- 1Departments of Pharmacology and Neuroscience, School of Biomedical Sciences, University of North Texas Health Science Center, Fort Worth, TX, United States
- 2School of Physical Education, Shanxi University, Taiyuan, China
- 3Internal Medicine, University of North Texas Health Science Center, Fort Worth, TX, United States
- 4Biostatistics and Epidemiology, School of Public Health, University of North Texas Health Science Center, Fort Worth, TX, United States
- 5Physiology and Anatomy, School of Biomedical Sciences, University of North Texas Health Science Center, Fort Worth, TX, United States
Background: Brief hypoxic exposures are increasingly applied as interventions for aging-related conditions. To optimize the therapeutic impact of hypoxia, knowledge of the sex-related differences in physiological responses to hypoxia is essential. This study compared hypoxia-induced hypoxemic responses in elderly men and women.
Methods: Seven elderly men (70.3 ± 6.0 years old) and nine women (69.4 ± 5.5 years old) breathed 10% O2 for 5 min while arterial (SaO2; transcutaneous photoplethysmography) and cerebral tissue O2 saturation (ScO2; near-infrared spectroscopy), ventilatory frequency, tidal volume, minute-ventilation, and partial pressures of end-tidal O2 (PETO2) and CO2 (mass spectrometry) were continuously monitored. Cerebral tissue oxygen extraction fraction (OEF) equaled (SaO2–ScO2)/SaO2.
Results: During 5 min hypoxia SaO2 fell from 97.0 ± 0.8% to 80.6 ± 4.6% in the men and from 96.3 ± 1.4% to 72.6 ± 4.0% in the women. The slope ΔSaO2/min was steeper in the women than the men (−4.71 ± 0.96 vs. −3.24 ± 0.76%/min; p = 0.005). Although SaO2 fell twice as sharply per unit decrease in PETO2 in the women than the men (−1.13 ± 0.11 vs. −0.54 ± 0.06%/mmHg; p = 0.003), minute-ventilation per unit hypoxemia increased less appreciably in the women (−0.092 ± 0.014 vs. −0.160 ± 0.021 L/min/%; p = 0.023). OEF fell with hypoxia duration in the women, but remained stable in the men.
Conclusion: During 5 min hypoxic breathing, elderly women experience more intense hypoxemia and reduced chemoreflex sensitivity vs. their male counterparts, which may lower OEF stability in women despite augmented O2 dissociation from hemoglobin during hypoxia. These sex-related differences merit attention when implementing brief hypoxic exposures for therapeutic purposes.
Introduction
The differences in oxygen carrying capacity in men vs. women are well recognized. Women have smaller lung volumes (Mead 1980; Sheel, et al., 2004), lower hematocrits and blood hemoglobin (Hb) contents (Tilling, et al., 2013; Tzounakas, et al., 2021), and smaller plasma and blood volumes (Diaz-Canestro, et al., 2022a; Diaz-Canestro, et al., 2022b). Collectively, these factors predict lower cardiorespiratory function and aerobic capacity in women vs. men (Harms 2006; Diaz-Canestro, et al., 2022a). Moreover, exercise induced arterial hypoxemia is more prevalent in women than men (Harms, et al., 1998; Richards, et al., 2004), which may be ascribed to less robust acute ventilatory responses to hypoxia in women (Guenette, et al., 2004). Cardiac baroreflex responses were attenuated in middle-aged women vs. age-matched men, while estrogen replacement in women abolished the sex difference in these responses (Huikuri, et al., 1996). Female sex hormones also dampen apnea-induced neurogenic vasoconstriction (Patel, et al., 2014). However, scant data are available regarding the sex-related differences in chemoreflex-mediated cardiac or ventilatory responses to hypoxemia, which could persist even after sex hormones have subsided in older women.
Intermittent hypoxic (IH) training involving cyclic ventilation with moderately hypoxic gas alternated with room air breathing, has been increasingly adopted to treat various age-related pathological conditions. IH training proved beneficial in patients with coronary artery disease (Burtscher, et al., 2004), chronic obstructive pulmonary disease (Burtscher, et al., 2009; Haider, et al., 2009), hypertension (Lyamina, et al., 2011), and mild cognitive impairment (Bayer, et al., 2017b; Serebrovska, et al., 2019; Wang, et al., 2020). Moreover, IH programs alternating moderately hypoxic and hyperoxic ventilation combined with physiotherapy or physical activity have found to improve cognitive performance and physical function and health in elderly adults (Bayer, et al., 2017a; Bayer, et al., 2017b; Bayer, et al., 2019; Serebrovska, et al., 2019; Behrendt, et al., 2022). In contrast, intense IH imposed by obstructive sleep apnea (hypoxia combined with hypercapnia) is an established risk factor for heart disease, stroke and cognitive impairment. Because the intensities of hypoxia-induced hypoxemia, tissue hypoxia and its related physiological responses are pivotal determinants of the benefits vs. detriments of hypoxic interventions (Navarrete-Opazo and Mitchell 2014), knowledge of sex differences in cardio-respiratory responses to hypoxia are indispensable to optimize the therapeutic efficacy of IH training.
This study compared the changes in arterial O2 saturation (SaO2), cerebral tissue oxygenation (ScO2) and cardiac and ventilatory responses during moderate hypoxic exposure in elderly men vs. women. The subjects breathed 10% O2 for 5 min, resulting in a mild to moderate hypoxemia which is well-tolerated (Zhang, et al., 2010; Liu, et al., 2017; Liu, et al., 2020) and improves cerebrovascular and cardioventilatory function in healthy adults (Zhang, et al., 2014; Zhang, et al., 2015; Wang, et al., 2020). Because women have lower aerobic capacity, cardiorespiratory function, acute ventilatory responses to hypoxia, and cardiac baroreflex responses than their male counterparts, this study tested the hypothesis that elderly women will experience more intense hypoxemia than elderly men when breathing moderately hypoxic air.
Materials and methods
Participants
Sixteen elderly men (n = 7) and women (n = 9) free of cardiorespiratory, metabolic and renal disease voluntarily participated in the study after providing written informed consent and passing a physical screening with arterial blood pressure below 140/90 mmHg. The men and women had similar body mass indices, although height and body surface area estimated from the DuBois formula (DuBois and DuBois, 1916) were significantly smaller in the women than men (Table 1). The study protocols were reviewed and approved by the Institutional Review Board for the Protection of Human Subjects at the University of North Texas Health Science Center (IRB Project #2016-070).
Hypoxia protocol
All subjects breathed poikilocapnic hypoxic air containing 10% O2. Before each experiment, a mass spectrometer gas analyzer (Perkin-Elmer, 1100 Medical Gas Analyzer, St Louis, Missouri) was calibrated with room air and medical gas (Instrumentational Laboratory, Lexington, MA) containing 10% O2 and 5% CO2 (balance N2). After calibration, the gas analyzer confirmed the hypoxic gas contained 10 ± 0.2% O2 (balance N2). All measurements were made with the subject wearing a disposable air-cushioned facemask (VacuMed, Ventura, CA) while resting in the supine position. After 10 min equilibration, baseline (pre-hypoxic) measurements were collected for approximately 3 min, and then normobaric, poikilocapnic hypoxia was applied for 5 min. No discomfort, distress, or dizziness was reported by any subjects or observed by the investigators, either before, during or after hypoxia. Ambient conditions were maintained throughout testing with barometric pressure 735–745 mmHg, relative humidity 50–58% and room temperature 24 ± 1°C.
Measurements
Measurements of O2, CO2 and ventilatory and cardiovascular function were described previously (Zhang, et al., 2010; Zhang, et al., 2014; Liu, et al., 2020) and are summarized here. Systemic arterial O2 saturation (SaO2) was measured by a transcutaneous sensor (TOSCA 500, Radiometer America Inc., Westlake, OH, United States) applied to the right earlobe. This measurement yielded SaO2 values essentially identical to values obtained from the finger using BIOPAC OXY100C (lab observation). Cerebral tissue O2 saturation (ScO2) of the prefrontal cortex was monitored by near-infrared spectroscopy with a sensor (Somanetics, 5100 INVOS Cerebral Oximeter, Troy, MI) placed on the right forehead. Cerebral tissue oxygen extraction fraction (OEF) was taken as (SaO2—ScO2)/SaO2.
Breath-by-breath inspired and expired fractions of O2 and CO2 were continuously monitored by mass spectrometry. Gas was sampled via a tubing embedded in the inlet of a Universal Ventilation Meter (UMV VacuMed, Ventura, CA), which recorded breath-by-breath ventilatory frequency (fBr) and tidal volume (VT). Minute ventilation equaled VT • fBr. Partial pressures of O2 and CO2 in end-tidal gas (PETO2 and PETCO2) were calculated by multiplying ambient barometric pressure by the expired fractions of O2 and CO2, respectively. Heart rate (HR) was monitored by standard limb lead II electrocardiography. Systolic and diastolic blood pressures (SBP and DBP) were measured by double finger cuffs placed on the proximal phalanges of the left index and middle fingers (CNAP 500, Graz, Austria). Mean arterial pressure (MAP) was calculated as 1/3 of SBP plus 2/3 of DBP. Analog data were continuously digitized at 250 Hz by a computer interfaced with a data acquisition system (MP150 BIOPAC, Santa Barbara, CA).
Data analyses
Baseline (i.e., 0 min hypoxia) variables were averaged from 60 s of continuous data collected immediately before initiating hypoxic breathing. During 5 min hypoxia, data from the last 30s of each minute were averaged to represent the minute-by-minute data (Zhang, et al., 2010; Liu, et al., 2017; Liu, et al., 2020). All ventilatory, cardiovascular, SaO2 and ScO2 data during hypoxic exposure passed the Shapiro-Wilk normality test, except breathing frequency in the female group. Demographic and baseline variables in male vs. female subjects were compared using two-sample t-test with Statistical Analysis System (SAS) software (Version 9.4, Cary, NC). Changes in SaO2 and ScO2 vs. hypoxia duration, i.e. slope, were determined using linear regression models in SAS. The effect-size index of both SaO2 and ScO2 slopes was estimated through the partial omega squared (
Results
Baseline variables
Baseline SaO2, ScO2, PETO2, PETCO2 and ventilatory function variables did not differ significantly between the elderly male and female subjects (Table 1). However, systolic, diastolic and mean arterial pressures were higher in the women than the men, and HR showed a strong trend toward higher values in the women.
SaO2 and ScO2 during hypoxic exposure
Arterial O2 saturation fell progressively with the duration of hypoxia exposure in both elderly men (−3.25 ± 0.31%/min, R2 = 0.96, p = 0.001) and women (−4.70 ± 0.40%/min, R2 = 0.97, p = 0.001). During the fifth minute of hypoxia, SaO2 was 80.6 ± 4.6% in the men and 72.6 ± 4.0% in the women, respectively. ANCOVA revealed a significant interaction of hypoxic duration x group factor (p = 0.021), indicating a significant difference in the slopes of the two groups (Figure 1A). The rate of SaO2 decline, was significantly greater (p = 0.005) in the elderly women (−4.71 ± 0.96%/min, n = 9) than men (−3.24 ± 0.76%/min, n = 7) (Figure 1B), indicating 5 min exposure to 10% O2 produced more rapid intensification of arterial hypoxemia in the elderly women. ScO2 paralleled the hypoxemia. During the 5-min hypoxia, ScO2 values fell from 67.9 ± 5.2% to 52.1 ± 5.3% in the women and from 70.1 ± 2.7% to 58.3 ± 2.6% in the men (Figure 1C). ANCOVA revealed a more rapid ScO2 decline (p = 0.052) in the women (−3.09 ± 0.25%/min, R2 = 0.97, p = 0.001) than men (−2.32 ± 0.22%/min, R2 = 0.96, p = 0.001). The slope ΔScO2/min was steeper (p = 0.004) in the elderly women (−3.09 ± 0.39%/min, n = 9) than men (−2.31 ± 0.50%/min, n = 7); thus, the elderly women experienced more intense cerebral tissue hypoxia during hypoxic breathing (Figure 1D). The partial Omega squared (
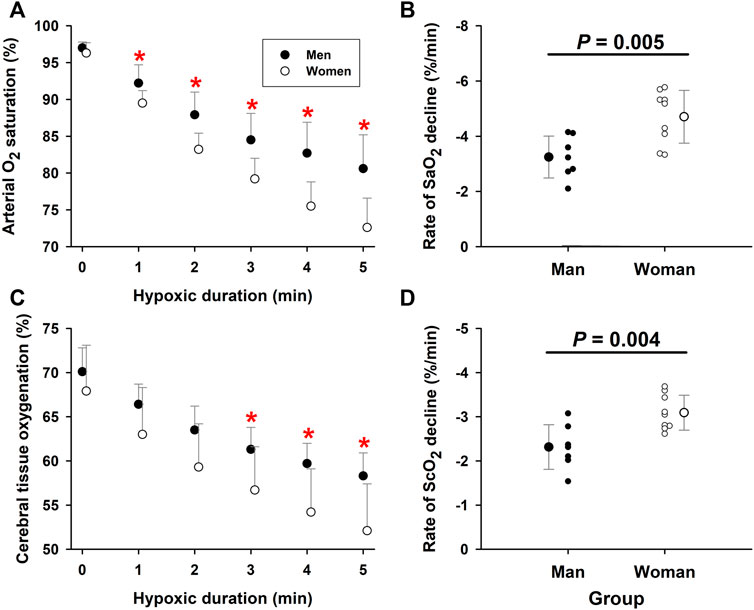
FIGURE 1. Arterial O2 saturation and cerebral tissue oxygenation during moderate hypoxia in elderly men vs. women. Panel (A): Arterial O2 saturation (SaO2) fell more rapidly in elderly women than men during 5-min moderate hypoxia. Panel (B): The individual and group rates of SaO2 decline during 5-min hypoxia were greater in the elderly women than men (unpaired t-test). Panel (C): ScO2 fell with hypoxia duration to a greater extent in elderly women than men. Panel (D): ScO2 fell more rapidly in elderly women than elderly men during 5-min hypoxia (unpaired t-test). *Post-hoc analysis: p < 0.05 vs. values in women. Mean values ±SD. Panels (B) and (D) also show values in individual subjects.
Respiratory and cardiovascular responses during hypoxic exposure
Both VT (time factor p = 0.003) and minute ventilation (time factor p = 0.001) increased during hypoxia, as expected. Ventilatory frequency (fBr) did not change during 5-min hypoxia (time factor p = 0.486) (Figure 2A), but remained higher in the women than men (group factor p = 0.001). Since fBr was unchanged, the increased minute ventilation could be ascribed to the hypoxemia-elicited increase in VT (Figures 2B,C). Both VT and minute ventilation were lower in the women than the men (group factor p = 0.001 for VT and p = 0.003 for minute ventilation).
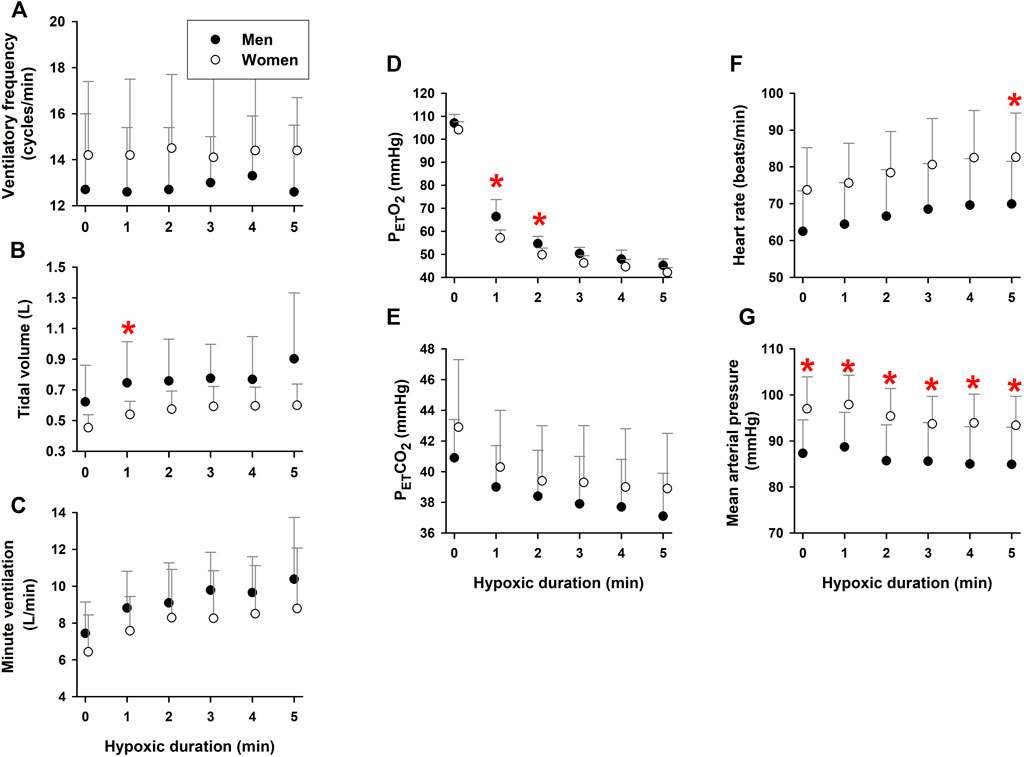
FIGURE 2. Respiratory and cardiovascular responses during 5-min hypoxia. Ventilatory frequency did not change [Panel (A)], while tidal volume [Panel (B)] and, thus, minute-ventilation [Panel (C)] increased during hypoxia in elderly men and women. The women had higher ventilatory frequencies and lower tidal volumes and minute ventilation than the men. PETO2 [Panel (D)] and PETCO2 [Panel (E)] fell during hypoxia. The women [had lower PETO2 and higher PETCO2 values than the men. Hypoxic exposure increased heart rate [Panel (F)], without affecting mean arterial pressure [Panel (G)]. Heart rate and mean arterial pressure were higher in the elderly women than men. *Post-hoc analysis: p < 0.05 vs. values in women. Mean values ±SD.
PETO2 fell as systemic hypoxemia intensified (time factor p = 0.003) during 5-min hypoxia (Figure 2D). PETCO2 also fell during hypoxia (time factor p = 0.008), due to increased minute ventilation (Figure 2E). PETO2 was lower (group factor p = 0.012) and PETCO2 was greater (group factor p = 0.001) in the women vs. the men. Heart rate increased during hypoxia (time factor p = 0.015) (Figure 2F), but mean arterial pressure did not (time factor p = 0.205), indicating that the hypoxemia elicited tachycardiac but not hypertensive responses (Figure 2G). Both HR and MAP were greater (group factor p = 0.001) in the women than men throughout hypoxia.
Sex-related differences in cardioventilatory responses to hypoxemia
During hypoxic exposure, the slope of SaO2 vs. PETO2 was doubled (p = 0.003) in the women (1.125 ± 0.106%/mmHg, R2 = 0.97, p = 0.002) vs. men (0.539 ± 0.062%/mmHg, R2 = 0.95, p = 0.003) (Figure 3). This apparent enhancement of O2-hemoglobin dissociation indicated that the elderly women reached the steeper portion of the O2-hemoglobin dissociation curve earlier in hypoxia than the men. Increases in minute ventilation could be ascribed to decreases in SaO2 in both the women (−0.092 ± 0.014 L/min/%; R2 = 0.90, p = 0.003) and men (−0.160 ± 0.020 L/min/%; R2 = 0.95, p = 0.002) (Figure 4A). However, the Δminute ventilation/ΔSaO2 slope was shallower (p = 0.023) in the women than men during hypoxic exposure, suggesting lower sensitivity of the chemoreflex-mediated ventilatory compensation in elderly women. On the other hand, the slope of minute ventilation vs. PETO2 (Figure 4B) was not different (p = 0.441) in the women (−0.035 ± 0.005 L/min/mmHg, R2 = 0.91, p = 0.002) vs. men (−0.042 ± 0.007 L/min/mmHg, R2 = 0.88, p = 0.003). Although the ΔHR/ΔSaO2 slope tended to be shallower in the women (-0.409 ± 0.027 bpm/%; R2 = 0.98, p < 0.001) vs. men (−0.480 ± 0.022 bpm/%; R2 = 0.99, p < 0.001) (Figure 4C), the difference was not statistically significant (p = 0.107). The ΔHR/ΔPETO2 slope was not different (p = 0.689) in the women (−0.131 ± 0.043 bpm/mmHg, R2 = 0.62, p = 0.040) vs. the men (−0.109 ± 0.025 bpm/mmHg, R2 = 0.78, p = 0.012) (Figure 4D). Cerebral tissue OEF in the women fell with increasing hypoxia duration (slope −0.0030 ± 0.0006 fraction/min; R2 = 0.88, p = 0.006), while in the men, OEF did not change during hypoxia (p = 0.248) (Figure 5).
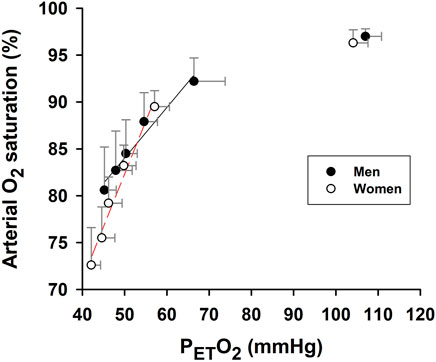
FIGURE 3. Association of arterial O2 saturation and partial pressure of end-tidal O2. Slope of SaO2 vs. end-tidal partial pressure O2 (PETO2) during 5-min hypoxia was significantly steeper in women than men. Baseline (pre-hypoxia) data (upper right) are not included in the linear regressions (solid and broken lines) or slope comparison. Symbols represent mean values ±SD at each min of the 5-min hypoxia exposure.
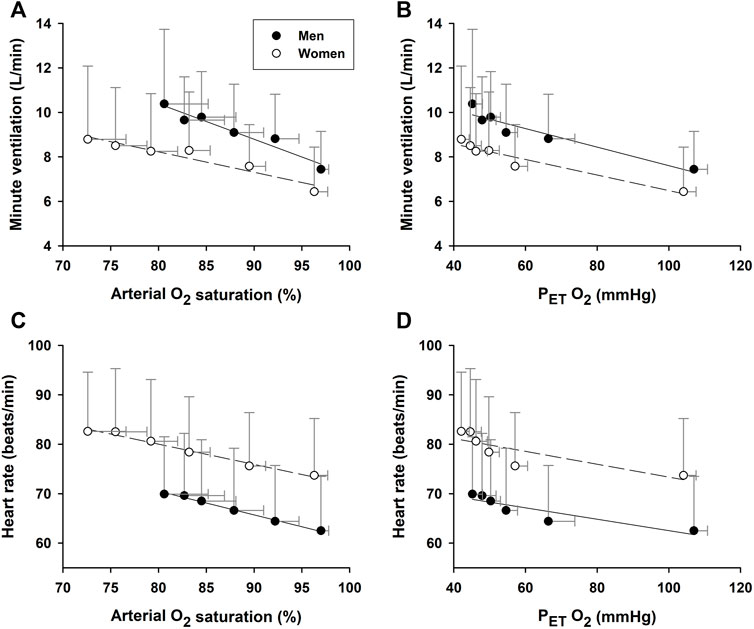
FIGURE 4. Associations of minute ventilation and heart rate vs. arterial O2 saturation. The increase of minute ventilation per unit decrease in arterial O2 saturation during hypoxic exposure [Panel (A)] was attenuated in the elderly women vs. men. However, the slopes of minute ventilation per unit decrease in PETO2 [Panel (B)] were not significantly different between the two groups. Neither the increase in heart rate per unit SaO2 decrease [Panel (C)] nor the heart rate decrease per unit decrease in PETO2 [Panel (D)] differed between the groups.
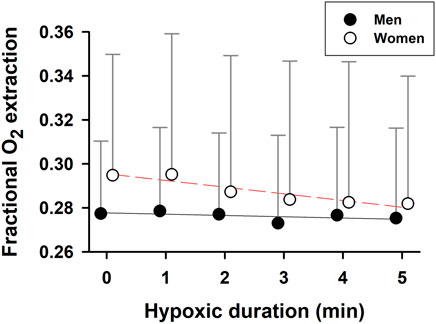
FIGURE 5. Cerebral tissue fractional oxygen extraction during hypoxia. Cerebral tissue fractional O2 extraction declined with hypoxic time in the elderly women, but was stable over 5-min hypoxia in the elderly men. Mean values ±SD.
Discussion
This study is the first to demonstrate sex differences in hypoxia-induced arterial hypoxemia and cerebral tissue hypoxia in elderly adults, where 5-min exposure to 10% O2 produced more precipitous declines in arterial O2 saturation and cerebral tissue oxygenation in women vs. men. In general, women have smaller physiques and, thus, lower blood volume and hemoglobin mass than their male counterparts (Falz et al., 2019; Diaz-Canestro et al., 2022a; Diaz-Canestro et al., 2022b); consequently, vascular PaO2 and O2 content are likely to fall more rapidly in women than men during hypoxia. Indeed, in this study O2-hemoglobin dissociation at a given decrease in PETO2 was greater in women than men exposed to the same intensity (10% O2) and duration (5 min) of hypoxic breathing. Furthermore, the increase in minute ventilation per unit decrease in SaO2 was smaller in the women, indicating less robust chemoreflex-mediated ventilatory compensation than in men. Consequently, cerebral tissue OEF progressively fell with hypoxic duration in the elderly women, but remained constant during hypoxia in the elderly men.
The mechanisms producing the more severe hypoxia-induced arterial hypoxemia in elderly women are not fully understood. We postulated that the lower oxygen carrying capacity and cardiorespiratory function in women could contribute to more intense hypoxemia during hypoxic exposure. Underlying factors could include lower blood hemoglobin content (Tilling, et al., 2013; Tzounakas, et al., 2021), lower circulating capacity, i.e., total blood volume (Diaz-Canestro, et al., 2022a; Diaz-Canestro, et al., 2022b), and smaller reservoir, i.e., body physique or surface area (see Table 1) in women. Collectively, these factors would cause blood O2 content to fall more rapidly in women than men at a given hypoxia intensity, producing a more severe arterial hypoxemia at the same hypoxic intensity and duration. A diminished chemoreflex-mediated ventilatory response in the elderly women vs. their age-matched male counterparts also may have contributed to the more intense hypoxemia during 10% O2 breathing in women vs. men.
We previously reported a shift toward decreased hemoglobin O2-binding affinity during hypoxia, a phenomenon which intensified with repeated hypoxic exposures (Zhang, et al., 2010; Zhang, et al., 2014). Enhanced O2-hemoglobin dissociation augments O2 release to O2-consuming tissues in proportion to the duration and number of hypoxic exposures, potentially offsetting the decreased arterial O2 content. However, increased O2 unloading also exacerbates arterial hypoxemia by depleting oxygenated hemoglobin. This mechanism may underlie the greater exercise-induced arterial hypoxemia in exercise trained young adults vs. age-matched, sedentary men and women (Guenette, et al., 2004). Our data indicate that the rate of SaO2 decrease per unit decrease in PETO2 was appreciably greater in elderly women vs. men (Figure 3). This phenomenon was likely a pivotal contributor to the greater hypoxia-induced hypoxemia in the elderly women in this study.
Evidence for sex-related differences in ventilatory responses to hypoxia remains inconclusive (Guenette, et al., 2004). Some studies reported no sex difference in ventilatory response to acute normobaric poikilocapnic or isocapnic hypoxia (10% O2) in young adults (Rispen, et al., 2017), yet others have shown less robust ventilatory responses to mild normobaric hypoxia (15% O2) associated with more intense hypoxemia in women (Camacho-Cardenosa, et al., 2022). In the present study, the ventilation increase per unit hypoxemia (i.e., decrease in SaO2) was significantly lower in the elderly women than men (Figure 4A: −0.10 vs. −0.16 L/min/%, p = 0.023). This attenuated chemoreflex-mediated ventilatory response could not be ascribed to counteraction by hyperventilation-induced hypocapnia, because PETCO2 was persistently higher in the women than men (Figure 2E). The tachycardic response per unit hypoxemia was not significant between the two groups (Figure 4B: −0.41 bpm/% in women vs. −0.48 bpm/% in men; p = 0.107).
During hypoxia, cerebral tissue fractional O2 extraction is increased or maintained in young adults (Liu, et al., 2017), whereas skeletal muscle OEF declines with exposure time (Zhang, et al., 2010). These tissue-specific differences in OEF, which may favor greater O2 release from hemoglobin in the brain vs. muscles, should serve to protect the brain, which is utterly dependent on O2 for ATP production. In this study, cerebral tissue OEF remained constant in elderly men, but fell in elderly women during hypoxia (Figure 5). The decreased OEF in the women was associated with more intense arterial hypoxemia combined with a diminished ventilatory response during hypoxia, could destabilize O2 delivery to the brain in elderly women vs. men.
This study confirmed that increases in minute ventilation in both elderly men and women during hypoxic breathing were predominantly driven by increases in tidal volume. Deeper breathing during hypoxia may lower thoracic and jugular venous pressures, thereby facilitating cerebral venous outflow to accommodate the increased cerebral arterial inflow in response to hypoxia-induced cerebral vasodilation (Liu, et al., 2017). This study further confirmed that moderate hypoxia-induced hypoxemia caused no hypertensive responses in elderly men and women. Although hypoxic exposure increased HR in both sexes, there was no increase in MAP associated with the tachycardic response. Since cardiac output could increase with the increased heart rate, MAP stability during hypoxia suggests that active vasodilation may have counteracted hypoxia-induced sympathoexcitation or increased heart rate.
Study limitations and perspectives
Study limitations include using PETO2 instead of partial pressure of dissolved O2 in arterial blood (PaO2) to evaluate O2-hemoglobin dissociation during hypoxic exposure. Nonetheless, breath-by-breath continuously measured PETO2 should change in lockstep with alveolar PO2 (Zhang, et al., 2010; Zhang, et al., 2014; Liu, et al., 2017) and, thus, report changes in PaO2 as well. Although the slope of minute ventilation vs. SaO2 was significantly smaller in the elderly women than men, there was no difference in the slopes of minute ventilation vs. PETO2 between the sexes, This discrepancy is probably related to a non-simply linear decrease in PETO2 during hypoxia (Figure 2D). The study sample size is small, especially in the male group (n = 7), since this study is not a priori in design. Moreover, since cardiorespiratory fitness or function may affect exercise-induced arterial hypoxemia, future studies with expanded sample size are required to define potential fitness-related differences in hypoxia-induced arterial hypoxemia in elderly men and women.
Despite these limitations, there is an important practical implication of this study: that the sex-related differences should be taken into account when applying normobaric poikilocapnic IH training as a non-pharmacological prophylactic and therapeutic strategy to improve neurocognitive performance, physiological function or physical well-being in elderly men and women. In elderly men, breathing moderately hypoxic air containing ≥10% O2 for ≤3 min may barely attain the threshold to trigger compensatory responses based on active cerebrovasodilation (Liu, et al., 2017). Most elderly men can tolerate ≥6 min exposure to 10% O2 without experiencing severe hypoxemia. On the other hand, in some elderly women ≥6 min exposure to 10% O2 may reduce SaO2 and/or ScO2 to critical levels, i.e., c. ≤65% SaO2 or ≤50% ScO2 (Figure 1). Since more severe hypoxemia cannot further augment cerebral vasodilation and blood flow to compensate for cerebral tissue hypoxia (Liu, et al., 2017), it may induce dizziness or lightheadedness.
In conclusion, this study demonstrated more intense arterial hypoxemia in elderly women than elderly men during hypoxic breathing. Physiologically, this greater hypoxia-induced hypoxemia may be explained by an increase in O2-hemoglobin dissociation and attenuation of the chemoreflex-mediated ventilatory compensation in the elderly women vs. their age-matched male counterparts. These sex-related differences in arterial hypoxemia and the hypoxemia-elicited ventilatory responses should be taken into account when implementing hypoxic regimens for elderly adults.
Data availability statement
The raw data supporting the conclusions of this article will be made available by the authors, without undue reservation.
Ethics statement
The studies involving human participants were reviewed and approved by The North Texas Regional IRB. The patients/participants provided their written informed consent to participate in this study.
Author contributions
Conceived and planned experiments: JZ, RM, and XS. Performed experiments: JZ, XS. Analyzed data: JZ, ZZ, and XS. Interpreted results of experiments: JZ, YD, GK, ZZ, RM, and XS. Prepared figures and drafted manuscript: JZ, ZZ, RM, and XS. Edited the manuscript: JZ, RM, and XS. Approved final version of manuscript: JZ, YD, GK, ZZ, RM, and XS.
Funding
This study was supported in part by Texas Alzheimer’s Research and Care Consortium Investigator Grant and R01AG076675 to XS and Grant #202103021224022 to JZ. Study data are available from XS on request.
Acknowledgments
We sincerely thank our volunteer subjects for their cheerful participation and Hannah Schenck for her technical assistance.
Conflict of interest
The authors declare that the research was conducted in the absence of any commercial or financial relationships that could be construed as a potential conflict of interest.
Publisher’s note
All claims expressed in this article are solely those of the authors and do not necessarily represent those of their affiliated organizations, or those of the publisher, the editors and the reviewers. Any product that may be evaluated in this article, or claim that may be made by its manufacturer, is not guaranteed or endorsed by the publisher.
References
Bayer U., Glazachev O. S., Likar R., Burtscher M., Kofler W., Pinter G., et al. (2017a). Adaptation to intermittent hypoxia-hyperoxia improves cognitive performance and exercise tolerance in elderly. Adv. Gerontol. 30 (2), 255–261.
Bayer U., Likar R., Pinter G., Stettner H., Demschar S., Trummer B., et al. (2019). Effects of intermittent hypoxia-hyperoxia on mobility and perceived health in geriatric patients performing a multimodal training intervention: A randomized controlled trial. BMC Geriatr. 19 (1), 167. doi:10.1186/s12877-019-1184-1
Bayer U., Likar R., Pinter G., Stettner H., Demschar S., Trummer B., et al. (2017b). Intermittent hypoxic-hyperoxic training on cognitive performance in geriatric patients. Alzheimers Dement. 3 (1), 114–122. doi:10.1016/j.trci.2017.01.002
Behrendt T., Bielitzki R., Behrens M., Glazachev O. S., Schega L. (2022). Effects of intermittent hypoxia-hyperoxia exposure prior to aerobic cycling exercise on physical and cognitive performance in geriatric patients-A randomized controlled trial. Front. Physiol. 13, 899096. doi:10.3389/fphys.2022.899096
Burtscher M., Haider T., Domej W., Linser T., Gatterer H., Faulhaber M., et al. (2009). Intermittent hypoxia increases exercise tolerance in patients at risk for or with mild COPD. Respir. Physiol. Neurobiol. 165 (1), 97–103. doi:10.1016/j.resp.2008.10.012
Burtscher M., Pachinger O., Ehrenbourg I., Mitterbauer G., Faulhaber M., Puhringer R., et al. (2004). Intermittent hypoxia increases exercise tolerance in elderly men with and without coronary artery disease. Int. J. Cardiol. 96 (2), 247–254. doi:10.1016/j.ijcard.2003.07.021
Camacho-Cardenosa A., Camacho-Cardenosa M., Tomas-Carus P., Timón R., Olcina G., Burtscher M. (2022). Acute physiological response to a normobaric hypoxic exposure: Sex differences. Int. J. Biometeorol. 66 (7), 1495–1504. doi:10.1007/s00484-022-02298-y
Diaz-Canestro C., Pentz B., Sehgal A., Montero D. (2022a). Sex differences in cardiorespiratory fitness are explained by blood volume and oxygen carrying capacity. Cardiovasc. Res. 118 (1), 334–343. doi:10.1093/cvr/cvab028
Diaz-Canestro C., Pentz B., Sehgal A., Montero D. (2022b). Sex differences in orthostatic tolerance are mainly explained by blood volume and oxygen carrying capacity. Crit. Care Explor. 4 (1), e0608. doi:10.1097/CCE.0000000000000608
DuBois D., DuBois E. F. (1916). A formula to estimate the approximate surface area if height and weight be known. Arch. Intern. Med. 17, 863–871. doi:10.1001/archinte.1916.00080130010002
Falz R. S., Fikenzer S. H., Busse M. (2019). Normal values of hemoglobin mass and blood volume in young, active women and men. Int. J. Sports Med. 40, 236–244. doi:10.1055/a-0826-9235
Guenette J. A., Diep T. T., Koehle M. S., Foster G. E., Richards J. C., Sheel A. W. (2004). Acute hypoxic ventilatory response and exercise-induced arterial hypoxemia in men and women. Respir. Physiol. Neurobiol. 143 (1), 37–48. doi:10.1016/j.resp.2004.07.004
Haider T., Casucci G., Linser T., Faulhaber M., Gatterer H., Ott G., et al. (2009). Interval hypoxic training improves autonomic cardiovascular and respiratory control in patients with mild chronic obstructive pulmonary disease. J. Hypertens. 27 (8), 1648–1654. doi:10.1097/HJH.0b013e32832c0018
Harms C. A. (2006). Does gender affect pulmonary function and exercise capacity? Respir. Physiol. Neurobiol. 151 (2-3), 124–131. doi:10.1016/j.resp.2005.10.010
Harms C. A., McClaran S. R., Nickele G. A., Pegelow D. F., Nelson W. B., Dempsey J. A. (1998). Exercise-induced arterial hypoxaemia in healthy young women. J. Physiol. 507, 619–628. doi:10.1111/j.1469-7793.1998.619bt.x
Huikuri H. V., Pikkujämsä S. M., Airaksinen K. E., Ikäheimo M. J., Rantala A. O., Kauma H., et al. (1996). Sex-related differences in autonomic modulation of heart rate in middle-aged subjects. Circulation 94 (2), 122–125. doi:10.1161/01.cir.94.2.122
Liu X., Chen X., Kline G., Ross S. E., Hall J. R., Ding Y., et al. (2020). Reduced cerebrovascular and cardioventilatory responses to intermittent hypoxia in elderly. Respir. Physiol. Neurobiol. 271, 103306. doi:10.1016/j.resp.2019.103306
Liu X., Xu D., Hall J. R., Ross S., Chen S., Liu H., et al. (2017). Enhanced cerebral perfusion during brief exposures to cyclic intermittent hypoxemia. J. Appl. Physiol. 123 (6), 1689–1697. doi:10.1152/japplphysiol.00647.2017
Lyamina N. P., Lyamina S. V., Senchiknin V. N., Mallet R. T., Downey H. F., Manukhina E. B. (2011). Normobaric hypoxia conditioning reduces blood pressure and normalizes nitric oxide synthesis in patients with arterial hypertension. J. Hypertens. 29 (11), 2265–2272. doi:10.1097/HJH.0b013e32834b5846
Mead J. (1980). Dysanapsis in normal lungs assessed by the relationship between maximal flow, static recoil, and vital capacity. Am. Rev. Respir. Dis. 121 (2), 339–342. doi:10.1164/arrd.1980.121.2.339
Navarrete-Opazo A., Mitchell G. S. (2014). Therapeutic potential of intermittent hypoxia: A matter of dose. Am. J. Physiol. Regul. Integr. Comp. Physiol. 307 (10), R1181–R1197. doi:10.1152/ajpregu.00208.2014
Olejnik S., Algina J. (2003). Generalized eta and omega squared statistics: Measures of effect size for some common research designs. Psychol. Methods 8 (4), 434–447. doi:10.1037/1082-989X.8.4.434
Patel H. M., Heffernan M. J., Ross A. J., Muller M. D. (2014). Sex differences in forearm vasoconstrictor response to voluntary apnea. Am. J. Physiol. Heart Circ. Physiol. 306 (3), H309–H316. doi:10.1152/ajpheart.00746.2013
Richards J. C., McKenzie D. C., Warburton D. E., Road J. D., Sheel A. W. (2004). Prevalence of exercise-induced arterial hypoxemia in healthy women. Med. Sci. Sports Exerc. 36 (9), 1514–1521. doi:10.1249/01.mss.0000139898.30804.60
Rispen L., Marks D., Green S. (2017). Dynamic ventilatory responses of females and males to acute isocapnic and poikilocapnic hypoxia. Respir. Physiol. Neurobiol. 245, 57–64. doi:10.1016/j.resp.2017.05.005
Serebrovska Z. O., Serebrovska T. V., Kholin V. A., Tumanovska L. V., Shysh A. M., Pashevin D. A., et al. (2019). Intermittent hypoxia-hyperoxia training improves cognitive function and decreases circulating biomarkers of alzheimer's disease in patients with mild cognitive impairment: A pilot study. Int. J. Mol. Sci. 20 (21), E5405. doi:10.3390/ijms20215405
Sheel A. W., Richards J. C., Foster G. E., Guenette J. A. (2004). Sex differences in respiratory exercise physiology. Sports Med. 34 (9), 567–579. doi:10.2165/00007256-200434090-00002
Tilling L., Hunt J., Jiang B., Sanders T. A., Clapp B., Chowienczyk P. (2013). Endothelial function does not relate to haemoglobin or serum erythropoietin concentrations and these do not explain the gender difference in endothelial function in healthy middle-aged men and women. Eur. J. Clin. Invest. 43 (3), 225–230. doi:10.1111/eci.12033
Tzounakas V. L., Anastasiadi A. T., Drossos P. V., Karadimas D. G., Valsami S., Stamoulis K. E., et al. (2021). Sex-related aspects of the red blood cell storage lesion. Blood Transfus. 19 (3), 224–236. doi:10.2450/2020.0141-20
Wang H., Shi X., Schenck H., Hall J. R., Ross S. E., Kline G. P., et al. (2020). Intermittent hypoxia training for treating mild cognitive impairment: A pilot study. Am. J. Alzheimers Dis. Other Demen. 35, 1533317519896725. doi:10.1177/1533317519896725
Zhang P., Downey H. F., Chen S., Shi X. (2014). Two-week normobaric intermittent hypoxia exposures enhance oxyhemoglobin equilibrium and cardiac responses during hypoxemia. Am. J. Physiol. Regul. Integr. Comp. Physiol. 307 (6), R721–R730. doi:10.1152/ajpregu.00191.2014
Zhang P., Downey H. F., Shi X. (2010). Acute intermittent hypoxia exposures enhance arterial oxygen delivery. Exp. Biol. Med. 235 (9), 1134–1141. doi:10.1258/ebm.2010.009393
Keywords: aging, chemoreflex, heart rate (HR), oxygen dissociation, oxygen extraction fraction (OEF), ventilation
Citation: Zhao J, Ding Y, Kline GP, Zhou Z, Mallet RT and Shi X (2022) Hypoxic breathing produces more intense hypoxemia in elderly women than in elderly men. Front. Physiol. 13:989635. doi: 10.3389/fphys.2022.989635
Received: 08 July 2022; Accepted: 12 October 2022;
Published: 26 October 2022.
Edited by:
Alba Camacho-Cardenosa, University of Extremadura, SpainReviewed by:
Vincent Joseph, Laval University, CanadaIsmael Martínez Guardado, Nebrija University, Spain
Copyright © 2022 Zhao, Ding, Kline, Zhou, Mallet and Shi. This is an open-access article distributed under the terms of the Creative Commons Attribution License (CC BY). The use, distribution or reproduction in other forums is permitted, provided the original author(s) and the copyright owner(s) are credited and that the original publication in this journal is cited, in accordance with accepted academic practice. No use, distribution or reproduction is permitted which does not comply with these terms.
*Correspondence: Xiangrong Shi, Xiangrong.Shi@unthsc.edu