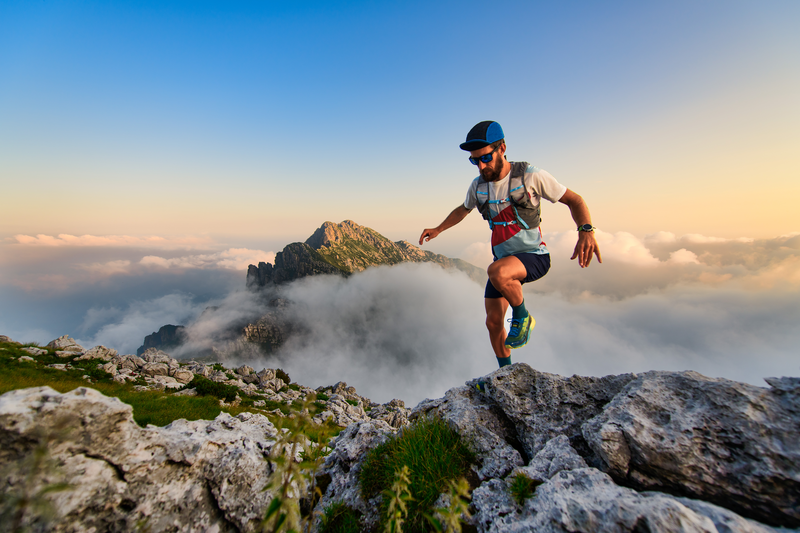
94% of researchers rate our articles as excellent or good
Learn more about the work of our research integrity team to safeguard the quality of each article we publish.
Find out more
ORIGINAL RESEARCH article
Front. Physiol. , 09 September 2022
Sec. Exercise Physiology
Volume 13 - 2022 | https://doi.org/10.3389/fphys.2022.986920
Purpose: The purpose of the present study was to compare the acute physiological and perceptual responses between resistance-type high-intensity interval training (R-HIIT)and cycling high-intensity interval training (C-HIIT).
Methods: Twelve healthy and active men randomly performed C-HIIT and R-HIIT. The C-HIIT protocol was performed on a cycle ergometer and consisted of ten 60 s working intervals at 90% PPO separated by a 60 s active recovery at 25% PPO. The R-HIIT protocol consisted of ten 60 s working intervals (barbell back squat with a load of 20% bodyweight, maximum 30 reps) separated by 60 s passive recovery period in an unloaded standing position. Oxygen consumption (
Results: Peak (p < 0.05) and average
Conclusion: The R-HIIT protocol can elicit similar cardiovascular, hormones, and perceptual responses as C-HIIT but with a higher contribution to the anaerobic glycolysis energy system. In contrast, C-HIIT is superior to R-HIIT for increasing oxygen consumption during exercise. Therefore, the two types of HIIT may lead to different metabolic and neuromuscular adaptations.
Lack of physical activity or exercise is a major cause of most chronic diseases (Booth et al., 2012). As mentioned in the World Health Organization guidelines, adults should regularly spend at least 150–300 min on moderate-intensity or 75–150 min on vigorous-intensity, or engage in combination of moderate to vigorous physical activity throughout the week resulting in an equivalent level. This can reduce the incidences of chronic diseases (World Health Organization, 2018). High-intensity interval training (HIIT) has become a popular exercise modality worldwide (Thompson, 2022) and is characterized by repeated vigorous exercise at near-maximal workloads (≥ 85% HRmax or ≥ 80%
R-HIIT is generally performed in a circuit manner that alternately stimulates different muscle groups using multiple resistance exercises. However, different resistance exercises produce different levels of stimulation of the cardiorespiratory systems. This causes large fluctuations in cardiorespiratory responses throughout the exercise and is lower than in traditional HIIT (Riegler et al., 2017; Schleppenbach et al., 2017; Bellissimo et al., 2022; Järvinen et al., 2022). In a study by Järvinen et al. (Järvinen et al., 2022), they found that performing R-HIIT involving whole-body muscle groups, oxygen uptake was lower when the movement mainly engaged upper limbs and trunk muscle groups, while oxygen uptake was highest when lower limb muscle groups were highly engaged. Additionally, inconsistent cardiorespiratory responses were also elicited by different lower extremity movements in R-HIIT (Bellissimo et al., 2022). Therefore, we hypothesize that R-HIIT using a single lower extremity dynamic exercise can continue to increase cardiorespiratory response in a similar way to that seen with traditional HIIT. The squat is a classic resistance training exercise that involves neuromuscular and movement patterns similar to numerous sports and daily activities. As a result, it can enhance the athletic performance of athletes or improve the health and quality of life of the general population. (Schoenfeld, 2010). An initial study by Takai et al. (2013) showed that 8 weeks of bodyweight squats significantly reduced body fat and increased lean body mass, muscle thickness, muscular strength of the knee extensors, and jump performance in adolescent boys. Another study by Falz et al. (2019) compared the acute physiological responses of cycling MICT, HIIT, and resistance-type HIIT. They found that among the five bodyweight movements (squats, push-ups, isometric back extension, isometric leg raise, and inverted rows), only squats could elicit comparable stroke volumes as traditional HIIT. Based on the above studies, we hypothesized that squat-based R-HIIT might achieve the same cardiorespiratory response as traditional cycling HIIT.
Exercise can disrupt physiological homeostasis and cause significant hormonal changes. The appropriate endocrine response elicited by exercise can cause beneficial adaptations in the body, thereby helping in enhancing physical health as well as sports performance (Hackney and Lane, 2015). Testosterone and cortisol have been identified as the anabolic and catabolic hormonal responses to exercise-induced physiological stress, respectively (Adlercreutz et al., 1986). However, few studies have reported that different exercise modalities elicit different hormonal responses (Harris et al., 2018; Velasco-Orjuela et al., 2018). Therefore, the acute changes in testosterone and cortisol levels may vary between resistance-type HIIT and cycling HIIT because of differences in muscle activity and metabolism characteristics.
The autonomic nervous system (ANS) increases cardiac activity and controls hemodynamics by reducing parasympathetic nerve activity and simultaneously diverting sympathetic innervation during exercise. It also regulates the cardiovascular system to promote recovery after exercise (Borresen and Lambert, 2008). It is important to consider the time required for recovery of ANS after the workout, in addition to the response of the cardiovascular system during exercise. A better understanding of the recovery duration indicated by cardiac parasympathetic reactivation following exercise can contribute to improved programming of training sessions that aim to induce metabolic and cardiorespiratory adaptations (Stanley et al., 2013). Changes in cardiac parasympathetic activity can be assessed rapidly and non-invasively by measuring heart rate variability (HRV) after exercise (Malik, 1996). However, there are limited studies comparing the differences in HRV responses between resistance-type HIIT and cycling HIIT.
The acute response and long-term adaptation of physiological systems could be affected by the combinations of different training variables (work/recovery ratios, intensity and duration of the work/recovery periods) in HIIT (Buchheit and Laursen, 2013). The cycling HIIT pattern used in this study was 60:60 s with a sub-maximum load. This pattern is considered safe and effective in improving the health and fitness of varied populations of adults, including those who are active (Raleigh et al., 2016), sedentary (Hood et al., 2011), and overweight/obese (Boyd et al., 2013). The purpose of this study was to compare the acute oxygen consumption (
Eighteen recreationally active male participants were initially recruited from Capital University of Physical Education and Sports (Haidian District, Beijing, China). The inclusion criteria were: aged between 18 and 28 years, performing structured exercise training at least twice a week (≥ 150 min of moderate-intensity or 75 min of vigorous-intensity/week). None of the subjects had previously participated in systematic resistance training or high-intensity interval training. The exclusion criteria were: any disease, injury, or other condition that could compromise the ability to perform the exercise. Since the six subjects did not meet the requirements, 12 subjects (21.9 ± 2.6 years) were included in the study. The height, body mass, percentage of body fat, and body mass index were 178.5 ± 4. 5 cm, 72.4 ± 6.0 kg, 18.2 ± 6.1%, and 22.2 ± 1.3 kg/m2, respectively (Table 1). Prior to study initiation, the participants were fully informed of the study procedures, and all of them signed a written informed consent form. The minimal sample size of 12 was determined by a priori analyses using G*Power software (version 3.1.9.2) based on the following parameters: an alpha level of 0.05, a power (1-beta) equal to 0.8, and an effect size of 0.4.
The current study employed a randomized crossover design. The experiments consisted of five visits to the laboratory. All visit sessions were separated by at least 72 h and at least 1 week washout period between two HIIT sessions. During the first visit, participants accepted anthropometric measurements and a maximal incremental cycling test to determine
FIGURE 1. Experimental design of the study. C-HIIT, cycling high-intensity interval training; R- HIIT, resistance-type high-intensity interval training; PPO, peak power output;
Participants’ height (cm) and bodyweight were measured using an ultrasonic tester (DHM-200, China) and BMI was calculated by dividing the subjects’ weight (kilograms) by the square of their height (meters). Body composition was assessed by dual x-ray absorptiometry (Lunar, United States). All anthropometric data are shown in Table 1.
Peak power output (PPO) was identified at the work rate coincident with volitional fatigue. When the exercise intensity could not be maintained for the full 60 s, PPO was calculated using the equation (Kuipers et al., 1985):
PPO is the peak power output (W), Wfinal is the last power output (W) completed for 60 s, t (s) is the amount of time reached in the final uncompleted stage, T (s) is the duration of each stage (60 s), and Winc (W) is the workload increment (15 W).
The participants performed two exercise sessions in random order and on two separate occasions (Figure 1). Participants were given verbal encouragement during both HIIT protocols. In our pilot experiment, we matched the intensity of the two HIIT conditions by assessing the perceived effort levels directly after exercise. This method has been used by other researchers (Rønnestad et al., 2020; Järvinen et al., 2022).
Before starting C-HIIT, participants performed approximately 10 min of warm-up, including dynamic stretches and 3 min cycling at 50 W. The warm-up was followed by a 1-min transition period before the start of the session. The C-HIIT protocol was performed on a cycle ergometer (ergoline 100 K, Germany) and consisted of ten 60 s working intervals at 90% PPO interspersed with a 60 s active recovery at 25% PPO. During the exercise, subjects were instructed to maintain pedal cadence between 60–65 revolutions per minute.
Before starting R-HIIT, participants performed approximately 10 min of warm-up, including dynamic stretches and squats without load. The R-HIIT protocol consisted of ten 60 s working intervals (barbell back squat with a load of 20% bodyweight) separated by 60 s recovery periods (put down the loaded barbell and rest in a standing position). The participant placed the barbell on the trapezius with the assistance of the researcher and held the barbell in both hands. During all repetitions, participants were asked to squat down to approximately 90° knee flexion, the feet were required to maintain contact with the floor (e.g., no jumping or lifting of the heels), and full hip and knee extension was required at the conclusion of each repetition. They were instructed to perform the eccentric phase of each squat for 1 s while performing the concentric phase of each squat as fast as possible to a standing position. The maximum number of squats completed per working interval is 30 reps. A metronome is used to control the tempo of the movements (60 beats/min). If the participant is unable to complete the movement at the prescribed tempo, a brief rest in a standing position with load is allowed. The researcher removed the barbell immediately after each working interval and repositioned the barbell in the participant’s trapezius 5 s before the next exercise bout.
During exercise, all
Blood lactate concentration from fingertip blood sample was measured with a portable device (Lactate Scout, H/P COSMOS, Germany) before warm-up, 3 min after exercise, and 30 min after exercise. Venous blood samples were collected before the warm-up, immediately after exercise, and 30 min after exercise for the determinutesation of testosterone and cortisol levels. The whole blood samples were allowed to clot at room temperature for 30 min, then centrifuged at 3,000 rpm for 10 min at 4°C. The separated serum samples were frozen and kept at −80°C. The serum testosterone and cortisol levels were measured with the radioimmunoassay method (XH6080, Xi’an Nuclear Instrument Factory, China).
All variables related to HRV were assessed in 4 moments: 1) before warm-up, 2) 5 min after exercise, 3) 35 min after exercise, and 4) 24 h after exercise. Autonomic nervous system activity in HRV was measured by an electrocardiographic workstation (Shenzhen Boying Medical Instrument Technology Co., China). Electrocardiogram electrodes were placed on their wrists with the subjects in the supine position. The values of every R-R interval were obtained by the system completely independent of human operation, and 5 min were selected for analysis. For the HRV data processing, the Kubios software (v.3.5.0, HRV analysis, Finland) (Tarvainen et al., 2014) was used. The time-domain variable of HRV used in the present analysis was the root mean square difference of successive normal R-R interval (RMSSD). The frequency domain variable was high-frequency power of 0.15–0.40 Hz (HF). Because the raw values of RMSSD and HF were skewed toward higher values and violated assumptions of normality, natural log(ln) transformation was applied (lnRMSSD and lnHF).
Data are presented as mean ± standard deviation (SD). Statistical analyses were performed with SPSS Statistics (version 26, IBM, United States). Shapiro–Wilk tests were used to check normality assumptions. The alpha level was set at a value of P of <0.05 for indication of statistical significance for all analyses. Two-tailed paired sample t-tests were used to identify statistical differences in measurements of peak or average of
FIGURE 2.
There was no significant difference in HRpeak, HRpeak (%), HRmean and HRmean (%) between C-HIIT and R-HIIT (p = 0.218, d = 0.27; p = 0.216, d = 0.42; p = 0.070, d = 0.26; p = 0.069, d = 0.48; Table 2). The changes in HR during the two exercise sessions are displayed in Figure 3. For all time points during exercise (0–19 min). No significant time × condition interaction effect was observed on HR (F = 0.906, p = 0.436, and η2 = 0.040). No significant main effect was observed for exercise condition on HR (F = 0.308, p = 0.584, and η2 = 0.014). A significant main effect was observed for time in which HR significantly increased across exercise (F = 141.184, p < 0.001, and η2 = 0.865).
Aerobic and total EE measured during C-HIIT was significantly higher than during R-HIIT (p < 0.001, d = 1.34; p = 0.001, d = 1.21; Table 2). Anaerobic glycolytic EE measured during R-HIIT was significantly higher than during C-HIIT (p = 0.002, d = 1.34; Table 2).
There was no significant difference in RPEpeak and RPEmean between C-HIIT and R-HIIT (p = 0.059, d = 0.89, p = 0.935, and d = 0.04; Table 2). The changes in RPE during the two exercise sessions are displayed in Figure 4. For all intervals during exercise (1–10 intervals). A significant time × condition interaction effect was observed on RPE (F = 6.233, p = 0.001, and η2 = 0.221). No significant main effect was observed for exercise condition on RPE (F = 0.005, p = 0.943, and η2 < 0.001). A significant main effect was observed for time in which RPE significantly increased across exercise (F = 166.795, p < 0.001, and η2 = 0.883).
For blood lactate (Table 3; Figure 5A). A significant time × condition interaction effect was observed (F = 7.339, p = 0.010, and η2 = 0.250). A significant main effect was observed for exercise condition (F = 5.653, p = 0.027, and η2 = 0.204). A significant main effect was observed for time (F = 392.073, p < 0.001, and η2 = 0.947). For both exercise conditions, lactate increased above baseline after exercise (p < 0.001) and remained elevated through 30 min (p < 0.001). Blood lactate was significantly higher at 3 min post-exercise for R-HIIT than C-HIIT (p = 0.014, d = 1.15). No differences were observed for blood lactate at baseline (p = 0.685, d = 0.33) or 30 min after exercise (p = 0.552, d = 0.25) between C-HIIT and R-HIIT.
FIGURE 5. Blood lactate (A) at baseline (Pre), 3 min after exercise (Post 3 min), and 30 min after exercise (Post 30 min). Testosterone (B) and Cortisol (C) at baseline (Pre), immediately after exercise (Post 0 min), and 30 min after exercise (Post 30 min). lnRMSSD (D) and lnHF (E) at baseline (Pre), 5 min after exercise (Post 5 min), 35 min after exercise (Post 35 min), and 24 h after exercise (Post 24 h). *p < 0.05 R-HIIT was significantly higher than C-HIIT during exercise, # < 0.05 significantly different from Pre.
For testosterone (Table 3; Figure 5B). No significant time × condition interaction effect was observed (F = 0.309, p = 0.735, and η2 = 0.014). No significant main effect was observed for exercise condition (F = 0.001, p = 0.970, and η2 < 0.001). A significant main effect was observed for time (F = 55.193, p < 0.001, and η2 = 0.418). For both exercise conditions, testosterone increased above baseline immediately after exercise (p < 0.05) and returned to baseline 30 min after exercise (p > 0.05). No differences were observed for testosterone at baseline (p = 0.756, d = 0.08), immediately after exercise (p = 0.564, d = 0.28) and 30 min after exercise (p = 0.776, d = 0.09) between C-HIIT and R-HIIT.
For cortisol (Table 3; Figure 5C). No significant time × condition interaction effect was observed (F = 0.352, p = 0.658, η2 = 0.016). No significant main effect was observed for exercise condition (F = 0.020, p = 0.887, η2 = 0.001). A significant main effect was observed for time (F = 17.172, p < 0.001, η2 = 0.715). For both conditions, cortisol increased above baseline immediately after exercise (p < 0.001) and returned to baseline 30 min after exercise (p > 0.05). No differences were observed for cortisol at baseline (p = 0.745, d = 0.12), immediately after exercise (p = 0.753, d = 0.14) and 30 min after exercise (p = 0.757, d = 0.13) between C-HIIT and R-HIIT.
For lnRMSSD (Table 3; Figure 5D). No significant time × condition interaction effect was observed (F = 0.589, p = 0.578, and η2 = 0.026). No significant main effect was observed for exercise condition (F = 0.460, p = 0.505, and η2 = 0.020). A significant main effect was observed for time (F = 44.152, p < 0.001, and η2 = 0.667). For both conditions, lnRMSSD dropped significantly from baseline at 5 and 35 min after exercise (p < 0.001) and returned to baseline 24 h after exercise (p > 0.05). No differences were observed for lnRMSSD at baseline (p = 0.564, d = 0.22), 5 min after exercise (p = 0.332, d = 0.41), 35 min after exercise (p = 0.633, d = 0.16) and 24 h after exercise (p = 0.791, d = 0.25) between C-HIIT and R-HIIT.
For lnHF (Table 3; Figure 5E). No significant time × condition interaction effect was observed (F = 0.789, p = 0.472, and η2 = 0.035). No significant main effect was observed for exercise condition (F = 0.046, p = 0.531, and η2 = 0.018). A significant main effect was observed for time (F = 65.510, p < 0.001, and η2 = 0.749). For both conditions, lnHF dropped significantly from baseline at 5 and 35 min after exercise (p < 0.001) and returned to baseline 24 h after exercise (p > 0.05). No differences were observed for lnHF at baseline (p = 0.661, d = 0.21), 5 min after exercise (p = 0.351, d = 0.40), 35 min after exercise (p = 0.477, d = 0.31) and 24 h after exercise (p = 0.752, d = 0.10) between C-HIIT and R-HIIT.
In this study, we compared the acute physiological and perceptual responses between resistance-type high-intensity interval training (R-HIIT) and cycling high-intensity interval training (C-HIIT). The major findings were that C-HIIT induced greater oxygen consumption and energy expenditure while R-HIIT elicited higher blood lactate levels. In contrast to our hypothesis, no significant differences in heart rate, testosterone, cortisol, heart rate variability, and perceived exertion ratings in the two HIIT conditions.
Our findings confirmed that squat-based R-HIIT could consistently elevate the cardiorespiratory response as traditional cycling HIIT (Figures 2, 3). On the other hand, the results also showed that the peak and mean oxygen consumption of R-HIIT remained lower than that of C-HIIT (Table 2). This result is likely attributed to the greater recruitment of lower threshold motor units during C-HIIT that relies more on aerobic metabolism for adenosine triphosphate supply (Wood et al., 2016). In contrast, R-HIIT recruits more high-threshold motor units because of the need to overcome external load as the requirement of “as fast as possible in concentric contraction” that relies more on anaerobic metabolic systems. This speculation could be supported by higher blood lactate levels observed after R-HIIT exercise (Table 3). Moreover, the type of muscle contraction could also affect oxygen demand. For instance, the primary contraction type of the lower limb muscle groups in cycling is concentric contraction (Bijker et al., 2002), whereas the squatting movement involves eccentric contraction that requires less oxygen (Douglas et al., 2017). Another critical factor is the mode of recovery during the recovery period. R-HIIT uses passive recovery, whereas C-HIIT uses low-intensity active recovery (25% PPO) which is the traditional HIIT model commonly used in research and exercise practice. Active recovery can lead to greater deoxygenation of the exercising muscle groups than passive recovery, which causes higher oxygen demand (Fennell and Hopker, 2021). Therefore, the aerobic and total energy expenditure during exercise was significantly greater in C-HIIT than in R-HIIT. These results are also consistent with several previous studies comparing traditional HIIT with resistance-type HIIT (Schleppenbach et al., 2017; Järvinen et al., 2022).
Although the oxygen consumption of R-HIIT is lower than that of C-HIIT during exercise, the heart rate response is similar to that of C-HIIT (Figure 3). Similarly, other studies have also observed the differences between oxygen uptake and heart rate response. For instance, Bellissimo et al. (2022) compared bodyweight high-intensity interval exercise (HIIE) consisting of various plyometric-based calisthenics to treadmill running HIIE and found that there was less difference in the HR response (%HRpeak ∼5%) than the
In the R-HIIT condition, higher post-exercise blood lactate indicates a greater proportion of anaerobic glycolytic energy system supply. The primary reason is that the muscles must overcome a higher force with each contraction during the squat. Thus, the recruitment of more glycolytic muscle fibers and a more activated glycolytic system leads to a greater accumulation of blood lactate. Moreover, the strong force of muscular contraction might lead to an obstructed blood flow by compressing the blood vessels. Therefore, a reduction in blood flow to the exercising muscles most likely decreased the amount of oxygen being delivered to the mitochondria and reduced the elimination of metabolic end-products (Gotshalk et al., 2004). Additionally, the low-intensity active recovery used in the C-HIIT condition may have facilitated the clearance of blood lactate (Mandroukas et al., 2011). There is some evidence that exercise-induced metabolic stress is associated with acute hormonal responses (Gordon et al., 1994; Goto et al., 2005; Wahl et al., 2010). A previous study has shown that the decreased blood pH during exercise acts as a stimulus for exercise-induced cortisol secretion (Wahl et al., 2010). However, the two HIIT conditions in the present study elicited a similar change in cortisol levels. Our results align with a study done by Harris et al. (2018), where they found that full body low-load, high repetition resistance endurance training elicited higher blood lactate and growth hormone than a matched time and caloric cost cycling protocol but no significant difference in cortisol levels. Therefore, we presume that blood lactate concentrations in R-HIIT may not be sufficient to cause a greater change in cortisol levels. Additionally, no difference in testosterone levels was observed between the two HIIT protocols. The acute increase in testosterone levels can promote protein synthesis, leading to skeletal muscle hypertrophy and increased muscle strength (Vingren et al., 2010; Hooper et al., 2017). It is worth noting that HIIT can modulate the expression of genes and proteins involved in muscle mass regulation, increase muscle protein synthesis and activates muscle satellite cells (Callahan et al., 2021). Since squatting can recruit more and faster muscle fibers than cycling, it can be speculated that in the long term, R-HIIT can offer a greater advantage in building muscle and improving muscle strength.
Cardiac parasympathetic activity is suppressed and is fully recovered after at least 24 h of a single aerobic-based training session (Stanley et al., 2013). The present study showed that both HIIT conditions suppressed parasympathetic activity at 5 and 35 min after exercise and returned to baseline after 24 h with no significant difference. These observations are consistence with a study by Schaun and Del Vecchio (2018), where they compared bodyweight HIIT and cycling HIIT in the Tabata model. Since exercise intensity is an essential factor influencing heart rate variability (Parekh and Lee, 2005), the similar heart rate responses of R-HIIT and C-HIIT during exercise might explain the consistency of HRV change and recovery rate after exercise. From the perspective of the autonomic nervous system, these findings indicate that the participants fully recovered in 24 h after exercise and were able to conduct a subsequent exercise session. However, it is worth noting that the recovery of cardiac parasympathetic activity is not synchronized with other physiological systems. For example, the recovery of circulating muscle creatine kinase levels and protracted muscle pain lags behind the parasympathetic activity after weightlifting training (Chen et al., 2011). Most subjects in our study reported that R-HIIT elicited a greater degree of muscle soreness than C-HIIT 24 h after exercise. This could be attributed to the more severe muscle damage caused by the eccentric muscle contraction involved in the squatting movement (Allen, 2001). The delayed-onset muscle soreness may prevent the practitioner from exercising the next time or may limit the timing and frequency of subsequent sessions. Therefore, it is important for exercise instructors to schedule the exercise session according to the recovery levels of different physiology systems.
There was a clear linear increase in RPE after each working interval throughout both the C-HIIT and the R-HIIT conditions with no significant difference (Figure 4). Previous studies have reported that lower RPE is induced by bodyweight HIIT than by traditional cycling HIIT. Therefore, it can be speculated that alternately activating whole-body muscle groups can reduce local muscle stress compared to constant cycling (Gist et al., 2014; Riegler et al., 2017). In contrast, the squat exercise used in this study was similar to cycling, activating mainly the lower extremity muscle groups and resulting in similar RPE. Despite a gradual increase in RPE, all subjects maintained a defined rpm during the C-HIIT session. We propose that subjects might be able to complete more bouts at the defined rpm and intensity. However, in R-HIIT, not all subjects could complete the prescribed number of reps in all working intervals. Two subjects in the last three bouts and one subject in the last four bouts could not achieve the prescribed 30 reps. Unlike the other studies, the R-HIIT protocol did not use “all-out” as a requirement in the working interval but a fixed number of 30 reps. Thus, we believe it is similar to the “keeping rpm” in cycling and can delay the onset of fatigue. In the case of “as many reps as possible” requirements, the practitioner may accumulate fatigue quickly and perhaps gradually reduce the number of reps during the exercise (Bellissimo et al., 2022). Although there were no statistically significant difference in RPE between C-HIIT and R-HIIT, the higher blood lactate levels in R-HIIT after the last bout indicated that R-HIIT might have accumulated more fatigue. In addition, the greater change magnitude of RPE in R-HIIT might also suggest that fatigue was accumulated more rapidly than in C-HIIT (Figure 4). Excessive fatigue during resistance exercise can undermine correct movement techniques and increase the risk of injury (Trafimow et al., 1993). In order to avoid extreme fatigue during resistance-type HIIT, the exercise intensity could be reduced by changing the load, or/and changing the number of reps completed per working interval, or/and inserting extra rest periods. However, the change of exercise intensity perhaps leads the cardiovascular response would no longer reach the target range.
There are several limitations of the present study that should be acknowledged. First, the definition of HIIT has been used inconsistently. Previous studies have defined HIIT depend on the physiological response induced rather than the type of physical challenge or activity. In this study, we classified HIIT according to the exercise modality. Our results show that the cardiorespiratory response induced by R-HIIT meets the definition of HIIT (>85%HRmax). However, disparities in oxygen uptake and metabolic characteristics between the two HIIT modalities indicate that there might be differences in physiological adaptation. In addition, although some studies have suggested that R-HIIT has advantages over traditional HIIT in improving muscle fitness (McRae et al., 2012; Buckley et al., 2015; Sheykhlouvand et al., 2022), R-HIIT might not be as good as traditional resistance training (RT) in increasing muscle mass and strength because RT allows for heavier loads and longer interval rest periods. Second, although our pilot experiment revealed that participants could perform two types of HIIT with similar perceived effort (RPE), the exercise intensity performed could not precisely match R-HIIT and C-HIIT due to the differences in the exercise forms. Another limitation is that we did not measure 1-repetition maximum to set the squat load but directly used 20% of the individual’s body weight, which may lead to various individual physiological responses during exercise because of differences in strength levels. Similarly, the
Our study demonstrates that the R-HIIT protocol can elicit similar cardiovascular, hormonal, and perceptual responses as C-HIIT but with a higher contribution to the anaerobic glycolysis energy system. In contrast, C-HIIT is superior to R-HIIT for increasing oxygen consumption during exercise. Therefore, the two types of HIIT may lead to different metabolic and neuromuscular adaptations. R-HIIT might be advantageous in simultaneously improving cardiovascular and muscle fitness.
The original contributions presented in the study are included in the article/Supplementary Material, further inquiries can be directed to the corresponding author.
The studies involving human participants were reviewed and approved by Capital University of Physical Education and Sports Ethical Committee. The patients/participants provided their written informed consent to participate in this study. Written informed consent was obtained from the individual(s) for the publication of any potentially identifiable images or data included in this article.
All authors contributed to the study. JM conceived and designed research. Material preparation, data collection and analysis were performed by JM, TW, LZ, and QL. The first draft of the manuscript was written by JM and SB, and all authors commented on previous versions of the manuscript. All authors read and approved the final manuscript.
The present study was funded by the Science and Technology Project of the Beijing Education Commission (KM201810029003).
The authors are grateful to the participants for their time and investment in the investigation.
The authors declare that the research was conducted in the absence of any commercial or financial relationships that could be construed as a potential conflict of interest.
All claims expressed in this article are solely those of the authors and do not necessarily represent those of their affiliated organizations, or those of the publisher, the editors and the reviewers. Any product that may be evaluated in this article, or claim that may be made by its manufacturer, is not guaranteed or endorsed by the publisher.
HR, Heart rate; HRmax, Maximal heart rate; HRmean, Mean heart rate;
Adlercreutz H., Härkönen M., Kuoppasalmi K., Näveri H., Huhtaniemi I., Tikkanen H., et al. (1986). Effect of training on plasma anabolic and catabolic steroid hormones and their response during physical exercise. Int. J. Sports Med. 07, S27–S28. doi:10.1055/s-2008-1025798
Allen D. G. (2001). Eccentric muscle damage: Mechanisms of early reduction of force. Acta Physiol. Scand. 171, 311–319. doi:10.1046/j.1365-201x.2001.00833.x
Bellissimo G. F., Ducharme J., Mang Z., Millender D., Smith J., Stork M. J., et al. (2022). The acute physiological and perceptual responses between bodyweight and treadmill running high-intensity interval exercises. Front. Physiol. 13, 824154. doi:10.3389/fphys.2022.824154
Bijker B., Groot G. de, Laye A., H. (2002). Differences in leg muscle activity during running and cycling in humans. Eur. J. Appl. Physiol. 87, 556–561. doi:10.1007/s00421-002-0663-8
Booth F. W., Roberts C. K., Laye M. J. (2012). “Lack of exercise is a major cause of chronic diseases,” in Comprehensive physiology. Editor R. Terjung (Wiley), 1143doi:10.1002/cphy.c110025
Borresen J., Lambert M. I. (2008). Autonomic control of heart rate during and after exercise : Measurements and implications for monitoring training status. Sports Med. 38 (8), 633–646. doi:10.2165/00007256-200838080-00002
Borst C., Wieling W., van Brederode J. F., Hond A., de Rijk L. G., Dunning A. J. (1982). Mechanisms of initial heart rate response to postural change. Am. J. Physiol. 243, H676–H681. doi:10.1152/ajpheart.1982.243.5.H676
Boyd J. C., Simpson C. A., Jung M. E., Gurd B. J. (2013). Reducing the intensity and volume of interval training diminishes cardiovascular adaptation but not mitochondrial biogenesis in overweight/obese men. PLoS ONE 8, e68091. doi:10.1371/journal.pone.0068091
Buchheit M., Laursen P. B. (2013). High-intensity interval training, solutions to the programming puzzle: Part I: Cardiopulmonary emphasis. Sports Med. 43, 313–338. doi:10.1007/s40279-013-0029-x
Buckley S., Knapp K., Lackie A., Lewry C., Horvey K., Benko C., et al. (2015). Multimodal high-intensity interval training increases muscle function and metabolic performance in females. Appl. Physiol. Nutr. Metab. 40, 1157–1162. doi:10.1139/apnm-2015-0238
Callahan M. J., Parr E. B., Hawley J. A., Camera D. M. (2021). Can high-intensity interval training promote skeletal muscle anabolism? Sports Med. 51, 405–421. doi:10.1007/s40279-020-01397-3
Chen J.-L., Yeh D.-P., Lee J.-P., Chen C.-Y., Huang C.-Y., Lee S.-D., et al. (2011). Parasympathetic nervous activity mirrors recovery status in weightlifting performance after training. J. Strength Cond. Res. 25, 1546–1552. doi:10.1519/JSC.0b013e3181da7858
Costa E. C., Hay J. L., Kehler D. S., Boreskie K. F., Arora R. C., Umpierre D., et al. (2018). Effects of high-intensity interval training versus moderate-intensity continuous training on blood pressure in adults with pre- to established hypertension: A systematic review and meta-analysis of randomized trials. Sports Med. 48, 2127–2142. doi:10.1007/s40279-018-0944-y
di Prampero P. E., Ferretti G. (1999). The energetics of anaerobic muscle metabolism: A reappraisal of older and recent concepts. Respir. Physiol. 118, 103–115. doi:10.1016/S0034-5687(99)00083-3
Dias K. A., Ingul C. B., Tjønna A. E., Keating S. E., Gomersall S. R., Follestad T., et al. (2018). Effect of high-intensity interval training on fitness, fat mass and cardiometabolic biomarkers in children with obesity: A randomised controlled trial. Sports Med. 48, 733–746. doi:10.1007/s40279-017-0777-0
Douglas J., Pearson S., Ross A., McGuigan M. (2017). Eccentric exercise: Physiological characteristics and acute responses. Sports Med. 47, 663–675. doi:10.1007/s40279-016-0624-8
Falz R., Fikenzer S., Holzer R., Laufs U., Fikenzer K., Busse M. (2019). Acute cardiopulmonary responses to strength training, high-intensity interval training and moderate-intensity continuous training. Eur. J. Appl. Physiol. 119, 1513–1523. doi:10.1007/s00421-019-04138-1
Fennell C. R. J., Hopker J. G. (2021). The acute physiological and perceptual effects of recovery interval intensity during cycling-based high-intensity interval training. Eur. J. Appl. Physiol. 121, 425–434. doi:10.1007/s00421-020-04535-x
Garber C. E., Blissmer B., Deschenes M. R., Franklin B. A., Lamonte M. J., Lee I.-M., et al. (2011). American college of sports medicine position stand. Quantity and quality of exercise for developing and maintaining cardiorespiratory, musculoskeletal, and neuromotor fitness in apparently healthy adults: Guidance for prescribing exercise. Med. Sci. Sports Exerc. 43, 1334–1359. doi:10.1249/MSS.0b013e318213fefb
Gist N. H., Freese E. C., Cureton K. J. (2014). Comparison of responses to two high-intensity intermittent exercise protocols. J. Strength Cond. Res. 28, 3033–3040. doi:10.1519/JSC.0000000000000522
Gordon S. E., Kraemer W. J., Vos N. H., Lynch J. M., Knuttgen H. G. (1994). Effect of acid-base balance on the growth hormone response to acute high-intensity cycle exercise. J. Appl. Physiol. 76, 821–829. doi:10.1152/jappl.1994.76.2.821
Goto K., Ishii N., Kizuka T., Takamatsu K. (2005). The impact of metabolic stress on hormonal responses and muscular adaptations. Med. Sci. Sports Exerc. 37 (6), 955. doi:10.1249/01.mss.0000170470.98084.39
Gotshalk L. A., Berger R. A., Kraemer W. J. (2004). Cardiovascular responses to a high-volume continuous circuit resistance training protocol. J. Strength Cond. Res. 18 (4), 760–764. doi:10.1519/14954.1
Hackney A. C., Lane A. R. (2015). “Exercise and the regulation of endocrine hormones,” in Progress in molecular biology and translational science (Elsevier), 293doi:10.1016/bs.pmbts.2015.07.001
Harris N., Kilding A., Sethi S., Merien F., Gottschall J. (2018). A comparison of the acute physiological responses to BODYPUMPTM versus iso-caloric and iso-time steady state cycling. J. Sci. Med. Sport 21, 1085–1089. doi:10.1016/j.jsams.2018.02.010
Hood M. S., Little J. P., Tarnopolsky M. A., Myslik F., Gibala M. J. (2011). Low-Volume interval training improves muscle oxidative capacity in sedentary adults. Med. Sci. Sports Exerc. 43, 1849–1856. doi:10.1249/MSS.0b013e3182199834
Hooper D. R., Kraemer W. J., Focht B. C., Volek J. S., DuPont W. H., Caldwell L. K., et al. (2017). Endocrinological roles for testosterone in resistance exercise responses and adaptations. Sports Med. 47 (9), 1709–1720. doi:10.1007/s40279-017-0698-y
Järvinen L., Lundin Petersdotter S., Chaillou T. (2022). High-intensity resistance exercise is not as effective as traditional high-intensity interval exercise for increasing the cardiorespiratory response and energy expenditure in recreationally active subjects. Eur. J. Appl. Physiol. 122, 459–474. doi:10.1007/s00421-021-04849-4
Kuipers H., Verstappen F., Keizer H., Geurten P., van Kranenburg G. (1985). Variability of aerobic performance in the laboratory and its physiologic correlates. Int. J. Sports Med. 06, 197–201. doi:10.1055/s-2008-1025839
MacInnis M. J., Gibala M. J. (2017). Physiological adaptations to interval training and the role of exercise intensity: Training adaptations and the nature of the stimulus. J. Physiol. 595, 2915–2930. doi:10.1113/JP273196
Malik M. (1996). Heart rate variability.: Standards of measurement, physiological interpretation, and clinical use: Task force of the European society of cardiology and the north American society for pacing and electrophysiology. Ann. Noninvasive Electrocardiol. 1, 151–181. doi:10.1111/j.1542-474X.1996.tb00275.x
Mandroukas A., Heller J., Metaxas T. I., Sendelides T., Riganas C., Vamvakoudis E., et al. (2011). Cardiorespiratory and metabolic alterations during exercise and passive recovery after three modes of exercise. J. Strength Cond. Res. 25, 1664–1672. doi:10.1519/JSC.0b013e3181da7831
McRae G., Payne A., Zelt J. G. E., Scribbans T. D., Jung M. E., Little J. P., et al. (2012). Extremely low volume, whole-body aerobic–resistance training improves aerobic fitness and muscular endurance in females. Appl. Physiol. Nutr. Metab. 37, 1124–1131. doi:10.1139/h2012-093
Milanović Z., Sporiš G., Weston M. (2015). Effectiveness of high-intensity interval training (hit) and continuous endurance training for VO2max improvements: A systematic review and meta-analysis of controlled trials. Sports Med. 45, 1469–1481. doi:10.1007/s40279-015-0365-0
Miyamura M., Honda Y. (1972). Oxygen intake and cardiac output during maximal treadmill and bicycle exercise. J. Appl. Physiol. (1985). 32 (2), 185–188. doi:10.1152/jappl.1972.32.2.-b185
Parekh A., Lee C. M. (2005). Heart rate variability after isocaloric exercise bouts of different intensities. Med. Sci. Sports Exerc. 37, 599–605. doi:10.1249/01.MSS.0000159139.29220.9A
Raleigh J. P., Giles M. D., Scribbans T. D., Edgett B. A., Sawula L. J., Bonafiglia J. T., et al. (2016). The impact of work-matched interval training on VO2peak and VO2 kinetics: Diminishing returns with increasing intensity. Appl. Physiol. Nutr. Metab. 41, 706–713. doi:10.1139/apnm-2015-0614
Ramos J. S., Dalleck L. C., Tjonna A. E., Beetham K. S., Coombes J. S. (2015). The impact of high-intensity interval training versus moderate-intensity continuous training on vascular function: A systematic review and meta-analysis. Sports Med. 45, 679–692. doi:10.1007/s40279-015-0321-z
Riegler M., Stotz G., Fitzgerald K., Munoz C. K., Lewis J., Ring S., et al. (2017). Acute responses to the 7-minute workout. J. Strength Cond. Res. 31, 2572–2578. doi:10.1519/JSC.0000000000002073
Rønnestad B. R., Hansen J., Nygaard H., Lundby C. (2020). Superior performance improvements in elite cyclists following short‐interval vs effort‐matched long‐interval training. Scand. J. Med. Sci. Sports 30, 849–857. doi:10.1111/sms.13627
Ryan B. J., Schleh M. W., Ahn C., Ludzki A. C., Gillen J. B., Varshney P., et al. (2020). Moderate-intensity exercise and high-intensity interval training affect insulin sensitivity similarly in obese adults. J. Clin. Endocrinol. Metab. 105, dgaa345–e2959. doi:10.1210/clinem/dgaa345
Schaun G. Z., Del Vecchio F. B. (2018). High-intensity interval exercises' acute impact on heart rate variability: Comparison between whole-body and cycle ergometer protocols. J. Strength Cond. Res. 32, 223–229. doi:10.1519/JSC.0000000000002180
Schleppenbach L. N., Ezer A. B., Gronemus S. A., Widenski K. R., Braun S. I., Janot J. M. (2017). Speed-and circuit-based high-intensity interval training on recovery oxygen consumption. Int. J. Exerc. Sci. 10 (7), 942
Schoenfeld B. J. (2010). Squatting kinematics and kinetics and their application to exercise performance. J. Strength Cond. Res. 24, 3497–3506. doi:10.1519/JSC.0b013e3181bac2d7
Sheykhlouvand M., Arazi H., Astorino T. A., Suzuki K. (2022). Effects of a new form of resistance-type high-intensity interval training on cardiac structure, hemodynamics, and physiological and performance adaptations in well-trained kayak sprint athletes. Front. Physiol. 13, 850768. doi:10.3389/fphys.2022.850768
Stanley J., Peake J. M., Buchheit M. (2013). Cardiac parasympathetic reactivation following exercise: Implications for training prescription. Sports Med. 43, 1259–1277. doi:10.1007/s40279-013-0083-4
Takai Y., Fukunaga Y., Fujita E., Mori H., Yoshimoto T., Yamamoto M., et al. (2013). Effects of body mass-based squat training in adolescent boys. J. Sports Sci. Med. 12 (1), 60
Tarvainen M. P., Niskanen J.-P., Lipponen J. A., Ranta-aho P. O., Karjalainen P. A. (2014). Kubios HRV – heart rate variability analysis software. Comput. Methods Programs Biomed. 113, 210–220. doi:10.1016/j.cmpb.2013.07.024
Thompson W. R. (2022). Worldwide survey of fitness trends for 2022. ACSM's. Health Fit. J. 26 (1), 11–20. doi:10.1249/fit.0000000000000732
Trafimow S., Trafimow J. H., Schipplein O. D., Novak G. J., Andersson G. B. (1993). The effects of quadriceps fatigue on the technique of lifting. Spine 18 (3), 364–367. doi:10.1097/00007632-199303000-00011
Velasco-Orjuela G. P., Domínguez-Sanchéz M. A., Hernández E., Correa-Bautista J. E., Triana-Reina H. R., García-Hermoso A., et al. (2018). Acute effects of high-intensity interval, resistance or combined exercise protocols on testosterone – cortisol responses in inactive overweight individuals. Physiol. Behav. 194, 401–409. doi:10.1016/j.physbeh.2018.06.034
Vingren J. L., Kraemer W. J., Ratamess N. A., Anderson J. M., Volek J. S., Maresh C. M. (2010). Testosterone physiology in resistance exercise and training: The up-stream regulatory elements. Sports Med. 40, 1037–1053. doi:10.2165/11536910-000000000-00000
Wahl P., Zinner C., Achtzehn S., Bloch W., Mester J. (2010). Effect of high- and low-intensity exercise and metabolic acidosis on levels of GH, IGF-I, IGFBP-3 and cortisol. Growth Horm. IGF Res. 20, 380–385. doi:10.1016/j.ghir.2010.08.001
Wewege M., van den Berg R., Ward R. E., Keech A. (2017). The effects of high-intensity interval training vs. moderate-intensity continuous training on body composition in overweight and obese adults: A systematic review and meta-analysis. Obes. Rev. 18, 635–646. doi:10.1111/obr.12532
Wood K. M., Olive B., LaValle K., Thompson H., Greer K., Astorino T. A. (2016). Dissimilar physiological and perceptual responses between sprint interval training and high-intensity interval training. J. Strength Cond. Res. 30, 244–250. doi:10.1519/JSC.0000000000001042
Keywords: high-intensity interval training, resistance-type HIIT, cardiorespiratory response, heart rate variability, acute physiological response
Citation: Mao J, Wang T, Zhang L, Li Q and Bo S (2022) Comparison of the acute physiological and perceptual responses between resistance-type and cycling high-intensity interval training. Front. Physiol. 13:986920. doi: 10.3389/fphys.2022.986920
Received: 05 July 2022; Accepted: 12 August 2022;
Published: 09 September 2022.
Edited by:
Ahmad Alkhatib, University of Taipei, TaiwanReviewed by:
Todd Anthony Astorino, California State University San Marcos, United StatesCopyright © 2022 Mao, Wang, Zhang, Li and Bo. This is an open-access article distributed under the terms of the Creative Commons Attribution License (CC BY). The use, distribution or reproduction in other forums is permitted, provided the original author(s) and the copyright owner(s) are credited and that the original publication in this journal is cited, in accordance with accepted academic practice. No use, distribution or reproduction is permitted which does not comply with these terms.
*Correspondence: Shumin Bo, Ym9zaHVtaW5AY3VwZXMuZWR1LmNu
Disclaimer: All claims expressed in this article are solely those of the authors and do not necessarily represent those of their affiliated organizations, or those of the publisher, the editors and the reviewers. Any product that may be evaluated in this article or claim that may be made by its manufacturer is not guaranteed or endorsed by the publisher.
Research integrity at Frontiers
Learn more about the work of our research integrity team to safeguard the quality of each article we publish.