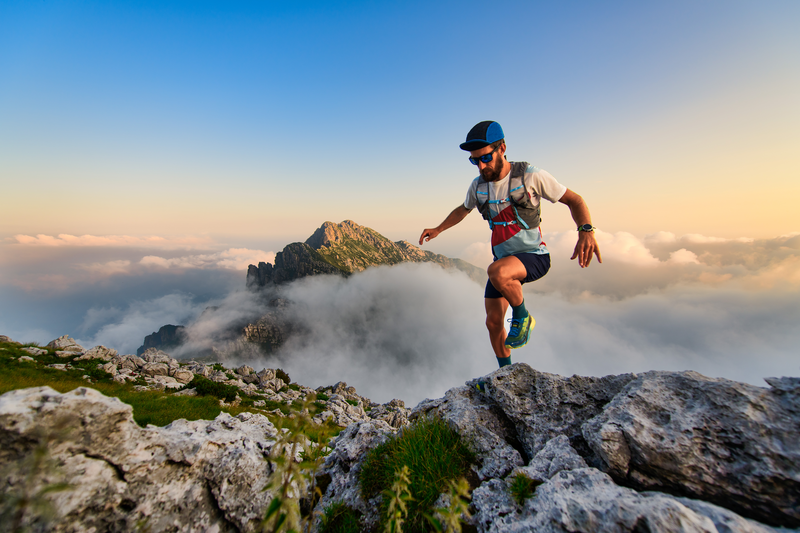
94% of researchers rate our articles as excellent or good
Learn more about the work of our research integrity team to safeguard the quality of each article we publish.
Find out more
REVIEW article
Front. Physiol. , 26 August 2022
Sec. Exercise Physiology
Volume 13 - 2022 | https://doi.org/10.3389/fphys.2022.981577
Ischemic diseases are a major cause of mortality or disability in the clinic. Surgical or medical treatment often has poor effect on patients with tissue and organ ischemia caused by diffuse stenoses. Promoting angiogenesis is undoubtedly an effective method to improve perfusion in ischemic tissues and organs. Although many animal or clinical studies tried to use stem cell transplantation, gene therapy, or cytokines to promote angiogenesis, these methods could not be widely applied in the clinic due to their inconsistent experimental results. However, exercise rehabilitation has been written into many authoritative guidelines in the treatment of ischemic diseases. The function of exercise in promoting angiogenesis relies on the regulation of blood glucose and lipids, as well as cytokines that secreted by skeletal muscle, which are termed as myokines, during exercise. Myokines, such as interleukin-6 (IL-6), chemokine ligand (CXCL) family proteins, irisin, follistatin-like protein 1 (FSTL1), and insulin-like growth factor-1 (IGF-1), have been found to be closely related to the expression and function of angiogenesis-related factors and angiogenesis in both animal and clinical experiments, suggesting that myokines may become a new molecular target to promote angiogenesis and treat ischemic diseases. The aim of this review is to show current research progress regarding the mechanism how exercise and exercise-induced myokines promote angiogenesis. In addition, the limitation and prospect of researches on the roles of exercise-induced myokines in angiogenesis are also discussed. We hope this review could provide theoretical basis for the future mechanism studies and the development of new strategies for treating ischemic diseases.
Ischemic diseases, including ischemic heart disease, ischemic stroke, and peripheral artery disease, are characterized by insufficient perfusion of tissues and organs caused by vascular stenosis or even occlusion. Inflammatory reactions, vasospasms, thromboembolisms, fat embolisms, and uneven blood distribution can all result in ischemic diseases, while atherosclerosis is the most common cause. Hypertension, hyperglycemia, and hyperlipidemia are important factors that promote atherosclerosis. When severe diffuse atherosclerosis occurs in cerebral vessels, coronary arteries, or peripheral arteries, surgical or medical treatment usually shows poor effect and the patients would have a poor prognosis. Exercise rehabilitation therapy can significantly improve the symptoms and prognosis of patients with ischemic stroke, ischemic heart disease, and peripheral artery disease (Treat-Jacobson et al., 2019; MacKay-Lyons et al., 2020; Pelliccia et al., 2021), and has already been used as a treating method along with medication and surgical operation. Skeletal muscle is the main organ involved in exercise, in the meantime, it is also the largest glycogen reserve organ and an important endocrine organ. Skeletal muscle can produce myokines that cause a series of biological effects through autocrine, paracrine, or remote secretion during exercise (Giudice and Taylor, 2017). It is reported that exercise can promote angiogenesis in the skeletal muscle, myocardium, and brain (Morland et al., 2017; Longchamp et al., 2018; Tryfonos et al., 2021). Neovascularization is an important mechanism for exercise to improve the clinical symptoms and prognosis of ischemic diseases. Some studies have shown that this effect of exercise is closely related to myokines. This review focuses on the current research progress of the mechanism underlying exercise-mediated promotion of angiogenesis through myokines (Figure 1).
FIGURE 1. Exercise and exercise-induced myokines in promoting angiogenesis. After exercise, the expression of IL-6, irisin, FSTL1, CXCL1, IGF-1 that promote angiogenesis increased in muscles, while the expression of CXCL10, which inhibits angiogenesis, decreased. These myokines regulate the expression of angiogenesis-related factors, such as VEGF, eNOS/NO and MMP, which play important roles in endothelial cell matrix degradation, endothelial cell migration and lumen formation, and synergistically promote angiogenesis. In addition, myokines also improve hyperglycemia and hyperlipidemia. Together, these effects of myokines promote angiogenesis in brain, heart and muscle tissue. VEGF, vascular endothelial growth factor; eNOS, endothelial nitric oxide synthase; MMP, matrix metalloproteinase (Create with BioRender.com).
In a narrow sense, angiogenesis refers to the process of degradation of the original capillary or microvascular endothelial matrix, the proliferation, migration and differentiation of endothelial cells, formation of a new vascular lumen in the form of budding, synthesis of new extravascular matrix, and the formation of new blood vessels (Diaz-Flores et al., 1994). While in a broader sense, angiogenesis includes the process of expanding, maturing, or forming arterioles containing a muscle layer on the basis of the original vascular lumen. At present, many cytokines regulating angiogenesis have been found, including vascular endothelial growth factor (VEGF), fibroblast growth factor (FGF), angiopoietin 1,2, transforming growth factor-β (TGF-β), erythropoietin, epidermal growth factor (EGF), interleukin-6 (IL-6), matrix metalloproteinase (MMP), and endothelial nitric oxide synthase (eNOS) (Table 1). Among them, VEGF is the most critical cytokine regulating angiogenesis. It participates in many processes of angiogenesis, such as proliferation and migration of endothelial cells, change of vascular permeability, and degeneration of extracellular matrix. MMP also plays an extremely important role in the degradation of collagen around microvessels in the early stage of angiogenesis. The complex formed by cell membrane-MMP and urokinase in cell pseudopodia can adhere to migrating endothelial cells and improve the migration rate and survival rate of endothelial cells (van Hinsbergh et al., 2006). FGF can promote endothelial cell migration, smooth muscle cell proliferation, and the gradual maturation of newly formed vascular lumen. Endothelial cells can synthesize NO under the regulation of eNOS. Under physiological conditions, NO can increase the level of cGMP in cells and affect the mRNA transcription process, and the angiogenic effects of FGF-2 and VEGF are closely related to the NO signaling pathway (Ziche and Morbidelli, 2000; Kivela et al., 2019).
Angiogenesis is an effective method to improve blood perfusion in ischemic tissues and organs, and alleviate ischemic symptoms. Current strategies to promote angiogenesis for treating ischemic diseases (including those under development) include cell therapy, gene therapy, cytokine therapy, and exercise rehabilitation (Losordo and Dimmeler, 2004a, b). Strauer et al. (Strauer et al., 2002) transplanted autologous bone marrow monocytes into the inner wall of blood vessels of myocardial infarction patient through an interventional balloon, and found that these bone marrow monocytes cells can significantly improve perfusion in the ischemic area by promoting angiogenesis. Besides, transplantation of autologous bone marrow stem cells at the ischemic site in patients with peripheral arterial disease can also improve the symptoms caused by ischemia (Tateishi-Yuyama et al., 2002). At present, the targets of gene and cytokine therapy to promote angiogenesis mainly include VEGF and its receptors, FGF family proteins, and HIF-1. Among them, VEGF and FGF have been found to promote myocardial perfusion in phase Ⅰ or phase Ⅱ clinical trials in the treatment of patients with ischemic heart disease (Losordo and Dimmeler, 2004a). Although many animal experiments using gene therapy successfully promoted peripheral angiogenesis, phase Ⅱ or phase Ⅲ randomized controlled clinical trials of gene therapy for peripheral arterial diseases have shown both positive and negative results. Therefore, the application prospect of gene therapy to promote peripheral angiogenesis is unclear yet (Simon et al., 2022). Compared with these above strategies, exercise rehabilitation has been incorporated into many authoritative guidelines as an improvement method for many ischemic diseases (Treat-Jacobson et al., 2019; MacKay-Lyons et al., 2020; Pelliccia et al., 2021). Exercise-mediated improvement of ischemic symptoms is closely related to angiogenesis promotion. The EXCITE clinical trial showed that exercise rehabilitation can promote coronary collateral circulation growth in patients with stable angina pectoris (Mobius-Winkler et al., 2016). The capillary density per unit of skeletal muscle in young men with good exercise practice was significantly higher than that of untrained men (Hermansen and Wachtlova, 1971). After aerobic training, it was found that the levels of VEGF and capillary density in the mouse brain increased significantly (Morland et al., 2017). Therefore, exercise therapy plays an important role in improving the symptoms and prognosis of ischemic diseases, an in-depth discussion of the function and mechanism of exercise in promoting angiogenesis is of great significance for improving the therapeutic effect of exercise therapy, formulating individualized treatment plans, and also discovering new therapeutic targets.
Many studies have reported that the role of exercise in promoting angiogenesis is related to the changes of the internal environment after exercise. For example, hyperlipidemia and hyperglycemia can damage endothelial function, cause vascular endothelial inflammatory reactions, promote atherosclerosis formation, and inhibit angiogenesis. Exercise can significantly increase the metabolic rate of blood lipids and glucose. Clinical observation shows that aerobic training improves the blood lipid levels of patients with hyperlipidemia (Zhao et al., 2021). Obesity induced by a high-fat diet also has a negative effect on angiogenesis. Aerobic exercise can improve obesity and promote the expressions of myokine and VEGF (Shin et al., 2015). Besides, skeletal muscle accounts for the main part of glucose utilization under insulin stimulation, thus plays an important role in blood glucose control and metabolic homeostasis. During muscle contraction, the circulation of glucose transporter four in the cell membrane of skeletal muscle cells is increased, which enhances glucose uptake and reduces blood glucose concentration (Nedachi et al., 2008). In addition, exercise can improve insulin resistance in obese patients and improve glucose utilization in muscle tissue (Coffey and Hawley, 2007).
Except for blood lipid and glucose metabolism, the expression level and distribution of angiogenesis-related cytokines are also regulated by exercise to promote angiogenesis (Bloor, 2005). Gavin et al. (Gavin et al., 2004) showed that the protein level of VEGF in muscle tissue was decreased after exercise, while the protein level of VEGF in the serum and myocardium was increased (Yazdani et al., 2020; Dastah et al., 2021). The difference of VEGF protein level in different tissues after exercise may be due to the increased blood volume in muscle during exercise, which resulted in VEGF protein redistribution. In addition, NO synthesis is also changed significantly during exercise (Dyakova et al., 2015). NO was first considered as an inflammatory factor that regulates vasoconstriction. Later, it was found that NO plays an important role in angiogenesis. NO synthesis is regulated by eNOS, and the effect of NO/eNOS is closely related to VEGF. The protein level of eNOS in the myocardium was increased after exercise, which was correlated with increased expression of VEGF (Iemitsu et al., 2006). The mRNA expression of VEGF receptors (Flt-1 and Flk-1) was increased after exercise, but this increase could be inhibited by NOS inhibitor, indicating that NO may be involved in regulating VEGF expression (Gavin and Wagner, 2002). Lee et al. (Lee et al., 2000) demonstrated that NO promoted endothelial cell migration and differentiation through upregulating αvβ3 integrin expression. If αvβ3 integrin function was inhibited in endothelial cells, even in the presence of VEGF, the downstream signaling pathway of VEGFR2 was damaged, so as the migration and adhesion of endothelial cells (Danilucci et al., 2019). Ranjbar et al. (Ranjbar et al., 2016) showed that the effect of aerobic exercise on myocardial artery neogenesis in rats with myocardial infarction was also correlated with increased TGF-β expression. Aerobic exercise may increase TGF-β expression to promote angiogenesis and protect cardiovascular function. After 8 weeks of aerobic training, the myocardium blood vessel density in diabetic rats increased significantly, and the expression of TGF-β and other cytokines that regulated angiogenesis in the myocardium also increased significantly (Yazdani et al., 2020).
Thus, exercise can promote angiogenesis through systemic changes in the internal environment, including blood lipids and glucose metabolism, as well as the expression of angiogenesis-related cytokines. Besides, the effect of exercise on local metabolism and cytokine synthesis, especially in the skeletal muscle, also plays an important role in promoting angiogenesis.
Myokines are proteins or polypeptides produced by muscle tissue during contraction. The earliest discovered myokine was IL-6. Since then, CXCL family members, irisin, FSTL1, and IGF-1 have been discovered gradually (Gomarasca et al., 2020; Kwon et al., 2020). Myokines can affect the function of muscle and other tissues and organs via autocrine, paracrine, or remote secretion. Myokines maintain the shape and function of muscle cells, promote the repair of damaged muscle cells and the proliferation of skeletal muscle cells through autocrine action. The paracrine effect of myokines plays an important role in exercise-induced skeletal muscle angiogenesis. Besides, myokines can also reach the liver, heart, and other organs through long-distance secretion and participate in the processes of blood glucose and lipid control as well as angiogenesis.
Exercise-induced myokine synthesis is affected by exercise intensity, type of motion, duration, and temperature. Endurance training, resistance exercise, and intermittent high-intensity aerobic exercise can all significantly increase follistatin-like protein 1 (FSTL1) protein concentration in circulation (Kon et al., 2020; Xi et al., 2020). Strength and endurance training can both increase IGF-1 concentration in the circulation of elderly men without difference (Arazi et al., 2021). In obese adolescents, serum IGF-1 concentration is reduced, 4 weeks of moderate exercise can increase the activity but not the concentration of IGF-1 (Yang et al., 2018). Although IL-6 mRNA expression in skeletal muscle of high-fat diet rats is higher than that in the control mice, it could be reduced to a much lower level with aerobic exercise (Gopalan et al., 2021; Shirvani et al., 2021). While for diabetic patients, 6 months of resistance training had no effect on serum IL-6 concentration under the same weight loss condition (Miller et al., 2017). Besides, IL-6 mRNA expression in the muscle of one hind limb of rat at 18 °C was significantly lower than that of the opposite limb at 37 °C, while CXCL1 mRNA expression was higher at 18°C than that at 37°C (Krapf et al., 2021). When human skeletal muscle cells were heated from 18 to 37°C, IL-6 and CXCL1 mRNA expression increased significantly.
So far, how exercise-induced myokine synthesis has not been demonstrated clearly. For example, the changes in NO, protein arginine methyltransferase1 (PRMT1), and histone deacetylase 5(HDAC5) all participate in the regulation of IL-6 expression after exercise. NO inhibitor could reduce exercise-induced IL-6 mRNA expression, while after administration of nitroglycerin, which could increase NO concentration, IL-6 mRNA expression increased significantly, suggesting that NO is involved in the regulation of IL-6 mRNA expression (Steensberg et al., 2007). The effect of NO on IL-6 expression may be attributed to its effect on increasing cGMP concentration. Besides, NF-κB is a transcription factor sensitive to redox reactions. Some studies have shown that NF-κB inhibition reduces the exercise-induced transcription and synthesis of IL-6 (Pedersen and Febbraio, 2008). However, other studies in obese people showed that exercise promotes the expression of IL-6 prior to NF-κB activation (Tantiwong et al., 2010). PRMT1 is an important protease involved in post-transcriptional modification of signaling molecules. It can methylate RelA, resulting in its inability to bind to DNA and consequently affect NF-κB activation (Reintjes et al., 2016). PRMT1 mRNA and protein expression levels are increased significantly after strenuous exercise, but not after long-term exercise (van Lieshout et al., 2019). In PRMT1-deficent mice, IL-6 expression in the liver was decreased, suggesting that PRMT1 is involved in the regulation of IL-6 expression (Zhao et al., 2019). Klymenko et al. (Klymenko et al., 2020) found that HDAC5 can negatively regulate IL-6 gene expression and then affect glucose uptake in skeletal muscle cells. By electrically stimulating C2C12 cells to generate a muscle contraction model, it was found that IL-6 expression in HDAC5-deficient myocytes was higher than that in wild-type cells, suggesting that HDAC5 was also involved in the regulation of exercise-induced IL-6 expression.
Exercise-induced myokines have been found to play important roles in promoting angiogenesis in the skeletal muscle and improving ischemic symptoms in the whole body (Table 2).
IL-6 is first thought to be a proinflammatory cytokine secreted by leukocytes. IL-6 can participate in the angiogenesis of tumor tissue, thus playing an important role in tumorigenesis and development (Ancrile et al., 2007; Lin et al., 2012). The role of IL-6 in promoting angiogenesis in tumor tissues is closely related to VEGF (Ando et al., 2019; Xiao et al., 2021). Similarly, aerobic exercise can promote the expression of IL-6 and VEGF and improve the metabolic status of the whole body in obese people. Except for that, IL-6 is also closely related to metabolic syndrome and insulin resistance (Yousefabadi et al., 2021; El-Toukhy et al., 2022). IL-6 treatment can promote glucose transporter transfer to the cell membrane surface and promote glucose absorption (Carey et al., 2006). Besides, IL-6 can induce glucagon-like peptide-1 expression, thereby improving insulin secretion and blood glucose levels (Ellingsgaard et al., 2011; Ellingsgaard et al., 2020). In obese people, IL-6 also regulates glucose metabolism and insulin release by affecting gluconeogenesis in hepatocytes to affect blood glucose (Knudsen et al., 2016; Peppler et al., 2019). In addition, it has been found that muscle-derived IL-6 improves insulin resistance through activating AMPK and inhibiting the p38/MAPK signaling pathway (Tang et al., 2019). Therefore, aerobic exercise-induced IL-6 may promote angiogenesis through increasing the expression of VEGF and improving the metabolic status of whole body.
CXCL family proteins have been divided into two categories according to their different domains: ELR + CXCL proteins contain a Glu-Leu-Arg sequence at the N-terminal, while ELR- CXCL proteins do not. The former includes CXCL1-3 and CXCL5-8, which binding to CXCR1 and/or CXCR2 to promote angiogenesis. The latter include CXCL4, CXCL4L1, and CXCL9-14, which can bind to CXCR3, CXCR4, CXCR5, or CXCR7 to exert an anti-angiogenic effect (Lee et al., 2018). Exercise can increase CXCL1 mRNA and protein levels in muscles earlier than IL-6, suggesting that CXCL1 may promote angiogenesis by regulating the expression of IL-6 (Pedersen et al., 2011). CXCL10, also known as interferon γ-inducible protein, is secreted by monocytes and endothelial cells and can regulate chemotaxis, cell proliferation and apoptosis (Sui et al., 2004). Animal experiments show that skeletal muscle can inhibit CXCL10 expression during contraction, and p38/MAPK signaling pathway may be involved in this regulatory process; cell experiments show that CXCL10 can inhibit endothelial cell activity (Ishiuchi et al., 2018). In the high-fat diet-induced obesity mouse model, CXCL10 expression in skeletal muscle is increased, and the capillary density is decreased, while after aerobic training, CXCL10 expression is reduced, and the capillary density is increased (Ishiuchi-Sato et al., 2020). Therefore, exercise-induced increased expression of CXCL1 and decreased expression of CXCL10 in muscles may play a synergistically role in promoting angiogenesis, however, the mechanism how they regulate angiogenesis remain largely unknown.
Irisin is derived from fibronectin III domain protein 5, which is regulated by the transcription factor PGC1α (Bostrom et al., 2012). The function of irisin is closely related to metabolism. In patients with gestational diabetes mellitus, the blood irisin level is significantly higher compared to healthy people, and is positively correlated with fasting insulin level (Ebert et al., 2014). Prenatal exercise can induce irisin production and promote blood glucose homeostasis and improve the metabolic disorders caused by pregnancy (Szumilewicz et al., 2017). Patients with polycystic ovary syndrome also show higher levels of irisin in serum and follicular fluid than healthy people, which is positively correlated with insulin resistance (Bousmpoula et al., 2019). Besides, a positive correlation between irisin level and islet resistance also exists in obese people. Although comprehensive intervention and weight control could not change irisin level, obese people with high irisin level showed better improvement in body fat rate, blood glucose, and triglyceride than obese people with low irisin level (Fukushima et al., 2016). However, in patients with type I diabetes, the plasma irisin level is significantly lower than that in healthy people (Tentolouris et al., 2018), thus irisin is expected to be a new treatment for diabetes (Leung, 2017). This suggested that irisin might also indirectly improve the symptoms of ischemic disease and promote angiogenesis through improving metabolism.
Indeed, irisin can repair human umbilical vein endothelial cell (HUVEC) injury caused by high glucose through the Notch signaling pathway, reduce the expression of apoptosis-related proteins and increase the expression of VEGFA in HUVECs (Wang et al., 2021). In lipoprotein-injured HUVEC, irisin promotes angiogenesis through the Akt/mTOR/S6K1/Nrf2 signaling pathway (Zhang and Cao, 2019).Wu et al. (Wu et al., 2015) also confirm that irisin increases the expression of MMP2 and MMP9 through the ERK signaling pathway and promotes angiogenesis in HUVECs and zebrafish embryos. In the myocardial infarction animal model, irisin treatment also shows myocardial protection, and better angiogenesis at the edge of infarction (Liao et al., 2019). Moreover, irisin can also stimulate adipocyte secretion of CXCL1 and improve endothelial cell adhesion (Shaw et al., 2021). Together, these results suggest that irisin has strong application value in promoting angiogenesis and improving the symptoms of ischemic diseases.
FSTL1, also known as TSC-36 or FRP, is a secretory glycoprotein produced by mesenchymal tissue cells (Chaly et al., 2014). It was first considered as a pro-inflammatory protein molecule induced by TGF-β and can induce IL-6 synthesis (Shibanuma et al., 1993; Miyamae et al., 2006). FSTL1 is expressed in many cells and tissues, animals lacking the FSTL1 gene die soon after birth (Geng et al., 2011). FSTL1 plays an important role in postnatal pulmonary vessel development, deficiency of FSTL1 in mouse endothelial cells affects pulmonary vessel remodeling (Tania et al., 2017). FSTL1 also participates in regulating metabolism, and its level is closely related to insulin resistance. In obese and diabetic patients, FSTL1 circulating level is significantly higher compared to healthy people. While FSTL1 expression is decreased significantly in hyperinsulinemia even with normal blood glucose (Xu et al., 2020). FSTL1 has been found to play an important role in promoting angiogenesis in tumor metastasis and in some ischemic diseases. Muscle-derived FSTL1 can induce cardiac angiogenesis in rats with myocardial infarction by regulating the DIP2A-SMAD2/3 signaling pathway (Xi et al., 2020). FSTL1 improves vascular endothelial cell function in ischemic tissue by affecting eNOS phosphorylation, further promoting angiogenesis (Ouchi et al., 2008). FSTL1 also regulates the gene expression of MMP1, MMP3, and MMP13 (Ni et al., 2019). Deficiency of FSTL1 decreases VEGF, α-SMA and other genes related to extracellular matrix synthesis in HUVECs, while overexpression of FSTL1 increases the expression of these genes (Niu et al., 2021). These results suggest that FSTL1 is closely related to extracellular matrix synthesis and degradation. Besides, deficiency of HDAC5 in endothelial cells of patients with Scleroderma increases FSTL1 expression and improves the ability of endothelial cells to form tubules in Matrigel, suggesting that FSTL1-mediated promotion of angiogenesis is dependent on HDAC5 (Tsou et al., 2016). Therefore, FSTL1 may be critical in promoting angiogenesis in ischemic diseases by regulating blood glucose, VEGF, and cell matrix synthesis, which can all improve the symptoms and prognosis of ischemic diseases.
IGF-1 consists of four subunits: A, B, C, and D. The structures of subunits A and B are similar to that of insulin, the function of subunit C is different from that of insulin, and insulin does not have subunit D. IGF-1 can promote cell proliferation and angiogenesis and resist apoptosis (Duan et al., 2010). Although IGF-1 secretion is increased during high-intensity of aerobic training, the serum concentration of its ligand remained unchanged (Nishida et al., 2010). Clinical observation shows that IGF-1 expression level is closely related to the degree of retinal angiogenesis in diabetic patients (Hellstrom et al., 2002). In the isoproterenol-induced myocardial infarction model, IGF-1 promotes angiogenesis through inducing IL-8 expression (Haleagrahara et al., 2011). IGF-1 treatment in rats with myocardial infarction can promote VEGF expression and improve the degree of myocardial ischemia (Lisa et al., 2011). Exercise and IGF-1 supplementation can reduce the degree of diabetes-induced neuropathy and increase the expression of VEGF-A and angiogenesis (Saboory et al., 2020). In the mouse model of subclinical muscle injury, IGF-1 alone could not significantly increase angiogenesis, but IGF-1 combined with exercise could increase muscle capillary density and promote muscle regeneration (Alcazar et al., 2020). These above studies show that the angiogenesis-promoting effect of IGF-1 is closely related to VEGF expression, and IGF-1 supplementation can enhance the exercise-mediated promotion of angiogenesis.
Although myokines have been found to promote angiogenesis, current studies regarding the detailed mechanism how exercise-induced myokines promote angiogenesis and improve the symptoms of ischemic diseases remain inadequate. First, glucose and lipid metabolism, cytokines are closely related to the angiogenesis, thus myokines that could regulate these factors are thought to have angiogenesis-promoting functions. However, the direct evidence that demonstrate the relationship between myokines and angiogenesis during exercise are missing. The molecular mechanism, such as the direct target or receptors and the downstream signaling of myokines in promoting angiogenesis need to be discovered in the future. Second, both animal and clinical research data on treating ischemic diseases using exercise or myokines are insufficient probably due to lack of effective indicators of angiogenesis except for metabolic markers and related cytokines. Although some studies have used exercise or myokines in different diseases and obtain positive results, the effect of disease severity, exercise types and the level of myokines on angiogenesis need to be demonstrated much more clearly. Third, some myokines, like interleukins and chemokines, are also proinflammatory cytokines which need to be taken into account carefully because inflammation also contribute to the development of ischemic diseases. Although their expressions might also be correlated with the level of metabolic status or even angiogenesis-related cytokines, it is hard to define the main function of these myokines in the internal environment. Therefore, future studies should clarify the effect of exercise or myokines on those intracellular and extracellular factors that directly promote angiogenesis in depth.
Promoting angiogenesis is undoubtedly an effective method to improve tissue and organ perfusion, especially for ischemic diseases that cannot be treated through surgery. The benefit and safety of exercise rehabilitation for patients with ischemic diseases have been widely recognized. During exercise, the expression of myokines that promote angiogenesis increased, including IL-6, irisin, FSTL1, CXCL1, IGF-1, while the expression of CXCL10, which inhibits angiogenesis, decreased. These myokines regulate angiogenesis-related factors, such as VEGF, NOS and MMP, which play important roles in endothelial cell matrix degradation, endothelial cell migration, and lumen formation, and synergistically promote angiogenesis. In addition, the improvement on hyperglycemia and hyperlipidemia by exercise also provides a favorable internal environment for angiogenesis.
Currently, the effect of surgical treatment is often poor for ischemic diseases caused by diffuse vascular diseases. Medical treatment mainly involves the regulation of blood glucose, blood lipids, and anti-atherosclerosis, as well as thrombosis prevention. However, there is no medication that can directly promote angiogenesis. Some clinical studies have confirmed that exercise rehabilitation is a safe and effective treatment for ischemic heart disease, stroke, and peripheral vascular diseases. Due to the promising effect of exercise rehabilitation on angiogenesis and tissue perfusion, its value in treating ischemic diseases will draw more and more attention in the future, especially in ischemic diseases caused by diffuse vascular diseases. Myokines generated during exercise have also shown their potential in promoting angiogenesis in vivo and are expected to be a new molecular target for the treatment of ischemic diseases. Moreover, the metabolic syndrome improvement effect of myokines can make it benefit a much more broader patients with obesity and diabetes. Therefore, it is worthy to carry on more detailed studies on the function and mechanisms of exercise and myokines in promoting angiogenesis. Future study could focus on: 1) How exercise induced myokine expressions in muscle cells, the effect of contraction pathway on epigenetic changes of myokine-related genes; 2) The direct function of myokines on angiogenesis, the receptor and downstream signaling of myokines on vascular endothelial cells, as well as the effect on organ angiogenesis using in vivo dynamic visual evidence; 3) The clinical data about the change of myokines before and after exercise in patients with ischemic diseases, the effect of exercise on angiogenesis in ischemic organs need to be systematically collected and carefully analyzed with the exercise type, disease severity and other disease history taken into account.
BL conceived this review, organized and revised the manuscript. CQ did major work of writing the manuscript. XS, HW, and YY made contributions to writing the manuscript. CQ, XS, HW, YY, and BL gave final approval of the version to be published.
This work was supported by grants from the Finance Department of Jilin Province, China [No.2019SCZT004], the Science and Technology Department of Jilin Province, China [No.20190905002SF], and National Key R and D Program of China [No.2016YFC1301100].
The authors declare that the research was conducted in the absence of any commercial or financial relationships that could be construed as a potential conflict of interest.
All claims expressed in this article are solely those of the authors and do not necessarily represent those of their affiliated organizations, or those of the publisher, the editors and the reviewers. Any product that may be evaluated in this article, or claim that may be made by its manufacturer, is not guaranteed or endorsed by the publisher.
Alcazar C. A., Hu C., Rando T. A., Huang N. F., Nakayama K. H. (2020). Transplantation of insulin-like growth factor-1 laden scaffolds combined with exercise promotes neuroregeneration and angiogenesis in a preclinical muscle injury model. Biomater. Sci. 8, 5376–5389. doi:10.1039/d0bm00990c
Ancrile B., Lim K. H., Counter C. M. (2007). Oncogenic Ras-induced secretion of IL6 is required for tumorigenesis. Genes. Dev. 21, 1714–1719. doi:10.1101/gad.1549407
Ando N., Hara M., Shiga K., Yanagita T., Takasu K., Nakai N., et al. (2019). Eicosapentaenoic acid suppresses angiogenesis via reducing secretion of IL6 and VEGF from colon cancerassociated fibroblasts. Oncol. Rep. 42, 339–349. doi:10.3892/or.2019.7141
Arazi H., Babaei P., Moghimi M., Asadi A. (2021). Acute effects of strength and endurance exercise on serum BDNF and IGF-1 levels in older men. BMC Geriatr. 21, 50. doi:10.1186/s12877-020-01937-6
Bloor C. M. (2005). Angiogenesis during exercise and training. Angiogenesis 8, 263–271. doi:10.1007/s10456-005-9013-x
Bostrom P., Wu J., Jedrychowski M. P., Korde A., Ye L., Lo J. C., et al. (2012). A PGC1-alpha-dependent myokine that drives brown-fat-like development of white fat and thermogenesis. Nature 481, 463–468. doi:10.1038/nature10777
Bousmpoula A., Benidis E., Demeridou S., Kapeta-Kourkouli R., Chasiakou A., Chasiakou S., et al. (2019). Serum and follicular fluid irisin levels in women with polycystic ovaries undergoing ovarian stimulation: Correlation with insulin resistance and lipoprotein lipid profiles. Gynecol. Endocrinol. 35, 803–806. doi:10.1080/09513590.2019.1594761
Carey A. L., Steinberg G. R., Macaulay S. L., Thomas W. G., Holmes A. G., Ramm G., et al. (2006). Interleukin-6 increases insulin-stimulated glucose disposal in humans and glucose uptake and fatty acid oxidation in vitro via AMP-activated protein kinase. Diabetes 55, 2688–2697. doi:10.2337/db05-1404
Chaly Y., Hostager B., Smith S., Hirsch R. (2014). Follistatin-like protein 1 and its role in inflammation and inflammatory diseases. Immunol. Res. 59, 266–272. doi:10.1007/s12026-014-8526-z
Coffey V. G., Hawley J. A. (2007). The molecular bases of training adaptation. Sports Med. 37, 737–763. doi:10.2165/00007256-200737090-00001
Danilucci T. M., Santos P. K., Pachane B. C., Pisani G. F. D., Lino R. L. B., Casali B. C., et al. (2019). Recombinant RGD-disintegrin DisBa-01 blocks integrin αvβ3 and impairs VEGF signaling in endothelial cells. Cell. Commun. Signal. 17, 27. doi:10.1186/s12964-019-0339-1
Dastah S., Tofighi A., Bonab S. B. (2021). The effect of aerobic exercise on the expression of mir-126 and related target genes in the endothelial tissue of the cardiac muscle of diabetic rats. Microvasc. Res. 138, 104212. doi:10.1016/j.mvr.2021.104212
Diaz-Flores L., Gutierrez R., Varela H. (1994). Angiogenesis: An update. Histol. Histopathol. 9, 807–843.
Duan C., Ren H., Gao S. (2010). Insulin-like growth factors (IGFs), IGF receptors, and IGF-binding proteins: Roles in skeletal muscle growth and differentiation. Gen. Comp. Endocrinol. 167, 344–351. doi:10.1016/j.ygcen.2010.04.009
Dyakova E. Y., Kapilevich L. V., Shylko V. G., Popov S. V., Anfinogenova Y. (2015). Physical exercise associated with NO production: Signaling pathways and significance in health and disease. Front. Cell. Dev. Biol. 3, 19. doi:10.3389/fcell.2015.00019
Ebert T., Stepan H., Schrey S., Kralisch S., Hindricks J., Hopf L., et al. (2014). Serum levels of irisin in gestational diabetes mellitus during pregnancy and after delivery. Cytokine 65, 153–158. doi:10.1016/j.cyto.2013.11.009
El-Toukhy S. E., Ashour M. N., Mohamed N. A., Gouhar S. A., Nazeeh N. N., Youness E. R. (2022). Potential role of novel myokine in rats-induced metabolic syndrome. Biointerface Res. App 12, 3305–3315.
Ellingsgaard H., Hauselmann I., Schuler B., Habib A. M., Baggio L. L., Meier D. T., et al. (2011). Interleukin-6 enhances insulin secretion by increasing glucagon-like peptide-1 secretion from L cells and alpha cells. Nat. Med. 17, 1481–1489. doi:10.1038/nm.2513
Ellingsgaard H., Seelig E., Timper K., Coslovsky M., Soederlund L., Lyngbaek M. P., et al. (2020). GLP-1 secretion is regulated by IL-6 signalling: A randomised, placebo-controlled study. Diabetologia 63, 362–373. doi:10.1007/s00125-019-05045-y
Fukushima Y., Kurose S., Shinno H., Thi Thu H. C., Takao N., Tsutsumi H., et al. (2016). Effects of body weight reduction on serum irisin and metabolic parameters in obese subjects. Diabetes Metab. J. 40, 386–395. doi:10.4093/dmj.2016.40.5.386
Gavin T. P., Robinson C. B., Yeager R. C., England J. A., Nifong L. W., Hickner R. C. (2004). Angiogenic growth factor response to acute systemic exercise in human skeletal muscle. J. Appl. Physiol. 96 (1985), 19–24. doi:10.1152/japplphysiol.00748.2003
Gavin T. P., Wagner P. D. (2002). Attenuation of the exercise-induced increase in skeletal muscle Flt-1 mRNA by nitric oxide synthase inhibition. Acta Physiol. Scand. 175, 201–209. doi:10.1046/j.1365-201X.2002.00987.x
Geng Y., Dong Y., Yu M., Zhang L., Yan X., Sun J., et al. (2011). Follistatin-like 1 (Fstl1) is a bone morphogenetic protein (BMP) 4 signaling antagonist in controlling mouse lung development. Proc. Natl. Acad. Sci. U. S. A. 108, 7058–7063. doi:10.1073/pnas.1007293108
Giudice J., Taylor J. M. (2017). Muscle as a paracrine and endocrine organ. Curr. Opin. Pharmacol. 34, 49–55. doi:10.1016/j.coph.2017.05.005
Gomarasca M., Banfi G., Lombardi G. (2020). Myokines: The endocrine coupling of skeletal muscle and bone. Adv. Clin. Chem. 94 94, 155–218. doi:10.1016/bs.acc.2019.07.010
Gopalan V., Yaligar J., Michael N., Kaur K., Anantharaj R., Verma S. K., et al. (2021). A 12-week aerobic exercise intervention results in improved metabolic function and lower adipose tissue and ectopic fat in high-fat diet fed rats. Biosci. Rep. 41, BSR20201707. doi:10.1042/BSR20201707
Haleagrahara N., Chakravarthi S., Mathews L. (2011). Insulin like growth factor-1 (IGF-1)Causes overproduction of IL-8, an angiogenic cytokine and stimulates neovascularization in isoproterenol-induced myocardial infarction in rats. Int. J. Mol. Sci. 12, 8562–8574. doi:10.3390/ijms12128562
Hellstrom A., Carlsson B., Niklasson A., Segnestam K., Boguszewski M., De Lacerda L., et al. (2002). IGF-I is critical for normal vascularization of the human retina. J. Clin. Endocrinol. Metab. 87, 3413–3416. doi:10.1210/jcem.87.7.8629
Hermansen L., Wachtlova M. (1971). Capillary density of skeletal muscle in well-trained and untrained men. J. Appl. Physiol. 30, 860–863. doi:10.1152/jappl.1971.30.6.860
Iemitsu M., Maeda S., Jesmin S., Otsuki T., Miyauchi T. (2006). Exercise training improves aging-induced downregulation of VEGF angiogenic signaling cascade in hearts. Am. J. Physiol. Heart Circ. Physiol. 291, H1290–H1298. doi:10.1152/ajpheart.00820.2005
Ishiuchi Y., Sato H., Tsujimura K., Kawaguchi H., Matsuwaki T., Yamanouchi K., et al. (2018). Skeletal muscle cell contraction reduces a novel myokine, chemokine (C-X-C motif) ligand 10 (CXCL10): Potential roles in exercise-regulated angiogenesis. Biosci. Biotechnol. Biochem. 82, 97–105. doi:10.1080/09168451.2017.1411778
Ishiuchi-Sato Y., Hiraiwa E., Shinozaki A., Nedachi T. (2020). The effects of glucose and fatty acids on CXCL10 expression in skeletal muscle cells. Biosci. Biotechnol. Biochem. 84, 2448–2457. doi:10.1080/09168451.2020.1814127
Kivela R., Hemanthakumar K. A., Vaparanta K., Robciuc M., Izumiya Y., Kidoya H., et al. (2019). Endothelial cells regulate physiological cardiomyocyte growth via VEGFR2-mediated paracrine signaling. Circulation 139, 2570–2584. doi:10.1161/CIRCULATIONAHA.118.036099
Klymenko O., Brecklinghaus T., Dille M., Springer C., de Wendt C., Altenhofen D., et al. (2020). Histone deacetylase 5 regulates interleukin 6 secretion and insulin action in skeletal muscle. Mol. Metab. 42, 101062. doi:10.1016/j.molmet.2020.101062
Knudsen J. G., Joensen E., Bertholdt L., Jessen H., van Hauen L., Hidalgo J., et al. (2016). Skeletal muscle IL-6 and regulation of liver metabolism during high-fat diet and exercise training. Physiol. Rep. 4, e12788. doi:10.14814/phy2.12788
Kon M., Tanimura Y., Yoshizato H. (2020). Effects of acute endurance exercise on follistatin-like 1 and apelin in the circulation and metabolic organs in rats. Arch. Physiol. Biochem., 1–5. doi:10.1080/13813455.2020.1764050
Krapf S., Schjolberg T., Asoawe L., Honkanen S. K., Kase E. T., Thoresen G. H., et al. (2021). Novel methods for cold exposure of skeletal muscle in vivo and in vitro show temperature-dependent myokine production. J. Therm. Biol. 98, 102930. doi:10.1016/j.jtherbio.2021.102930
Kwon J. H., Moon K. M., Min K. W. (2020). Exercise-induced myokines can explain the importance of physical activity in the elderly: An overview. Healthc. (Basel) 8, E378. doi:10.3390/healthcare8040378
Lee N. H., Nikfarjam M., He H. (2018). Functions of the CXC ligand family in the pancreatic tumor microenvironment. Pancreatology 18, 705–716. doi:10.1016/j.pan.2018.07.011
Lee P. C., Kibbe M. R., Schuchert M. J., Stolz D. B., Watkins S. C., Griffith B. P., et al. (2000). Nitric oxide induces angiogenesis and upregulates alpha(v)beta(3) integrin expression on endothelial cells. Microvasc. Res. 60, 269–280. doi:10.1006/mvre.2000.2265
Leung P. S. (2017). The potential of irisin as a therapeutic for diabetes. Future Med. Chem. 9, 529–532. doi:10.4155/fmc-2017-0035
Liao Q., Qu S., Tang L. X., Li L. P., He D. F., Zeng C. Y., et al. (2019). Irisin exerts a therapeutic effect against myocardial infarction via promoting angiogenesis. Acta Pharmacol. Sin. 40, 1314–1321. doi:10.1038/s41401-019-0230-z
Lin Z. Y., Chuang Y. H., Chuang W. L. (2012). Cancer-associated fibroblasts up-regulate CCL2, CCL26, IL6 and LOXL2 genes related to promotion of cancer progression in hepatocellular carcinoma cells. Biomed. Pharmacother. 66, 525–529. doi:10.1016/j.biopha.2012.02.001
Lisa M., Haleagrahara N., Chakravarthi S. (2011). Insulin-Like Growth Factor-1 (IGF-1) Reduces ischemic changes and increases circulating angiogenic factors in experimentally - induced myocardial infarction in rats. Vasc. Cell. 3, 13. doi:10.1186/2045-824X-3-13
Longchamp A., Mirabella T., Arduini A., MacArthur M. R., Das A., Trevino-Villarreal J. H., et al. (2018). Amino acid restriction triggers angiogenesis via GCN2/ATF4 regulation of VEGF and H2S production. Cell. 173, 117–129. doi:10.1016/j.cell.2018.03.001
Losordo D. W., Dimmeler S. (2004b). Therapeutic angiogenesis and vasculogenesis for ischemic disease: Part II: Cell-based therapies. Circulation 109, 2692–2697. doi:10.1161/01.CIR.0000128596.49339.05
Losordo D. W., Dimmeler S. (2004a). Therapeutic angiogenesis and vasculogenesis for ischemic disease. Part I: Angiogenic cytokines. Circulation 109, 2487–2491. doi:10.1161/01.CIR.0000128595.79378.FA
MacKay-Lyons M., Billinger S. A., Eng J. J., Dromerick A., Giacomantonio N., Hafer-Macko C., et al. (2020). Aerobic exercise recommendations to optimize best practices in care after stroke: AEROBICS 2019 update. Phys. Ther. 100, 149–156. doi:10.1093/ptj/pzz153
Miller E. G., Sethi P., Nowson C. A., Dunstan D. W., Daly R. M. (2017). Effects of progressive resistance training and weight loss versus weight loss alone on inflammatory and endothelial biomarkers in older adults with type 2 diabetes. Eur. J. Appl. Physiol. 117, 1669–1678. doi:10.1007/s00421-017-3657-2
Miyamae T., Marinov A. D., Sowders D., Wilson D. C., Devlin J., Boudreau R., et al. (2006). Follistatin-like protein-1 is a novel proinflammatory molecule. J. Immunol. 177, 4758–4762. doi:10.4049/jimmunol.177.7.4758
Mobius-Winkler S., Uhlemann M., Adams V., Sandri M., Erbs S., Lenk K., et al. (2016). Coronary collateral growth induced by physical exercise: Results of the impact of intensive exercise training on coronary collateral circulation in patients with stable coronary artery disease (EXCITE) trial. Circulation 133, 1438–1448. doi:10.1161/CIRCULATIONAHA.115.016442
Morland C., Andersson K. A., Haugen O. P., Hadzic A., Kleppa L., Gille A., et al. (2017). Exercise induces cerebral VEGF and angiogenesis via the lactate receptor HCAR1. Nat. Commun. 8, 15557. doi:10.1038/ncomms15557
Nedachi T., Fujita H., Kanzaki M. (2008). Contractile C2C12 myotube model for studying exercise-inducible responses in skeletal muscle. Am. J. Physiol. Endocrinol. Metab. 295, E1191–E1204. doi:10.1152/ajpendo.90280.2008
Ni S., Li C. K., Xu N. W., Liu X., Wang W., Chen W. Y., et al. (2019). Follistatin-like protein 1 induction of matrix metalloproteinase 1, 3 and 13 gene expression in rheumatoid arthritis synoviocytes requires MAPK, JAK/STAT3 and NF-kappa B pathways. J. Cell. Physiol. 234, 454–463. doi:10.1002/jcp.26580
Nishida Y., Matsubara T., Tobina T., Shindo M., Tokuyama K., Tanaka K., et al. (2010). Effect of low-intensity aerobic exercise on insulin-like growth factor-I and insulin-like growth factor-binding proteins in healthy men. Int. J. Endocrinol. 2010, 452820. doi:10.1155/2010/452820
Niu R., Nie Z. T., Liu L., Chang Y. W., Shen J. Q., Chen Q., et al. (2021)., 13. Albany NY, 8643–8664. doi:10.18632/aging.202678Follistatin-like protein 1 functions as a potential target of gene therapy in proliferative diabetic retinopathy Aging.
Ouchi N., Oshima Y., Ohashi K., Higuchi A., Ikegami C., Izumiya Y., et al. (2008). Follistatin-like 1, a secreted muscle protein, promotes endothelial cell function and revascularization in ischemic tissue through a nitric-oxide synthase-dependent mechanism. J. Biol. Chem. 283, 32802–32811. doi:10.1074/jbc.M803440200
Pedersen B. K., Febbraio M. A. (2008). Muscle as an endocrine organ: Focus on muscle-derived interleukin-6. Physiol. Rev. 88, 1379–1406. doi:10.1152/physrev.90100.2007
Pedersen L., Pilegaard H., Hansen J., Brandt C., Adser H., Hidalgo J., et al. (2011). Exercise-induced liver chemokine CXCL-1 expression is linked to muscle-derived interleukin-6 expression. J. Physiol. 589, 1409–1420. doi:10.1113/jphysiol.2010.200733
Pelliccia A., Sharma S., Gati S., Back M., Borjesson M., Caselli S., et al. (2021). 2020 ESC Guidelines on sports cardiology and exercise in patients with cardiovascular disease. Eur. Heart J. 42, 17–96. doi:10.1093/eurheartj/ehaa605
Peppler W. T., Townsend L. K., Meers G. M., Panasevich M. R., MacPherson R. E. K., Rector R. S., et al. (2019). Acute administration of IL-6 improves indices of hepatic glucose and insulin homeostasis in lean and obese mice. Am. J. Physiol. Gastrointest. Liver Physiol. 316, G166–G178. doi:10.1152/ajpgi.00097.2018
Ranjbar K., Rahmani-Nia F., Shahabpour E. (2016). Aerobic training and l-arginine supplementation promotes rat heart and hindleg muscles arteriogenesis after myocardial infarction. J. Physiol. Biochem. 72, 393–404. doi:10.1007/s13105-016-0480-x
Reintjes A., Fuchs J. E., Kremser L., Lindner H. H., Liedl K. R., Huber L. A., et al. (2016). Asymmetric arginine dimethylation of RelA provides a repressive mark to modulate TNFα/NF-κB response. Proc. Natl. Acad. Sci. U. S. A. 113, 4326–4331. doi:10.1073/pnas.1522372113
Saboory E., Gholizadeh-Ghaleh Aziz S., Samadi M., Biabanghard A., Chodari L. (2020). Exercise and insulin-like growth factor 1 supplementation improve angiogenesis and angiogenic cytokines in a rat model of diabetes-induced neuropathy. Exp. Physiol. 105, 783–792. doi:10.1113/EP088069
Shaw A., Toth B. B., Kiraly R., Arianti R., Csomos I., Poliska S., et al. (2021). Irisin stimulates the release of CXCL1 from differentiating human subcutaneous and deep-neck derived adipocytes via upregulation of NF kappa B pathway. Front. Cell. Dev. Biol. 9, 737872. doi:10.3389/fcell.2021.737872
Shibanuma M., Mashimo J., Mita A., Kuroki T., Nose K. (1993). Cloning from a mouse osteoblastic cell line of a set of transforming-growth-factor-beta 1-regulated genes, one of which seems to encode a follistatin-related polypeptide. Eur. J. Biochem. 217, 13–19. doi:10.1111/j.1432-1033.1993.tb18212.x
Shin K. O., Bae J. Y., Woo J., Jang K. S., Kim K. S., Park J. S., et al. (2015). The effect of exercise on expression of myokine and angiogenesis mRNA in skeletal muscle of high fat diet induced obese rat. J. Exerc. Nutr. Biochem. 19, 91–98. doi:10.5717/jenb.2015.15061006
Shirvani H., Mirnejad R., Soleimani M., Arabzadeh E. (2021). Swimming exercise improves gene expression of PPAR-gamma and downregulates the overexpression of TLR4, MyD88, IL-6, and TNF-alpha after high-fat diet in rat skeletal muscle cells. Gene 775, 145441. doi:10.1016/j.gene.2021.145441
Simon F., Duran M., Garabet W., Schelzig H., Jacobs M., Gombert A. (2022). Gene therapy of chronic limb-threatening ischemia: Vascular medical perspectives. J. Clin. Med. 11, 1282. doi:10.3390/jcm11051282
Steensberg A., Keller C., Hillig T., Frosig C., Wojtaszewski J. F. P., Pedersen B. K., et al. (2007). Nitric oxide production is a proximal signaling event controlling exercise-induced mRNA expression in human skeletal muscle. Faseb J. 21, 2683–2694. doi:10.1096/fj.06-7477com
Strauer B. E., Brehm M., Zeus T., Kostering M., Hernandez A., Sorg R. V., et al. (2002). Repair of infarcted myocardium by autologous intracoronary mononuclear bone marrow cell transplantation in humans. Circulation 106, 1913–1918. doi:10.1161/01.cir.0000034046.87607.1c
Sui Y. J., Potula R., Dhillon N., Pinson D., Li S. P., Nath A., et al. (2004). Neuronal apoptosis is mediated by CXCL10 overexpression in simian human immunodeficiency virus encephalitis. Am. J. Pathol. 164, 1557–1566. doi:10.1016/S0002-9440(10)63714-5
Szumilewicz A., Worska A., Piernicka M., Kuchta A., Kortas J., Jastrzebski Z., et al. (2017). The exercise-induced irisin is associated with improved levels of glucose homeostasis markers in pregnant women participating in 8-week prenatal group fitness Program: A pilot study. Biomed. Res. Int. 2017, 9414525. doi:10.1155/2017/9414525
Tang H., Deng S., Cai J. G., Ma X. N., Liu M., Zhou L. (2019). Muscle-derived IL-6 improved insulin resistance of C2C12 cells through activating AMPK and inhibiting p38MAPK signal pathway in vitro. Int. J. Diabetes Dev. Ctries. 39, 486–498. doi:10.1007/s13410-018-0680-8
Tania N. P., Maarsingh H., Is T. B., Mattiotti A., Prakash S., Timens W., et al. (2017). Endothelial follistatin-like-1 regulates the postnatal development of the pulmonary vasculature by modulating BMP/Smad signaling. Pulm. Circ. 7, 219–231. doi:10.1177/2045893217702340
Tantiwong P., Shanmugasundaram K., Monroy A., Ghosh S., Li M., DeFronzo R. A., et al. (2010). NF-κB activity in muscle from obese and type 2 diabetic subjects under basal and exercise-stimulated conditions. Am. J. Physiol. Endocrinol. Metab. 299, E794–E801. doi:10.1152/ajpendo.00776.2009
Tateishi-Yuyama E., Matsubara H., Murohara T., Ikeda U., Shintani S., Masaki H., et al. (2002). Therapeutic angiogenesis for patients with limb ischaemia by autologous transplantation of bone-marrow cells: A pilot study and a randomised controlled trial. Lancet 360, 427–435. doi:10.1016/S0140-6736(02)09670-8
Tentolouris A., Eleftheriadou I., Tsilingiris D., Anastasiou I. A., Kosta O. A., Mourouzis I., et al. (2018). Plasma irisin levels in subjects with type 1 diabetes: Comparison with healthy controls. Horm. Metab. Res. 50, 803–810. doi:10.1055/a-0748-6170
Treat-Jacobson D., McDermott M. M., Bronas U. G., Campia U., Collins T. C., Criqui M. H., et al. (2019). Optimal exercise programs for patients with peripheral artery disease: A scientific statement from the American heart association. Circulation 139, e10–e33. doi:10.1161/CIR.0000000000000623
Tryfonos A., Tzanis G., Pitsolis T., Karatzanos E., Koutsilieris M., Nanas S., et al. (2021). Exercise training enhances angiogenesis-related gene responses in skeletal muscle of patients with chronic heart failure. Cells 10 (8), 1915. doi:10.3390/cells10081915
Tsou P. S., Wren J. D., Amin M. A., Schiopu E., Fox D. A., Khanna D., et al. (2016). Histone deacetylase 5 is overexpressed in Scleroderma endothelial cells and impairs angiogenesis via repression of proangiogenic factors. Arthritis Rheumatol. 68, 2975–2985. doi:10.1002/art.39828
van Hinsbergh V. W., Engelse M. A., Quax P. H. (2006). Pericellular proteases in angiogenesis and vasculogenesis. Arterioscler. Thromb. Vasc. Biol. 26, 716–728. doi:10.1161/01.ATV.0000209518.58252.17
vanLieshout T. L., Bonafiglia J. T., Gurd B. J., Ljubicic V. (2019). Protein arginine methyltransferase biology in humans during acute and chronic skeletal muscle plasticity. J. Appl. Physiol. 127 (1985), 867–880. doi:10.1152/japplphysiol.00142.2019
Wang H., Pei S., Fang S., Jin S., Deng S., Zhao Y., et al. (2021). Irisin restores high glucose-induced cell injury in vascular endothelial cells by activating Notch pathway via Notch receptor 1. Biosci. Biotechnol. Biochem. 85, 2093–2102. doi:10.1093/bbb/zbab137
Wu F., Song H., Zhang Y., Zhang Y., Mu Q., Jiang M., et al. (2015). Irisin induces angiogenesis in human umbilical vein endothelial cells in vitro and in zebrafish embryos in vivo via activation of the ERK signaling pathway. PLoS One 10, e0134662. doi:10.1371/journal.pone.0134662
Xi Y., Hao M., Liang Q., Li Y., Gong D. W., Tian Z. (2020). Dynamic resistance exercise increases skeletal muscle-derived FSTL1 inducing cardiac angiogenesis via DIP2A-Smad2/3 in rats following myocardial infarction. J. Sport Health Sci. 10, 594–603. doi:10.1016/j.jshs.2020.11.010
Xiao L., Wang Z., Lu N., He Y., Qiao L., Sheng X., et al. (2021). GPER mediates the IL6/JAK2/STAT3 pathway involved in VEGF expression in swine ovary GCs. J. Mol. Endocrinol. 68, 23–33. doi:10.1530/JME-21-0125
Xu X., Zhang T., Mokou M., Li L., Li P., Song J., et al. (2020). Follistatin-like 1 as a novel adipomyokine related to insulin resistance and physical activity. J. Clin. Endocrinol. Metab. 105, dgaa629. doi:10.1210/clinem/dgaa629
Yang H. F., Lin X. J., Wang X. H. (2018). Effects of aerobic exercise plus diet control on serum levels of total IGF-1 and IGF-1 binding protein-3 in female obese youths and adolescents. Zhongguo Ying Yong Sheng Li Xue Za Zhi 34, 78–82. doi:10.12047/j.cjap.5571.2018.020
Yazdani F., Shahidi F., Karimi P. (2020). The effect of 8 weeks of high-intensity interval training and moderate-intensity continuous training on cardiac angiogenesis factor in diabetic male rats. J. Physiol. Biochem. 76, 291–299. doi:10.1007/s13105-020-00733-5
Yousefabadi H. A., Niyazi A., Alaee S., Fathi M., Rahimi G. R. M. (2021). Anti-inflammatory effects of exercise on metabolic syndrome patients: A systematic review and meta-analysis. Biol. Res. Nurs. 23, 280–292. doi:10.1177/1099800420958068
Zhang Q., Cao X. (2019). Epigenetic regulation of the innate immune response to infection. Nat. Rev. Immunol. 19, 417–432. doi:10.1038/s41577-019-0151-6
Zhao J., O'Neil M., Vittal A., Weinman S. A., Tikhanovich I. (2019). PRMT1-Dependent macrophage IL-6 production is required for alcohol-induced HCC progression. Gene Expr. 19, 137–150. doi:10.3727/105221618X15372014086197
Zhao S., Zhong J., Sun C., Zhang J. (2021). Effects of aerobic exercise on tc, hdl-C, ldl-C and tg in patients with hyperlipidemia: A protocol of systematic review and meta-analysis. Med. Baltim. 100, e25103. doi:10.1097/MD.0000000000025103
Keywords: myokine, exercise, angiogenesis, ischemic diseases, cytokines
Citation: Qi C, Song X, Wang H, Yan Y and Liu B (2022) The role of exercise-induced myokines in promoting angiogenesis. Front. Physiol. 13:981577. doi: 10.3389/fphys.2022.981577
Received: 29 June 2022; Accepted: 05 August 2022;
Published: 26 August 2022.
Edited by:
Joon-Young Park, Baylor University, United StatesReviewed by:
Joo Young Huh, Chonnam National University, South KoreaCopyright © 2022 Qi, Song, Wang, Yan and Liu. This is an open-access article distributed under the terms of the Creative Commons Attribution License (CC BY). The use, distribution or reproduction in other forums is permitted, provided the original author(s) and the copyright owner(s) are credited and that the original publication in this journal is cited, in accordance with accepted academic practice. No use, distribution or reproduction is permitted which does not comply with these terms.
*Correspondence: Bin Liu, bGl1YmluMzMzM0B2aXAuc2luYS5jb20=
Disclaimer: All claims expressed in this article are solely those of the authors and do not necessarily represent those of their affiliated organizations, or those of the publisher, the editors and the reviewers. Any product that may be evaluated in this article or claim that may be made by its manufacturer is not guaranteed or endorsed by the publisher.
Research integrity at Frontiers
Learn more about the work of our research integrity team to safeguard the quality of each article we publish.