- 1Department of Veterinary and Biomedical Sciences, University of Minnesota, Falcon Heights, MN, United States
- 2Department of Food Science and Human Nutrition, Michigan State University, East Lansing, MI, United States
- 3Department of Animal Sciences, The Ohio State University/Ohio Agricultural Research and Development Center, Wooster, OH, United States
Thermal stress poses a threat to agricultural systems through increased risk to animal growth, health, and production. Exposure of poultry, especially hatchlings, to extreme temperatures can seriously affect muscle development and thus compromise subsequent meat quality. This study was designed to characterize transcriptional changes induced in turkey muscle satellite cells (SCs) cultured from commercial birds under thermal challenge to determine the applicability of previous results obtained for select research lines. Satellite cells isolated from the pectoralis major muscle of 1-week old commercial fast-growing birds (Nicholas turkey, NCT) and from a slower-growing research line (RBC2) were proliferated in culture at 38°C or 43°C for 72 h. RNAseq analysis found statistically significant differences in gene expression among treatments and between turkey lines with a greater number of genes altered in the NCT SCs suggesting early myogenesis. Pathway analysis identified cell signaling and regulation of Ca2+ as important responses. Expression of the intercellular signaling Wnt genes, particularly Wnt5a and 7a was significantly altered by temperature with differential response between lines. The peripheral calcium channel RYR3 gene was among the genes most highly upregulated by heat stress. Increased expression of RYR3 would likely result in higher resting cytosolic calcium levels and increased overall gene transcription. Although responses in the calcium signaling pathway were similar among the RBC2 and NCT lines, the magnitude of expression changes was greater in the commercially selected birds. These results provide evidence into how SC activity, cellular fate, and ultimately muscle development are altered by heat stress and commercial selection.
Introduction
A major agricultural concern is a decline in food and feed production in geographic regions experiencing adverse effects of climate change. Temperature and humidity extremes are especially detrimental to livestock, including poultry, with hatchlings and pre-market birds being particularly vulnerable at this critical stage of development. Contemporary commercial poultry have been genetically selected over several decades for increased growth, feed conversion, and breast (pectoralis major) muscle yield, resulting in larger, faster growing birds with altered muscle morphology, especially at the microscopic level. These changes result in muscle that generates greater metabolic heat (Konarzewski et al., 2000) coupled with reduced capillary supply (relative to mass) that increases the concentration of anaerobic respiration products (Hoving-Bolink et al., 2000; Velleman et al., 2003; Joiner et al., 2014). As a result, faster-growing commercial birds have lower heat tolerance and suffer increased muscle defects due to heat stress (Wilson et al., 1990; Berrong and Washburn, 1998; Velleman et al., 2003; Chiang et al., 2008). The potential economic losses due to reduced production and meat quality are of particular concern to poultry producers.
Growth and development of muscle are dependent on the activity of multipotent stem cells (satellite cells, SCs) that have the ability to follow myogenic, osteogenic, or adipogenic cellular pathways (Asakura et al., 2001; Powell et al., 2016). The number of muscle myofibers is fixed at hatch (Smith, 1963) and post-hatch growth is the result of active proliferation of progenitor SCs followed by fusion to form myofibers (Moss and Leblond, 1971). During the first week of life of the post-hatch period, SCs have high levels of mitotic activity needed for hypertrophic growth; and after this period the cells become quiescent until they are needed for repair (Mozdziak et al., 1994; Halevy et al., 2001; Bi and Kuang, 2012). In response to heat stress, avian SCs show accelerated proliferation and differentiation (Halevy et al., 2001) with cells being more sensitive to environmental temperatures during proliferation (Xu et al., 2021a). Thus, post-hatch thermal stress can potentially change myogenic potential by altering SC heterogeneity. These observations are of particular importance because of the nature of the poultry industry. Following hatch birds are transported to farms, frequently over long distances, and are potentially exposed to temperature extremes. Because they have immature thermoregulatory systems, their body temperatures, especially near the body surface, may be significantly elevated thereby triggering adverse muscle development when SCs are highly active.
In a previous study (Reed et al., 2017a), we contrasted effects of thermal challenge on proliferating turkey skeletal muscle SC transcriptomes from select research lines: RBC2 (Randombred control line 2) and F line (selected for only 16 weeks body weight from the RBC2 line) (Nestor, 1977; 1984). The SCs used in that study (isolated from 7-week old male birds) showed a general increase in expression of genes related to muscle system development and differentiation with heat-treatment. The activation and proliferation of SCs is controlled by signaling molecules and myogenic regulatory transcription factors such as MYOD1 (Myogenic Differentiation 1) and interacting genes. These genes were significantly upregulated in SCs of the growth selected line with heat treatment (Clark et al., 2016; Reed et al., 2017a). The “Wingless-related integration site” Wnt gene (Wnt7a) important in the induction of satellite cells, is implicated in several developmental processes, including regulation of cell fate and patterning during embryogenesis (Eubelen et al., 2018). This gene was also significantly upregulated indicating potential differential control of SC proliferation with growth selection. Thus, these studies identified important genes and pathways of potential significance to heat response in commercial turkeys.
The present study was designed to determine the applicability of previous results obtained for select research lines to that of commercial birds by examining transcriptional changes induced in SCs under thermal challenge. Here we examine gene expression in SCs derived from 1-week old commercial fast-growing birds and from the slower-growing RBC2 line. Importantly, this corresponds to the period of maximal mitotic activity and period of SC response to external stimuli like temperature. We hypothesize that satellite cell activity, cellular fate, and muscle development are altered by age of the SCs and heat stress. We contrast the results from SCs of commercial birds with that of the research lines used in previous studies. Understanding the effects of heat stress on satellite cell-mediated mechanisms will allow development of effective breeding, nutritional, and management strategies to enhance production of consistent, high-quality muscle food products and minimize impact of climatic stressors.
Methods
Turkey myogenic satellite cells
Satellite cells were previously isolated from the p. major muscle of 1-week-old male Nicholas Commercial (NCT) turkeys and RBC2 turkeys as described by Velleman et al. (2000) and stored in liquid nitrogen until use. The NCT turkeys are modern commercial meat-type turkeys selected for increased growth rate and breast muscle yield and were obtained from Nicholas Turkeys (Lewisburg, WV). The RBC2 turkeys were initiated in 1966 by crossing two commercial large white turkey strains representing the genetics of the commercial turkeys at the time (Nestor et al., 1969). The RBC2 line was then maintained at the Poultry Research Center of the Ohio Agricultural Research Development Center/The Ohio State University, Wooster, OH without conscious selection for any trait. Only SCs from males were used to avoid any sex effects (Velleman et al., 2000).
Turkey p. major SCs were cultured as described in Reed et al. (2017a). Briefly, cells were plated and incubated at 38°C for 24 h in plating medium consisting of Dulbecco’s Modified Eagle’s Medium (DMEM, Sigma Aldrich, St. Louis, MO), 10% chicken serum (Gemini BioProducts, West Sacramento, CA), 5% horse serum (Gemini BioProducts), 1% antibiotics-antimycotics, and 0.1% gentamicin. After 24 h the cells were fed growth medium and cultured at an experimental temperature (38° or 43°C) for 72 h. The control temperature of 38°C is approximately that measured in newly hatched poults (38.0–38.5°C, Strasburg, unpublished data). The heat stress temperature of 43°C exceeds the approximate body temperature of mature turkeys (41.5°C) and has been shown to have significant effects on SC proliferation (Clark et al., 2016; Xu et al., 2021a). Growth medium was changed every 24 h during the 72 h treatment. At harvest, cell medium was removed and the cells were collected into RNAzol RT (Sigma-Aldrich) and held at −80°C until RNA isolation.
RNA isolation and sequencing
Total RNA from each sample was isolated by RNAzol RT extraction, DNase-treated (Turbo DNA-freeTM Kit, Ambion, Inc.), and stored at −80°C. Initial RNA concentration and quality were assessed by spectrophotometry (Nanodrop 1000), and samples were submitted for library preparation and sequencing at the University of Minnesota Genomics Center. Each sample was further quantified by RiboGreen Assay (Invitrogen Corp.) and RNA integrity confirmed on the 2100 Bioanalyzer (Agilent Technologies). For each treatment group, replicate samples were prepped for sequencing (n = 2 biological replicates per treatment group). RNA Integrity Numbers (RIN) ranged between 5.2 and 9.0. Indexed libraries were constructed with 1 μg of total RNA/sample with the TruSeq Stranded mRNA Sample Preparation Kit (Illumina, Inc.) and size selected for approximately 200 bp inserts. Libraries were multiplexed and sequenced on the NovaSeq SP platform using v1.5 chemistry (Illumina, Inc.) to produce 51-bp paired-end reads.
RNAseq data analyses
Sequence adapters were removed and low quality bases were trimmed using Trimmomatic (Bolger et al., 2014) enabled with the optional “−q” option; 3 bp sliding-window trimming from 3’ end requiring minimum Q30. Quality control checks on raw sequence data for each sample were performed with FastQC (Andrews, 2010). Read mapping was performed via Bowtie (v2.2.4.0) using the turkey genome (UMD 5.1, ENSEMBL Annotation 104). Read counts were normalized in CLC Genomics Workbench (CLCGWB v. 10.1, CLC Bio).
Identifiers for annotated genes were iteratively obtained from several sources. The majority were retrieved directly from the ENSEMBL annotation. For genes with empty ENSEMBL ID fields, additional gene IDs were obtained from individual searches of DAVID, Uniprot and NCBI using the Ensembl IDs. For the remaining “novel” genes, individual searches were conducted in ENSEMBL for orthologs in the chicken genome (GRCg6a). This resulted in approximately 2500 genes in the turkey gene list remaining as uncharacterized or novel protein coding genes.
Hierarchical clustering of samples (based on Euclidean sample distances with single linkage) was performed in CLCGWB using normalized reads counts. Empirical analysis of differential gene expression and ANOVA was performed in CLCGWB on original expression values (Bonferroni and FDR corrected). Principal component analysis (PCA) was performed in CLCGWB and pair-wise comparisons between treatment groups were made in the Bioconductor (3.2) R package DESeq2 (Love et al., 2014) following the standard workflow. Gene enrichment tests were performed using the PANTHER Overrepresentation Test [GO Consortium release 20150430 (Mi et al., 2013); http://geneontology.org/]. For GO analysis the reference gene list of the chicken (Gallus gallus) was utilized with ∼66% of the turkey loci (Annotation 105) having ID homologs as presented in Supplementary Table S1. Additional analyses were conducted through the use of Qiagen Ingenuity Pathway Analysis (Krämer et al., 2014).
Results
Gene expression
Total RNA isolated from SC cultures (n = 8) was used to construct individual barcoded libraries. Sequencing produced over 181M paired-end reads (accessioned as part of SRA BioProject PRJNA842679). The number of reads per library ranged from 20.7 to 25.7M (average 22.7M) (Table 1). After read trimming and filtering, read quality was consistently high and Q values ranged from 35.8 to 36.3. Replicate libraries produced comparable results with an average difference between replicates of 2.2M reads.
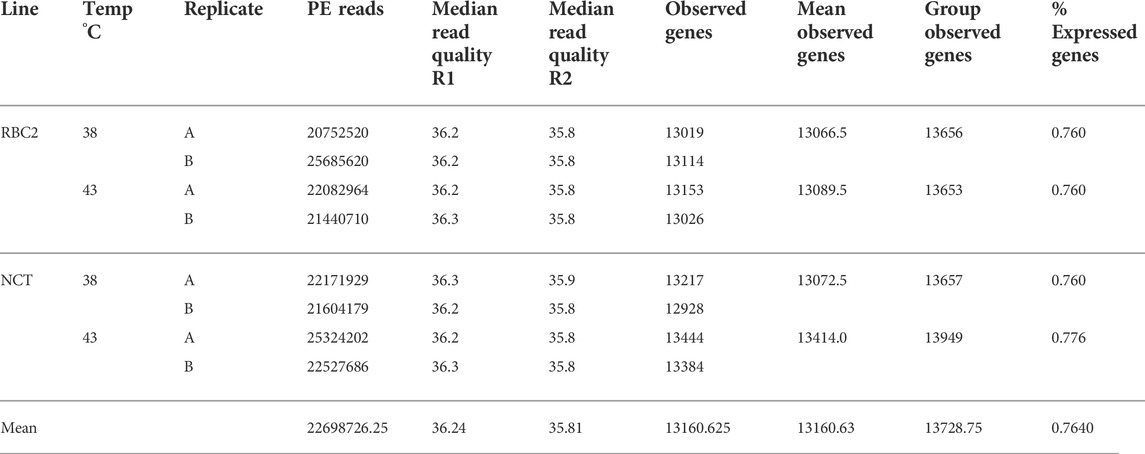
TABLE 1. Summary of RNAseq data for proliferation experiment. For each library the total number of raw reads, median read qualities (R1 and R2), the number of observed genes (mapped reads >1) by library and treatment group and the percentage of expressed genes (group read count >1.0) are given.
Evidence for expression (at least one mapped read in at least one treatment group) was observed for 15,118 genes and 76.4% of the ENSEMBL turkey gene set (80.3% of protein-coding genes) (Supplementary Table S1). The number of observed genes per library ranged from 12,928 to 13,444 (average 13,160.6), whereas the number of observed genes per treatment group ranged from 13,653 to 13,949 (average 13,728.7) (Table 1). Variation among treatment groups was visualized by principal component analysis (PCA) based on normalized read counts (Figure 1). Treatment groups clustered distinctly (Temp/Time) within the first two principal components irrespective of line. Replicate treatment pairs occurred as nearest neighbors within the PCA space, supporting the pooling of replicates for expression analyses.
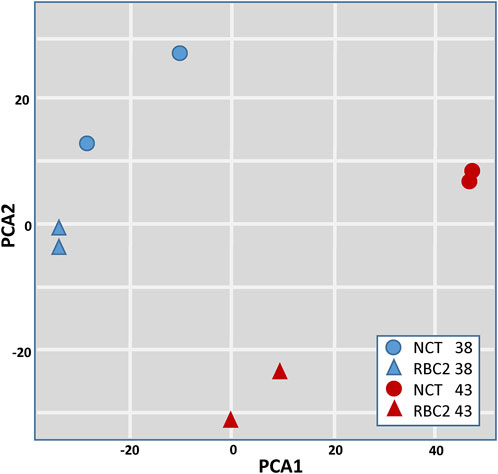
FIGURE 1. Principal component (PCA) plot of RNAseq data based on normalized read counts. Sample to sample distances (within- and between-treatments) are illustrated on the first two principal components. Samples are plotted according to treatment.
Differences in gene expression among groups is demonstrated in the distribution of unique and shared expressed genes among treatment groups as illustrated in Table 2. On average 13,031 genes were expressed in the between-treatment group comparisons within lines (Temp effect), and 12,080 being shared. Characterization of the suite of expressed genes in the SCs derived from muscle from 1-week old birds was consistent with that observed in our previous study of cells from 7-week birds with enzymes, transcription regulators and transporter being the most represented functional class separate of structural proteins (Supplementary Table S1). Numbers of uniquely expressed genes were higher at the higher temperature (460, and 650 in the RBC2 and NCT lines, respectively). Within temperature an average of 12,972 genes were expressed between lines, with 12,140 being shared. This reflects an overall similarity in response of cultured SCs between the turkey lines. Here, the number of uniquely expressed genes were higher in the RBC2 at 38°C but higher in the NCT lines at 43°C.
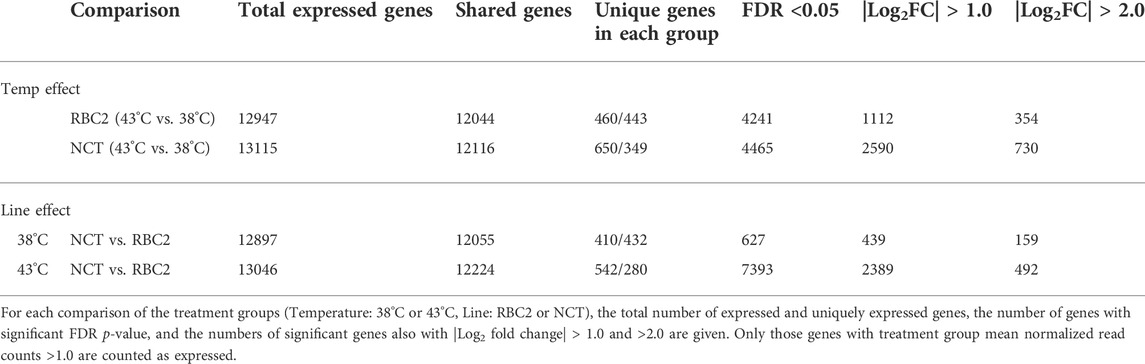
TABLE 2. Summary of gene expression and significant differential expression (DE) in pair-wise comparisons of proliferating cells.
Temperature effects
Temperature effects were examined in two pairwise, within-line comparisons: RBC2 hot-versus control (43 vs. 38°C) and NCT hot versus control (43 vs. 38°C) (Supplementary Table S2). Each of these within-line comparisons identified over 4000 significant differentially expressed (DE) genes (FDR adjusted p-value < 0.05, Table 2) in the SCs; a greater number of DE genes was observed in the NCT SCs (4465) compared to the RBC2 (4241). These numbers are substantially reduced when limited by fold change. For example, the number of DE genes in the RBC2 group comparison is reduced to 1,112 and 354 (|Log2FC| > 1.0, and >2.0, respectively).
As illustrated in Figure 2, a total of 880 genes were identified with significant DE (p < 0.05 and |Log2FC| > 2.0) in the comparison of SCs proliferated at 43 versus 38°C with 204 shared between the lines. Of the 354 DEGs significant in the RBC2 SCs, 217 were upregulated and 137 down regulated with heat treatment (43°C compared to 38°C) (Supplementary Table S2). Unique to the RBC2 cells were 150 DEGs altered by heat challenge. The 40 significant DEGs showing the greatest fold change are listed in Figure 3. Genes down regulated in the RBC2 line included two loci (ENSMGAG00000003778 and ENSMGAG00000020934) with similarity to selectin E (SELE). In mammals, this protein-coding gene is expressed in cytokine-stimulated endothelial cells and is involved in inflammation by mediating adhesion of cells to the vascular lining. Also included are genes for structural proteins {MPZL2 (myelin protein zero like 2), ENSMGAG00000000617 (Tubulin beta chain), integral membrane proteins [TMEM81 (transmembrane protein 81), TMEM132E (transmembrane protein 132E), SLC6A4 (solute carrier family 6 member 4)], and growth factors [FGF19 (fibroblast growth factor 19), GDF9 (growth differentiation factor 9), IGFALS (insulin like growth factor binding protein acid labile subunit)]}; some of these genes have potential roles in development. Up regulated genes include WNT7a, a Wnt family member. Genes in this family are involved in several developmental processes including regulation of cell fate and patterning. Several genes associated with muscle function are also included: [LOC100549331 (myosin-7-like), MYL1 (myosin light chain 1), and MYL10 (myosin light chain 10), and TUBAL3 (tubulin alpha-3 chain)]. Pathway analysis (PANTHER) of the 354 RBC2 DEGs identified the inflammation mediated by chemokine and cytokine signaling pathway (P00031), Wnt signaling pathway (GO:P00057), and Angiogenesis (GO:P00005) as affected pathways. Overrepresentation test of the up- and down-regulated gene sets suggest heat treatment up regulates muscle function and down regulates endocrine response (Table 3).
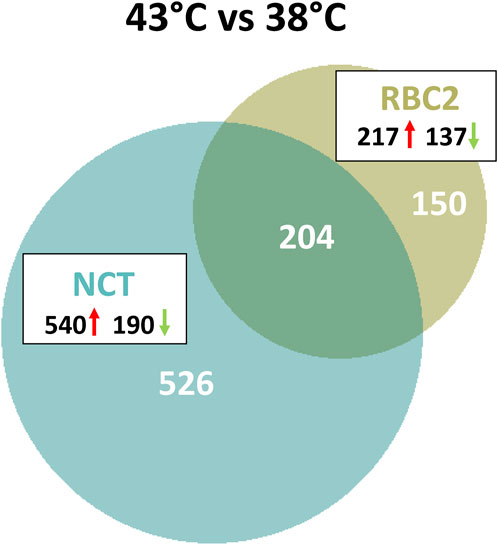
FIGURE 2. Distribution of differentially expressed genes during proliferation of cultured turkey p. major satellite cells. For each temperature comparison, the number of genes (FDR pval <0.05 and |Log2FC| > 2.0) shared or unique to each line (RBC2 and NCT) are indicated in the Venn diagram. Circle size is proportional to the number of genes.
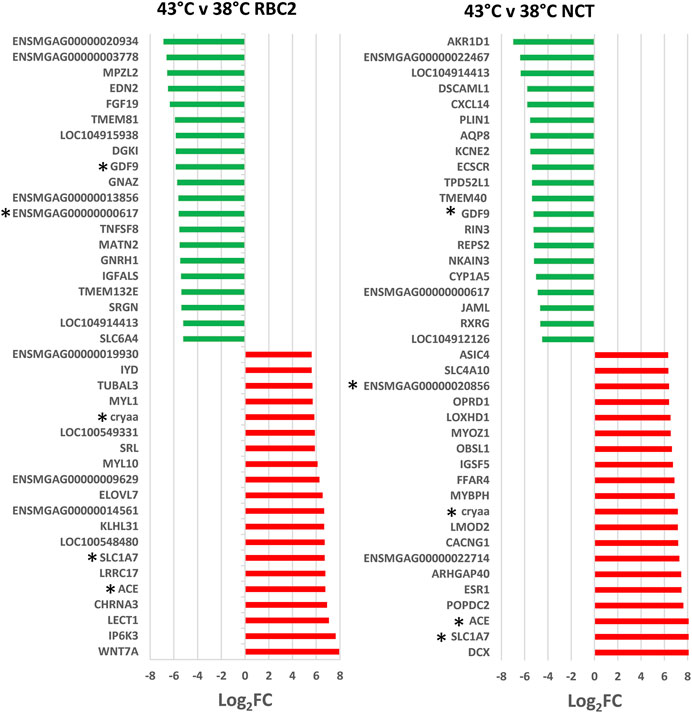
FIGURE 3. Directional change in the genes showing the highest expression differences between lines (NCT and RBC2) at 38 and 43°C. For each with-in temperature comparison the Log2FC for each gene is plotted (FDR pval <0.05). Genes shared between temperatures are indicated by asterisks.
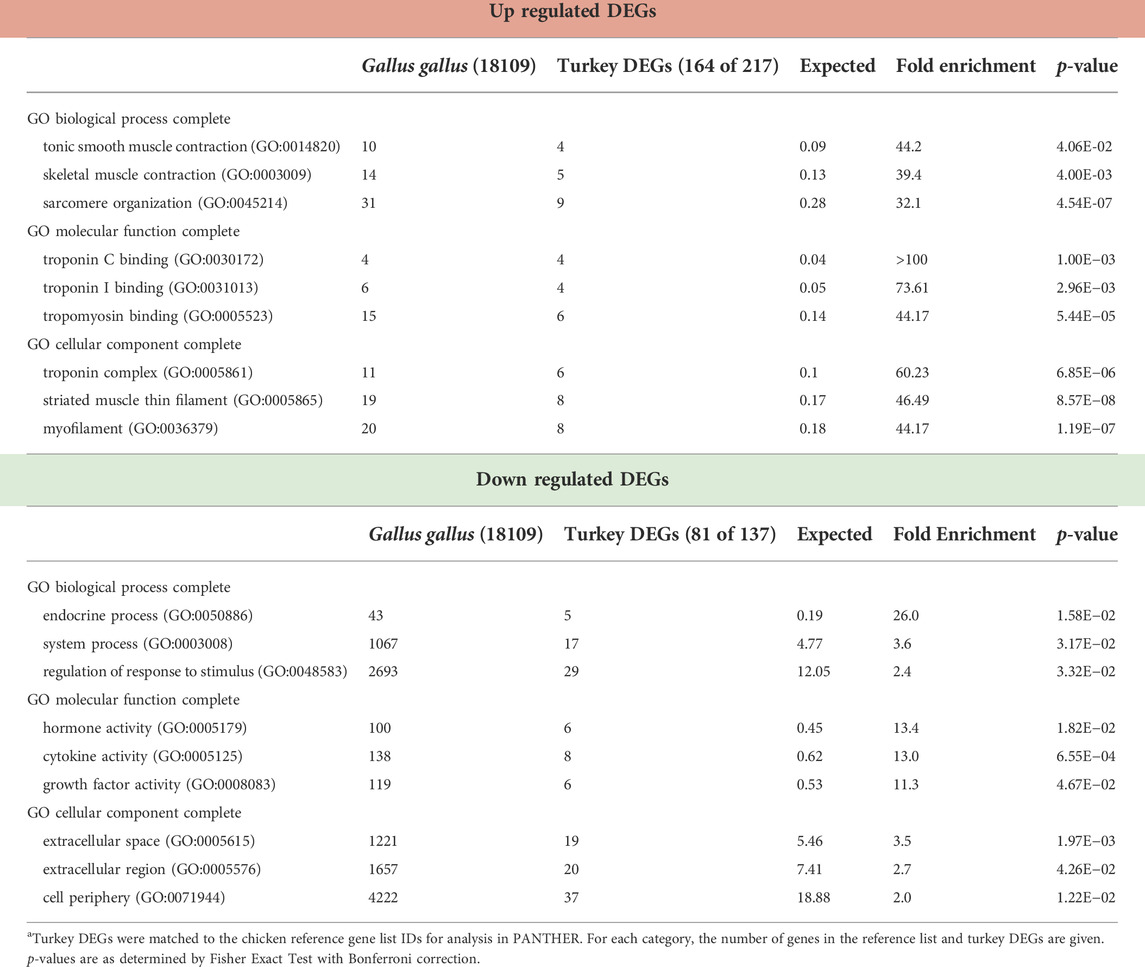
TABLE 3. PANTHER Overrepresentation test of 354 DEGs in the RBC2 line temperature comparison (43°C vs. 38°C) after 72 h of proliferation. Shown are the three gene ontology categories with the greatest fold enrichment in each category for the up and down-regulated genesa.
A greater number of genes were affected by heat treatment (730 total DEGs) in the NCT SCs, with 540 being upregulated and 190 down regulated (Figure 2, Supplementary Table S2). Unique to the NCT cells were 526 DEGs altered by heat challenge. Genes showing the largest fold change (Figure 3) include a suite of diverse structural, enzymatic, physiological, and immunological functions. Muscle-associated genes such as MYOZ1 (myozenin 1), MYBPH (myosin binding protein H) and CACNG1 (calcium voltage-gated channel auxiliary subunit gamma 1) were significantly upregulated by heat treatment. However, genes with putative vascular/endothelial functions were both up-regulated [ACE (angiotensin I converting enzyme)] and down-regulation [ECSCR (endothelial cell surface expressed chemotaxis and apoptosis regulator), KCNE2 (potassium voltage-gated channel subfamily E regulatory subunit 2), and LOC104912126 (type-1 angiotensin II receptor-like)]. Pathway analysis (PANTHER) of the 730 NCT DEGs identified the Nicotinic acetylcholine receptor signaling (4.04 fold) and the Inflammation mediated by chemokine and Cytokine signaling (2.97 fold, p = 7.70E−03) as significantly enriched pathways. Overrepresentation test of the up- and down-regulated gene sets found heat treatment to up regulate muscle structural genes and down regulate regulation of multicellular organismal processes (GO:0051240) (Table 4).
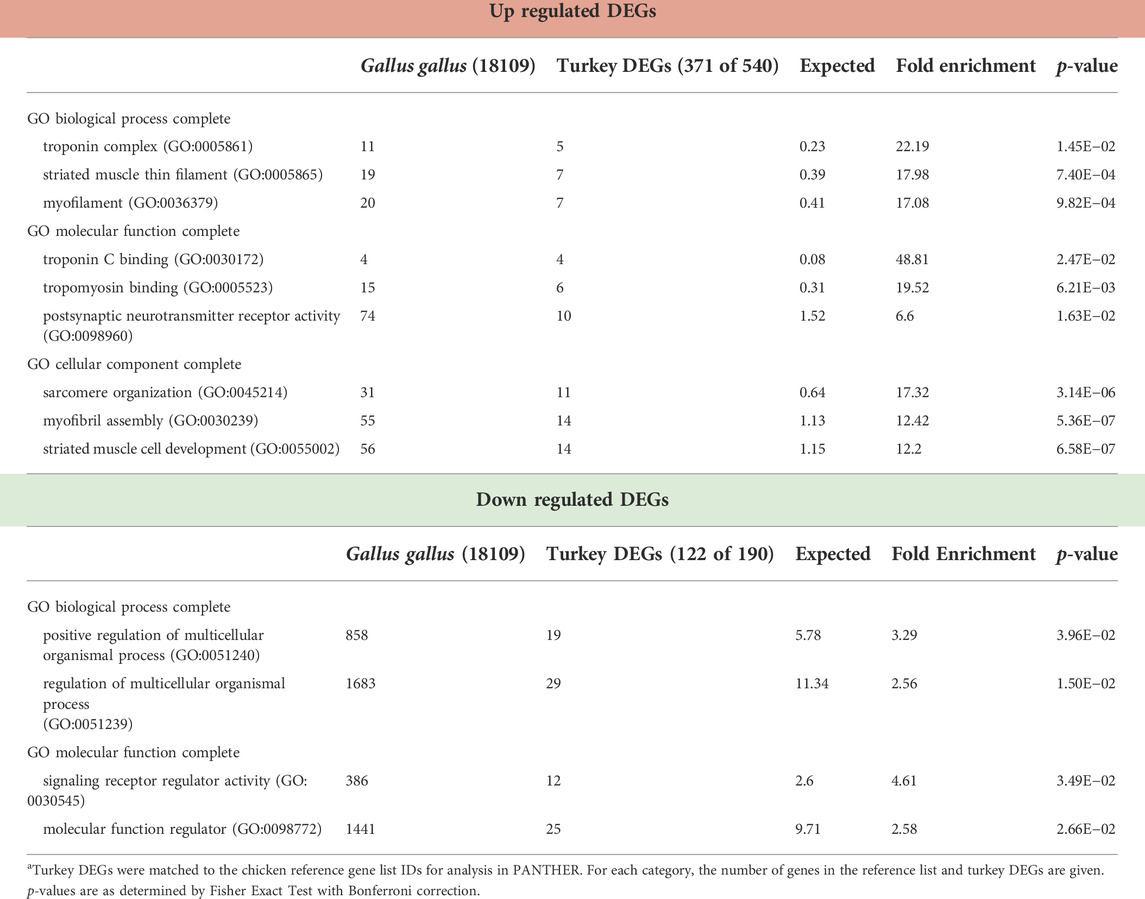
TABLE 4. PANTHER Overrepresentation test of 730 DEGs in the NCT line temperature comparison (43°C vs. 38°C) after 72 h of proliferation. Shown are the gene ontology categories with the greatest fold enrichment in each category for the up and down-regulated genesa.
Among the 204 DEGs shared between lines, 159 were upregulated at 43°C compared to 38°C and 45 down regulated in both lines (denoted in Supplementary Table S2). This identifies a set of and similarly responding genes common to both lines. PANTHER analysis shows significant overrepresentation in these DEGs of genes associated with multiple GO biological process categories including tonic smooth muscle contraction (GO:0014820, 61.18 fold enrichment), regulation of cellular extravasation (GO:0002693, 48.94) regulation of myotube differentiation (GO:0010831, 40.79) sarcomere organization (GO:0045214, 31.58), myofibril assembly (GO:0030239, 22.25) and striated muscle cell development (GO:0055002, 21.85) as well as others.
Ingenuity pathway analysis (IPA, Krämer et al., 2022) was also used to infer biological functions in SCs significantly affected by heat treatment of RBC2 and NCT SCs. Approximately 68% of the turkey genes mapped to IPA identifiers. Canonical pathways were identified for both the RBC2 and NCT temperature comparisons (43°C vs. 38°C) and are summarized in Table 5. Activation z-score represents the change in activation state and thus implicated biological function. Of the pathways with the highest absolute activation z-scores, the majority were signaling pathways with calcium signaling ranking among the highest in both lines.
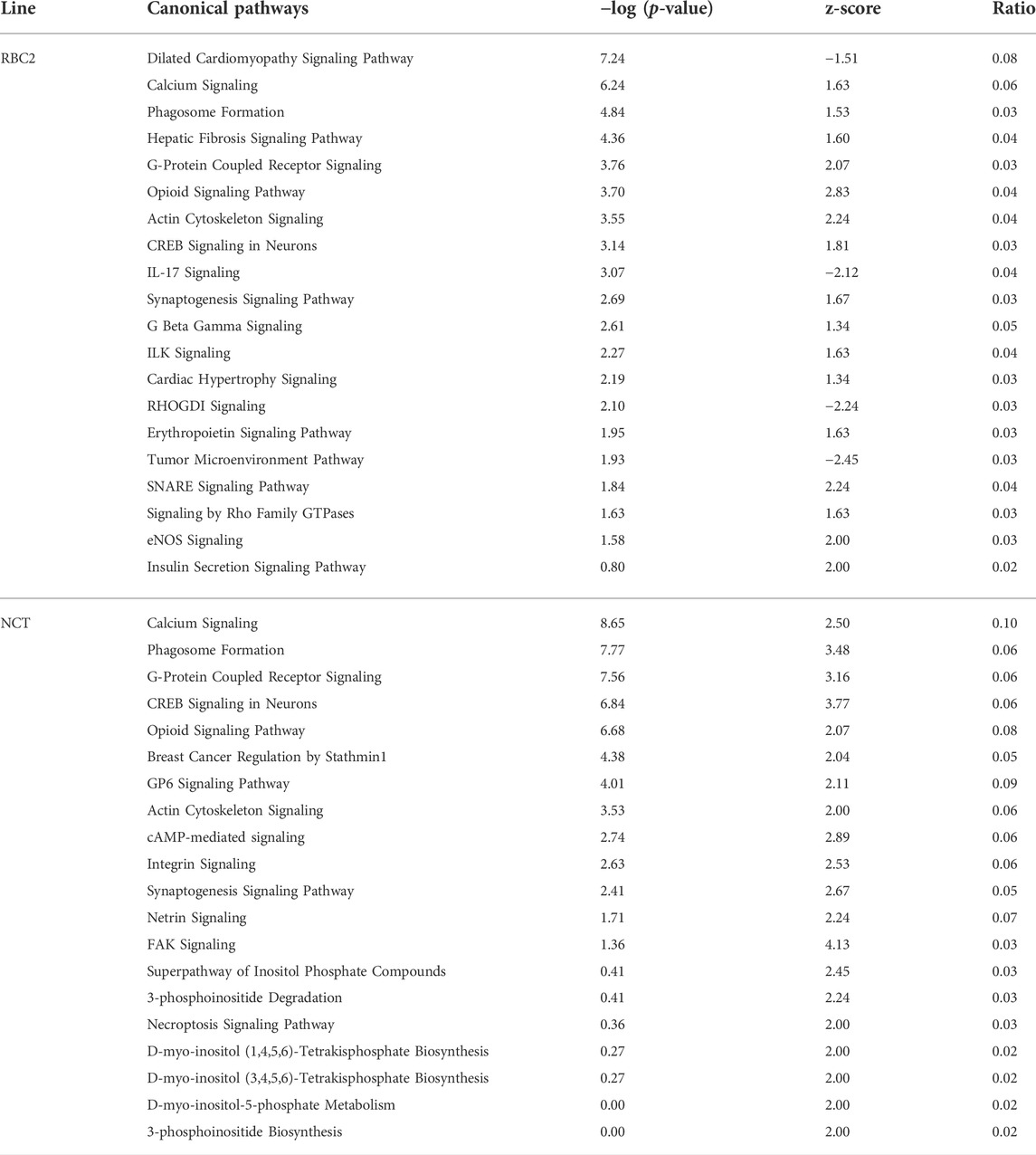
TABLE 5. Significant canonical pathways identified by Ingenuity Pathway Analysis (IPA) for turkey satellite cells following thermal stress. For each line, the 20 pathways with the greatest absolute activation score are given. Pathways are sorted by −log (p-value).
Effects of selection (line differences)
Line effects were examined in two pairwise within-temperature comparisons: Control NCT versus RBC2 (38°C) and heat-treated NCT versus RBC2 (43°C) (Table 2). Comparisons between lines at 38°C identified 627 significant DEGs (FDR adjusted p-value < 0.05, Table 2) with 159 having |Log2FC > 2.0|. Greater gene expression response was observed at 43°C where 7393 DEGs with p < 0.05 were identified and 492 of these with |Log2FC > 2.0|.
A total of 593 combined genes were identified with significant DE (p < 0.05 and |Log2FC| > 2.0) in the comparison between lines. Of these DEGs, 101 were unique to the 38°C comparison, 434 unique at 43°C and 58 shared between the two temperatures (Figure 4). Of the 159 DEGs significant at 38°C, 67 were upregulated and 92 down regulated in the NCT cells compared to RBC2 (Supplementary Table S2).
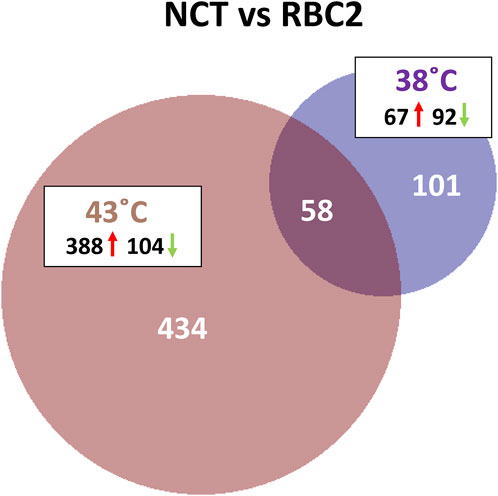
FIGURE 4. Distribution of differentially expressed genes between lines (F-line versus RBC2) during proliferation of p. major satellite cells. For each temperature comparison, the number of genes with FDR pval <0.05 and |Log2FC| > 2.0 that were shared or unique to each incubation temperature are indicated. The number and direction of expression change (↑or ↓) for the genes included in each temperature group are listed outside the Venn diagram. Circle size is proportional to the number of genes.
The 40 significant DEGs showing the greatest expression differences between the lines are listed in Figure 5. Highly upregulated in NCT cells relative to RBC2 (Log2FC = 7.81) was a gene with orthology to SPTSSB (serine palmitoyltransferase, small subunit B). In mammals, this enzyme catalyzes the initial rate-limiting step in sphingolipid biosynthesis (Merrill, 1983). Also upregulated were EFEMP1 (EGF containing fibulin extracellular matrix protein 1) an extracellular matrix glycoproteins of the fibulin family, LGR5 (leucine rich repeat containing G protein-coupled receptor 5, Log2FC = 3.85), a receptor for R-spondins with a potential role in canonical Wnt signaling in the formation and maintenance of adult stem cells, and PTPRR (protein tyrosine phosphatase receptor type R, Log2FC = 3.75) a member of the protein tyrosine phosphatase (PTP) family that regulate cell growth, and differentiation.
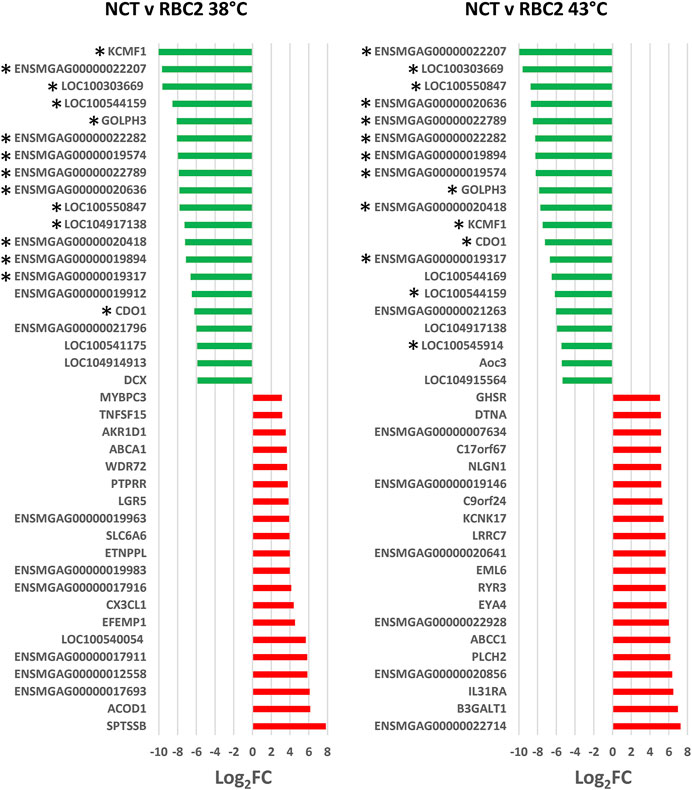
FIGURE 5. Directional change in the genes showing the highest expression differences at 43°C compared to 38°C. For each with-in line comparison (RBC2 and NCT) the Log2FC for each gene is plotted (FDR pval <0.05). Genes shared between temperatures are indicated by asterisks.
Several ubiquitylation proteins such as KCMF1 (E3 ubiquitin-protein ligase), LOC100303669 (ubiquitin-associated protein 2), and ENSMGAG00000022282 (ubiquitin-conjugating enzyme E2 R2-like), were highly down regulated in NCT compared to RBC2 at 38°C. Ubiquitylation can result in several important enzymatic post-translational modifications. Pathway analysis of the 92 down regulated genes identified the Nicotinic acetylcholine receptor signaling (12.78 fold) and Wnt signaling pathway (8.83 fold) as significantly enriched pathways. Overrepresentation test of the down-regulated genes found highest enrichment for genes involved in cell differentiation and morphogenesis (Table 6).
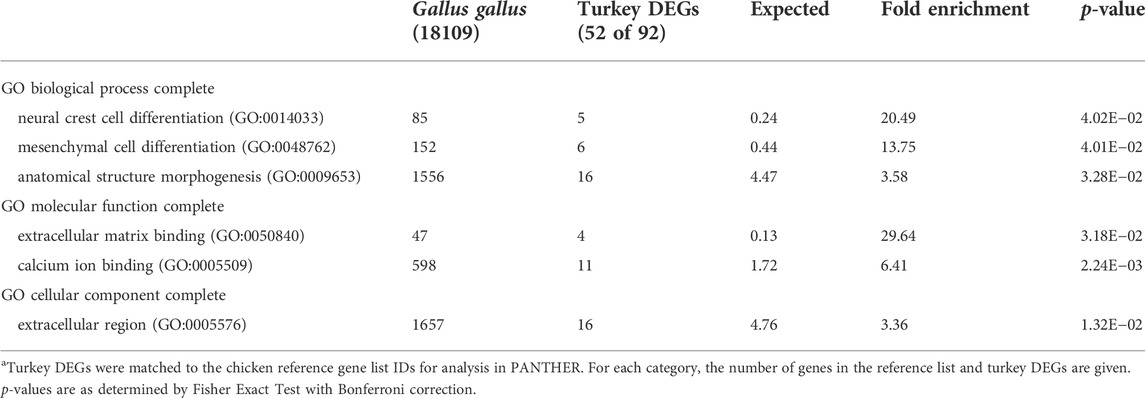
TABLE 6. PANTHER Overrepresentation test of 92 DEGs down regulated in the NCT cells relative to the RBC2 at 38°C after 72 h of proliferation. Shown are the gene ontology categories with the greatest fold enrichment in each categorya.
There was a greater number of differentially expressed genes between the lines at 43°C (492 total DEGs) compared to 38°C (Figure 4) and 434 responded uniquely at 43°C (Supplementary Table S2). Expression of 388 genes was significantly higher in the NCT cells with 104 down regulated compared to the RBC2 cells. Among the top up-regulated genes in the NCT line is the ryanodine receptor RYR3 (Figure 5), one of two sarcoplasmic reticulum calcium channel isoforms (the other being RYR1) expressed in skeletal muscle. Both channels are involved in muscle contraction. The RYR3 gene product is a calcium channel that is activated by elevated cytosolic [Ca2+] (calcium-induced calcium release) in contrast to RYR1 which is activated by cell depolarization. Of the genes showing the greatest down regulation in the NCT cells at 43°C, 15 were also down regulated at 38°C. Largest fold change was seen for ENSMGAG00000022207 (a novel protein with BLAST similarity to HINTWL2, histidine triad nucleotide binding protein W-like), LOC100303669 (ubiquitin-associated protein 2), and LOC100550847 (spindling-like). Spindlin is of interest as it is an activator of Wnt signaling pathway (Su et al., 2014).
Overrepresentation analysis of the 104 down-regulated genes identified two significant GO biological processes; anatomical structure formation involved in morphogenesis (GO:0048646, 6.33-fold enrichment) and multicellular organismal process (GO:0032501, 2.37 fold enrichment). Analysis of the 388 up-regulated genes identified significant enrichment for genes associated with muscle cell differentiation (GO:0042692), muscle structure development (GO:0061061), and vasculature development (GO:0001944) (Table 7). Taken as a whole, NCT cells responded to heat treatment through increased expression of genes involved in muscle development.
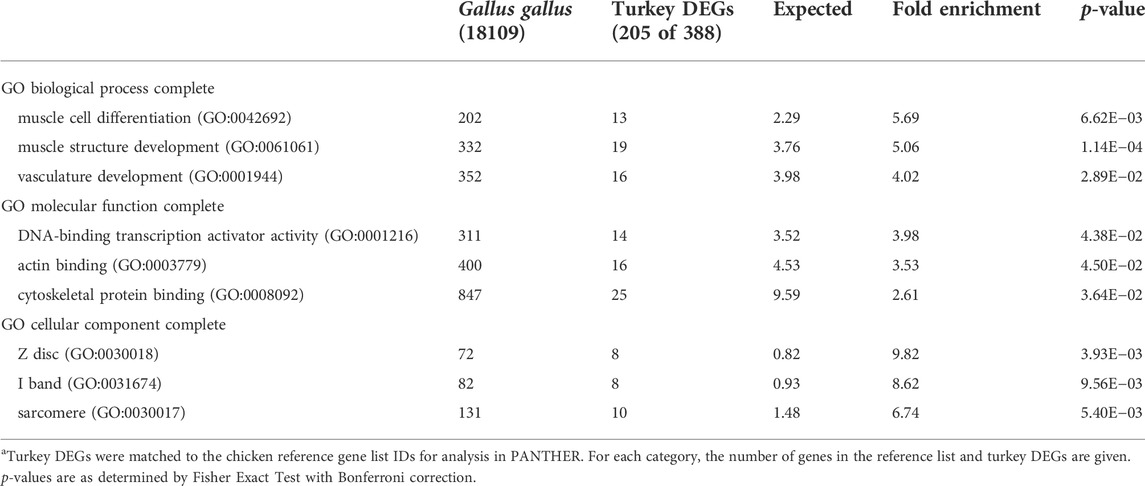
TABLE 7. PANTHER Overrepresentation test of 388 DEGs up regulated in the NCT cells relative to the RBC2 at 43°C after 72 h of proliferation. Shown are the gene ontology categories with the greatest fold enrichment in each categorya.
Among the 58 DEGs shared between the temperature treatments, 19 showed higher expression in the NCT cells and 39 showed higher expression in RBC2 at 38°C, identifying a set of genes where expression is similarly changed in both lines regardless of temperature (denoted in Supplementary Table S2). Many of these were among those with expression most highly altered by the heat treatment (Figure 5). In comparison, 56 DEGs show similar expression directionality and only two genes responded directionally different. The first, aldo-keto reductase family 1 member D1 (AKR1D1), had significantly higher expression in NCT cells at 38°C (Log2FC = 2.4) and lower expression in NCT cells at 43°C (Log2FC = −3.8) compared to RBC2 cells. Although function of this gene is mostly associated with catalysis of bile acid intermediates (5-beta-reduction), it also functions in steroid hormone metabolism (Nikolaou et al., 2019). The second gene, doublecortin (DCX) had significantly lower expression in NCT cells (Log2FC = −5.86) at 38°C and higher expression in at 43°C (Log2FC = 2.4) compared to the RBC2 cells. In mammals, DCX has been shown to promote the stability of microtubules (Horesh et al., 1999).
Gene expression differences between the NCT and RBC2 SCs at 43°C were also examined with IPA (11,256 mapped IDs). Here, the Wnt/Ca2+ canonical pathway had the highest z-score (analysis cutoffs p-value 0.05 and |expression log ratio| > 2.0). Although Wnt7a is significantly up regulated in both NCT and RBC2 cells under thermal challenge, Wnt5a that signals the Wnt/Ca + pathway was significantly down regulated in NCT versus RBC2. Without restricting log ratio, EIF2 signaling (z = −3.22); members of which were down regulated in NCT cells. As primary targets of several signaling pathways, eIFs regulate gene expression by altering translation in response to stress-related signals.
Direct comparison between the responses of the 1 week SCs in the present study to those of the 7 weeks SCs previously examined (Reed et al., 2017a) is difficult due to the use of different genome annotations for analysis. To facilitate comparison, a composite gene list was created using common gene IDs and the DEGs identified in the two studies (43°C versus 38°C) and these were used to run a Comparison analysis in IPA. Comparison analysis of the RBC2 cells found the majority of the significant canonical pathways to have similar activation (z-score) characteristics. Notable exceptions were Calcium signaling (z = 1.195 and −1.265 in 1 and 7 weeks respectively), EIF2 signaling (z = −0.200 and 2.714 in 1 and 7 weeks respectively) and NRF2-mediated oxidative stress response (z = 0.577 and 2.00 in 1 and 7 weeks respectively). Within the Calcium signaling pathway notable differences included the down regulation of the inositol-triphosphate sarcoplasmic reticulum receptor ITPR1 [IP3R] in both RBC2 cell lines with heat challenge.
Similar comparison between the responses of NCT SCs to those of SCs from the 16 weeks bodyweight line F (Reed et al., 2017a) was run in IPA. Significant canonical pathways were obtained and the 20 pathways with highest z-score, primarily signaling pathways, are presented in Figure 6. In general, the lines responded similarly within these canonical pathways but with the NCT cells showing often greater z-score activation. Directional changes between the lines are seen for the Pulmonary Fibrosis Idiopathic Signaling and TCA Cycle II pathways. It is unknown whether these differences are due to the age of the birds from which the SCs were isolated (1 versus 7 weeks) or genetic differences resulting from differential selection. Functional differences might be expected between SCs derived from 1 week poults (more actively proliferating SC population) as compared to a more quiescent SC population in 7 weeks birds (Picard and Marcelle, 2013).
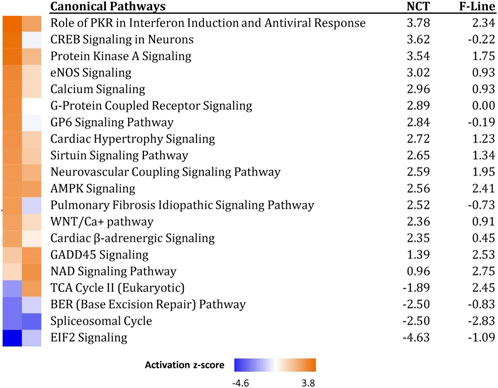
FIGURE 6. Significant canonical pathways identified in IPA Comparison analysis of genes expressed in NCT versus F-Line turkey skeletal muscle SCs at 43°C. Only the 20 pathways with highest composite Z-scores are shown.
Discussion
Post-hatch thermal stress affects the growth and subsequent structure of poultry breast muscle (Piestun et al., 2017; Patael et al., 2019; Halevy, 2020). Thermal stress has significant effects on the proliferation, differentiation and adipogenic potential of SCs isolated from different genetic lines of turkeys (Clark et al., 2016; Reed et al., 2017a,b) and from commercial birds (Xu et al., 2021a, b). The activation and proliferation of SCs is modulated by signaling molecules which direct myogenesis through signaling pathways. Identification of these pathways provides insight on how selection for growth rate and increased muscle mass may alter the biological response to thermal challenge.
Notch signaling is crucial in the processes by which SCs proliferate, generate differentiating cells, or self-renew. Signaling through HES1 controls transcription of the myogenic transcription factor MYOD1 and the Notch ligand DLL1 thereby determining the activation state of SCs. Although heat stress may alter cell proliferation (Halevy et al., 2001; Xu et al., 2021a), expression of the transcription factor Pax7, a marker of proliferation, was similar across treatments. This gene is essential for regulating the expansion and differentiation of SCs (von Maltzahn et al., 2013). Expression of SC proliferation factor MYOD1 was significantly increased in the 1 week skeletal muscle SCs of both turkey lines with heat stress (Log2FC = 2.02 and 1.40 in RBC2 and NCT, respectively). This nuclear protein is a muscle-specific transcriptional regulatory factor. Similar findings for MYOD1 expression were observed in our previous studies (Reed et al., 2017a; Xu et al., 2022). Like MYOD1, significant upregulation in response to heat stress was seen for DLL1 in both RBC2 and NCT SCs (Log2FC = 2.13 and 2.72, respectively). Appropriate oscillation of DLL1 expression in myogenic cells is important in determining cell fate (Lahmann et al., 2021; Zhang et al., 2021).
Growth processes in the turkey SCs are likely accelerated with heat stress as expression of the differentiation factor myogenin (MYOG, Log2FC = 5.35 and 3.99 in RBC2 and NC, respectively) was also up regulated. Myogenin is required for differentiation and the formation of multinucleated myotubes. This up-regulation is consistent with the findings of Xu et al. (2022) who measured MYOG expression by qRT-PCR. Also consistent with Xu et al. (2022), we observed slightly reduced expression of the peroxisome proliferator-activated receptor PPARγ in both lines with heat stress. Expression of myogenic factor 6 (MYF6 [MRF4]), trended slightly lower with heat stress (Log2FC = −1.83 and −1.37 in RBC2 and NCT, respectively). Recent studies in mice found this myogenic regulator checks muscle stem cell exhaustion by transcriptionally regulating multiple myokines and blocking premature differentiation (Lazure et al., 2020).
Other signaling molecules importantly modulate activation of SCs. Three fibroblast growth factors (FGFs), a family of proteins important in cell signaling for normal cellular development, showed significant expression differences. Notably, FGF12 was upregulated in the NCT cells at 43°C compared to control (38°C) and FGF10 was significantly upregulated in the NCT cells relative to the RBC2 at 43°C (Log2FC = 2.02). A third FGF (FGF19) was down regulated by heat stress in the RBC2 cells (43 versus 38°C) and was lower in NCT cells compared to RBC2 in controls (38°C). Although specific function has not been assigned, in mammals FGF10 binds to fibroblast growth factor receptors (FGFRs) but FGF12 does not. Expression of FGF19 in humans is reported in the ileum in response to bile acid absorption (Potthoff et al., 2012). The cytokine LIF (leukemia inhibitory factor) involved in the induction of differentiation, was significantly down regulated in both the RBC2 and NCT cells (Log2FC = −2.60 and −1.83, respectively) with heat stress.
Previous transcriptome analysis of SCs from 7-week old birds (Reed et al., 2017a) found thermal stress alters expression of several Wnt genes. In the present study of SCs from 1-week old birds, Wnt7a was the most highly upregulated gene in the RBC2 line (Log2FC = 7.94) and was significantly upregulated in NCT SCs (Log2FC = 3.37) under heat stress. In the mouse, the Wnt/PCP pathway is regulated through the frizzled-7 (Fzd7) receptor and stimulates SC proliferation, promotes hypertrophy of myofibers resulting in increased skeletal muscle mass (Otto et al., 2008; Le Grand et al., 2009). Up regulation of Wnt7a during the period of SC peak mitotic activity, suggests that thermal stress affects turkey skeletal muscle through a similar mechanism. Supporting evidence is provided by knockdown experiments where in the same cultured 1-week turkey SCs used in this study, knockdown of Fzd7 significantly decreased proliferation and MYOD expression especially under heat stress (Xu et al., 2022). The NCT SCs being less affected than RBC2 SCs. Results from these combined studies show that growth selection appears to have altered the response to thermal stress through the Wnt/PCP pathway affecting poultry breast muscle growth (myofiber hypertrophy), structure, and protein to fat ratio (fat deposition).
As a ubiquitous intracellular signal, Ca2+ is vital in regulation of skeletal muscle development, maintenance and regulation through calcium signaling. Calcium regulation in SCs appears to be largely affected by heat stress and the Calcium signaling pathway ranked among the highest in IPA by z-score. The spatiotemporal pattern of Ca2+ signaling in developing muscle is dependent on stores of Ca2+ (Tu et al., 2016). Expression of the two major calcium channels inositol-triphosphate receptors (IP3R) and RYR (ryanodine receptors) are developmentally regulated. Expression of the IP3 receptor was not significantly altered by heat stress in the turkey SCs, but RYR3 was among the most highly upregulated genes. RYR3 is a peripheral calcium channel associated with the sarcoplasmic reticulum (SR). In mice, RYR3 has a distinct developmental expression profile with maximum expression in neonates followed by decline, most noticeable in fast-twitch muscles (Tarroni et al., 1997). Unlike RYR1, which is coupled to the voltage-gated calcium channel and is activated by depolarization, RYR3 is only susceptible to calcium-induced Ca release (CICR, not voltage-gated). Expression of the SR luminal calcium-binding protein calsequestrin (CASQ2) is also elevated. CASQ is responsible for storage of Ca2+ within the SR when the muscle is at rest, and appears to be involved in regulation of RYR1 and possibly RYR3 calcium release activity. Studies in turkeys suggest that splice variants of ryanodine receptors are altered by heat stress, which could affect postmortem calcium homeostasis and meat quality (Strasburg and Chiang, 2009).
Perez et al. (2005) used mouse dyspedic myotubes lacking genes for all ryanodine receptors (RYR1, RYR2, and RYR3) to investigate the effects of differential expression of RYR1 and RYR3. They showed that increasing expression of RYR3 resulted in increased cytosolic [Ca2+]. Because increased temperatures result in increased expression of RYR3, it is likely that will result in increased resting cytosolic calcium levels and increased gene transcription. Predicted upregulation of plasma membrane voltage-gated calcium channel would also likely increase cytosolic calcium via increased channel abundance although compensatory mechanisms may control the flow of Ca2+ through the channels. Also, a potential effector is CICR, releasing ions when Ca2+ levels reach a concentration threshold. Higher cytosolic Ca2+ levels activate calmodulin with downstream effects resulting in enhanced transcription leading to cell growth and development, consistent with the increased growth of the SC cells. The trends in the calcium signaling pathway were similar among the RBC2 and NCT lines but the magnitude of fold changes was greater in the commercially selected NCT birds. Modulation of calcium-regulating genes in early development could potentially be associated with aberrant postmortem Ca2+ regulation tied to meat quality defects in heat-stressed birds (Sporer et al., 2012).
Response to heat stress, particularly in NCT cells is reflected in gene expression supporting early myogenesis. Pathway analysis also highlighted increased in expression in myofibrillar proteins (e.g., troponin T, alpha-actin). Most of these genes appear to be of the fast muscle isoforms. Expression of the short transient receptor potential channel 3 (TRPC3) was down regulated (but only in the NCT cells). Ca2+ signaling through calcineurin via TRPCs has downstream phosphorylation of Ca2+-sensitive transcription factors (NFAT, nuclear factor of activated T cells) (Tu et al., 2016). Heat-stressed NCT SCs highly upregulated NFATC2 compared to control (Log2FC = 5.79) and expression was significantly greater in NCT cells compared to RBC2 cells at 43°C. In mice, deficiency of NFATC2 results in impaired myoblast fusion (Horsley et al., 2001). Expression of NFATC3 was also significantly higher in NCT SCs at 43°C (Log2FC = 2.07). Knockout of NFATC3 in early myogenesis results in reduced muscle mass (Kegley et al., 2001). Differential response of these transcription factors may be the result of selection for muscle growth and confirmation characteristics in commercial birds.
Conclusions
This study has identified genes and gene pathways in proliferating 1 week turkey SCs altered by phenotypic selection and heat stress. This RNAseq analysis provides a snapshot, along a continuum of gene expression changes that may translate into functional differences in gene products. Although these SCs are still proliferating, and are not yet differentiated into muscle fibers, this study provides clues into how SC activity, cellular fate, and ultimately muscle development are altered by heat stress and highlight cellular processes for future study. Heat-treated SCs respond with significant expression changes in several key signaling pathways and muscle-related structural genes. In general, SCs responded by upregulating muscle components with differential response from growth-selected birds in key signaling pathways including Wnt and calcium signaling. We hypothesize that heat stress causes higher resting cytosolic calcium levels in part due to changes in RYR3 and IP3R expression. Further studies will examine the downstream effects of these gene expression changes on cytosolic calcium in SCs and skeletal muscle of developing poults.
Data availability statement
The datasets presented in this study can be found in online repositories. The names of the repository/repositories and accession number(s) can be found below: https://www.ncbi.nlm.nih.gov/genbank/, PRJNA842679.
Author contributions
KR, SV, and GS conceived and designed the experiments. SV and KM performed the experiments. KR and KM analyzed the data. KR drafted the manuscript. All authors read and approved the final manuscript.
Funding
This work was financially supported by the United States Department of Agriculture, National Institute of Food Agriculture, AFRI competitive grant no. 2020-67015-30827 to GS, KR, and SV.
Acknowledgments
The authors thank Cindy Coy for technical assistance in culturing the satellite cells used in this study. Juan Abrahante, University of Minnesota Informatics Institute, assisted with data processing.
Conflict of interest
The authors declare that the research was conducted in the absence of any commercial or financial relationships that could be construed as a potential conflict of interest.
Publisher’s note
All claims expressed in this article are solely those of the authors and do not necessarily represent those of their affiliated organizations, or those of the publisher, the editors and the reviewers. Any product that may be evaluated in this article, or claim that may be made by its manufacturer, is not guaranteed or endorsed by the publisher.
Supplementary material
The Supplementary Material for this article can be found online at: https://www.frontiersin.org/articles/10.3389/fphys.2022.970243/full#supplementary-material
References
Andrews S. (2010). FastQC: A quality control tool for high throughput sequence data. Available at: http://www.bioinformatics.babraham.ac.uk/projects/fastqc/.
Asakura A., Komaki M., Rudnicki M. (2001). Muscle satellite cells are multipotential stem cells that exhibit myogenic, osteogenic, and adipogenic differentiation. Differentiation. 68, 245–253. doi:10.1046/j.1432-0436.2001.680412.x
Berrong S. L., Washburn K. W. (1998). Effects of genetic variation on total plasma protein, body weight gains, and body temperature responses to heat stress. Poult. Sci. 77, 379–385. doi:10.1093/ps/77.3.379
Bi P., Kuang S. (2012). Meat science and muscle biology symposium: Stem cell niche and postnatal muscle growth. J. Anim. Sci. 90, 924–935. doi:10.2527/jas.2011-4594
Bolger A. M., Lohse M., Usadel B. (2014). Trimmomatic: A flexible trimmer for illumina sequence data. Bioinformatics 30, 2114–2120. doi:10.1093/bioinformatics/btu170
Chiang W. A., Booren A., Strasburg G. (2008). The effect of heat stress on thyroid hormone response and meat quality in turkeys of two genetic lines. Meat Sci. 80, 615–622. doi:10.1016/j.meatsci.2008.02.012
Clark D. L., Coy C. S., Strasburg G. M., Reed K. M., Velleman S. G. (2016). Temperature effect on proliferation and differentiation of satellite cells from turkeys with different growth rates. Poult. Sci. 95, 934–947. doi:10.3382/ps/pev437
Eubelen M., Bostaille N., Cabochette P., Gauquier A., Tebabi P., Dumitru A. C., et al. (2018). A molecular mechanism for Wnt ligand-specific signaling. Science 361, eaat1178. doi:10.1126/science.aat1178
Halevy O., Krispin A., Leshem Y., McMurtry J. P., Yahav S. (2001). Early-age heat exposure affects skeletal muscle satellite cell proliferation and differentiation in chicks. Am. J. Physiol. Regul. Integr. Comp. Physiol. 281, R302–R309. doi:10.1152/ajpregu.2001.281.1.R302
Halevy O. (2020). Timing is everything - the high sensitivity of avian satellite cells to thermal conditions during embryonic and posthatch periods. Front. Physiol. 11, 235. doi:10.3389/fphys.2020.00235
Horesh D., Sapir T., Francis F., Wolf S. G., Caspi M., Elbaum M., et al. (1999). Doublecortin, a stabilizer of microtubules. Hum. Mol. Genet. 8, 1599–1610. doi:10.1093/hmg/8.9.1599
Horsley V., Friday B. B., Matteson S., Kegley K. M., Gephart J., Pavlath G. K., et al. (2001). Regulation of the growth of multinucleated muscle cells by an NFATC2-dependent pathway. J. Cell Biol. 153, 329–338. doi:10.1083/jcb.153.2.329
Hoving-Bolink A. H., Kranen R. W., Klont R. E., Gerritsen C. L., de Greef K. H. (2000). Fibre area and capillary supply in broiler breast muscle in relation to productivity and ascites. Meat Sci. 56, 397–402. doi:10.1016/s0309-1740(00)00071-1
Joiner K. S., Hamlin G. A., Lien A. R., Bilgili S. F. (2014). Evaluation of capillary and myofiber density in the pectoralis major muscles of rapidly growing, high-yield broiler chickens during increased heat stress. Avian Dis. 58, 377–382. doi:10.1637/10733-112513-Reg.1
Kegley K. M., Gephart J., Warren G. L., Pavlath G. K. (2001). Altered primary myogenesis in NFATC3(−/−) mice leads to decreased muscle size in the adult. Dev. Biol. 232, 115–126. doi:10.1006/dbio.2001.0179
Konarzewski M. A., Gavin A., McDevitt R., Wallis I. R. (2000). Metabolic and organ mass responses to selection for high growth rates in the domestic chicken (Gallus domesticus). Physiol. Biochem. Zool. 73, 237–248. doi:10.1086/316729
Krämer A., Green J., Pollard J., Tugendreich S. (2014). Causal analysis approaches in ingenuity pathway analysis. Bioinformatics 30, 523–530. doi:10.1093/bioinformatics/btt703
Lahmann I., Zhang Y., Baum K., Wolf J., Birchmeier C. (2021). An oscillatory network controlling self-renewal of skeletal muscle stem cells. Exp. Cell Res. 409, 112933. doi:10.1016/j.yexcr.2021.112933
Lazure F., Blackburn D. M., Corchado A. H., Sahinyan K., Karam N., Sharanek A., et al. (2020). Myf6/MRF4 is a myogenic niche regulator required for the maintenance of the muscle stem cell pool. EMBO Rep. 21, e49499. doi:10.15252/embr.201949499
Le Grand F., Jones A. E., Seale V., Scimè A., Rudnicki M. A. (2009). Wnt7a activates the planar cell polarity pathway to drive the symmetric expansion of satellite stem cells. Cell Stem Cell 4, 535–547. doi:10.1016/j.stem.2009.03.013
Love M. I., Huber W., Anders S. (2014). Moderated estimation of fold change and dispersion for RNA-seq data with DESeq2. Genome Biol. 15, 550. doi:10.1186/s13059-014-0550-8
Merrill A. H. (1983). Characterization of serine palmitoyltransferase activity in Chinese hamster ovary cells. Biochim. Biophys. Acta 754, 284–291. doi:10.1016/0005-2760(83)90144-3
Mi H., Muruganujan A., Thomas P. D. (2013). PANTHER in 2013: modeling the evolution of gene function, and other gene attributes, in the context of phylogenetic trees. Nucleic Acids Res. 41 (Database issue), D377–D386. doi:10.1093/nar/gks1118
Moss F., Leblond C. (1971). Satellite cells as the source of nuclei in muscles of growing rats. Anat. Rec. 170, 421–435. doi:10.1002/ar.1091700405
Mozdziak P., Schultz E., Cassens R. (1994). Satellite cell mitotic activity in posthatch Turkey skeletal muscle growth. Poult. Sci. 73, 547–555. doi:10.3382/ps.0730547
Nestor K. E., McCartney M. G., Bachev N. (1969). Relative contributions of genetics and environment to turkey improvement. Poult. Sci. 48, 1944–9.
Nestor K. E. (1977). Genetics of growth and reproduction in the Turkey. Poult. Sci. 56, 337–347. doi:10.3382/ps.0560337
Nestor K. E. (1984). Genetics of growth and reproduction in the Turkey. 9. Long-term selection for increased 16-week body weight. Poult. Sci. 63, 2114–2122. doi:10.3382/ps.0632114
Nikolaou N., Gathercole L. L., Marchand L., Althari S., Dempster N. J., Green C. J., et al. (2019). AKR1D1 is a novel regulator of metabolic phenotype in human hepatocytes and is dysregulated in non-alcoholic fatty liver disease. Metabolism. 99, 67–80. doi:10.1016/j.metabol.2019.153947
Otto A., Schmidt C., Luke G., Allen S., Valasek P., Muntoni F., et al. (2008). Canonical Wnt signalling induces satellite-cell proliferation during adult skeletal muscle regeneration. J. Cell Sci. 121, 2939–2950. doi:10.1242/jcs.026534
Patael T., Piestun Y., Soffer A., Mordechay S., Yahav S., Velleman S. G., et al. (2019). Early posthatch thermal stress causes long-term adverse effects on pectoralis muscle development in broilers. Poult. Sci. 98, 3268–3277. doi:10.3382/ps/pez123
Perez C. F., López J. R., Allen P. D. (2005). Expression levels of RyR1 and RyR3 control resting free Ca2+ in skeletal muscle. Am. J. Physiol. Cell Physiol. 288, C640–C649. doi:10.1152/ajpcell.00407.2004
Picard C. A., Marcelle C. (2013). Two distinct muscle progenitor populations coexist throughout amniote development. Dev. Biol. 373, 141–148. doi:10.1016/j.ydbio.2012.10.018
Piestun Y., Patael T., Yahav S., Velleman S. G., Halevy O. (2017). Early posthatch thermal stress affects breast muscle development and satellite cell growth and characteristics in broilers. Poult. Sci. 96, 2877–2888. doi:10.3382/ps/pex065
Potthoff M. J., Kliewer S. A., Mangelsdorf D. J. (2012). Endocrine fibroblast growth factors 15/19 and 21: From feast to famine. Genes Dev. 26, 312–324. doi:10.1101/gad.184788.111
Powell D. J., Velleman S. G., Cowieson J., Singh M., Muir W. I. (2016). Influence of hatch time and access to feed on intramuscular adipose tissue deposition in broilers. Poult. Sci. 95, 1449–1456. doi:10.3382/ps/pew063
Reed K. M., Mendoza K. M., Strasburg G. M., Velleman S. G. (2017b). Response of Turkey muscle satellite cells to thermal challenge. II. Transcriptome effects in differentiating cells. Front. Physiol. 8, 948. doi:10.3389/fphys.2017.00948
Reed K. M., Mendoza K. M., Abrahante J. E., Barnes N. E., Velleman S. G., Strasburg G. M., et al. (2017a). Response of Turkey muscle satellite cells to thermal challenge. I. Transcriptome effects in proliferating cells. BMC Genomics 18, 352. doi:10.1186/s12864-017-3740-4
Smith J. H. (1963). Relation of body size to muscle cell size and number in the chicken. Poult. Sci. 42, 283–290. doi:10.3382/ps.0420283
Sporer K. R. B., Zhuo H. R., Linz J. E., Booren A. M., Strasburg G. M. (2012). Differential expression of calcium-regulating genes in heat-stressed Turkey breast muscle is associated with meat quality. Poult. Sci. 91, 1418–1424. doi:10.3382/ps.2011-02039
Strasburg G. M., Chiang W. (2009). Pale, soft, exudative Turkey - the role of ryanodine receptor variation in meat quality. Poult. Sci. 88, 1497–1505. doi:10.3382/ps.2009-00181
Su X., Zhu G., Ding X., Lee S. Y., Dou Y., Zhu B., et al. (2014). Molecular basis underlying histone H3 lysine-arginine methylation pattern readout by Spin/Ssty repeats of Spindlin1. Genes Dev. 28, 622–636. doi:10.1101/gad.233239.113
Tarroni P., Rossi D., Conti A., Sorrentino V. (1997). Expression of the ryanodine receptor type 3 calcium release channel during development and differentiation of mammalian skeletal muscle cells. J. Biol. Chem. 272, 19808–19813. doi:10.1074/jbc.272.32.19808
Tu M. K., Levin J. B., Hamilton A. M., Borodinsky L. N. (2016). Calcium signaling in skeletal muscle development, maintenance and regeneration. Cell Calcium 59, 91–97. doi:10.1016/j.ceca.2016.02.005
Velleman S. G., Anderson J. W., Coy C. S., Nestor K. E. (2003). Effect of selection for growth rate on muscle damage during Turkey breast muscle development. Poult. Sci. 82, 1069–1074. doi:10.1093/ps/82.7.1069
Veleman S. G., Liu X., Nestor K. E., McFarland D. C. (2000). Heterogeneity in growth and differentiation characteristics in male and female satellite cells isolated from Turkey lines with different growth rates. Comp. Biochem. Physiol. A Mol. Integr. Physiol. 125, 503–509. doi:10.1016/s1095-6433(00)00178-1
von Maltzahn J., Jones A. E., Parks R. J., Rudnicki M. A. (2013). Pax7 is critical for the normal function of satellite cells in adult skeletal muscle. Proc. Natl. Acad. Sci. U. S. A. 110, 16474–16479. doi:10.1073/pnas.1307680110
Wilson B., Nieberg P., Buhr R., Kelly B., Shultz F. (1990). Turkey muscle growth and focal myopathy. Poult. Sci. 69, 1553–1562. doi:10.3382/ps.0691553
Xu J., Strasburg G. M., Reed K. M., Velleman S. G. (2021b). Effect of temperature and selection for growth on intracellular lipid accumulation and adipogenic gene expression in Turkey pectoralis major muscle satellite cells. Front. Physiol. 12, 667814. doi:10.3389/fphys.2021.667814
Xu J., Strasburg G. M., Reed K. M., Velleman S. G. (2021a). Response of Turkey pectoralis major muscle satellite cells to hot and cold thermal stress: Effect of growth selection on satellite cell proliferation and differentiation. Comp. Biochem. Physiol. A Mol. Integr. Physiol. 252, 110823. doi:10.1016/j.cbpa.2020.110823
Xu J., Strasburg G. M., Reed K. M., Velleman S. G. (2022). Temperature and growth selection effects on proliferation, differentiation, and adipogenic potential of Turkey myogenic satellite cells through frizzled-7-mediated Wnt planar cell polarity pathway. Front. Physiol. 13, 892887. doi:10.3389/fphys.2022.892887
Keywords: satellite cell, proliferation, skeletal muscle, growth selection, Turkey
Citation: Reed KM, Mendoza KM, Strasburg GM and Velleman SG (2022) Transcriptome response of proliferating muscle satellite cells to thermal challenge in commercial turkey. Front. Physiol. 13:970243. doi: 10.3389/fphys.2022.970243
Received: 15 June 2022; Accepted: 15 July 2022;
Published: 25 August 2022.
Edited by:
Paul Siegel, Virginia Tech, United StatesReviewed by:
Shannon Carroll, Harvard Medical School, United StatesKi Ho Park, The Ohio State University, United States
Copyright © 2022 Reed, Mendoza, Strasburg and Velleman. This is an open-access article distributed under the terms of the Creative Commons Attribution License (CC BY). The use, distribution or reproduction in other forums is permitted, provided the original author(s) and the copyright owner(s) are credited and that the original publication in this journal is cited, in accordance with accepted academic practice. No use, distribution or reproduction is permitted which does not comply with these terms.
*Correspondence: Kent M. Reed, a21yZWVkQHVtbi5lZHU=