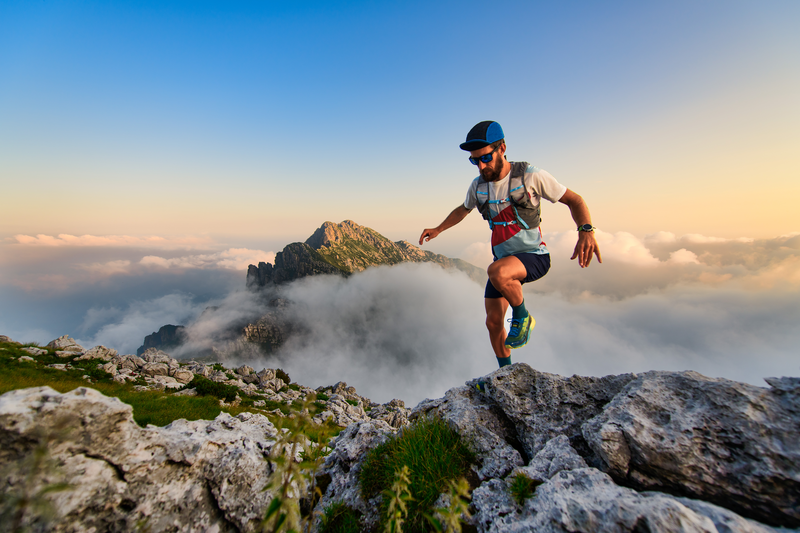
94% of researchers rate our articles as excellent or good
Learn more about the work of our research integrity team to safeguard the quality of each article we publish.
Find out more
REVIEW article
Front. Physiol. , 30 August 2022
Sec. Exercise Physiology
Volume 13 - 2022 | https://doi.org/10.3389/fphys.2022.951899
This article is part of the Research Topic Physical Activity and Exercise among Children: Health Implications View all 14 articles
Background: Neuromuscular Electrical Stimulation (NMES) is an emerging assistive technology applied through surface or implanted electrodes to augment skeletal muscle contraction. NMES has the potential to improve function while reducing the neuromuscular impairments of spastic cerebral palsy (CP). This scoping review examines the application of NMES to augment lower extremity exercises for individuals with spastic CP and reports the effects of NMES on neuromuscular impairments and function in spastic CP, to provide a foundation of knowledge to guide research and development of more effective treatment.
Methods: A literature review of Scopus, Medline, Embase, and CINAHL databases were searched from 2001 to 2 November 2021 with identified inclusion and exclusion criteria.
Results: Out of 168 publications identified, 33 articles were included. Articles on three NMES applications were identified, including NMES-assisted strengthening, NMES-assisted gait, and NMES for spasticity reduction. NMES-assisted strengthening included the use of therapeutic exercises and cycling. NMES-assisted gait included the use of NMES to improve gait patterns. NMES-spasticity reduction included the use of transcutaneous electrical stimulation or NMES to decrease tone. Thirteen studies investigated NMES-assisted strengthening, eleven investigated therapeutic exercise and demonstrated significant improvements in muscle structure, strength, gross motor skills, walking speed, and functional mobility; three studies investigated NMES-assisted cycling and demonstrated improved gross motor skills and walking distance or speed. Eleven studies investigated NMES-assisted gait and demonstrated improved muscle structure, strength, selective motor control, gross motor skills, and gait mechanics. Seven studies investigated NMES for spasticity reduction, and five of the seven studies demonstrated reduced spasticity.
Conclusion: A growing body of evidence supports the use of NMES-assisted strengthening, NMES-assisted gait, and NMES for spasticity reduction to improve functional mobility for individuals with spastic CP. Evidence for NMES to augment exercise in individuals with spastic CP remains limited. NMES protocols and parameters require further clarity to translate knowledge to clinicians. Future research should be completed to provide richer evidence to transition to more robust clinical practice.
Cerebral palsy (CP) is the most common motor disability in childhood, affecting 1.5 to 4 per 1,000 live births and presenting as spastic, dyskinetic, and ataxic types of CP, depending on the location of early brain injury (Bax et al., 2005). Spastic CP is the most common type of CP characterized by four interrelated neuromuscular impairments associated with corticospinal tract injury: muscle weakness, short muscle-tendon length relative to bone, spasticity, and impaired selective motor control (SMC) (Bax et al., 2005; Wright M. et al., 2012; Zhou et al., 2017). Dyskinetic CP is characterized by involuntary muscle contractions imposed on purposeful movement, limiting functional mobility, and is thought to be associated with basal ganglia injury (Sanger, 2015). Ataxic CP impairs balance and coordination associated with an injury in the cerebellum of the brain (Imamura et al., 1992; Rankin et al., 2010). Depending on the location of brain injury, an individual may present with symptoms of more than one type of CP (Schiariti et al., 2018). This review focuses on neuromuscular electrical stimulation (NMES) application to augment lower limb exercise for individuals with spastic CP, affecting around 80% of children with CP (Novak, 2014; CDC, 2020). Spastic CP can involve unilateral or bilateral limbs. In milder cases of CP, the lower limb is more affected distally, than proximally. Functional mobility in spastic CP is described by the Gross Motor Function Classification System (GMFCS). GMFCS levels range from I to V, with GMFCS I being mild and GMFCS V being the most severe (Palisano et al., 2007), and are reported in this review.
NMES is an emerging assistive technology applied as surface stimulation through electrodes placed over the skin or directly to the muscle via implanted electrodes to initiate or augment skeletal muscle contraction through intact peripheral nerves (Mooney and Rose, 2019; Wright et al., 2012). NMES applied through surface electrodes is the most common application as it is a non-invasive technique and generally well tolerated (Mooney and Rose, 2019). Electrodes are commonly placed over the motor point where the motor nerve innervates the muscle (Botter et al., 2011). The application of NMES to achieve functional movements is often referred to as Functional Electrical Stimulation (FES) (Masani and Popovic, 2011). The application of low-intensity electrical stimulation primarily targeting nerves, referred to as Transcutaneous Electrical Nerve Stimulation (TENS), is routinely used for pain management and has the potential to improve motor function in patients with neurodegenerative disorders (Levin and Hui-Chan, 1992; Vance et al., 2012; Kroeling et al., 2013). NMES applications include the use of NMES-assisted strengthening, NMES-assisted gait, and NMES spasticity reduction.
NMES parameters that control stimulation vary based on clinical application, targeted muscles, and individual tolerance (Maffiuletti, 2010). Parameters reported in this review include stimulation frequency, intensity, pulse width, timing (on/off ratio), and ramp. The frequency of electrical stimulation refers to the number of times a pulse of current is applied within one second, measured in Hertz (Hz). Higher frequencies generally produce more muscle activation as long as the individual pulses reach muscle fibers after their refractory period, do not result in neurotransmitter depletion, or do not block nerves otherwise (e.g., nerve blocking with monophasic high-frequency stimulation or with charge-balanced kilohertz frequency alternating current), therefore, it generates more force and can lead to increased fatigue and lower tolerance (Chaudhuri and Behan, 2004; Gorgey et al., 2009; Wegrzyk et al., 2015). Intensity or pulse amplitude refers to the amount of current delivered, or the voltage applied to the electrodes (respectively resulting in change of the current delivered) during each pulse. It is measured in milliamperes (mA) for current-controlled and Volts for voltage-controlled stimulation, where the current is proportional to the voltage. Pulse width refers to the duration between the start and end of each electrical pulse and is typically reported in microseconds (μs). Longer pulse widths are associated with increased muscle force; however, shorter pulse widths may provide patients with more comfort and increased tolerance (Mogyoros et al., 1996; Knash et al., 2003; Mang et al., 2011, 2011). Timing (on/off) refers to the duration the stimulation with a given frequency is turned on versus turned off, typically reported in seconds, whereas ramp refers to the gradual increase followed by a gradual decrease in stimulation intensity to facilitate adaptation, reduce the likelihood of discomfort, and promote smooth gradations of tetany between different muscle groups (Baker et al., 2000; Bijak et al., 2005).
A growing body of evidence supports the use of NMES in the treatment and care of individuals with CP (Mooney and Rose, 2019; Novak et al., 2020). In this review, treatments were categorized into NMES-assisted strengthening exercises (therapeutic exercise and cycling), NMES-assisted gait (overground and treadmill walking for neuroprosthetic and neurotherapeutic effects), and NMES for spasticity reduction (during strengthening exercise and gait which typically targets spastic muscles with lower frequency stimulation using TENS parameters). The ultimate goal of NMES for individuals with CP is to improve functional mobility and quality of life.
Muscle weakness is a common impairment in individuals with CP and significantly impacts their ability to function and participate in activities. Weakness is primarily caused by neurological impairment, including reduced motor-unit firing and by muscle structural changes including in the muscle fascicles such as fatty replacement, in sarcomeres, and in muscle fiber size variability (Huijing, 1998; Elder et al., 2003; Lieber et al., 2004; Foran et al., 2005; Rose and McGill, 2005; Malaiya et al., 2007; Stackhouse et al., 2007; Barber et al., 2012; Noble et al., 2014; Zhou et al., 2017). Evidence indicates that use of NMES for augmenting exercise increases microvascular perfusion in the stimulated skeletal muscle (Clemente et al., 1991; Moloney et al., 2006; Bahadori et al., 2017). This decreases the diffusion distance in the stimulated muscle tissue and enhances the exchange of nutrients and metabolites between the blood and tissue, improving physiological muscle function. Given the vital role of muscle tissue (e.g., in maintaining stable glucose metabolism), NMES might further benefit the overall quality of life in individuals across all GMFCS levels.
Accurate interpretation of research requires relevant, validated outcome measures. Therefore, this review includes studies that report outcome measures recommended as Common Data Elements (CDE) by The National Institute of Neurological Disorders and Stroke (NINDS) (Grinnon et al., 2012). The CDE database is structured by diagnosis and includes CDEs recommended for CP.
Using the NINDS CDE database, there are several ways to measure and assess changes in strength in individuals with CP (Table 1). These include both direct strength measures, such as Manual Muscle Testing and Maximum Voluntary Isometric Contraction Testing, as well as measures of functional mobility, such as temporal-spatial parameters of gait (Lee et al., 2008), 3D gait analysis of kinematics and kinetics including the Gait Deviation Index (GDI) (Schwartz and Rozumalski, 2008), 6 Minute Walk Test (6MWT) (Maher et al., 2008) which reflects gait distance, Timed Up and Go (TUG) (Kaya Kara et al., 2019), and Gross Motor Function Measure (GMFM) (Russell et al., 2000). Although not CDE outcomes, dynamometry and timed sit to stand are often used to reflect changes in muscle strength and function in individuals with CP. Changes in muscle physiology can be assessed indirectly through muscle structure using musculoskeletal ultrasound (US) and Magnetic Resonance Imaging (MRI). Our review also identified in certain studies the CDE measures of Selective Control Assessment of the Lower Extremity (SCALE) (Fowler et al., 2009) for assessment of SMC.
TABLE 1. NINDS Common Data Elements (Grinnon et al., 2012) outcome measures identified in the articles reviewed, assessing motor function, spasticity, movement, functional mobility, and Quality of Life.
This scoping review examines the application of NMES to augment lower extremity exercises for individuals with spastic CP, and reports the effects of NMES on neuromuscular impairments and function in spastic CP, to provide a foundation of knowledge that can guide research to advance the field and provide more effective treatment.
Given the extent of the literature, we determined that the most appropriate type of review for this field is a scoping review (Pollock et al., 2022). The primary goal of our review was to give a comprehensive assessment of the current use of NMES for augmenting exercise for individuals with spastic CP. We also sought to identify knowledge gaps to guide future research directions. The Preferred Reporting Items for Systematic Reviews and Meta-Analyses extension for Scoping Reviews (PRISMA-ScR) checklist was utilized to guide this review (Tricco et al., 2018).
A literature search was completed using Scopus, Medline, Embase, and CINAHL databases with additional publications referenced through the primary search. The search was completed on 2 November 2021, using the following keywords and Boolean operators: “spastic cerebral palsy” AND “neuromuscular electrical stimulation” OR “functional electrical stimulation”. The inclusion criteria for the articles were as follows: 1) the study involved individuals with CP, 2) the study reported outcome measures recommended by CDE for CP and were related to muscle strength and function, gait temporal-spatial parameters, and kinematics as identified in Table 1; 3) the study incorporated a known NMES dosage (session, duration, and frequency) with a known exercise component, such as strengthening, cycling, gait training; 4) the study was available in English; and 5) the study was published as a full-text manuscript. The exclusion criteria for the articles were as follows: 1) NMES was not a component of the study, 2) exercise was not a component of the study, 3) duration of treatment period was less than 4 weeks or not reported; 4) investigated muscles were not involving lower extremities; 5) articles were from dissertations, conference posters, or abstracts, 6) studies were published before 2001.
Using recommendations by the National Institute of Neurological Disorders and Stroke (NINDS), the authors used publications reporting at least one common data element (CDE) outcome measures specific to the diagnosis of CP. Each publication was given a level of evidence based on the Oxford Centre for Evidence-Based Medicine 2011 Level of Evidence guidelines (Howick, 2011). Data were extracted by the authors (KG, CJ, KS, BB) for each publication but unblinded to the results of other authors.
The initial 5-database search resulted in 168 publications, and an additional 41 articles were identified from references. Fifty-one articles were duplicates. The authors used titles and abstracts to screen the publications for the relevance of exercise programs involving the lower extremity. Fifty-seven articles were discarded due to diagnoses other than spastic CP or study aims outside the scope of exercise. One hundred and one articles, including seven review articles, met criteria and were fully reviewed by the authors; however, 68 were excluded upon further review for different populations (n = 5), absence of CDE for CP outcome measures (n = 6), lack of NMES intervention (n = 10), inadequate or unreported treatment duration (n = 10), lack of exercise component (n = 11), language other than English (n = 2), muscle groups other than lower extremities (n = 6), non-qualifying publication type (n = 15), and published before 2001 (n = 3). Based on these inclusion criteria, this scoping review includes a total of 33 articles, 26 intervention studies, and seven reviews. See Figure 1 for the publication search flow chart.
The literature was categorized by the application of NMES, including NMES-assisted strengthening, NMES-assisted gait, and NMES for spasticity reduction. Extracted variables included the study’s aim study design, age of participants, sample size, limb involvement (bilateral and/or unilateral), GMFCS level, device type, targeted muscle, NMES dose (number of weeks, sessions per week, and time per session), NMES parameters (frequency, intensity, pulse width, timing, ramp, waveform, and mode), and CDE outcomes recommended by NINDS, detailed in Tables 2–4.
TABLE 2. Articles reviewed reporting level of evidence, participant characteristics, NMES intervention, and outcomes measures.
A total of fourteen articles were included for NMES-assisted strengthening, as shown in Table 2. NMES-assisted strengthening interventions included NMES augmenting therapeutic exercise, pre-operative surgical preparation, post-operative recovery, and NMES-assisted cycling. Several articles overlapped in the type of intervention, such as strengthening and spasticity reduction.
Eleven studies reported NMES-assisted therapeutic exercise intervention: one case report (Daichman et al., 2003), one case series (Greve and Colvin, 2021), one pilot study (Stackhouse et al., 2007), two prospective trials (Nunes et al., 2008; Rajalaxmi et al., 2017), two prospective controlled studies (Karabay et al., 2015; Mukhopadhyay et al., 2017), and four randomized controlled trials (RCT) (Kerr et al., 2006; Khalili and Hajihassanie, 2008; Arya et al., 2012; Qi et al., 2018). Strengthening involved both home and clinic interventions using portable NMES devices with surface or implanted electrodes focused on the quadriceps, gastrocnemius, and tibialis anterior muscles. NMES was applied during positioning, stretching, facilitated exercises, strengthening, activities of daily living, balance, posture, and gait exercises (Daichman et al., 2003; Kerr et al., 2006; Stackhouse et al., 2007; Khalili and Hajihassanie, 2008; Nunes et al., 2008; Arya et al., 2012; Karabay et al., 2015; Mukhopadhyay et al., 2017; Rajalaxmi et al., 2017; Qi et al., 2018; Greve and Colvin, 2021). Dosage consisted of 15–60 min, one to seven times per week for 4–16 weeks. See Table 3 for specific NMES parameters and dosage for each study.
Ten studies using NMES-assisted therapeutic exercise reported improvements in muscle structure, strength, gross motor skills, WS, and functional mobility (Daichman et al., 2003; Stackhouse et al., 2007; Khalili and Hajihassanie, 2008; Nunes et al., 2008; Arya et al., 2012; Karabay et al., 2015; Mukhopadhyay et al., 2017; Rajalaxmi et al., 2017; Qi et al., 2018; Greve and Colvin, 2021). Two studies examined muscle cross-sectional area (CSA) using ultrasound or MRI and found an increase in CSA values of the quadriceps (Stackhouse et al., 2007), tibialis anterior (Karabay et al., 2015), and gastrocnemius (Karabay et al., 2015). Two studies reported an increase in quadriceps strength assessed with dynamometry (Daichman et al., 2003; Stackhouse et al., 2007). Six studies conducted the GMFM (Kerr et al., 2006; Nunes et al., 2008; Arya et al., 2012; Mukhopadhyay et al., 2017; Qi et al., 2018; Greve and Colvin, 2021), and four of the six studies reported positive changes in gross motor skills (Nunes et al., 2008; Mukhopadhyay et al., 2017; Qi et al., 2018; Greve and Colvin, 2021). Two studies reported improvement in functional mobility using the PEDI (Daichman et al., 2003) and FMS (Greve and Colvin, 2021). Studies also reported improvement in gait (Daichman et al., 2003; Arya et al., 2012; Mukhopadhyay et al., 2017; Rajalaxmi et al., 2017), WS (Stackhouse et al., 2007; Arya et al., 2012; Mukhopadhyay et al., 2017; Qi et al., 2018), and endurance (Greve and Colvin, 2021) following NMES. Five studies (Daichman et al., 2003; Kerr et al., 2006; Stackhouse et al., 2007; Khalili and Hajihassanie, 2008; Greve and Colvin, 2021) commented on adherence with 90–100% tolerance for using NMES by individuals participating in these studies. See Table 4 for CDE outcomes and results of each study.
Three studies reported NMES-assisted cycling for exercise, where multichannel NMES was applied using surface electrodes while the participant rode an indoor tricycle or stationary bicycle. One case report (Johnston and Wainwright, 2011) and two RCTs (Armstrong et al., 2020; Özen et al., 2021) reported on multichannel NMES used to target multiple muscles during cycling, including the gluteals, quadriceps, hamstrings, gastrocnemius, and/or anterior tibialis. NMES was applied during cycling alone or in addition to interventions, such as ROM, strengthening, and balance. NMES intervention dosage ranged from 30 min, 3–5 times per week for 4–12 weeks.
Three studies using NMES-assisted cycling reported boosting gross motor skills, walking distance, and speed (Johnston and Wainwright, 2011; Armstrong et al., 2020; Özen et al., 2021). Two studies (Armstrong et al., 2020; Özen et al., 2021) reported improvement in gross motor skills assessed with the GMFM. Studies also reported an increase in walking distance assessed with 6MWT (Johnston and Wainwright, 2011; Özen et al., 2021) and speed assessed using the TUG (Johnston and Wainwright, 2011). NMES was well-tolerated in one study (Özen et al., 2021) and variable in two studies (Johnston and Wainwright, 2011; Armstrong et al., 2020). See Tables 2, 3, 4 for details of each study’s NMES application and CDE outcomes.
Table 2 reports the results of NMES-assisted gait, which includes interventions using NMES during gait for treadmill or overground walking with a known therapeutic dosage.
Eleven studies reported NMES-assisted gait for strengthening and improving gait pattern, including one case report (Robinson et al., 2015), three single-subject research design studies (SSRD) (Chan et al., 2004; Pool et al., 2014; Gonçalves et al., 2019), three prospective trials (Johnston et al., 2004; Prosser et al., 2012; Damiano et al., 2013), and four RCTs (Pool et al., 2016; 2015, van der Linden et al., 2003, 2008). Various NMES devices were used, including surface electrodes for non-wearable units targeting the gluteals, quadriceps, gastrocnemius, and tibialis anterior. Wearable units targeted hip adductors, gluteus maximus and medius, quadriceps, tibialis anterior, and gastrocnemius. NMES was applied during walking overground or performing functional task training. Only one study applied NMES while on a treadmill (Chan et al., 2004). NMES dosage ranged from 15 min to 8 h per day, 3–7 days per week for 4–40 weeks. See Table 3 for details of each study’s NMES application and parameters.
The eleven studies that investigated NMES-assisted gait found improved muscle structure, strength, SMC, gross motor skills, and gait (van der Linden et al., 2003, 2008; Chan et al., 2004; Johnston et al., 2004; Prosser et al., 2012; Damiano et al., 2013; Pool et al., 2016, 2015, 2014; Robinson et al., 2015; Gonçalves et al., 2019). NMES-assisted gait resulted in increased muscle volume of tibialis anterior as assessed on MRIs (Pool et al., 2016), increased tibialis anterior CSA as assessed on ultrasound (Damiano et al., 2013), increased strength as assessed by dynamometers (Pool et al., 2016, 2014), improved SMC as assessed by SCALE (Pool et al., 2016), improved gross motor skills as assessed by GMFM (van der Linden et al., 2003; Chan et al., 2004; Johnston et al., 2004; Gonçalves et al., 2019), and improved gait as assessed by kinematics, kinetics, and temporal-spatial parameters (Chan et al., 2004; Johnston et al., 2004; van der Linden et al., 2008; Prosser et al., 2012; Pool et al., 2015; Robinson et al., 2015). Compliance was reported to be high for NMES intervention (Chan et al., 2004; Prosser et al., 2012; Pool et al., 2016, 2015). Tolerance was reported as ranging from good (Damiano et al., 2013; Pool et al., 2014) to variable (van der Linden et al., 2003, 2008). See Table 4 for CDE outcome measures and results for each NMES-assisted gait study.
Seven studies reported on the effects of NMES on spasticity. One case report (Daichman et al., 2003), one prospective controlled study (Karabay et al., 2015), one prospective trial (Rajalaxmi et al., 2017), and four RCTs (Khalili and Hajihassanie, 2008; AlAbdulwahab and Al-Gabbani, 2010; Pool et al., 2015; Özen et al., 2021). The targeted muscles for NMES included hip adductors, quadriceps, hamstrings, gastrocnemius, and tibialis anterior. NMES was applied to the antagonist muscle during exercises, including ROM, balance, strengthening, and gait training (Daichman et al., 2003; Khalili and Hajihassanie, 2008; Pool et al., 2015; Rajalaxmi et al., 2017; Özen et al., 2021). TENS was applied to the antagonist muscle during ROM and gait training exercises (AlAbdulwahab and Al-Gabbani, 2010). In addition, NMES-assisted strengthening and NMES-assisted gait were investigated (Pool et al., 2015; Özen et al., 2021). The dosage varied between 5 and 240 min per session, 3–7 days per week for 1–8 weeks.
Among the seven studies of NMES for spasticity reduction, five studies reported reduced spasticity in the antagonistic muscle when using electrical stimulation (Khalili and Hajihassanie, 2008; AlAbdulwahab and Al-Gabbani, 2010; Pool et al., 2015; Rajalaxmi et al., 2017; Özen et al., 2021). Study results included decreased resistance of the hip adductors (AlAbdulwahab and Al-Gabbani, 2010), hamstrings (Khalili and Hajihassanie, 2008; Özen et al., 2021), and gastrocnemius muscles (Pool et al., 2015; Rajalaxmi et al., 2017; Özen et al., 2021) assessed by the Modified Ashworth Scale (MAS) or Tardieu Scale; while two studies found no change in spasticity (Daichman et al., 2003; Karabay et al., 2015). Application and results of NMES-assisted spasticity reduction can be found in Tables 2, 3, 4.
Our search identified seven studies reviewing NMES as an intervention for individuals with CP, including reviews (Khamis et al., 2018; Wright M. et al., 2012), scoping reviews (Mooney and Rose, 2019; Walhain et al., 2021), and systematic reviews with meta-analysis (Salazar et al., 2019) and without meta-analysis (Chiu and Ada, 2014; Moll et al., 2017). These reviews explicitly focused on the effects of NMES on muscle morphology (Walhain et al., 2021), gait (Mooney and Rose, 2019, p. 2; Wright P. A. et al., 2012), gross motor function (Salazar et al., 2019), ankle dorsiflexion (Moll et al., 2017), activities (Chiu and Ada, 2014), and improvement in gait deviations when using FES (Khamis et al., 2018). None of the listed reviews were specific to our scoping review looking at the NMES application as a lower extremity exercise for individuals with spastic CP.
The findings of this scoping review indicate that NMES applied to strengthening exercise, gait, and spasticity reduction demonstrate potential benefits for improving muscle physiology, neuromuscular impairments, gait patterns, and functional mobility in individuals with spastic CP. The twenty-six intervention publications, dating from 2003 to 2021, included a total of 558 individuals aged 3–57 years with CP, GMFCS levels I-IV with unilateral or bilateral involvement. The dosage of NMES intervention varied by study, as noted in Table 2. In addition, while using NMES, the exercise activities varied and included ROM, strengthening (i.e., isometric contractions, progressive resistance exercises, cycling), positioning, functional tasks, and gait. NMES included both wearable and non-wearable devices with surface electrodes, with the exception of two studies that utilized implanted electrodes (Johnston et al., 2004; Stackhouse et al., 2007).
The NMES parameters utilized in these studies included frequencies between 10 and 50 Hz, stimulation intensities between 4 and 100 mA, with typical values below 40 mA, and pulse width between 3 and 350 μs, as shown in Table 3. The most substantial variation was in pulse width, which could be attributed to individual preferences and tolerances and to the sequence of adjusting NMES parameters during treatment. Although pulse width affects muscle force production, currently, there is no evidence suggesting the range of optimal pulse width, therefore, more studies are needed. Clinical experience suggests that electrode size and adherence to the skin and pulse width contribute most to NMES comfort level.
NMES-assisted strengthening was found to increase strength, WS, walking distance, gross motor skills, and functional mobility. Three studies reported that NMES applied during exercise provided better outcomes than exercise alone (Khalili and Hajihassanie, 2008; Arya et al., 2012; Qi et al., 2018). This may be attributed to increased sensory attention to task and motor learning. Weaker muscles are likely to gain more from NMES strengthening than stronger muscles. Physical therapy, as well as surgical preparation and recovery, provide opportunities to initiate NMES strengthening of weakened muscles. Clinical expertise suggests that voluntary contraction is an important element of strengthening and motor control versus NMES stimulation alone. The results of this scoping review found further evidence that supports the use of NMES-assisted strengthening as a clinical treatment for individuals with spastic CP. Future studies need to study the impact of NMES-assisted strengthening on biological aspects of muscle physiology and chronic health conditions in individuals with spastic CP.
Another benefit to muscle strengthening is increasing overall muscle-tendon length across the joint, which may improve ROM (Zhou et al., 2017). Increasing muscle fiber diameter through strengthening theoretically increases overall muscle-tendon length due to the diagonal muscle fiber pennation angle relative to the axis of the bone (Zhou et al., 2017). Several studies identified that muscle CSA was increased with NMES-assisted strengthening, which likely would translate to increased overall muscle-tendon length and improved ROM (Stackhouse et al., 2007; Karabay et al., 2015). Future studies need to examine the impact of NMES-assisted strengthening on overall muscle-tendon length and joint ROM.
NMES-assisted gait was found to improve strength, motor control, gait pattern, and temporal-spatial parameters. Similar to NMES-assisted strengthening, the repetitive movement of walking on a treadmill combined with NMES was found to have an advantage over treadmill gait or NMES alone for improving ankle power and gross motor skills of standing and walking (Chan et al., 2004). Furthermore, another study suggested that intensive use of NMES-assisted gait in home and community settings may facilitate motor learning (Pool et al., 2014). The results of this scoping review further strengthens the evidence to support NMES-assisted gait as a clinical treatment for individuals with spastic CP. Wearable single-channel NMES units are widely available and allow for home and community use to improve foot clearance in swing; however wearable multi-channel units are not widely available. Wearable multi-channel units are needed to treat gait abnormalities other than limited foot clearance in swing. Further research and development are needed in this area.
NMES was also found to reduce spasticity, as assessed by Tardieu or MAS in five of seven studies reviewed; one study used TENS (AlAbdulwahab and Al-Gabbani, 2010), and four studies used NMES (Khalili and Hajihassanie, 2008; Pool et al., 2015; Rajalaxmi et al., 2017; Özen et al., 2021). Corticospinal tract injury results in a loss of descending neural signal activation and inhibition. Muscle spasticity is a neuromuscular impairment that results from loss of inhibition. Further research needs to investigate the potential inhibitory effects of NMES and how to optimize spasticity reduction and duration of treatment effects. The location of ideal electrode placement along the lumbar spine, over relevant dermatomes, directly over spastic muscle, or to elicit antagonist inhibition requires further research.
Limitations of this scoping review include the exclusion of some NMES-related studies that did not meet inclusion criteria due to NMES treatment duration of fewer than 4 weeks, the absence of an exercise component, technology development trials for NMES-assisted gait on a treadmill (Zahradka et al., 2021) or robotics (Shideler et al., 2020). These limitations may have eliminated some evidence in the field. However, with respect to treatment duration, a recent publication recommended at least 8–20 weeks of exercise training to facilitate meaningful changes in muscle structure and improve function in individuals with CP (Moreau and Lieber, 2022). This suggests that 8–20 weeks of exercise duration may be required, and therefore, it is possible that some of the studies in our review lacked the proper dosage to produce a meaningful change. While 11 out of 26 studies in this scoping review were RCTs, further studies with larger sample sizes and more consistent protocols using CDE outcome measures are needed to move the field forward.
This scoping review indicates that further research is needed to determine optimal NMES protocols and dosage using sensitive CDE outcome measures. Furthermore, device development of wearable NMES units that can be easily applied for NMES-assisted strengthening, gait, and spasticity reduction is needed for individuals with spastic CP. Understanding the relationship between NMES strength training and functional results, as well as the optimal NMES protocol and dosage, requires research with a larger sample size and longer treatment duration (i.e., 8–20 weeks). Identifying changes in neuromuscular impairments of weakness, short-muscle tendon unit, spasticity, and impaired SMC as well as motor learning, and utilizing CDEs with careful attention to minimal clinically important differences will allow us to better comprehend the therapeutic effects of NMES. Finally, advancing new NMES technology, such as wireless multichannel NMES devices and hybrid robotic and exoskeleton NMES systems, will provide evidence-based, clinically feasible interventions for individuals with CP to improve functional mobility.
Findings from this scoping review provide evidence that supports the use of NMES-assisted strengthening with therapeutic exercise and cycling, NMES-assisted gait, and NMES for spasticity reduction to improve mobility in individuals with spastic CP, based on validated CDE outcome measures. Wearable and non-wearable units were utilized with surface or implanted electrodes targeting the gluteals, hip adductors, hamstrings, quadriceps, gastrocnemius, and tibialis anterior to augment exercise and mobility. NMES was found to improve muscle structure, strength, gross motor skills, gait kinematics, WS, and walking distance and reduce spasticity. Clinicians can consider NMES to be an effective treatment for individuals with spastic CP. Additional research is needed to further investigate optimal parameters, dosage, and impact of NMES on neuromuscular impairments and functional mobility in individuals with spastic CP.
All authors listed have made a substantial, direct, and intellectual contribution to the work and approved it for publication.
The authors of this study did not receive any funding for their research.
We would like to thank Dr. James McCarthy for sharing his expertise.
The authors declare that the research was conducted in the absence of any commercial or financial relationships that could be construed as a potential conflict of interest.
All claims expressed in this article are solely those of the authors and do not necessarily represent those of their affiliated organizations, or those of the publisher, the editors and the reviewers. Any product that may be evaluated in this article, or claim that may be made by its manufacturer, is not guaranteed or endorsed by the publisher.
AlAbdulwahab S. S., Al-Gabbani M. (2010). Transcutaneous electrical nerve stimulation of hip adductors improves gait parameters of children with spastic diplegic cerebral palsy. NeuroRehabilitation 26, 115–122. doi:10.3233/NRE-2010-0542
Armstrong E. L., Boyd R. N., Horan S. A., Kentish M. J., Ware R. S., Carty C. P. (2020). Functional electrical stimulation cycling, goal‐directed training, and adapted cycling for children with cerebral palsy: A randomized controlled trial. Dev. Med. Child. Neurol. 62, 1406–1413. doi:10.1111/dmcn.14648
Arya B. K., Mohapatra J., Subramanya K., Prasad H., Kumar R., Mahadevappa M. (2012). “Surface EMG analysis and changes in gait following electrical stimulation of quadriceps femoris and tibialis anterior in children with spastic cerebral palsy,” in Annual International Conference of the IEEE Engineering in Medicine and Biology Society, San Diego, CA, 28 August 2012 - 01 September 2012 (IEEE), 5726–5729. doi:10.1109/EMBC.2012.6347295
Bahadori S., Immins T., Wainwright T. W. (2017). The effect of calf neuromuscular electrical stimulation and intermittent pneumatic compression on thigh microcirculation. Microvasc. Res. 111, 37–41. doi:10.1016/j.mvr.2017.01.001
Baker L. L., Wederich C., McNeal D. R., Newsam C. J., Waters R. L. (2000). Neuro muscular electrical stimulation: A practical guide. Los Amigos Res. Educ. Inst.
Barber L., Barrett R., Lichtwark G. (2012). Medial gastrocnemius muscle fascicle active torque-length and Achilles tendon properties in young adults with spastic cerebral palsy. J. Biomech. 45, 2526–2530. doi:10.1016/j.jbiomech.2012.07.018
Bax M., Goldstein M., Rosenbaum P., Leviton A., Paneth N., Dan B., et al. (2005). Proposed definition and classification of cerebral palsy, April 2005. Dev. Med. Child. Neurol. 47, 571–576. doi:10.1017/S001216220500112X
Bijak M., Rakos M., Hofer C., Mayr W., Strohhofer M., Raschka D., et al. (2005). Stimulation parameter optimization for FES supported standing up and walking in SCI patients. Artif. Organs 29, 220–223. doi:10.1111/j.1525-1594.2005.29039.x
Botter A., Oprandi G., Lanfranco F., Allasia S., Maffiuletti N. A., Minetto M. A. (2011). Atlas of the muscle motor points for the lower limb: Implications for electrical stimulation procedures and electrode positioning. Eur. J. Appl. Physiol. 111, 2461–2471. doi:10.1007/s00421-011-2093-y
Boyd R. N., Graham H. K. (1999). Objective measurement of clinical findings in the use of botulinum toxin type A for the management of children with cerebral palsy. Eur. J. Neurol 6, s23–s35. doi:10.1111/j.1468-1331.1999.tb00031.x
CDC, 2020. Data and statistics for cerebral palsy CDC. Centers for disease control and prevention. Available at: https://www.cdc.gov/ncbddd/cp/data.html. (Accessed 5, 6, 22)
Chan N. N. C., Smith A. W., Lo S. K. (2004). Efficacy of neuromuscular electrical stimulation in improving ankle kinetics during walking in children with cerebral palsy. Hong Kong Physiother. J. 22, 50–56. doi:10.1016/S1013-7025(09)70050-8
Chaudhuri A., Behan P. O. (2004). Fatigue in neurological disorders. Lancet 363, 978–988. doi:10.1016/S0140-6736(04)15794-2
Chiu H.-C., Ada L. (2014). Effect of functional electrical stimulation on activity in children with cerebral palsy: A systematic review. Pediatr. Phys. Ther. 26, 283–288. doi:10.1097/PEP.0000000000000045
Clemente F. R., Matulionis D. H., Barron K. W., Currier D. P. (1991). Effect of motor neuromuscular electrical stimulation on microvascular perfusion of stimulated rat skeletal muscle. Phys. Ther. 71, 397–404. doi:10.1093/ptj/71.5.397
Coster W., Law M., Bedell G., Khetani M., Cousins M., Teplicky R. (2010). Participation and environment measure for children and youth (PEM-CY). Boston, MA: Boston University.
Daichman J., Johnston T. E., Evans K., Tecklin J. S. (2003). The effects of a neuromuscular electrical stimulation home program on impairments and functional skills of a child with spastic diplegic cerebral palsy: A case report. Pediatr. Phys. Ther. 15, 153–158. doi:10.1097/01.PEP.0000083121.26982.1D
Damiano D. L., Prosser L. A., Curatalo L. A., Alter K. E. (2013). Muscle plasticity and ankle control after repetitive use of a functional electrical stimulation device for foot drop in cerebral palsy. Neurorehabil. Neural Repair 27, 200–207. doi:10.1177/1545968312461716
Elder G. C., Kirk J., Stewart G., Cook K., Weir D., Marshall A., et al. (2003). Contributing factors to muscle weakness in children with cerebral palsy. Dev. Med. Child. Neurol. 45, 542–550. doi:10.1017/S0012162203000999
Foran J. R., Steinman S., Barash I., Chambers H. G., Lieber R. L. (2005). Structural and mechanical alterations in spastic skeletal muscle. Dev. Med. Child. Neurol. 47, 713–717. doi:10.1017/S0012162205001465
Fowler E. G., Staudt L. A., Greenberg M. B., Oppenheim W. L. (2009). Selective control assessment of the lower extremity (SCALE): Development, validation, and interrater reliability of a clinical tool for patients with cerebral palsy. Dev. Med. Child. Neurol. 51, 607–614. doi:10.1111/j.1469-8749.2008.03186.x
Gonçalves R. V., Fonseca S. T., de Araújo P. A., Souza T. R., Resende R. A., Mancini M. C. (2019). Functional task training combined with electrical stimulation improves motor capacity in children with unilateral cerebral palsy: A single-subject design. Pediatr. Phys. Ther. 31, 208–215. doi:10.1097/PEP.0000000000000588
Gorgey A. S., Black C. D., Elder C. P., Dudley G. A. (2009). Effects of electrical stimulation parameters on fatigue in skeletal muscle. J. Orthop. Sports Phys. Ther. 39, 684–692. doi:10.2519/jospt.2009.3045
Gracies J.-M., Burke K., Clegg N. J., Browne R., Rushing C., Fehlings D., et al. (2010). Reliability of the Tardieu scale for assessing spasticity in children with cerebral palsy. Arch. Phys. Med. Rehabil. 91, 421–428. doi:10.1016/j.apmr.2009.11.017
Greve K., Colvin C. (2021). Neuromuscular electrical stimulation to immobilized lower extremities directly following orthopaedic surgery in three children with cerebral palsy: A case series. Sensors 21, 7661. doi:10.3390/s21227661
Grinnon S. T., Miller K., Marler J. R., Lu Y., Stout A., Odenkirchen J., et al. (2012). National Institute of neurological disorders and Stroke common data element project - approach and methods. Clin. Trials 9, 322–329. doi:10.1177/1740774512438980
Haley S. M. (1992). Pediatric evaluation of disability inventory (PEDI). Dev. Stand. Adm. Man. Ther. Ski. Build.
Howick J. (2011). The philosophy of evidence-based medicine. Chichester, West Sussex, UK: Wiley-Blackwell, BMJ Books.
Huijing P. A. (1998). Muscle, the motor of movement: Properties in function, experiment and modelling. J. Electromyogr. Kinesiol. 8, 61–77. doi:10.1016/S1050-6411(97)00023-0
Imamura S., Tachi N., Tsuzuki A., Sasaki K., Hirano S., Tanabe C., et al. (1992). Ataxic cerebral palsy and brain imaging. No Hattatsu 24. 441–448.
Johnston T. E., Wainwright S. F. (2011). Cycling with functional electrical stimulation in an adult with spastic diplegic cerebral palsy. Phys. Ther. 91, 970–982. doi:10.2522/ptj.20100286
Johnston T. E., Finson R. L., McCarthy J. J., Smith B. T., Betz R. R., Mulcahey M. J. (2004). Use of functional electrical stimulation to augment traditional orthopaedic surgery in children with cerebral palsy. J. Pediatr. Orthop. 24, 283–291. doi:10.1097/00004694-200405000-00009
Karabay İ., Öztürk G. T., Malas F. Ü., Kara M., Tiftik T., Ersöz M., et al. (2015). Short-term effects of neuromuscular electrical stimulation on muscle architecture of the tibialis anterior and gastrocnemius in children with cerebral palsy: Preliminary results of a prospective controlled study. Am. J. Phys. Med. Rehabil. 94, 728–733. doi:10.1097/PHM.0000000000000238
Kaya Kara O., Livanelioglu A., Yardımcı B. N., Soylu A. R. (2019). The effects of functional progressive strength and power training in children with unilateral cerebral palsy. Pediatr. Phys. Ther. 31, 286–295. doi:10.1097/PEP.0000000000000628
Kerr C., McDowell B., Cosgrove A., Walsh D., Bradbury I., McDonough S. (2006). Electrical stimulation in cerebral palsy: A randomized controlled trial. Dev. Med. Child. Neurol. 48, 870–876. doi:10.1017/S0012162206001915
Khalili M. A., Hajihassanie A. (2008). Electrical simulation in addition to passive stretch has a small effect on spasticity and contracture in children with cerebral palsy: A randomised within-participant controlled trial. Aust. J. Physiother. 54, 185–189. doi:10.1016/S0004-9514(08)70025-4
Khamis S., Herman T., Krimus S., Danino B. (2018). Is functional electrical stimulation an alternative for orthotics in patients with cerebral palsy? A literature review. Eur. J. Paediatr. Neurol. 22, 7–16. doi:10.1016/j.ejpn.2017.10.004
Knash M. E., Kido A., Gorassini M., Chan K. M., Stein R. B. (2003). Electrical stimulation of the human common peroneal nerve elicits lasting facilitation of cortical motor-evoked potentials. Exp. Brain Res. 153, 366–377. doi:10.1007/s00221-003-1628-9
Kroeling P., Gross A., Graham N., Burnie S. J., Szeto G., Goldsmith C. H., et al. (2013). Electrotherapy for neck pain. Cochrane Database Syst. Rev. 1 (8), CD004251. doi:10.1002/14651858.CD004251.pub5
Law M., Baptiste S., McColl M., Opzoomer A., Polatajko H., Pollock N. (1990). The Canadian occupational performance measure: An outcome measure for occupational therapy. Can. J. Occup. Ther. 57, 82–87. doi:10.1177/000841749005700207
Lee J. H., Sung I. Y., Yoo J. Y. (2008). Therapeutic effects of strengthening exercise on gait function of cerebral palsy. Disabil. Rehabil. 30, 1439–1444. doi:10.1080/09638280701618943
Levin M. F., Hui-Chan C. W. Y. (1992). Relief of hemiparetic spasticity by TENS is associated with improvement in reflex and voluntary motor functions. Electroencephalogr. Clin. Neurophysiol. 85, 131–142. doi:10.1016/0168-5597(92)90079-Q
Lieber R. L., Steinman S., Barash I. A., Chambers H. (2004). Structural and functional changes in spastic skeletal muscle. Muscle Nerve 29, 615–627. doi:10.1002/mus.20059
Mackey A. H., Lobb G. L., Walt S. E., Stott N. S. (2003). Reliability and validity of the Observational Gait Scale in children with spastic diplegia. Dev. Med. Child. Neurol. 45, 4–11. doi:10.1017/S0012162203000021
Mackie P. C., Jessen E. C., Jarvis S. N. (1998). The lifestyle assessment questionnaire: An instrument to measure the impact of disability on the lives of children with cerebral palsy and their families. Child. Care Health Dev. 24, 473–486. doi:10.1046/j.1365-2214.1998.00083.x
Maffiuletti N. A. (2010). Physiological and methodological considerations for the use of neuromuscular electrical stimulation. Eur. J. Appl. Physiol. 110, 223–234. doi:10.1007/s00421-010-1502-y
Maher C. A., Williams M. T., Olds T. S. (2008). The six-minute walk test for children with cerebral palsy. Int. J. Rehabil. Res. 31, 185–188. doi:10.1097/MRR.0b013e32830150f9
Malaiya R., McNee A. E., Fry N. R., Eve L. C., Gough M., Shortland A. P. (2007). The morphology of the medial gastrocnemius in typically developing children and children with spastic hemiplegic cerebral palsy. J. Electromyogr. Kinesiol. 17, 657–663. doi:10.1016/j.jelekin.2007.02.009
Mang C. S., Clair J. M., Collins D. F. (2011). Neuromuscular electrical stimulation has a global effect on corticospinal excitability for leg muscles and a focused effect for hand muscles. Exp. Brain Res. 209, 355–363. doi:10.1007/s00221-011-2556-8
Masani K., Popovic M. R. (2011). “Functional electrical stimulation in rehabilitation and neurorehabilitation,” in Springer handbook of medical technology. Editors R. Kramme, K.-P. Hoffmann, and R. S. Pozos (Berlin, Heidelberg: Springer Handbooks. Springer), 877–896. doi:10.1007/978-3-540-74658-4_44
Mogyoros I., Kiernan M. C., Burke D. (1996). Strength-duration properties of human peripheral nerve. Brain 119, 439–447. doi:10.1093/brain/119.2.439
Moll I., Vles J. S. H., Soudant D. L. H. M., Witlox A. M. A., Staal H. M., Speth L. A. W. M., et al. (2017). Functional electrical stimulation of the ankle dorsiflexors during walking in spastic cerebral palsy: A systematic review. Dev. Med. Child. Neurol. 59, 1230–1236. doi:10.1111/dmcn.13501
Moloney M. C., Lyons G. M., Breen P., Burke P. E., Grace P. A. (2006). Haemodynamic study examining the response of venous blood flow to electrical stimulation of the gastrocnemius muscle in patients with chronic venous disease. Eur. J. Vasc. Endovasc. Surg. 31, 300–305. doi:10.1016/j.ejvs.2005.08.003
Mooney J. A., Rose J. (2019). A scoping review of neuromuscular electrical stimulation to improve gait in cerebral palsy: The arc of progress and future strategies. Front. Neurol. 10, 887. doi:10.3389/fneur.2019.00887
Moreau N. G., Lieber R. L. (2022). Effects of voluntary exercise on muscle structure and function in cerebral palsy. Dev. Med. Child. Neurol. 64, 700–708. doi:10.1111/dmcn.15173
Mukhopadhyay R., Lenka P. K., Biswas A., Mahadevappa M. (2017). Evaluation of functional mobility outcomes following electrical stimulation in children with spastic cerebral palsy. J. Child. Neurol. 32, 650–656. doi:10.1177/0883073817700604
Mutlu A., Livanelioglu A., Gunel M. K. (2008). Reliability of Ashworth and modified Ashworth scales in children with spastic cerebral palsy. BMC Musculoskelet. Disord. 9, 44. doi:10.1186/1471-2474-9-44
Noble J. J., Fry N. R., Lewis A. P., Keevil S. F., Gough M., Shortland A. P. (2014). Lower limb muscle volumes in bilateral spastic cerebral palsy. Brain Dev. 36, 294–300. doi:10.1016/j.braindev.2013.05.008
Novak S., Guerron G., Zou Z., Cheung G., Berteau J.-P. (2020). New guidelines for electrical stimulation parameters in adult patients with knee osteoarthritis based on a systematic review of the current literature. Am. J. Phys. Med. Rehabil. 99, 682–688. doi:10.1097/PHM.0000000000001409
Novak I. (2014). Evidence-based diagnosis, health care, and rehabilitation for children with cerebral palsy. J. Child. Neurol. 29, 1141–1156. doi:10.1177/0883073814535503
Nunes L., Quevedo A. a. F., Magdalon E. C. (2008). Effects of neuromuscular electrical stimulation on tibialis anterior muscle of spastic hemiparetic children. Rev. Bras. Fisioter. 12, 317–323. doi:10.1590/S1413-35552008000400011
Ottenbacher K. J., Msall M. E., Lyon N., Duffy L. C., Ziviani J., Granger C. V., et al. (2000). The WeeFIM instrument: Its utility in detecting change in children with developmental disabilities. Arch. Phys. Med. Rehabil. 81, 1317–1326. doi:10.1053/apmr.2000.9387
Özen N., Unlu E., Karaahmet O. Z., Gurcay E., Gundogdu I., Umay E. (2021). Effectiveness of functional electrical stimulation - cycling treatment in children with cerebral palsy. Malawi Med. J. 33, 144–152. doi:10.4314/mmj.v33i3.1
Palisano R., Rosenbaum P., Walter S., Russell D., Wood E., Galuppi B. (2007). Expanded and revised gross motor function classification system. Can Child Centre for Childhood Disability Research. Canada: McMaster University.
Pollock D., Tricco A. C., Peters M. D. J., Mclnerney P. A., Khalil H., Godfrey C. M., et al. (2022). Methodological quality, guidance, and tools in scoping reviews: A scoping review protocol. JBI Evid. Synth. 20, 1098–1105. doi:10.11124/JBIES-20-00570
Pool D., Blackmore A. M., Bear N., Valentine J. (2014). Effects of short-term daily community walk aide use on children with unilateral spastic cerebral palsy. Pediatr. Phys. Ther. 26, 308–317. doi:10.1097/PEP.0000000000000057
Pool D., Valentine J., Bear N., Donnelly C. J., Elliott C., Stannage K. (2015). The orthotic and therapeutic effects following daily community applied functional electrical stimulation in children with unilateral spastic cerebral palsy: A randomised controlled trial. BMC Pediatr. 15, 154. doi:10.1186/s12887-015-0472-y
Pool D., Elliott C., Bear N., Donnelly C. J., Davis C., Stannage K., et al. (2016). Neuromuscular electrical stimulation-assisted gait increases muscle strength and volume in children with unilateral spastic cerebral palsy. Dev. Med. Child. Neurol. 58, 492–501. doi:10.1111/dmcn.12955
Prosser L. A., Curatalo L. A., Alter K. E., Damiano D. L. (2012). Acceptability and potential effectiveness of a foot drop stimulator in children and adolescents with cerebral palsy: Functional Electrical Stimulation in CP. Dev. Med. Child. Neurol. 54, 1044–1049. doi:10.1111/j.1469-8749.2012.04401.x
Qi Y.-C., Niu X.-L., Gao Y.-R., Wang H., Hu M., Dong L.-P., et al. (2018). Therapeutic effect evaluation of neuromuscular electrical stimulation with or without strengthening exercise on spastic cerebral palsy. Clin. Pediatr. 57, 580–583. doi:10.1177/0009922817732619
Rajalaxmi V., Sudhakar S., Kuppuswamy P., Anusiya K., Balathandayutham K. (2017). Effectiveness of neuromuscular electrical stimulation and cryotherapy on reducing spasticity in diplegic cerebral palsy. Biomedicine 37 (3), 369–374.
Rankin J., Cans C., Garne E., Colver A., Dolk H., Uldall P., et al. (2010). Congenital anomalies in children with cerebral palsy: A population-based record linkage study. Dev. Med. Child. Neurol. 52, 345–351. doi:10.1111/j.1469-8749.2009.03415.x
Robinson B. S., Williamson E. M., Cook J. L., Harrison K. S., Lord E. M. (2015). Examination of the use of a dual-channel functional electrical stimulation system on gait, balance and balance confidence of an adult with spastic diplegic cerebral palsy. Physiother. Theory Pract. 31, 214–220. doi:10.3109/09593985.2014.982774
Rose J., McGill K. C. (2005). Neuromuscular activation and motor-unit firing characteristics in cerebral palsy. Dev. Med. Child. Neurol. 47, 329–336. doi:10.1017/S0012162205000629
Russell D. J., Avery L. M., Rosenbaum P. L., Raina P. S., Walter S. D., Palisano R. J. (2000). Improved scaling of the gross motor function measure for children with cerebral palsy: Evidence of reliability and validity. Phys. Ther. 80, 873–885. doi:10.1093/ptj/80.9.873
Salazar A. P., Pagnussat A. S., Pereira G. A., Scopel G., Lukrafka J. L. (2019). Neuromuscular electrical stimulation to improve gross motor function in children with cerebral palsy: A meta-analysis. Braz. J. Phys. Ther. 23, 378–386. doi:10.1016/j.bjpt.2019.01.006
Sanger T. (2015). Movement disorders in cerebral palsy. J. Pediatr. Neurol. 13, 198–207. doi:10.1055/s-0035-1558866
Schiariti V., Longo E., Shoshmin A., Kozhushko L., Besstrashnova Y., Król M., et al. (2018). Implementation of the international classification of functioning, disability, and health (ICF) core sets for children and youth with cerebral palsy: Global initiatives promoting optimal functioning. Int. J. Environ. Res. Public Health 15, 1899. doi:10.3390/ijerph15091899
Schwartz M. H., Rozumalski A. (2008). The gait deviation index: A new comprehensive index of gait pathology. Gait Posture 28, 351–357. doi:10.1016/j.gaitpost.2008.05.001
Shideler B. L., Bulea T. C., Chen J., Stanley C. J., Gravunder A. J., Damiano D. L. (2020). Toward a hybrid exoskeleton for crouch gait in children with cerebral palsy: Neuromuscular electrical stimulation for improved knee extension. J. Neuroeng. Rehabil. 17, 121. doi:10.1186/s12984-020-00738-7
Stackhouse S. K., Binder-Macleod S. A., Stackhouse C. A., McCarthy J. J., Prosser L. A., Lee S. C. K. (2007). Neuromuscular electrical stimulation versus volitional isometric strength training in children with spastic diplegic cerebral palsy: A preliminary study. Neurorehabil. Neural Repair 21, 475–485. doi:10.1177/1545968306298932
Tricco A. C., Lillie E., Zarin W., O’Brien K. K., Colquhoun H., Levac D., et al. (2018). PRISMA extension for scoping reviews (PRISMA-ScR): Checklist and explanation. Ann. Intern. Med. 169, 467–473. doi:10.7326/M18-0850
van der Linden M., Hazlewood M., Aitchison A., Hillman S., Robb J. (2003). Electrical stimulation of gluteus maximus in children with cerebral palsy: Effects on gait characteristics and muscle strength. Dev. Med. Child. Neurol. 45, 385–390. doi:10.1017/S0012162203000732
van der Linden M. L., Hazlewood M. E., Hillman S. J., Robb J. E. (2008). Functional electrical stimulation to the dorsiflexors and quadriceps in children with cerebral palsy. Pediatr. Phys. Ther. 20, 23–29. doi:10.1097/PEP.0b013e31815f39c9
Vance C. G. T., Rakel B. A., Blodgett N. P., DeSantana J. M., Amendola A., Zimmerman M. B., et al. (2012). Effects of transcutaneous electrical nerve stimulation on pain, pain sensitivity, and function in people with knee osteoarthritis: A randomized controlled trial. Phys. Ther. 92, 898–910. doi:10.2522/ptj.20110183
Walhain F., Desloovere K., Declerck M., Van Campenhout A., Bar‐On L. (2021). Interventions and lower‐limb macroscopic muscle morphology in children with spastic cerebral palsy: A scoping review. Dev. Med. Child. Neurol. 63, 274–286. doi:10.1111/dmcn.14652
Wegrzyk J., Fouré A., Vilmen C., Ghattas B., Maffiuletti N. A., Mattei J.-P., et al. (2015). Extra Forces induced by wide-pulse, high-frequency electrical stimulation: Occurrence, magnitude, variability and underlying mechanisms. Clin. Neurophysiol. 126, 1400–1412. doi:10.1016/j.clinph.2014.10.001
Wright M., Wallman L., Campbell S., Palisano R., Orlin M. (2012). Physical therapy for children. St. Louis, MO: Saunders Elsevier.
Wright P. A., Durham S., Ewins D. J., Swain I. D. (2012). Neuromuscular electrical stimulation for children with cerebral palsy: A review. Arch. Dis. Child. 97, 364–371. doi:10.1136/archdischild-2011-300437
Zahradka N., Behboodi A., Sansare A., Lee S. C. K. (2021). Evaluation of individualized functional electrical stimulation-induced acute changes during walking: A case series in children with cerebral palsy. Sensors 21, 4452. doi:10.3390/s21134452
Keywords: cerebral palsy, exercise, transcutanenous electric nerve stimulation, gait, neuromuscular electrical simulation
Citation: Greve KR, Joseph CF, Berry BE, Schadl K and Rose J (2022) Neuromuscular electrical stimulation to augment lower limb exercise and mobility in individuals with spastic cerebral palsy: A scoping review. Front. Physiol. 13:951899. doi: 10.3389/fphys.2022.951899
Received: 24 May 2022; Accepted: 25 July 2022;
Published: 30 August 2022.
Edited by:
Ferdinand Von Walden, Karolinska Institutet (KI), SwedenReviewed by:
Annika Kruse, University of Graz, AustriaCopyright © 2022 Greve, Joseph, Berry, Schadl and Rose. This is an open-access article distributed under the terms of the Creative Commons Attribution License (CC BY). The use, distribution or reproduction in other forums is permitted, provided the original author(s) and the copyright owner(s) are credited and that the original publication in this journal is cited, in accordance with accepted academic practice. No use, distribution or reproduction is permitted which does not comply with these terms.
*Correspondence: Kelly R. Greve, a2VsbHkuZ3JldmVAY2NobWMub3Jn
†ORCID: Kelly R. Greve, orcid.org/0000-0001-9744-4326
Disclaimer: All claims expressed in this article are solely those of the authors and do not necessarily represent those of their affiliated organizations, or those of the publisher, the editors and the reviewers. Any product that may be evaluated in this article or claim that may be made by its manufacturer is not guaranteed or endorsed by the publisher.
Research integrity at Frontiers
Learn more about the work of our research integrity team to safeguard the quality of each article we publish.