- 1School of Sport, Exercise and Rehabilitation Sciences, University of Birmingham, Birmingham, United Kingdom
- 2Centre for Human Brain Health, University of Birmingham, Birmingham, United Kingdom
The growing prevalence of physical inactivity in the population highlights the urgent need for a more comprehensive understanding of how sedentary behaviour affects health, the mechanisms involved and what strategies are effective in counteracting its negative effects. Physical inactivity is an independent risk factor for different pathologies including atherosclerosis, hypertension and cardiovascular disease. It is known to progressively lead to reduced life expectancy and quality of life, and it is the fourth leading risk factor for mortality worldwide. Recent evidence indicates that uninterrupted prolonged sitting and short-term inactivity periods impair endothelial function (measured by flow-mediated dilation) and induce arterial structural alterations, predominantly in the lower body vasculature. Similar effects may occur in the cerebral vasculature, with recent evidence showing impairments in cerebral blood flow following prolonged sitting. The precise molecular and physiological mechanisms underlying inactivity-induced vascular dysfunction in humans are yet to be fully established, although evidence to date indicates that it may involve modulation of shear stress, inflammatory and vascular biomarkers. Despite the steady increase in sedentarism in our societies, only a few intervention strategies have been investigated for their efficacy in counteracting the associated vascular impairments. The current review provides a comprehensive overview of the evidence linking acute and short-term physical inactivity to detrimental effects on peripheral, central and cerebral vascular health in humans. We further examine the underlying molecular and physiological mechanisms and attempt to link these to long-term consequences for cardiovascular health. Finally, we summarize and discuss the efficacy of lifestyle interventions in offsetting the negative consequences of physical inactivity.
1 Introduction
Over the past few decades, the increasing prevalence of physical inactivity has become one of the most critical public health problems of our society (Dumith et al., 2011; Harvey et al., 2013). According to the World Health Organization recommended physical activity guidelines (Bull et al., 2020), physical inactivity refers to an insufficient level of moderate (at least 150 min per week) or vigorous intensity aerobic physical activity (at least 75 min per week). Physical inactivity is recognized as an independent risk factor for different pathologies including atherosclerosis (Bertoni et al., 2009), hypertension (Huai et al., 2013), cardiovascular disease (CVD) (Fletcher et al., 1996), type 2 diabetes (Admiraal et al., 2011), and breast (Pizot et al., 2016) and colon cancer (Liu et al., 2016). Globally, it is estimated that 1 in 4 adults and over 80% of adolescents are physically inactive (Guthold et al., 2018; Guthold et al., 2020). However, in many geographical areas, such as Latin America and Caribbean, and high-income Western countries, the average inactivity level is above one-third of the adult population (Guthold et al., 2018). This is a public health concern given that physical inactivity is the fourth leading risk factor for mortality (British Heart 2017) accounting for more than five million deaths per year (Ekelund et al., 2016). Furthermore, physical inactivity has been shown to have detrimental effects on brain function in children (Chaddock et al., 2011) and older adults (Arnardottir et al., 2016) and be associated with an increased risk of dementia (Laurin et al., 2001; Hamer and Chida 2009; Kivimäki et al., 2019).
Importantly, being physically active may not be sufficient to prevent the detrimental effects on health. There is accumulating evidence from both epidemiological and observational studies showing that sedentary behaviour per se is a major modifiable risk factor for chronic disease and is predictive of cardiovascular and all-cause mortality (Ekelund et al., 2016; Carter et al., 2017). Sedentary behaviour is characterized by any waking behaviour (e.g., TV viewing, computer use) in a sitting, reclining or lying position in which the energy expenditure is ≤ 1.5 metabolic equivalents (METs) (Tremblay et al., 2017). Numerous studies indicate that links between sedentary time and mortality may be apparent even among those who are physically active (Carter et al., 2017; Patterson et al., 2018). Notably, others report that physical activity (more than 60 min per day of moderate intensity, or more than 150 min per week of moderate-to-vigorous intensity) can be effective in attenuating or even eliminating the increased mortality risks associated with sedentary time (Ekelund et al., 2016; Stamatakis et al., 2019). The prevalence of sedentary behaviour has increased drastically in western countries within the last 50 years (Church et al., 2011; Archer et al., 2013), with young adults spending approximately 8.5 h daily in sedentary activities (Staiano et al., 2018) most of which are work-related (approx. 60% of total daily sitting time) (Clemes et al., 2014; Kazi et al., 2014). This is more accentuated in older adults, with reports showing that individuals over 60 years old spend more than 9.5 h per day being sedentary (Matthews et al., 2008; Sagelv et al., 2019). This is particularly relevant given that spending more than 9.5 h per day being sedentary increases the risk of all-cause mortality by approximately 30% (i.e., hazard ratio: ∼1.3) (Ekelund et al., 2019). Moreover, to aggravate the already critical situation, future predictions estimated that the amount of time spent in sedentary behaviour will increase in the foreseeable future (Ng and Popkin 2012).
Importantly, randomized controlled human trials show that even acute bouts of uninterrupted sitting can have adverse effects on peripheral (Thosar et al., 2014; Thosar et al., 2015a; Thosar et al., 2015b; Restaino et al., 2015; Morishima et al., 2016; Restaino et al., 2016; Morishima et al., 2017; Vranish et al., 2018), central (Dempsey et al., 2016a; Dempsey et al., 2016b; Credeur et al., 2019) and cerebral vascular health (Carter et al., 2018) as well as on glucose metabolism (Dunstan et al., 2012; Bailey and Locke 2015). It is likely that repeated transient impairments in vascular and metabolic function due to acute sitting may contribute to the well-known, long-term detrimental effects on cardiovascular (Padilla and Fadel 2017), metabolic (Kim et al., 2018), and brain health (Falck et al., 2017; Siddarth et al., 2018). However, the underlying mechanisms linking acute/short-term effects of sitting and long-term consequences for human health are not well understood. Uncovering such mechanistic underpinnings will be key to optimize existent strategies (e.g., standing, walking) and foster new alternative strategies (e.g., dietary approaches) that can minimize or counteract the detrimental effects of acute sedentariness in humans.
In this review, we summarize the evidence supporting the impact of acute and short-term physical inactivity on peripheral and cerebral vascular function in humans. We further discuss the potential molecular and physiological mechanisms that underlie such detrimental effects and attempt to link these to long-term consequences for health. Additionally, we summarize and discuss interventions that are efficacious in offsetting the immediate negative consequences of inactivity.
2 Acute effects of physical inactivity on vascular function in humans
2.1 Endothelial function
Studies examining the acute effects of physical inactivity on human vascular health have been primarily focused on models of uninterrupted sitting, lasting from 10 min to 7 h, in young healthy individuals, middle-aged overweight/obese adults, adults with type 2 diabetes, and women with polycystic ovary syndrome (PCOS) (Table 1). Restaino et al. (2015) showed that prolonged sitting (lasting 6 h) resulted in a decline in endothelial function, measured by flow-mediated dilation (FMD), in the popliteal artery in the lower limbs in young healthy men. Conversely, FMD of the brachial artery in the upper limbs remained unaffected by sitting (Restaino et al., 2015), which is consistent with what was observed in similar studies (Thosar et al., 2014; Carter et al., 2017).
First introduced in 1992 (Celermajer et al., 1992), FMD is now a widely popular, non-invasive tool for examining nitric oxide (NO)-dependent endothelial function of peripheral conduit arteries (Thijssen et al., 2019). The FMD technique requires the use of an ultrasound device (ideally, high-resolution, and duplex); the protocol consists of inducing a post-ischemic increase in blood flow (and therefore shear stress) that results in a transient increase in diameter (i.e., vasodilation) of the imaged conduit artery (Thijssen et al., 2019). Peripheral conduit arteries such as the brachial (Green et al., 2014) and superficial femoral artery (Kooijman et al., 2008) have been demonstrated to be largely mediated by NO, therefore reflecting endothelium-dependent vascular function. FMD decline reflects endothelial dysfunction, which is typically an early sign of atherosclerosis (Mudau et al., 2012; Thijssen et al., 2019). FMD is affected by sedentary behaviour, particularly, as showed in a recent study, large amount of total daily sitting time has been associated with reductions in FMD (Yamaji et al., 2022). Whilst brachial FMD is reflective of future risk of CVD (decline of 1% in FMD is associated with an increased risk of a future CVD event of up to 13%) (Inaba et al., 2010), lower limb FMD seems to be more closely associated with lower limb atherosclerosis and peripheral arterial disease (PAD) (Heinen et al., 2015). Indeed, in an asymptomatic population, higher sedentary time has been associated with a 22% increased risk of a future diagnosis with PAD (Kulinski et al., 2015).
Although only a limited number of studies have assessed the impact of prolonged sitting on endothelial function on both upper and lower limbs, most have found that sitting affects mainly endothelial function in the lower limb arteries (Thosar et al., 2014; Restaino et al., 2015; Climie et al., 2018). To date, only two studies have shown significant decline in brachial FMD in response to uninterrupted sitting (i.e., 2.5, and 4 h) (Cho et al., 2020; Headid et al., 2020), with one of those two studies showing concomitantly a marked decline in popliteal FMD (Headid et al., 2020). In agreement with Restaino et al. (2015), many others have shown that shorter sitting trials (1–3 h) have detrimental effects on endothelial function not only in the popliteal artery (2.3–4.1% FMD) (Morishima et al., 2016; Restaino et al., 2016; Morishima et al., 2017; Vranish et al., 2017; Morishima et al., 2020a; Morishima et al., 2020b) but also in the upstream superficial femoral artery (2.4–2.7% FMD) in young healthy adults (Thosar et al., 2014; Thosar et al., 2015a; Thosar et al., 2015b; Ballard et al., 2017). In addition, recent studies have found that the common femoral artery is affected by sitting-induced vascular dysfunction, measured by passive leg movement (PLM)-induced hyperaemia (Garten et al., 2019a; Garten et al., 2019b; Decker et al., 2021), an alternative method to assess endothelial function (Gifford and Richardson 2017).
Significant declines in lower limb vascular function, specifically superficial femoral artery (4.1–4.3% FMD), seem to be observed consistently within the first hour of sitting, with no significant further declines during longer periods of sitting (Thosar et al., 2014; Thosar et al., 2015a; Thosar et al., 2015b; Climie et al., 2018). Interestingly, among those studies, only one showed a small recovery in FMD detectable from the second hour of sitting that was maintained up to 5 h (Climie et al., 2018). Despite the majority of the studies indicating that sitting-induced impairments in vascular function occur in the lower limbs, a few studies report no statistical significant differences in FMD in femoral (Carter et al., 2019; Caldwell et al., 2020; Taylor et al., 2020; Hartman et al., 2021; Taylor et al., 2021), popliteal (Padilla et al., 2009; Peddie et al., 2021), and posterior tibial artery (Credeur et al., 2019) in response to 2–7 h of sitting (Table 1).
The level of physical fitness may also play an important role in counteracting sitting-induced vascular dysfunction, but the literature is rather limited and shows discrepancy in results. Specifically, one of these studies has shown that endurance-trained men, contrary to their untrained counterparts, maintained lower limb endothelial function throughout a 3-h sitting period (Morishima et al., 2020a), whereas other studies do not confirm these findings (Garten et al., 2019a; Liu et al., 2021). In this regard, Liu et al. (2021) have shown that higher fitness levels were associated with more pronounced sitting-induced declines in popliteal FMD, despite having higher FMD prior to sitting (Liu et al., 2021). Additionally, another study has found similar sitting-induced declines in lower limb vascular function between trained and untrained individuals (Garten et al., 2019a), although in this study endothelial function was assessed using the PLM technique, and not the gold-standard FMD. As such, it is not clear whether physical fitness is sufficient to provide a protective effect against sitting-induced endothelial dysfunction and this is an area that should be investigated in future studies.
In children, sitting-induced negative consequences on vasculature are remarkably similar. For example, a study in 7–10 year-old girls showed that sitting-induced impairments in endothelial function in the superficial femoral artery may also be apparent within similar time frames (i.e., 3 h) (McManus et al., 2015) and to a similar degree (2.3% FMD) to young healthy adults (Thosar et al., 2014; Thosar et al., 2015a; Thosar et al., 2015b; Ballard et al., 2017). This is relevant considering that children (9–11 years old) spend on average more than 8 h per day in sedentary activities (LeBlanc et al., 2015), while their recommended daily time for being physical active is 60 min (more than twice that of adults) (Gibson-Moore 2019).
The detrimental effects of sitting have been also observed in at-risk populations. In particular, recent studies in sedentary overweight/obese adults have shown FMD declines in both the femoral (4.1%) and popliteal artery (1.5%) in response to 1 and 4 h of sitting, respectively (Climie et al., 2018; Kruse et al., 2018). No changes in brachial FMD were detected in this population (Climie et al., 2018). On the other hand, more recent studies in women with PCOS (Taylor et al., 2020), adults with type 2 diabetes (Taylor et al., 2021) and with increased cardiovascular risk (Hartman et al., 2021), did not detect any sitting-induced decline in FMD in lower limb arteries, specifically the superficial femoral artery (Taylor et al., 2020; Hartman et al., 2021; Taylor et al., 2021).
Furthermore, it remains unclear whether there are sex differences in vascular responses to prolonged sitting, with a few studies comparing the impact of sitting between males and females. Vranish et al. (2017) reported sitting-induced declines in popliteal artery FMD in men, while in pre-menopausal women (assessed during the early follicular phase) vascular function was preserved, likely due to the influence of oestrogen, which is protective of endothelial function (Maturana et al., 2007). However, more recent studies report similar declines in micro and macrovascular function in the popliteal and common femoral artery in both males and females (O’Brien et al., 2019; Decker et al., 2021). This highlights the need to design future studies that can evaluate the sex differences in vascular function during sitting, also taking into consideration menopausal status.
Altogether, these studies indicate that uninterrupted prolonged sitting in children, healthy young adults and at-risk populations affects peripheral conduit arteries by impairing endothelial-dependent vascular function. Particularly, lower limb arteries are the most affected by sitting, while vascular impairments in upper limb arteries are often not observed. This is important to consider, given that lower limb arteries are more prone to circulatory problems such as atherosclerosis (Kröger et al., 1999; Laclaustra et al., 2016) and peripheral arterial disease (Thachil et al., 2011). However, it remains to be established how the severity of vascular deficits changes with sitting time, but findings to date indicate that endothelial dysfunction can be detected after only 1 h of sitting, with additional sitting time not resulting in more severe impairments in both healthy young adults and sedentary overweight/obese middle-aged adults. Currently, it is unclear how the severity of sitting-induced endothelial dysfunction in at-risk populations (e.g., obese individuals) compares with healthy young adults, given that no studies have directly compared these groups within the same human trial. Finally, the impact of sex and fitness in endothelial function post-sitting requires further examination.
2.2 Blood flow haemodynamics
In addition to endothelial function, other parameters such as blood flow and shear rate/stress are also modulated by prolonged sitting in both lower and upper limb arteries (Thosar et al., 2014; Morishima et al., 2017; Vranish et al., 2018). Blood flow and shear rate/stress play important roles in the regulation of vascular tone by stimulating the endothelium to release vasoactive factors such as NO (Paniagua et al., 2001). Marked declines in both blood flow and shear rate in the popliteal artery have been reported in response to very short periods of sitting (10 min) in both healthy young men and women (Vranish et al., 2017; Vranish et al., 2018). Interestingly, this reduction occurred within the first minute of sitting and remained consistently lower until the end of the sitting trial (Vranish et al., 2018). Although popliteal FMD was unaffected after 10 min of sitting, hyperaemic blood velocity (area under the curve) was significantly reduced, suggesting that short periods of sitting affect not only resting blood flow and shear rate, but also functional blood flow dynamics (i.e., reactive hyperaemia response). Reactive hyperaemia, a measure of peripheral microvascular function, is the transient increase in blood flow above baseline that occurs following a short period of ischemia due to arterial occlusion (Rosenberry and Nelson 2020). This is important given that reactive hyperaemia is a predictor of future cardiovascular events, and its reduction has been associated with increased risk of mortality and cardiovascular disease (Huang et al., 2007; Philpott and Anderson 2007; Paine et al., 2016).
Similar declines in blood velocity and shear rate are detected in other lower limb arteries, such as posterior tibial and superficial femoral artery, in young healthy adults (Thosar et al., 2014; Thosar et al., 2015a; Thosar et al., 2015b; Younger et al., 2016; Ballard et al., 2017). However, in at-risk populations, there are contradictory reports, with some studies showing sitting-induced reductions in shear rate and/or blood flow in the lower limb arteries (i.e., posterior tibial and popliteal artery) (Kruse et al., 2018; Credeur et al., 2019), whilst others seeing no changes in blood flow and/or shear rate in the superficial femoral artery (Climie et al., 2018; Taylor et al., 2020; Taylor et al., 2021). It is currently unclear whether these differences are of significance. On the other hand, shear rate declines in the brachial artery appear to be less accentuated when exposed to prolonged sitting. In fact, some studies show no significant reductions in shear rate in both healthy (Carter et al., 2017) and at-risk populations (Climie et al., 2018), whilst in young healthy adults, some show significant declines (Restaino et al., 2015) but also no effects on mean shear rate (Thosar et al., 2014) in young healthy individuals.
Similarly, blood flow has also shown to be reduced in the brachial artery (by approximately 42%) following 2 h of sitting (Restaino et al., 2015). This suggests that the contribution of resting blood flow/shear rate to changes in endothelial function after sitting might be different in upper and lower limbs. Overall, declines in blood flow and/or shear rate after sitting seem to be generally paralleled by reductions in FMD, at least in the lower limbs, suggesting that such haemodynamic changes might contribute to sitting-induced endothelial dysfunction.
2.3 Arterial stiffness
Recent studies suggest that sitting (3 h) can negatively impact aortic stiffness in young adults as indicated by an increase in pulse wave velocity (PWV) (∼0.35 m⋅s−1) (Credeur et al., 2019; Evans et al., 2019), which is the gold standard measure of arterial stiffness (Townsend 2016). Likewise, an increase in PWV has also been observed in at-risk middle-aged adults during a simulated seated workday (total sitting time: >7 h) (Alansare et al., 2020). These sitting-induced effects on PWV have been reported in young healthy adults (Evans et al., 2019), overweight/obese adults (Credeur et al., 2019) and middle-aged at-risk adults (overweight/obese, hypertensive) (Alansare et al., 2020), highlighting that sitting can have broader effects on vascular health beyond lower limb arterial function. However, similar to FMD findings, there are inconsistencies across the literature with some studies reporting that PWV remains unaltered following uninterrupted prolonged sitting (Kowalsky et al., 2019; Perdomo et al., 2019; Tremblay et al., 2019; Caldwell et al., 2020; Headid et al., 2020). Nevertheless, while such studies fail to show any acute change in PWV that reached the clinically significant threshold of 1 m⋅s−1 (corresponding to an increased risk of 14% and 15% in cardiovascular events and all-cause mortality, respectively) (Vlachopoulos et al., 2010), it is plausible to consider that repeated prolonged periods of sitting may aggravate aortic stiffness in the long-term. Indeed, numerous studies reported a positive association between sedentary time and arterial stiffness (Huynh et al., 2014; Horta et al., 2015; Ahmadi-Abhari et al., 2017). Also, this is particularly relevant given that increases in arterial stiffness are associated with higher risk of hypertension (Safar et al., 2018), cardiovascular disease (Mitchell et al., 2010), stroke (Mattace-Raso et al., 2006), chronic kidney disease (Oh 2018), and structural brain damage (Palta et al., 2019).
Sitting also induces increases in oscillatory shear index (OSI) in the brachial artery (Thosar et al., 2014), whilst resulting in either a reduction or no effect in the lower limb arteries (McManus et al., 2015; Garten et al., 2019a; Credeur et al., 2019). OSI is a haemodynamic parameter that reflects fluctuations in blood flow (Zhang et al., 2014), and is negatively correlated with wall shear stress (Fytanidis et al., 2014). Numerous studies have reported that a high OSI value may contribute to the development of atherosclerotic disease and arterial wall thickening (Goubergrits et al., 2008; Wen et al., 2010; Fytanidis et al., 2014; Meirson et al., 2015), which is shown to correlate with arterial stiffness (Gómez-Marcos et al., 2011; Suceava et al., 2013). Therefore, while most studies that have investigated upper limb arteries have not shown impairments in endothelial function after sitting (e.g., Thosar et al., 2014), the changes in OSI reported to date suggest that upper limb arteries may still be susceptible to atherosclerosis due to prolonged sitting. More studies are needed to clearly establish the short and long-term effects of sitting on markers of vascular stiffness.
2.4 Arterial blood pressure
Prolonged sitting has also been shown to have detrimental effects on blood pressure (BP). However, the literature remains unclear with some studies reporting no sitting-induced variations in BP (e.g., Restaino et al., 2016; Carter et al., 2019; Headid et al., 2020), whilst several studies have detected significant increases in either systolic (SBP), diastolic (DBP), or mean arterial pressure (MAP) in response to short bouts of sitting (i.e., 3–7 h) (e.g., O’Brien et al., 2019; Morishima et al., 2020a; Morishima et al., 2020b). For example, Younger et al. (2016) have reported that 5 h of sitting leads to increases in MAP of approximately 5 mmHg, in healthy young adults. In addition to this, Dempsey et al. (2016a) showed increases in SBP (10 mmHg) and DBP (5 mmHg) after 7 h of uninterrupted sitting in late middle-aged/elderly adults (∼60 years old) with cardiovascular risk factors. Effectively, these BP changes reached clinically relevant pre-hypertensive range (above 130/80), which are known to be associated with an increased risk of developing hypertension (Chobanian et al., 2003) and future cardiovascular events (Huang et al., 2013). Importantly, data pooled from 4 randomized controlled trials indicate that sitting might increase BP in a dose-response manner and that hypertensive individuals might be more susceptible to sitting-induced increases in BP (∆SBP = 6 mmHg; ∆DBP = 3 mmHg) than normotensives (∆SBP = 5; ∆DBP = 2 mmHg), as observed following 6 h of sitting (Dempsey et al., 2018). This is key, as acute increases in BP during sitting might put this already vulnerable population at even higher risk of future cardiovascular events (Luo et al., 2020).
In summary, uninterrupted prolonged sitting induces detrimental effects on endothelial function in the peripheral vasculature, increases arterial stiffness and raises blood pressure, as well as modulates blood flow and shear rate/stress in both healthy and middle-aged obese/overweight populations. The lower limb arteries appear to be much more susceptible to sitting-induced vascular impairments compared to upper body arteries (e.g., brachial artery), although only a few studies have addressed this question specifically.
3 Short-term effects of physical inactivity in the peripheral vasculature
The literature investigating the effects of physical inactivity on human vascular function over short periods of time is currently limited, but there is evidence to indicate that periods of reduced/abolished physical activity ranging from 1 to 8 weeks can have detrimental consequences (Thijssen et al., 2010) (Figure 1) (Table 2). In particular, step-reduction models have shown marked declines in the popliteal artery FMD response (approx. 3.5%) after 5 days of reduced activity (from >12,000 to <4,000 steps per day) in healthy active men (Boyle et al., 2013; Teixeira et al., 2017). Whilst no changes in FMD were detected in the brachial artery, a significant reduction in resting arterial diameter was reported (0.23 mm) (Boyle et al., 2013). Interestingly, when in young healthy adults the daily step count was further reduced (from >12,000 to approx. 2,500) and for a longer period of time (i.e., 14 days) there were observed reductions in brachial FMD (by 1.8%) while the diameter remained unaltered (Bowden Davies et al., 2021). This may suggest that intensity and duration of the physical inactivity protocol play an important role in mediating inactivity-induced vascular dysfunction. To date, the effects of step-reduction on vascular health in older adults or other at-risk populations have not been investigated. This would be particularly important future work given that in the United Kingdom, older adults perform an average of 4,000–6,500 steps per day (Harris et al., 2009; Davis et al., 2011; Ludwig et al., 2018), with over 80 year-olds performing less than 3,000 steps daily (Davis et al., 2011). Additionally, increases in circulating levels of endothelial microparticles (EMPs) have also been observed (Boyle et al., 2013). EMPs are small membrane-enclosed vesicles released in response to endothelial cell injury/apoptosis and represent an accurate biological marker of endothelial dysfunction (Deng et al., 2016). Indeed, direct relationships between microparticle concentrations and clinical manifestations of cardiovascular diseases, such as atherosclerosis (Paudel et al., 2016), coronary artery disease (França et al., 2015) and myocardial infarction (Deng et al., 2016), have been observed.
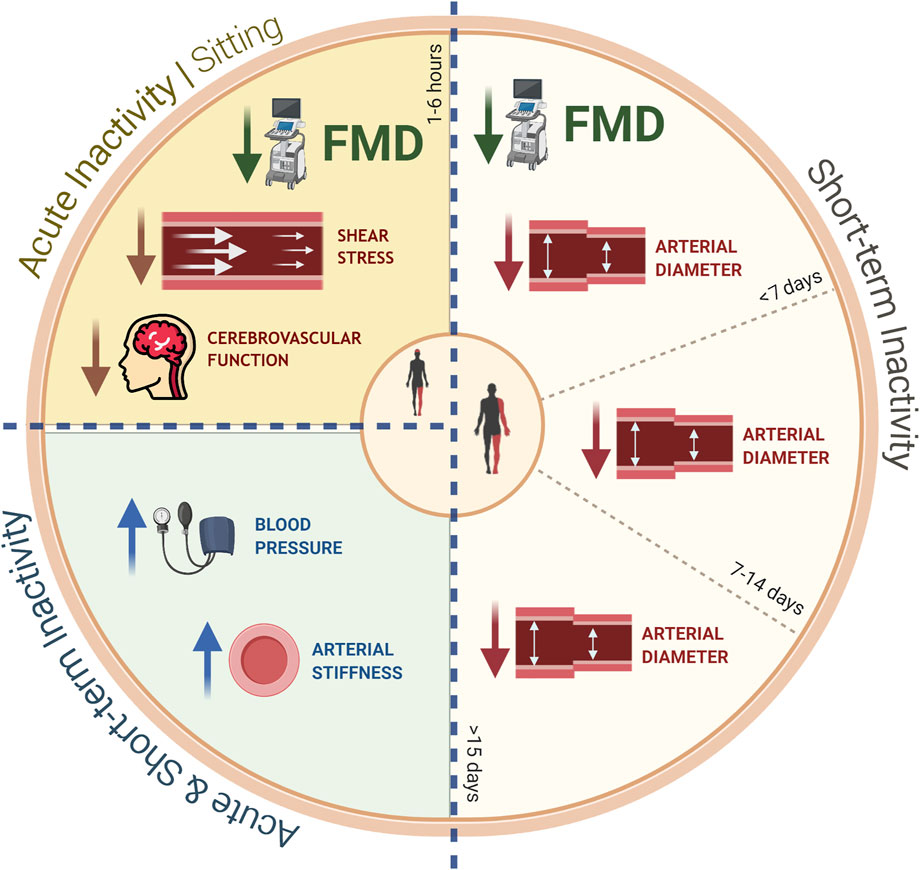
FIGURE 1. Summary of the negative effects of both acute (sitting) and short-term (e.g., step-reduction, limb immobilization, bed rest) inactivity protocols on peripheral vascular health in humans: sitting for up to 6 h results in a reduction in FMD, shear stress/rate, and cerebrovascular function; short-term reductions in physical activity of up to 15 days (or more) result in declines in arterial diameter. All inactivity protocols (acute and short-term) have been reported to increase blood pressure and arterial stiffness. FMD: Flow-mediated dilation.
Prolonged bed rest is an alternative method of restricting physical activity in humans and has been widely used in research in recent years to evaluate its impact on human metabolic and vascular function (Jost 2008; Hargens and Vico 2016). In contrast with step-reduction models, in which the main activity (i.e., walking) has an energy expenditure of approx. 3 METs, bed rest abolishes any form of activity resulting in an energy expenditure of only 1 MET (Mendes et al., 2018), corresponding to resting metabolic rate (Jetté et al., 1990). Prolonged bed rest is widely prevalent during hospitalization, however, although traditionally recommended as a primary treatment for many medical conditions, prolonged bed rest can be considered as a deleterious form of therapy (Allen et al., 1999). Nosova et al. (2014) have shown that a 5-day bed rest intervention induces a significant decline in FMD in brachial and superficial femoral arteries (approx. 2% in both), an increase in DBP (approx. 4 mmHg) and an increase in central augmentation index (9%, as an indirect measure of arterial stiffness) in young healthy individuals (Nosova et al., 2014). A similar period of bed rest was shown to result in further reductions in the diameter of the brachial artery, and an increase in SBP (approx. 7 mmHg), although there were no significant changes in blood flow, blood velocity and FMD detected in this study (Hamburg et al., 2007). Impairments in microvascular function, as shown by decreased reactive hyperaemia, were also detected in both upper and lower limbs (Hamburg et al., 2007). For example, the aforementioned study by Hamburg and colleagues also observed a reduction in peak hyperaemia blood flow, which is traditionally used as a surrogate measure of resistance vessel remodelling and may indicate the presence of inward remodelling of resistance arteries (Thijssen et al., 2011). This finding is consistent with the inward remodelling of the brachial conduit artery which was also reported, as indicated by the reduction in brachial artery diameter (Hamburg et al., 2007). This aspect is clinically relevant given that structural inward remodelling of resistance vessels is associated with an increased risk of future cardiovascular events (Brown et al., 2018).
Longer bed rest protocols, as expected, seem to induce more severe effects on the vasculature. For example, Bleeker et al. (2005) reported that 52 days of horizontal bed rest resulted in diameter reductions of the brachial (5%), superficial (16%) and common femoral artery (17%), indicating that arterial inward remodelling may have occurred as a consequence of short-term inactivity. However, no alterations in BP, and in baseline blood flow in all examined arteries were observed. Interestingly, the reduction in diameter observed in the superficial femoral artery was paralleled by an increase in FMD and endothelium-independent dilation, possibly due to increased smooth muscle sensitivity to vasodilators (e.g., NO), as suggested by the authors (Bleeker et al., 2005), or simply because of the inverse relationship between FMD and baseline diameter (Silber et al., 2001). However, a similar study using the same model found functional impairments in the popliteal vein whilst no significant alterations in diameter were observed (van Duijnhoven et al., 2008).
Localized physical inactivity in the lower limbs have also been achieved experimentally by using unilateral leg casting. Similar to bed rest, this method is used in medical practice, but mainly as a primary treatment for immobilizing a limb after an acute hard and/or soft-tissue injury. To investigate injury-related vascular impact, Sugawara et al. (2004) evaluated the effects of short-term (i.e., 7 days) cast immobilization of the right lower limb (left leg used as an internal control) in healthy young men. They reported significant reductions in resting blood flow, lumen diameter and vascular conductance in the common femoral artery of the immobilized leg; however, there were no significant changes in BP and femoral artery intima-media thickness. Similarly, the study of Rakobowchuk et al. (2011) involved a similar immobilization protocol for a longer period (i.e., 12 days) and showed a decline in diameter, and an increase in shear rate and FMD in the popliteal artery of the immobilized leg in comparison to the control leg. Interestingly, the common femoral artery reported a decline in arterial diameter in both legs, likely driven by an overall reduction in physical activity (Rakobowchuk et al., 2011). These reductions in diameter observed in lower limb arteries are consistent with the findings of a more recent study that used a 14-day leg immobilization protocol (Cohen et al., 2021). Specifically, a reduction in femoral artery diameter was observed whilst FMD, shear rate, and PWV remained unaltered (Cohen et al., 2021). This type of intervention has also been shown to induce a decrease in vascular function (measured by PLM technique), and vascular endothelial growth factor (VEGF) (Hyldahl et al., 2021), which plays an important role in maintaining vascular function (Koyama et al., 2002).
Interestingly, although localized inactivity of lower limbs appears to induce localized vascular impairments, that does not seem to be the case for upper limb arteries. For example, a study investigating specifically the immobilization of the upper limbs reported that brachial artery diameter, FMD and endothelium-independent vasodilation did not change significantly in healthy individuals in response to an 8-day intervention (Birk et al., 2013). However, Birk and colleagues did observe a decline in peak blood flow (Birk et al., 2013), possibly indicating vascular inward remodelling of forearm resistance arteries (Thijssen et al., 2011; Birk et al., 2013). This finding might also be an indication that inactivity-induced vascular remodelling in large conduit arteries and resistance vessels have a different time-course (reviewed in Thijssen et al., 2011). Collectively, these studies suggest that the lower body arteries are more susceptible to localized acute and short-term inactivity than the upper body arteries.
There are other models that reflect local or systemic physical inactivity such as unilateral lower limb suspension, dry immersion, water immersion, and head-down tilt bed rest. However, these inactivity protocols are used primarily to investigate the physiological consequences of microgravity conditions that are relevant for human space travel (Hackney and Ploutz-Snyder 2012; Tesch et al., 2016; Watenpaugh 2016; Tomilovskaya et al., 2019; Pandiarajan and Hargens 2020), and do not represent relevant earth-based sedentary activity, and therefore are out of the scope of this review.
In summary, there is evidence to indicate that short-term inactivity has a detrimental impact on peripheral and central vascular health. Whilst short periods of step-reduction and bed rest induce impairments in peripheral vascular function (as seen in FMD decline), longer systemic and lower limb inactivity protocols seem to impact arterial structure (vascular inward remodelling), potentially reflecting the time-course of vascular adaptations to inactivity. However, most studies have focused on young healthy adults, making the translation of these observations to other populations difficult. Furthermore, upper limb arteries seem to be more resistant than lower body arteries in response to short-term inactivity, particularly following local inactivity protocols, which is consistent with observations from acute sitting studies.
4 Effects of physical inactivity in the cerebral vasculature
The body of research looking at the impact of physical inactivity on the cerebral vasculature is currently very limited, mostly focused on the acute effects of prolonged sitting. A recent study in young healthy desk workers reported a decrease in blood velocity in the middle cerebral artery (MCAv; –2.9 cm⋅s−1), measured by transcranial Doppler ultrasound, and impaired cerebral autoregulation following 6 h of uninterrupted sitting. However, other cerebrovascular functional measures were not altered (i.e., cerebrovascular CO2 reactivity [5% CO2]) nor was there a measurable effect on cognitive performance (Carter et al., 2021). Prior studies in the same population using shorter sitting protocols (i.e., 4 h) have reported a reduction in MCAv (–1.4 cm⋅s−1), whilst cerebrovascular CO2 reactivity (5% CO2) and cerebral autoregulation remained unaltered (Carter et al., 2018). Furthermore, in young healthy adults, 3 h of sitting did not result in changes in prefrontal cortex haemodynamics (i.e., total haemoglobin concentration, and tissue saturation index) and executive function (Stoner et al., 2019). In addition, Wheeler et al. (2019) reported larger reductions in MCAv in response to 2 (approx. 6 cm⋅s−1) and 4 h (approx. 8 cm⋅s−1) of sitting in overweight/obese older adults (Wheeler et al., 2019), which may indicate that age or health status (e.g., endothelial dysfunction) can play a role in cerebrovascular responses to inactivity (Iadecola and Davisson 2008; Stefanidis et al., 2019). Conversely, in response to a much shorter period of sitting (i.e., 30 min), no significant changes in MCAv were observed in middle-aged overweight adults (Perdomo et al., 2019).
Further studies in older adults also report a significant increase in cerebrovascular resistance (Maasakkers et al., 2020), which has been associated with mild cognitive impairment and Alzheimer’s disease (Yew et al., 2017; Nation 2018). Only a limited number of studies have investigated the impact of longer periods of inactivity (e.g., lower limb immobilization, bed rest) in blood flow to the brain, with studies to date reporting no changes in carotid blood velocity, blood flow, arterial compliance and arterial diameter in young healthy individuals (Bleeker et al., 2005; Rakobowchuk et al., 2011).
In summary, the current literature on the impact of short-term periods of inactivity on cerebrovascular function is very limited, yet this is an important area requiring attention, given the evidence suggesting that chronic cerebral hypoperfusion is a key contributing factor to the pathogenesis of dementia (Roher et al., 2012; Wolters et al., 2017; Park et al., 2019) and the epidemiological links between sedentary behaviour and incidence of dementia (Yan et al., 2020). Preventing transient and/or permanent declines in cerebral blood flow by reducing physical inactivity, may be key for delaying the onset of dementia.
5 Mechanisms underlying inactivity-induced impairments in vascular function
5.1 Vascular biomarkers
The molecular mechanisms underlying the effects on the vasculature of prolonged uninterrupted sitting, remain largely unexplored. It is well-established that endothelial function (measured by FMD) is dependent on a balance between key vasoconstrictors, such as endothelin-1 (ET-1), and vasodilators, such as NO (Sanders et al., 2000; Bourque et al., 2011) (Figure 2). NO is produced by endothelial nitric oxide synthase (eNOS) in response to changes in shear stress (Ballermann et al., 1998; Davies 2009; Sriram et al., 2016) and plays a role in preventing arterial stiffness and platelet aggregation (Sugawara et al., 2007; Sandoo et al., 2010). Currently, there is limited evidence for the role of NO on physical inactivity-induced vascular dysfunction in humans. Climie et al. (2018) reported no changes in levels of circulating nitrate and nitrite after 5 h of sitting in sedentary overweight/obese adults, despite significant declines in femoral FMD. In agreement with this, no changes in plasma levels of arginine and its related metabolites (indirect indicators of NO status) were observed after 3 h of sitting in healthy men (Ballard et al., 2017). Although acute periods of prolonged sitting do not seem to elicit changes in NO status, there are studies reporting significant differences in circulating NO metabolites (e.g., nitrite, nitrate) between sedentary and non-sedentary older adults (Bjork et al., 2012; Carmeli et al., 2015). However, the quantification of nitrite and nitrate in circulation may not be an exact reflection of eNOS activity or NO production (Lauer et al., 2001), thus caution should be taken when these biomarkers are considered for assessing the impact of inactivity on NO levels. Instead, nitrite rather than nitrate may be a more robust measure of endogenous NO production (Lauer et al., 2001). Consistent with human studies, animal studies report lower concentrations of plasma NO and expression of eNOS in sedentary mice compared to their active age-matched counterparts (Suvorava et al., 2004; Song et al., 2009; Kang et al., 2019). Similarly, 14 days of hindlimb unweighting in mice resulted in a reduction of eNOS expression in soleus-feed arteries and this was accompanied by impairments of endothelial vasodilatory function (Jasperse et al., 1999; Woodman et al., 2001). This indicates that NO might play a role in the long-term effects of physical inactivity but does not necessarily explain the more acute and transient effects on endothelial function.
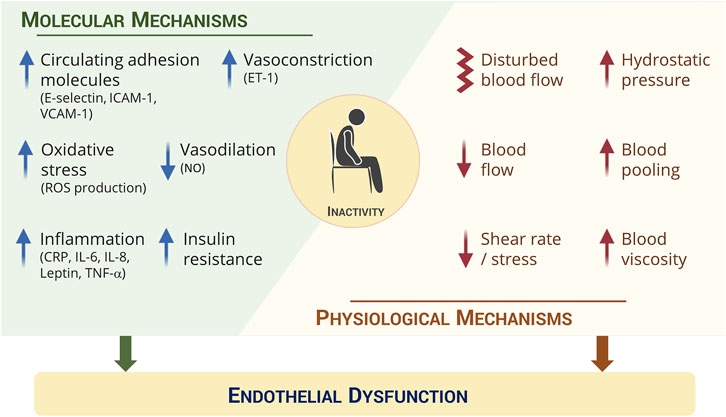
FIGURE 2. Proposed underpinning mechanisms by which physical inactivity induces endothelial dysfunction in humans include modulation of i) molecular components associated with vasodilation/vasoconstriction, vascular adhesion, inflammation, oxidative stress and insulin resistance, as well as ii) physiological components, mainly in the lower limbs, such as declines in blood flow and shear stress/rate and increases in hydrostatic pressure, blood pooling and viscosity. CRP: C-reactive protein; ET-1: Endothelin-1; ICAM-1: Intercellular adhesion molecule-1; IL-6: Interleukin-6; IL-8: Interleukin-8; NO: Nitric oxide; ROS: Reactive oxygen species; TNF-α: Tumor necrosis factor alpha; VCAM-1: Vascular cell adhesion molecule-1.
Vascular smooth muscle sensitivity to NO may also play an important role in maintaining endothelial function following long periods of inactivity (Bleeker et al., 2005). For example, Jasperse et al. (1999) showed an increased endothelium-independent vasodilation in soleus-feed arteries in response to administration of sodium nitroprusside, a NO donor, following 14 days of hindlimb unweighting in male rats. This enhanced response during physical deconditioning indicates an increase in NO sensitivity by endothelial smooth muscle cells (Jasperse et al., 1999), which is likely a compensatory mechanism in response to chronic reduction of shear stress and NO bioavailability.
Circulating levels of ET-1 have been shown to increase after prolonged sitting, along with significant declines in femoral FMD and shear rate in humans (Ballard et al., 2017). For example, ET-1 concentrations after 5 h of sitting were significantly higher than sitting with intermittent resistance activity and were found to be significantly correlated with blood flow and shear rate, although not with FMD (Climie et al., 2018). The authors suggest that in sedentary populations, interactions between blood flow, shear stress, and ET-1 may contribute to sitting-induced impairment in arterial function, but additional studies are needed to establish a direct relationship with endothelial function. Importantly, increases in levels of ET-1 have been associated with reduced eNOS expression (Rosiansky-Sultan et al., 2006) and impairments in FMD (Nishiyama et al., 2017), as well as elevations in inflammatory markers (i.e., serum interleukin-6), blood pressure and PWV (du Plooy et al., 2015). Furthermore, increased concentrations of ET-1 appear to play a key role in the pathogenesis of hypertension (Schiffrin 2001) and atherosclerosis (Dashwood and Tsui 2002).
Circulating adhesion molecules, such as intercellular (ICAM) and vascular cell adhesion molecules (VCAM), have been reported to be higher in physically inactive women (Mora et al., 2006) and sedentary hypertensive men (DeSouza et al., 1997), and positively associated with TV viewing time in children (Gabel et al., 2016) and adolescents (Martinez-Gomez et al., 2012). Chronic changes in circulating adhesion molecules may be clinically relevant molecular biomarkers for atherosclerosis (Hwang et al., 1997; Blann et al., 1998; Gross et al., 2012; Varona et al., 2019). However, no significant changes have been observed after prolonged sitting or bed rest (Nosova et al., 2014; Climie et al., 2018; Duvivier et al., 2018), so future studies are needed to establish the significance of adhesion molecules to endothelial dysfunction during periods of physical inactivity.
5.2 Insulin resistance
Several studies have reported positive associations between sedentary time and insulin resistance in both adults and children (Sardinha et al., 2008; Cooper et al., 2012; Lahjibi et al., 2013; Kim et al., 2018). Insulin is a peptide hormone secreted by the pancreas (specifically, β cells of the islets of Langerhans) that maintains normal blood glucose concentrations throughout the day (glucose homeostasis) (El Sayed and Mukherjee 2017), with insulin resistance typically defined as the state of attenuated biological response to normal or elevated insulin concentrations (Hauner 2002; Wilcox 2005). Insulin resistance is associated with the occurrence of vascular-related conditions including hypertension (Tarray et al., 2014), atherosclerosis (Semenkovich 2006), and endothelial dysfunction (Steinberg et al., 1996), and insulin plays an important role in conduit arteries compliance, modulation of blood flow and relaxation of resistance arterioles (Zheng and Liu 2015). Particularly, insulin is known to stimulate NO production via eNOS activation, and also regulates the release of ET-1 and the proliferation of vascular smooth muscle cells (Janus et al., 2016), which all have an effect on vascular function. Importantly, numerous experimental studies indicate that both acute and short-term inactivity protocols have negative effects on insulin profile. Most studies report significant impairments in insulin action/concentration in response to prolonged sitting (5–9 h) compared with sitting interrupted with regular active breaks (Dunstan et al., 2012; Peddie et al., 2013; Dempsey et al., 2016a; Dempsey et al., 2016b; Henson et al., 2016; Yates et al., 2020), although others have reported no changes (Miyashita et al., 2016). Generally, longer inactivity protocols such as, multiple days of prolonged sitting (Stephens et al., 2011; Larsen et al., 2015), step-reduction (5–14 days) (Krogh-Madsen et al., 2010; Knudsen et al., 2012; Breen et al., 2013; Reynolds et al., 2015; Davies et al., 2018; McGlory et al., 2018), or bed rest (3–9 days) (Stuart et al., 1988; Smorawinski et al., 2000; Alibegovic et al., 2009; Biensø et al., 2012; Dirks et al., 2016) seem to consistently induce signs of insulin resistance compared with observations after one prolonged sitting session. Interestingly, such effects on the insulin profile appear to occur within a similar time frame as inactivity-induced endothelial dysfunction, although very few studies have investigated these outcomes together. As such, Hamburg et al. (2007) showed signs of insulin resistance which were accompanied by vascular impairments following 5 days of bed rest. Contrary to this, Nosova and colleagues observed no significant changes in metabolic biomarkers despite reporting declines in both upper and lower limb vascular function (Nosova et al., 2014).
Considering the number of functions that insulin presents within the vascular endothelium (e.g., NO/ET-1 regulation), it is possible that insulin resistance observed following inactivity protocols might be a mechanism by which insulin resistance mediates inactivity-induced endothelial dysfunction. However, this aspect is not yet clearly elucidated, thus requiring future investigations within the context of acute inactivity, vascular function and insulin concentrations.
5.3 Inflammatory markers
Inflammation is strongly associated with vascular dysfunction and risk of CVD (Savoia et al., 2011; Teixeira et al., 2014). In this regard, increases in inflammatory markers such as tumor necrosis factor alpha (TNF-α), interleukin (IL)-1, IL-6, and IL-12 are associated with higher risk of developing hypertension (Jayedi et al., 2019); they also have been shown to have detrimental consequences on vasculature, particularly by promoting atherosclerotic lesions, leukocytes adhesion to the endothelium, and increase in atherosclerotic plaque formation (Galkina and Ley 2009). Furthermore, increases in IL-6 and C-reactive protein (CRP) are known to downregulate NO bioavailability (by attenuating eNOS activity) and upregulate ET-1 levels (Teixeira et al., 2014), and therefore impair endothelial function (Wassmann et al., 2004; Esteve et al., 2007; Hein et al., 2009; Devaraj et al., 2011; Nishiyama et al., 2017). As such, inflammation has been explored and hypothesized as a potential contributing mechanism underlying inactivity-related vascular dysfunction.
Several epidemiological studies showed that sedentary time, both self-reported and objectively measured, is associated with higher levels of several inflammatory biomarkers including leptin, CRP, IL-6, and TNF-α, in both children and adults (healthy and at-risk) (Healy et al., 2011; Allison et al., 2012; Yates et al., 2012; Henson et al., 2013; Falconer et al., 2014; Farah et al., 2016; Gabel et al., 2016). Interestingly, interrupting sedentary time with physical activity breaks, or partially replacing it with standing, or stepping, results in significant reductions in IL-6, CRP, and leptin (Healy et al., 2011; Henson et al., 2018).
Despite the limited number of human randomized controlled trials, some acute and short-term inactivity models also suggest significant modulation of inflammatory markers. Recently, Dogra et al. (2019) reported an increase in salivary IL-8 concentration in response to 4 h of uninterrupted sitting in active young healthy adults. However, no assessments of endothelial function were performed during this sitting intervention, so no direct association with vascular function could be established. Further, using a 2-week step reduction model, short-term inactivity increases circulatory levels of CRP, TNF-α (Breen et al., 2013), and IL-6 concentrations (McGlory et al., 2018) in older adults, but not in young adults (Krogh-Madsen et al., 2010). In agreement with this, short periods of bed rest (i.e., 5 days) appear to not cause detectable changes in systemic inflammatory markers in young healthy adults (Hamburg et al., 2007; Nosova et al., 2014).
Overall, these findings indicate that acute, short and long-term inactivity can induce increases in inflammatory markers particularly in older adults or at-risk populations. Although modulation of inflammatory profile is frequently shown to occur in concomitance with a decline in vascular function in the context of physical inactivity, a causative link between inflammation and inactivity-induced endothelial dysfunction is yet to be established.
5.4 Oxidative stress
Oxidative stress has also been suggested as a likely physiological mechanism by which physical inactivity may impair vascular function. Oxidative stress is characterized by an imbalance between elevated reactive oxygen species (ROS) formation and a low antioxidant defence in the body (Pizzino et al., 2017). Elevated levels of ROS are considered a contributing factor for the development of hypertension and atherosclerosis (Cai 2005; Suvorava et al., 2005; Münzel et al., 2017), possibly due to their involvement in vasoconstriction within resistance vessels (Jones and Morice 2000). This idea of oxidative stress as a potential mechanism is supported by observations that supplementation with dietary antioxidants (e.g., vitamin C) prevents declines in endothelial function during prolonged sitting in humans (Thosar et al., 2015a) and attenuates hydrogen peroxide (H2O2) production and muscle atrophy in rodent models of immobilization (Min et al., 2011). This may be of significance given the known association between low skeletal muscle mass and increased risk of arterial stiffness, coronary artery calcification, and reduced arterial size (Radegran and Saltin 2000; Ko et al., 2016; Yang et al., 2020).
However, there is limited direct evidence for modifications in oxidative stress markers as a consequence of inactivity in humans. Gram et al. (2015) examined the effects of a short-term inactivity intervention (i.e., 2 weeks of unilateral lower limb immobilization) followed by an exercise intervention (i.e., 6 weeks of aerobic cycle training) on mitochondrial ROS production within the vastus lateralis muscle in healthy young and older men. Their findings showed that lower limb immobilization resulted in augmented mitochondrial H2O2 production, and this was reversed by exercise. In line with this, 5 weeks of bed rest in healthy young men resulted in increased protein carbonylation, a marker of oxidative stress, in vastus lateralis muscle (Dalla Libera et al., 2009). Further, cross-sectional studies reported higher levels of global oxidative stress in sedentary older adults in comparison to non-sedentary (Carmeli et al., 2015). Conversely, a short-term lower limb immobilization protocol showed no significant changes in oxidative stress markers (i.e., protein carbonyls, and 4-hydroxy-2-nonenal adducts) (Glover et al., 2010), and no changes in circulating lipid peroxidation (malondialdehyde) were observed after prolonged sitting in humans (Ballard et al., 2017).
Animal studies report increased production of ROS and enhanced activity of NADPH oxidase (an enzyme responsible for the release of superoxide) in the endothelium and the media of the aortic wall after 6 weeks of reduced physical inactivity, which was accompanied by impaired endothelium-dependent vasodilation and accelerated atherosclerotic plaque development (Laufs et al., 2005). Similarly, hindlimb immobilization (12; 14 days) and unloading (28 days) in rodents also resulted in mitochondrial production of ROS (i.e., hydrogen peroxide) and reduced skeletal muscle mass (Kondo et al., 1991; Lawler et al., 2003; Min et al., 2011). Despite some evidence in animal and human studies that inactivity can affect levels of ROS and NADPH oxidase activity in muscle and vascular tissue, the evidence for the role of oxidative stress on physical inactivity-induced endothelial dysfunction remains to be established.
5.5 Haemodynamics and rheological factors
The importance of shear stress and its role in maintaining and regulating vascular health and function is well-established (Gnasso et al., 2001; Thosar et al., 2012; Green et al., 2017). Shear stress is defined as the frictional force that blood flow exerts on the inner layer of the vessel wall (i.e., endothelium) (Fernandes et al., 2018), and is quantified by multiplying shear rate (equal to velocity/diameter) by blood viscosity (Pyke and Tschakovsky 2005). The majority of studies investigating inactivity do not measure blood viscosity, and therefore, shear stress. Typically, shear rate is measured instead, which represents an acceptable surrogate measure of shear stress (Pyke and Tschakovsky 2005). Several studies have shown that exercise-induced increases in shear rate represent an important physiological stimulus for improving endothelial function (Tinken et al., 2009; Tinken et al., 2010; Birk et al., 2012). On the other hand, prolonged sitting has been shown to consistently lead to reductions in shear rate in both upper and lower limb arteries, as described previously in this review. Importantly, continuous/intermittent increases in shear rate, through local heating, prevents declines in endothelial function (measured by FMD) observed during periods of restricted/reduced physical activity (Restaino et al., 2016; Teixeira et al., 2017). This suggests that shear rate is likely underpinning changes in vascular function during periods of inactivity. Indeed, the majority of studies (e.g., Thosar et al., 2015a; Morishima et al., 2016; Morishima et al., 2017) consistently showed that declines in FMD in lower limb arteries are accompanied by reductions in shear rate during prolonged sitting. However, this relationship is less clear in the upper limb arteries, where consistent reductions in shear rate are not always paralleled by impairments in FMD (Thosar et al., 2014; Restaino et al., 2015).
Viscosity is a measure of fluid friction that directly influences the magnitude of shear rate and has also been observed to increase in response to sitting in the lower, but not the upper limb arteries (Hitosugi et al., 2000). This may explain, at least partially, the specificity of the vascular deficits observed in the lower limb arteries during sitting studies. Given that typically lower shear rate results in higher blood viscosity (Chien 1970; Dormandy 1970; Sriram et al., 2014), it is plausible to hypothesize that declines in shear rate observed during sitting may contribute to increases in viscosity, and together these are likely to impact vascular function (e.g., FMD). However, to date, no studies have specifically explored this relationship during inactivity protocols.
In short-term inactivity models, the relationship between blood flow/shear rate and lower limb FMD may be less consistent than in prolonged sitting. For example, Bleeker et al. (2005) did not observe any significant change in blood flow and FMD (corrected for shear rate) in the superficial femoral artery in response to 52 days of bed rest. On the contrary, 12 days of unilateral lower limb immobilization induced increases in both mean shear rate and FMD in the popliteal artery (Rakobowchuk et al., 2011). These contrasting relationships might be driven by the reduction in arterial size observed after longer periods of physical inactivity, which is known to influence mean shear rate and consequently FMD (Pyke and Tschakovsky 2005). However, looking at mean shear rate during inactive periods might not be as informative as looking at its components (anterograde and retrograde shear rate), when trying to establish links with endothelial function. For example, a recent study showed that artificially modulating anterograde and retrograde shear rate patterns alone, without altering mean shear rate, results in increases in FMD (Holder et al., 2019). Indeed, controlled increases and decreases in anterograde shear rate alone result in enhancement and attenuation of FMD, respectively (Tinken et al., 2009). In addition, both acute and prolonged experimental increases in retrograde shear rate reduce brachial FMD/diameter in young healthy men (Thijssen et al., 2009; Thijssen et al., 2015), highlighting the importance of anterograde and retrograde shear rate in modulating vascular function in humans. Indeed, a few studies report declines in anterograde shear rate in brachial and superficial femoral arteries during sitting (Thosar et al., 2014; Thosar et al., 2015a; Thosar et al., 2015b), while others do not (McManus et al., 2015; Carter et al., 2019). However, it is currently difficult to clearly define the impact of altered shear rate patterns (anterograde and retrograde shear rate) in inactivity-induced endothelial dysfunction, as the majority of studies measured only mean shear rate.
The arterial bending of lower limbs that accompany sitting positions can further affect shear rate/stress characteristics. Under normal conditions and based on their geometrical properties, straight conduit arteries (e.g., brachial artery) have typically laminar blood flow (Rabby et al., 2014; Evju and Mardal 2015) and therefore, high wall shear rate/stress (Paszkowiak and Dardik 2003; Chiu and Chien 2011). However, alteration in arterial shape due to arterial bending reduces blood flow and may predispose the artery to experience disturbed blood flow distal to the bending site (Walsh et al., 2017). Disturbed blood flow, a nonuniform and irregular flow, is mainly characterized by low and reciprocating shear stress (due to a periodic flow in which its velocity oscillates in backward and forward direction) (Chiu and Chien 2011), and is typically observed in areas prone to atherosclerosis (i.e., arterial branches and curvatures), such as femoral arteries (Chiu and Chien 2011; Heo et al., 2014). Accumulating evidence indicates that disturbed blood flow has negative effects on the vasculature (Chiu and Chien 2011; Heo et al., 2014), including reductions of eNOS expression (Gambillara et al., 2006), increase of ROS production (Heo et al., 2011), and promotion of endothelial cell activation and apoptosis (Jenkins et al., 2013).
Interestingly, sitting studies report reductions in blood flow and/or shear rate once participants transition from supine to the sitting position (Restaino et al., 2016; Vranish et al., 2017; Vranish et al., 2018), and a recovery of blood flow when moving back to supine position (Morishima et al., 2017). In agreement with this, Walsh et al. (2017) have shown that when the leg is bent at 90° at the hip and knee during the lateral lying down position, there is a significant decline in popliteal artery FMD, similarly to what has been observed during sitting. This was further accompanied by reductions in mean, anterograde, and retrograde shear rate within the first hour of arterial bending, which partially reflects what has been observed during prolonged sitting (Thosar et al., 2014; Thosar et al., 2015a; Thosar et al., 2015b; Morishima et al., 2020a; Morishima et al., 2020b). This highlights that turbulent flow patterns induced by arterial bending might also contribute to declines in endothelial function during prolonged sitting.
In summary, there is some evidence to suggest that reduced anterograde shear rate, higher blood viscosity, and disturbed blood flow may contribute to endothelial dysfunction during physical inactivity periods, particularly during prolonged sitting, but more studies are needed to establish these relationships.
5.6 Hydrostatic pressure
As outlined above, the vasculature in the upper and lower limb arteries seems to be differentially affected during periods of inactivity (Thosar et al., 2014; Restaino et al., 2015; Climie et al., 2018). The mechanisms underlying these differences are not clear, however, it is known that blood pressure in the lower limbs is generally higher in comparison with upper limbs (Sheppard et al., 2019) independently of the body posture adopted (Pollack and Wood 1949; Gemignani et al., 2012). These differences in blood pressure, particularly during sitting and standing (Pollack and Wood 1949; Gemignani et al., 2012), may be attributed to elevated hydrostatic pressure, which is a component of blood pressure that refers to the force that blood exerts on the blood vessels due to the effect of gravity (Badeer 2001; Darwish and Lui 2020). Indeed, in vitro studies show that whilst physiological hydrostatic pressure (i.e., ∼11 mmHg) for short periods (i.e., 45 min) protects endothelial cells integrity (Muller-Marschhausen et al., 2008), sustained exposure stimulates the proliferation of human endothelial cells (Acevedo et al., 1993; Schwartz et al., 1999)—a response that has been linked to atherosclerosis (Fuster et al., 2010). Furthermore, elevated hydrostatic pressure (i.e., ≥50 mmHg) results in a reduction in endothelial barrier function (Prystopiuk et al., 2018) and VE-cadherin protein expression (Ohashi et al., 2007), both of which have been associated with endothelial cells apoptosis (Carmeliet et al., 1999). A study in humans reported that acute exposure of the upper limb arteries to increased hydrostatic pressure induced declines in FMD (Padilla et al., 2009), suggesting a link between hydrostatic pressure and endothelial dysfunction.
The lower body vasculature is chronically exposed to higher hydrostatic pressure and this may explain why lower limb arteries have reduced vascular responsiveness and increased vascular susceptibility to inactivity-induced detrimental effects (Bleeker et al., 2005; Thosar et al., 2014; Restaino et al., 2015; Climie et al., 2018). However, it is currently not clear to what extent hydrostatic pressure contributes to the difference in vascular function responses between upper and lower limbs in the context of physical inactivity. In addition, it has been suggested that increased hydrostatic pressure in lower extremities during sitting may further induce blood pooling (Padilla and Fadel 2017), which refers to the slowing of blood within the venous circulation due to lack of skeletal muscle activity (Tansey et al., 2019). The increase in hydrostatic pressure within the vascular tree forces the fluid to filter out from blood vessels into the interstitial space, leading to localized edema (Hall and Hall 2020). In support of this, several studies have shown that sitting-induced declines in FMD are accompanied by blood pooling in lower limbs, as reflected by marked increase in calf (Restaino et al., 2015; Vranish et al., 2017; Kruse et al., 2018; Credeur et al., 2019; O’Brien et al., 2019) and ankle circumference (Morishima et al., 2016).
Increased lower limb blood pooling contributes to a cascade of physiological events, in particular a reduction in central venous return, ventricular filling, stroke volume and blood pressure, which results in an increase in sympathetic nervous system activity by stimulation of baroreflexes (Freeman 2006; Stone et al., 2016). This can be clinically relevant given that increase in sympathetic nervous system activity, which is known to induce peripheral vasoconstriction, affects vascular function through possible inhibition of shear-mediated NO production (Amiya et al., 2014).
Overall, sitting may induce increase in hydrostatic pressure in lower limb blood vessels that results in leg blood pooling. Both hydrostatic pressure and blood pooling appear to have negative effects on the vasculature, and therefore, they might contribute to the underlying physiological mechanisms by which sitting affects lower limb vascular function.
6 Long-term consequences of physical inactivity
6.1 Arterial remodelling and hypertension
Arterial remodelling refers to a set of functional and structural adaptations of the blood vessel wall in response to disease, injury, aging (Van Varik et al., 2012) and also exposure to regular periods of physical activity/inactivity (Thijssen et al., 2011; Thijssen et al., 2012). Vascular remodelling includes inward remodelling (reduction in arterial lumen) and outward remodelling (increase in arterial lumen), and depending on variations in vascular wall thickness, vessels may undergo hypotrophic (reduction in arterial wall), eutrophic (no alteration in arterial wall), and hypertrophic (increase in arterial wall) remodelling (Van Varik et al., 2012).
Vascular inward eutrophic remodelling in blood vessels appears to take place in response to short-term inactivity protocols. In particular, bed rest, limb immobilization and step reduction result in declines in arterial diameter in the brachial, femoral (common and superficial), and popliteal arteries (Sugawara et al., 2004; Bleeker et al., 2005; Hamburg et al., 2007; Rakobowchuk et al., 2011; Boyle et al., 2013), without alterations in femoral artery intima-media thickness and intima-media thickness/lumen ratio (Sugawara et al., 2004). Importantly, there is currently well-established evidence linking vascular inward remodelling to hypertension (Baumbach and Heistad 1989; Skov et al., 1992; Rizzoni et al., 1996; Mulvany 1999; Intengan and Schiffrin 2001; Rizzoni et al., 2009; Schiffrin 2012; Brown et al., 2018). However, the literature focuses particularly on the link between hypertension and inward remodelling of small arteries, such as resistance and cerebral arteries, while the link between hypertension and inward remodelling of conduit arteries (e.g., femoral, and brachial arteries) is not yet established. Nonetheless, inactivity might contribute to the development of hypertension through the inward remodelling process of resistance vessels. In fact, individuals with essential hypertension show signs of inward eutrophic remodelling in resistance vessels, which result from an increased media-to-lumen ratio without altering the cross-sectional area of the tunica media (Mulvany 1999; Brown et al., 2018). This type of alteration seems to occur in both the upper and lower body vasculature in response to inactivity protocols (i.e., bed rest, and limb immobilization), as indicated by local reduction in peak blood flow (Hamburg et al., 2007; Birk et al., 2013), which is a surrogate measure of resistance vessel remodelling (Thijssen et al., 2011). This may be clinically relevant considering that structural alteration of resistance arteries is a powerful predictor of cardiovascular events in high-risk populations (Rizzoni et al., 2003; Mathiassen et al., 2007). Indeed, several epidemiological studies show an association between sedentary behaviour and increased risk of developing hypertension (Beunza et al., 2007; Lee and Wong 2015; Lim et al., 2017).
Collectively, these observations highlight that inactivity can induce vascular remodelling in resistance and conduit arteries and this is likely linked to increased incidence of hypertension in sedentary individuals.
6.2 Atherosclerosis
Atherosclerosis is a chronic inflammatory disease characterized by a progressive accumulation of fatty deposits and fibrous components (forming plaques) in the inner layer of the artery (tunica intima), resulting in narrowing of the lumen and thickening of the arterial wall (Ross 1999; Lusis 2000; Rafieian-Kopaei et al., 2014). This results in inadequate blood perfusion to organs (e.g., heart, brain) or extremities (e.g., lower limbs) (Ross 1999; Rafieian-Kopaei et al., 2014) that can lead to serious complications such as heart attack, stroke, or PAD (Banerjee and Chimowitz 2017; Shu and Santulli 2018; Palasubramaniam et al., 2019). In particular, PAD is characterized by the accumulation of atherosclerotic plaques in the peripheral arteries in the lower extremities (Zemaitis et al., 2017). Several studies have shown that atherosclerotic lesions typically develop in areas characterized with low anterograde shear stress (Gnasso et al., 1997; Cheng et al., 2006; Heo et al., 2014) and high OSI (Wu et al., 2004). These atherosclerosis-prone areas are typically vessel curvatures, bifurcations, and branch points in which the blood flow changes its pattern from laminar to disturbed blood flow (Owen and Roberts 2007; Jenkins et al., 2013). Alterations in blood flow patterns predispose the vasculature to accumulation of atherosclerotic plaques by inducing expression of atherogenic genes and proteins (Nigro et al., 2011). Indeed, a recent longitudinal study has shown a higher prevalence of atherosclerosis within the femoral arteries in comparison with coronary and carotid arteries, and a more robust association between femoral atherosclerosis and cardiovascular risk factors (Laclaustra et al., 2016).
During sitting, the bending of the lower limbs further predisposes those arteries (e.g., popliteal and femoral arteries) to atherogenic disturbed blood flow distal to the bending site (Walsh et al., 2017), which contributes to altered shear patterns and reduced endothelial function (Chiu and Chien 2011; Jenkins et al., 2013). In line with this and as described previously in this review, acute and short-term inactivity interventions consistently result in decreases in shear rate and impairments in vascular function in the lower limb arteries (e.g., Thosar et al., 2014; Thosar et al., 2015a; Thosar et al., 2015b; Restaino et al., 2015; Morishima et al., 2016). This is also relevant given that impairments in endothelial function in the popliteal artery seem closely related to increases in intima-media thickness (Iwamoto et al., 2016), which is a valuable marker of subclinical atherosclerosis (de Groot et al., 2004; Harrington et al., 2010; Bauer et al., 2012). Collectively, these findings suggest that lower limb atherosclerosis is likely one of the long-term consequences of inactivity-induced endothelial dysfunction.
In agreement with this, a large population-based study showed a dose-response relationship between sedentary time and increased risk of atherosclerotic PAD (Unkart et al., 2020). Further studies in both healthy and at-risk populations have reported that time spent in sedentary activities (e.g., TV viewing) is directly associated with the progression of coronary artery calcification, and carotid intima-media thickness (Kozàkovà et al., 2010; Delaney et al., 2013; García-Hermoso et al., 2015; Diaz et al., 2016; Lazaros et al., 2019). Other studies have shown associations between sedentary time and either low ankle-brachial index values or PWV, which are both non-invasive tools for detecting PAD development, and arterial stiffness, respectively (Horta et al., 2015; Kulinski et al., 2015; Ahmadi-Abhari et al., 2017). The impact of physical inactivity on the progression of PAD has also been demonstrated in epidemiological and experimental studies in spinal cord injury patients (Bell et al., 2011; Su et al., 2015).
Collectively, these findings highlight that vascular alterations induced by inactivity may play an important role in the development of atherosclerosis, particularly in the lower limbs.
6.3 Skeletal muscle function/mass
It is well-established that increased sedentary behaviour is associated with reduced skeletal muscle mass and greater risk of developing sarcopenia (Gianoudis et al., 2015; Aggio et al., 2016; Smith et al., 2020). Sarcopenia is an age-related condition characterized by a progressive reduction of skeletal muscle mass and function (Santilli et al., 2014; Cruz-Jentoft and Sayer 2019) that can develop as early as the 4th decade of life (Metter et al., 1997). Studies have shown that inactivity interventions such as bed rest (10 days–17 weeks) (LeBlanc et al., 1992; Paddon-Jones et al., 2004; Kortebein et al., 2008; Coker et al., 2015), step reduction (14 days) (Krogh-Madsen et al., 2010; Breen et al., 2013; Davies et al., 2018; McGlory et al., 2018), unilateral arm (Kitahara et al., 2003; Birk et al., 2013) and leg immobilization (5–21 days) (Glover et al., 2008; Suetta et al., 2009; Dirks et al., 2014), result in reductions in muscle protein synthesis rate, muscle function, and muscle mass, which occur mainly in the lower limbs.
Reduced blood perfusion during inactivity, which may be the consequence of multiple factors including impairments in NO signalling and skeletal muscle capillarity (Gram et al., 2014), may contribute to the muscular functional impairments observed, particularly within the context of sarcopenia (Zempo et al., 2017; Hendrickse and Degens 2019). Consistent with this, Rasmussen et al. (2006) showed increases in leg blood flow and muscle protein synthesis in response to insulin infusion in young healthy adults and further reported that insulin-induced increases in blood flow were positively correlated with changes in muscle protein synthesis. Similarly, Volpi et al. (2000) showed that an increase in endogenous insulin concentrations due to amino acid-glucose administration resulted in increased blood flow and muscle protein anabolism in young individuals. Importantly, when insulin or amino acids were infused in combination with the NO donor sodium nitroprusside, improvements in muscle protein anabolism in both young and elderly adults were observed (Timmerman et al., 2010; Dillon et al., 2011). Collectively, these findings support a role for blood flow in regulating muscle protein anabolism. Therefore, the decline in blood flow that results from physical inactivity and particularly sitting may contribute to impairments in protein anabolism and loss of muscle mass linked to sedentary behaviour. Another aspect that may contribute to functional and structural impairments in skeletal muscle tissue is arterial stiffness. Several studies have indeed shown negative associations between arterial stiffness and skeletal muscle mass/strength (Ochi et al., 2010; Im et al., 2017; Zhang et al., 2019). As such, given the experimental evidence showing increases in arterial stiffness (i.e., PWV) in response to short bouts of sitting (Credeur et al., 2019; Evans et al., 2019), this may indicate another mechanism by which inactivity can contribute to muscular impairments. However, the links between vascular and muscular dysfunction in the context of inactivity have not been experimentally established. Currently, it is not clear whether inactivity-induced vascular dysfunction is causally contributing to a decline of functional and structural aspects of skeletal muscle tissue.
7 Preventive strategies for counteracting negative effects of physical inactivity
7.1 Physical exercise
Physical activity is naturally one of the main interventions used to attempt to prevent vascular detrimental effects of prolonged inactivity. Many epidemiological studies have highlighted the beneficial associations between physical activity and prognostic outcomes for vascular health, whilst sedentary activity tends to be associated with poorer outcomes (Gomez-Marcos et al., 2014; Horta et al., 2015). A longitudinal study in elderly adults, reported that increases in arterial stiffness over a 5-year period (specifically, 0.76 m⋅s−1 increase in carotid-femoral PWV) were significantly attenuated by 0.02 m⋅s−1 for every additional hour per week of moderate-to-vigorous physical activity, whilst increased sedentary time was associated with higher rates of arterial stiffness (Ahmadi-Abhari et al., 2017). Furthermore, among highly active individuals the association between sedentary time and the relative risk for all-cause mortality is 30% lower in comparison with less active individuals (Biswas et al., 2015). Moreover, replacing 1 h per day of sitting with moderate-to-vigorous cycling exercise resulted in improvements in biomarkers of endothelial dysfunction (i.e., ICAM-1, E-selectin) in young healthy and at-risk adults (Duvivier et al., 2018). Similarly, in children, replacing 1 h per day of sedentary time with light/moderate-to-vigorous physical activity showed beneficial effects on the clustering of cardio-metabolic risk (i.e., systolic and diastolic blood pressure, waist circumference, and HDL cholesterol) (Wijndaele et al., 2019). Overall, these studies indicate that habitual physical activity even in small increments can have beneficial long-term consequences for cardiovascular health (Palombo and Kozakova 2016).
Human randomized experimental studies have further assessed the efficacy of bouts of physical activity as a strategy to interrupt prolonged sitting (Figure 3) (Table 3). Specifically, interrupting sitting every 30–60 min with short walking/cycling breaks (2–10 min) has been shown to be effective in preventing declines in lower limb endothelial function across different populations (Thosar et al., 2015a; McManus et al., 2015; Hartman et al., 2021). Similarly, 3 min of simple resistance activities, such as half squats, calf raises, and single knee raises, every 30 min (within a 7-h sitting period) are sufficient to prevent sitting-induced declines in femoral FMD, resting blood flow and shear rate in middle-aged/elderly adults (Climie et al., 2018), as well as reported benefits in young women with PCOS (Taylor et al., 2020) and adults with type 2 diabetes (Taylor et al., 2021). Furthermore, intermittent leg fidgeting (1 min on, 4 min off during a 3-h sitting protocol) has been shown to ameliorate popliteal FMD and attenuate declines in shear rate in young healthy adults (Morishima et al., 2016). The beneficial impact of physical activity is also observed in upper limb arteries, albeit to a lesser extent, with a recent study reporting that callisthenic exercises (2-min set every 20 min) during 1.5 h of sitting elicited an increase in brachial artery shear rate in young healthy adults (Carter et al., 2017). Finally, a more recent study has found, within the same population, that performing stair climbing breaks (5 min every hour) during a 4-h sitting intervention, improves FMD and shear rate in brachial and popliteal artery, respectively (Cho et al., 2020). However, not all studies have shown such positive effects. For example, Kruse and colleagues found no benefits in the popliteal artery with brief intermittent bouts of light-intensity desk pedalling during a 4-h sitting period in early middle-aged overweight/obese adults (Kruse et al., 2018), and Carter and colleagues did not observe any significant positive effects on a range of vascular outcomes (e.g., FMD and shear rate), with the exception of marginal benefits on blood flow and vascular conductance, in young adults when utilising activity breaks (i.e., 2-min walking every 30 min; 8-min walking every 120 min) during their 4-h prolonged sitting protocol (Carter et al., 2019). Collectively, these findings may indicate that higher exercise intensity/duration may be needed in some populations in order to counteract the negative vascular effects induced by uninterrupted prolonged sitting.
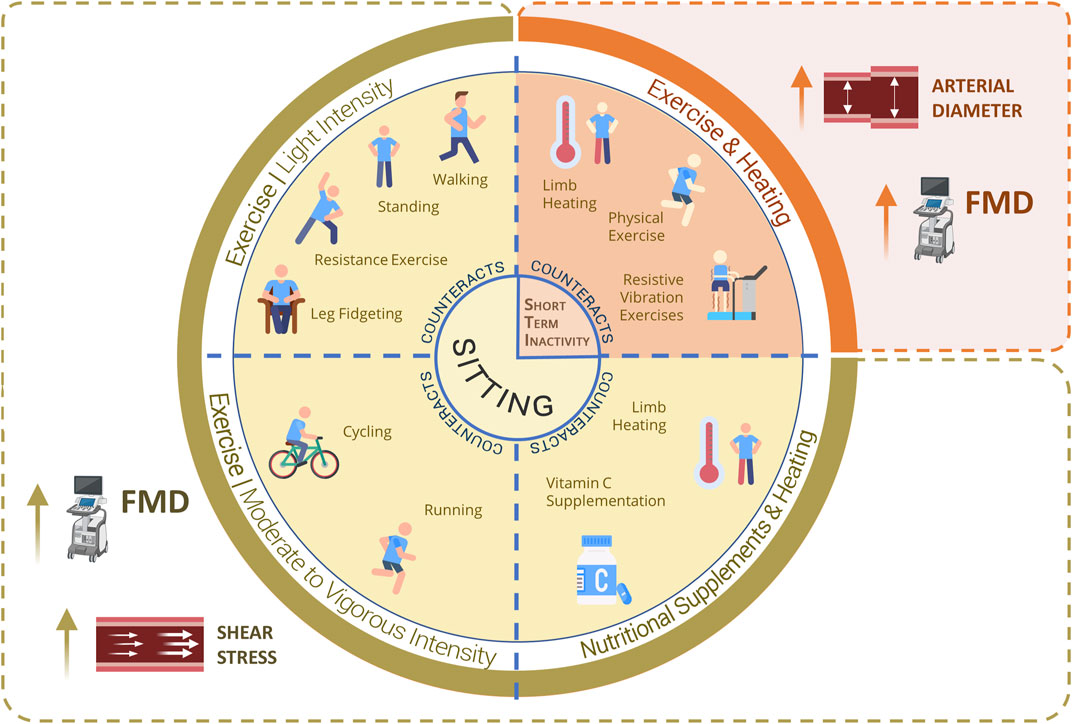
FIGURE 3. Summary of intervention strategies that have been shown to be effective at preventing detrimental effects on vascular health during periods of physical inactivity. Standing, intermittent leg fidgeting, walking, running, cycling, resistance exercises, limb heating and vitamin C supplementation were shown to be effective at improving FMD and/or shear stress/rate in the context of prolonged sitting. Resistive vibration exercises, limb heating and general physical activity were shown to be effective at preventing declines in FMD and/or arterial diameter during inactivity protocols such as bed rest, step-reduction, and leg immobilization. FMD: Flow-mediated dilation.
The performance of physical activity before or immediately after a prolonged period of sedentary behaviour also seems to be beneficial for vascular function. For example, Restaino et al. (2015) reported that 10 min of walking at the end of a 6-h sitting trial reversed the decline in mean blood flow (resting, and hyperaemic), shear rate, and popliteal artery FMD, with no benefits detected in the brachial artery (Restaino et al., 2015). Recent studies also showed that 45 min of aerobic exercise on a cycle ergometer (Morishima et al., 2017) and on a treadmill (Ballard et al., 2017) prior to a 3-h sitting bout effectively prevented reductions in FMD on the popliteal and superficial femoral artery, respectively. Importantly, exercise-induced vascular improvements during prolonged sitting are also accompanied by metabolic changes, such as reductions in postprandial plasma glucose (Bailey and Locke 2015; Bhammar et al., 2017; Champion et al., 2018) and insulin concentrations (Dunstan et al., 2012; Peddie et al., 2013; Larsen et al., 2015; Henson et al., 2016; Yates et al., 2020), as well as benefits to systolic/diastolic BP (Larsen et al., 2014; Zeigler et al., 2015; Dempsey et al., 2016a; Zeigler et al., 2016; Bhammar et al., 2017; Champion et al., 2018) and vascular biomarkers (i.e., ET-1) (Climie et al., 2018). Overall, this highlights a crucial role that physical activity can play in preventing peripheral vascular and cardiometabolic damages induced by prolonged sitting.
In the context of longer periods of physical inactivity, there are only a few studies investigating the efficacy of controlled physical activity interventions. Reynolds et al. (2015) observed that 1 day of normal ambulatory activity (>12,000 steps per day) following 5 days of step reduction (<4,000 steps per day) was not sufficient to exert any significant improvement in insulin sensitivity (which was reduced by inactivity), or insulin-stimulated blood flow responses and vascular conductance in the femoral and brachial artery (Reynolds et al., 2015). As such, longer periods of inactivity may require more intense physical activity or a greater duration of time (i.e., number of days) to recover from the inactivity-induced vascular impairments, but more studies are needed to establish this. Alternatively, exercising with the use of a resistive vibration footplate, specifically by performing squats, heel raises, toe raises, and ‘kicks’ (twice daily for 30 min), during horizontal bed rest (52 days), is reported to prevent arterial size reduction in the lower limbs (Bleeker et al., 2005). A similar physical intervention has been used during 60 days of head-down tilt bed rest and resulted in attenuations in the decline of the superficial femoral artery diameter and prevented the increase in intima-media thickness (in carotid, and superficial femoral artery). However, when the exercise was performed without the use of the resistive vibration footplate, it was not sufficient to attenuate the inactivity-induced reduction in diameter of the superficial femoral artery (van Duijnhoven et al., 2010a; van Duijnhoven et al., 2010b). Similarly, a combination of aerobic (35 min running; 3–4 times per week) and resistance exercise (∼30 min; every 3 days) prevented increases in circulating endothelial cells, a biomarker of endothelial damage, and declines of endothelium-dependent vasodilation in cutaneous microcirculation during head-down tilt bed rest (Demiot et al., 2007). Finally, a recent study has shown that a 16-week intervention of reduced sitting combined with low-intensity physical activity improved the FMD response and increased the diameter of the superficial femoral artery in elderly individuals with increased cardiovascular risk (Hartman et al., 2021).
In addition to the benefits described for the peripheral vasculature, there is also some evidence showing physical activity-induced improvements in cerebrovascular function during prolonged sitting. For example, Carter et al. (2018) reported beneficial effects on brain vasculature in response to walking breaks (i.e., 2-min walking breaks every 30 min, and 8-min walking breaks every 120 min). Interestingly, shorter and more frequent walking breaks improved cerebral autoregulation and prevented the reductions in MCAv observed following 4 h of uninterrupted sitting. On the other hand, longer and less-frequent walking breaks were not sufficient to prevent sitting-induced declines in MCAv. This suggests that frequency of physical activity may be important to prevent declines in blood flow during prolonged sitting (Carter et al., 2018). Additionally, performing moderate-intensity walking (30 min) alone or in combination with walking breaks during prolonged sitting (8 h) has been shown to ameliorate changes in MCAv towards the end of the sitting period in comparison to sitting alone (Wheeler et al., 2019).
Collectively, there is encouraging evidence suggesting that different types of physical activity are effective in preventing impairments in both peripheral and cerebral vasculature function in the context of acute and short-term inactivity periods in young healthy adults, but benefits in other populations need further research.
7.2 Standing
Office-based workers spend a considerable amount of time seated daily (Fisher et al., 2018) and sit-stand workstations have proven effective in reducing sitting time in the office environment (Hedge and Ray 2004; Alkhajah et al., 2012; Pronk et al., 2012), although their efficacy at reducing vascular impairments is less clear. For example, Morishima et al. (2017) investigated the effects of two different 3-h interventions (standing vs. sitting) on lower limb vascular function in young healthy individuals. Interestingly, prolonged standing was effective in preventing sitting-induced declines in shear rate and FMD in the popliteal artery (Morishima et al., 2017). Similarly, two more recent studies showed that replacing sitting with standing for 2–6 h was effective in preventing the sitting-induced declines in vascular function in lower limb arteries (Caldwell et al., 2020; Peddie et al., 2021). Other studies have further shown that alternating sitting and standing (every 30/60 min) is an effective strategy for reducing SBP (Zeigler et al., 2016), DBP, MAP, and carotid-ankle PWV (Barone Gibbs et al., 2017). However, Kruse and colleagues reported that interrupting 4 h of sitting with standing (10-min bouts of standing at the beginning of each hour) was not effective at preventing sitting-induced decline in FMD in the popliteal artery in sedentary middle-aged adults (Kruse et al., 2018). This suggests that populations that are chronically sedentary may need longer and/or more intense physical interventions (rather than standing) to prevent transient vascular impairments caused by sitting. Graves et al. (2015) showed that the use of a sit-stand workstation during 8 weeks in a group of healthy middle-aged adults resulted in only a significant reduction in total cholesterol, whilst BP and brachial artery FMD were not affected significantly. However, no objective measurements of sitting, standing, and walking time were taken in that study.
Despite some of the experimental studies indicating vascular benefits of standing, epidemiological studies suggest that long periods in this particular posture may be deleterious for vascular health. Indeed, the majority of studies report associations between prolonged standing at work and high prevalence of venous vascular diseases (Krijnen et al., 1997; Tomei et al., 1999; Tüchsen et al., 2000; Tüchsen et al., 2005; Yun et al., 2018). Furthermore, standing at work has been associated with increase in carotid intima-media thickness (Krause et al., 2000), and decline in blood pressure (measured on the wrist), which is suggested to be the result of impaired venoconstriction and blood pooling in the lower extremities (Ngomo et al., 2008).
Overall, standing may be a valid alternative for preventing vascular dysfunction associated with short periods of prolonged sitting. However, recommendations of standing desks in the office environment, as a way to break sitting time, need to be accompanied by more specific guidelines on frequency and duration of standing that elicit maximal benefits and minimize other vascular complications.
7.3 Thermal stress
Local heating has been shown to result in rapid increases in blood flow, shear rate and FMD in upper and lower limb conduit arteries (Tinken et al., 2009), similarly to those occurring as a result of cycling and handgrip exercise (Tinken et al., 2009). In the context of prolonged sitting, unilateral foot heating exposure (at 42–49°C) during an uninterrupted sitting protocol (2–3 h) prevented the sitting-induced reduction in mean shear rate and FMD in the popliteal and femoral artery in a group of healthy young adults (Restaino et al., 2016; Caldwell et al., 2020). In line with this, intermittent foot heating (at 42°C; 3 times per day) during 5 days of reduced activity (from >12,000 to <4,000 steps per day) appears effective at preventing inactivity-induced decline in the popliteal artery FMD, although no significant changes in reactive hyperaemia and arterial diameter were detected (Teixeira et al., 2017). The positive effects observed here are likely due to the increase in leg blood flow and mean shear rate in response to each heating session (Teixeira et al., 2017). Finally, a daily 2-h heating treatment (via pulsed shortwave diathermy on the vastus lateralis) during 10 days of leg immobilization increases the hyperaemic response to passive leg movement, as well as attenuates the reductions in femoral artery diameter and VEGF (Hyldahl et al., 2021).
Collectively, these findings indicate that local heating interventions may also be an effective strategy to prevent endothelial dysfunction induced by acute and short-term physical inactivity, by modulating blood flow and shear rate. However, from a practical perspective, it might be a challenging alternative and not feasible in most situations (e.g., office workplace).
7.4 Diet
Eating and sitting are often concurrent behaviours, presenting a unique opportunity to use dietary strategies to counteract the negative effects of sitting. Despite this, the topic has received little attention to date. Of the limited studies that have explored this in human participants, Thosar and colleagues showed that vitamin C ingestion during 3 h of uninterrupted sitting (1 tablet of 1 g after 30 min; 1 tablet of 500 mg after 90 min) was effective in preventing sitting-induced reductions in shear rate (i.e., anterograde and mean) and FMD in the superficial femoral artery in healthy young men (Thosar et al., 2015a). Given the high antioxidant capacity of vitamin C (Kim and Lee 2004), their study highlighted that sitting-induced impairments in endothelial function may be at least partially driven by increased oxidative stress within the vasculature, although this was not directly investigated within this study. Interestingly, a more recent study showed that vitamin C ingestion (1 g) 90 min prior to the testing session was effective in preventing sitting-induced reductions in leg microvascular function. However, these positive effects were observed only in male participants and not in female participants (Decker et al., 2021).
In addition, Morishima and colleagues investigated the effects of 8 weeks of fish oil supplementation (containing omega-3 polyunsaturated fatty acids) on popliteal artery vascular function following a 3-h sitting protocol (Morishima et al., 2020a). They reported that fish oil was ineffective in counteracting sitting-induced endothelial dysfunction, with no improvements in shear rate or FMD observed (Morishima et al., 2020a). Considering the beneficial properties of omega-3 fatty acid supplementation on vascular function (Wang et al., 2012), even for a shorter period of time (i.e., 3 weeks) (Żebrowska et al., 2015), the findings from the study of Morishima et al. (2020a) may suggest that the vasculature (especially in lower limb arteries) responds to short-term dietary interventions differently within the context of inactivity. However, caution must be taken before drawing these conclusions as the evidence available is currently very scarce.
8 Summary: Conclusion
Evidence from epidemiological and observational studies show that sedentary behaviour is a major modifiable risk factor for future chronic diseases, increasing the risk of CVD and all-cause mortality, independently of physical activity. Sedentary behaviour is alarmingly high, particularly in older adults (accounting of more than 720 million people worldwide) (Kamiya et al., 2020), with almost 70% estimated to spend more than 8 h daily on sitting or low energy expenditure activities (Harvey et al., 2013). This situation is expected to worsen as demographic projections show in the near future a considerable increase in the elderly population worldwide (Kamiya et al., 2020). Importantly, the performing of physical exercise, particularly at low volume and intensity, may not be sufficient to prevent the harmful impact that sedentary behaviour has on health. However, there is evidence indicating that physical exercise at higher volume and intensity may instead be effective in attenuating but not always eliminating the increased mortality risks associated with sedentary time. Therefore, considering the detrimental health impact of sedentary behaviour, reducing sedentary time in daily life is a crucial first step for improving health status and quality of life. Currently, our understanding of the physiological abnormalities that underlie sedentary behaviour and direct links to long-term health issues is only starting to emerge. This combined knowledge will be critical for the development of effective strategies to offset the negative impact of sedentariness across the lifespan.
Acute (hours) and short-term (days; weeks) physical inactivity periods can have a detrimental impact on peripheral, central, and cerebral vascular health. In particular, prolonged sitting (1–7 h), which has been one of the most studied models of inactivity, affects blood pressure and endothelial function, particularly in the lower body arteries (e.g., femoral, and popliteal arteries), with consistent declines in endothelial-dependent dilation, blood flow, shear rate and microvascular reactivity. These acute modifications experienced repeatedly are likely to contribute to the onset of hypertension and atherosclerosis in the long-term. Short-term inactivity (days; weeks) tend to further affect arterial structure (arterial inward remodelling), suggesting that the duration of the period of inactivity can play a role in the type and severity of impairments. In some cases, reductions in arterial diameter were also accompanied by increases in vascular function which is plausible considering that FMD and shear stress (during reactive hyperaemia) are inversely related to baseline diameter (Silber et al., 2001), thus resulting in greater NO production. In such a case, increase in vascular function should not be considered as a positive outcome. Changes in vascular function during sitting may also extend to the brain, with reductions in blood flow velocity being observed across healthy and at-risk populations. However, the data available linking inactivity and cerebrovascular function is currently limited, especially regarding effects on cognition. This is an area that needs urgent attention given the established epidemiological links between sedentary behaviour, cerebral hypoperfusion and dementia.
Importantly, the effects of inactivity on the body vasculature do not appear to be homogenous, with the upper limb arteries reported to be more resistant to inactivity-induced endothelial dysfunction in comparison to the lower limb arteries. However, one important aspect to consider is that in most of these studies, upper limbs were not completely inactive, and those movements, although light, may have been sufficient for preventing the inactivity-induced reductions in blood flow and shear stress in the brachial artery. Therefore, this might be among the factors behind the lack of impairments in the brachial artery endothelial function as shown in some of these studies. Furthermore, the observed higher susceptibility of the lower limb vasculature, particularly during prolonged sitting, may depend to a certain extent to unique aspects affecting specifically lower limb arteries. These aspects, particularly a more acute arterial bending and a higher hydrostatic pressure result in alterations of arterial haemodynamics which are known to have negative consequences on vasculature. Overall, lower limb vasculature is more susceptible to inactivity, and this may explain the higher incidence of atherosclerotic plaque formation in such arteries as well as the higher blood pressure in lower limbs as compared to upper limbs. Increased disturbed blood flow due to the presence of vessel curvatures, branch points, and bifurcations are likely to play a part, which may ultimately result in low anterograde shear stress and increased expression/synthesis of proatherogenic genes and proteins. Indeed, the established associations between sedentary behaviour and increased risk of atherosclerotic PAD and hypertension may be a consequence of the elevated susceptibility of lower limb vasculature to physical inactivity. However, a causal link between the vascular impairments observed following acute and short-term inactivity interventions and long-term disease is yet to be established.
Many other underlying mechanisms have been explored in the literature and may contribute to inactivity-induced endothelial dysfunction, including a reduction in circulatory levels of vasodilators and increases in vasoconstrictors, modulation of circulating adhesion molecules, oxidative stress biomarkers, inflammatory cytokines, and increases in insulin resistance. However, modulation of these biomarkers does not explain the artery-specific (e.g., lower vs. upper limbs) differences in vascular dysfunction observed during sitting, particularly considering the difficulties in clearly establishing the causal relationship between systemic biomarkers and local changes. In this sense, clinical biomarkers should be ideally collected in situ (e.g., local arterial blood sampling), but this is not always possible especially considering the several potential complications that could derive from it, including nerve damage and excessive bleeding (World Health 2010). Concomitantly, such molecular/physiological mechanisms are poorly understood, with very limited evidence available in human studies, making it challenging to draw firm conclusions. In particular, there is a lack of randomized controlled trials combining gold standard measures of endothelial function in humans and assessment of underpinning molecular mechanisms of action. Therefore, future studies aiming to establish mechanistic causal links between vascular impairments in humans and inactivity-induced endothelial dysfunction are urgently needed.
A variety of strategies have been explored to prevent either acute or short-term inactivity-induced vascular dysfunction, including walking breaks, upright standing, physical exercise (endurance and resistance), local heating, and nutritional strategies. Walking breaks and physical exercise seem to be the most effective at improving vascular function during inactivity periods, whilst data on standing, local heating, and nutritional strategies are rather limited, and in some cases inconsistent. For example, standing interventions have reported selected vascular benefits following periods of inactivity, but epidemiological studies indicate that prolonged periods of standing can also have detrimental consequences for vascular health (e.g., venous vascular diseases, and blood pooling). From a practical point of view, it may be difficult for young adults to use planned exercise/walking breaks or heating interventions routinely, particularly in the office/working environments. Similarly, for older adults it may not be always possible to exercise/walk so frequently, given the numerous barriers to physical activity within this population (e.g., poor physical health, fear and negative experiences, environmental factors), particularly for those who already have mobility limitations (Rasinaho et al., 2007; Spiteri et al., 2019). On the other hand, dietary strategies may be useful in combination with walking/exercise breaks to help counteract the negative effects of prolonged periods of inactivity. Eating and sitting are often concurrent behaviours, presenting a unique opportunity to use dietary strategies to counteract the negative effects of sitting, however, this area of research remains largely unexplored. This is especially relevant in older adults, where prolonged periods of sitting are more likely. Dietary interventions, for example, those using food sources rich in inorganic nitrate or plant-derived polyphenols (e.g., flavonoids), might constitute an effective strategy for preventing impairments in endothelial function associated with inactivity. Current evidence clearly shows clinically relevant improvements in cardiovascular health and endothelial function in response to acute and sub-chronic dietary intake of foods rich in inorganic nitrate (d’El-Rei et al., 2016; Lara et al., 2016; Walker et al., 2019) or plant-derived flavonoids (Hodgson and Croft 2006; Schroeter et al., 2006; Heiss et al., 2007; Rodriguez-Mateos et al., 2013; Heiss et al., 2015; Rees et al., 2018), making these dietary options interesting to explore in the context of sitting-induced impairments in endothelial function and in blood pressure, when longer periods of inactivity are unavoidable (e.g., in old age, during long-haul travel).
In summary, the impact of sedentariness on health is a global problem that affects individuals across the lifespan. This review clearly highlights the need for mechanistic information that can support the evidence-based recommendations/guidelines to counteract the detrimental impact of sedentary behaviour in the population, whilst further highlighting the importance of finding novel strategies to help reduce the negative effects of sitting on health.
Author contributions
Conceptualisation and drafting of the work was carried out by AD and CR. Critical revision and editing was carried out by SL. All authors have read and approved the final manuscript.
Funding
AD PhD studentship is funded by the College of Life and Environmental Sciences, University of Birmingham (2018–2023).
Acknowledgments
Figures were created with BioRender.com in collaboration with João Guerreiro and some icons in Figures 1, 3 were produced by Freepik, Monkik, and Smashicons from flaticon.com. We would like to express our sincere gratitude to Professor Carolyn Greig from the University of Birmingham for the valuable and constructive feedback provided for refining this narrative review.
Conflict of Interest
The authors declare that the research was conducted in the absence of any commercial or financial relationships that could be construed as a potential conflict of interest.
Publisher’s note
All claims expressed in this article are solely those of the authors and do not necessarily represent those of their affiliated organizations, or those of the publisher, the editors and the reviewers. Any product that may be evaluated in this article, or claim that may be made by its manufacturer, is not guaranteed or endorsed by the publisher.
References
Acevedo A. D., Bowser S. S., Gerritsen M. E., Bizios R. (1993). Morphological and proliferative responses of endothelial cells to hydrostatic pressure: Role of fibroblast growth factor. J. Cell. Physiol. 157 (3), 603–614. doi:10.1002/jcp.1041570321
Admiraal W. M., van Valkengoed I. G. M., L de Munter J. S., Stronks K., Hoekstra J. B. L., Holleman F. (2011). The association of physical inactivity with Type 2 diabetes among different ethnic groups. Diabet. Med. 28 (6), 668–672. doi:10.1111/j.1464-5491.2011.03248.x
Aggio D. A., Sartini C., Papacosta O., Lennon L. T., Ash S., Whincup P. H., et al. (2016). Cross-sectional associations of objectively measured physical activity and sedentary time with sarcopenia and sarcopenic obesity in older men. Prev. Med. 91, 264–272. doi:10.1016/j.ypmed.2016.08.040
Ahmadi-Abhari S., Sabia S., Shipley M. J., Kivimäki M., Singh‐Manoux A., Tabak A., et al. (2017). Physical activity, sedentary behavior, and long‐term changes in aortic stiffness: The whitehall II study. J. Am. Heart Assoc. 6 (8), e005974. doi:10.1161/JAHA.117.005974
Alansare A. B., Kowalsky R. J., Jones M. A., Perdomo S. J., Stoner L., Gibbs B. B. (2020). The effects of a simulated workday of prolonged sitting on seated versus supine blood pressure and pulse wave velocity in adults with overweight/obesity and elevated blood pressure. J. Vasc. Res. 57 (6), 355–366. doi:10.1159/000510294
Alibegovic A. C., Højbjerre L., Sonne M. P., van Hall G., Stallknecht B., Dela F., et al. (2009). Impact of 9 days of bed rest on hepatic and peripheral insulin action, insulin secretion, and whole-body lipolysis in healthy young male offspring of patients with type 2 diabetes. Diabetes 58 (12), 2749–2756. doi:10.2337/db09-0369
Alkhajah T. A., Reeves M. M., Eakin E. G., Winkler E. A. H., Owen N., Healy G. N. (2012). Sit–stand workstations: A pilot intervention to reduce office sitting time. Am. J. Prev. Med. 43 (3), 298–303. doi:10.1016/j.amepre.2012.05.027
Allen C., Glasziou P., Del Mar C. (1999). Bed rest: A potentially harmful treatment needing more careful evaluation. Lancet 354 (9186), 1229–1233. doi:10.1016/s0140-6736(98)10063-6
Allison M. A., Jensky N. E., Marshall S. J., Bertoni A. G., Cushman M. (2012). Sedentary behavior and adiposity-associated inflammation: The multi-ethnic study of atherosclerosis. Am. J. Prev. Med. 42 (1), 8–13. doi:10.1016/j.amepre.2011.09.023
Amiya E., Watanabe M., Komuro I. (2014). The relationship between vascular function and the autonomic nervous system. Ann. Vasc. Dis. 7 (2), 109–119. doi:10.3400/avd.ra.14-00048
Archer E., Shook R. P., Thomas D. M., Church T. S., Katzmarzyk P. T., Hebert J. R., et al. (2013). 45-Year trends in women’s use of time and household management energy expenditure. PloS one 8 (2), e56620. doi:10.1371/journal.pone.0056620
Arnardottir N. Y., Koster A., Van Domelen D. R., Brychta R. J., Caserotti P., Eiriksdottir G., et al. (2016). Association of change in brain structure to objectively measured physical activity and sedentary behavior in older adults: Age, Gene/Environment Susceptibility-Reykjavik Study. Behav. Brain Res. 296, 118–124. doi:10.1016/j.bbr.2015.09.005
Badeer H. S. (2001). Hemodynamics for medical students. Adv. Physiol. Educ. 25 (1), 44–52. doi:10.1152/advances.2001.25.1.44
Bailey D. P., Locke C. D. (2015). Breaking up prolonged sitting with light-intensity walking improves postprandial glycemia, but breaking up sitting with standing does not. J. Sci. Med. Sport 18 (3), 294–298. doi:10.1016/j.jsams.2014.03.008
Ballard K. D., Duguid R. M., Berry C. W., Dey P., Bruno R. S., Ward R. M., et al. (2017). Effects of prior aerobic exercise on sitting-induced vascular dysfunction in healthy men. Eur. J. Appl. Physiol. 117 (12), 2509–2518. doi:10.1007/s00421-017-3738-2
Ballermann B. J., Dardik A., Eng E., Liu A. (1998). Shear stress and the endothelium. Kidney Int. Suppl. 54, S100–S108. doi:10.1046/j.1523-1755.1998.06720.x
Banerjee C., Chimowitz M. I. (2017). Stroke caused by atherosclerosis of the major intracranial arteries. Circ. Res. 120 (3), 502–513. doi:10.1161/CIRCRESAHA.116.308441
Barone Gibbs B., Kowalsky R. J., Perdomo S. J., Taormina J. M., Balzer J. R., Jakicic J. M. (2017). Effect of alternating standing and sitting on blood pressure and pulse wave velocity during a simulated workday in adults with overweight/obesity. J. Hypertens. 35 (12), 2411–2418. doi:10.1097/HJH.0000000000001463
Bauer M., Caviezel S., Teynor A., Erbel R., Mahabadi A. A., Schmidt-Trucksäss A. (2012). Carotid intima-media thickness as a biomarker of subclinical atherosclerosis. Swiss Med. Wkly. 142 (4344), w13705. doi:10.4414/smw.2012.13705
Baumbach G. L., Heistad D. D. (1989). Remodeling of cerebral arterioles in chronic hypertension. Hypertension 13 (6), 968–972. doi:10.1161/01.hyp.13.6.968
Bell J. W., Chen D., Bahls M., Newcomer S. C. (2011). Evidence for greater burden of peripheral arterial disease in lower extremity arteries of spinal cord-injured individuals. Am. J. Physiol. Heart Circ. Physiol. 301 (3), H766–H772. doi:10.1152/ajpheart.00507.2011
Bertoni A. G., Whitt-Glover M. C., Chung H., Le K. Y., Barr R. G., Mahesh M., et al. (2009). The association between physical activity and subclinical atherosclerosis: The multi-ethnic study of atherosclerosis. Am. J. Epidemiol. 169 (4), 444–454. doi:10.1093/aje/kwn350
Beunza J. J., Martínez-González M. Á., Ebrahim S., Bes-Rastrollo M., Núñez J., Martínez J. A., et al. (2007). Sedentary behaviors and the risk of incident hypertension: The SUN cohort. Am. J. Hypertens. 20 (11), 1156–1162. doi:10.1016/j.amjhyper.2007.06.007
Bhammar D. M., Sawyer B. J., Tucker W. J., Gaesser G. A. (2017). Breaks in sitting time: Effects on continuously monitored glucose and blood pressure. Med. Sci. Sports Exerc. 49 (10), 2119–2130. doi:10.1249/MSS.0000000000001315
Biensø R. S., Ringholm S., Kiilerich K., Aachmann-Andersen N.-J., Krogh-Madsen R., Guerra B., et al. (2012). GLUT4 and glycogen synthase are key players in bed rest–induced insulin resistance. Diabetes 61 (5), 1090–1099. doi:10.2337/db11-0884
Birk G. K., Dawson E. A., Atkinson C., Haynes A., Cable N. T., Thijssen D. H. J., et al. (2012). Brachial artery adaptation to lower limb exercise training: Role of shear stress. J. Appl. Physiol. 112 (10), 1653–1658. doi:10.1152/japplphysiol.01489.2011
Birk G. K., Dawson E. A., Cable N. T., Green D. J., Thijssen D. H. J. (2013). Effect of unilateral forearm inactivity on endothelium-dependent vasodilator function in humans. Eur. J. Appl. Physiol. 113 (4), 933–940. doi:10.1007/s00421-012-2505-7
Biswas A., Oh P. I., Faulkner G. E., Bajaj R. R., Silver M. A., Mitchell M. S., et al. (2015). Sedentary time and its association with risk for disease incidence, mortality, and hospitalization in adults: A systematic review and meta-analysis. Ann. Intern. Med. 162 (2), 123–132. doi:10.7326/M14-1651
Bjork L., Jenkins N. T., Witkowski S., Hagberg J. M. (2012). Nitro-oxidative stress biomarkers in active and inactive men. Int. J. Sports Med. 33 (04), 279–284. doi:10.1055/s-0032-1301891
Blann A. D., Seigneur M., Steiner M., Miller J. P., McCollum C. N. (1998). Circulating ICAM-1 and VCAM-1 in peripheral artery disease and hypercholesterolaemia: Relationship to the location of atherosclerotic disease, smoking, and in the prediction of adverse events. Thromb. Haemost. 79 (06), 1080–1085. doi:10.1055/s-0037-1615019
Bleeker M. W. P., De Groot P. C. E., Rongen G. A., Rittweger J., Felsenberg D., Smits P., et al. (2005). Vascular adaptation to deconditioning and the effect of an exercise countermeasure: Results of the berlin bed rest study. J. Appl. Physiol. 99 (4), 1293–1300. doi:10.1152/japplphysiol.00118.2005
Bourque S. L., Davidge S. T., Adams M. A. (2011). The interaction between endothelin-1 and nitric oxide in the vasculature: New perspectives. Am. J. Physiol. Regul. Integr. Comp. Physiol. 300 (6), R1288–R1295. doi:10.1152/ajpregu.00397.2010
Bowden Davies K. A., Norman J. A., Thompson A., Mitchell K. L., Harrold J. A., Halford J. C. G., et al. (2021). Short-term physical inactivity induces endothelial dysfunction. Front. Physiol. 12, 659834. doi:10.3389/fphys.2021.659834
Boyle L. J., Credeur D. P., Jenkins N. T., Padilla J., Leidy H. J., Thyfault J. P., et al. (2013). Impact of reduced daily physical activity on conduit artery flow-mediated dilation and circulating endothelial microparticles. J. Appl. Physiol. 115 (10), 1519–1525. doi:10.1152/japplphysiol.00837.2013
Breen L., Stokes K. A., Churchward-Venne T. A., Moore D. R., Baker S. K., Smith K., et al. (2013). Two weeks of reduced activity decreases leg lean mass and induces “anabolic resistance” of myofibrillar protein synthesis in healthy elderly. J. Clin. Endocrinol. Metab. 98 (6), 2604–2612. doi:10.1210/jc.2013-1502
British Heart F. (2017). Physical inactivity and sedentary behaviour report 2017. Birmingham, UK: British Heart Foundation Birmingham.
Brown I. A. M., Diederich L., Good M. E., DeLalio L. J., Murphy S. A., Cortese-Krott M. M., et al. (2018). Vascular smooth muscle remodeling in conductive and resistance arteries in hypertension. Arterioscler. Thromb. Vasc. Biol. 38 (9), 1969–1985. doi:10.1161/ATVBAHA.118.311229
Bull F. C., Al-Ansari S. S., Biddle S., Borodulin K., Buman M. P., Cardon G., et al. (2020). World Health Organization 2020 guidelines on physical activity and sedentary behaviour. Br. J. Sports Med. 54 (24), 1451–1462. doi:10.1136/bjsports-2020-102955
Cai H. (2005). Hydrogen peroxide regulation of endothelial function: Origins, mechanisms, and consequences. Cardiovasc. Res. 68 (1), 26–36. doi:10.1016/j.cardiores.2005.06.021
Caldwell A. R., Jansen L. T., Rosa-Caldwell M. E., Howie E. K., Gallagher K. M., Turner R. C., et al. (2020). Combined effects of standing and passive heating on attenuating sitting-induced vascular dysfunction. bioRxiv.
Carmeli E., Bachar A., Rom O., Aizenbud D. (2015). “Oxidative stress and nitric oxide in sedentary older adults with intellectual and developmental disabilities,” in Pathophysiology of respiration (Berlin, Germany: Springer), 21–27.
Carmeliet P., Lampugnani M.-G., Moons L., Breviario F., Compernolle V., Bono F., et al. (1999). Targeted deficiency or cytosolic truncation of the VE-cadherin gene in mice impairs VEGF-mediated endothelial survival and angiogenesis. Cell. 98 (2), 147–157. doi:10.1016/s0092-8674(00)81010-7
Carter S. E., Draijer R., Holder S. M., Brown L., Thijssen D. H. J., Hopkins N. D. (2019). Effect of different walking break strategies on superficial femoral artery endothelial function. Physiol. Rep. 7 (16), e14190. doi:10.14814/phy2.14190
Carter S. E., Draijer R., Holder S. M., Brown L., Thijssen D. H. J., Hopkins N. D. (2018). Regular walking breaks prevent the decline in cerebral blood flow associated with prolonged sitting. J. Appl. Physiol. 125 (3), 790–798. doi:10.1152/japplphysiol.00310.2018
Carter S. E., Draijer R., Stewart C. E., Moss A. D., Thijssen D. H. J., Hopkins N. D. (2021). Are acute sitting-induced changes in inflammation and cerebrovascular function related to impaired mood and cognition? Sport Sci. Health 17, 753–762. doi:10.1007/s11332-021-00753-8
Carter S., Hartman Y., Holder S., Thijssen D. H., Hopkins N. D. (2017). Sedentary behavior and cardiovascular disease risk: Mediating mechanisms. Exerc. Sport Sci. Rev. 45 (2), 80–86. doi:10.1249/JES.0000000000000106
Celermajer D. S., Sorensen K. E., Gooch V. M., Spiegelhalter D. J., Miller O. I., Sullivan I. D., et al. (1992). Non-invasive detection of endothelial dysfunction in children and adults at risk of atherosclerosis. Lancet 340 (8828), 1111–1115. doi:10.1016/0140-6736(92)93147-f
Chaddock L., Pontifex M. B., Hillman C. H., Kramer A. F. (2011). A review of the relation of aerobic fitness and physical activity to brain structure and function in children. J. Int. Neuropsychol. Soc. 17 (6), 975–985. doi:10.1017/S1355617711000567
Champion R. B., Smith L. R., Smith J., Hirlav B., Maylor B. D., White S. L., et al. (2018). Reducing prolonged sedentary time using a treadmill desk acutely improves cardiometabolic risk markers in male and female adults. J. Sports Sci. 36 (21), 2484–2491. doi:10.1080/02640414.2018.1464744
Cheng C., Tempel D., Van Haperen R., Van Der Baan A., Grosveld F., Daemen M. J., et al. (2006). Atherosclerotic lesion size and vulnerability are determined by patterns of fluid shear stress. Circulation 113 (23), 2744–2753. doi:10.1161/CIRCULATIONAHA.105.590018
Chien S. (1970). Shear dependence of effective cell volume as a determinant of blood viscosity. Science 168 (3934), 977–979. doi:10.1126/science.168.3934.977
Chiu J.-J., Chien S. (2011). Effects of disturbed flow on vascular endothelium: Pathophysiological basis and clinical perspectives. Physiol. Rev. 91 (1), 327–387. doi:10.1152/physrev.00047.2009
Cho M. J., Kanokwan B., Jeong K. H., Sun Y. E., Young J. S. (2020). The acute effects of interrupting prolonged sitting with stair climbing on vascular and metabolic function after a high-fat meal. Eur. J. Appl. Physiol. 120 (4), 829–839. doi:10.1007/s00421-020-04321-9
Chobanian A. V., Bakris G. L., Black H. R., Cushman W. C., Green L. A., Izzo J. L., et al. (2003). The seventh report of the joint national committee on prevention, detection, evaluation, and treatment of high blood pressure: The JNC 7 report. Jama 289 (19), 2560–2572. doi:10.1001/jama.289.19.2560
Church T. S., Thomas D. M., Tudor-Locke C., Katzmarzyk P. T., Earnest C. P., Rodarte R. Q., et al. (2011). Trends over 5 decades in US occupation-related physical activity and their associations with obesity. PloS one 6 (5), e19657. doi:10.1371/journal.pone.0019657
Clemes S. A., Patel R., Mahon C., Griffiths P. L. (2014). Sitting time and step counts in office workers. Occup. Med. 64 (3), 188–192. doi:10.1093/occmed/kqt164
Climie R. E., Wheeler M. J., Grace M., Lambert E. A., Cohen N., Owen N., et al. (2018). Simple intermittent resistance activity mitigates the detrimental effect of prolonged unbroken sitting on arterial function in overweight and obese adults. J. Appl. Physiol. (1985). 125 (6), 1787–1794. doi:10.1152/japplphysiol.00544.2018
Cohen J. N., Slysz J. T., King T. J., Coates A. M., King R. T., Burr J. F. (2021). Blood flow restriction in the presence or absence of muscle contractions does not preserve vasculature structure and function following 14–days of limb immobilization. Eur. J. Appl. Physiol. 121, 2437–2447. doi:10.1007/s00421-021-04715-3
Coker R. H., Hays N. P., Williams R. H., Wolfe R. R., Evans W. J. (2015). Bed rest promotes reductions in walking speed, functional parameters, and aerobic fitness in older, healthy adults. J. Gerontol. A Biol. Sci. Med. Sci. 70 (1), 91–96. doi:10.1093/gerona/glu123
Cooper A. R., Sebire S., Montgomery A. A., Peters T. J., Sharp D. J., Jackson N., et al. (2012). Sedentary time, breaks in sedentary time and metabolic variables in people with newly diagnosed type 2 diabetes. Diabetologia 55 (3), 589–599. doi:10.1007/s00125-011-2408-x
Credeur D. P., Miller S. M., Jones R., Stoner L., Dolbow D. R., Fryer S. M., et al. (2019). Impact of prolonged sitting on peripheral and central vascular health. Am. J. Cardiol. 123 (2), 260–266. doi:10.1016/j.amjcard.2018.10.014
Cruz-Jentoft A. J., Sayer A. A. (2019). Sarcopenia. Lancet 393 (10191), 2636–2646. doi:10.1016/S0140-6736(19)31138-9
Dalla Libera L., Ravara B., Gobbo V., Tarricone E., Vitadello M., Biolo G., et al. (2009). A transient antioxidant stress response accompanies the onset of disuse atrophy in human skeletal muscle. J. Appl. Physiol. 107 (2), 549–557. doi:10.1152/japplphysiol.00280.2009
Darwish A., Lui F. (2020). “Physiology, colloid osmotic pressure,” in StatPearls [internet] (Treasure Island, FL: StatPearls Publishing).
Dashwood M. R., Tsui J. C. S. (2002). Endothelin-1 and atherosclerosis: Potential complications associated with endothelin-receptor blockade. Atherosclerosis 160 (2), 297–304. doi:10.1016/s0021-9150(01)00586-x
Davies K. A. B., Sprung V. S., Norman J. A., Thompson A., Mitchell K. L., Halford J. C. G., et al. (2018). Short-term decreased physical activity with increased sedentary behaviour causes metabolic derangements and altered body composition: Effects in individuals with and without a first-degree relative with type 2 diabetes. Diabetologia 61 (6), 1282–1294. doi:10.1007/s00125-018-4603-5
Davies P. F. (2009). Hemodynamic shear stress and the endothelium in cardiovascular pathophysiology. Nat. Clin. Pract. Cardiovasc. Med. 6 (1), 16–26. doi:10.1038/ncpcardio1397
Davis M. G., Fox K. R., Hillsdon M., Sharp D. J., Coulson J. C., Thompson J. L. (2011). Objectively measured physical activity in a diverse sample of older urban UK adults. Med. Sci. Sports Exerc. 43 (4), 647–654. doi:10.1249/MSS.0b013e3181f36196
de Groot E., Hovingh G. K., Wiegman A., Duriez P., Smit A. J., Fruchart J.-C., et al. (2004). Measurement of arterial wall thickness as a surrogate marker for atherosclerosis. Circulation 109 (23), III33–III38. doi:10.1161/01.CIR.0000131516.65699.ba
Decker K. P., Feliciano P. G., Kimmel M. T., Hogwood A. C., Weggen J. B., Darling A. M., et al. (2021). Examining sex differences in sitting-induced microvascular dysfunction: Insight from acute vitamin C supplementation. Microvasc. Res. 135, 104147. doi:10.1016/j.mvr.2021.104147
d’El-Rei J., Cunha A. R., Trindade M., Neves M. F. (2016). Beneficial effects of dietary nitrate on endothelial function and blood pressure levels. Int. J. Hypertens. 2016, 6791519. doi:10.1155/2016/6791519
Delaney J. A. C., Jensky N. E., Criqui M. H., Whitt-Glover M. C., Lima J. A. C., Allison M. A. (2013). The association between physical activity and both incident coronary artery calcification and ankle brachial index progression: The multi-ethnic study of atherosclerosis. Atherosclerosis 230 (2), 278–283. doi:10.1016/j.atherosclerosis.2013.07.045
Demiot C., Dignat-George F., Fortrat J.-O., Sabatier F., Gharib C., Larina I., et al. (2007). WISE 2005: Chronic bed rest impairs microcirculatory endothelium in women. Am. J. Physiol. Heart Circ. Physiol. 293 (5), H3159–H3164. doi:10.1152/ajpheart.00591.2007
Dempsey P. C., Larsen R. N., Dunstan D. W., Owen N., Kingwell B. A. (2018). Sitting less and moving more: Implications for hypertension. Hypertension 72 (5), 1037–1046. doi:10.1161/HYPERTENSIONAHA.118.11190
Dempsey P. C., Larsen R. N., Sethi P., Sacre J. W., Straznicky N. E., Cohen N. D., et al. (2016a). Benefits for type 2 diabetes of interrupting prolonged sitting with brief bouts of light walking or simple resistance activities. Diabetes care 39 (6), 964–972. doi:10.2337/dc15-2336
Dempsey P. C., Sacre J. W., Larsen R. N., Straznicky N. E., Sethi P., Cohen N. D., et al. (2016b). Interrupting prolonged sitting with brief bouts of light walking or simple resistance activities reduces resting blood pressure and plasma noradrenaline in type 2 diabetes. J. Hypertens. 34 (12), 2376–2382. doi:10.1097/HJH.0000000000001101
Deng F., Wang S., Zhang L. (2016). Endothelial microparticles act as novel diagnostic and therapeutic biomarkers of diabetes and its complications: A literature review. Biomed. Res. Int. 2016, 9802026. doi:10.1155/2016/9802026
DeSouza C. A., Dengel D. R., Macko R. F., Cox K., Seals D. R. (1997). Elevated levels of circulating cell adhesion molecules in uncomplicated essential hypertension. Am. J. Hypertens. 10 (12), 1335–1341. doi:10.1016/s0895-7061(97)00268-9
Devaraj S., Kumaresan P. R., Jialal I. (2011). C-Reactive protein induces release of both endothelial microparticles and circulating endothelial cells in vitro and in vivo: Further evidence of endothelial dysfunction. Clin. Chem. 57 (12), 1757–1761. doi:10.1373/clinchem.2011.169839
Diaz K. M., Booth J. N., Seals S. R., Hooker S. P., Sims M., Dubbert P. M., et al. (2016). Sedentary behavior and subclinical atherosclerosis in african Americans: Cross-sectional analysis of the jackson heart study. Int. J. Behav. Nutr. Phys. Act. 13 (1), 31–39. doi:10.1186/s12966-016-0349-y
Dillon E. L., Casperson S. L., Durham W. J., Randolph K. M., Urban R. J., Volpi E., et al. (2011). Muscle protein metabolism responds similarly to exogenous amino acids in healthy younger and older adults during NO-induced hyperemia. Am. J. Physiol. Regul. Integr. Comp. Physiol. 301 (5), R1408–R1417. doi:10.1152/ajpregu.00211.2011
Dirks M. L., Wall B. T., Nilwik R., Weerts D. H. J. M., Verdijk L. B., van Loon L. J. C. (2014). Skeletal muscle disuse atrophy is not attenuated by dietary protein supplementation in healthy older men. J. Nutr. 144 (8), 1196–1203. doi:10.3945/jn.114.194217
Dirks M. L., Wall B. T., van de Valk B., Holloway T. M., Holloway G. P., Chabowski A., et al. (2016). One week of bed rest leads to substantial muscle atrophy and induces whole-body insulin resistance in the absence of skeletal muscle lipid accumulation. Diabetes 65 (10), 2862–2875. doi:10.2337/db15-1661
Dogra S., Wolf M., Jeffrey M. P., Foley R. C. A., Logan-Sprenger H., Jones-Taggart H., et al. (2019). Disrupting prolonged sitting reduces IL-8 and lower leg swell in active young adults. BMC Sports Sci. Med. Rehabil. 11 (1), 23–27. doi:10.1186/s13102-019-0138-4
Dormandy J. A. (1970). Clinical significance of blood viscosity. Ann. R. Coll. Surg. Engl. 47 (4), 211–228.
du Plooy C. S., Mels C. M. C., Huisman H. W., Kruger R. (2015). The association of endothelin-1 with markers of arterial stiffness in black South African women: The SABPA study. J. Amino Acids 2015, 481517. doi:10.1155/2015/481517
Dumith S. C., Hallal P. C., Reis R. S., Kohl H. W. (2011). Worldwide prevalence of physical inactivity and its association with human development index in 76 countries. Prev. Med. 53 (1-2), 24–28. doi:10.1016/j.ypmed.2011.02.017
Dunstan D. W., Kingwell B. A., Larsen R., Healy G. N., Cerin E., Hamilton M. T., et al. (2012). Breaking up prolonged sitting reduces postprandial glucose and insulin responses. Diabetes care 35 (5), 976–983. doi:10.2337/dc11-1931
Duvivier B. M. F. M., Bolijn J. E., Koster A., Schalkwijk C. G., Savelberg H. H. C. M., Schaper N. C. (2018). Reducing sitting time versus adding exercise: Differential effects on biomarkers of endothelial dysfunction and metabolic risk. Sci. Rep. 8 (1), 8657–7. doi:10.1038/s41598-018-26616-w
Ekelund U., Steene-Johannessen J., Brown W. J., Fagerland M. W., Owen N., Powell K. E., et al. (2016). Does physical activity attenuate, or even eliminate, the detrimental association of sitting time with mortality? A harmonised meta-analysis of data from more than 1 million men and women. Lancet 388 (10051), 1302–1310. doi:10.1016/S0140-6736(16)30370-1
Ekelund U., Tarp J., Steene-Johannessen J., Hansen B. H., Jefferis B., Fagerland M. W., et al. (2019). Dose-response associations between accelerometry measured physical activity and sedentary time and all cause mortality: Systematic review and harmonised meta-analysis. BMJ 366, l4570. doi:10.1136/bmj.l4570
El Sayed S. A., Mukherjee S. (2017). “Physiology, pancreas,” in StatPearls [internet] (Treasure Island, FL: StatPearls Publishing).
Esteve E., Castro A., López-Bermejo A., Vendrell J., Ricart W., Fernández-Real J.-M. (2007). Serum interleukin-6 correlates with endothelial dysfunction in healthy men independently of insulin sensitivity. Diabetes Care 30 (4), 939–945. doi:10.2337/dc06-1793
Evans W. S., Stoner L., Willey Q., Kelsch E., Credeur D. P., Hanson E. D. (2019). Local exercise does not prevent the aortic stiffening response to acute prolonged sitting: A randomized crossover trial. J. Appl. Physiol. 127 (3), 781–787. doi:10.1152/japplphysiol.00318.2019
Evju Ø., Mardal K.-A. (2015). “On the assumption of laminar flow in physiological flows: Cerebral aneurysms as an illustrative example,” in Modeling the heart and the circulatory system (Berlin, Germany: Springer), 177–195.
Falck R. S., Davis J. C., Liu-Ambrose T. (2017). What is the association between sedentary behaviour and cognitive function? A systematic review. Br. J. Sports Med. 51 (10), 800–811. doi:10.1136/bjsports-2015-095551
Falconer C. L., Cooper A. R., Walhin J. P., Thompson D., Page A. S., Peters T. J., et al. (2014). Sedentary time and markers of inflammation in people with newly diagnosed type 2 diabetes. Nutr. Metab. Cardiovasc. Dis. 24 (9), 956–962. doi:10.1016/j.numecd.2014.03.009
Farah B. Q., Ritti-Dias R. M., Montgomery P. S., Casanegra A. I., Silva-Palacios F., Gardner A. W. (2016). Sedentary behavior is associated with impaired biomarkers in claudicants. J. Vasc. Surg. 63 (3), 657–663. doi:10.1016/j.jvs.2015.09.018
Fernandes D. C., Araujo T. L. S., Laurindo F. R. M., Tanaka L. Y. (2018). Hemodynamic forces in the endothelium: From mechanotransduction to implications on development of atherosclerosis. Endothelium and cardiovascular diseases. Amsterdam, Netherlands: Elsevier, 85–95.
Fisher A., Ucci M., Smith L., Sawyer A., Spinney R., Konstantatou M., et al. (2018). Associations between the objectively measured office environment and workplace step count and sitting time: Cross-sectional analyses from the active buildings study. Int. J. Environ. Res. Public Health 15 (6), 1135. doi:10.3390/ijerph15061135
Fletcher G. F., Balady G., Blair S. N., Blumenthal J., Caspersen C., Chaitman B., et al. (1996). Statement on exercise: Benefits and recommendations for physical activity programs for all Americans: A statement for health professionals by the committee on exercise and cardiac rehabilitation of the council on clinical cardiology, American heart association. Circulation 94 (4), 857–862. doi:10.1161/01.cir.94.4.857
França C. N., Izar M. C. d. O., Amaral J. B. d., Tegani D. M., Fonseca F. A. H. (2015). Microparticles as potential biomarkers of cardiovascular disease. Arq. Bras. Cardiol. 104 (2), 169–174. doi:10.5935/abc.20140210
Freeman R. (2006). Assessment of cardiovascular autonomic function. Clin. Neurophysiol. 117 (4), 716–730. doi:10.1016/j.clinph.2005.09.027
Fuster J. J., Fernández P., González-Navarro H., Silvestre C., Nabah Y. N. A., Andrés V. (2010). Control of cell proliferation in atherosclerosis: Insights from animal models and human studies. Cardiovasc. Res. 86 (2), 254–264. doi:10.1093/cvr/cvp363
Fytanidis D. K., Soulis J. V., Giannoglou G. D. (2014). Patient-specific arterial system flow oscillation. Hippokratia 18 (2), 162–165.
Gabel L., Ridgers N. D., Della Gatta P. A., Arundell L., Cerin E., Robinson S., et al. (2016). Associations of sedentary time patterns and TV viewing time with inflammatory and endothelial function biomarkers in children. Pediatr. Obes. 11 (3), 194–201. doi:10.1111/ijpo.12045
Galkina E., Ley K. (2009). Immune and inflammatory mechanisms of atherosclerosis (*). Annu. Rev. Immunol. 27, 165. doi:10.1146/annurev.immunol.021908.132620
Gambillara V., Chambaz C., Montorzi G., Roy S., Stergiopulos N., Silacci P. (2006). Plaque-prone hemodynamics impair endothelial function in pig carotid arteries. Am. J. Physiol. Heart Circ. Physiol. 290 (6), H2320–H2328. doi:10.1152/ajpheart.00486.2005
García-Hermoso A., Martínez-Vizcaíno V., Recio-Rodríguez J. I., Sánchez-López M., Gómez-Marcos M. Á., García-Ortiz L., et al. (2015). Sedentary behaviour patterns and carotid intima-media thickness in Spanish healthy adult population. Atherosclerosis 239 (2), 571–576. doi:10.1016/j.atherosclerosis.2015.02.028
Garten R. S., Hogwood A. C., Weggen J. B., Fralin R. C., LaRosa K., Lee D., et al. (2019a). Aerobic training status does not attenuate prolonged sitting-induced lower limb vascular dysfunction. Appl. Physiology, Nutr. Metabolism 44 (4), 425–433. doi:10.1139/apnm-2018-0420
Garten R. S., Scott M. C., Zúñiga T. M., Hogwood A. C., Fralin R. C., Weggen J. (2019b). A prior high-intensity exercise bout attenuates the vascular dysfunction resulting from a prolonged sedentary bout. J. Phys. Act. Health 16 (10), 916–924. doi:10.1123/jpah.2018-0568
Gemignani T., Matos-Souza J. R., Franchini K. G., Nadruz W. (2012). Leg blood pressure measured in orthostatic posture is associated with left ventricular mass in normotensive subjects. Am. J. Hypertens. 25 (10), 1083–1087. doi:10.1038/ajh.2012.101
Gianoudis J., Bailey C. A., Daly R. M. (2015). Associations between sedentary behaviour and body composition, muscle function and sarcopenia in community-dwelling older adults. Osteoporos. Int. 26 (2), 571–579. doi:10.1007/s00198-014-2895-y
Gibson-Moore H. (2019). UK Chief Medical Officers’ physical activity guidelines 2019: What’s new and how can we get people more active? Nutr. Bull. 44 (4), 320–328. doi:10.1111/nbu.12409
Gifford J. R., Richardson R. S. (2017). CORP: Ultrasound assessment of vascular function with the passive leg movement technique. J. Appl. Physiol. 123 (6), 1708–1720. doi:10.1152/japplphysiol.00557.2017
Glover E. I., Phillips S. M., Oates B. R., Tang J. E., Tarnopolsky M. A., Selby A., et al. (2008). Immobilization induces anabolic resistance in human myofibrillar protein synthesis with low and high dose amino acid infusion. J. Physiol. 586 (24), 6049–6061. doi:10.1113/jphysiol.2008.160333
Glover E. I., Yasuda N., Tarnopolsky M. A., Abadi A., Phillips S. M. (2010). Little change in markers of protein breakdown and oxidative stress in humans in immobilization-induced skeletal muscle atrophy. Appl. Physiology, Nutr. Metabolism 35 (2), 125–133. doi:10.1139/H09-137
Gnasso A., Carallo C., Irace C., De Franceschi M. S., Mattioli P. L., Motti C., et al. (2001). Association between wall shear stress and flow-mediated vasodilation in healthy men. Atherosclerosis 156 (1), 171–176. doi:10.1016/s0021-9150(00)00617-1
Gnasso A., Irace C., Carallo C., De Franceschi M. S., Motti C., Mattioli P. L., et al. (1997). In vivo association between low wall shear stress and plaque in subjects with asymmetrical carotid atherosclerosis. Stroke 28 (5), 993–998. doi:10.1161/01.str.28.5.993
Gómez-Marcos M. Á., Recio-Rodríguez J. I., Patino-Alonso M. C., Agudo-Conde C., Gómez-Sánchez L., Rodríguez-Sánchez E., et al. (2011). Relationship between intima-media thickness of the common carotid artery and arterial stiffness in subjects with and without type 2 diabetes: A case-series report. Cardiovasc. Diabetol. 10 (1), 3–8. doi:10.1186/1475-2840-10-3
Gomez-Marcos M. A., Recio-Rodríguez J. I., Patino-Alonso M. C., Agudo-Conde C., Lasaosa-Medina L., Rodriguez-Sanchez E., et al. (2014). Relationship between objectively measured physical activity and vascular structure and function in adults. Atherosclerosis 234 (2), 366–372. doi:10.1016/j.atherosclerosis.2014.02.028
Goubergrits L., Kertzscher U., Schöneberg B., Wellnhofer E., Petz C., Hege H.-C. (2008). CFD analysis in an anatomically realistic coronary artery model based on non-invasive 3D imaging: Comparison of magnetic resonance imaging with computed tomography. Int. J. Cardiovasc. Imaging 24 (4), 411–421. doi:10.1007/s10554-007-9275-z
Gram M., Dahl R., Dela F. (2014). Physical inactivity and muscle oxidative capacity in humans. Eur. J. Sport Sci. 14 (4), 376–383. doi:10.1080/17461391.2013.823466
Gram M., Vigelsø A., Yokota T., Helge J. W., Dela F., Hey‐Mogensen M. (2015). Skeletal muscle mitochondrial H2O2 emission increases with immobilization and decreases after aerobic training in young and older men. J. Physiol. 593 (17), 4011–4027. doi:10.1113/JP270211
Graves L. E. F., Murphy R. C., Shepherd S. O., Cabot J., Hopkins N. D. (2015). Evaluation of sit-stand workstations in an office setting: A randomised controlled trial. BMC public health 15 (1), 1145. doi:10.1186/s12889-015-2469-8
Green D. J., Dawson E. A., Groenewoud H. M. M., Jones H., Thijssen D. H. J. (2014). Is flow-mediated dilation nitric oxide mediated? A meta-analysis. Hypertension 63 (2), 376–382. doi:10.1161/HYPERTENSIONAHA.113.02044
Green D. J., Hopman M. T. E., Padilla J., Laughlin M. H., Thijssen D. H. J. (2017). Vascular adaptation to exercise in humans: Role of hemodynamic stimuli. Physiol. Rev. 97 (2), 495–528. doi:10.1152/physrev.00014.2016
Gross M. D., Bielinski S. J., Suarez-Lopez J. R., Reiner A. P., Bailey K., Thyagarajan B., et al. (2012). Circulating soluble intercellular adhesion molecule 1 and subclinical atherosclerosis: The coronary artery risk development in young adults study. Clin. Chem. 58 (2), 411–420. doi:10.1373/clinchem.2011.168559
Guthold R., Stevens G. A., Riley L. M., Bull F. C. (2020). Global trends in insufficient physical activity among adolescents: A pooled analysis of 298 population-based surveys with 1· 6 million participants. Lancet. Child. Adolesc. Health 4 (1), 23–35. doi:10.1016/S2352-4642(19)30323-2
Guthold R., Stevens G. A., Riley L. M., Bull F. C. (2018). Worldwide trends in insufficient physical activity from 2001 to 2016: A pooled analysis of 358 population-based surveys with 1· 9 million participants. Lancet. Glob. Health 6 (10), e1077–e1086. doi:10.1016/S2214-109X(18)30357-7
Hackney K. J., Ploutz-Snyder L. L. (2012). Unilateral lower limb suspension: Integrative physiological knowledge from the past 20 years (1991–2011). Eur. J. Appl. Physiol. 112 (1), 9–22. doi:10.1007/s00421-011-1971-7
Hall J. E., Hall M. E. (2020). Guyton and Hall textbook of medical physiology e-Book. Amsterdam, Netherlands: Elsevier Health Sciences.
Hamburg N. M., McMackin C. J., Huang A. L., Shenouda S. M., Widlansky M. E., Schulz E., et al. (2007). Physical inactivity rapidly induces insulin resistance and microvascular dysfunction in healthy volunteers. Arterioscler. Thromb. Vasc. Biol. 27 (12), 2650–2656. doi:10.1161/ATVBAHA.107.153288
Hamer M., Chida Y. (2009). Physical activity and risk of neurodegenerative disease: A systematic review of prospective evidence. Psychol. Med. 39 (1), 3–11. doi:10.1017/S0033291708003681
Hargens A. R., Vico L. (2016). Long-duration bed rest as an analog to microgravity. J. Appl. Physiol. 120 (8), 891–903. doi:10.1152/japplphysiol.00935.2015
Harrington J., Peña A. S., Gent R., Hirte C., Couper J. (2010). Aortic intima media thickness is an early marker of atherosclerosis in children with type 1 diabetes mellitus. J. Pediatr. 156 (2), 237–241. doi:10.1016/j.jpeds.2009.08.036
Harris T. J., Owen C. G., Victor C. R., Adams R., Ekelund U. L. F., Cook D. G. (2009). A comparison of questionnaire, accelerometer, and pedometer: Measures in older people. Med. Sci. Sports Exerc. 41 (7), 1392–1402. doi:10.1249/MSS.0b013e31819b3533
Hartman Y. A. W., Tillmans L. C. M., Benschop D. L., Hermans A. N. L., Nijssen K. M. R., Eijsvogels T. M. H., et al. (2021). Long-term and acute benefits of reduced sitting on vascular flow and function. Med. Sci. Sports Exerc. 53, 341–350. doi:10.1249/MSS.0000000000002462
Harvey J. A., Chastin S. F. M., Skelton D. A. (2013). Prevalence of sedentary behavior in older adults: A systematic review. Int. J. Environ. Res. Public Health 10 (12), 6645–6661. doi:10.3390/ijerph10126645
Hauner H. (2002). Insulin resistance and the metabolic syndrome—A challenge of the new millennium. Eur. J. Clin. Nutr. 56 (1), S25–S29. doi:10.1038/sj.ejcn.1601350
Headid R. J., Pekas E. J., Wooden T. K., Son W.-M., Layec G., Shin J., et al. (2020). Impacts of prolonged sitting with mild hypercapnia on vascular and autonomic function in healthy recreationally active adults. Am. J. Physiol. Heart Circ. Physiol. 319 (2), H468–H480. doi:10.1152/ajpheart.00354.2020
Healy G. N., Matthews C. E., Dunstan D. W., Winkler E. A. H., Owen N. (2011). Sedentary time and cardio-metabolic biomarkers in US adults: NHANES 2003–06. Eur. Heart J. 32 (5), 590–597. doi:10.1093/eurheartj/ehq451
Hedge A., Ray E. J. (2004). Effects of an electronic height-adjustable worksurface on computer worker musculoskeletal discomfort and productivity. Los Angeles, CA: SAGE Publications Sage CA.
Hein T. W., Singh U., Vasquez-Vivar J., Devaraj S., Kuo L., Jialal I. (2009). Human C-reactive protein induces endothelial dysfunction and uncoupling of eNOS in vivo. Atherosclerosis 206 (1), 61–68. doi:10.1016/j.atherosclerosis.2009.02.002
Heinen Y., Stegemann E., Sansone R., Benedens K., Wagstaff R., Balzer J., et al. (2015). Local association between endothelial dysfunction and intimal hyperplasia: Relevance in peripheral artery disease. J. Am. Heart Assoc. 4 (2), e001472. doi:10.1161/JAHA.114.001472
Heiss C., Finis D., Kleinbongard P., Hoffmann A., Rassaf T., Kelm M., et al. (2007). Sustained increase in flow-mediated dilation after daily intake of high-flavanol cocoa drink over 1 week. J. Cardiovasc. Pharmacol. 49 (2), 74–80. doi:10.1097/FJC.0b013e31802d0001
Heiss C., Sansone R., Karimi H., Krabbe M., Schuler D., Rodriguez-Mateos A., et al. (2015). Impact of cocoa flavanol intake on age-dependent vascular stiffness in healthy men: A randomized, controlled, double-masked trial. Age 37 (3), 9794. doi:10.1007/s11357-015-9794-9
Hendrickse P., Degens H. (2019). The role of the microcirculation in muscle function and plasticity. J. Muscle Res. Cell. Motil. 40 (2), 127–140. doi:10.1007/s10974-019-09520-2
Henson J., Davies M. J., Bodicoat D. H., Edwardson C. L., Gill J. M. R., Stensel D. J., et al. (2016). Breaking up prolonged sitting with standing or walking attenuates the postprandial metabolic response in postmenopausal women: A randomized acute study. Diabetes care 39 (1), 130–138. doi:10.2337/dc15-1240
Henson J., Edwardson C. L., Bodicoat D. H., Bakrania K., Davies M. J., Khunti K., et al. (2018). Reallocating sitting time to standing or stepping through isotemporal analysis: Associations with markers of chronic low-grade inflammation. J. Sports Sci. 36 (14), 1586–1593. doi:10.1080/02640414.2017.1405709
Henson J., Yates T., Edwardson C. L., Khunti K., Talbot D., Gray L. J., et al. (2013). Sedentary time and markers of chronic low-grade inflammation in a high risk population. PloS one 8 (10), e78350. doi:10.1371/journal.pone.0078350
Heo K.-S., Fujiwara K., Abe J.-i. (2011). Disturbed-flow-mediated vascular reactive oxygen species induce endothelial dysfunction. Circ. J. 75 (12), 2722–2730. doi:10.1253/circj.cj-11-1124
Heo K.-S., Fujiwara K., Abe J.-i. (2014). Shear stress and atherosclerosis. Mol. Cells 37 (6), 435–440. doi:10.14348/molcells.2014.0078
Hitosugi M., Niwa M., Takatsu A. (2000). Rheologic changes in venous blood during prolonged sitting. Thromb. Res. 100 (5), 409–412. doi:10.1016/s0049-3848(00)00348-0
Hodgson J. M., Croft K. D. (2006). Dietary flavonoids: Effects on endothelial function and blood pressure. J. Sci. Food Agric. 86 (15), 2492–2498. doi:10.1002/jsfa.2675
Holder S. M., Dawson E. A., Brislane Á., Hisdal J., Green D. J., Thijssen D. H. J. (2019). Fluctuation in shear rate, with unaltered mean shear rate, improves brachial artery flow-mediated dilation in healthy, young men. J. Appl. Physiol. 126 (6), 1687–1693. doi:10.1152/japplphysiol.00009.2019
Horta B. L., Schaan B. D., Bielemann R. M., Vianna C. Á., Gigante D. P., Barros F. C., et al. (2015). Objectively measured physical activity and sedentary-time are associated with arterial stiffness in Brazilian young adults. Atherosclerosis 243 (1), 148–154. doi:10.1016/j.atherosclerosis.2015.09.005
Huai P., Xun H., Reilly K. H., Wang Y., Ma W., Xi B. (2013). Physical activity and risk of hypertension: A meta-analysis of prospective cohort studies. Hypertension 62 (6), 1021–1026. doi:10.1161/HYPERTENSIONAHA.113.01965
Huang A. L., Silver A. E., Shvenke E., Schopfer D. W., Jahangir E., Titas M. A., et al. (2007). Predictive value of reactive hyperemia for cardiovascular events in patients with peripheral arterial disease undergoing vascular surgery. Arterioscler. Thromb. Vasc. Biol. 27 (10), 2113–2119. doi:10.1161/ATVBAHA.107.147322
Huang Y., Wang S., Cai X., Mai W., Hu Y., Tang H., et al. (2013). Prehypertension and incidence of cardiovascular disease: A meta-analysis. BMC Med. 11 (1), 177–179. doi:10.1186/1741-7015-11-177
Huynh Q. L., Blizzard C. L., Sharman J. E., Magnussen C. G., Dwyer T., Venn A. J. (2014). The cross-sectional association of sitting time with carotid artery stiffness in young adults. BMJ open 4 (3), e004384. doi:10.1136/bmjopen-2013-004384
Hwang S.-J., Ballantyne C. M., Sharrett A. R., Smith L. C., Davis C. E., Gotto A. M., et al. (1997). Circulating adhesion molecules VCAM-1, ICAM-1, and E-selectin in carotid atherosclerosis and incident coronary heart disease cases: The atherosclerosis risk in communities (ARIC) study. Circulation 96 (12), 4219–4225. doi:10.1161/01.cir.96.12.4219
Hyldahl R. D., Hafen P. S., Nelson W. B., Ahmadi M., Pfeifer B., Mehling J., et al. (2021). Passive muscle heating attenuates the decline in vascular function caused by limb disuse. J. Physiol. 599 (20), 4581–4596. doi:10.1113/JP281900
Iadecola C., Davisson R. L. (2008). Hypertension and cerebrovascular dysfunction. Cell. Metab. 7 (6), 476–484. doi:10.1016/j.cmet.2008.03.010
Im I. J., Choi H. J., Jeong S. M., Kim H. J., Son J. S., Oh H. J. (2017). The association between muscle mass deficits and arterial stiffness in middle-aged men. Nutr. Metab. Cardiovasc. Dis. 27 (12), 1130–1135. doi:10.1016/j.numecd.2017.10.002
Inaba Y., Chen J. A., Bergmann S. R. (2010). Prediction of future cardiovascular outcomes by flow-mediated vasodilatation of brachial artery: A meta-analysis. Int. J. Cardiovasc. Imaging 26 (6), 631–640. doi:10.1007/s10554-010-9616-1
Intengan H. D., Schiffrin E. L. (2001). Vascular remodeling in hypertension: Roles of apoptosis, inflammation, and fibrosis. Hypertension 38 (3), 581–587. doi:10.1161/hy09t1.096249
Iwamoto A., Kajikawa M., Maruhashi T., Iwamoto Y., Oda N., Kishimoto S., et al. (2016). Vascular function and intima-media thickness of a leg artery in peripheral artery disease: A comparison of buerger disease and atherosclerotic peripheral artery disease. J. Atheroscler. Thromb. 23, 1261–1269. doi:10.5551/jat.35436
Janus A., Szahidewicz-Krupska E., Mazur G., Doroszko A. (2016). Insulin resistance and endothelial dysfunction constitute a common therapeutic target in cardiometabolic disorders. Mediat. Inflamm. 2016, 3634948. doi:10.1155/2016/3634948
Jasperse J. L., Woodman C. R., Price E. M., Hasser E. M., Laughlin M. H. (1999). Hindlimb unweighting decreases ecNOS gene expression and endothelium-dependent dilation in rat soleus feed arteries. J. Appl. Physiol. 87 (4), 1476–1482. doi:10.1152/jappl.1999.87.4.1476
Jayedi A., Rahimi K., Bautista L. E., Nazarzadeh M., Zargar M. S., Shab-Bidar S. (2019). Inflammation markers and risk of developing hypertension: A meta-analysis of cohort studies. Heart 105 (9), 686–692. doi:10.1136/heartjnl-2018-314216
Jenkins N. T., Padilla J., Boyle L. J., Credeur D. P., Laughlin M. H., Fadel P. J. (2013). Disturbed blood flow acutely induces activation and apoptosis of the human vascular endothelium. Hypertension 61 (3), 615–621. doi:10.1161/HYPERTENSIONAHA.111.00561
Jetté M., Sidney K., Blümchen G. (1990). Metabolic equivalents (METS) in exercise testing, exercise prescription, and evaluation of functional capacity. Clin. Cardiol. 13 (8), 555–565. doi:10.1002/clc.4960130809
Jones R. D., Morice A. H. (2000). Hydrogen peroxide—an intracellular signal in the pulmonary circulation: Involvement in hypoxic pulmonary vasoconstriction. Pharmacol. Ther. 88 (2), 153–161. doi:10.1016/s0163-7258(00)00089-9
Kamiya Y., Lai N. M. S., Schmid K. (2020). World population ageing 2020 highlights: Living arrangements of older persons.
Kang J. J., Treadwell T. A., Bodary P. F., Shayman J. A. (2019). Voluntary wheel running activates Akt/AMPK/eNOS signaling cascades without improving profound endothelial dysfunction in mice deficient in α-galactosidase A. Plos one 14 (5), e0217214. doi:10.1371/journal.pone.0217214
Kazi A., Duncan M., Clemes S., Haslam C. (2014). A survey of sitting time among UK employees. Occup. Med. 64 (7), 497–502. doi:10.1093/occmed/kqu099
Kim D.-O., Lee C. Y. (2004). Comprehensive study on vitamin C equivalent antioxidant capacity (VCEAC) of various polyphenolics in scavenging a free radical and its structural relationship. Crit. Rev. Food Sci. Nutr. 44 (4), 253–273. doi:10.1080/10408690490464960
Kim K. S., Kim S. J., Kim S., Choi D.-W., Ju Y. J., Park E.-C. (2018). Association of self-reported sedentary time with insulin resistance among Korean adults without diabetes mellitus: A cross-sectional study. BMC public health 18 (1), 1335–1338. doi:10.1186/s12889-018-6237-4
Kitahara A., Hamaoka T., Murase N., Homma T., Kurosawa Y., Ueda C., et al. (2003). Deterioration of muscle function after 21-day forearm immobilization. Med. Sci. Sports Exerc. 35 (10), 1697–1702. doi:10.1249/01.MSS.0000089339.07610.5F
Kivimäki M., Singh-Manoux A., Pentti J., Sabia S., Nyberg S. T., Alfredsson L., et al. (2019). Physical inactivity, cardiometabolic disease, and risk of dementia: An individual-participant meta-analysis. BMJ 365, l1495. doi:10.1136/bmj.l1495
Knudsen S. H., Hansen L. S., Pedersen M., Dejgaard T., Hansen J., Hall G. V., et al. (2012). Changes in insulin sensitivity precede changes in body composition during 14 days of step reduction combined with overfeeding in healthy young men. J. Appl. Physiol. 113 (1), 7–15. doi:10.1152/japplphysiol.00189.2011
Ko B.-J., Chang Y., Jung H.-S., Yun K. E., Kim C.-W., Park H. S., et al. (2016). Relationship between low relative muscle mass and coronary artery calcification in healthy adults. Arterioscler. Thromb. Vasc. Biol. 36 (5), 1016–1021. doi:10.1161/ATVBAHA.116.307156
Kondo H., Miura M., Itokawa Y. (1991). Oxidative stress in skeletal muscle atrophied by immobilization. Acta Physiol. Scand. 142 (4), 527–528. doi:10.1111/j.1748-1716.1991.tb09191.x
Kooijman M., Thijssen D. H. J., De Groot P. C. E., Bleeker M. W. P., Van Kuppevelt H. J. M., Green D. J., et al. (2008). Flow‐mediated dilatation in the superficial femoral artery is nitric oxide mediated in humans. J. Physiol. 586 (4), 1137–1145. doi:10.1113/jphysiol.2007.145722
Kortebein P., Symons T. B., Ferrando A., Paddon-Jones D., Ronsen O., Protas E., et al. (2008). Functional impact of 10 days of bed rest in healthy older adults. J. Gerontol. A Biol. Sci. Med. Sci. 63 (10), 1076–1081. doi:10.1093/gerona/63.10.1076
Kowalsky R. J., Jakicic J. M., Hergenroeder A., Rogers R. J., Gibbs B. B. (2019). Acute cardiometabolic effects of interrupting sitting with resistance exercise breaks. Appl. Physiology, Nutr. Metabolism 44 (10), 1025–1032. doi:10.1139/apnm-2018-0633
Koyama S., Sato E., Haniuda M., Numanami H., Nagai S., Izumi T. (2002). Decreased level of vascular endothelial growth factor in bronchoalveolar lavage fluid of normal smokers and patients with pulmonary fibrosis. Am. J. Respir. Crit. Care Med. 166 (3), 382–385. doi:10.1164/rccm.2103112
Kozàkovà M., Palombo C., Morizzo C., Nolan J. J., Konrad T., Balkau B., et al. (2010). Effect of sedentary behaviour and vigorous physical activity on segment-specific carotid wall thickness and its progression in a healthy population. Eur. Heart J. 31 (12), 1511–1519. doi:10.1093/eurheartj/ehq092
Krause N., Lynch J. W., Kaplan G. A., Cohen R. D., Salonen R., Salonen J. T. (2000). Standing at work and progression of carotid atherosclerosis. Scand. J. Work Environ. Health 26, 227–236. doi:10.5271/sjweh.536
Krijnen R. M. A., de Boer E. M., Adèr H. J., Bruynzeel D. P. (1997). Venous insufficiency in male workers with a standing profession. Part 1: Epidemiology. Dermatology 194 (2), 111–120. doi:10.1159/000246077
Kröger K., Kucharczik A., Hirche H., Rudofsky G. (1999). Atherosclerotic lesions are more frequent in femoral arteries than in carotid arteries independent of increasing number of risk factors. Angiology 50 (8), 649–654. doi:10.1177/000331979905000805
Krogh-Madsen R., Thyfault J. P., Broholm C., Mortensen O. H., Olsen R. H., Mounier R., et al. (2010). A 2-wk reduction of ambulatory activity attenuates peripheral insulin sensitivity. J. Appl. Physiol. 108 (5), 1034–1040. doi:10.1152/japplphysiol.00977.2009
Kruse N. T., Hughes W. E., Benzo R. M., Carr L. J., Casey D. P. (2018). Workplace strategies to prevent sitting-induced endothelial dysfunction. Med. Sci. Sports Exerc. 50 (4), 801–808. doi:10.1249/MSS.0000000000001484
Kulinski J. P., Sanghavi M., Ayers C. R., Das S. R., Banerjee S., Berry J. D., et al. (2015). Association between low ankle-brachial index and accelerometer-derived sedentary and exercise time in the asymptomatic general population. Vasc. Med. 20 (4), 332–338. doi:10.1177/1358863X15573837
Laclaustra M., Casasnovas J. A., Fernández-Ortiz A., Fuster V., León-Latre M., Jiménez-Borreguero L. J., et al. (2016). Femoral and carotid subclinical atherosclerosis association with risk factors and coronary calcium: The AWHS study. J. Am. Coll. Cardiol. 67 (11), 1263–1274. doi:10.1016/j.jacc.2015.12.056
Lahjibi E., Heude B., Dekker J. M., Højlund K., Laville M., Nolan J., et al. (2013). Impact of objectively measured sedentary behaviour on changes in insulin resistance and secretion over 3 years in the RISC study: Interaction with weight gain. Diabetes Metab. 39 (3), 217–225. doi:10.1016/j.diabet.2012.12.006
Lara J., Ashor A. W., Oggioni C., Ahluwalia A., Mathers J. C., Siervo M. (2016). Effects of inorganic nitrate and beetroot supplementation on endothelial function: A systematic review and meta-analysis. Eur. J. Nutr. 55 (2), 451–459. doi:10.1007/s00394-015-0872-7
Larsen R. N., Kingwell B. A., Robinson C., Hammond L., Cerin E., Shaw J. E., et al. (2015). Breaking up of prolonged sitting over three days sustains, but does not enhance, lowering of postprandial plasma glucose and insulin in overweight and obese adults. Clin. Sci. 129 (2), 117–127. doi:10.1042/CS20140790
Larsen R. N., Kingwell B. A., Sethi P., Cerin E., Owen N., Dunstan D. W. (2014). Breaking up prolonged sitting reduces resting blood pressure in overweight/obese adults. Nutr. Metab. Cardiovasc. Dis. 24 (9), 976–982. doi:10.1016/j.numecd.2014.04.011
Lauer T., Preik M., Rassaf T., Strauer B. E., Deussen A., Feelisch M., et al. (2001). Plasma nitrite rather than nitrate reflects regional endothelial nitric oxide synthase activity but lacks intrinsic vasodilator action. Proc. Natl. Acad. Sci. U. S. A. 98 (22), 12814–12819. doi:10.1073/pnas.221381098
Laufs U., Wassmann S., Czech T., Münzel T., Eisenhauer M., Böhm M., et al. (2005). Physical inactivity increases oxidative stress, endothelial dysfunction, and atherosclerosis. Arterioscler. Thromb. Vasc. Biol. 25 (4), 809–814. doi:10.1161/01.ATV.0000158311.24443.af
Laurin D., Verreault R., Lindsay J., MacPherson K., Rockwood K. (2001). Physical activity and risk of cognitive impairment and dementia in elderly persons. Arch. Neurol. 58 (3), 498–504. doi:10.1001/archneur.58.3.498
Lawler J. M., Song W., Demaree S. R. (2003). Hindlimb unloading increases oxidative stress and disrupts antioxidant capacity in skeletal muscle. Free Radic. Biol. Med. 35 (1), 9–16. doi:10.1016/s0891-5849(03)00186-2
Lazaros G., Oikonomou E., Vogiatzi G., Christoforatou E., Tsalamandris S., Goliopoulou A., et al. (2019). The impact of sedentary behavior patterns on carotid atherosclerotic burden: Implications from the corinthia epidemiological study. Atherosclerosis 282, 154–161. doi:10.1016/j.atherosclerosis.2019.01.026
LeBlanc A. D., Schneider V. S., Evans H. J., Pientok C., Rowe R., Spector E. (1992). Regional changes in muscle mass following 17 weeks of bed rest. J. Appl. Physiol. 73 (5), 2172–2178. doi:10.1152/jappl.1992.73.5.2172
LeBlanc A. G., Katzmarzyk P. T., Barreira T. V., Broyles S. T., Chaput J.-P., Church T. S., et al. (2015). Correlates of total sedentary time and screen time in 9–11 year-old children around the world: The international study of childhood obesity, lifestyle and the environment. PloS one 10 (6), e0129622. doi:10.1371/journal.pone.0129622
Lee P. H., Wong F. K. Y. (2015). The association between time spent in sedentary behaviors and blood pressure: A systematic review and meta-analysis. Sports Med. 45 (6), 867–880. doi:10.1007/s40279-015-0322-y
Lim M. S., Park B., Kong I. G., Sim S., Kim S. Y., Kim J.-H., et al. (2017). Leisure sedentary time is differentially associated with hypertension, diabetes mellitus, and hyperlipidemia depending on occupation. BMC Public Health 17 (1), 278–279. doi:10.1186/s12889-017-4192-0
Liu H., O’Brien M. W., Johns J. A., Kimmerly D. S. (2021). Does aerobic fitness impact prolonged sitting-induced popliteal artery endothelial dysfunction? Eur. J. Appl. Physiol. 121 (11), 3233–3241. doi:10.1007/s00421-021-04796-0
Liu L., Shi Y., Li T., Qin Q., Yin J., Pang S., et al. (2016). Leisure time physical activity and cancer risk: Evaluation of the WHO's recommendation based on 126 high-quality epidemiological studies. Br. J. Sports Med. 50 (6), 372–378. doi:10.1136/bjsports-2015-094728
Ludwig V. M., Bayley A., Cook D. G., Stahl D., Treasure J. L., Asthworth M., et al. (2018). Association between depressive symptoms and objectively measured daily step count in individuals at high risk of cardiovascular disease in south london, UK: A cross-sectional study. BMJ open 8 (4), e020942. doi:10.1136/bmjopen-2017-020942
Luo D., Cheng Y., Zhang H., Ba M., Chen P., Li H., et al. (2020). Association between high blood pressure and long term cardiovascular events in young adults: Systematic review and meta-analysis. BMJ 370, m3222. doi:10.1136/bmj.m3222
Maasakkers C. M., Melis R. J. F., Kessels R. P. C., Gardiner P. A., Rikkert M. G. M. O., Thijssen D. H. J., et al. (2020). The short-term effects of sedentary behaviour on cerebral hemodynamics and cognitive performance in older adults: A cross-over design on the potential impact of mental and/or physical activity. Alzheimers Res. Ther. 12 (1), 76–11. doi:10.1186/s13195-020-00644-z
Martinez-Gomez D., Eisenmann J. C., Healy G. N., Gomez-Martinez S., Diaz L. E., Dunstan D. W., et al. (2012). Sedentary behaviors and emerging cardiometabolic biomarkers in adolescents. J. Pediatr. 160 (1), 104–110. doi:10.1016/j.jpeds.2011.06.037
Mathiassen O. N., Buus N. H., Sihm I., Thybo N. K., Mørn B., Schroeder A. P., et al. (2007). Small artery structure is an independent predictor of cardiovascular events in essential hypertension. J. Hypertens. 25 (5), 1021–1026. doi:10.1097/HJH.0b013e32805bf8ed
Mattace-Raso F. U. S., van der Cammen T. J. M., Hofman A., van Popele N. M., Bos M. L., Schalekamp M. A. D. H., et al. (2006). Arterial stiffness and risk of coronary heart disease and stroke: The rotterdam study. Circulation 113 (5), 657–663. doi:10.1161/CIRCULATIONAHA.105.555235
Matthews C. E., Chen K. Y., Freedson P. S., Buchowski M. S., Beech B. M., Pate R. R., et al. (2008). Amount of time spent in sedentary behaviors in the United States, 2003–2004. Am. J. Epidemiol. 167 (7), 875–881. doi:10.1093/aje/kwm390
Maturana M. A., Irigoyen M. C., Spritzer P. M. (2007). Menopause, estrogens, and endothelial dysfunction: Current concepts. Clinics 62, 77–86. doi:10.1590/s1807-59322007000100012
McGlory C., von Allmen M. T., Stokes T., Morton R. W., Hector A. J., Lago B. A., et al. (2018). Failed recovery of glycemic control and myofibrillar protein synthesis with 2 wk of physical inactivity in overweight, prediabetic older adults. J. Gerontol. A Biol. Sci. Med. Sci. 73 (8), 1070–1077. doi:10.1093/gerona/glx203
McManus A. M., Ainslie P. N., Green D. J., Simair R. G., Smith K., Lewis N. (2015). Impact of prolonged sitting on vascular function in young girls. Exp. Physiol. 100 (11), 1379–1387. doi:10.1113/EP085355
Meirson T., Orion E., Di Mario C., Webb C., Patel N., Channon K. M., et al. (2015). Flow patterns in externally stented saphenous vein grafts and development of intimal hyperplasia. J. Thorac. Cardiovasc. Surg. 150 (4), 871–878. doi:10.1016/j.jtcvs.2015.04.061
Mendes M. d. A., Da Silva I., Ramires V., Reichert F., Martins R., Ferreira R., et al. (2018). Metabolic equivalent of task (METs) thresholds as an indicator of physical activity intensity. PLoS One 13 (7), e0200701. doi:10.1371/journal.pone.0200701
Metter E. J., Conwit R., Tobin J., Fozard J. L. (1997). Age-associated loss of power and strength in the upper extremities in women and men. J. Gerontol. A Biol. Sci. Med. Sci. 52 (5), B267–B276. doi:10.1093/gerona/52a.5.b267
Min K., Smuder A. J., Kwon O.-s., Kavazis A. N., Szeto H. H., Powers S. K. (2011). Mitochondrial-targeted antioxidants protect skeletal muscle against immobilization-induced muscle atrophy. J. Appl. Physiol. 111 (5), 1459–1466. doi:10.1152/japplphysiol.00591.2011
Mitchell G. F., Hwang S.-J., Vasan R. S., Larson M. G., Pencina M. J., Hamburg N. M., et al. (2010). Arterial stiffness and cardiovascular events: The framingham heart study. Circulation 121 (4), 505–511. doi:10.1161/CIRCULATIONAHA.109.886655
Miyashita M., Edamoto K., Kidokoro T., Yanaoka T., Kashiwabara K., Takahashi M., et al. (2016). Interrupting sitting time with regular walks attenuates postprandial triglycerides. Int. J. Sports Med. 37 (02), 97–103. doi:10.1055/s-0035-1559791
Mora S., Lee I. M., Buring J. E., Ridker P. M. (2006). Association of physical activity and body mass index with novel and traditional cardiovascular biomarkers in women. Jama 295 (12), 1412–1419. doi:10.1001/jama.295.12.1412
Morishima T., Restaino R. M., Walsh L. K., Kanaley J. A., Fadel P. J., Padilla J. (2016). Prolonged sitting-induced leg endothelial dysfunction is prevented by fidgeting. Am. J. Physiol. Heart Circ. Physiol. 311 (1), H177–H182. doi:10.1152/ajpheart.00297.2016
Morishima T., Restaino R. M., Walsh L. K., Kanaley J. A., Padilla J. (2017). Prior exercise and standing as strategies to circumvent sitting-induced leg endothelial dysfunction. Clin. Sci. 131 (11), 1045–1053. doi:10.1042/CS20170031
Morishima T., Tsuchiya Y., Padilla J., Ochi E. (2020a). Eight weeks of fish oil supplementation does not prevent sitting-induced leg endothelial dysfunction. Appl. Physiology, Nutr. Metabolism 45 (1), 55–60. doi:10.1139/apnm-2019-0138
Morishima T., Tsuchiya Y., Ueda H., Tsuji K., Ochi E. (2020b). Sitting-induced endothelial dysfunction is prevented in endurance-trained individuals. Med. Sci. Sports Exerc. 52 (8), 1770–1775. doi:10.1249/MSS.0000000000002302
Mudau M., Genis A., Lochner A., Strijdom H. (2012). Endothelial dysfunction: The early predictor of atherosclerosis. Cardiovasc. J. Afr. 23 (4), 222–231. doi:10.5830/CVJA-2011-068
Muller-Marschhausen K., Waschke J., Drenckhahn D. (2008). Physiological hydrostatic pressure protects endothelial monolayer integrity. Am. J. Physiol. Cell. Physiol. 294 (1), C324–C332. doi:10.1152/ajpcell.00319.2007
Mulvany M. J. (1999). Vascular remodelling of resistance vessels: Can we define this? Cardiovasc. Res. 41 (1), 9–13. doi:10.1016/s0008-6363(98)00289-2
Münzel T., Camici G. G., Maack C., Bonetti N. R., Fuster V., Kovacic J. C. (2017). Impact of oxidative stress on the heart and vasculature: Part 2 of a 3-part series. J. Am. Coll. Cardiol. 70 (2), 212–229. doi:10.1016/j.jacc.2017.05.035
Nation D. A. (2018). Blood pressure and cerebral blood flow in alzheimer disease. Hypertension 72, 68–69. doi:10.1161/HYPERTENSIONAHA.118.11019
Ng S. W., Popkin B. M. (2012). Time use and physical activity: A shift away from movement across the globe. Obes. Rev. 13 (8), 659–680. doi:10.1111/j.1467-789X.2011.00982.x
Ngomo S., Messing K., Perrault H., Comtois A. (2008). Orthostatic symptoms, blood pressure and working postures of factory and service workers over an observed workday. Appl. Ergon. 39 (6), 729–736. doi:10.1016/j.apergo.2007.11.004
Nigro P., Abe J.-i., Berk B. C. (2011). Flow shear stress and atherosclerosis: A matter of site specificity. Antioxid. Redox Signal. 15 (5), 1405–1414. doi:10.1089/ars.2010.3679
Nishiyama S. K., Zhao J., Wray D. W., Richardson R. S. (2017). Vascular function and endothelin-1: Tipping the balance between vasodilation and vasoconstriction. J. Appl. Physiol. 122 (2), 354–360. doi:10.1152/japplphysiol.00772.2016
Nosova E. V., Yen P., Chong K. C., Alley H. F., Stock E. O., Quinn A., et al. (2014). Short-term physical inactivity impairs vascular function. J. Surg. Res. 190 (2), 672–682. doi:10.1016/j.jss.2014.02.001
O’Brien M. W., Johns J. A., Al-Hinnawi A., Kimmerly D. S. (2020). Popliteal flow-mediated dilatory responses to an acute bout of prolonged sitting between earlier and later phases of natural menstrual and oral contraceptive pill cycles. J. Appl. Physiol. 129 (4), 637–645. doi:10.1152/japplphysiol.00424.2020
O’Brien M. W., Johns J. A., Williams T. D., Kimmerly D. S. (2019). Sex does not influence impairments in popliteal endothelial-dependent vasodilator or vasoconstrictor responses following prolonged sitting. J. Appl. Physiol. 127 (3), 679–687. doi:10.1152/japplphysiol.00887.2018
Ochi M., Kohara K., Tabara Y., Kido T., Uetani E., Ochi N., et al. (2010). Arterial stiffness is associated with low thigh muscle mass in middle-aged to elderly men. Atherosclerosis 212 (1), 327–332. doi:10.1016/j.atherosclerosis.2010.05.026
Oh Y. S. (2018). Arterial stiffness and hypertension. Clin. Hypertens. 24 (1), 17. doi:10.1186/s40885-018-0102-8
Ohashi T., Sugaya Y., Sakamoto N., Sato M. (2007). Hydrostatic pressure influences morphology and expression of VE-cadherin of vascular endothelial cells. J. Biomech. 40 (11), 2399–2405. doi:10.1016/j.jbiomech.2006.11.023
Owen C. A., Roberts M. (2007). Arterial vascular hemodynamics. J. Diagnostic Med. Sonogr. 23 (3), 129–140. doi:10.1177/8756479307302338
Paddon-Jones D., Sheffield-Moore M., Urban R. J., Sanford A. P., Aarsland A., Wolfe R. R., et al. (2004). Essential amino acid and carbohydrate supplementation ameliorates muscle protein loss in humans during 28 days bedrest. J. Clin. Endocrinol. Metab. 89 (9), 4351–4358. doi:10.1210/jc.2003-032159
Padilla J., Fadel P. J. (2017). Prolonged sitting leg vasculopathy: Contributing factors and clinical implications. Am. J. Physiol. Heart Circ. Physiol. 313 (4), H722–H728. doi:10.1152/ajpheart.00326.2017
Padilla J., Sheldon R. D., Sitar D. M., Newcomer S. C. (2009). Impact of acute exposure to increased hydrostatic pressure and reduced shear rate on conduit artery endothelial function: A limb-specific response. Am. J. Physiol. Heart Circ. Physiol. 297 (3), H1103–H1108. doi:10.1152/ajpheart.00167.2009
Paine N. J., Hinderliter A. L., Blumenthal J. A., Adams K. F., Sueta C. A., Chang P. P., et al. (2016). Reactive hyperemia is associated with adverse clinical outcomes in heart failure. Am. Heart J. 178, 108–114. doi:10.1016/j.ahj.2016.05.008
Palasubramaniam J., Wang X., Peter K. (2019). Myocardial infarction—from atherosclerosis to thrombosis: Uncovering new diagnostic and therapeutic approaches. Arterioscler. Thromb. Vasc. Biol. 39 (8), e176–e185. doi:10.1161/ATVBAHA.119.312578
Palombo C., Kozakova M. (2016). Arterial stiffness, atherosclerosis and cardiovascular risk: Pathophysiologic mechanisms and emerging clinical indications. Vasc. Pharmacol. 77, 1–7. doi:10.1016/j.vph.2015.11.083
Palta P., Sharrett A. R., Wei J., Meyer M. L., Kucharska-Newton A., Power M. C., et al. (2019). Central arterial stiffness is associated with structural brain damage and poorer cognitive performance: The ARIC study. J. Am. Heart Assoc. 8 (2), e011045. doi:10.1161/JAHA.118.011045
Pandiarajan M., Hargens A. R. (2020). Ground-based analogs for human spaceflight. Front. Physiol. 11, 716. doi:10.3389/fphys.2020.00716
Paniagua O. A., Bryant M. B., Panza J. A. (2001). Role of endothelial nitric oxide in shear stress–induced vasodilation of human microvasculature: Diminished activity in hypertensive and hypercholesterolemic patients. Circulation 103 (13), 1752–1758. doi:10.1161/01.cir.103.13.1752
Park J.-H., Hong J.-H., Lee S.-W., Ji H. D., Jung J.-A., Yoon K.-W., et al. (2019). The effect of chronic cerebral hypoperfusion on the pathology of alzheimer's disease: A positron emission tomography study in rats. Sci. Rep. 9 (1), 14102–14109. doi:10.1038/s41598-019-50681-4
Paszkowiak J. J., Dardik A. (2003). Arterial wall shear stress: Observations from the bench to the bedside. Vasc. Endovasc. Surg. 37 (1), 47–57. doi:10.1177/153857440303700107
Patterson R., McNamara E., Tainio M., de Sá T. H., Smith A. D., Sharp S. J., et al. (2018). Sedentary behaviour and risk of all-cause, cardiovascular and cancer mortality, and incident type 2 diabetes: A systematic review and dose response meta-analysis. Eur. J. Epidemiol. 33 (9), 811–829. doi:10.1007/s10654-018-0380-1
Paudel K. R., Panth N., Kim D.-W. (2016). Circulating endothelial microparticles: A key hallmark of atherosclerosis progression. Scientifica 2016, 8514056. doi:10.1155/2016/8514056
Peddie M. C., Bone J. L., Rehrer N. J., Skeaff C. M., Gray A. R., Perry T. L. (2013). Breaking prolonged sitting reduces postprandial glycemia in healthy, normal-weight adults: A randomized crossover trial. Am. J. Clin. Nutr. 98 (2), 358–366. doi:10.3945/ajcn.112.051763
Peddie M. C., Kessell C., Bergen T., Gibbons T. D., Campbell H. A., Cotter J. D., et al. (2021). The effects of prolonged sitting, prolonged standing, and activity breaks on vascular function, and postprandial glucose and insulin responses: A randomised crossover trial. Plos one 16 (1), e0244841. doi:10.1371/journal.pone.0244841
Perdomo S. J., Balzer J. R., Jakicic J. M., Kline C. E., Gibbs B. B. (2019). Acute effects of aerobic exercise duration on blood pressure, pulse wave velocity and cerebral blood flow velocity in middle-aged adults. Sport Sci. Health 15 (3), 647–658. doi:10.1007/s11332-019-00566-w
Philpott A., Anderson T. J. (2007). Reactive hyperemia and cardiovascular risk. Arterioscler. Thromb. Vasc. Biol. 27, 2065–2067. doi:10.1161/ATVBAHA.107.149740
Pizot C., Boniol M., Mullie P., Koechlin A., Boniol M., Boyle P., et al. (2016). Physical activity, hormone replacement therapy and breast cancer risk: A meta-analysis of prospective studies. Eur. J. Cancer 52, 138–154. doi:10.1016/j.ejca.2015.10.063
Pizzino G., Irrera N., Cucinotta M., Pallio G., Mannino F., Arcoraci V., et al. (2017). Oxidative stress: Harms and benefits for human health. Oxidative Med. Cell. Longev. 2017, 8416763. doi:10.1155/2017/8416763
Pollack A. A., Wood E. H. (1949). Venous pressure in the saphenous vein at the ankle in man during exercise and changes in posture. J. Appl. Physiol. 1 (9), 649–662. doi:10.1152/jappl.1949.1.9.649
Pronk N. P., Katz A. S., Lowry M., Payfer J. R. (2012). Peer reviewed: Reducing occupational sitting time and improving worker health: The take-a-stand project, 2011. Prev. chronic Dis. 9, E154. doi:10.5888/pcd9.110323
Prystopiuk V., Fels B., Simon C. S., Liashkovich I., Pasrednik D., Kronlage C., et al. (2018). A two-phase response of endothelial cells to hydrostatic pressure. J. Cell. Sci. 131 (12), jcs206920. doi:10.1242/jcs.206920
Pyke K. E., Tschakovsky M. E. (2005). The relationship between shear stress and flow‐mediated dilatation: Implications for the assessment of endothelial function. J. Physiol. 568 (2), 357–369. doi:10.1113/jphysiol.2005.089755
Rabby M. G., Sultana R., Shupti S. P., Molla M. M. (2014). Laminar blood flow through a model of arterial stenosis with oscillating wall. Inter. J. Fluid Mech. Res. 41 (5), 417–429. doi:10.1615/interjfluidmechres.v41.i5.30
Radegran G., Saltin B. (2000). Human femoral artery diameter in relation to knee extensor muscle mass, peak blood flow, and oxygen uptake. Am. J. Physiol. Heart Circ. Physiol. 278 (1), H162–H167. doi:10.1152/ajpheart.2000.278.1.H162
Rafieian-Kopaei M., Setorki M., Doudi M., Baradaran A., Nasri H. (2014). Atherosclerosis: Process, indicators, risk factors and new hopes. Int. J. Prev. Med. 5 (8), 927–946.
Rakobowchuk M., Crozier J., Glover E. I., Yasuda N., Phillips S. M., Tarnopolsky M. A., et al. (2011). Short-term unilateral leg immobilization alters peripheral but not central arterial structure and function in healthy young humans. Eur. J. Appl. Physiol. 111 (2), 203–210. doi:10.1007/s00421-010-1636-y
Rasinaho M., Hirvensalo M., Leinonen R., Lintunen T., Rantanen T. (2007). Motives for and barriers to physical activity among older adults with mobility limitations. J. Aging Phys. Act. 15 (1), 90–102. doi:10.1123/japa.15.1.90
Rasmussen B. B., Fujita S., Wolfe R. R., Mittendorfer B., Roy M., Rowe V. L., et al. (2006). Insulin resistance of muscle protein metabolism in aging. FASEB J. 20 (6), 768–769. doi:10.1096/fj.05-4607fje
Rees A., Dodd G. F., Spencer J. P. E. (2018). The effects of flavonoids on cardiovascular health: A review of human intervention trials and implications for cerebrovascular function. Nutrients 10 (12), 1852. doi:10.3390/nu10121852
Restaino R. M., Holwerda S. W., Credeur D. P., Fadel P. J., Padilla J. (2015). Impact of prolonged sitting on lower and upper limb micro‐and macrovascular dilator function. Exp. Physiol. 100 (7), 829–838. doi:10.1113/EP085238
Restaino R. M., Walsh L. K., Morishima T., Vranish J. R., Martinez-Lemus L. A., Fadel P. J., et al. (2016). Endothelial dysfunction following prolonged sitting is mediated by a reduction in shear stress. Am. J. Physiol. Heart Circ. Physiol. 310 (5), H648–H653. doi:10.1152/ajpheart.00943.2015
Reynolds L. J., Credeur D. P., Holwerda S. W., Leidy H. J., Fadel P. J., Thyfault J. P. (2015). Acute inactivity impairs glycemic control but not blood flow to glucose ingestion. Med. Sci. Sports Exerc. 47 (5), 1087–1094. doi:10.1249/MSS.0000000000000508
Rizzoni D., De Ciuceis C., Porteri E., Paiardi S., Boari G. E. M., Mortini P., et al. (2009). Altered structure of small cerebral arteries in patients with essential hypertension. J. Hypertens. 27 (4), 838–845. doi:10.1097/HJH.0b013e32832401ea
Rizzoni D., Porteri E., Boari G. E. M., De Ciuceis C., Sleiman I., Muiesan M. L., et al. (2003). Prognostic significance of small-artery structure in hypertension. Circulation 108 (18), 2230–2235. doi:10.1161/01.CIR.0000095031.51492.C5
Rizzoni D., Porteri E., Castellano M., Bettoni G., Muiesan M. L., Muiesan P., et al. (1996). Vascular hypertrophy and remodeling in secondary hypertension. Hypertension 28 (5), 785–790. doi:10.1161/01.hyp.28.5.785
Rodriguez-Mateos A., Rendeiro C., Bergillos-Meca T., Tabatabaee S., George T. W., Heiss C., et al. (2013). Intake and time dependence of blueberry flavonoid–induced improvements in vascular function: A randomized, controlled, double-blind, crossover intervention study with mechanistic insights into biological activity. Am. J. Clin. Nutr. 98 (5), 1179–1191. doi:10.3945/ajcn.113.066639
Roher A. E., Debbins J. P., Malek-Ahmadi M., Chen K., Pipe J. G., Maze S., et al. (2012). Cerebral blood flow in Alzheimer’s disease. Vasc. Health Risk Manag. 8, 599–611. doi:10.2147/VHRM.S34874
Rosenberry R., Nelson M. D. (2020). Reactive hyperemia: A review of methods, mechanisms, and considerations. Am. J. Physiol. Regul. Integr. Comp. Physiol. 318 (3), R605–R618. doi:10.1152/ajpregu.00339.2019
Rosiansky-Sultan M., Klipper E., Spanel-Borowski K., Meidan R. (2006). Inverse relationship between nitric oxide synthases and endothelin-1 synthesis in bovine corpus luteum: Interactions at the level of luteal endothelial cell. Endocrinology 147 (11), 5228–5235. doi:10.1210/en.2006-0795
Ross R. (1999). Atherosclerosis—An inflammatory disease. N. Engl. J. Med. 340 (2), 115–126. doi:10.1056/NEJM199901143400207
Safar M. E., Asmar R., Benetos A., Blacher J., Boutouyrie P., Lacolley P., et al. (2018). Interaction between hypertension and arterial stiffness: An expert reappraisal. Hypertension 72 (4), 796–805. doi:10.1161/HYPERTENSIONAHA.118.11212
Sagelv E. H., Ekelund U., Pedersen S., Brage S., Hansen B. H., Johansson J., et al. (2019). Physical activity levels in adults and elderly from triaxial and uniaxial accelerometry. The Tromsø Study. PloS one 14 (12), e0225670. doi:10.1371/journal.pone.0225670
Sanders D. B., Kelley T., Larson D. (2000). The role of nitric oxide synthase/nitric oxide in vascular smooth muscle control. Perfusion 15 (2), 97–104. doi:10.1177/026765910001500203
Sandoo A., van Zanten J. J. C. S. V., Metsios G. S., Carroll D., Kitas G. D. (2010). The endothelium and its role in regulating vascular tone. Open cardiovasc. Med. J. 4, 302–312. doi:10.2174/1874192401004010302
Santilli V., Bernetti A., Mangone M., Paoloni M. (2014). Clinical definition of sarcopenia. Clin. Cases Min. Bone Metab. 11 (3), 177–180.
Sardinha L. B., Andersen L. B., Anderssen S. A., Quitério A. L., Ornelas R., Froberg K., et al. (2008). Objectively measured time spent sedentary is associated with insulin resistance independent of overall and central body fat in 9-to 10-year-old Portuguese children. Diabetes care 31 (3), 569–575. doi:10.2337/dc07-1286
Savoia C., Sada L., Zezza L., Pucci L., Lauri F. M., Befani A., et al. (2011). Vascular inflammation and endothelial dysfunction in experimental hypertension. Int. J. Hypertens. 2011, 281240. doi:10.4061/2011/281240
Schiffrin E. L. (2001). Role of endothelin-1 in hypertension and vascular disease. Am. J. Hypertens. 14 (S3), 83S–89S. doi:10.1016/s0895-7061(01)02074-x
Schiffrin E. L. (2012). Vascular remodeling in hypertension: Mechanisms and treatment. Hypertension 59 (2), 367–374. doi:10.1161/HYPERTENSIONAHA.111.187021
Schroeter H., Heiss C., Balzer J., Kleinbongard P., Keen C. L., Hollenberg N. K., et al. (2006). (–)-Epicatechin mediates beneficial effects of flavanol-rich cocoa on vascular function in humans. Proc. Natl. Acad. Sci. U. S. A. 103 (4), 1024–1029. doi:10.1073/pnas.0510168103
Schwartz E. A., Bizios R., Medow M. S., Gerritsen M. E. (1999). Exposure of human vascular endothelial cells to sustained hydrostatic pressure stimulates proliferation: Involvement of the αv integrins. Circ. Res. 84 (3), 315–322. doi:10.1161/01.res.84.3.315
Semenkovich C. F. (2006). Insulin resistance and atherosclerosis. J. Clin. Investig. 116 (7), 1813–1822. doi:10.1172/JCI29024
Sheppard J. P., Albasri A., Franssen M., Fletcher B., Pealing L., Roberts N., et al. (2019). Defining the relationship between arm and leg blood pressure readings: A systematic review and meta-analysis. J. Hypertens. 37 (4), 660–670. doi:10.1097/HJH.0000000000001958
Shu J., Santulli G. (2018). Update on peripheral artery disease: Epidemiology and evidence-based facts. Atherosclerosis 275, 379–381. doi:10.1016/j.atherosclerosis.2018.05.033
Siddarth P., Burggren A. C., Eyre H. A., Small G. W., Merrill D. A. (2018). Sedentary behavior associated with reduced medial temporal lobe thickness in middle-aged and older adults. PloS one 13 (4), e0195549. doi:10.1371/journal.pone.0195549
Silber H. A., Bluemke D. A., Ouyang P., Du Y. P., Post W. S., Lima J. A. C. (2001). The relationship between vascular wall shear stress and flow-mediated dilation: Endothelial function assessed by phase-contrast magnetic resonance angiography. J. Am. Coll. Cardiol. 38 (7), 1859–1865. doi:10.1016/s0735-1097(01)01649-7
Skov K., Mulvany M. J., Korsgaard N. (1992). Morphology of renal afferent arterioles in spontaneously hypertensive rats. Hypertension 20 (6), 821–827. doi:10.1161/01.hyp.20.6.821
Smith L., Tully M., Jacob L., Blackburn N., Adlakha D., Caserotti P., et al. (2020). The association between sedentary behavior and sarcopenia among adults aged≥ 65 years in low-and middle-income countries. Int. J. Environ. Res. Public Health 17 (5), 1708. doi:10.3390/ijerph17051708
Smorawinski J., Kaciuba-Uscilko H., Nazar K., Kubala P., Kaminska E., Ziemba A. W., et al. (2000). Effects of three-day bed rest on metabolic, hormonal and circulatory responses to an oral glucose load in endurance or strength trained athletes and untrained subjects. J. Physiol. Pharmacol. 51 (2), 279–289.
Song W., Kwak H.-B., Kim J.-H., Lawler J. M. (2009). Exercise training modulates the nitric oxide synthase profile in skeletal muscle from old rats. J. Gerontol. A Biol. Sci. Med. Sci. 64 (5), 540–549. doi:10.1093/gerona/glp021
Spiteri K., Broom D., Bekhet A. H., de Caro J. X., Laventure B., Grafton K. (2019). Barriers and motivators of physical activity participation in middle-aged and older adults—A systematic review. J. Aging Phys. Act. 27 (6), 929–944. doi:10.1123/japa.2018-0343
Sriram K., Intaglietta M., Tartakovsky D. M. (2014). Non‐Newtonian flow of blood in arterioles: Consequences for wall shear stress measurements. Microcirculation 21 (7), 628–639. doi:10.1111/micc.12141
Sriram K., Laughlin J. G., Rangamani P., Tartakovsky D. M. (2016). Shear-induced nitric oxide production by endothelial cells. Biophys. J. 111 (1), 208–221. doi:10.1016/j.bpj.2016.05.034
Staiano A. E., Martin C. K., Champagne C. M., Rood J. C., Katzmarzyk P. T. (2018). Sedentary time, physical activity, and adiposity in a longitudinal cohort of nonobese young adults. Am. J. Clin. Nutr. 108 (5), 946–952. doi:10.1093/ajcn/nqy191
Stamatakis E., Gale J., Bauman A., Ekelund U., Hamer M., Ding D. (2019). Sitting time, physical activity, and risk of mortality in adults. J. Am. Coll. Cardiol. 73 (16), 2062–2072. doi:10.1016/j.jacc.2019.02.031
Stefanidis K. B., Askew C. D., Klein T., Lagopoulos J., Summers M. J. (2019). Healthy aging affects cerebrovascular reactivity and pressure-flow responses, but not neurovascular coupling: A cross-sectional study. PLoS One 14 (5), e0217082. doi:10.1371/journal.pone.0217082
Steinberg H. O., Chaker H., Leaming R., Johnson A., Brechtel G., Baron A. D. (1996). Obesity/insulin resistance is associated with endothelial dysfunction. Implications for the syndrome of insulin resistance. J. Clin. Investig. 97 (11), 2601–2610. doi:10.1172/JCI118709
Stephens B. R., Granados K., Zderic T. W., Hamilton M. T., Braun B. (2011). Effects of 1 day of inactivity on insulin action in healthy men and women: Interaction with energy intake. Metabolism. 60 (7), 941–949. doi:10.1016/j.metabol.2010.08.014
Stone K. J., Fryer S. M., Ryan T., Stoner L. (2016). The validity and reliability of continuous-wave near-infrared spectroscopy for the assessment of leg blood volume during an orthostatic challenge. Atherosclerosis 251, 234–239. doi:10.1016/j.atherosclerosis.2016.06.030
Stoner L., Willey Q., Evans W. S., Burnet K., Credeur D. P., Fryer S., et al. (2019). Effects of acute prolonged sitting on cerebral perfusion and executive function in young adults: A randomized cross‐over trial. Psychophysiology 56 (12), e13457. doi:10.1111/psyp.13457
Stuart C. A., Shangraw R. E., Prince M. J., Peters E. J., Wolfe R. R. (1988). Bed-rest-induced insulin resistance occurs primarily in muscle. Metabolism. 37 (8), 802–806. doi:10.1016/0026-0495(88)90018-2
Su T.-W., Chou T.-Y., Jou H.-J., Yang P.-Y., Lin C.-L., Sung F.-C., et al. (2015). Peripheral arterial disease and spinal cord injury: A retrospective nationwide cohort study. Medicine 94 (41), e1655. doi:10.1097/MD.0000000000001655
Suceava I., Lighezan D., Serban C., Lighezan R., Gurban C., Dragan S. (2013). The association between arterial stiffness and carotid intima-media thickness in patients with known cardiovascular risk factors. Clujul Med. 86 (3), 222–226.
Suetta C., Hvid L. G., Justesen L., Christensen U., Neergaard K., Simonsen L., et al. (2009). Effects of aging on human skeletal muscle after immobilization and retraining. J. Appl. Physiol. 107 (4), 1172–1180. doi:10.1152/japplphysiol.00290.2009
Sugawara J., Hayashi K., Kaneko F., Yamada H., Kizuka T., Tanaka H. (2004). Reductions in basal limb blood flow and lumen diameter after short-term leg casting. Med. Sci. Sports Exerc. 36 (10), 1689–1694. doi:10.1249/01.mss.0000142410.45142.28
Sugawara J., Komine H., Hayashi K., Yoshizawa M., Yokoi T., Otsuki T., et al. (2007). Effect of systemic nitric oxide synthase inhibition on arterial stiffness in humans. Hypertens. Res. 30 (5), 411–415. doi:10.1291/hypres.30.411
Suvorava T., Lauer N., Kojda G. (2004). Physical inactivity causes endothelial dysfunction in healthy young mice. J. Am. Coll. Cardiol. 44 (6), 1320–1327. doi:10.1016/j.jacc.2004.06.030
Suvorava T., Lauer N., Kumpf S., Jacob R., Meyer W., Kojda G. (2005). Endogenous vascular hydrogen peroxide regulates arteriolar tension in vivo. Circulation 112 (16), 2487–2495. doi:10.1161/CIRCULATIONAHA.105.543157
Tansey E. A., Montgomery L. E. A., Quinn J. G., Roe S. M., Johnson C. D. (2019). Understanding basic vein physiology and venous blood pressure through simple physical assessments. Adv. Physiol. Educ. 43 (3), 423–429. doi:10.1152/advan.00182.2018
Tarray R., Saleem S., Yousuf I., Gulnar A., Laway B., Verma S., et al. (2014). Role of insulin resistance in essential hypertension. Cardiovasc. Endocrinol. 3 (4), 129–133. doi:10.1097/xce.0000000000000032
Taylor F. C., Dunstan D. W., Fletcher E., Townsend M. K., Larsen R. N., Rickards K., et al. (2020). Interrupting prolonged sitting and endothelial function in polycystic ovary syndrome. Med. Sci. Sports Exerc. 10, 479–486. doi:10.1249/MSS.0000000000002513
Taylor F. C., Dunstan D. W., Homer A. R., Dempsey P. C., Kingwell B. A., Climie R. E., et al. (2021). Acute effects of interrupting prolonged sitting on vascular function in type 2 diabetes. Am. J. Physiol. Heart Circ. Physiol. 320 (1), H393–H403. doi:10.1152/ajpheart.00422.2020
Teixeira A. L., Padilla J., Vianna L. C. (2017). Impaired popliteal artery flow-mediated dilation caused by reduced daily physical activity is prevented by increased shear stress. J. Appl. Physiol. 123 (1), 49–54. doi:10.1152/japplphysiol.00001.2017
Teixeira B. C., Lopes A. L., Macedo R. C. O., Correa C. S., Ramis T. R., Ribeiro J. L., et al. (2014). Inflammatory markers, endothelial function and cardiovascular risk. J. Vasc. Bras. 13, 108–115. doi:10.1590/jvb.2014.054
Tesch P. A., Lundberg T. R., Fernandez-Gonzalo R. (2016). Unilateral lower limb suspension: From subject selection to “omic” responses. J. Appl. Physiol. 120 (10), 1207–1214. doi:10.1152/japplphysiol.01052.2015
Thachil E. J., Prasad I. R. V., Narsimulu G. (2011). Vasculopathies of peripheral arterial disease: Uncommon etiologies. J. Assoc. Physicians India 59, 215.
Thijssen D. H. J., Black M. A., Pyke K. E., Padilla J., Atkinson G., Harris R. A., et al. (2011). Assessment of flow-mediated dilation in humans: A methodological and physiological guideline. Am. J. Physiol. Heart Circ. Physiol. 300 (1), H2–H12. doi:10.1152/ajpheart.00471.2010
Thijssen D. H. J., Bruno R. M., van Mil A. C. C. M., Holder S. M., Faita F., Greyling A., et al. (2019). Expert consensus and evidence-based recommendations for the assessment of flow-mediated dilation in humans. Eur. Heart J. 40 (30), 2534–2547. doi:10.1093/eurheartj/ehz350
Thijssen D. H. J., Cable N. T., Green D. J. (2012). Impact of exercise training on arterial wall thickness in humans. Clin. Sci. 122 (7), 311–322. doi:10.1042/CS20110469
Thijssen D. H. J., Dawson E. A., Tinken T. M., Cable N. T., Green D. J. (2009). Retrograde flow and shear rate acutely impair endothelial function in humans. Hypertension 53 (6), 986–992. doi:10.1161/HYPERTENSIONAHA.109.131508
Thijssen D. H. J., Maiorana A. J., O’Driscoll G., Cable N. T., Hopman M. T. E., Green D. J. (2010). Impact of inactivity and exercise on the vasculature in humans. Eur. J. Appl. Physiol. 108 (5), 845–875. doi:10.1007/s00421-009-1260-x
Thijssen D. H. J., Schreuder T. H. A., Newcomer S. W., Laughlin M. H., Hopman M. T. E., Green D. J. (2015). Impact of 2-weeks continuous increase in retrograde shear stress on brachial artery vasomotor function in young and older men. J. Am. Heart Assoc. 4 (10), e001968. doi:10.1161/jaha.115.001968
Thosar S. S., Bielko S. L., Mather K. J., Johnston J. D., Wallace J. P. (2015a). Effect of prolonged sitting and breaks in sitting time on endothelial function. Med. Sci. Sports Exerc 47, 843. doi:10.1249/MSS.0000000000000479
Thosar S. S., Bielko S. L., Wiggins C. C., Wallace J. P. (2014). Differences in brachial and femoral artery responses to prolonged sitting. Cardiovasc. Ultrasound 12 (1), 50. doi:10.1186/1476-7120-12-50
Thosar S. S., Bielko S. L., Wiggins C. S., Klaunig J. E., Mather K. J., Wallace J. P. (2015b). Antioxidant vitamin C prevents decline in endothelial function during sitting. Med. Sci. Monit. 21, 1015–1021. doi:10.12659/MSM.893192
Thosar S. S., Johnson B. D., Johnston J. D., Wallace J. P. (2012). Sitting and endothelial dysfunction: The role of shear stress. Med. Sci. Monit. 18 (12), RA173–RA180. doi:10.12659/msm.883589
Timmerman K. L., Lee J. L., Dreyer H. C., Dhanani S., Glynn E. L., Fry C. S., et al. (2010). Insulin stimulates human skeletal muscle protein synthesis via an indirect mechanism involving endothelial-dependent vasodilation and mammalian target of rapamycin complex 1 signaling. J. Clin. Endocrinol. Metab. 95 (8), 3848–3857. doi:10.1210/jc.2009-2696
Tinken T. M., Thijssen D. H. J., Hopkins N., Black M. A., Dawson E. A., Minson C. T., et al. (2009). Impact of shear rate modulation on vascular function in humans. Hypertension 54 (2), 278–285. doi:10.1161/HYPERTENSIONAHA.109.134361
Tinken T. M., Thijssen D. H. J., Hopkins N., Dawson E. A., Cable N. T., Green D. J. (2010). Shear stress mediates endothelial adaptations to exercise training in humans. Hypertension 55 (2), 312–318. doi:10.1161/HYPERTENSIONAHA.109.146282
Tomei F., Baccolo T. P., Tomao E., Palmi S., Rosati M. V. (1999). Chronic venous disorders and occupation. Am. J. Ind. Med. 36 (6), 653–665. doi:10.1002/(sici)1097-0274(199912)36:6<653::aid-ajim8>3.0.co;2-p
Tomilovskaya E., Shigueva T., Sayenko D., Rukavishnikov I., Kozlovskaya I. (2019). Dry immersion as a ground-based model of microgravity physiological effects. Front. Physiol. 10, 284. doi:10.3389/fphys.2019.00284
Townsend R. R. (2016). Arterial stiffness: Recommendations and standardization. Pulse 4 (1), 3–7. doi:10.1159/000448454
Tremblay J. C., Stimpson T. V., Murray K. M., Pyke K. E. (2019). Sitting cross-legged for 30 min alters lower limb shear stress pattern but not flow-mediated dilation or arterial stiffness. Appl. Physiology, Nutr. Metabolism 44 (2), 221–224. doi:10.1139/apnm-2018-0339
Tremblay M. S., Aubert S., Barnes J. D., Saunders T. J., Carson V., Latimer-Cheung A. E., et al. (2017). Sedentary behavior research network (SBRN)–terminology consensus project process and outcome. Int. J. Behav. Nutr. Phys. Act. 14 (1), 75. doi:10.1186/s12966-017-0525-8
Tüchsen F., Hannerz H., Burr H., Krause N. (2005). Prolonged standing at work and hospitalisation due to varicose veins: A 12 year prospective study of the Danish population. Occup. Environ. Med. 62 (12), 847–850. doi:10.1136/oem.2005.020537
Tüchsen F., Krause N., Hannerz H., Burr H., Kristensen T. S. (2000). Standing at work and varicose veins. Scand. J. Work Environ. Health 26, 414–420. doi:10.5271/sjweh.562
Unkart J. T., Allison M. A., Parada H., Criqui M. H., Qi Q., Diaz K. M., et al. (2020). Sedentary time and peripheral artery disease: The hispanic community health study/study of latinos. Am. Heart J. 222, 208–219. doi:10.1016/j.ahj.2020.02.005
van Duijnhoven N. T. L., Bleeker M. W. P., de Groot P. C. E., Thijssen D. H. J., Felsenberg D., Rittweger J., et al. (2008). The effect of bed rest and an exercise countermeasure on leg venous function. Eur. J. Appl. Physiol. 104 (6), 991–998. doi:10.1007/s00421-008-0854-z
van Duijnhoven N. T. L., Green D. J., Felsenberg D., Belavy D. L., Hopman M. T. E., Thijssen D. H. J. (2010a). Impact of bed rest on conduit artery remodeling: Effect of exercise countermeasures. Hypertension 56 (2), 240–246. doi:10.1161/HYPERTENSIONAHA.110.152868
van Duijnhoven N. T. L., Thijssen D. H. J., Green D. J., Felsenberg D., Belavý D. L., Hopman M. T. E. (2010b). Resistive exercise versus resistive vibration exercise to counteract vascular adaptations to bed rest. J. Appl. Physiol. 108 (1), 28–33. doi:10.1152/japplphysiol.00760.2009
Van Varik B., Rennenberg R., Reutelingsperger C., Kroon A., de Leeuw P., Schurgers L. J. (2012). Mechanisms of arterial remodeling: Lessons from genetic diseases. Front. Genet. 3, 290. doi:10.3389/fgene.2012.00290
Varona J. F., Ortiz-Regalón R., Sánchez-Vera I., López-Melgar B., García-Durango C., Vázquez J. M. C., et al. (2019). Soluble ICAM 1 and VCAM 1 blood levels alert on subclinical atherosclerosis in non smokers with asymptomatic metabolic syndrome. Arch. Med. Res. 50 (2), 20–28. doi:10.1016/j.arcmed.2019.05.003
Vlachopoulos C., Aznaouridis K., Stefanadis C. (2010). Prediction of cardiovascular events and all-cause mortality with arterial stiffness: A systematic review and meta-analysis. J. Am. Coll. Cardiol. 55 (13), 1318–1327. doi:10.1016/j.jacc.2009.10.061
Volpi E., Mittendorfer B., Rasmussen B. B., Wolfe R. R. (2000). The response of muscle protein anabolism to combined hyperaminoacidemia and glucose-induced hyperinsulinemia is impaired in the elderly. J. Clin. Endocrinol. Metab. 85 (12), 4481–4490. doi:10.1210/jcem.85.12.7021
Vranish J. R., Young B. E., Kaur J., Patik J. C., Padilla J., Fadel P. J. (2017). Influence of sex on microvascular and macrovascular responses to prolonged sitting. Am. J. Physiol. Heart Circ. Physiol. 312 (4), H800–H805. doi:10.1152/ajpheart.00823.2016
Vranish J. R., Young B. E., Stephens B. Y., Kaur J., Padilla J., Fadel P. J. (2018). Brief periods of inactivity reduce leg microvascular, but not macrovascular, function in healthy young men. Exp. Physiol. 103 (10), 1425–1434. doi:10.1113/EP086918
Walker M. A., Bailey T. G., McIlvenna L., Allen J. D., Green D. J., Askew C. D. (2019). Acute dietary nitrate supplementation improves flow mediated dilatation of the superficial femoral artery in healthy older males. Nutrients 11 (5), 954. doi:10.3390/nu11050954
Walsh L. K., Restaino R. M., Martinez-Lemus L. A., Padilla J. (2017). Prolonged leg bending impairs endothelial function in the popliteal artery. Physiol. Rep. 5 (20), e13478. doi:10.14814/phy2.13478
Wang Q., Liang X., Wang L., Lu X., Huang J., Cao J., et al. (2012). Effect of omega-3 fatty acids supplementation on endothelial function: A meta-analysis of randomized controlled trials. Atherosclerosis 221 (2), 536–543. doi:10.1016/j.atherosclerosis.2012.01.006
Wassmann S., Stumpf M., Strehlow K., Schmid A., Schieffer B., Böhm M., et al. (2004). Interleukin-6 induces oxidative stress and endothelial dysfunction by overexpression of the angiotensin II type 1 receptor. Circ. Res. 94 (4), 534–541. doi:10.1161/01.RES.0000115557.25127.8D
Watenpaugh D. E. (2016). Analogs of microgravity: Head-down tilt and water immersion. J. Appl. Physiol. 120 (8), 904–914. doi:10.1152/japplphysiol.00986.2015
Wen C.-Y., Yang A.-S., Tseng L.-Y., Chai J.-W. (2010). Investigation of pulsatile flowfield in healthy thoracic aorta models. Ann. Biomed. Eng. 38 (2), 391–402. doi:10.1007/s10439-009-9835-6
Wheeler M. J., Dunstan D. W., Smith B., Smith K. J., Scheer A., Lewis J., et al. (2019). Morning exercise mitigates the impact of prolonged sitting on cerebral blood flow in older adults. J. Appl. Physiol. 126 (4), 1049–1055. doi:10.1152/japplphysiol.00001.2019
Wijndaele K., White T., Andersen L. B., Bugge A., Kolle E., Northstone K., et al. (2019). Substituting prolonged sedentary time and cardiovascular risk in children and youth: A meta-analysis within the international children’s accelerometry database (ICAD). Int. J. Behav. Nutr. Phys. Act. 16 (1), 96–10. doi:10.1186/s12966-019-0858-6
Wolters F. J., Zonneveld H. I., Hofman A., van der Lugt A., Koudstaal P. J., Vernooij M. W., et al. (2017). Cerebral perfusion and the risk of dementia: A population-based study. Circulation 136 (8), 719–728. doi:10.1161/CIRCULATIONAHA.117.027448
Woodman C. R., Schrage W. G., Rush J. W. E., Ray C. A., Price E. M., Hasser E. M., et al. (2001). Hindlimb unweighting decreases endothelium-dependent dilation and eNOS expression in soleus not gastrocnemius. J. Appl. Physiol. 91 (3), 1091–1098. doi:10.1152/jappl.2001.91.3.1091
World Health O. (2010). WHO guidelines on drawing blood: Best practices in phlebotomy. Geneva: World Health Organization.
Wu S. P., Ringgaard S., Oyre S., Hansen M. S., Rasmus S., Pedersen E. M. (2004). Wall shear rates differ between the normal carotid, femoral, and brachial arteries: An in vivo MRI study. J. Magn. Reson. Imaging 19 (2), 188–193. doi:10.1002/jmri.10441
Yamaji T., Harada T., Hashimoto Y., Nakano Y., Kajikawa M., Yoshimura K., et al. (2022). Self-reported total sitting time on a non-working day is associated with blunted flow-mediated vasodilation and blunted nitroglycerine-induced vasodilation. Sci. Rep. 12 (1), 6366. doi:10.1038/s41598-022-10242-8
Yan S., Fu W., Wang C., Mao J., Liu B., Zou L., et al. (2020). Association between sedentary behavior and the risk of dementia: A systematic review and meta-analysis. Transl. Psychiatry 10 (1), 112–118. doi:10.1038/s41398-020-0799-5
Yang M., Zhang X., Ding Z., Wang F., Wang Y., Jiao C., et al. (2020). Low skeletal muscle mass is associated with arterial stiffness in community-dwelling Chinese aged 45 years and older. BMC public health 20 (1), 226–229. doi:10.1186/s12889-020-8323-7
Yates T., Edwardson C. L., Celis-Morales C., Biddle S. J. H., Bodicoat D., Davies M. J., et al. (2020). Metabolic effects of breaking prolonged sitting with standing or light walking in older south asians and white Europeans: A randomized acute study. J. Gerontol. A Biol. Sci. Med. Sci. 75 (1), 139–146. doi:10.1093/gerona/gly252
Yates T., Khunti K., Wilmot E. G., Brady E., Webb D., Srinivasan B., et al. (2012). Self-reported sitting time and markers of inflammation, insulin resistance, and adiposity. Am. J. Prev. Med. 42 (1), 1–7. doi:10.1016/j.amepre.2011.09.022
Yew B., Nation D. A., Alzheimer’s Disease Neuroimaging I. (2017). Cerebrovascular resistance: Effects on cognitive decline, cortical atrophy, and progression to dementia. Brain 140 (7), 1987–2001. doi:10.1093/brain/awx112
Younger A. M., Pettitt R. W., Sexton P. J., Maass W. J., Pettitt C. D. (2016). Acute moderate exercise does not attenuate cardiometabolic function associated with a bout of prolonged sitting. J. Sports Sci. 34 (7), 658–663. doi:10.1080/02640414.2015.1068435
Yun M.-J., Kim Y.-K., Kang D.-M., Kim J.-E., Ha W.-C., Jung K.-y., et al. (2018). A study on prevalence and risk factors for varicose veins in nurses at a University hospital. Saf. Health Work 9 (1), 79–83. doi:10.1016/j.shaw.2017.08.005
Żebrowska A., Mizia-Stec K., Mizia M., Gąsior Z., Poprzęcki S. (2015). Omega-3 fatty acids supplementation improves endothelial function and maximal oxygen uptake in endurance-trained athletes. Eur. J. Sport Sci. 15 (4), 305–314. doi:10.1080/17461391.2014.949310
Zeigler Z. S., Mullane S. L., Crespo N. C., Buman M. P., Gaesser G. A. (2016). Effects of standing and light-intensity activity on ambulatory blood pressure. Med. Sci. Sports Exerc. 48 (2), 175–181. doi:10.1249/MSS.0000000000000754
Zeigler Z. S., Swan P. D., Bhammar D. M., Gaesser G. A. (2015). Walking workstation use reduces ambulatory blood pressure in adults with prehypertension. J. Phys. Act. Health 12 (1), S119–S127. doi:10.1123/jpah.2013-0487
Zemaitis M. R., Boll J. M., Dreyer M. A. (2017).“Peripheral arterial disease,” in StatPearls [internet] (Treasure Island, FL: StatPearls Publishing).
Zempo H., Isobe M., Naito H. (2017). Link between blood flow and muscle protein metabolism in elderly adults. J. Phys. Fit. Sports Med. 6 (1), 25–31. doi:10.7600/jpfsm.6.25
Zhang L., Guo Q., Feng B. L., Wang C. Y., Han P. P., Hu J., et al. (2019). A cross-sectional study of the association between arterial stiffness and sarcopenia in Chinese community-dwelling elderly using the asian working group for sarcopenia criteria. J. Nutr. Health Aging 23 (2), 195–201. doi:10.1007/s12603-018-1147-9
Zhang Q., Gao B., Gu K., Chang Y., Xu J. (2014). The study on hemodynamic effect of varied support models of BJUT-II VAD on coronary artery: A primary CFD study. Asaio J. 60 (6), 643–651. doi:10.1097/MAT.0000000000000137
Keywords: sitting, sedentary, inactivity, vascular, cerebrovascular, endothelial
Citation: Daniele A, Lucas SJE and Rendeiro C (2022) Detrimental effects of physical inactivity on peripheral and brain vasculature in humans: Insights into mechanisms, long-term health consequences and protective strategies. Front. Physiol. 13:998380. doi: 10.3389/fphys.2022.998380
Received: 19 July 2022; Accepted: 25 August 2022;
Published: 27 September 2022.
Edited by:
Irena Levitan, University of Illinois at Chicago, United StatesReviewed by:
Mark Daniel Ross, Heriot-Watt University, United KingdomJames Lang, Iowa State University, United States
Copyright © 2022 Daniele, Lucas and Rendeiro. This is an open-access article distributed under the terms of the Creative Commons Attribution License (CC BY). The use, distribution or reproduction in other forums is permitted, provided the original author(s) and the copyright owner(s) are credited and that the original publication in this journal is cited, in accordance with accepted academic practice. No use, distribution or reproduction is permitted which does not comply with these terms.
*Correspondence: Catarina Rendeiro, Yy5yZW5kZWlyb0BiaGFtLmFjLnVr