- 1Department of Internal Medicine, University of Chicago Medicine, Chicago, IL, United States
- 2Section of Cardiology, Center for Arrhythmia Care, University of Chicago Medicine, Chicago, IL, United States
Cardiac resynchronization therapy (CRT) via biventricular pacing (BiVP) is an established treatment for patients with left ventricular systolic heart failure and intraventricular conduction delay resulting in wide QRS. Seminal trials demonstrating mortality benefit from CRT were conducted in patients with wide left bundle branch block (LBBB) pattern on electrocardiogram (ECG) and evidence of clinical heart failure. The presence of conduction block was assumed to correlate with commonly applied criteria for LBBB. More recent data has challenged this assertion, revealing that LBBB pattern may include distinct underlying pathophysiology, including patients with complete conduction block, either at the left-sided His fibers or the proximal left bundle, intact Purkinje activation with wide LBBB-like QRS, and patients demonstrating both proximal block and distal delay. Currently, BiVP-CRT is indicated for all QRS duration ≥150 ms and may be considered for BBB patterns from 130 to 149 ms with robust clinical data to support its use. Despite this, however, there remains a significant number of non-responders to BVP. Conduction system pacing (CSP) has emerged as an alternative approach to deliver CRT and correct QRS in patients with conduction block. Newer hybrid approaches which combine CSP and traditional BiVP-CRT and may hold promise for patients with IP or mixed-level block. As various approaches to CRT continue to be studied, physiologic phenotyping of the LBBB pattern remains an important consideration.
Introduction
For over a century, left bundle branch block (LBBB) has been recognized as a clinical entity. The clinical significance of LBBB has changed over the years from being first perceived as a non-harmful electrocardiogram (ECG) finding to more recently being associated with poorer prognosis, particularly in patients with severe, symptomatic heart failure (Master et al., 1940; Tabrizi et al., 2007). Cardiac resynchronization therapy (CRT) via biventricular pacing (BiVP) has been established to reduce mortality in patients with electrical dyssynchrony and left ventricular systolic dysfunction (Moss et al., 2009; Zareba et al., 2011; Gold et al., 2012; Goldenberg et al., 2014). Large clinical trials have shown that the presence of LBBB on surface ECG is one of the best predictors of CRT response (Linde et al., 2008; Moss et al., 2009). Multiple guidelines have adopted this definition and emphasized presence of LBBB as a criterion when selecting patients for CRT (Tracy et al., 2012; Glikson et al., 2021; Allaw et al., 2022). However, despite this, there remains a significant percentage of patients who do not experience reduction in morbidity or mortality from CRT (Mullens et al., 2009). Recent observations of suggest that the LBBB pattern recognized on surface ECG may distinct underlying pathophysiology: complete conduction block through the left bundle of the His-Purkinje system, wide LBBB-like QRS due to left ventricular hypertrophy or diffuse fibrosis leading to interventricular conduction delay with preserved His-Purkinje activation (IVCD with IPA), or a combination of both proximal block and concomitant distal disease (Upadhyay et al., 2019a; Tung and Upadhyay, 2020). CRT response has been shown to depend on the mechanism of the bundle branch block (Caputo et al., 2018; Jastrzębski et al., 2018). Conduction system pacing (CSP) and hybrid pacing are emerging strategies for CRT but may not be suitable for all types of LBBB patterns.
How to define left bundle branch block?
Surface ECG
Most definitions of LBBB are based on surface ECG patterns. The American Heart Association (AHA), American College of Cardiology (ACC), and Heart Rhythm Society (HRS) collaborated on the currently adopted definition in 2009 and was restated in 2018 (Surawicz et al., 2009; Kusumoto et al., 2019). By their definition, the LBBB pattern is defined by a QRS duration ≥120 ms with a broad notched or slurred R-wave in leads I, aVL, V5, and V6; delayed time to R-wave peak; absent Q waves; ST segment and T-wave changes. Importantly, the precise definition of some of the components of this definition, including the assessing timing of slurs or notches, remains subjective.
In 2011, the landmark Multicenter Automatic Defibrillator Implantation with Cardiac Resynchronization Therapy (MADIT-CRT) trial suggested that patients with reduced ejection fraction <30% and prolonged QRS >130 ms derived significant benefit in composite heart failure events and death from CRT-D compared to ICD if they had a LBBB pattern compared to non-LBBB (i.e.,: right bundle branch block or nonspecific interventricular conduction delay) (Goldenberg et al., 2014). Notably, the study was underpowered to assess death alone as there was a trend but no significance in subgroup analysis. The MADIT-CRT study altered the definition of LBBB pattern and incorporated the presence of a QS or rS pattern in lead V1 and removed the criteria of delayed R-wave peak and ST segment and T-wave changes (Zareba et al., 2011). This definition was adopted by the European Society of Cardiology (ESC) in 2013 and incorporated into their guidelines (Glikson et al., 2021; Brignole et al., 2013).
Strauss and colleagues in 2011 observed a growing body of evidence that CRT had greatest benefit in patients with LBBB due to conduction block rather than hypertrophy, underscoring the importance of accurate patient identification. By reviewing serial ECGs and with the understanding that hypertrophy was a gradual process whereas conduction block was an abrupt process, the authors proposed a modification to the LBBB criteria. What later was known as the Strauss criteria, they suggested that LBBB be more stringently defined as a QRS duration of ≥130 ms in women and ≥140 ms in men; a QS or rS pattern in lead V1 and V2; and mid-QRS notching or slurring in at least two of the leads V1, V2, V5, V6, I, and aVL (Strauss et al., 2011).
When compared systematically in a large series of patients, however, no single set of criteria was superior at identifying response to CRT (van Stipdonk et al., 2020). Given the absence of a “gold standard” for determining whether a conduction block is present or not, there may be inherent limitations to the use of surface ECG in predicting patient response to CRT (Perrin et al., 2012; Caputo et al., 2018; Jastrzębski et al., 2018). Indeed, up to one third of the patients who meet ACC/AHA/HRS criteria for LBBB demonstrated intact activation of their ventricles via the His-Purkinje system (Auricchio et al., 2004).
Intracardiac electroanatomic mapping
While distinguishing LBBB due to conduction block versus IVCD with IPA may be challenging on surface ECG, intracardiac recordings of ventricular activation patterns can more readily distinguish these two entities. In 1984, Vassallo et al., used endocardial catheter mapping on patients with LBBB on surface ECG and concluded that left ventricular endocardial activation was heterogenous (Vassallo et al., 1984). Several decades later in 2003, Rodriguez et al., used 3D endocardial mapping systems and observed 2 types of septal activation patterns in patients with LBBB and heart failure (Rodriguez et al., 2003). Auricchio and colleagues utilized non-contact mapping to identify patterns of ventricular activation in patients with LBBB pattern, and similarly concluded that transseptal conduction was normal in one-third (Auricchio et al., 2004). Subsequently, Derval et al., used high density electroanatomic mapping to demonstrate that patients with LBBB had distinct left ventricular activation patterns compared to patients with nonspecific intraventricular conduction delay (Derval et al., 2017). More recently, Upadhyay and colleagues employed multielectrode mapping catheters to assess left-sided septal activation among 72 patients with LBBB. They found that the mechanism of LBBB pattern was due to complete conduction block (CCB) within the proximal left conduction system in 64% of patients, while intact Purkinje activation (IPA)—a finding usually attributed to IVCD—was noted in the remaining 36%. Importantly, the population studied included patients both referred for conventional device indications along with those referred for electrophysiology (EP) study in the treatment of ventricular tachycardia, and therefore may have included patients more advanced disease (Upadhyay et al., 2019a). Regardless, the conclusion from these studies is that determining conduction block from surface ECG alone remains limited.
While assessing LBBB via intracardiac mapping remains the most rigorous modality to assess for CCB, it is generally only utilized in patients who already have another indication for EP study. It is unclear whether routinely performing left-sided septal mapping as an isolated diagnostic study in the absence of concomitant indications is justified due to the incremental risks of arterial access. The risk profile of left ventricular septal mapping is likely comparable to routine diagnostic coronary angiography with left ventricular pressure assessment. An additional barrier may be the justification of economic costs and time associated with EP study to guide CRT implant. Presently, there are no published guidelines to suggest which patient populations would most benefit from invasive studies to further clarify their electrical activation pattern.
Transthoracic echocardiogram
One of the main hypotheses as to why CRT improves mortality in patients with heart failure is because LBBB causes left ventricular (LV) dyssynchrony thus leading to unfavorable cardiac remodeling. Thus, transthoracic echocardiogram (TTE) has been used as a non-invasive tool to visually assess for LV dyssynchrony which are correlated with conduction block from complete LBBB. There are a number of TTE patterns which are indicative of this degree of LV dyssynchrony including septal flash or septal beak (the interventricular septum [IVS] rapidly and briefly moves leftward before or during ejection); septal systolic rebound stretch (IVS elongates during early systole); apical rocking (apex moves towards septum during early contraction and then swings to the lateral wall during late contraction); LV lateral wall hypertrophy with late contraction; or short filling and ejection times with a longer isovolumic phase due to late aortic valve opening (DILLON et al., 1974; Voigt, 2015; Walmsley et al., 2016). Contraction pattern assessment by 2-dimensional strain echocardiography to assess for typical LBBB mechanical activation has been shown to improve selection of CRT responders when used in conjunction with surface ECG (Risum et al., 2015). Tissue doppler index and 3D approaches have also been studied to assess for LV dyssynchrony (Dohi et al., 2005; Marsan et al., 2008; Van de Veire et al., 2008; Surkova et al., 2017). It has been shown that TTE patterns of LV dyssynchrony may play a role in identifying CRT responders, and correction of these patterns after CRT is independently associated with lower all-cause mortality and reverse LV remodeling (Stankovic et al., 2016). In fact, two randomized trials showed that echo guided placement of the LV lead to areas of imaged dyssynchrony improved rates of death and HF hospitalization (Khan et al., 2012; Saba et al., 2013).
There are several ‘mimics’ to LBBB induced LV dyssynchrony on TTE, particularly for septal flash. These ‘mimics’ include coronary artery disease, post-cardiac surgery, right ventricular dysfunction, severe mitral stenosis, pericardial disease, other conduction system abnormalities (e.g., ventricular pacing, pre-excitation, premature contractions), or extra-cardiac posterior compression (e.g., hiatal hernia, pregnancy, ascites) (Surkova et al., 2017). Additionally, the presence of these patterns may be difficult to elucidate, particularly in cases of borderline dyssynchrony. Finally, while TTE patterns of dyssynchrony may be valuable visual representations of underlying physiology, there has been no study to day showing that TTE patterns of dyssynchrony predict response to CRT. In fact, the presence of LV dyssynchrony alone, in the absence of wide QRS, does not predict CRT response. This was shown in the 2013 EchoCRT trial which randomized 809 patients with QRS < 130 ms and TTE evidence of LV dyssynchrony (Ruschitzka et al., 2013). The trial was terminated early due to increased mortality observed in the patients randomized to CRT, and this observation of harm from BiVP in narrow QRS patients is perhaps one of the most important findings reported among CRT trials in the last decade.
Emerging modalities
Given the interest in accurately defining LBBB to identify patients who would benefit from CRT, there are several emerging modalities presently being explored. The use of ultra-high frequency ECG (UHF-ECG) has shown early promise in increasing accuracy of patient selection for CRT. UHF-ECG utilizes much higher acquisition frequencies to allow for a much more detailed understanding of electrical activity during ventricular depolarization (corresponding to the QRS complex seen on standard 12-lead ECG, along with additional recording at sites V7, V8, and V9) (Jurak et al., 2017; Jurak et al., 2020). The main limitations of UHF-ECG include the requirement of new hardware and lack of validation against intracardiac assessment of LBBB.
Another area of active interest is the use of non-invasive body surface mapping to improve accuracy in identifying ventricular dyssynchrony beyond QRS duration and morphology. Ploux and collegues studied the use of non-invasive mapping in 2013 and found ventricular electrical uncoupling on body mapping predicted clinical CRT response better than presence of LBBB on standard surface ECG (Ploux et al., 2013). Similarly, other groups are studying machine learning algorithms that may be harnessed to identify surface ECG waveforms beyond LBBB morphology and QRS duration which may better predict CRT response (Feeny et al., 2020). Recently, QRS area has been shown to be associated with clinical and echocardiographic response to CRT (van Stipdonk et al., 2018; Emerek et al., 2019). Okafor et al. even found that QRS area was superior to QRS duration and QRS morphology in predicting mortality after CRT (Okafor et al., 2019).
Advanced cardiac imaging technique have also been explored as non-invasive ways to better characterize LV dyssynchrony. Single photon emission computed tomography (SPECT) and 18F-fluorodeoxyglucose positron emission tomography (PET) studies have demonstrated that LBBB results in relative reduction in coronary flow to the septum and hyperperfusion to the lateral wall (Koepfli et al., 2009; Claridge et al., 2015). Other techniques such as quantitative gated SPECT have shown to feasibly demonstrate LV dyssynchrony and predict CRT response with a sensitivity of 83% and a specificity of 81% (Boogers et al., 2009). Similar to TTE, cardiac magnetic resonance imaging (CMR) can be utilized to assess for mechanical LV dyssynchrony using strain patterns, timining of myocardial thickness, and volume changes. CMR has superior imaging resolution as compared to TTE and can additionally provide information on the extent of myocardial scar and detailed anatomy of the coronary veins, both important in the planning of CRT (Sohal et al., 2014; Surkova et al., 2017). The major advantages and limitations of each modality is outlined in Table 1.
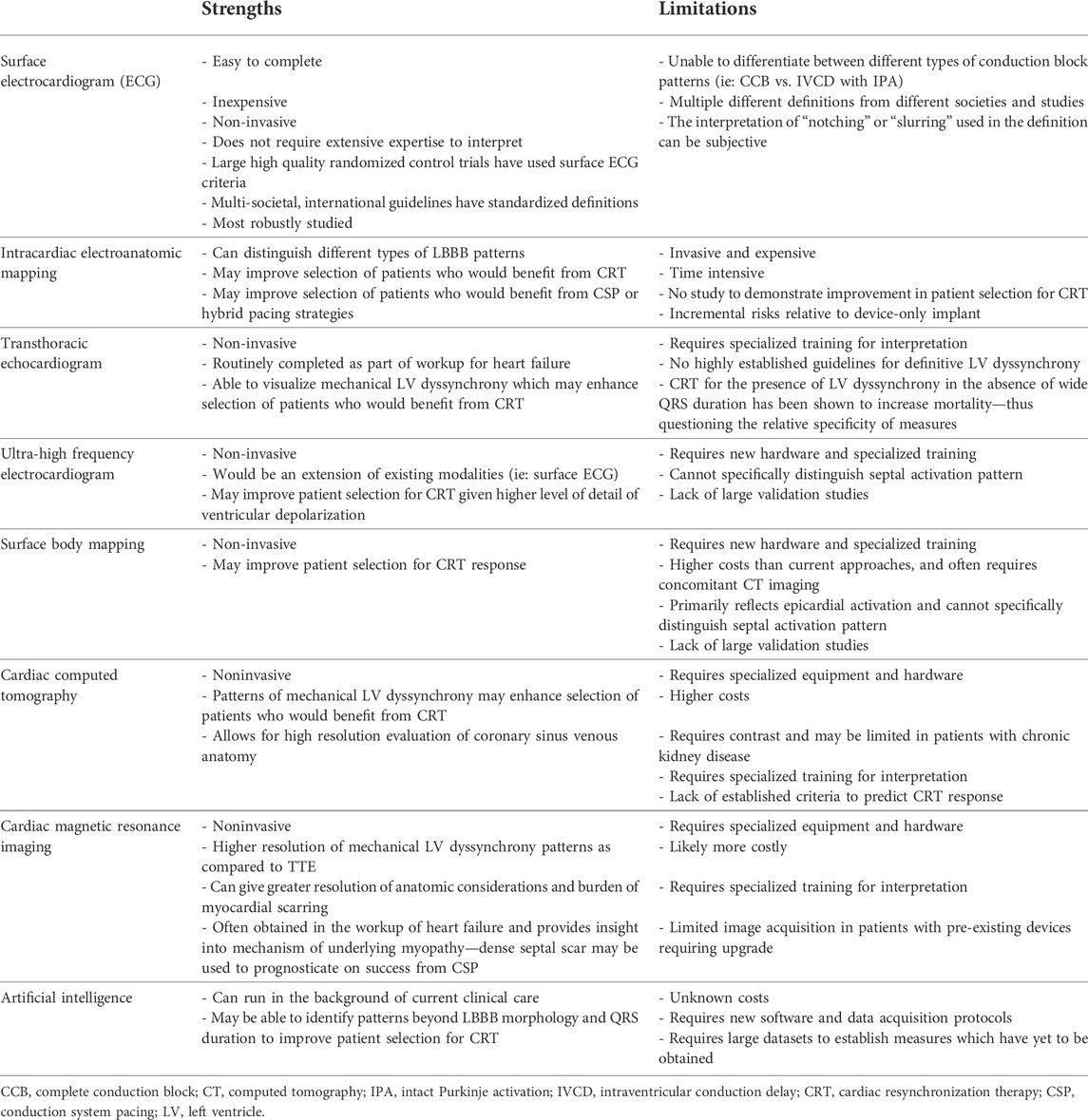
TABLE 1. Strength and limitations of different modalities to characterize left bundle branch block (LBBB) pattern.
Anatomic considerations of LBBB
Intracardiac electroanatomic mapping in patients with LBBB patterns on surface ECG have found heterogenous activation sequences of His-Purkinje conduction, ranging from CCB to IVCD with IPA (Figure 1) (Upadhyay et al., 2019a). CCB comprises of proximal block at the level of the left-sided His fibers (“intrahisian”) and slightly more distal block at the level of left-sided Purkinje fibers (“true left bundle branch block”). In patients who have wide QRS durations but intact His-Purkinje activation on intracardiac mapping, it is hypothesized that the wide QRS duration is from diffuse myopathy, mostly from hypertrophy or fibrosis. It is believed that both processes may be present simultaneously as well (“mixed block”).
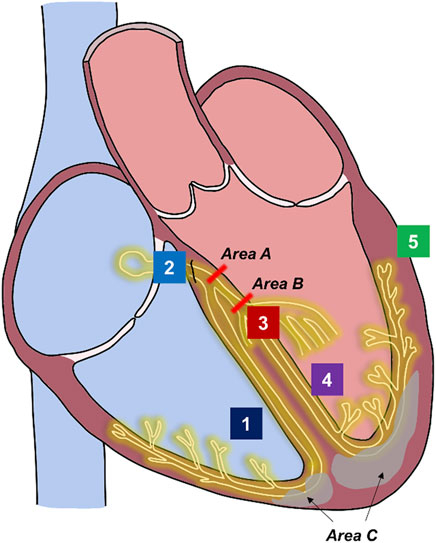
FIGURE 1. Sites of conduction block in left bundle block pattern and sites of pacing for cardiac resynchronization therapy. Underlying pathophysiology of left bundle block pattern. Course of His-Purkinje system is shown in yellow. Fibrosis within the myocardium in illustrated in gray. Complete conduction block (His fibers or proximal left-sided conduction): areas A or B. Interventricular conduction delay from hypertrophy or fibrosis with intact Purkinje activation: area C. Mixed or multi-level block: areas A + area B + area C. Possible pacing sites for cardiac resynchronization therapy. Site 1: Right Ventricular endocardium pacing (lead tip does not reach conduction system). Site 2: His Bundle pacing (lead tip at or adjacent to His fibers). Site 3: Left bundle branch area pacing (lead tip at or adjacent to left-sided conduction system fibers). Site 4: Left septal pacing (lead tip at left ventricular endocardial surface). Site 5: Left ventricular epicardial pacing. Biventricular pacing: Site 1 and Site 5 or fusion with right bundle activation. Conduction system pacing: Site 2 OR Site 3. Site 4 may also engage fast fibers at the left ventricular endocardial surface. His-optimized cardiac resynchronization therapy: Site 2 in combination with Site 5. Left bundle branch optimized cardiac resynchronization therapy: Site 3 in combination with Site 5.
Cardiac resynchronization therapy approaches based on LBBB anatomic site
Biventricular pacing
CRT for patients with heart failure and LBBB is achieved through placement of both a right ventricular endocardial lead and a left ventricular epicardial lead that is located in the coronary sinus (Figure 1). BiVP is the most robustly studied approach to CRT with multiple large, randomized control trials showing consistent clinical and mortality benefit in patients with severe, clinical heart failure and wide QRS durations with LBBB patterns. The Multisite Stimulation in Cardiomyopathies (MUSTIC), Pacing Therapies in Congestive Heart Failure (PATH-HF), and Multicenter InSync Randomized Clinical Evaluation (MIRACLE) trials found that CRT compared to no CRT improved quality of life metrics (Abraham et al., 2002; Auricchio et al., 2002; Linde et al., 2002). The Comparison of Medical Therapy, Pacing and Defibrillation in Heart Failure (COMPANION) trial, the Cardiac Resynchronization-Heart Failure (CARE-HF) study, the Multicenter Automatic Defibrillator Implantation Trial with Cardiac Resynchronization Therapy (MADIT-CRT), and the Resynchronization/Defibrillation for Ambulatory Heart Failure (RAFT) trial found that CRT reduced death and hospitalizations (Bristow et al., 2004; Cleland et al., 2005; Cleland et al., 2006; Moss et al., 2009; Tang et al., 2010).
Taken together, these landmark clinical trials have firmly established CRT via BiVP as the standard of care for patients with poor systolic function and a wide QRS duration on surface ECG. This is reflected in multi-societal guidelines which give a class I indication for CRT in this subset of patients (Kusumoto et al., 2019; Glikson et al., 2021). Currently, the use of BiVP for CRT should be considered in all patients with LBBB pattern on surface ECG, regardless of the underlying anatomic physiology of LBBB given the numerous large, randomized controlled trials showing significant clinical benefit. With that noted, roughly 30% of patients who undergo CRT do not improve after therapy (Auricchio and Prinzen, 2011). The high number of CRT “non-responders” to BiVP led some experts to consider other locations for pacing, such as CSP and hybrid pacing.
Conduction system pacing
CRT via CSP relies on inserting a LV lead into IVS to capture in the area of the His bundle (“His bundle pacing”, HBP) or to the left bundle of the Purkinje fibers (“left bundle branch area pacing”, LBBAP) (Figure 1). CSP attempts to counteract cardiac dyssynchrony by capturing sites distal to proximal block to re-engage the native His-Purkinje system and thus shorten QRS. The feasibility of HBP in lieu of an LV lead was shown in 2017 by Ajijola and collegues who successfully completed His-CRT in 16 of the 21 (76%) enrolled patients (Ajijola et al., 2017). Significant improvements in LV ejection fraction (LVEF) and functional class were noted. The His-SYNC pilot study was the first prospective, multi-center, single-blinded randomized, controlled trial to evaluate HBP compared to BiVP for CRT. Among patients with HF who met class I or II guideline indications for CRT, both HBP and BiVP showed improvement in LVEF at 6 months but were not significantly different between the two groups. HBP did significantly reduce QRS duration when compared to BiVP. Importantly, the trial revealed high rates of cross-over as a limitation to HBP as a means to deliver CRT, and that patients with IVCD on surface ECG could not be corrected with HBP (Upadhyay et al., 2019b; Upadhyay et al., 2019c). In the largest trial to date, the His-Alternative Study randomized patients with NYHA class II-IV heart failure symptoms, LVEF ≤ 35%, and LBBB pattern by Strauss’ criteria to HBP versus BiVP (Vinther et al., 2021), and reported results which were in line with the His-SYNC pilot. Similarly, intention-to-treat showed no difference between HBP and BiVP, but per-protocol analysis demonstrated that HBP treated patients had significantly higher LVEF compared to BiVP at 6 months. Rates of procedural success were higher as patients with IVCD were excluded. Improvement in clinical and physical outcomes were similar between groups.
More recently, left bundle branch area pacing (LBBAP) was reported as a novel means to pursue CSP, with reduced procedural complexity and more stable capture thresholds than HBP (Huang et al., 2017). LBBAP as an alternative for HBP was shown by Huang and colleagues in 2020 who successfully implemented LBBAP in 97% of patients with non-ischemic cardiomyopathy, complete LBBB, LVEF ≤ 50% with a CRT indication or who failed to achieve acceptable HBP thresholds (Huang et al., 2020). There was a significant improvement in LVEF and NYHA functional class and 75% achieved normal LVEF (≥ 50%) at 1 year follow-up. The LBBAP Collaborative Study Group retrospectively analyzed over 300 patients who underwent LBBAP and found significant improvement in LVEF with 72% of patients having a clinical response and 73% of patients having an echocardiographic response (Vijayaraman et al., 2021). Finally, LBBAP was compared to HBP and BiVP in a single center, prospective observation study of 127 patients with LVEF ≤ 40%, LBBB, and a CRT indication (Wu et al., 2021). LBBAP improved LVEF and NYHA functional class at a significantly higher rate than BiVP but inferences are limited given the lack of randomization.
While the results of CSP appear promising, there remains a lack of high quality, large, randomized, control trials with blinded assessment of key endpoints and an emphasis on clinical outcomes. Notably, the majority of data reported on CSP have explored echocardiographic endpoints. Given the anatomic consideration of LBBB, it is also worth noting that CSP is likely not appropriate for patients with IPA. Upadhyay et al. found that no patients with IPA had correction of wide QRS duration with HBP (Upadhyay et al., 2019a). This has led some experts to advocate that intracardiac mapping should be employed for patients undergoing CSP. If intracardiac mapping reveals that the patient has IVCD with IPA, then it is argued that CSP should not be used. This would ultimately help to refine patient selection of CSP with the potential to reduce the high cross-over numbers seen in previous RCTs (Tung and Upadhyay, 2020). It is worth noting that BiVP overcomes the anatomic considerations of the LBBB and IPA as it paces directly on the LV epicardium. While there are limited early data suggesting that CSP may have a role in treating nonresponse to BiV nonresponse to CSP is a salient concern which remains to be characterized (Vijayaraman et al., 2022).
Hybrid pacing
Hybrid methods of pacing have been developed which use sequential or simultaneous fusion of CSP pacing with traditional coronary sinus leads. His-optimized cardiac resynchronization therapy (HOT-CRT) or left bundle branch optimized cardiac resynchronization therapy (LOT-CRT) use a LV epicardial lead with the addition of a His-bundle lead or a left bundle area lead, respectively, to achieve the shortest QRS width. The first experience of HOT-CRT was reported in 2019 by the HIS-Sync Investigators in patients whom successful QRS correction could not be achieved with HBP alone (Upadhyay et al., 2019c). HOT-CRT was successfully implemented in 25 of 27 patients and clinical response was noted in 84% of patients and an echocardiographic response in 92% of patients. Zweeink et al. found that HOT-CRT decreased LV activation time to a significantly greater extent than HBP or BiV pacing alone (Zweerink et al., 2021). LOT-CRT was first reported on by Jastrzębski et al., in 2021 who found that there was improvement in LVEF and reduction in NYHA function class at 3-months follow-up (Jastrzębski et al., 2022). It is also worth noting that in the study, LOT-CRT reduced QRS duration to a greater extent than BiVP or LBBAP. Hybrid methods of pacing appear to show early promise, particularly for patients with mixed block or conduction block on multiple levels.
Conclusion
Intracardiac EP studies of patients with wide QRS duration and LBBB pattern on surface ECG have demonstrated three distinct entities: CCB, IVCD with IPA, or mixed blocks. The heterogeneity of the underlying pathophysiology of LBBB pattern on surface ECG has pushed investigators to study other forms of assessing LV dyssynchrony or CRT response such as intracardiac recordings, TTE, UHF-ECG, SPECT, and CMR. The highest quality data support CRT via BiVP for patients with clinical heart failure and wide QRS durations. Alternative pacing strategies such as CSP, HOT-CRT, and LOT-CRT show early clinical promise but have yet to show improvement in hard clinical outcomes compared to BiVP. CSP should be reserved for patients with CCB while hybrid models of pacing may be suitable for patients with IPA or mixed levels of conduction block.
Author contributions
NK contributed to writing the manuscript and creation of figures and tables. GU contributed to writing the manuscript, editing the manuscript, and editing the figures and tables.
Conflict of interest
The authors declare that the research was conducted in the absence of any commercial or financial relationships that could be construed as a potential conflict of interest.
Publisher’s note
All claims expressed in this article are solely those of the authors and do not necessarily represent those of their affiliated organizations, or those of the publisher, the editors and the reviewers. Any product that may be evaluated in this article, or claim that may be made by its manufacturer, is not guaranteed or endorsed by the publisher.
References
Abraham W. T., Fisher W. G., Smith A. L., Delurgio D. B., Leon A. R., Loh E., et al. (2002). Cardiac resynchronization in chronic heart failure. N. Engl. J. Med. 346, 1845–1853. doi:10.1056/NEJMoa013168
Ajijola O. A., Upadhyay G. A., Macias C., Shivkumar K., Tung R. (2017). Permanent His-bundle pacing for cardiac resynchronization therapy: Initial feasibility study in lieu of left ventricular lead. Heart rhythm. 14, 1353–1361. doi:10.1016/j.hrthm.2017.04.003
Allaw A. B., Mittal S., Merchant F. M., Besser S. A., Beaser A. D., Aziz Z., et al. (2022). Population-level impact of the guidelines update on patient selection and outcomes after cardiac resynchronization therapy. JACC. Clin. Electrophysiol. 8, 651–661. doi:10.1016/j.jacep.2022.01.026
Auricchio A., Fantoni C., Regoli F., Carbucicchio C., Goette A., Geller C., et al. (2004). Characterization of left ventricular activation in patients with heart failure and left bundle-branch block. Circulation 109, 1133–1139. doi:10.1161/01.CIR.0000118502.91105.F6
Auricchio A., Prinzen F. W. (2011). Non-responders to cardiac resynchronization therapy: The magnitude of the problem and the issues. Circ. J. 75, 521–527. doi:10.1253/circj.cj-10-1268
Auricchio A., Stellbrink C., Sack S., Block M., Vogt J., Bakker P., et al. (2002). Long-term clinical effect of hemodynamically optimized cardiac resynchronization therapy in patients with heart failure and ventricular conduction delay. J. Am. Coll. Cardiol. 39, 2026–2033. doi:10.1016/s0735-1097(02)01895-8
Boogers M. M., Van Kriekinge S. D., Henneman M. M., Ypenburg C., Van Bommel R. J., Boersma E., et al. (2009). Quantitative gated SPECT-derived phase analysis on gated myocardial perfusion SPECT detects left ventricular dyssynchrony and predicts response to cardiac resynchronization therapy. J. Nucl. Med. 50, 718–725. doi:10.2967/jnumed.108.060657
Brignole M., Auricchio A., Baron-Esquivias G., Bordachar P., Boriani G., Breithardt O.-A., et al. (2013). ESC Guidelines on cardiac pacing and cardiac resynchronization therapy: The Task Force on cardiac pacing and resynchronization therapy of the European Society of Cardiology (ESC). Developed in collaboration with the European Heart Rhythm Association (EHRA). EP Eur. 15, 1070–1118.
Bristow M. R., Saxon L. A., Boehmer J., Krueger S., Kass D. A., De Marco T., et al. (2004). Cardiac-resynchronization therapy with or without an implantable defibrillator in advanced chronic heart failure. N. Engl. J. Med. 350, 2140–2150. doi:10.1056/NEJMoa032423
Caputo M. L., van Stipdonk A., Illner A., D'Ambrosio G., Regoli F., Conte G., et al. (2018). The definition of left bundle branch block influences the response to cardiac resynchronization therapy. Int. J. Cardiol. 269, 165–169. doi:10.1016/j.ijcard.2018.07.060
Claridge S., Chen Z., Jackson T., Sammut E., Sohal M., Behar J., et al. (2015). Current concepts relating coronary flow, myocardial perfusion and metabolism in left bundle branch block and cardiac resynchronisation therapy. Int. J. Cardiol. 181, 65–72. doi:10.1016/j.ijcard.2014.11.194
Cleland J. G., Daubert J. C., Erdmann E., Freemantle N., Gras D., Kappenberger L., et al. (2006). Longer-term effects of cardiac resynchronization therapy on mortality in heart failure [the CArdiac REsynchronization-Heart Failure (CARE-HF) trial extension phase]. Eur. Heart J. 27, 1928–1932. doi:10.1093/eurheartj/ehl099
Cleland J. G. F., Daubert J-C., Erdmann E., Freemantle N., Gras D., Kappenberger L., et al. (2005). The effect of cardiac resynchronization on morbidity and mortality in heart failure. N. Engl. J. Med. Overseas. Ed. 352, 1539–1549. doi:10.1056/nejmoa050496
Derval N., Duchateau J., Mahida S., Eschalier R., Sacher F., Lumens J., et al. (2017). Distinctive left ventricular activations associated with ECG pattern in heart failure patients. Circ. Arrhythm. Electrophysiol. 10, e005073. doi:10.1161/CIRCEP.117.005073
Dillon J. C., Chang S., Feigenbaum H. (1974). Echocardiographic manifestations of left bundle branch block. Circulation 49, 876–880. doi:10.1161/01.cir.49.5.876
Dohi K., Suffoletto M. S., Schwartzman D., Ganz L., Pinsky M. R., Gorcsan J. (2005). Utility of echocardiographic radial strain imaging to quantify left ventricular dyssynchrony and predict acute response to cardiac resynchronization therapy. Am. J. Cardiol. 96, 112–116. doi:10.1016/j.amjcard.2005.03.032
Emerek K., Friedman D. J., Sørensen P. L., Hansen S. M., Larsen J. M., Risum N., et al. (2019). Vectorcardiographic QRS area is associated with long-term outcome after cardiac resynchronization therapy. Heart rhythm. 16, 213–219. doi:10.1016/j.hrthm.2018.08.028
Feeny A. K., Rickard J., Trulock K. M., Patel D., Toro S., Moennich L. A., et al. (2020). Machine learning of 12-lead QRS waveforms to identify cardiac resynchronization therapy patients with differential outcomes. Circ. Arrhythm. Electrophysiol. 13, e008210. doi:10.1161/CIRCEP.119.008210
Glikson M., Nielsen J. C., Kronborg M. B., Michowitz Y., Auricchio A., Barbash I. M., et al. (2021). ESC Guidelines on cardiac pacing and cardiac resynchronization therapy. Eur. Heart J. 42, 3427–3520. doi:10.1093/eurheartj/ehab364
Gold M. R., Thébault C., Linde C., Abraham W. T., Gerritse B., Ghio S., et al. (2012). Effect of QRS duration and morphology on cardiac resynchronization therapy outcomes in mild heart failure: Results from the resynchronization reverses remodeling in systolic left ventricular dysfunction (REVERSE) study. Circulation 126, 822–829. doi:10.1161/CIRCULATIONAHA.112.097709
Goldenberg I., Kutyifa V., Klein H. U., Cannom D. S., Brown M. W., Dan A., et al. (2014). Survival with cardiac-resynchronization therapy in mild heart failure. N. Engl. J. Med. 370, 1694–1701. doi:10.1056/NEJMoa1401426
Huang W., Su L., Wu S., Xu L., Xiao F., Zhou X., et al. (2017). A novel pacing strategy with low and stable output: Pacing the left bundle branch immediately beyond the conduction block. Can. J. Cardiol. 33, 1736.e1–1736. doi:10.1016/j.cjca.2017.09.013
Huang W., Wu S., Vijayaraman P., Su L., Chen X., Cai B., et al. (2020). Cardiac resynchronization therapy in patients with nonischemic cardiomyopathy using left bundle branch pacing. JACC. Clin. Electrophysiol. 6, 849–858. doi:10.1016/j.jacep.2020.04.011
Jastrzębski M., Kukla P., Kisiel R., Fijorek K., Moskal P., Czarnecka D. (2018). Comparison of four LBBB definitions for predicting mortality in patients receiving cardiac resynchronization therapy. Ann. Noninvasive Electrocardiol. 23, e12563. doi:10.1111/anec.12563
Jastrzębski M., Moskal P., Huybrechts W., Curila K., Sreekumar P., Rademakers L. M., et al. (2022). Left bundle branch–optimized cardiac resynchronization therapy (LOT-CRT): Results from an international LBBAP collaborative study group. Heart rhythm. 19, 13–21. doi:10.1016/j.hrthm.2021.07.057
Jurak P., Curila K., Leinveber P., Prinzen F. W., Viscor I., Plesinger F., et al. (2020). Novel ultra-high-frequency electrocardiogram tool for the description of the ventricular depolarization pattern before and during cardiac resynchronization. J. Cardiovasc. Electrophysiol. 31, 300–307. doi:10.1111/jce.14299
Jurak P., Halamek J., Meluzin J., Plesinger F., Postranecka T., Lipoldova J., et al. (2017). Ventricular dyssynchrony assessment using ultra-high frequency ECG technique. J. Interv. Card. Electrophysiol. 49, 245–254. doi:10.1007/s10840-017-0268-0
Khan F. Z., Virdee M. S., Palmer C. R., Pugh P. J., O'Halloran D., Elsik M., et al. (2012). Targeted left ventricular lead placement to guide cardiac resynchronization therapy: The TARGET study: A randomized, controlled trial. J. Am. Coll. Cardiol. 59, 1509–1518. doi:10.1016/j.jacc.2011.12.030
Koepfli P., Wyss C. A., Gaemperli O., Siegrist P. T., Klainguti M., Schepis T., et al. (2009). Left bundle branch block causes relative but not absolute septal underperfusion during exercise. Eur. Heart J. 30, 2993–2999. doi:10.1093/eurheartj/ehp372
Kusumoto F. M., Schoenfeld M. H., Barrett C., Edgerton J. R., Ellenbogen K. A., Gold M. R., et al. (2019). ACC/AHA/HRS guideline on the evaluation and management of patients with bradycardia and cardiac conduction delay: A report of the American College of Cardiology/American heart association task Force on clinical practice guidelines and the heart rhythm society. Circulation 140, e382–e482. doi:10.1161/CIR.0000000000000628
Linde C., Abraham W. T., Gold M. R., John Sutton M., Ghio S., Daubert C., et al. (2008). Randomized trial of cardiac resynchronization in mildly symptomatic heart failure patients and in asymptomatic patients with left ventricular dysfunction and previous heart failure symptoms. J. Am. Coll. Cardiol. 52, 1834–1843. doi:10.1016/j.jacc.2008.08.027
Linde C., Leclercq C., Rex S., Garrigue S., Lavergne T., Cazeau S., et al. (2002). Long-term benefits of biventricular pacing in congestive heart failure: Results from the MUltisite STimulation in cardiomyopathy (MUSTIC) study. J. Am. Coll. Cardiol. 40, 111–118. doi:10.1016/s0735-1097(02)01932-0
Marsan N. A., Bleeker G. B., Ypenburg C., Van Bommel R. J., Ghio S., Van de Veire N. R., et al. (2008). Real-time three-dimensional echocardiography as a novel approach to assess left ventricular and left atrium reverse remodeling and to predict response to cardiac resynchronization therapy. Heart rhythm. 5, 1257–1264. doi:10.1016/j.hrthm.2008.05.021
Master A. M., Kalter H., Dack S., Jaffe H. L. (1940). The relation between bundle branch block and cardiac enlargement. Am. Heart J. 20, 186–194. doi:10.1016/s0002-8703(40)90763-3
Moss A. J., Hall W. J., Cannom D. S., Klein H., Brown M. W., Daubert J. P., et al. (2009). Cardiac-resynchronization therapy for the prevention of heart-failure events. N. Engl. J. Med. 361, 1329–1338. doi:10.1056/NEJMoa0906431
Mullens W., Grimm R. A., Verga T., Dresing T., Starling R. C., Wilkoff B. L., et al. (2009). Insights from a cardiac resynchronization optimization clinic as part of a heart failure disease management program. J. Am. Coll. Cardiol. 53, 765–773. doi:10.1016/j.jacc.2008.11.024
Okafor O., Zegard A., van Dam P., Stegemann B., Qiu T., Marshall H., et al. (2019). Changes in QRS area and QRS duration after cardiac resynchronization therapy predict cardiac mortality, heart failure hospitalizations, and ventricular arrhythmias. J. Am. Heart Assoc. 8, e013539. doi:10.1161/JAHA.119.013539
Perrin M. J., Green M. S., Redpath C. J., Nery P. B., Keren A., Beanlands R. S., et al. (2012). Greater response to cardiac resynchronization therapy in patients with true complete left bundle branch block: A PREDICT substudy. Europace 14, 690–695. doi:10.1093/europace/eur381
Ploux S., Lumens J., Whinnett Z., Montaudon M., Strom M., Ramanathan C., et al. (2013). Noninvasive electrocardiographic mapping to improve patient selection for cardiac resynchronization therapy: Beyond QRS duration and left bundle branch block morphology. J. Am. Coll. Cardiol. 61, 2435–2443. doi:10.1016/j.jacc.2013.01.093
Risum N., Tayal B., Hansen T. F., Bruun N. E., Jensen M. T., Lauridsen T. K., et al. (2015). Identification of typical left bundle branch block contraction by strain echocardiography is additive to electrocardiography in prediction of long-term outcome after cardiac resynchronization therapy. J. Am. Coll. Cardiol. 66, 631–641. doi:10.1016/j.jacc.2015.06.020
Rodriguez L. M., Timmermans C., Nabar A., Beatty G., Wellens H. J. (2003). Variable patterns of septal activation in patients with left bundle branch block and heart failure. J. Cardiovasc. Electrophysiol. 14, 135–141. doi:10.1046/j.1540-8167.2003.02421.x
Ruschitzka F., Abraham W. T., Singh J. P., Bax J. J., Borer J. S., Brugada J., et al. (2013). Cardiac-resynchronization therapy in heart failure with a narrow QRS complex. N. Engl. J. Med. 369, 1395–1405. doi:10.1056/NEJMoa1306687
Saba S., Marek J., Schwartzman D., Jain S., Adelstein E., White P., et al. (2013). Echocardiography-guided left ventricular lead placement for cardiac resynchronization therapy: Results of the speckle tracking assisted resynchronization therapy for electrode region trial. Circ. Heart Fail. 6, 427–434. doi:10.1161/CIRCHEARTFAILURE.112.000078
Sohal M., Duckett S. G., Zhuang X., Shi W., Ginks M., Shetty A., et al. (2014). A prospective evaluation of cardiovascular magnetic resonance measures of dyssynchrony in the prediction of response to cardiac resynchronization therapy. J. Cardiovasc. Magn. Reson. 16, 58. doi:10.1186/s12968-014-0058-0
Stankovic I., Prinz C., Ciarka A., Daraban A. M., Kotrc M., Aarones M., et al. (2016). Relationship of visually assessed apical rocking and septal flash to response and long-term survival following cardiac resynchronization therapy (PREDICT-CRT). Eur. Heart J. Cardiovasc. Imaging 17, 262–269. doi:10.1093/ehjci/jev288
Strauss D. G., Selvester R. H., Wagner G. S. (2011). Defining left bundle branch block in the era of cardiac resynchronization therapy. Am. J. Cardiol. 107, 927–934. doi:10.1016/j.amjcard.2010.11.010
Surawicz B., Childers R., Deal B. J., Gettes L. S., Bailey J. J., Gorgels A., et al. (2009). AHA/ACCF/HRS recommendations for the standardization and interpretation of the electrocardiogram: Part III: Intraventricular conduction disturbances: A scientific statement from the American heart association electrocardiography and arrhythmias committee, council on clinical Cardiology; the American College of Cardiology foundation; and the heart rhythm society. Endorsed by the international society for computerized electrocardiology. J. Am. Coll. Cardiol. 53, 976–981. doi:10.1016/j.jacc.2008.12.013
Surkova E., Badano L. P., Bellu R., Aruta P., Sambugaro F., Romeo G., et al. (2017). Left bundle branch block: From cardiac mechanics to clinical and diagnostic challenges. Europace 19, 1251–1271. doi:10.1093/europace/eux061
Tabrizi F., Englund A., Rosenqvist M., Wallentin L., Stenestrand U. (2007). Influence of left bundle branch block on long-term mortality in a population with heart failure. Eur. Heart J. 28, 2449–2455. doi:10.1093/eurheartj/ehm262
Tang A. S. L., Wells G. A., Talajic M., Arnold M. O., Sheldon R., Connolly S., et al. (2010). Cardiac-resynchronization therapy for mild-to-moderate heart failure. N. Engl. J. Med. 363, 2385–2395. doi:10.1056/NEJMoa1009540
Tracy C. M., Epstein A. E., Darbar D., DiMarco J. P., Dunbar S. B., Estes N. A. M., et al. (2012). ACCF/AHA/HRS focused update of the 2008 guidelines for device-based therapy of cardiac rhythm abnormalities: A report of the American College of Cardiology foundation/American heart association task Force on practice guidelines and the heart rhythm society. [corrected]. Circulation 126, 1784–1800. doi:10.1161/CIR.0b013e3182618569
Tung R., Upadhyay G. A. (2020). Defining left bundle branch block patterns in cardiac resynchronisation therapy: A return to His bundle recordings. Arrhythm. Electrophysiol. Rev. 9, 28–33. doi:10.15420/aer.2019.12
Upadhyay G. A., Cherian T., Shatz D. Y., Beaser A. D., Aziz Z., Ozcan C., et al. (2019). Intracardiac delineation of septal conduction in left bundle-branch block patterns. Circulation 139, 1876–1888. doi:10.1161/CIRCULATIONAHA.118.038648
Upadhyay G. A., Vijayaraman P., Nayak H. M., Verma N., Dandamudi G., Sharma P. S., et al. (2019). His corrective pacing or biventricular pacing for cardiac resynchronization in heart failure. J. Am. Coll. Cardiol. 74, 157–159. doi:10.1016/j.jacc.2019.04.026
Upadhyay G. A., Vijayaraman P., Nayak H. M., Verma N., Dandamudi G., Sharma P. S., et al. (2019). On-treatment comparison between corrective His bundle pacing and biventricular pacing for cardiac resynchronization: A secondary analysis of the his-SYNC pilot trial. Heart rhythm. 16, 1797–1807. doi:10.1016/j.hrthm.2019.05.009
Van de Veire N. R., Yu C. M., Ajmone-Marsan N., Bleeker G. B., Ypenburg C., De Sutter J., et al. (2008). Triplane tissue Doppler imaging: A novel three-dimensional imaging modality that predicts reverse left ventricular remodelling after cardiac resynchronisation therapy. Heart 94, e9. doi:10.1136/hrt.2007.122564
van Stipdonk A. M. W., Hoogland R., Ter Horst I., Kloosterman M., Vanbelle S., Crijns H. J. G. M., et al. (2020). Evaluating electrocardiography-based identification of cardiac resynchronization therapy responders beyond current left bundle branch block definitions. JACC. Clin. Electrophysiol. 6, 193–203. doi:10.1016/j.jacep.2019.10.009
van Stipdonk A. M. W., Ter Horst I., Kloosterman M., Engels E. B., Rienstra M., Crijns H. J. G. M., et al. (2018). QRS area is a strong determinant of outcome in cardiac resynchronization therapy. Circ. Arrhythm. Electrophysiol. 11, e006497. doi:10.1161/CIRCEP.118.006497
Vassallo J. A., Cassidy D. M., Marchlinski F. E., Buxton A. E., Waxman H. L., Doherty J. U., et al. (1984). Endocardial activation of left bundle branch block. Circulation 69, 914–923. doi:10.1161/01.cir.69.5.914
Vijayaraman P., Herweg B., Verma A., Sharma P. S., Atiqa Batul S., Sundaram Ponnusamy S., et al. (2022). Rescue left bundle branch area pacing in coronary venous lead failure or nonresponse to biventricular pacing: Results from International LBBAP Collaborative Study Group. Amsterdam, Netherlands: Heart Rhythm.
Vijayaraman P., Ponnusamy S., Cano Ó., Sharma P. S., Naperkowski A., Subsposh F. A., et al. (2021). Left bundle branch area pacing for cardiac resynchronization therapy: Results from the international LBBAP collaborative study group. JACC. Clin. Electrophysiol. 7, 135–147. doi:10.1016/j.jacep.2020.08.015
Vinther M., Risum N., Svendsen J. H., Møgelvang R., Philbert B. T. (2021). A randomized trial of His pacing versus biventricular pacing in symptomatic HF patients with left bundle branch block (His-Alternative). JACC. Clin. Electrophysiol. 7, 1422–1432. doi:10.1016/j.jacep.2021.04.003
Voigt J-U. (2015). Cardiac resynchronization therapy responders can be better identified by specific signatures in myocardial function. Eur. Heart J. Cardiovasc. Imaging 17, 132–133. doi:10.1093/ehjci/jev291
Walmsley J., Huntjens P. R., Prinzen F. W., Delhaas T., Lumens J. (2016). Septal flash and septal rebound stretch have different underlying mechanisms. Am. J. Physiol. Heart Circ. Physiol. 310, H394–H403. doi:10.1152/ajpheart.00639.2015
Wu S., Su L., Vijayaraman P., Zheng R., Cai M., Xu L., et al. (2021). Left bundle branch pacing for cardiac resynchronization therapy: Nonrandomized on-treatment comparison with His bundle pacing and biventricular pacing. Can. J. Cardiol. 37, 319–328. doi:10.1016/j.cjca.2020.04.037
Zareba W., Klein H., Cygankiewicz I., Hall W. J., McNitt S., Brown M., et al. (2011). Effectiveness of cardiac resynchronization therapy by QRS morphology in the multicenter automatic defibrillator implantation trial-cardiac resynchronization therapy (MADIT-CRT). Circulation 123, 1061–1072. doi:10.1161/CIRCULATIONAHA.110.960898
Keywords: left bundle branch block, cardiac resynchronization therapy, biventricular pacing, conduction system pacing, left bundle branch area pacing
Citation: Kong NW and Upadhyay GA (2022) Cardiac resynchronization considerations in left bundle branch block. Front. Physiol. 13:962042. doi: 10.3389/fphys.2022.962042
Received: 05 June 2022; Accepted: 30 August 2022;
Published: 15 September 2022.
Edited by:
Aldo Rinaldi, Wolfson Centre for Age-Related Diseases, King’s College London, United KingdomReviewed by:
Francisco Leyva, Queen Elizabeth Hospital Birmingham, United KingdomDaniel Friedman, Duke University Health System, United States
Copyright © 2022 Kong and Upadhyay. This is an open-access article distributed under the terms of the Creative Commons Attribution License (CC BY). The use, distribution or reproduction in other forums is permitted, provided the original author(s) and the copyright owner(s) are credited and that the original publication in this journal is cited, in accordance with accepted academic practice. No use, distribution or reproduction is permitted which does not comply with these terms.
*Correspondence: Gaurav A. Upadhyay, upadhyay@uchicago.edu