- 1Universidade de São Paulo, Pirassununga, São Paulo, Brazil
- 2Novus International Inc., St. Charles, MO, United States
- 3Cobb Vantress, São Paulo, Brazil
Trace mineral minerals Zn, Cu, and Mn play important roles in breeder production and progeny performance. The objective of this study was to determine maternal supplementation of trace mineral minerals on breeder production and progeny growth and development. A total of 540 broiler breeders, Cobb 500 (Slow feathering; 0–66 weeks old) were assigned to one of three treatment groups with the same basal diet and three different supplemental trace minerals: ITM–inorganic trace minerals in sulfates: 100, 16, and 100 ppm of Zn, Cu, and Mn respectively; MMHAC -mineral methionine hydroxy analog chelate: 50, 8, and 50 ppm of bis-chelated MINTREX®Zn, Cu and Mn (Novus International, Inc.), and TMAAC - trace minerals amino acid complex: 50, 8, and 50 ppm of Zn, Cu, and Mn. At 28 weeks of age, eggs from breeder treatments were hatched for progeny trial, 10 pens with 6 males and 6 female birds per pen were fed a common diet with ITM for 45 days. Breeder production, egg quality, progeny growth performance, mRNA expression of gut health associated genes in breeder and progeny chicks were measured. Data were analyzed by one-way ANOVA; means were separated by Fisher’s protected LSD test. A p-Value ≤ 0.05 was considered statistically different and 0.1 was considered numerical trend. Breeders on ITM treatment had higher (p < 0.05) body weight (BW), weight gain and lower (p < 0.05) feed conversion ratio (FCR) from 0 to 10 weeks, when compared to birds fed MMHAC. MMHAC significantly improved egg mass by 3 g (p < 0.05) and FCR by 34 points (0.05 < p < 0.1) throughout the reproductive period (26–66 weeks) in comparison to ITM. MMHAC improved (p < 0.01) egg yolk color versus (vs.) ITM and TMAAC in all periods, except 28 weeks, increased (p < 0.01) eggshell thickness and resistance vs. TMAAC at 58 weeks, and reduced (p < 0.05) jejunal NF-κB gene expression vs. TMAAC at 24 weeks. There was a significant reduction in tibial dry matter weight, Seedor index and resistance for the breeders that received MMHAC and/or TMAAC when compared to ITM at 18 weeks. Lower seedor index but numerically wider tibial circumference was seen in hens fed MMHAC at 24 weeks, and wider tibial circumference but lower tibial resistance in hens fed TMAAC at 66 weeks. Maternal supplementation of MMHAC in breeder hens increased (p < 0.0001) BW vs. ITM and TMAAC at hatching, reduced (p < 0.05) feed intake vs. ITM at d14 and d28, and improved (p < 0.01) FCR and performance index vs. TMAAC at d28, reduced (p < 0.01) NF-κB gene expression and increased (p < 0.05) A20 gene expression vs. TMAAC on d0 and vs. ITM on d14, reduced (p < 0.05) TLR2 gene expression vs. ITM on d0 and vs. TMAAC on d14, increased (p < 0.05) MUC2 gene expression vs. both ITM and TMAAC on d45 in progeny jejunum. Overall, these results suggest that supplementation with lower levels of MHA-chelated trace minerals improved breeder production and egg quality and reduced breeder jejunal inflammation while maintaining tibial development in comparison to those receiving higher inorganic mineral supplementation, and it also carried over the benefits to progeny with better growth performance, less jejunal inflammation and better innate immune response and gut barrier function in comparison to ITM and/or TMAAC.
Introduction
Trace minerals such as zinc (Zn), copper (Cu), and manganese (Mn) are essential cofactors for hundreds of cellular enzymes and transcription factors in all animal species, including poultry, and are integral in a wide variety of biochemical and physiological processes. Zn plays a significant role in many biological processes, such as cell proliferation and animal growth, immune system development and response, reproduction, gene regulation, and protection against oxidative stress and damage (Zhao et al., 2016; Qi et al., 2019; Londero et al., 2020). Like Zn, Cu is essential for a wide variety of health and performance-related functions in all animal species. For instance, Cu regulates collagen crosslinking therefore promoting skin, bone, tendon and intestinal integrity (Rath et al., 1999). Mn is essential for growth and fertility and plays a very important role in bone development in the embryo and after hatching in birds (Gallup and Norris, 1939; Fawcett, 1994; Underwood and Suttle, 2001).
Historically, animal feeds have been supplemented with low cost, inorganic forms of Zn, Cu, and Mn, such as oxides and sulfates. However, these inorganic salts tend to dissociate in the low pH environment of the upper gastrointestinal tract, leaving the minerals susceptible to reaction with other compounds that might impair absorption thereby decreasing mineral bioavailability and increasing mineral excretion and oxidative stress (Underwood and Suttle, 2001). Organic trace mineral (OTM) varies between products and are determined by the type of ligands used to bind with the mineral. Mineral–organic ligand combinations can result in the production of one of several classes of OTMs. These ligands are usually amino acids, peptides, polysaccharides and organic acids (Byrne et al., 2021). In general, organic microminerals are absorbed anywhere in the small intestine by intestinal carriers of amino acids and peptides while inorganic metals are generally in duodenum by intestinal transporters, so competition between minerals for the same absorption mechanism is reduced. Therefore, not only is the bioavailability of nutrients higher, but additionally minerals in organic form are readmitted to the tissues, and kept stored for a longer time compared to inorganic minerals (Lopes, et al., 2017). It should also be noted that not all organic trace mineral forms are equally stable at low pH and consequently the bioavailability of nutrients can be variable (Brown and Zeringue, 1994; Cao et al., 2000; Guo et al., 2001). Since organic trace minerals have greater bioavailability than inorganic trace minerals, the bis-chelated trace minerals, MMHAC, are chelated to a hydroxy methionine (MHA) analog, which is not an amino acid, but an organic acid. MHA is the precursor of methionine and differs from methionine by having a hydroxyl group instead of an amino group on the alpha carbon. The bis-chelates form 2 structural rings, which provide a higher protection for the metal in challenging environments such as the upper gastro-intestinal tract. This results in a much smaller dissociation rate versus other structures, such as the organic metal amino acid complexes or proteinates. MMHAC has been shown to be highly bioavailable with higher metallothionein synthesis in the enterocytes and/or greater metal deposition in bones than is seen with inorganic trace minerals (Yan and Waldroup, 2006; Richards et al., 2015; Min et al., 2019). Numerous studies have shown organic trace minerals to improve characteristics such as production performance, egg quality, reproduction, antioxidant status, mineral bioavailability and fecal mineral excretion in breeders, and bone development in broilers (Araújo et al., 2019; Wang et al., 2019a, 2019b; Güz et al., 2019; Londero et al., 2020). However, there are few studies evaluating the effects of feeding lower levels of organic trace minerals on breeder development and progeny growth and development. Therefore, the aim of this study was to evaluate the effect of two different organic trace minerals at low levels compared with inorganic trace minerals at high levels on performance (starter, growth and pre-reproduction, and reproduction stages), egg quality, gut health and bone development of broiler breeders from 1 day to 66 weeks of age and on progeny growth and development.
Materials and methods
Animals and management
The project was approved by the Ethics Committee on animal use (CEUA) of the Faculty of Animal Science and Food Engineering (FZEA) of the University of São Paulo. The study was conducted over a total period of 66 weeks between April 2019 and September 2020 at the Poultry Research Laboratory of the Department of Nutrition and Animal Production of the Faculty of Veterinary Medicine and Animal Science (FMVZ), Fernando Costa campus, Pirassununga, São Paulo - Brazil.
A total of 540 females 1-day old Cobb 500 (Slow feathering) broiler breeders with a uniform body weight (47.35 ± 0.013 g) were randomly assigned to three treatment groups with 15 replicates/treatment and 12 birds/replicate. The three treatment groups were: ITM-inorganic trace minerals in sulfates: 100, 16, and 100 ppm of Zn, Cu, and Mn respectively; MMHAC - mineral methionine hydroxy analog chelate: 50, 8, and 50 ppm of MINTREX®Zn, Cu, and Mn (Novus International, Inc.), respectively, and TMAAC - trace minerals amino acid complex: 50, 8, and 50 ppm of Zn, Cu, and Mn, respectively.
Feeding, water and lighting program were performed as recommended by genetic company, following a daily program the birds were fed ad libitum until the 7th day of age and feed intake was then controlled to ensure the birds reached sexual maturity at the appropriate age and feed intake of all birds was controlled as recommended by management guide. From the 1st to the 24th week, feed supply was adjusted weekly according to the management guide of the genetics company (Cobb, 2020). From the 24th to 66th week, the birds were weighed every 2 weeks to minimize the stress experienced by breeders in the laying phase.
In order for the breeding flock to have good uniformity, it is important to ensure that all birds have the same weight. In the present study, selection of breeding stock was performed twice. One way to accomplish this is to separate overweight and underweight animals from the average, ideal weight at 4 weeks of age, sort and monitor body weight during the growth period. This was followed by repeated sorting at 10 weeks of age, handling all males and removing suboptimal males with visual defects including crooked and bent fingers, abnormalities and bent toes, spinal abnormalities, eye and beak abnormalities. At 4 weeks, 3 birds per box were removed from the study and at 18 weeks another 2 birds per box were removed from the study, according to their body weight (±10% of the reference weight average).
Diet and experimental groups
Basal diets that contained corn, soybean meal, wheat bran and trace minerals (Tables 1, 2) were formulated according to the nutritional requirement recommended in the guide from the genetics company (Cobb, 2020). The feeding program was divided into 6 phases: Starter (0–4 weeks), grower (5–18 weeks), pre-reproduction (19–22 weeks), reproduction I (23–40 weeks), reproduction II (41–50 weeks) and reproduction III (51–66 weeks).
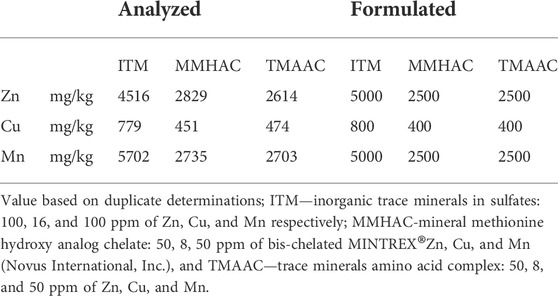
TABLE 2. Concentrations of zinc (Zn) Copper (Cu) and Manganese (Mg) in mineral premix at inclusion 20 kg/MT.
Evaluation of live performance
The growth and development of the broiler breeders was evaluated from 0 to 24 weeks. At the end of each phase (4; 10; 18, and 24 weeks), the birds were evaluated individually for the following characteristics: average body weight (kg), average daily body weight gain (g/bird/day), average feed intake (g) and corrected feed conversion ratio (FCR) (Sakomura and Rostagno, 2016).
At 24 weeks old, the birds started the production phase. The productive performance of the birds was evaluated daily after the stabilization of laying (26 weeks) and a cycle of (26–40, 26–50, and 26–66) was considered. The characteristics evaluated included daily egg production (percentage), daily egg mass (g) and FCR per egg mass. The data obtained were corrected if there was mortality in the group. At 28 weeks, the broiler breeder hens were inseminated with 0.5 ml fresh semen, which was collected by means of abdominal massaging. The eggs were collected from day 3 until day 10 after insemination and placed temporarily in a holding room at 18°C. Subsequently, the eggs from the broiler breeders were incubated in a commercial incubator with automatic turning. Incubation was performed at a temperature of 37.7°C and with relative air humidity of 55%–60%. The egg was turned automatically every hour through an angle of 45°.
Progeny trial
From the hatching eggs of the breeders, a total of 360-day old chicks (180 males and 180 females) were used for part of the study. They were distributed according to their maternal diet in 10 pens with 6 males and 6 female birds per pen for a period of 45 days of age. Progeny received the same basal diet (Table 3) to investigate the effect of nutrient transfer from breeders to progeny.
The broiler diets were based on corn and soybean meal with supplementation of ITM—inorganic trace minerals: 100, 16, and 100 ppm of Zn, Cu, and Mn respectively, as sulfates. The feeding program was divided into 3 phases: starter (1–14 days); grower (15–28 days) and finisher (29–45 days). Water and feed were provided ad libitum. At the end of each phase, the following performance evaluations were measured: body weight (BW), daily body weight gain (DBWG), daily feed intake (DFI), FCR and performance index (PEI) (Bird, 1955).
Egg quality
Egg quality was evaluated at 28, 40, 50, 58, and 65 weeks of age. For two consecutive days, a total 120 eggs per treatment were collected for analysis, identified and, the egg weight (g), yolk coloration, eggshell thickness (mm) and resistance (kgf) were measured using the Digital Egg Tester machine (DET6000, Nabel Co.). For egg quality data, the average of the eggs laid by each experimental unit (box) within the two consecutive days was taken.
Gut health
To determine whether trace minerals affect gut health, expression of nuclear factor kappa B (NF-kB) was measured in the jejunum of 15 breeders per treatment (1 breeder per box) at 24°weeks of age, expression of NF-kB, interleukin 6 (IL6), mucin-2 protein (MUC2), interleukin-1β (IL-1β) and toll like receptor 2 (TLR2) was measured in 10 progeny broilers per treatment (1 bird per pen) at 1, 14, and 45 days of age by qRT-PCR. For extraction of genetic material from the jejunum, the 2-cm segments of jejunum were cut down to Meckel’s diverticulum, then the tissue was carefully opened with sterile scissors (70% alcohol) and very gently cleaned with cold sterile PBS. It was then placed in an aluminum foil, which was closed quickly and immediately and placed into liquid nitrogen for at least 24 h and kept in a freezer (−80°C) until analysis.
Total RNA was extracted from jejunal tissue using the Trizol® (Invitrogen - Thermo Fisher Scientific, Waltham, Massachusetts, United States). Samples were treated with DNaseI (Invitrogen-Thermo Fisher Scientific, Waltham, Massachusetts, United States) and reverse transcription was carried out with High-Capacity cDNA Reverse Transcription Kits (Applied Biosystems - Thermo Fisher Scientific, Waltham, Massachusetts, United States).
The RT-qPCR analysis was conducted using SYBR® Green PCR Master Mix (Applied Biosystems - Thermo Fisher Scientific, Waltham, Massachusetts, United States) on the Step One Plus Real-Time PCR System (Applied Biosystems- Thermo Fisher Scientific, Waltham, Massachusetts, United States). Primers were designed using OligoAnalyzer 3.1 (Integrated DNA Technologies, Coralville, Iowa, United States) (Table 4) and purchased from Invitrogen (Thermo Fisher Scientific, Waltham, Massachusetts, United States). The concentration of primers was optimized and primers were verified with 95%–105% efficiency and linearity of amplification r2 greater than 0.99. Relative fold change in mRNA quantity was normalized to house-keeping gene β-actin and calculated according to 2-ΔΔCT (Livak and Schmittgen, 2001), and the relative expression of all genes were then normalized to ITM treatment.
Bone quality
Bone quality was evaluated in 15 breeders per treatment (1 breeder per box) at 18, 24, and 66 weeks of age. The right tibia was removed from the birds slaughtered for evaluation of wet weight (WW), dry weight (DW), dry matter (DM), length (LGTH), circumference (CIR) and resistance (R) were measured. For bone density, the Seedor index (bone weight/bone length) was calculated.
The tibias were desiccated to determine bone resistance using a universal EMIC® test machine, model DL 3000, with load applied at a speed of 5 mm/min and a load cell of 2000 N. In the three-point flexion test, the tibias were held in the horizontal position with support span distance of 3/4 the size of the bone and the force was applied at a point half way between the device and the tibia.
Dry matter of the tibia was evaluated using the methodology described by the Association of Official Analytical Chemists (AOAC, 2007). The right tibia was deboned. The weight of the clean crucible (g) and the weight of the sample (g) were measured. Then, the crucibles with the sample were placed in a forced ventilation oven at 105°C for 24 h. The dry matter weight of the sample was determined to be the difference between final weight of sample and crucible less the weight of the crucible. The quotient was multiplied by 1,000 for conversion between mass units (g and kg).
Statistical analysis
The results were analyzed and tested for normality. Observations with a studentized residual greater than three (in absolute value) were considered outliers.
The data were submitted to one way ANOVA analysis and the means of treatments compared by Tukey test using the MIXED PROC of the Statistical Analysis System (SAS) software (Version 9.4, 2013). A p value ≤ 0.05 was considered as significant and 0.05 < p value ≤ 0.1 was considered as a trend.
Results
Performance of broiler breeders during rearing phase
There was no significant difference in average body weight (BW), daily body weight gain (DBWG) and feed conversion (FCR) between treatments except for the period 0–10 weeks (grower phase) (Table 5). During the grower phase, BW and DBWG were lower for the breeders in MMHAC than those in ITM but not different from TMAAC. FCR was better in the ITM group than MMHAC but there was no difference between ITM and TMAAC groups. There was no significant difference in daily feed intake (DFI) between any groups in the evaluated periods.
Performance of broiler breeders in the reproduction phase
There was no significant difference in egg production (%) and FCR per egg mass (Table 6).
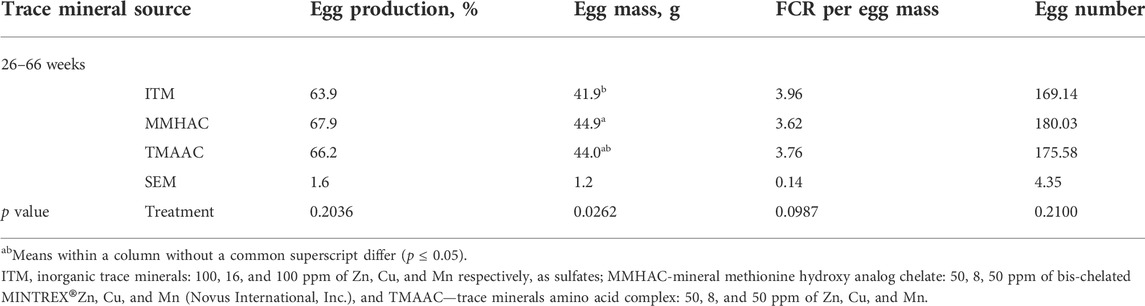
TABLE 6. Effect of feeding supplemental inorganic or organic trace minerals on the performance of broiler breeders (26–66 weeks).
For egg mass, a significant difference (p = 0.0262) was observed between treatments at 26–66 weeks (Table 6). MMHAC improved egg mass vs. ITM during weeks 26–66 (p = 0.0262, Table 6).
Egg quality
There was no significant difference in egg weight at any time points (Table 7). MMHAC supplementation enhanced (p < 0.009) yolk coloration in comparison to both ITM and TMAAC at all time points except for week 28, improved eggshell resistance vs. TMAAC at week 50 (p = 0.0913) and week 58 (p = 0.0027), decreased (p = 0.0196) eggshell thickness vs. ITM but not different from TMAAC at week 28 and increased (p = 0.002) eggshell thickness vs. TMAAC at week 58 (Table 7).
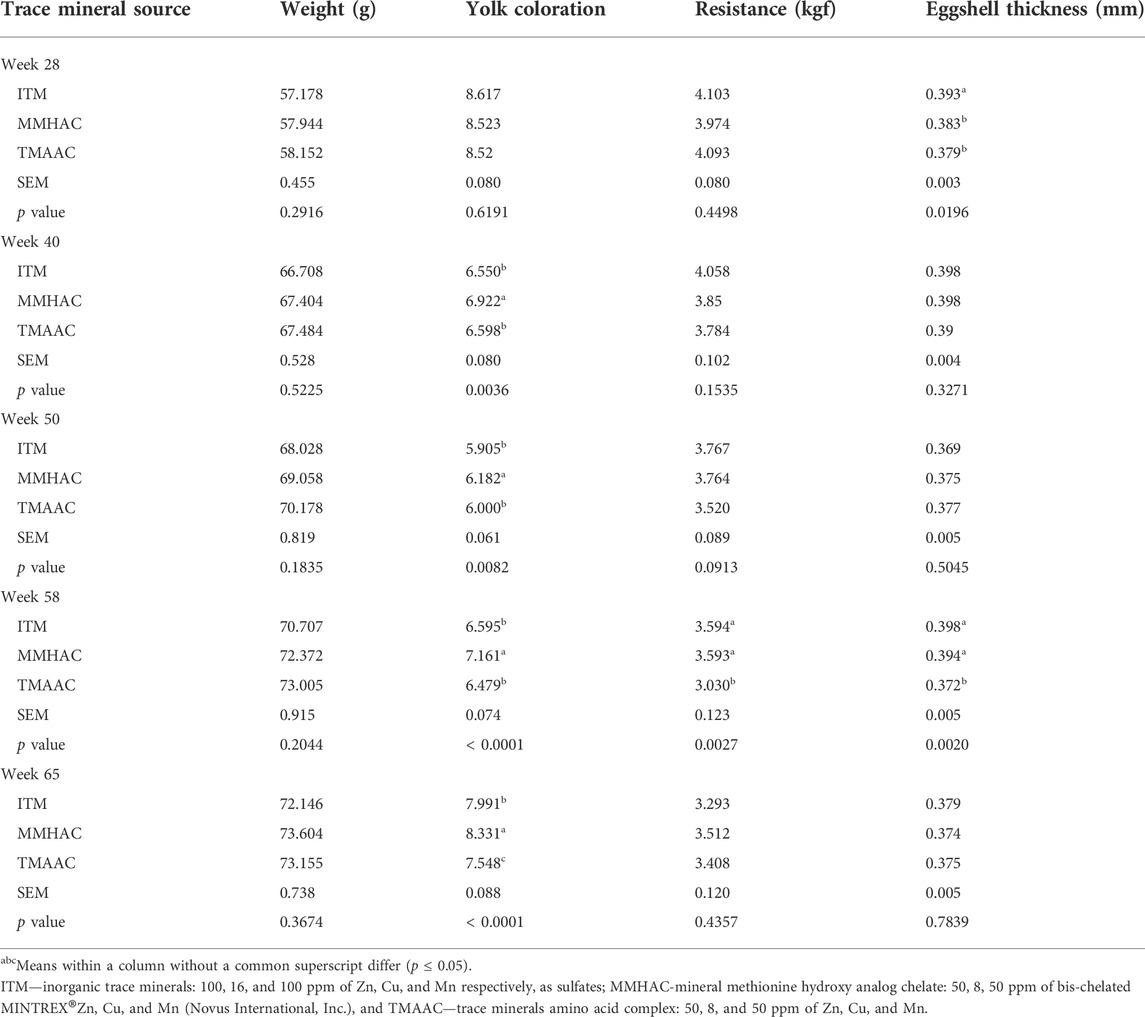
TABLE 7. Effect of inorganic or organic trace minerals on egg quality parameters of broiler breeders.
Gut health of broiler breeders
To determine whether trace minerals affect gut health, NF-κB mRNA expression was measured in the jejunum of breeders at 24 weeks of age. In the groups with diets supplemented with MMHAC, NF-κB mRNA expression was significantly lower than TMAAC and numerically lower than ITM (Figure 1), indicating that supplementation with MMHAC reduced jejunal inflammation in broiler breeder.
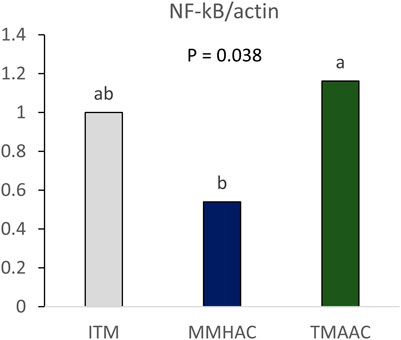
FIGURE 1. Effect of inorganic or organic trace minerals on jejunal NFκB gene expression in broiler breeders at 24 weeks of age. ab Means without a common superscript differ (p ≤ 0.05). ITM—inorganic trace minerals: 100, 16, and 100 ppm of Zn, Cu, and Mn respectively, as sulfates; MMHAC-mineral methionine hydroxy analog chelate: 50, 8, 50 ppm of bis-chelated MINTREX–Zn, Cu, and Mn (Novus International, Inc.), and TMAAC—trace minerals amino acid complex: 50, 8, and 50 ppm of Zn, Cu, and Mn.
Bone quality
At 18 weeks of age, there was no significant difference between treatments for wet weight (WW), length and circumference of the tibia (Table 8). Dry weight, dry matter, Seedor index and resistance were significantly reduced in MMHAC treatment when compared to ITM, but not different from TMAAC.
At 24 weeks of age, MMHAC significantly (p = 0.0399) reduced seeder index and numerically (p = 0.092) increased tibial circumference in comparison to ITM and TMAAC. There were no significant differences in other tibial parameters between treatments.
At the end of the study, 66 weeks, MMHAC supplementation did not significantly alter bone characteristic parameters in comparison with ITM and TMAAC except for a lower (p < 0.0001) tibial circumference and numerically higher tibial resistance than TMAAC. Although TMAAC treatment resulted in a higher tibial circumference than ITM and MMHAC, tibial resistance was lower (p = 0.0133) than ITM.
Performance of the progeny
The body weight of progeny chicks at hatch was significantly higher in MMHAC treatment than TMAAC and ITM treatments (Table 9). During starter phase (1–14 days), there was no significant difference in BW, DBWG, FCR, and PEI between treatments, however, FI was significantly (p = 0.0231) reduced in MMHAC when compared to ITM, but not different from TMAAC (Table 9).
During 1–28 days, maternal supplementation of MMHAC significantly (p = 0.0118) reduced FI vs. ITM and improved FCR (p = 0.0051) and PEI (p = 0.0262) vs. TMAAC (Table 9).
Considering performance characteristics across the entire period, 0–45 days, BW, DBWG, FCR and PEI were not different between treatments, FI was numerically (p = 0.0665) reduced in MMHAC and TMAAC treatments compared with ITM.
Gut health of progeny
To determine whether trace minerals in the maternal diet affect gut health of the progeny, MUC2, IL6, NF-κB, IL1β, A20, and TLR2 mRNA expression was measured in the jejunum of progeny at 1, 14, and 45 days of age (Figure 2).
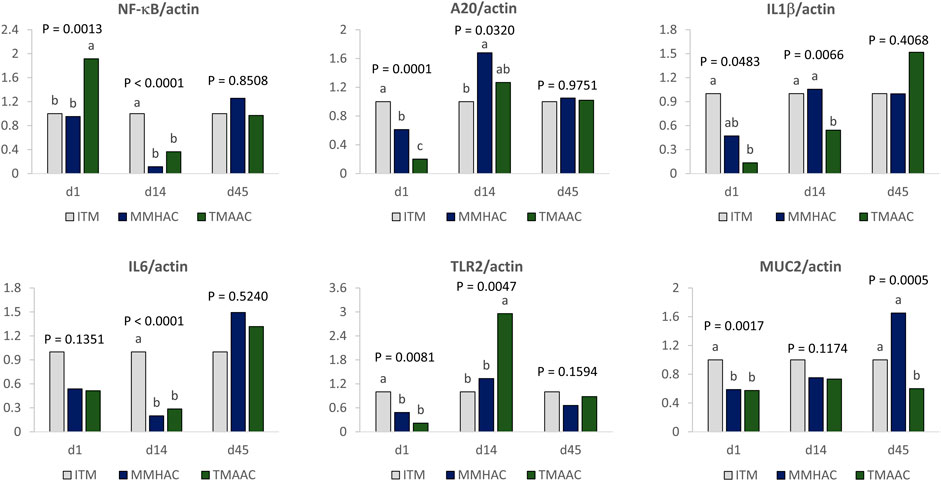
FIGURE 2. Effect of inorganic or organic trace minerals on jejunal anti-inflammatory gene expression in progeny broilers at 1-, 14-, and 45-days of age. ab Means without a common superscript differ (p ≤ 0.05). ITM—inorganic trace minerals: 100, 16, and 100 ppm of Zn, Cu and Mn respectively, as sulfates; MMHAC-mineral methionine hydroxy analog chelate: 50, 8, 50 ppm of bis-chelated MINTREX®Zn, Cu, and Mn (Novus International, Inc.), and TMAAC—trace minerals amino acid complex: 50, 8, and 50 ppm of Zn, Cu, and Mn.
At hatch, in the groups with maternal diets supplemented with MMHAC, TLR2, and MUC2 mRNA expression was lower than ITM but not different from TMAAC, NF-κB mRNA expression was significantly lower than TMAAC but no different from ITM, A20 gene expression was lower than ITM and higher than TMAAC (Figure 2).
At the end of starter stage, 14 days of age, MMHAC maternal supplementation resulted in significantly (p < 0.0001) lower IL6 and NF-κB mRNA expression for the progeny in comparison with ITM but there was no difference from TMAAC. Progeny from maternal breeders with MMHAC supplementation also had higher IL1β and lower TLR2 expression than TMAAC, and higher A20 expression than ITM.
At the end of the study, at 45 days of age, the only gut health parameter significantly altered by maternal MMHAC supplementation in comparison with ITM and TMAAC was MUC2 mRNA expression. Maternal MMHAC supplementation significantly (p = 0.0005) increased MUC2 mRNA expression in comparison with ITM and TMAAC.
Discussion
In this study, supplementation of low dose of MMHAC and TMAAC had similar development and growth performance as high dose of ITM in broiler breeders, and tended to improve egg production % and egg mass and FCR per egg mass as compared to high dose of ITM. MMHAC also improved egg yolk color in comparison to ITM and TMAAC at most time points and eggshell resistance and thickness vs. TMAAC at 58 weeks. These benefits are probably due to higher bioavailability of chelated Zn, Cu, and Mn-methionine hydroxy analogs than inorganic trace minerals as reported previously (Yan and Waldroup, 2006; Richards et al., 2015; Min et al., 2019).
Consistent with the findings in this study, a number of other studies have showed beneficial effects of chelated Zn-MHA and chelated Zn, Cu, and Mn-MHA in breeder reproduction performance and egg quality. For example, supplementation of 0.5 and 1 g/kg MMHAC analog and organic Se significantly improved egg production by 8.4–9.3% points, egg mass by 6.28–7.23 g of egg/hen/day and feed conversion ratio by 28–32 points in comparison to ITM in commercial laying hens raised under high ambient temperature. It also increased eggshell thickness and albumen width, reduced total cholesterol in serum and egg yolk and malondialdehyde in egg yolk, and increased antibody titers against avian influenza H9N1. This suggests that supplementation with MMHAC enhances reproductive performance, shell quality characteristics, plasma profile, yolk mineral concentration, yolk lipid oxidation, and immune response in laying hens under high ambient temperature (Saleh et al., 2020). Consistent with these findings, MMHAC supplementation in breeders over the 80 weeks produced a 4.1% increase in total egg production per hen housed (326 vs. 339) and a 4.9% increase in hatchable eggs per hen housed (119 vs. 124) vs. ITM (Parker, 2013). Li et al. (2019) reported that shell strength was mostly related to mammillary cone width in the ultrastructure, which is affected by the pattern of calcium deposition during eggshell formation. Furthermore, the supplementation of 80 mg/kg chelated Zn-methionine hydroxy analog in Hyline Grey layers significantly increased egg weight and eggshell strength (Li et al., 2019). This was probably caused by Zn-induced changes in Ca deposits in the eggshell. The addition of Zn increases the utilization of Ca to improve the qualitative parameters of the eggshell.
Similarly, Min et al. (2018) found that supplementation of 40 or 80 mg/kg chelated Zn-methionine hydroxy analog significantly increased eggshell thickness and eggshell strength by promoting Ca deposition and carbonic anhydrase activity and reduced the percentage of broken eggs in comparison to 80 mg/kg ZnSO4 (Min et al., 2018).
Araújo et al. (2019) observed that low levels of organic trace minerals Zn:Cu:Mn:Fe, in the form of carbo-amino-phosphates, increased egg production and eggshell thickness and breaking strength in broiler breeders at 34, 46, and 52 weeks of age when compared to high levels of inorganic trace minerals (Araújo et al., 2019). Wang et al., 2019a reported that organic trace minerals Zn, Cu, Mn, Fe, and Se, in the form of proteinate, increased laying rate by 9.56% in comparison to the same levels of ITM, but not with respect to high levels of ITM (Wang et al., 2019b). However, the same supplementation did not improve egg weight and egg quality vs. the same or higher levels of ITM (Wang et al., 2019a). Although Umar Yaqoob et al., 2020 observed no significant difference of the productive performance, higher eggshell thickness was seen when broiler breeders were fed low levels of organic trace minerals Zn, Cu, Mn, and Fe in the form of complexed glycinate in comparison to commercially recommended high levels of ITM during weeks 23–37 (Umar Yaqoob et al., 2020).
The influence of Zn supplementation levels on productive indices of laying hens is inconsistent. A meta-analysis from 11 studies showed that dietary organic and/or inorganic Zn supplementation reduced FCR and increased egg weight and egg mass compared with control diets without Zn supplementation (Ogbuewu and Mbajiorgu, 2022). The results of meta-analysis were affected by the age and breed/strain of hen, and supplementation levels and duration. In this meta-analysis, Zn source (organic vs. inorganic) had no effect on feed intake, but organic Zn significantly improved FCR, egg production%, egg quality (including egg weight, egg mass, Haugh unit, eggshell thickness, eggshell weight), and blood Zn concentrations in laying hens. Supplementation of 25, 50, 75 or 100 ppm of zinc methionine had no effect on body weight, body weight gain or feed conversion ratio in comparison to a control diet without Zn supplementation in Hisex Brown laying hens from 22 to 34 weeks of age, and 100 ppm of zinc methionine significantly increased egg number, egg weight and egg mass compared to other groups (Abd El-Hack et al., 2018). These findings suggest that organic Zn has greater bioavailability than inorganic Zn, leading to better nutrient digestibility and absorption in the gastrointestinal tract thereby greater reproduction performance.
The changes in breeder productivity with different forms of organic trace minerals may be related to the type of ligand on the mineral. In the present study, each molecule of Zn, Cu, and Mn was chelated to 2 molecules of methionine hydroxy analog by covalent bonds, which are far more stable than ionic bonds (Kratzer, 2017). The bis-chelate structure of MMHAC protects the minerals during transit through the gastrointestinal tract and ensures the mineral is delivered to the absorption site in the small intestine. The ligand, methionine hydroxy analog, is not an amino acid but an organic acid, 2-hydroxy-4-methyliobutanoic. It is the precursor of Met, and rapidly absorbed and converted into L-methionine in the bird (Dibner, 2003). Bis-chelated Zn has been shown to have greater bioavailability, with a higher metallothionein expression in the enterocytes or a higher amount of Zn deposition in bones, than other forms of organic Zn, such as Zn-Met, Zn proteinate and Zn amino acid complex (Richards et al., 2008a; Richards et al., 2008b). Supplementation of methionine hydroxy analog bis-chelated Zn to breeders also increased apparent retention of minerals such as Cu, Zn, and Ca and nutrients such as crude protein and energy, expression of liver metallothionein, tibial strength and tibial Zn and Ca deposition in aged hens (Min et al., 2019). The numerically higher egg production, egg mass, egg quality and better FCR per egg mass in MMHAC group is probably due to the greater bioavailability and nutrient retention of MMHAC than the TMAAC in this study.
It has been reported that the effect of maternal dietary organic trace mineral supplementation can be felt beyond the embryonic stage (Zorzetto et al., 2021). It is well recognized that the trace minerals Zn, Cu, and Mn could potentially impact optimal gut health of young monogastric animals (Shannon and Hill, 2019). Zn is known to be essential in many biological functions in mammals, such as anti-inflammation, anti-diarrhea, and maintenance of the integrity of the epithelial barrier (Roselli et al., 2003; Patel et al., 2010). Supplementation with zinc oxide with small particle size improved gut morphometry and gut barrier function in Ross 308 broiler breeder hens (Barzegar et al., 2021) and increased anti-sheep red blood cells antibody titer, IgM, the total number of leukocytes, and the percentage of lymphocytes in Cobb500 breeder hens (Sharideh et al., 2019). Adequate or higher maternal Zn supplementation to breeder hens not only improved breeder performance with 4%–7% higher laying rate, but also enhanced progeny immune response and antioxidant ability via epigenetic mechanisms (Huang et al., 2019). For example, supplementation of bis-chelated Zn-MHA in breeder hens improved progeny immunity by down-regulation of NF-kB mediated intestinal inflammation (Li et al., 2015). The NF-κB signaling pathway is one of the major pathways regulating chronic inflammation and inflammation induced by external stimuli such as bacteria, infection (Neurath et al., 1998). Zinc finger protein A20 is a signaling molecule that blocks the phosphorylation and activation of NF-κB, thereby inhibiting translocation of NF-κB and suppressing the inflammatory cascade and reducing expression of proinflammatory cytokines (Ma and Malynn, 2012; Catrysse et al., 2014). Therefore, upregulation of A20 would downregulate the NF-κB pathway and reduce inflammation. In the current study, MMHAC supplementation significantly reduced NF-κB mRNA expression vs. TMAAC in breeders and decreased NF-κB mRNA expression in progeny chicks vs. either ITM or TMAAC on d0 and d14, and upregulated A20 gene expression vs. ITM on d14. These results suggest that the downregulation of the NF-κB pathway in progeny birds might be caused by the upregulation of A20 gene expression. This is consistent with the study by Li et al. (2015), in which chelated Zn supplementation to breeder hens exhibited greater effects than ZnSO4 in reducing gene expression of NF-κB p65 and its downstream inflammatory cytokine IL6 by upregulating A20 gene expression via epigenetic modification in the jejunum of progeny. MUC2 is the main intestinal mucin of the mucus layer that protects the integrity of gut epithelial cells and an increase of MUC2 gene expression indicates better gut barrier function. TLR2 is one of the toll-like receptors that plays an important role in the identification of distinct molecular patterns from invading pathogens including bacteria, fungi, parasites, and viruses (Mukherjee et al., 2016). As the intestine is the first line barrier for pathogens, the greater reduction of jejunal TLR2 expression in progeny from MMHAC fed breeders than either ITM or TMAAC fed breeders suggests that maternal MMHAC supplementation probably decreased pathogen challenge in progeny birds. Taken together, these findings suggest that supplementation of the breeder diet with bis-chelated trace minerals, MMHAC, reduces jejunal inflammation and improves the innate immune response and gut barrier function in comparison to ITM and/or mineral amino acid complex.
Another important characteristic in broiler breeder development is bone structure. Bone development occurs early in life. Longitudinal growth in long bones occurs by endochondral ossification and the long bones widen by a process involving intramembranous ossification. At the onset of sexual maturity, osteoblasts undergo a dramatic change, from forming lamellar cortical bone, to producing a woven bone called medullary bone, which is a labile source of calcium for eggshell formation. Medullary bone accumulates rapidly on the surfaces of structural bones, especially leg bones. In early laying stages this is in spicules within the medullary cavities and it continues to accumulate slowly over the remainder laying period (Whitehead, 2004). The medullary bone is weaker than the structural bone, but its bone content is positively correlated with humerus strength (Fleming et al., 1998).
Trace minerals play important roles in bone development. Zinc regulates collagen synthesis (Starcher et al., 1980), osteoblast-associated cellular invasion of cartilage matrix (Dibner et al., 2007) and endochondral ossification (Hatori et al., 1995; Ho et al., 2003; Fraker, 2005). Cu regulates crosslinking of collagen and elastin, which provides tensile strength and elasticity for bone (Carlton and Henderson, 1964). Using organic forms of trace minerals to increase their bioavailability could improve bone mineralization and development. For example, feeding methionine hydroxy analog chelated Zn to breeder hens increased Zn and Ca concentrations in tibias and tibial strength (Min et al., 2019); feeding Zn, Cu, and Mn chelated methionine hydroxy analog to turkeys increased tibial breaking strength and cortical bone width (Ferket et al., 2009). In the current study, overall, most of tibial parameters were not different between groups, indicating that half dose of MMHAC and TMAAC were enough to provide minerals needed for tibial growth and development as compared to high dose of ITM, which is probably also due to the greater bioavailability as shown by increased tibial Zn deposition as reported previously (Richards et al., 2015).
Maternal nutrition can affect embryonic development and progeny growth since all nutrients required for embryo development are acquired by nutrient transfer from the breeders (Richards et al., 2010; Özlü et al., 2018; Emamverdi et al., 2019). More trace minerals could be transferred from breeders to progeny chicks when breeders were fed highly bioavailable source of minerals, MMHAC. This hypothesis is confirmed by a previous study in which Zn-MHAC supplementation to breeders increased Zn deposition in egg yolk and albumin than Zn sulfate (Li et al., 2015). The improvement of progeny growth performance in this study could results from increased amount of trace minerals transferred from breeders to progeny, less inflammation and better gut barrier function and innate immunity. Consistent with this study, supplementation of MHA bis-chelated Zn:Cu:Mn and organic Se to breeder hens increased body weight and FCR of 42-day-old offspring birds compared to the same inclusion levels (50:8:60:0.3 ppm of Zn:Cu:Mn:Se) of ITM from sulfate and Se from Na2SeO3 (Sun et al., 2012). These findings suggest that supplementation of bis-chelated trace minerals MMHAC in the breeder diet improved progeny growth performance in comparison to ITM and mineral amino acid complex.
In summary, in breeder, supplementation with lower levels of MHA-chelated trace minerals improved egg production performance and egg quality and reduced intestinal inflammation while maintaining tibial development in comparison to ITM and/or mineral amino acid complex. In the resultant progeny, maternal supplementation of bis-chelated trace minerals, MMHAC, in the breeder diet improved growth performance and reduced jejunal inflammation in comparison to ITM and mineral amino acid complex. In conclusion, MHA bis-chelated trace minerals performed better, or similarly, to higher concentrations of inorganic trace mineral and/or similar concentrations of trace mineral amino acid complexes in breeders and progeny in this study.
Data availability statement
The original contributions presented in the study are included in the article/Supplementary Materials, further inquiries can be directed to the corresponding authors.
Ethics statement
The animal study was reviewed and approved by Ethics Committee on animal use (CEUA) of the Faculty of Animal Science and Food Engineering (FZEA) of the University of São Paulo.
Author contributions
FR, RA, AM, DT, MA, DH, CA, and LA contributed to conception and design of the study. FR, BL, MT, CG, and PP contributed to desenvolviment of the study. FR and RA organized the database. RC, JC and CG performed the statistical analysis. FR, JC, and LFA wrote sections of the manuscript. All authors contributed to manuscript revision, read, and approved the submitted version.
Funding
Novus International Inc., United States, donated the different sources of organic mineral.
Acknowledgments
This work was carried out with the support of the Coordenação de Aperfeiçoamento de Pessoal do Ensino Superior—Brasil (CAPES)—Código de Financiamento 001. Novus International Inc., United States, donated the different sources of organic mineral.
Conflict of interest
JC, RA, DT, MV-A and DH were employed by Novus International Inc.
The remaining authors declare that the research was conducted in the absence of any commercial or financial relationships that could be construed as a potential conflict of interest.
Publisher’s note
All claims expressed in this article are solely those of the authors and do not necessarily represent those of their affiliated organizations, or those of the publisher, the editors and the reviewers. Any product that may be evaluated in this article, or claim that may be made by its manufacturer, is not guaranteed or endorsed by the publisher.
References
AAFCO (1998). Publicação oficial da Associação Americana Oficial de Controle de Alimentos. Paul. M. Bachman, 237–238.
Abd El-Hack M. E., Alagawany M., Amer S. A., Arif M., Wahdan K. M. M., El-Kholy M. S. (2018). Effect of dietary supplementation of organic zinc on laying performance, egg quality and some biochemical parameters of laying hens. J. Anim. Physiol. Anim. Nutr. 102 (2), e542–e549. doi:10.1111/jpn.12793
Afrouziyeh M., Zukiwsky N. M., Zuidhof M. J. (2021). Timing of growth affected broiler breeder feeding motivation and reproductive traits. Poult. Sci. 100 (9), 101375. doi:10.1016/j.psj.2021.101375
Akaike H. (1973). “Information theory and an extension of the maximum likelihood principle,” in Int. Symp. Inf. Theory. Editors B. N. Petrov, and F. Csaki, 267–281.
Araújo C. S. S., Hermes R. G., Bittencourt L. C., Silva C. C., Araújo L. F., Granghelli C. A., et al. (2019). Different dietary trace mineral sources for broiler breeders and their progenies. Poult. Sci. 98 (10), 4716–4721. doi:10.3382/ps/pez182
Barzegar M., Zagharim M., Zhandi M., Sadeghi M. (2021). Effects of zinc dosage and particle size on gut morphology, tight junctions and TNF-α expression in broiler breeder hens. J. Anim. Physiol. Anim. Nutr. Berl. 106, 772–782. doi:10.1111/jpn.13638
Bird H. R. (1955). performance index” of growing chickens. Poult. Sci. 34 (5), 1163–1164. doi:10.3382/ps.0341163
Brown T. F., Zeringue L. K. (1994). Laboratory Evaluations of solubility and structural integrity of complexed and chelated trace mineral supplements. J. Dairy Sci. 77, 181–189. doi:10.3168/jds.s0022-0302(94)76940-x
Byrne L., Hynes M. J., Connolly C. D., Murphy R. A. (2021). Influence of the chelation process on the stability of organic trace mineral supplements used in animal nutrition. Animals. 11 (6), 1730. doi:10.3390/ani11061730
Cao J., Henry P. R., Guo R., Holwerda R. A., Toth J. P., Littell R. C., et al. (2000). Chemical characteristics and relative bioavailability of supplemental organic zinc sources for poultry and ruminants. J. Anim. Sci. 78, 2039–2054. doi:10.2527/2000.7882039x
Carlton W. W., Henderson W. (1964). Skeletal lesions in experimental copper-deficiency in chickens. Avian Dis. 8, 48–55. doi:10.2307/1587818
Catrysse L., Vereecke L., Beyaert R., Van Loo G. (2014). A20 in inflammation and autoimmunity. Trends Immunol. 35 (1), 22–31. doi:10.1016/j.it.2013.10.005
De Beer M., Coon C. N. (2009). The effect of feed restriction programs and growth curves on reproductive performance, in vitro lipogenesis and heterophil to lymphocyte ratios in broiler breeder hens. Int. J. Poult. Sci. 8, 373–388. doi:10.3923/ijps.2009.373.388
Dibner J. J. (2003). Review of the metabolism of 2-hydroxy-4-(methylthio)butanoic acid. World's. Poult. Sci. J. 59, 99–110. doi:10.1079/WPS20030006
Dibner J. J., Richards J. D., Kitchell M. L., Quiroz M. A. (2007). Metabolic challenges and early bone development. J. Appl. Poult. Res. 16, 126–137. doi:10.1093/japr/16.1.126
Emamverdi M., Zare-Shahneh A., Zhandi M., Zaghari M., Minai-Tehrani D., Khodaei-Motlagh M. (2019). An improvement in productive and reproductive performance of aged broiler breeder hens by dietary supplementation of organic selenium. Theriogenology 126, 279–285. doi:10.1016/j.theriogenology.2018.12.001
Ferket P. R., Oviedo-Rondón E. O., Mente P. L., Bohórquez D. V., Santos A. A., Grimes J. L., et al. (2009). Organic trace minerals and 25-hydroxycholecalciferol affect performance characteristics, leg abnormalities, and biomechanical properties of leg bones of turkeys. Poult. Sci. 88 (1), 118–131. doi:10.3382/ps.2008-00200
Fleming R. H., McCormack H. A., McTeir L., Whitehead C. C. (1998). Medullary bone and humeral breaking strength in laying hens. Res. Vet. Sci. 64 (1), 63–67. doi:10.1016/s0034-5288(98)90117-5
Fraker P. J. (2005). Roles for cell death in zinc deficiency. J. Nutr. 135, 359–362. doi:10.1093/jn/135.3.359
Gallup W., Norris L. (1939). The effect of a deficiency of manganese in the diet of the hen. Poult. Sci. 18, 83–88. doi:10.3382/ps.0180083
Guo R., Henry P. R., Holwerda R. A., Cao J., Littell R. C., Miles R. D., et al. (2001). Chemical characteristics and relative bioavailability of supplemental organic copper sources for poultry. J. Anim. Sci. 79, 1132–1141. doi:10.2527/2001.7951132x
Güz B. C., Molenaar R., De Jong I. C., Kemp B., Van Den Brand H., Van Krimpen M. (2019). Effects of dietary organic minerals. fish oil. and hydrolyzed collagen on growth performance and tibia characteristics of broiler chickens. Poult. Sci. 98, 6552–6563. doi:10.3382/ps/pez427
Hatori M., Klatte K. J., Teixeira C. C., Shapiro I. M. (1995). End labeling studies of fragmented DNA in the avian growth plate: Evidence of apoptosis in terminally differentiated chondrocytes. J. Bone Min. Res. 10, 1960–1968. doi:10.1002/jbmr.5650101216
Ho E., Courtemanche C., Ames B. (2003). Zinc deficiency induces oxidative DNA damage and increases p53 expression in human lung fibroblasts. J. Nutr. 133, 2543–2548. doi:10.1093/jn/133.8.2543
Huang L., Li X., Wang W., Yang L., Zhu Y. (2019). The role of zinc in poultry breeder and hen nutrition: An update. Biol. Trace Elem. Res. 192 (2), 308–318. doi:10.1007/s12011-019-1659-0
Joseph N. S., Robinson F. E., Korver D. R., Renema R. A. (2000). Effect of dietary protein intake during the pullet-to-breeder transition period on early egg weight and production in broiler breeders. Poult. Sci. 79, 1790–1796. doi:10.1093/ps/79.12.1790
Li C., Guo S., Gao J., Guo Y., Du E., Lv Z., et al. (2015). Maternal high-zinc diet attenuates intestinal inflammation by reducing DNA methylation and elevating H3K9 acetylation in the A20 promoter of offspring chicks. J. Nutr. Biochem. 26, 173–183. doi:10.1016/j.jnutbio.2014.10.005
Li L., Miao L., Zhu M., Wang L., Zou X. (2019). Dietary addition of zinc-methionine influenced eggshell quality by affecting calcium deposition in eggshell formation of laying hens. Br. J. Nutr.Br J. Nutr. 122123 (94), 961480–961973. doi:10.1017/S000711451900206X
Livak K. J., Schmittgen T. D. (2001). Analysis of relative gene expression data using real-time quantitative PCR and the 2(-Delta Delta C(T)) Method. Methods 25 (4), 402–408. doi:10.1006/meth.2001.1262
Londero A., Pires A. R., Golin L. F., Oliveira F. M., Guterres A., Moura S. D., et al. (2020). Effect of supplementation with organic and inorganic minerals on the performance. egg and sperm quality and. hatching characteristics of laying breeder hens. Anim. Reprod. Sci. 215, 106309. doi:10.1016/j.anireprosci.2020.106309
Lopes M., Paroul N., Barbosa J., Valduga E., Cansian R. L., Toniazzo G., et al. (2017). Effect of partial and total replacement of inorganic by organic microminerals sources on the quality of broiler carcasses. Braz. Arch. Biol. Technol. 60, e17160082. doi:10.1590/1678-4324-2017160082
Ma A., Malynn B. A. (2012). A20: linking a complex regulator of ubiquitylation to immunity and human disease. Nat. Rev. Immunol. 12 (11), 774–785. doi:10.1038/nri3313
Min Y. N., Liu F. X., Qi X., Ji S., Cui L., Wang Z. P., et al. (2019). Effects of organic zinc on tibia quality, mineral deposit, and metallothionein expression level of aged hens. Poult. Sci. 98 (1), 366–372. doi:10.3382/ps/pey386
Min Y. N., Liu F. X., Qi X., Ji S., Ma S. X., Liu X., et al. (2018). Effects of methionine hydroxyl analog chelated zinc on laying performance, eggshell quality, eggshell mineral deposition, and activities of Zn-containing enzymes in aged laying hens. Poult. Sci. 97 (10), 3587–3593. doi:10.3382/ps/pey203
Mukherjee S., Karmakar S., Babu S. P. (2016). TLR2 and TLR4 mediated host immune responses in major infectious diseases: a review. Braz. J. Infect. Dis. 20, 193–204. doi:10.1016/j.bjid.2015.10.011
Neurath M. F., Becker C., Barbulescu K. (1998). Role of NF-kappaB in immune and inflammatory responses in the gut. Gut 43, 856–860. doi:10.1136/gut.43.6.856
Ogbuewu I. P., Mbajiorgu C. A. (2022). Meta-analysis of zinc supplementation on laying performance, egg quality characteristics, and blood zinc concentrations in laying hens. Biol. Trace Elem. Res. doi:10.1007/s12011-021-03080-8
Özlü S., Elibol O., Brake J. (2018). Effect of storage temperature fluctuation on embryonic development and mortality, and hatchability of broiler hatching eggs. Poult. Sci. 97, 3878–3883. doi:10.3382/ps/pey253
Parker D. (2013).“Benefits of trace minerals, chelated with methionine hydroxy analog, on breeder performance and chick development,” in Feed compounder, 22–25.
Patel A., Mamtani M., Dibley M. J., Badhoniya N., Kulkarni H. (2010). Therapeutic value of zinc supplementation in acute and persistent diarrhea: a systematic review. PLoS One 5, e10386. doi:10.1371/journal.pone.0010386
Qi X., Shuxue M., Liu X., Wang Y., Liu Y., Gao Y., et al. (2019). Effects of the methionine hydroxyl analog chelate zinc on antioxidant capacity and liver metabolism using 1H-NMR-Based metabolomics in aged laying hens. Animals. 9, 898–910. doi:10.3390/ani9110898
Rath N. C., Balog J. M., Huff W. E., Huff G. R., Kulkarni G. B., Tierce J. F. (1999). Comparative differences in the composition and biomechanical properties of tibiae of seven and seventy-two-week-old male and female broiler breeder chickens. Poult. Sci. 78, 1232–1239. doi:10.1093/ps/78.8.1232
Renema R. A., Robinson F. E., Zuidhof M. J. (2007). Reproductive efficiency and metabolism of female broiler breeders as affected by genotype, feed allocation, and age at photostimulation. 2. Sexual maturation. Poult. Sci. 86, 2267–2277. doi:10.1093/ps/86.10.2267
Richards J. D., Atwell C. A., Winkelbauer P., Wuelling C. W., Wehmeyer M. E., Dibner J. J. (2008a). “MINTREX organic trace minerals are more bioavailable and support the antioxidant response more than other trace mineral forms,” in Poultry science association annual meeting, niagara falls (Ontario, Canada.
Richards J. D., Dibner J. J., Winkelbauer P., Atwell C. A., Quiroz M. A., York T., et al. (2008b). “Organic trace minerals for poultry enhance mineral bioavailability and can improve performance and reduce environmental impact,” in 16th European symposium on poultry nutrition.
Richards J. D., Fisher P. M., Evans J. L., Wedekind K. J. (2015). Greater bioavailability of chelated compared with inorganic zinc in broiler chicks in the presence or absence of elevated calcium and phosphorus. Open Access Anim. Physiol. 7, 97–110. doi:10.2147/OAAP.S83845
Richards J. D., Zhao J., Harrell R. J., Atwell C. A., Dibner J. J. (2010). Trace mineral nutrition in poultry and swine. Asian-Australas. J. Anim. Sci. 23 (11), 1527–1534. doi:10.5713/ajas.2010.r.07
Roselli M., Finamore A., Garaguso I., Britti M. S., Mangheri E. (2003). Zinc oxide protects cultured enterocytes from the damage induced by Escherichia coli. J. Nutr. 133, 4077–4082. doi:10.1093/jn/133.12.4077
Rutz F., Murphy R. (2009). “Minerais orgânicos para aves e suínos,” in CBNA: Congresso Internacional sobre Uso da Levedura na Alimentação Animal, 21–36. Campinas. SP. Anais . Campinas.
Sakomura N. K., Rostagno H. S. (2016). “Métodos de pesquisa em nutrição de monogástricos. Editores Nilva Kazue Sakomura,” in Horácio santiago Rostagno. 2ed - jaboticabal:funep, 01–30.
Saleh A. A., Eltantawy M. S., Gawish E. M., Younis H. H., Amber K. A., Abd El-Moneim A. E., et al. (2020). Impact of dietary organic mineral supplementation on reproductive performance, egg quality characteristics, lipid oxidation, ovarian follicular development, and immune response in laying hens under high ambient temperature. Biol. Trace Elem. Res. 195, 506–514. doi:10.1007/s12011-019-01861-w
Seedor J. G., Quarruccio H. A., Thompson D. D. (1991). The bisphosphonate alendronate (MK-217) inhibits bone loss due to ovariectomy in rats. J. Bone Min. Res. 6, 339–346. doi:10.1002/jbmr.5650060405
Shannon M. C., Hill G. M. (2019). Trace mineral supplementation for the intestinal health of young monogastric animals. Front. Vet. Sci. 13, 73. doi:10.3389/fvets.2019.00073
Sharideh H., Zhandi M., Zaghari M., Akhlaghi A., Hussaini S. M. H., Yousefi A. R. (2019). Changes in broiler breeder hen's immunity by zinc oxide and phytase. Iran. J. Vet. Res. 20 (2), 120–125. PMID: 31531034; PMCID: PMC6716284.
Starcher B. C., Hill C. H., Madaras J. G. (1980). Effect of zinc deficiency on bone collagenase and collagen turnover. J. Nutr. 110, 2095–2102. doi:10.1093/jn/110.10.2095
Sun J., Coon C. N. (2005). The effects of body weight, dietary fat, and feed withdrawal rate on the performance of broiler breeders. J. Appl. Poult. Res. 14, 728–739. doi:10.1093/japr/14.4.728
Sun Q., Guo Y., Ma S., Yuan J., An S., Li J. (2012). Dietary mineral sources altered lipid and antioxidant profiles in broiler breeders and posthatch growth of their offsprings. Biol. Trace Elem. Res. 145 (3), 318–324. doi:10.1007/s12011-011-9196-5
Umar Yaqoob M., Wang G., Sun W., Pei X., Liu L., Tao W., et al. (2020). Effects of inorganic trace minerals replaced by complexed glycinates on reproductive performance, blood profiles, and antioxidant status in broiler breeders. Poult. Sci. 99 (5), 2718–2726. doi:10.1016/j.psj.2019.11.058
Underwood E. J., Suttle N. F. (1999). The mineral nutrition of livestock. 3rd Edition. New York: CABI Publishing.
Underwood E. J., Suttle N. F. (2001). The mineral nutrition of livestock. London. UK: CABI Publishing.
Van Emous R. A., Kwakkel R. P., van Krimpen M. M., Hendriks W. H. (2013). Effects of growth patterns and dietary crude protein levels during rearing on body composition and performance in broiler breeder females during the rearing and laying period. Poult. Sci. 92, 2091–2100. doi:10.3382/ps.2012-02987
Wang G., Liu L. J., Tao W. J., Xiao Z. P., Pei X., Liu B. J., et al. (2019a). Effects of replacing inorganic trace minerals with organic trace minerals on the production performance. blood profiles and antioxidant status of broiler breeders. Poult. Sci. 98, 2888–2895. doi:10.3382/ps/pez035
Wang G., Liu L., Wang Z., Pei X., Tao W., Xiao Z., et al. (2019b). Comparison of inorganic and organically bound trace minerals on tissue mineral deposition and fecal excretion in broiler breeders. Biol. Trace Elem. Res. 189, 224–232. doi:10.1007/s12011-018-1460-5
Whitehead C. C. (2004). Overview of bone biology in the egg-laying hen. Poult. Sci. 83 (2), 193–199. doi:10.1093/ps/83.2.193
Williams B., Waddington D., Murray D. H., Farquharson C. (2004). Bone strength during growth: Influence of growth rate on cortical porosity and mineralization. Calcif. Tissue Int. 74, 236–245. doi:10.1007/s00223-002-2124-0
Yan F., Waldroup P. W. (2006). Evaluation of Mintrex manganese as a source of manganese for young broilers. Int. J. Poult. Sci. 5, 708–713. doi:10.3923/ijps.2006.708.713
Zhao J., Shirley R. B., Dibner J. J., Wedekind K. J., Yan F., Fisher P., et al. (2016). Superior growth performance in broiler chicks fed chelated compared to inorganic zinc in presence of elevated dietary copper. J. Anim. Sci. Biotechnol. 7, 13. eCollection. doi:10.1186/s40104-016-0072-1
Zorzetto P. S., Araújo C. S. S., Araújo L. F., Roque F. A., Granghelli C. A., Leite B. G. C., et al. (2021). Replacing dietary sodium selenite with a lower level of hydroxy-selenomethionine improves the performance of broiler breeders and their progeny. Ital. J. Anim. Sci. 20, 1749–1758. doi:10.1080/1828051x.2021.1977727
Keywords: breeder reproduction, bone quality, progeny, intestinal inflammation, egg quality, gut health, chelated trace minerals, maternal supplementation
Citation: de Arruda Roque F, Chen J, Araujo RB, Murcio AL, de Souza Leite BG, Dias Tanaka MT, Granghelli CA, Pelissari PH, Bueno Carvalho RS, Torres D, Vázquez‐Añón M, Hancock D, Soares da Silva Araujo C and Araujo LF (2022) Maternal supplementation of different trace mineral sources on broiler breeder production and progeny growth and gut health. Front. Physiol. 13:948378. doi: 10.3389/fphys.2022.948378
Received: 19 May 2022; Accepted: 08 August 2022;
Published: 23 September 2022.
Edited by:
Federico Sirri, University of Bologna, ItalyCopyright © 2022 de Arruda Roque, Chen, Araujo, Murcio, de Souza Leite, Dias Tanaka, Granghelli, Pelissari, Bueno Carvalho, Torres, Vázquez‐Añón, Hancock, Soares da Silva Araujo and Araujo. This is an open-access article distributed under the terms of the Creative Commons Attribution License (CC BY). The use, distribution or reproduction in other forums is permitted, provided the original author(s) and the copyright owner(s) are credited and that the original publication in this journal is cited, in accordance with accepted academic practice. No use, distribution or reproduction is permitted which does not comply with these terms.
*Correspondence: Fabricia de Arruda Roque, fabricia.roque@usp.br; Juxing Chen, juxing.chen@novusint.com