- 1Zoology Department, Faculty of Science, Assiut University, Assiut, Egypt
- 2Zoology Department, Faculty of Science, New Valley University, New Valley, Egypt
It is known that silver nanoparticles (Ag NPs) and AgNO3 have harmful effects on the surrounding organisms, which may cause damage to these organisms. Therefore, the aim of this study is to detect damage caused by Ag NPs and silver nitrate to land slugs (Lehmannia nyctelia). In this study, the slugs were exposed to various concentrations of Ag NPs and AgNO3 for 15 days. The biochemical, antioxidant, lipid peroxidation (LPO), DNA fragmentation, and histopathological endpoints were assessed after 15 days of exposure to different concentrations of Ag NPs (0.04, 0.08, 0.4, and 0.8 g/L) and silver nitrate (0.04, 0.08, 0.4, and 0.8 g/L). The results show a significant decrease in total protein, total carbohydrate, superoxide dismutase, and GST and a significant increase in total lipid, LPO, and DNA fragmentation after exposure to Ag NPs and AgNO3 for 15 days compared with the control group. Histopathiological alterations were observed in the digestive glands which were indicated by histochemical staining. We concluded that exposure to AgNO3 and Ag NPs caused oxidative stress, genetic damage and alterations in the profile of muscle proteins and histological structure in L. nyctelia.
1 Introduction
Over the past decades, the impressive progress of nanotechnology has led to the widespread use of engineered nanoparticles in a wide variety of fields. However, their release into the environment draws attention to the effects and risks to organisms (Mekkawy et al., 2019; Naguib et al., 2020). Nanoparticles can accumulate in soil through sewage sludge application, accidental spills and airborne deposition, agrochemicals, or soil remediation (Cornelis et al., 2010). In addition, soil has been shown to be a sink for many (common) pollutants; therefore, long-term effects on soil organisms are likely (Sánchez-Aguayo et al., 1987; Rodríguez et al., 2007). These representations make soil organisms a target to be taken into account.
In recent years, the production of products containing silver nanoparticles (Ag NPs) has increased dramatically, and the release of Ag NPs into the environment is probably already occurring (Blaser et al., 2008; Luoma, 2008). Ag NPs are used as an antimicrobial component in many commercial products with a wide range of applications (Mendes et al., 2015; Vance et al., 2015). For example, they find their uses in paints, cosmetics, clothing, food packaging, electrical appliances, and biomedical products (McGillicuddy et al., 2017). This widespread use of Ag NPs has raised concerns about their environmental impact (Sakka et al., 2016).
Potential release of industrial products and wastes based on Ag NPs into the environment can lead to the accumulation of Ag NPs in soil, which have impact on soil invertebrates (Schlich et al., 2013; Bicho et al., 2016), soil microbial communities (Grün et al., 2018; Parada et al., 2019), and plants (Yan and Chen, 2019). Ag NPs after entering the environment undergo physical, chemical, and biological transformations that change their physicochemical properties, which in turn affect their toxicity (Levard et al., 2012; Levard et al., 2013; Sharma et al., 2015).
The toxicity mechanism of Ag NPs is the induction oxidative stress due to the formation of reactive oxygen species (ROS) which damage components of cells (Yang et al., 2012; Patricia et al., 2017). The effect of the presence of Ag NPs in the soil environment has not been adequately studied, but the impact of Ag NPs on earthworms has been shown (Diez et al., 2015; Garcia et al., 2016; Patricia et al., 2017). Because Ag ions dissociate from Ag NPs, it is important to understand the impact of both forms of silver, which can help us in risk assessment.
Gastropods are known to be efficient mineral scavengers and respond to pollution in a sensitive and measurable manner; therefore, they are widely used as indicators of environmental pollution in soil (Viard et al., 2004; Snyman et al., 2005). Land snails and slugs have a high capacity to accumulate heavy metals and are considered important species for monitoring the bioavailability of metal constituents in soil compared to other invertebrates (Lanno and McCarty, 1997). The digestive gland is mainly where heavy metals accumulate in slugs (Marigómez et al., 2002; Johnston et al., 2010) and plays a critical role in metal detoxification (Kammenga et al., 2000). In slugs, the digestive gland consists of three different types of cells: digestive cells (the most abundant), excretory cells, and calcium (basophilic) cells (Shiroky et al., 1993).
Biomarkers such as total protein (TP), total carbohydrate (TC), total lipid (TL), superoxide dismutase (SOD), and GST enzymes, as well as lipid peroxidation (LPO) and DNA fragmentation at the suborganism level, are considered indicators of stress response (Huggett, 2018).
Several biomarkers have been used as effective tools at the cellular level due to their sensitivity, rapid response, and precise relationship between toxicant exposure and associated biological responses (Connon et al., 2012). Oxidative stress is a process caused by a steady-state imbalance between oxidants and antioxidants that plays a role in the pathogenesis of many diseases, including those caused by environmental agents, including metal ions (Samet and Wages, 2018). This can damage proteins and DNA and cause LPO (Wible and Bratton, 2018). The major toxicity of Ag NPs in environmental organisms causes ROS generation and LPO (Terevinto et al., 2010; J Cataldo R et al., 2011).
This study aimed to investigate the toxicity of different concentrations of Ag NPs on slugs compared with the same concentrations of the soluble form of the metal (AgNO3) using multiple biomarkers such as antioxidants and biochemical biomarkers, genotoxic damage, and histopathological changes.
2 Materials and Methods
2.1 Chemicals
Silver nitrate (AgNO3; with 99.8% purity and a molecular weight of 169.873 g/mol) as colorless crystals was obtained from Sigma-Aldrich (St. Louis, MO, United States).
The Ag NPs as powder with spherical-shaped particles (with 99.9% purity, a molecular weight of 107.87 g/mol, and an average particle size of <100 nm) were purchased from Sigma-Aldrich (St. Louis, MO, United States).
2.2 Characterization of AgNO3 and the Ag NPs
2.2.1 TEM
NP characterization was performed using transmission electron microscopy (TEM) at TEMU, Assiut University (JEOL JEM-1200 EX II).
2.2.2 UV–Vis Spectroscopy
The surface plasmons of AgNO3 and the Ag NPs were determined by collecting an absorption profile in the wavelength range of 250–600 nm using UV–visible (UV–Vis) spectroscopy (SHIMADZU UV-3101PC UV–Vis–NIR).
2.2.3 X-ray Diffraction Patterns
The structures of AgNO3 and the Ag NPs were examined by X-ray diffraction (XRD). The spectra were recorded at scanning mode on PW 2103 from Philips (Netherlands). The X-ray diffractometer used Cu Kα radiation (λ = 1.5406 Å) in the range of 2θ = 4°–80°.
2.3 Stock Preparation
The stock solution (AgNO3 and Ag NPs) was prepared according to production protocol and stored at 4°C in the dark. The stock solution (1 g/L) was prepared using purified water (Milli-Q) and sonicated before each use. Dilutions of the experimental concentrations were prepared from this stock solution before starting each experiment.
2.4 Sample Collection
Specimens of Lehmannia nyctelia (about 180 samples) (with a weight of 1 ± 0.4 g and a length of 1.5 ± 0.5 cm) were harvested during autumn at the Assiut University Farm, Assiut Governorate, Egypt, and transported to the Ecology Laboratory at Assiut University. The slugs were kept under normal laboratory conditions, kept in plastic containers with soil from their natural habitat, and fed fresh lettuce daily for 15 days until acclimatization.
2.5 Experimental Design
The slugs were randomly divided into nine groups (one control group and eight groups treated). Each group consisted of 20 samples and kept in plastic containers containing a mass of soil (30 g). Each container was covered with a perforated cloth for ventilation.
The control group was fed fresh lettuce impressed in 10 ml of distilled water. On the other hand, the treated groups were fed fresh lettuce impressed in various concentrations of Ag NPs and AgNO3 mixed with distilled water.
The first group was the control group, and the second, third, fourth, and fifth groups were exposed to 0.04-, 0.08-, 0.4-, and 0.8-g/L Ag NPs, respectively, for 15 days. The sixth, seventh, eighth, and ninth groups were exposed to 0.04-, 0.08-, 0.4-, and 0.8-g/L AgNO3, respectively, for 15 days. At the end of the experiments, the surviving slugs were used for biochemical analysis, antioxidant biomarkers, and histological studies.
2.6 Estimation of the Silver Ions
The estimation of the Ag by element and atomic mass in the sample was done by EDX (JSM-5400LV scanning microscope) at EMU at Assiut University with a link ISIS operator’s manual.
2.7 Measurement of the Biochemical Parameters
The whole body of L. nyctelia was homogenized in 1 ml of TCA (20%), and the TP concentration was determined based on the shift observed in the absorption maximum of an acidic solution of Coomassie brilliant blue G-250 from 465 to 595 nm when protein binding occurs in accordance with the method by (Bradford, 1976). The determination of TC was performed on an aliquot (100 μL) in accordance with the method by (Moreau et al., 1984). This method uses anthrone as the reagent and glucose as the standard. The absorbance was evaluated on a spectrophotometer at a wavelength of 620 nm. The TL of the whole body of L. nyctelia was measured according to the method of (Goldsworthy et al., 1972).
2.8 Antioxidant Biomarkers
SOD was measured based on its ability to inhibit the phenazine-methosulfate-mediated reduction of nitro blue tetrazolium dye to form a red product (Nishikimi et al., 1972).
GST was determined by the spectrophotometric assay of (Ålin et al., 1985). This method measures the total GST at an absorbance of 340 nm.
2.9 LPO and DNA Fragmentation
LPO was measured according to the method by (Ohkawa et al., 1979) at an absorbance of 535 nm. The LPO rate was expressed in nanomoles of thiobarbituric acid reactive substance formed per hour per milligram of protein using a molar extinction coefficient of 1.56 M/cm. DNA fragmentation was measured according to the method by (Kurita-Ochiai et al., 1999) using a spectrophotometer at 575 or 600 nm against a reagent blank. The percentage of fragmented DNA was estimated using the following formula: % of fragmented DNA = fragmented DNA/(fragmented + intact DNA) × 100.
2.10 Histochemical Change
At the end of the experiments, the whole bodies of the slugs were immediately fixed in 70% alcohol. The fixed slugs were processed through a paraffin embedding technique. The embedded tissues were sectioned at 5–7 µm in thickness and then stained with Sudan Black, periodic acid–Schiff (PAS) and hematoxylin stain, and toluidine blue. The sectioned digestive gland was examined using an Olympus microscope (Olympus Optical Co., Ltd., Japan).
2.11 Statistical Analysis
The mean and standard error were analyzed using the SPSS package (SPSS 1998) at the 0.05 significance level. Data were tested for normality (Shapiro–Wilk test) and then tested for homogeneity of variances using one-way analysis of variance.
2.12 Ethical Statement
All experiments were carried out in accordance with Egyptian laws and university guidelines for the care of experimental animals.
3 Results
3.1 Characterization of AgNO3 and Ag NPs
3.1.1 TEM
The TEM images showed that the Ag NPs were spherical in shape with small size (Figure 1A).
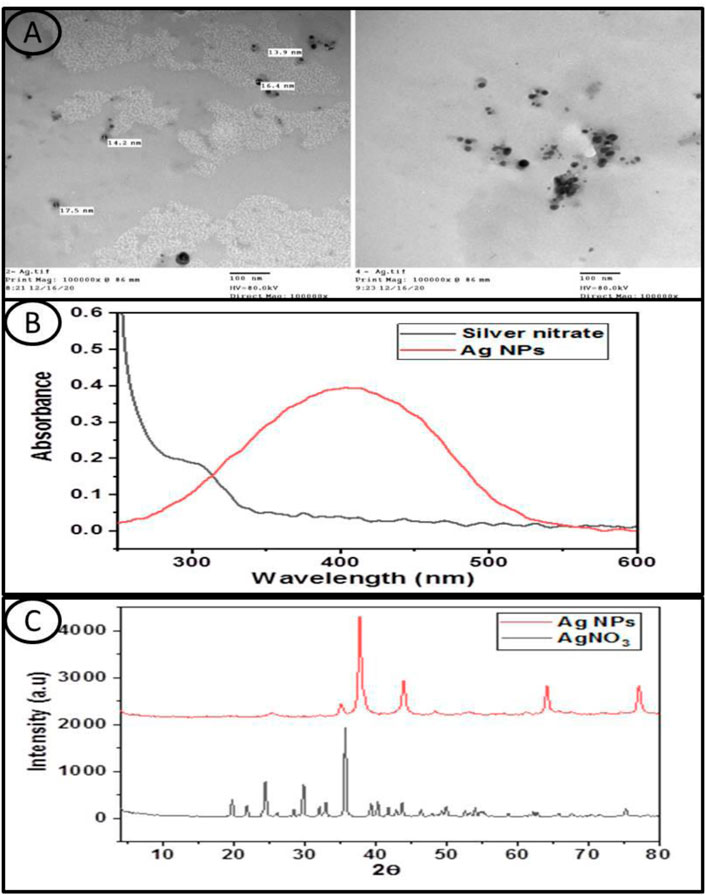
FIGURE 1. (A) Transmission electron microscope images of the investigated Silver Nanoparticles, (B) shows the UV-Vis absorption spectra of the silver nitrate and Ag NPs aqueous solution and (C) displayed the XRD patterns of silver nitrate and the Ag NPs used in this study.
3.1.2 UV–Vis Spectroscopy
The spectrum of silver nitrate has no absorption characteristic peak, whereas the spectrum of the Ag NPs showed an absorption peak centered at about 405 nm (Figure 1B).
3.1.3 XRD Patterns
The XRD pattern of silver nitrate exhibited several diffraction peaks, where the main higher peaks appeared at 2θ values of 35.7°, 29.8°, 24.5°, 21.8°, and 19.7°. On the other hand, the XRD pattern of the Ag NPs showed four peaks observed at 2θ values of 38°, 44°, 64.2°, and 77.1°, which can be attributed to the crystal planes (1 1 1), (2 0 0), (2 2 0), and (3 1 1), respectively, which have face-centered cubic silver crystal structures, and these values were similar with those of the standard card (JCPDS No: 04-0783) (Figure 1C).
3.2 Percentage of Silver
The percentage of Ag by element and atomic mass after exposure to different concentrations of Ag NPs (0.04, 0.08, 0.4, and 0.8 g/L) and silver nitrate (0.04, 0.08, 0.4, and 0.8 g/L) for 15 days showed a significant (p < 0.05) increase when compared to the control group (Figure 2).
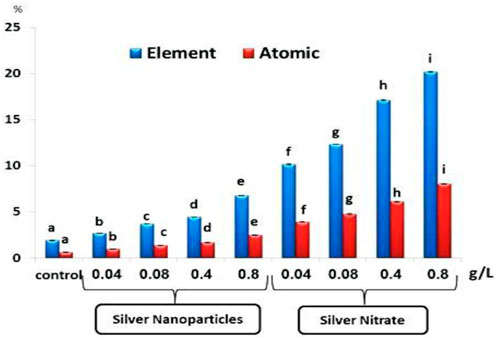
FIGURE 2. The percentage of silver as element and atomic in the total body of the slugs, (Lehmannia nyctelia), after 15 days of exposure. Bars represent the mean ± SE of the control and experimental groups. Different letters indicate significant differences amongst treatments (p < 0.05).
3.3 Biochemical Parameters
The TP and TC after exposure to 0.04, 0.08, 0.4, and 0.8 g/L of Ag NPs and 0.04, 0.08, 0.4, and 0.8 g/L of silver nitrate for 15 days showed a significant (p < 0.05) decrease when compared to the control group (Figure 3). On the other hand, the TL showed a significant (p < 0.05) increase after exposure to 0.04, 0.08, 0.4, and 0.8 g/L of Ag NPs and 0.04, 0.08, 0.4, and 0.8 g/L of silver nitrate for 15 days when compared to the control group (Figure 3).
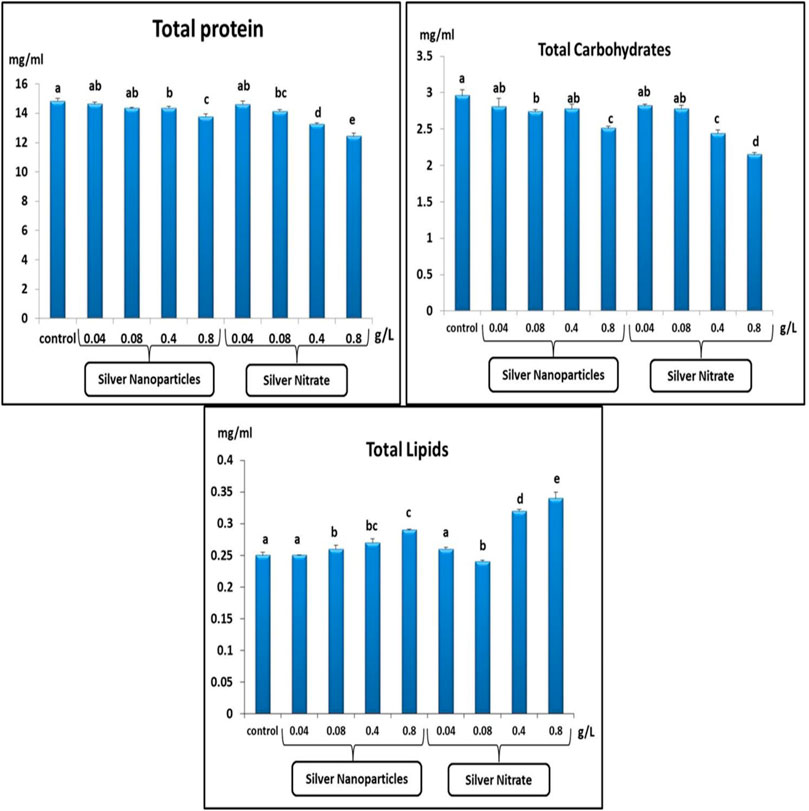
FIGURE 3. Total protein (mg/ml), total carbohydrates (mg/ml) and total lipids (mg/ml) in slugs (Lehmannia nyctelia) after silver nanoparticles and silver nitrite exposure for 15 days. Bars represent the mean ± SE of the control and experimental groups. Different letters indicate significant differences amongst treatments (p < 0.05).
3.4 Antioxidant Enzymes
The SOD and GST activities in the slugs showed a significant decrease (p < 0.05) after exposure to 0.04, 0.08, 0.4, and 0.8 g/L of Ag NPs and 0.04, 0.08, 0.4, and 0.8 g/L of silver nitrate for 15 days in comparison with the control group (Table 1).

TABLE 1. Effects of silver nanoparticles and silver nitrite on the antioxidants parameters of the slugs, (Lehmannia nyctelia), after 15 days of exposure. Data are presented as mean ± SE. Values in the same row with different superscript letter are significantly different (p < 0.05). Superoxide dismutase (SOD), Glutathione -S-transfer (GST), Lipid peroxidation (LOP) and DNA fragmentation.
3.5 DNA Fragmentation and LPO
LPO and DNA fragmentation after exposure to 0.04, 0.08, 0.4, and 0.8 g/L of Ag NPs and 0.04, 0.08, 0.4, and 0.8 g/L of silver nitrate for 15 days showed a significant increase (p < 0.05) in comparison with the control group (Table 1).
3.6 Histochemical Change
3.6.1 PAS and Hematoxylin Reaction for Carbohydrates in the Digestive Gland of the Slugs (L. nyctelia)
3.6.1.1 Control Group
PAS staining for carbohydrates in the digestive gland showed a positive reaction of polysaccharides and a high amount of carbohydrates in the control group, as shown in Figures 4A,B.
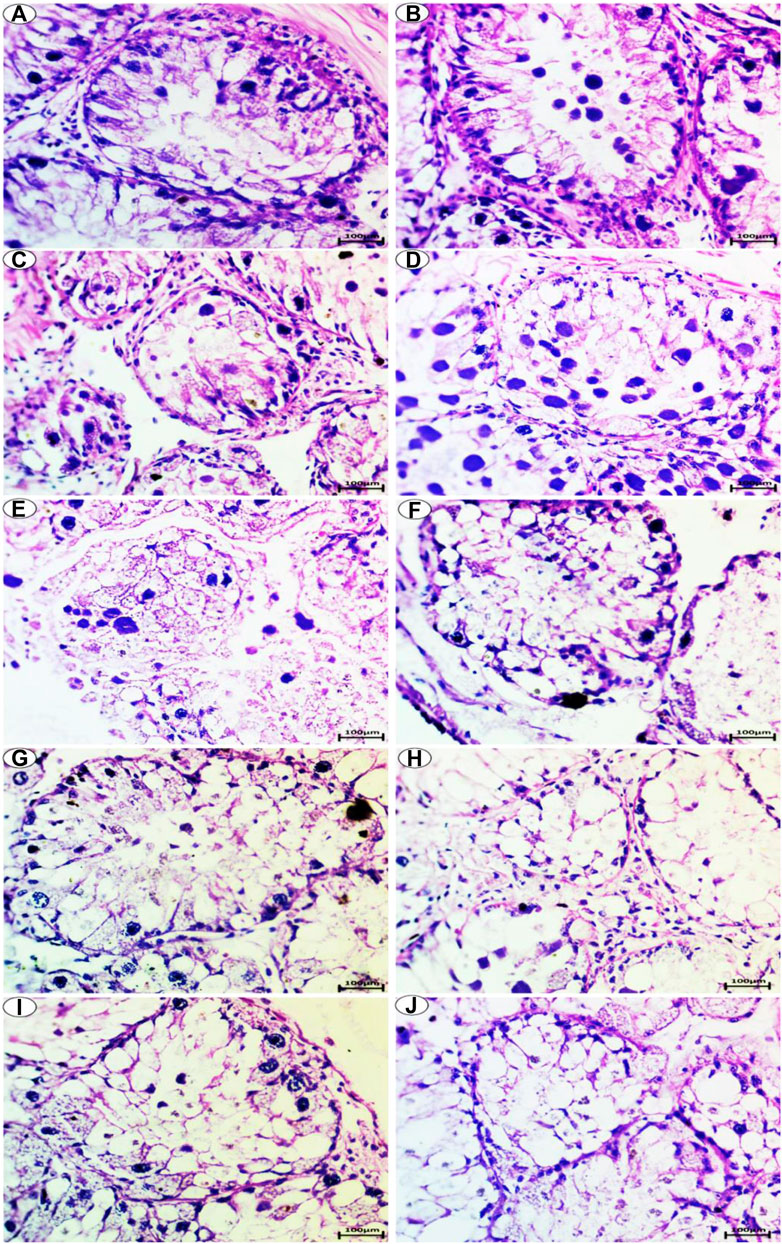
FIGURE 4. Photomicrograph of digestive gland of Slug Lehmannia nyctelia after the exposure to (A,B) Control group, (C); 0.04 g/L of Ag NPs, (D); 0.08 g/L of Ag NPs, (E); 0.4 g/L of Ag NPs and (F); 0.8 g/L of Ag NPs, (G); 0.04 g/L of silver nitrate, (H); 0.08 g/L of silver nitrate, (I); 0.4 g/L of silver nitrate, (J); 0.8 g/L of silver nitrate. (PAS and hematoxylin, scale bar 100 μm).
3.6.1.2 Treatment Exposure to Ag NPs
In the group treated with 0.04-g/L Ag NPs, a slight decrease in the amount of carbohydrates was observed, as shown in Figure 4C. In the group treated with 0.08-g/L Ag NPs, a moderate amount of carbohydrates was observed, as shown in Figure 4D.
The group exposed to 0.4-g/L Ag NPs showed depletion in carbohydrates, as shown in Figure 4E, whereas the group exposed to 0.8-g/L Ag NPs showed more depletion in the amount of carbohydrates, as shown in Figure 4F.
3.6.1.3 Treatment Exposure to Silver Nitrate
The group treated with 0.04-g/L silver nitrate showed a moderate amount of carbohydrates, as shown in Figure 4G, whereas the group exposed to 0.08-g/L silver nitrate showed depletion in the amount of carbohydrates, as shown in Figure 4H.
The group exposed to 0.4-g/L silver nitrate showed more depletion in the amount of carbohydrates, as shown in Figure 4I, whereas the group exposed to 0.8-g/L silver nitrate showed greater depletion in the amount of carbohydrates, as shown in Figure 4J.
3.6.2 Toluidine Blue for Proteins or Mucopolysaccharide Contents in the Digestive Gland of the Slugs (L. nyctelia)
Toluidine blue staining (pH of 2.5) was performed for acidic structures in the nucleus and cytoplasm and for colored structures with the same color of the dye termed as orthochromatic structures.
3.6.2.1 Control Group
The control group showed a normal intensity of blue color for localization of mucopolysaccharide contents (orthochromatic structures) in the cells of both the nuclei and cytoplasm and the digestive gland basement membrane and boundary of lining cells, as shown in Figures 5A,B.
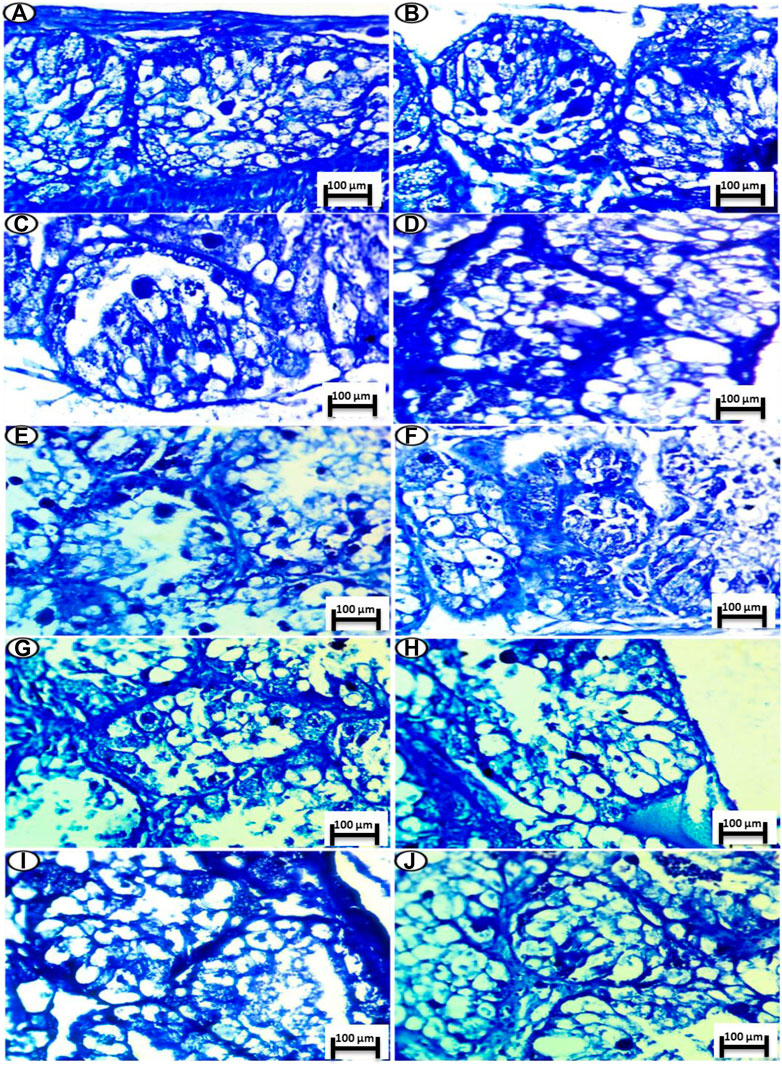
FIGURE 5. Photomicrograph of digestive gland of Slug Lehmannia nyctelia after the exposure to (A,B) Control group, (C); 0.04 g/L of Ag NPs, (D); 0.08 g/L of Ag NPs, (E); 0.4 g/L of Ag NPs and (F); 0.8 g/L of Ag NPs, (G); 0.04 g/L of silver nitrate, (H); 0.08 g/L of silver nitrate, (I); 0.4 g/L of silver nitrate, (J); 0.8 g/L of silver nitrate. (Toluidine blue, scale bar 100 μm.
3.6.2.2 Treatment Exposure to Ag NPs
The group exposed to 0.04 g/L of Ag NPs showed a slight decline in the intensity of staining in comparison with the control group (Figure 5C), while this intensity was decreased again in the group exposed to 0.08 g/L of Ag NPs and with more deposition at its basement membrane (Figure 5D).
The groups exposed to 0.4 and 0.8 g/L of Ag NPs showed severe depletion of mucopolysaccharide contents, and this shortage was noticed in the last groups. In addition, the main deepest coloration was noticed in the nuclei and faint reactions were noticed in the cytoplasm (Figures 5E,F).
3.6.2.3 Treatment Exposure to Silver Nitrate
In the silver-nitrate-treated groups, there is amelioration in the color deposition of the mucopolysaccharide contents especially in the basement membrane and the fine granules inside the lining cells, and this decrement was noticed for concentrations of 0.04 and 0.08 g/L of silver nitrate (Figures 5G,H), whereas a slight decline was noticed for concentrations of 0.4 and 0.8 g/L of silver nitrate (Figures 5I, J).
3.6.3 Sudan Black for Conjugated Lipids in the Digestive Gland of the Slugs (L. nyctelia)
The Sudan Black reaction showed a moderate accumulation of lipids in the digestive gland of the slugs (L. nyctelia) in the control group, whereas a large amount of lipids (Figure 6) was accumulated and observed in the digestive gland for the Ag NP- and silver-nitrate-treated groups.
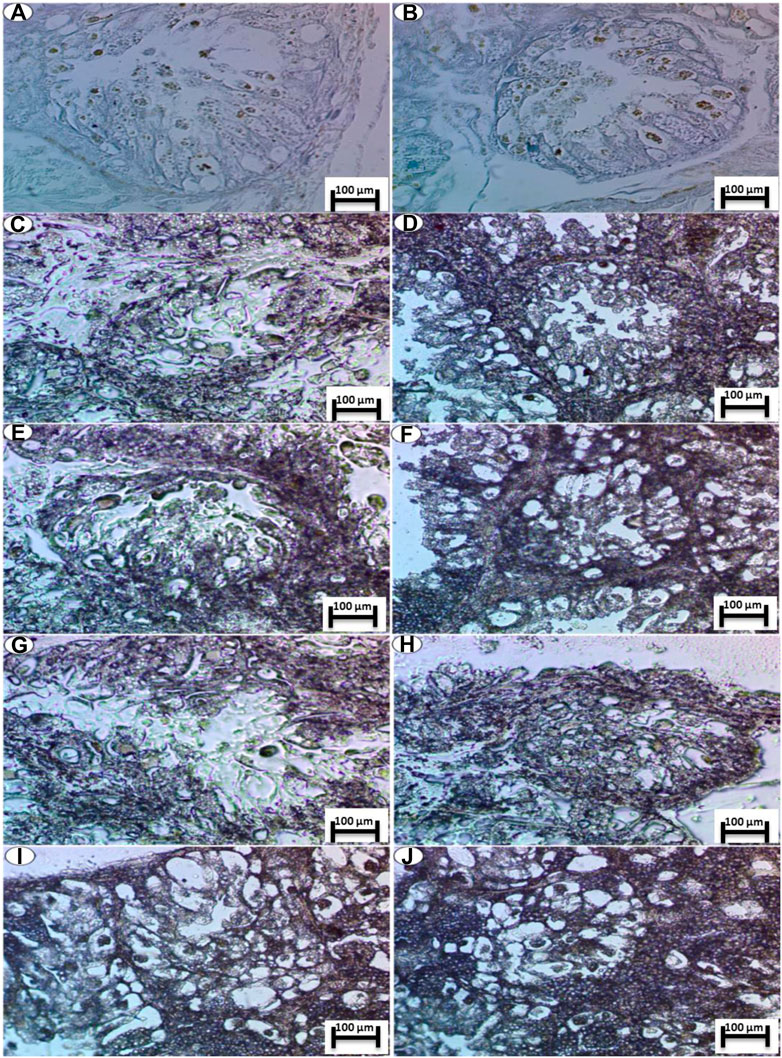
FIGURE 6. Photomicrograph of digestive gland of Slug Lehmannia nyctelia after the exposure to (A,B) Control group, (C); 0.04 g/L of Ag NPs, (D); 0.08 g/L of Ag NPs, (E); 0.4 g/L of Ag NPs and (F); 0.8 g/L of Ag NPs, (G); 0.04 g/L of silver nitrate, (H); 0.08 g/L of silver nitrate, (I); 0.4 g/L of silver nitrate, (J); 0.8 g/L of silver nitrate. (Sudan black, scale bar 100 μm).
4 Discussion
Little is known about the negative effects of AgNO3 and Ag NPs on L. nyctelia in soil, while many industries are currently using Ag NPs, and there is a possibility of increased environmental and human exposure with harmful toxicological consequences (Martirosyan et al., 2014). The characterization of AgNO3 and Ag NPs explains many properties of the materials used in the experiments, and the result of UV–Vis spectroscopy and the XRD patterns indicate that the Ag NPs have a spherical shape with a small size and a regular distribution, which is a characteristic of the surface plasmon resonance of silver in accordance with that by (Vu et al., 2018).
The slugs died during the experiments at a high rate with the AgNO3 treatments but at a low rate with the Ag NP treatments, indicating that AgNO3 does exhibit very acutely toxicity to L. nyctelia within 15 days, whereas the Ag NPs does exhibit acutely toxicity to L. nyctelia within 15 days. Many studies have shown that much of the toxicity of heavy metals is closely related to the production of ROS in biological systems (Valko et al., 2005). Oxidative stress determines the ability of ROS to damage cellular components such as cell membranes, proteins, DNA, and RNA (Radwan et al., 2010). In mollusks, heavy metals can cause a state of general stress, resulting in a decrease in their ability to adapt to hypoxia.
Proteins are involved in the structure of the cell. In chronic stress, proteins become a source of energy (Radwan et al., 2008). The results showed a significant reduction in the TP content. Similarly (Laila and Genena, 2011), found that the level of TP in Monacha cantiana and Eobania vermiculata decreased after exposure to molluscicides, while (Radwan and Mohamed, 2013) observed a significant increase in the protein rate in snails treated with 0.6 LD of imidacloprid. The accumulation of heavy metals in mollusks is often associated with a decrease in proteins belonging to metallothioneins, which play an important role in metal homeostasis.
Carbohydrates are the main and immediate source of energy. When oxidative stress occurs, carbohydrate stores are depleted to meet the increased need for energy (Arasta et al., 1996). Our results show a significant decrease in carbohydrate rates. The study by (Radwan et al., 2008) indicated a significant decrease in carbohydrate rate in E. vermiculata after exposure to pesticides. Unlike in the work by (Barky et al., 2012), atrazine caused a toxic effect by increasing the glucose concentration in snails (Biomphalaria alexandrina).
Under adverse conditions, slugs needed the energy to eliminate toxicants and stress. Slugs have a limited amount of carbohydrates, and proteins are the next alternative source of energy to meet the increased energy requirements (Radwan et al., 2008). (Padmaja and Balapara 1994) suggested that the reduction in protein content in the tissues of freshwater snails (Bellamya dissimilis) after exposure to pesticides may be due to some mechanisms: 1) the formation of lipoproteins, which are used to repair damaged cells and tissues, and 2) direct use by cells to meet energy needs (Radwan et al., 2008). Lipids are also a source of energy and important for the formation of cells and tissues (Araghi and Bastami, 2011). In our results, the TLs were significantly increased, which is consistent with the results by (El-Shenawy et al., 2012), which showed a significant increase in TLs in E. vermiculata collected from contaminated areas. Also, these results are consistent with the results by (Laila and Genena, 2011); they found that the treatment with all tested molluscicides for 3 days increased the TL levels in E. vermiculata.
SOD plays an important role in the protection against free radicals and oxidative stress (Menvielle-Bourg, 2005; Roubalová et al., 2015). SOD inhibition under the influence of NPs is one of the most important biomarkers of oxidative stress. In our study, a decrease in SOD activity was observed, as in the results by (Mwaanga et al., 2014), which recorded a decrease in SOD after exposure to Cu, CuO, and ZnO NPs on earthworms in soil. As noted by researchers elsewhere (Gill and Tuteja, 2010), GST plays an important role as an antioxidant, maintaining the integrity of proteins and enzymes. It is known that the restoration of GST in antioxidant processes is carried out due to several other processes (Lushchak, 2012). Thus, in this study, GST depletion could be caused by several processes (Gomes et al., 2015). showed that exposure of Eisenia fetida to Ag+ mixed with OECD soil at 25–200 mg/kg for 28 days resulted in a decrease in CAT and GST activities. Inhibition of GST activity can occur both due to the direct action of the metal on the enzyme and indirectly through the production of ROS that directly interacts with the enzyme and the depletion of its GSH (Roling and Baldwin, 2006). This explanation may be the reason for the decrease in GST activity that was induced in the present study in the case of slugs exposed to Ag NPs and silver nitrate.
Two mechanisms of Ag NP action have been proposed: 1) the release of Ag+ ions during long-term incubation in soil and the induction of ROS (McShan et al., 2014) and 2) the direct induction of ROS (Wijnhoven et al., 2009). Both mechanisms can have negative effects on oxidative stress and genotoxicity associated with exposure to Ag NPs, which can damage lipids, proteins, and nucleic acids and stimulate SOD, CAT, and GST production (McShan et al., 2014; Durán et al., 2016; Wang et al., 2017). Ag NPs can also cause toxicity through surface oxidation, which results in the release of Ag+, which can interact with lipid molecules, nucleic acids, and proteins in biological systems (McShan et al., 2014).
LPO has been studied in programs of environmental monitoring. Increased LPO has been reported in different snails exposed to laboratory or environmental pollutants (Al-Daihan et al., 2010; Barky et al., 2012; El-Shenawy et al., 2012). Mendes et al. (2015) showed in a similar study when Folsomia candida was exposed to Ag+ and Ag NPs and found that the increase in LPO was more pronounced in those exposed to Ag+ than those exposed to Ag NPs. This result is consistent with previous results that showed that copper exposure induces oxidative stress in snail tissues, as evidenced by an increase in LPO (Viarengo et al., 1990).
Genotoxicity is one of the most important chemical toxicity tests and risk assessments; however, little is known about the genotoxicity of AgNO3 and Ag NPs (Sayed, 2016; Sayed and Soliman, 2017; Sayed and Younes, 2017; Mahmoud et al., 2019; Mekkawy et al., 2019; Naguib et al., 2020), especially for terrestrial organisms L. nyctelia. It is known that ROS reacts with the DNA molecule, causing damage to purine and pyrimidine bases, as well as to the DNA backbone (Ali et al., 2015).
The comparison between silver nitrate and Ag NPs with the same concentrations showed that the antioxidant enzyme activity in the slugs exposed to silver nitrate was higher than that in the slugs exposed to the Ag NPs. There are many reasons why silver nitrate may be more toxic than Ag NPs: 1) The solubility of silver nitrate is much higher than the solubility of Ag NPs; 2) in the molar ratio, the concentration of silver nitrate ions is higher than the concentration of Ag NPs of equal mass; and 3) the elimination rate of Ag NPs is higher than that of silver nitrate (Ribeiro et al., 2015).
Histochemical examination of the digestive gland of L. nyctelia treated with AgNO3 and Ag NPs highlighted important qualitative changes because of the different concentrations tested. Indeed, nanoparticle exposure can cause very significant cytological alterations in the digestive gland, which plays a crucial role in the detoxification of pollutants (Frías-Espericueta et al., 2008). The histochemical changes are expected to be useful biomarkers of AgNO3 and Ag NP exposure (Snyman et al., 2009). The histopathological responses of the several organs (digestive gland) of L. nyctelia exposed to increasing concentrations of AgNO3 and Ag NPs in food occurring through reactions involving epithelial hyperplasia at the lowest doses tested the cell destruction and are accompanied by a proliferation of connective tissues and necrosis at high concentrations.
The results of this study can demonstrate the environmental consequences of the release of AgNO3 and Ag NPs into ecosystems. These biomarkers have also opened up broad prospects in essential toxicology as L. nyctelia is exposed to environmental pollutants. Our results provide regulators and industries with important information to determine whether AgNO3 and Ag NPs need to be monitored and regulated.
5 Conclusion
In conclusion, exposure to AgNO3 and Ag NPs caused changes in the antioxidant biomarkers (SOD and GST), DNA damage, LPO, and changes in the profile of muscle proteins with changes in the histopathological reactions of L. nyctelia, which can be considered as the cause of death of L. nyctelia.
Data Availability Statement
The original contributions presented in the study are included in the article/supplementary materials, further inquiries can be directed to the corresponding author.
Author Contributions
ZB, SS, WM, GA, NE; Conceptualization, ZB: Methodology, ZB, SS: Visualization, Investigation, ZB, SS, WM, GA, NE; Data curation, Writing- Original draft preparation. NE and GA contributed all stages of this work. All authors: Final Draft Writing-Reviewing and Editing.
Conflict of Interest
The authors declare that the research was conducted in the absence of any commercial or financial relationships that could be construed as a potential conflict of interest.
Publisher’s Note
All claims expressed in this article are solely those of the authors and do not necessarily represent those of their affiliated organizations, or those of the publisher, the editors and the reviewers. Any product that may be evaluated in this article, or claim that may be made by its manufacturer, is not guaranteed or endorsed by the publisher.
Acknowledgments
This study was extracted from a master thesis of ZB.
References
Al-Daihan S., Kaggwa J. S., El-Ansary A. K. (2010). The Effect of a Sublethal Concentration of Solanum nigrum on Some Antioxidants in Biomphalaria Arabica. J. Egypt Soc. Parasitol. 40, 205–214.
Ali D., Ahmed M., Alarifi S., Ali H. (2015). Ecotoxicity of Single-Wall Carbon Nanotubes to Freshwater snailLymnaea luteolaL.: Impacts on Oxidative Stress and Genotoxicity. Environ. Toxicol. 30, 674–682. doi:10.1002/tox.21945
Ålin P., Danielson U. H., Mannervik B. (1985). 4-Hydroxyalk-2-enals Are Substrates for Glutathione Transferase. FEBS Lett. 179, 267–270. doi:10.1016/0014-5793(85)80532-9
Araghi P., Bastami K. D. (2011). An Integrated Approach on Interconnected Effects between Selected Environmental Parameters and Fatty Acid Composition in Mollusks. Res. J. Environ. Sci. 5, 310. doi:10.3923/rjes.2011.134.141
Arasta T., Bais V., Thakur P. (1996). Effect of Nuvan on Some Biochemical Parameters of Indian Cat Fish, Mystus Vittatus. J. Environ. Biol. 17, 167–169.
Barky F. A., Abdelsalam H. A., Mahmoud M. B., Hamdi S. A. H. (2012). Influence of Atrazine and Roundup Pesticides on Biochemical and Molecular Aspects of Biomphalaria Alexandrina Snails. Pesticide Biochem. physiology 104, 9–18. doi:10.1016/j.pestbp.2012.05.012
Bicho R. C., Ribeiro T., Rodrigues N. P., Scott-Fordsmand J. J., Amorim M. J. B. (2016). Effects of Ag Nanomaterials (NM300K) and Ag Salt (AgNO3) Can Be Discriminated in a Full Life Cycle Long Term Test with Enchytraeus Crypticus. J. Hazard. Mater. 318, 608–614. doi:10.1016/j.jhazmat.2016.07.040
Blaser S. A., Scheringer M., MacLeod M., Hungerbühler K. (2008). Estimation of Cumulative Aquatic Exposure and Risk Due to Silver: Contribution of Nano-Functionalized Plastics and Textiles. Sci. total Environ. 390, 396–409. doi:10.1016/j.scitotenv.2007.10.010
Bradford M. M. (1976). A Rapid and Sensitive Method for the Quantitation of Microgram Quantities of Protein Utilizing the Principle of Protein-Dye Binding. Anal. Biochem. 72, 248–254. doi:10.1016/0003-2697(76)90527-3
Connon R. E., Geist J., Werner I. (2012). Effect-based Tools for Monitoring and Predicting the Ecotoxicological Effects of Chemicals in the Aquatic Environment. Sensors 12, 12741–12771. doi:10.3390/s120912741
Cornelis G., Kirby J. K., Beak D., Chittleborough D., McLaughlin M. J. (2010). A Method for Determination of Retention of Silver and Cerium Oxide Manufactured Nanoparticles in Soils. Environ. Chem. 7, 298–308. doi:10.1071/en10013
Diez-Ortiz M., LahiveLahive E., George S., Ter Schure A., Van Gestel C. A. M., Jurkschat K., et al. (2015). Short-term Soil Bioassays May Not Reveal the Full Toxicity Potential for Nanomaterials; Bioavailability and Toxicity of Silver Ions (AgNO3) and Silver Nanoparticles to Earthworm Eisenia fetida in Long-Term Aged Soils. Environ. Pollut. 203, 191–198. doi:10.1016/j.envpol.2015.03.033
Durán N., Durán M., De Jesus M. B., Seabra A. B., Fávaro W. J., Nakazato G. (2016). Silver Nanoparticles: A New View on Mechanistic Aspects on Antimicrobial Activity. Nanomedicine Nanotechnol. Biol. Med. 12, 789–799. doi:10.1016/j.nano.2015.11.016
El-Shenawy N. S., Mohammadden A., Al-Fahmie Z. H. (2012). Using the Enzymatic and Non-enzymatic Antioxidant Defense System of the Land Snail Eobania Vermiculata as Biomarkers of Terrestrial Heavy Metal Pollution. Ecotoxicol. Environ. Saf. 84, 347–354. doi:10.1016/j.ecoenv.2012.08.014
Friasespericueta M., Abad-Rosales S., Nevárez-Velázquez A. C., Osuna-López I., Páez-Osuna F., Lozano-Olvera R., et al. (2008). Histological Effects of a Combination of Heavy Metals on Pacific White Shrimp Litopenaeus Vannamei Juveniles. Aquat. Toxicol. 89, 152–157. doi:10.1016/j.aquatox.2008.06.010
Garcia-Velasco N., GandariasbeitiaGandariasbeitia M., Irizar A., Soto M. (2016). Uptake Route and Resulting Toxicity of Silver Nanoparticles in Eisenia fetida Earthworm Exposed through Standard OECD Tests. Ecotoxicology 25, 1543–1555. doi:10.1007/s10646-016-1710-2
Gill S. S., Tuteja N. (2010). Reactive Oxygen Species and Antioxidant Machinery in Abiotic Stress Tolerance in Crop Plants. Plant physiology Biochem. 48, 909–930. doi:10.1016/j.plaphy.2010.08.016
Goldsworthy G. J., Mordue W., Guthkelch J. (1972). Studies on Insect Adipokinetic Hormones. General Comp. Endocrinol. 18, 545–551. doi:10.1016/0016-6480(72)90034-2
Gomes S. I. L., Hansen D., Scott-Fordsmand J. J., Amorim M. J. B. (2015). Effects of Silver Nanoparticles to Soil Invertebrates: Oxidative Stress Biomarkers in Eisenia fetida. Environ. Pollut. 199, 49–55. doi:10.1016/j.envpol.2015.01.012
Grün A.-L., Straskraba S., Schulz S., Schloter M., Emmerling C. (2018). Long-term Effects of Environmentally Relevant Concentrations of Silver Nanoparticles on Microbial Biomass, Enzyme Activity, and Functional Genes Involved in the Nitrogen Cycle of Loamy Soil. J. Environ. Sci. 69, 12–22. doi:10.1016/j.jes.2018.04.013
Huggett R. J. (2018). Biomarkers: Biochemical, Physiological, and Histological Markers of Anthropogenic Stress. Boca Raton, Florida: CRC Press.
J Cataldo R., M E Hidalgo L., Neaman A., Gaete O H. (2011). Use of Molecular Biomarkers in Eisenia Foetida to Assess Copper Toxicity in Agricultural Soils Affected by Mining Activities. J. soil Sci. plant Nutr. 11, 57–70. doi:10.4067/S0718-95162011000300005
Johnston H. J., Hutchison G. R., Christensen F. M., Aschberger K., Stone V. (2010). The Biological Mechanisms and Physicochemical Characteristics Responsible for Driving Fullerene Toxicity. Toxicol. Sci. 114, 162–182. doi:10.1093/toxsci/kfp265
Kammenga J. E., Dallinger R., Donker M. H., Köhler H. R., Simonsen V., Triebskorn R., et al. (2000). Biomarkers in Terrestrial Invertebrates for Ecotoxicological Soil Risk Assessment. Rev. Environ. Contam. Toxicol. 164, 93–147.
Kurita-Ochiai T., Fukushima K., Ochiai K. (1999). Lipopolysaccharide Stimulates Butyric Acid-Induced Apoptosis in Human Peripheral Blood Mononuclear Cells. Infect. Immun. 67, 22–29. doi:10.1128/iai.67.1.22-29.1999
Laila R., Genena M. (2011). Biochemical Effect of Three Molluscicide Baits against the Two Land Snails, Monacha Cantiana and Eobania Vermiculata (Gastropoda: Helicidae). Int. J. Agric. Res. 6, 682–690. doi:10.3923/ijar.2011.682.690
Lanno R. P., McCarty L. S. (1997). Earthworm Bioassays: Adopting Techniques from Aquatic Toxicity Testing. Soil Biol. Biochem. 29, 693–697. doi:10.1016/s0038-0717(96)00197-6
Levard C., Hotze E. M., Colman B. P., Dale A. L., Truong L., Yang X. Y., et al. (2013). Sulfidation of Silver Nanoparticles: Natural Antidote to Their Toxicity. Environ. Sci. Technol. 47, 13440–13448. doi:10.1021/es403527n
Levard C., Hotze E. M., Lowry G. V., Brown G. E. (2012). Environmental Transformations of Silver Nanoparticles: Impact on Stability and Toxicity. Environ. Sci. Technol. 46, 6900–6914. doi:10.1021/es2037405
Luoma S. N. (2008). Silver Nanotechnologies and the Environment. Proj. Emerg. nanotechnologies Rep. 15, 12–13.
Lushchak V. I. (2012). Glutathione Homeostasis and Functions: Potential Targets for Medical Interventions. J. amino acids 2012, 736837. doi:10.1155/2012/736837
Mahmoud U. M., Mekkawy I. A. A., Naguib M., Sayed A. E.-D. H. (2019). Silver Nanoparticle-Induced Nephrotoxicity in Clarias gariepinus: Physio-Histological Biomarkers. Fish. Physiol. Biochem. 45, 1895–1905. doi:10.1007/s10695-019-00686-7
Marigómez I., Soto M., Cajaraville M. P., Angulo E., Giamberini L. (2002). Cellular and Subcellular Distribution of Metals in Molluscs. Microsc. Res. Tech. 56, 358–392. doi:10.1002/jemt.10040
Martirosyan A., Bazes A., Schneider Y.-J. (2014). In Vitrotoxicity Assessment of Silver Nanoparticles in the Presence of Phenolic Compounds - Preventive Agents against the Harmful Effect? Nanotoxicology 8, 573–582. doi:10.3109/17435390.2013.812258
McGillicuddy E., Murray I., Kavanagh S., Morrison L., Fogarty A., Cormican M., et al. (2017). Silver Nanoparticles in the Environment: Sources, Detection and Ecotoxicology. Sci. total Environ. 575, 231–246. doi:10.1016/j.scitotenv.2016.10.041
McShan D., Ray P. C., Yu H. (2014). Molecular Toxicity Mechanism of Nanosilver. J. food drug analysis 22, 116–127. doi:10.1016/j.jfda.2014.01.010
Mekkawy I. A., Mahmoud U. M., Hana M. N., Sayed A. E.-D. H. (2019). Cytotoxic and Hemotoxic Effects of Silver Nanoparticles on the African Catfish, Clarias gariepinus (Burchell, 1822). Ecotoxicol. Environ. Saf. 171, 638–646. doi:10.1016/j.ecoenv.2019.01.011
Mendes L., Maria V., Scott-Fordsmand J., Amorim M. (2015). Ag Nanoparticles (Ag NM300K) in the Terrestrial Environment: Effects at Population and Cellular Level in Folsomia candida (Collembola). Ijerph 12, 12530–12542. doi:10.3390/ijerph121012530
Menvielle-Bourg F. J. (2005). La Superoxyde Dismutase, Puissant Antioxydant Naturel, Désormais Disponible Par Voie Orale. Phytotherapy 3, 118–121. doi:10.1007/s10298-005-0087-9
Moreau R., Gourdoux L., Dutrieu J., Benkhay A. (1984). Hemolymph Trehalose and Carbohydrates in Starved Male Adult Locust a Migratoria: Possibility of Endocrine Modification. Comp. Biochem. Physiology Part A Physiology 78, 481–485. doi:10.1016/0300-9629(84)90582-6
Mwaanga P., Carraway E. R., van den Hurk P. (2014). The Induction of Biochemical Changes in Daphnia Magna by CuO and ZnO Nanoparticles. Aquat. Toxicol. 150, 201–209. doi:10.1016/j.aquatox.2014.03.011
Naguib M., Mahmoud U. M., Mekkawy I. A., Sayed A. E.-D. H. (2020). Hepatotoxic Effects of Silver Nanoparticles on Clarias gariepinus; Biochemical, Histopathological, and Histochemical Studies. Toxicol. Rep. 7, 133–141. doi:10.1016/j.toxrep.2020.01.002
Nishikimi M., Appaji Rao N., Yagi K. (1972). The Occurrence of Superoxide Anion in the Reaction of Reduced Phenazine Methosulfate and Molecular Oxygen. Biochem. biophysical Res. Commun. 46, 849–854. doi:10.1016/s0006-291x(72)80218-3
Ohkawa H., Ohishi N., Yagi K. (1979). Assay for Lipid Peroxides in Animal Tissues by Thiobarbituric Acid Reaction. Anal. Biochem. 95, 351–358. doi:10.1016/0003-2697(79)90738-3
Padmaja J., Balapara M. (1994). Effect of an Organochlorine and Three Organophosphate Pesticides on Glucose, Glycogen, Lipid, and Protein Contents in Tissues of the Freshwater Snail Bellamya Dissimilis (Müller). Bull. Environ. Contam. Toxicol. 53, 142–148. doi:10.1007/bf00205151
Parada J., Rubilar O., Fernández-Baldo M. A., Bertolino F. A., Durán N., Seabra A. B., et al. (2019). The Nanotechnology Among US: Are Metal and Metal Oxides Nanoparticles a Nano or Mega Risk for Soil Microbial Communities? Crit. Rev. Biotechnol. 39, 157–172. doi:10.1080/07388551.2018.1523865
Patricia C. S., Nerea G.-V., Erik U., Elena S. M., Eider B., Darío D. M. W., et al. (2017). Responses to Silver Nanoparticles and Silver Nitrate in a Battery of Biomarkers Measured in Coelomocytes and in Target Tissues of Eisenia fetida Earthworms. Ecotoxicol. Environ. Saf. 141, 57–63. doi:10.1016/j.ecoenv.2017.03.008
Radwan M. A., El-Gendy K. S., Gad A. F. (2010). Oxidative Stress Biomarkers in the Digestive Gland of Theba Pisana Exposed to Heavy Metals. Arch. Environ. Contam. Toxicol. 58, 828–835. doi:10.1007/s00244-009-9380-1
Radwan M. A., Essawy A. E., Abdelmeguied N. E., Hamed S. S., Ahmed A. E. (2008). Biochemical and Histochemical Studies on the Digestive Gland of Eobania Vermiculata Snails Treated with Carbamate Pesticides. Pesticide Biochem. physiology 90, 154–167. doi:10.1016/j.pestbp.2007.11.011
Radwan M. A., Mohamed M. S. (2013). Imidacloprid Induced Alterations in Enzyme Activities and Energy Reserves of the Land Snail, Helix Aspersa. Ecotoxicol. Environ. Saf. 95, 91–97. doi:10.1016/j.ecoenv.2013.05.019
Ribeiro M., Maria V., Scott-Fordsmand J., Amorim M. (2015). Oxidative Stress Mechanisms Caused by Ag Nanoparticles (NM300K) Are Different from Those of AgNO3: Effects in the Soil Invertebrate Enchytraeus Crypticus. Ijerph 12, 9589–9602. doi:10.3390/ijerph120809589
Rodríguez C., Sanchez H., Juan C. (2007). Earthworm Biomarkers of Pesticide Contamination: Current Status and Perspectives. J. Pesticide Sci. 32, 360. doi:10.1584/jpestics.R07-14
Roling J. A., Baldwin W. S. (2006). Alterations in Hepatic Gene Expression by Trivalent Chromium in Fundulus heteroclitus. Mar. Environ. Res. 62, S122–S127. doi:10.1016/j.marenvres.2006.04.008
Roubalová R., Procházková P., Dvořák J., Škanta F., Bilej M. (2015). The Role of Earthworm Defense Mechanisms in Ecotoxicity Studies. Invertebr. Surviv. J. 12, 203–213.
Sakka Y., Skjolding L. M., Mackevica A., Filser J., Baun A. (2016). Behavior and Chronic Toxicity of Two Differently Stabilized Silver Nanoparticles to Daphnia Magna. Aquat. Toxicol. 177, 526–535. doi:10.1016/j.aquatox.2016.06.025
Samet J. M., Wages P. A. (2018). Oxidative Stress from Environmental Exposures. Curr. Opin. Toxicol. 7, 60–66. doi:10.1016/j.cotox.2017.10.008
Sánchez-Aguayo I., Ballesteros F., Hidalgo J., López-Campos J. L. (1987). Epithelial Cell Types of the Primary Ureter of Helix Aspersa: Ultrastructural and Cytochemical Characteristics. Tissue Cell. 19, 265–273. doi:10.1016/0040-8166(87)90011-5
Sayed A. E.-D. H., Soliman H. A. M. (2017). Developmental Toxicity and DNA Damaging Properties of Silver Nanoparticles in the Catfish ( Clarias gariepinus ). Mutat. Research/Genetic Toxicol. Environ. Mutagen. 822, 34–40. doi:10.1016/j.mrgentox.2017.07.002
Sayed A. E. D. H. (2016). Genotoxicity Detection Following Exposure to Silver Nanoparticles in African Catfish (Clarias gariepinus). Ijnp 9, 41–53. doi:10.1504/ijnp.2016.078531
Sayed A., Younes H. M. (2017). Melanomacrophage Centers in Clarias gariepinus as an Immunological Biomarker for Toxicity of Silver Nanoparticles. J. Microsc. Ultrastruct. 5, 97–104. doi:10.1016/j.jmau.2016.07.003
Schlich K., Klawonn T., Terytze K., Hund-Rinke K. (2013). Effects of Silver Nanoparticles and Silver Nitrate in the Earthworm Reproduction Test. Environ. Toxicol. Chem. 32, 181–188. doi:10.1002/etc.2030
Sharma V. K., Filip J., Zboril R., Varma R. S. (2015). Natural Inorganic Nanoparticles - Formation, Fate, and Toxicity in the Environment. Chem. Soc. Rev. 44, 8410–8423. doi:10.1039/c5cs00236b
Shiroky J. B., Neville C., Esdaile J. M., Choquette D., Zummer M., Hazeltine M., et al. (1993). Low-dose Methotrexate with Leucovorin (Folinic Acid) in the Management of Rheumatoid Arthritis. Results of a Multicenter Randomized, Double-Blind, Placebo-Controlled Trial. Arthritis & Rheumatism 36, 795–803. doi:10.1002/art.1780360609
Snyman R. G., Reinecke A. J., Reinecke S. A. (2009). Quantitative Changes in Digestive Gland Cells and Oocytes of Helix Aspersa, as Biomarkers of Copper Oxychloride Exposure under Field Conditions. Bull. Environ. Contam. Toxicol. 83, 19–22. doi:10.1007/s00128-009-9721-9
Snyman R. G., Reinecke A. J., Reinecke S. A. (2005). Quantitative Changes in the Digestive Gland Cells of the Snail Helix Aspersa after Exposure to the Fungicide Copper Oxychloride. Ecotoxicol. Environ. Saf. 60, 47–52. doi:10.1016/j.ecoenv.2003.11.008
Terevinto A., Ramos A., Castroman G., Cabrera M. C., Saadoun A. (2010). Oxidative Status, In Vitro Iron-Induced Lipid Oxidation and Superoxide Dismutase, Catalase and Glutathione Peroxidase Activities in Rhea Meat. Meat Sci. 84, 706–710. doi:10.1016/j.meatsci.2009.11.007
Valko M., Morris H., Cronin M. (2005). Metals, Toxicity and Oxidative Stress. Cmc 12, 1161–1208. doi:10.2174/0929867053764635
Vance M. E., Kuiken T., Vejerano E. P., McGinnis S. P., Hochella M. F., Rejeski D., et al. (2015). Nanotechnology in the Real World: Redeveloping the Nanomaterial Consumer Products Inventory. Beilstein J. Nanotechnol. 6, 1769–1780. doi:10.3762/bjnano.6.181
Viard B., Pihan F., Promeyrat S., Pihan J.-C. (2004). Integrated Assessment of Heavy Metal (Pb, Zn, Cd) Highway Pollution: Bioaccumulation in Soil, Graminaceae and Land Snails. Chemosphere 55, 1349–1359. doi:10.1016/j.chemosphere.2004.01.003
Viarengo A., Canesi L., Pertica M., Poli G., Moore M. N., Orunesu M. (1990). Heavy Metal Effects on Lipid Peroxidation in the Tissues of mytilus Gallopro Vincialis Lam. Comp. Biochem. Physiology Part C Comp. Pharmacol. 97, 37–42. doi:10.1016/0742-8413(90)90168-9
Vu X. H., Duong T. T. T., Pham T. T. H., Trinh D. K., Nguyen X. H., Dang V.-S. (2018). Synthesis and Study of Silver Nanoparticles for Antibacterial Activity against Escherichia coli and Staphylococcus aureus. Adv. Nat. Sci. Nanosci. Nanotechnol. 9, 025019. doi:10.1088/2043-6254/aac58f
Wang J., Che B., Zhang L. W., Dong G., Luo Q., Xin L. (2017). Comparative Genotoxicity of Silver Nanoparticles in Human Liver HepG2 and Lung Epithelial A549 Cells. J. Appl. Toxicol. 37, 495–501. doi:10.1002/jat.3385
Wible D. J., Bratton S. B. (2018). Reciprocity in ROS and Autophagic Signaling. Curr. Opin. Toxicol. 7, 28–36. doi:10.1016/j.cotox.2017.10.006
Wijnhoven S. W. P., Peijnenburg W. J. G. M., Herberts C. A., Hagens W. I., Oomen A. G., Heugens E. H. W., et al. (2009). Nano-silver - a Review of Available Data and Knowledge Gaps in Human and Environmental Risk Assessment. Nanotoxicology 3, 109–138. doi:10.1080/17435390902725914
Yan A., Chen Z. (2019). Impacts of Silver Nanoparticles on Plants: a Focus on the Phytotoxicity and Underlying Mechanism. Ijms 20, 1003. doi:10.3390/ijms20051003
Keywords: silver nanoparticles, nitrate, Lehmannia nyctelia, oxidative stress, DNA
Citation: Bakr Z, Said SM, Mohammad WA, Aboulnasr GN and Elshimy NA (2022) Silver-Nanoparticle- and Silver-Nitrate-Induced Antioxidant Disbalance, Molecular Damage, and Histochemical Change on the Land Slug (Lehmannia nyctelia) Using Multibiomarkers. Front. Physiol. 13:945776. doi: 10.3389/fphys.2022.945776
Received: 16 May 2022; Accepted: 15 June 2022;
Published: 01 August 2022.
Edited by:
Francesco Fazio, University of Messina, ItalyCopyright © 2022 Bakr, Said, Mohammad, Aboulnasr and Elshimy. This is an open-access article distributed under the terms of the Creative Commons Attribution License (CC BY). The use, distribution or reproduction in other forums is permitted, provided the original author(s) and the copyright owner(s) are credited and that the original publication in this journal is cited, in accordance with accepted academic practice. No use, distribution or reproduction is permitted which does not comply with these terms.
*Correspondence: Zeinab Bakr, emVpbmFiX2Jha3JAc2NpZW5jZS5hdW4uZWR1LmVn