- 1Center of Excellence for Poultry Science, University of Arkansas, Fayetteville, AR, United States
- 2Department of Agricultural and Food Sciences, Alma Mater Studiorum—University of Bologna, Bologna, Italy
With the growing global demand for animal protein and rising temperatures caused by climate change, heat stress (HS) is one of the main emerging environmental challenges for the poultry industry. Commercially-reared birds are particularly sensitive to hot temperatures, so adopting production systems that mitigate the adverse effects of HS on bird performance is essential and requires a holistic approach. Feeding and nutrition can play important roles in limiting the heat load on birds; therefore, this review aims to describe the effects of HS on feed intake (FI) and nutrient digestibility and to highlight feeding strategies and nutritional solutions to potentially mitigate some of the deleterious effects of HS on broiler chickens. The reduction of FI is one of the main behavioral changes induced by hot temperatures as birds attempt to limit heat production associated with the digestion, absorption, and metabolism of nutrients. Although the intensity and length of the heat period influences the type and magnitude of responses, reduced FI explains most of the performance degradation observed in HS broilers, while reduced nutrient digestibility appears to only explain a small proportion of impaired feed efficiency following HS. Targeted feeding strategies, including feed restriction and withdrawal, dual feeding, and wet feeding, have showed some promising results under hot temperatures, but these can be difficult to implement in intensive rearing systems. Concerning diet composition, feeding increased nutrient and energy diets can potentially compensate for decreased FI during HS. Indeed, high energy and high crude protein diets have both been shown to improve bird performance under HS conditions. Specifically, positive results may be obtained with increased added fat concentrations since lipids have a lower thermogenic effect compared to proteins and carbohydrates. Moreover, increased supplementation of some essential amino acids can help support increased amino acid requirements for maintenance functions caused by HS. Further research to better characterize and advance these nutritional strategies will help establish economically viable solutions to enhance productivity, health, welfare, and meat quality of broilers facing HS.
Introduction
The poultry industry continues to play a critical role in meeting the growing demand for animal protein. The global production of chicken and turkey meat has doubled over the last 20 years, reaching 125.5 million tons in 2020 (FAO, 2022). This accounts for approximately 37% of global meat production, while poultry meat only represented 29% in the early 2000s. With the increasing global population projected to rise from 7.8 to 9.9 billion in 2050 (PRB, 2020) and better access to animal products in developing areas, it is predicted that animal-based food demand will grow by nearly 70% in the same timeline (Searchinger et al., 2019). Meanwhile, climate change represents one of the major concerns for livestock production in the coming decades. Some reports indicate that industrialized farming systems may lose 25% of their animal production, and this scenario may be worse for some tropical regions where extensive farming systems are more abundant (Nardone et al., 2010). Emerging estimates by the Intergovernmental Panel on Climate Change emphasized that global warming of more than 2°C will occur during the 21st century unless large reductions in CO2 and other greenhouse gas emissions are acheived soon (IPCC, 2021). Also, the authors indicated that climate change is already and will continue increasing the frequency and intensity of extreme weather events like hot temperature waves. Therefore, the poultry industry needs to continue adopting technologies and practices that reduce its impact on the environment, but it should also adopt production systems that are resilient in the face of rising global temperatures.
Modern broiler chickens are particularly sensitive to hot temperatures due to their rapid growth rates resulting from genetic selection to enhance production efficiency, as well as from limitations in heat dissipation caused by feathering, an absence of sweat glands, and relatively high stocking densities in intensive commercial rearing facilities (Lara and Rostagno, 2013; Emami et al., 2020). Heat stress (HS) occurs when the amount of heat produced by an animal surpasses its capacity to dissipate the heat to the surrounding environment. When the environmental temperature rises above the thermoneutral zone, birds typically reduce their physical activity and feed intake (FI) to limit heat production (HP), as well as increase their panting and water consumption to favor heat loss by evaporation (Renaudeau et al., 2012). Indeed, elevated temperatues trigger important physiologic and metabolic changes as described in Part I of this review (Brugaletta et al., 2022), and chronic HS exposure results in significant losses in bird performance, negatively affects welfare, challenges food safety, and reduces the overall economic efficiency of poultry production (Lara and Rostagno, 2013; Pawar et al., 2016). Consequently, HS has been estimated to cause annual economic losses of $128 to $165 million for the United States poultry industry (St-Pierre et al., 2003), but these figures probably underestimate current and future losses due to the growth of the poultry industry over the last decade and the worsening of climate change predictions.
Mitigating the adverse effects caused by hot temperatures in poultry productions requires a holistic and multi-factorial approach. Housing (Oloyo, 2018), management practices (Saeed et al., 2019), genetic selection (Kumar et al., 2021), and feeding and nutrition (Syafwan et al., 2011; Fouad et al., 2016; Sugiharto et al., 2017; Wasti et al., 2020; Abdel-Moneim et al., 2021; Chowdhury et al., 2021) can all provide some benefit to birds under HS conditions and have been the topics of several recent global reviews (Lin et al., 2006b; Nawab et al., 2018; Goel et al., 2021; Vandana et al., 2021). This review aims to assess the effects of HS on FI and nutrient digestibility, as well as to evaluate different feeding strategies and nutritional solutions to mitigate some of the adverse effects of HS on poultry. Effects on broiler chickens will be emphasized, though research with other types of poultry will be discussed where relevant. Further, this review will focus on macro-nutritional solutions as carbohydrates, fat, and proteins are the main source of energy, and their oxidation results in HP (Costa-Pinto and Gantner, 2020), which needs to be limited under HS.
Impact of heat stress on feed intake regulation and nutrient digestibility
Nearly all studies that have investigated the effects of HS in poultry have observed reductions in FI of heat-stressed birds compared with those in thermoneutral conditions, including meta-analyses conducted in broilers (Liu et al., 2020) and laying hens (Mignon-Grasteau et al., 2015). This reduction of FI observed under HS conditions reduces endogenous HP associated with digestion, absorption, and metabolism of nutrients (Lara and Rostagno, 2013). However, the magnitude of the FI reduction depends on several parameters related to the characteristics of the HS model imposed on the birds, and this can complicate comparisons among studies. Temperature, length and cyclicality of the heat period, and age of the birds at the beginning and the end of the HS period are all potential factors that can influence the intensity of the FI reduction. Many studies have used a constant HS model with high temperatures applied over a long period of time (Baziz et al., 1996; Geraert et al., 1996; Bonnet et al., 1997; Faria Filho et al., 2007). However, more recent studies have employed cyclic HS models combining higher temperatures during the day and lower temperatures during the night which may better simulate field conditions in temperate areas of the world (De Souza et al., 2016; Flees et al., 2017; Greene et al., 2021). When compared within the same experiment, cyclic HS decreased FI by 15% on average, while constant HS resulted in higher reductions ranging from 25% to 45% (De Souza et al., 2016; Awad et al., 2018; Teyssier et al., 2022). Therefore, cyclic HS resulted in a 1.5% reduction in FI per degree Celsius, while the values obtained under constant HS corroborate the expected response proposed by Baziz et al. (1996) of about a 3.5% reduction in FI per degree Celsius increase between 22°C and 35°C.
Interestingly, the reduction of growth observed under HS is greater than expected due to the reduced FI alone, leading to a lower feed efficiency (Renaudeau et al., 2012). The use of pair-feeding techniques, where birds under thermoneutral conditions are fed the same amount of feed consumed by heat-stressed birds, have shown that the reduction in growth due to decreased FI ranges between 60% and 99% (Geraert et al., 1996; Bonnet et al., 1997; Garriga et al., 2006; Lu et al., 2007; Zuo et al., 2015; De Souza et al., 2016; Zeferino et al., 2016; De Antonio et al., 2017; Emami et al., 2021; Ma et al., 2021; Teyssier et al., 2022). Therefore, the lower FI is the main factor explaining impaired performance of chickens observed under HS, with the remainder of the growth reduction attributable to impaired digestibility or physiological and metabolic changes that influence feed efficiency (Dale and Fuller, 1980; Geraert et al., 1996; Renaudeau et al., 2012).
Several studies have reported reduced dry matter (DM) digestibility in quails (Orhan et al., 2020) and laying hens (Kim et al., 2020) under HS conditions. In broilers, Bonnet et al. (1997) and De Souza et al. (2016) observed decreases of 1.6% and 3.9% in DM digestibility under constant HS. However, other studies have reported no DM digestibility losses due to HS (Faria Filho et al., 2007; Attia et al., 2016, 2017). At the nutrient level, even though no change in crude protein (CP) digestibility were observed by several authors (Faria Filho et al., 2007; Habashy et al., 2017b; Kim et al., 2020), numerous studies have reported decreases in CP or nitrogen digestibility ranging between 1.5% and 10% under hot temperatures (Zuprizal et al., 1993; Bonnet et al., 1997; Soleimani et al., 2010; Attia et al., 2016, 2017; De Souza et al., 2016; Orhan et al., 2020). The detrimental effect of HS has also been measured on amino acid (AA) digestibility. Wallis and Balnave (1984) observed a slight decrease in the digestibility for Thr, Ala, Met, Ile, and Leu, with greater impacts in male than in female birds. Standardized and apparent digestibility values of several AA (i.e., Arg, His, Thr, Val, Lys, Ile, Leu, Phe, Cys, Gly, Ser, Ala, Pro, and Tyr) were also reduced by approximately 5.5%, in the study of Soleimani et al. (2010). Regarding other nutrients, none of these studies observed an impact on crude fat digestibility, and only Kim et al. (2020) measured a reduction in NDF digestibility with laying hens.
Several mechanisms have been proposed to explain possible negative effects of HS on nutrient digestibility. Lower expression and activity of digestive enzymes, including trypsin, chymotrypsin, lipase, amylase, and maltase, have been observed in broilers reared under high temperatures (Hai et al., 2000; Song et al., 2018; Al-Zghoul et al., 2019). As described in Part I, oxidative stress induced by HS aggravates intestinal barrier disorders (Brugaletta et al., 2022), and hyperthermia has been associated with a reduction in upper gastrointestinal tract blood flow that can induce degradation of the intestinal mucosa (Song et al., 2014; Chegini et al., 2018). Following hot temperature exposure, the absorptive surface area of the small intestine is decreased due to a reduction in villi height, crypt depth (Song et al., 2018; He et al., 2019), and relative jejunal weight (Garriga et al., 2006). Heat stress also modulates the gene expression of several macronutrient transporters. Expression of glucose transporters SGLT1 and GLUT2 is downregulated when HS persists for several days (Sun et al., 2015; Habashy et al., 2017b; Al-Zghoul et al., 2019; Abdelli et al., 2021; Goel et al., 2021), whereas the expression of GLUT5 for the transport of fructose is increased (Habashy et al., 2017b). Despite the relatively greater decrease in AA digestibility compared to other macronutrients, several studies observed no influence of HS exposure on expression of AA transporters, including CAT1, y+LAT1, PePT1, and r-Bat (Sun et al., 2015; Habashy et al., 2017b; Al-Zghoul et al., 2019). On the other hand, Habashy et al. (2017a) measured a decrease in expression of several AA transporters (i.e., CAT1, LAT1, SNAT1, SNAT 2, SNAT 7, B0AT) after 12 days of HS. However, this reduction was not consistent with the slight increase in AA digestibility ( + 3%) observed in the same study.
Furthermore, even though HS does not seem to markedly affect fat digestibility, several studies have reported decreased intestinal expression of FABP and CD36 which are both involved in the uptake of fatty acids (Sun et al., 2015; Habashy et al., 2017b; Al-Zghoul et al., 2019), whereas the expression of FATP1 was increased under chronic HS (Habashy et al., 2017b).
While the regulation of nutrient transporter gene expression might be directly related to physiological adaptations to HS, it is important to consider that structural damages and the degradation of the epithelium induced by HS might be a potential factor indirectly causing the reduction of intestinal transporters (Habashy et al., 2017b). Overall, the slight decrease and inconsistent results regarding nutrient digestibility seem to indicate that reduced digestibility likely explains only a small proportion of reduced feed efficiency under HS conditions.
Feeding strategies
Lowering HP and improving heat dissipation are two ways to reduce the adverse effects of HS in poultry. While the reduction of HP is achievable by improving digestibility and by feeding the birds closer to their nutrient and energy requirements, an increased heat dissipation is possible by increasing the amount of water loss by evaporation (Syafwan et al., 2011). Several feeding strategies have been tested to attempt to mitigate the negative impact of hot temperatures through these means.
Feed restriction and withdrawal
Early studies focused on feed restriction before HS exposure, and its effects on HP and performance. In broiler breeders, feed restriction from 44 to 48 weeks before exposure to 4 days of elevated temperatures resulted in 23% decrease in HP compared with ad libitum fed birds. However, fed-restricted birds had a higher HP when adjusted for body weight (BW) differences and expressed per unit of metabolic body size (BW0.75). The lower BW of fed-restricted birds was therefore responsible for the reduction in HP and not the feed restriction per se (MacLeod and Hocking, 1993). In broilers, no beneficial effect of a preventative feed restriction was measured on performance and carcass quality (Plavnik and Yahav, 1998), but more promising results were obtained when feed restriction was applied during the HS period. Abu-Dieyeh (2006) observed that feed restriction to 75% and 50% of the feed consumption of ad-libitum fed broilers reduced rectal temperature, mortality, and feed conversion ratio (FCR). However, feed restriction diminished the rate of BW gain (BWG) and delayed marketing age of the birds.
Similarly, feed withdrawal for at least 6 h during HS decreased the corporal temperature (Yalçin et al., 2001; Özkan et al., 2003; Lozano et al., 2006), mortality (Yalçin et al., 2001) and heterophil-to-lymphocyte ratio (Yalçin et al., 2003) of broilers, indicating a reduction of the adverse effects of HS. Nevertheless, effects on performance were not consistent throughout the studies, with some observing a growth improvement (Yalçin et al., 2001; Mohamed et al., 2019) and others reporting a growth degradation (Lozano et al., 2006) likely due to the timing and magnitude of feed restriction (Özkan et al., 2003). Therefore, a short feed withdrawal during the hottest period of the day appears to be the best strategy to minimize the negative effects of HS on growth and delayed market ages. Removing the feed a few hours before the HS period could also be beneficial to avoid the potential increased in HP induced by anticipatory feeding behavior observed in birds exposed to repeated intermittent fasting (Fondevila et al., 2020).
Dual feeding
Dual feeding is characterized by the distribution of two different diets, one more concentrated in protein and the other more concentrated in energy, that are provided either simultaneously for self-selection or in sequential order. Dietary proteins are known to have a higher thermogenic effect compared with carbohydrates (Geraert, 1991), and feeding high protein diets during the coolest period of the day has been hypothesized to improve the thermotolerance of birds. Sequential feeding of high energy and high protein diets decreased body temperature (De Basilio et al., 2001; Lozano et al., 2006) and mortality (De Basilio et al., 2001), but reduced or did not improve the growth of broilers. Syafwan et al. (2012) tested self-selection under hot temperatures by providing a high-protein diet (CP: 299 g/kg; ME: 2,780 kcal/kg) and a high-energy diet (CP: 150.7 g/kg; ME: 3,241 kcal/kg) and showed that choice-fed and control-fed birds with a standard diet (CP: 215 g/kg; ME: 2,895 kcal/kg) performed similarly, although the former had 14% lower protein intake and 6.4% higher energy intake. However, no data on carcass composition were reported, and a lower protein intake could reduce muscle deposition. While a dual-feeding approach might be feasible in tropical areas and less-intensive production systems, Iyasere et al. (2021) estimated that it is not suitable for most commercial production operations due to cost and logistical constraints.
Wet feeding
Water is the most important nutrient in broiler nutrition, and it plays an essential role for thermoregulation under hot temperatures. Heat stress increases water loss through the respiratory tract as birds pant to increase heat loss by evaporative cooling (Richards, 1970; Bruno et al., 2011). In the light of the importance of water for the nutrition and physiology of broilers, wet feeding attempts to maximize water intake and utilization. Several studies have investigated the effect of wet feeding, i.e., the use of high moisture diets, on poultry performance under thermoneutral conditions (Moritz et al., 2001; Shariatmadari and Forbes, 2005; Khoa, 2007) and during HS. In heat-stressed broilers, Kutlu (2001) measured increased BWG, DM intake, carcass weight, protein content, but also increased abdominal fat and lipid content per unit of carcass weight, and reduced DM conversion efficiency (DM intake/BWG), when feed was mixed with the same amount of water. Similarly, Awojobi et al. (2009) and Dei and Bumbie (2011) observed increased BWG with wet-fed birds (addition from 1 to 2 parts of water to 1 part of dry feed) reared in tropical conditions. In laying hens, Tadtiyanant et al. (1991) reported that wet feeding increased DM intake, but no beneficial effects were found on performance. In contrast to these results, egg production and egg weight were increased by wet feeding in Japanese quails (Okan et al., 1996a; 1996b). Despite somewhat positive impacts of wet feeding in poultry, its application in the field remains limited due to an increased risk of fungal growth and resulting mycotoxicosis in birds (Wasti et al., 2020).
Feed form (mash vs. crumble vs. pellets) and feed structure (particle size)
Three different forms of feed are generally used in the poultry industry: mash, crumble, and pellets. Under thermoneutral conditions, pelleted feed is known to increase FI and BWG and improve digestibility (Massuquetto et al., 2018; Massuquetto et al., 2019). During summer, increased feed efficiency and egg production of laying hens have been observed for pelleted diets compared with mash diets (Almirall et al., 1997). In broilers exposed to cyclic HS, Cardoso et al. (2022) measured increased FI ( + 10%), BWG ( + 8.3%), CP digestibility ( + 2.3%), and energy utilization (apparent metabolizable energy, AME and nitrogen-corrected apparent metabolizable energy, AMEn) when feeding a pelleted diet compared with a mash diet. However, pelleting did not improve FCR, livability, or the feed production cost to kg of bird produced ratio. Likewise, Hosseini and Afshar (2017a) observed beneficial effects of pelleting on performance and digestibility when comparing mash, crumbled and pelleted diets under similar cyclic HS conditions. These authors also reported improved carcass weight and yield in heat-stressed broilers fed pelleted diets. Comparable performance improvements were obtained by feeding pelleted diets under thermoneutral and HS conditions (Serrano et al., 2013), so it is likely that mechanisms responsible for the positive effects of pelleting under thermoneutrality can be applied to HS conditions. Pelleting feed has been shown to lower feed wastage (Gadzirayi et al., 2006) and increase feed consumption, while concomitantly reducing physical activity and HP (Skinner-Noble et al., 2005; Latshaw and Moritz, 2009). Furthermore, as observed under thermoneutral (Abdollahi et al., 2011; Serrano et al., 2013) and HS conditions (Hosseini and Afshar, 2017b), pelleted diets reduce the relative weight of the digestive tract compared with birds fed mash diets. The pelleting process can further reduce ingredient particle size, reducing the mechanical stimulation of the gizzard and could therefore lower the energy requirements for maintenance. It also could release some inaccessible nutrients and enhance energy utilization, which could explain the increase in abdominal fat observed by Hosseini and Afshar (2017a) with pelleted diets fed under cyclic HS. Other potential benefits of feeding pelleted feeds during HS shown by these authors include increased villus length and villus to crypt depth ratio in the jejunum (Hosseini and Afshar, 2017b) as well as decreased breast HSP70 mRNA expression, breast creatine kinase protein level, and heterophil-to-lymphocyte ratio (Hosseini and Afshar, 2017a). Collectively, these reports indicate that pelleting attenuates the harmful effects of high ambient temperature in broiler chickens.
Concerning particle size, the use of coarse particles (2,280 µm) of corn increased panting compared to finer particles (605 µm) in broilers fed a mash diet under natural HS conditions (Santos et al., 2019). Similar results were found in laying hens under a semiarid environment, where coarser corn particles increased rectal temperature, respiratory rate, and decreased eggshell quality (De Souza et al., 2015). However, while coarse particles may increase the thermal challenge, they are also known to increase FI and improve performance in broilers under thermoneutral conditions (Amerah et al., 2008; Naderinejad et al., 2016). Thus, more research on broiler performance would be required to fully understand the role of ingredient particle size during HS.
Dietary energy density and lipid supplementation
The marked decrease in FI and in turn, energy intake, caused by elevated temperatures negatively affects bird performance. The effect of HS on energy utilization of feedstuffs, which is usually represented as AME, is still not well defined. Indeed, responses probably depend on the parameters of the HS imposed and characteristics of the diet, as some studies observed an increase in AME due to hot temperatures (Keshavarz and Fuller, 1980; Geraert et al., 1992), some observed no difference between thermoneutral and HS conditions (Yamazaki and Zi-Yi, 1982; Faria Filho et al., 2007; De Souza et al., 2016), and some have reported a decrease in AME with HS birds (Bonnet et al., 1997). However, three studies using the comparative slaughter technique with broilers placed under thermoneutral and HS conditions from d 28 to 42 (Geraert et al., 1996), or d 21 to 42 (Faria Filho et al., 2007; De Souza et al., 2016), indicate a decrease in retained energy and increase in HP per unit of feed when birds are placed under hot temperatures. Similarly, a quadratic effect of the temperature on the energy requirement for maintenance functions was measured by Sakomura et al. (2005), with the lowest requirements estimated at 25.2°C: MEm = BW0.75 x (307.87 + 15.63 T + 0.31 T2), with T being the temperature (°C) and BW0.75 the metabolic body size. Therefore, the relative contribution of maintenance energy requirements to total energy requirements is partly increased by the lower growth of HS birds, but also directly impacted by the increased temperature, which results in a diminishing effect on feed efficiency.
To compensate for lower energy intake of birds during HS, it has become common for producers in hot climate areas to feed higher energy diets (Wasti et al., 2020). Early studies suggested that high dietary energy concentrations could improve bird performance under constant (Dale and Fuller, 1979) and cyclic HS (Dale and Fuller, 1980), but it should be noted that the CP content of the diets were adjusted to energy levels and thus higher in high energy diets. Nonetheless, more recent studies using isonitrogenous diets have confirmed previous observations and showed that an increase in dietary metabolizable energy (ME) between 100 and 200 kcal/kg for broilers improved BWG up to 17% and FCR up to 10% (Raju et al., 2004; Ghazalah et al., 2008; Attia et al., 2011, 2018; Attia and Hassan, 2017) when reared under hot conditions. In addition, decreased skin and rectal temperatures have been observed in HS poultry fed diets with increased ME content (Al-Harthi et al., 2002; Attia et al., 2011). Increasing dietary ME content also improved ready-to-cook yield (Raju et al., 2004), although no improvement in carcass yield was observed by Ghazalah et al. (2008). However, both research groups reported an increased abdominal fat yield, thus the risk of increasing carcass yield from lipid and not protein deposition is a potential disadvantage of increasing dietary ME in HS broilers.
Increasing ME density in the diet is usually achieved by increasing the concentration of added lipid, and this strategy presents several potential advantages for HS birds. Feeding isocaloric diets with either higher proportions of carbohydrates or fat under HS conditions revealed that broilers had better performance when diets were supplemented with poultry fat, palm oil, or soybean oil compared to no fat supplementation (Zulkifli et al., 2006; Ghazalah et al., 2008). These observations are likely explained by the lower heat increment of fat oxidation compared with carbohydrates and proteins. Indeed, as measured by Fuller and Rendon (1977), high fat diets lead to lower heat increment than low-fat diets. Moreover, lipid inclusion improves nutrient digestion by slowing rate of passage (Mateos et al., 1982) and increasing the energy value of other nutrients (Aardsma et al., 2017). Lipid metabolism also generates more metabolic water than carbohydrate and protein catabolism, which can in turn be used for heat dissipation by evaporation (Barboza et al., 2009). Thus, as suggested by Ghazalah et al. (2008), a potential dietary recommendation for broilers exposed to hot temperatures could be to increase the ME level up to 3,300 kcal/kg, with lipid inclusion up to 5%, especially during the finishing period when birds are the most sensitive to high temperatures.
Although increasing dietary lipid additions has been shown to be a promising way to increase bird performance under HS conditions, less research has been conducted to compare the efficacy of different lipid sources. Zulkifli et al. (2006) did not observe a difference in BWG and FCR among broilers exposed to 34°C and supplemented either with 8% of palm oil or 8% of soybean oil. Abdominal fat and breast intramuscular fat deposition were also unaffected by the fat source. However, in broilers exposed to HS from 32 to 42 days post-hatch and fed isocaloric diets, improved FCR and BWG were observed when feeding diets with coconut oil or beef tallow than with diets containing olive or soybean oil (Seifi et al., 2020). The fatty acids within coconut oil and tallow are rich in saturated fatty acids and have chain lengths of mainly 12 and 16 carbons, respectively, while olive oil and soybean oil are rich in unsaturated fatty acids and have predominantly 18 carbon fatty acids. Short and medium chain fatty acids (SCFAs/MCFAs), containing up to 12 carbon atoms, are absorbed and metabolized more rapidly than longer chains, as they are transported to the portal vein as free fatty acid and do not require any transporter to get absorbed (Guillot et al., 1993), which could reduce the HP induced by digestion. Recent research also suggests saturated fatty acids, SCFAs, and MCFAs could have a beneficial impact on the mitochondrial metabolism and electron transport chain (Schönfeld and Wojtczak, 2016; Seifi et al., 2018, 2020; Hecker et al., 2021), which are known to be disrupted under HS condition (Akbarian et al., 2016).
Influence of dietary crude protein content
Proteins have a higher caloric increment than carbohydrates and fat (Musharaf and Latshaw, 1999) and therefore increase the diet-induced HP. When AA are metabolized for energy by birds, much of the HP is caused by deamination reactions and incorporation of N into uric acid (Smith et al., 1978; Swick et al., 2013). Therefore, optimizing dietary CP composition to better fit bird requirements decreases the heat produced during AA oxidation. So, in an effort to reduce the energy released during digestion, absorption, and metabolism of nutrients, dietary CP reductions have been proposed as a strategy to mitigate the harmful effects of HS in poultry (Furlan et al., 2004). Numerous studies in broilers have tested the effects of feeding a reduced CP diet versus a standard CP diet under constant HS (Alleman and Leclercq, 1997; Cheng et al., 1999; Faria Filho et al., 2005; Gonzalez-Esquerra and Leeson, 2005; Awad et al., 2018), cyclic HS (Cheng et al., 1999; Liu et al., 2016; Awad et al., 2018; Zulkifli et al., 2018; Amiri et al., 2019; Lin Law et al., 2019; Soares et al., 2020) and hot climates (Zaman et al., 2008; Laudadio et al., 2012; Awad et al., 2014, 2015, 2017; Lin Law et al., 2019; Attia et al., 2020). Table 1 summarizes 21 HS broiler trials comparing reduced CP diets (ranging from 143 to 190 g/kg CP) and standard CP diets (ranging from 183 to 223 g/kg CP), with both diets in each study formulated to meet or exceed a specific nutritional requirement, such as the Nutrient Requirements of Poultry (NRC, 1994) or breeder recommendations, or to contain similar AA profiles. Approximately half of these studies observed a significant reduction in performance when feeding broilers the reduced CP diet compared to the standard CP diet, while the other half did not observe dietary effects. The response variability can be partly explained by the range of low and standard CP levels, as well as the intensity and duration of the HS period, but it is important to note that feeding a low CP diet without degrading performance is still beneficial for reducing nitrogen excretion. Results for BWG, presented in Figure 1, indicate that regardless of the HS challenge type, reduced CP diets decreased BWG by 10.8% on average (ranging from a reduction of 40.1% to an improvement of 2.5%). Similar results were obtained with FCR, with an average increase of 6.9% (ranging from a decrease of 0.9% to an increase of 19.7%) when dietary CP was reduced (Figure 2). Some studies also reported a decreased FI with reduced CP diets (Cheng et al., 1999; Awad et al., 2014, 2015, 2017, 2018). In addition to a reduced CP diet, some researchers tested the effects of a higher CP diet, with CP levels above the standard recommendations. During HS, high CP diets resulted in a decrease (Cheng et al., 1999) or an increase in BWG (Faria Filho et al., 2005) and a decrease in FCR (Cheng et al., 1999; Faria Filho et al., 2005; Gonzalez-Esquerra and Leeson, 2005). However, other studies reported no effect of high versus standard CP diets (Zaman et al., 2008; Laudadio et al., 2012; Soares et al., 2020) and the increased diet cost associated with high CP diets could result in detrimental economical scenarios (Cardoso et al., 2022).
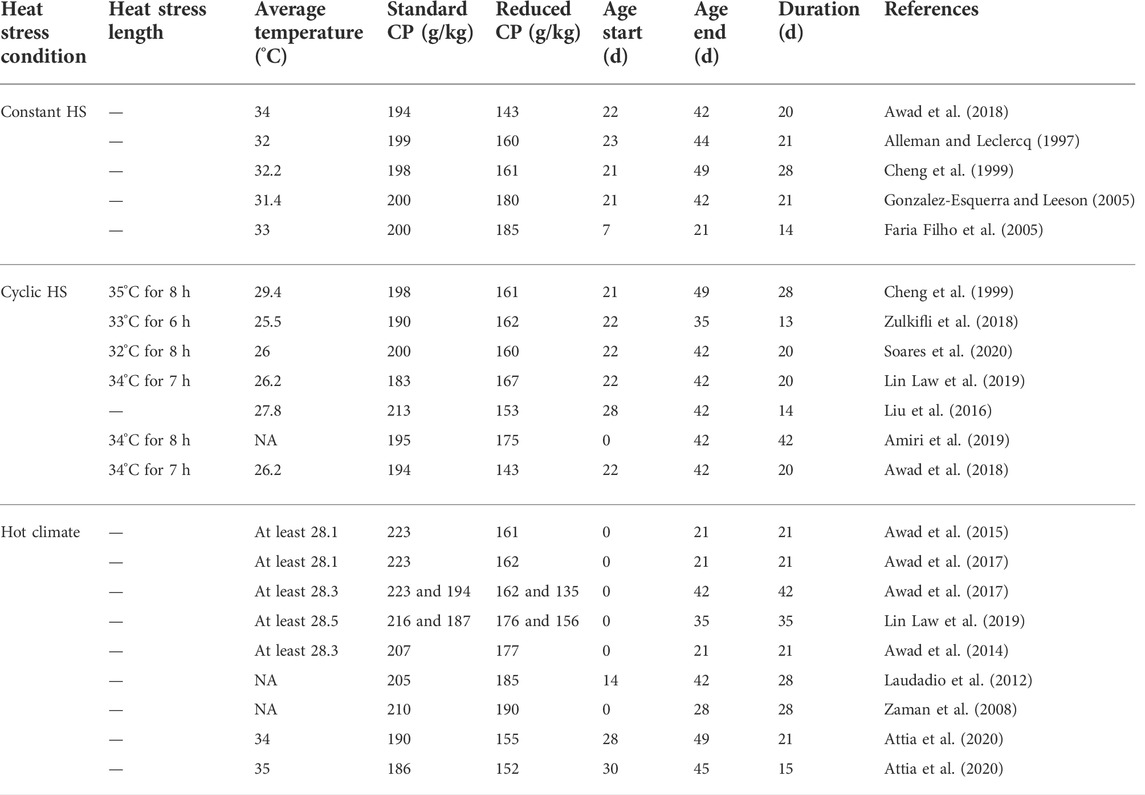
TABLE 1. Summary of experimental conditions of broiler studies comparing reduced and standard CP diets under HS conditions.
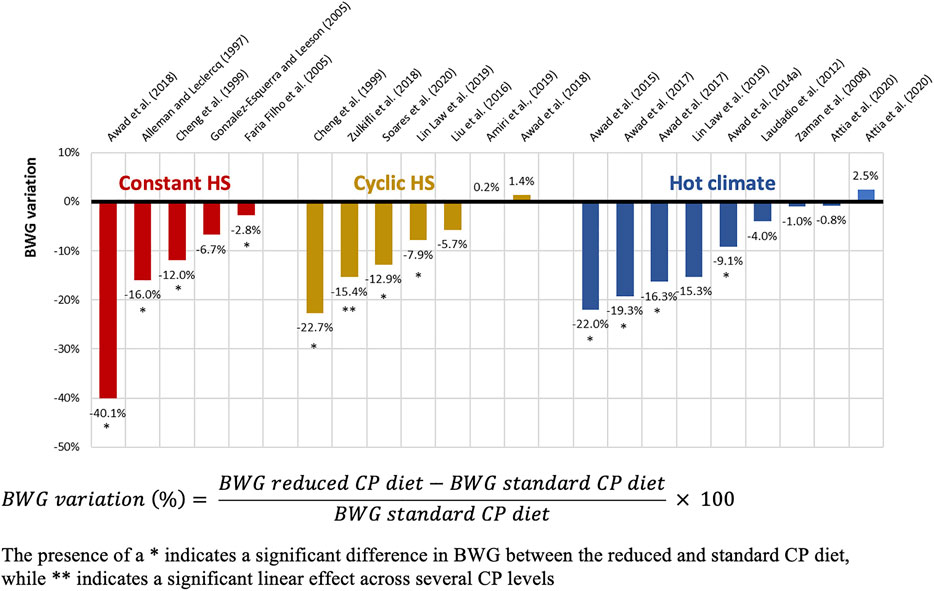
FIGURE 1. Effect of reduced and standard CP diets on BWG of broilers exposed to different HS conditions.
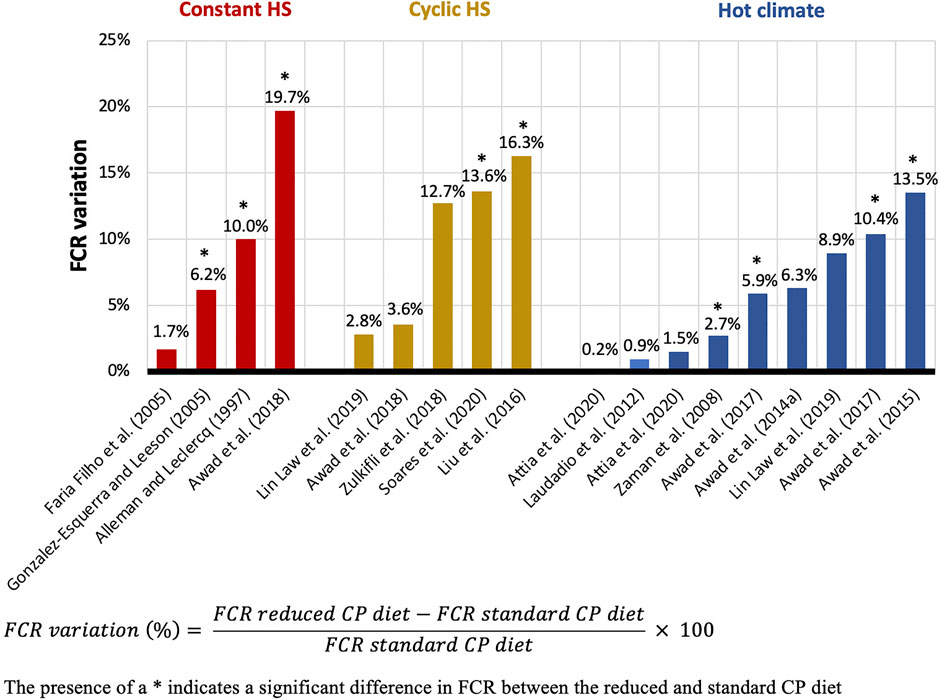
FIGURE 2. Effect of reduced and standard CP diets on FCR of broilers exposed to different HS conditions.
Feed-grade AA, which are included at higher levels in reduced CP diets to meet digestible AA requirements, allow to provide a balanced AA diet, and minimize the HP caused by AA oxidation, which is not possible to reach when relying on feed sources only. They also do not need enzymes for digestion and, as such, do not contribute to the digestion-related production of body heat (Morales et al., 2020). However, the performance degradations reported with reduced CP diets aligns with the lower HP observed in birds fed a high CP diet (220 g/kg) versus a low CP diet (160 g/kg) under cyclic HS conditions (Soares et al., 2020). Similar results have also been obtained under constant HS when comparing a high (230 g/kg), standard (200 g/kg), and low (170 g/kg) CP diets (Faria Filho et al., 2007). The lack of interaction between the CP level and environmental temperature reported by these authors is supported by studies conducted under thermoneutral conditions, where no difference (Noblet et al., 2003, 2007) or an increase (Swennen et al., 2004) in HP was measured with low CP diets, indicating that HS is not the cause per se of the higher HP with reduced CP diets. These results are surprising due to the higher caloric increment of proteins, but a possible explanation is that standard CP diets are usually formulated with a higher oil inclusion rate to reach the same amount of energy than reduced CP diets which generally have higher inclusion of corn (Soares et al., 2020). The extra-metabolic effect of dietary lipids, where the metabolizable energy value of the lipid exceeds its gross energy value (Aardsma et al., 2017), could compensate for the possible increase in heat increment derived from protein (Soares et al., 2020). Interestingly, reduced CP diets with AA deficiencies have also been associated with a greater plasma level of triiodothyronine (Carew et al., 1983, 1997; Buyse et al., 1992), which is known for its thermogenic effect (Collin et al., 2003).
Overall, simultaneously increasing dietary energy and CP could be a potentially beneficial strategy to limit the adverse effects of HS on broiler growth and feed efficiency. Indeed, improved performance has been demonstrated under HS conditions when broilers were fed both a high dietary ME and CP contents (Attia et al., 2006; Attia and Hassan, 2017). However, in a similar study in which broilers were exposed to thermoneutral temperatures or cyclic HS from day 19–42 and were fed with a dietary ME and CP content of 3,152 kcal/kg and 194.8 g/kg or 3,253 kcal/kg and 210.3 g/kg, respectively, no improvement in performance was observed with the higher nutrient and energy density diet in either environment. Consequently, an economic evaluation actually showed a decrease in overall profitability with the higher density diets (Cardoso et al., 2022).
The conflicting evidence of higher caloric increment of dietary protein and impaired performance of broilers fed reduced CP diets led Gonzalez-Esquerra and Leeson (2006) to conclude that no consensus has been reached on protein requirements of heat-stressed birds. More recent trials on reduced CP diets have shown no performance improvements or amelioration of HP reduction and do not support this dietary strategy under HS conditions. Nonetheless, when following the “ideal protein” concept, where all essential digestible AA are provided in balance (Baker and Chung, 1992), the supplementation of unbound feed-grade AA in reduced CP diets to satisfy the bird’s requirements should result in similar performance as when feeding standard CP diets. Furthermore, most of the studies presented above based their requirements on NRC or breeder recommendations, albeit broiler’s AA requirements under HS conditions still remain undefined. More importantly, although those studies met specific nutritional requirements for essential AA, some potentially limiting AA such as Arg, Thr, Ile, Leu, His, and Phe were not equally balanced between diets. Diets with AA imbalance can lead to adverse effects especially under HS condition as they normally increase HP (Sekiz et al., 1975). Also, the FI reduction triggered by HS reduces the amount of CP and AA ingested by the birds, potentially resulting in deficiency when compared with reduced dietary concentrations. Therefore, even if the inclusion level for all AA was formulated to meet or exceed a target nutritional requirement under thermoneutral conditions, the effective AA consumption may not have reached the bird’s requirements for some AA under HS conditions.
Further research on dietary CP and its interaction with energy and AA content would be required to better characterize the biological response induced by those diet changes under HS conditions. This would allow for a better understanding on the utilization of those nutrients in poultry reared under hot temperature to ultimately facilitate better prediction of economic outcomes associated with nutritional dietary variation.
Supplementation of amino acids
Amino acid density
Altering dietary density of essential AA has been shown to have promising results in heat-stressed broilers. In broilers under hot temperatures, Maharjan et al. (2020) fed five levels of digestible Lys (dLys) from 80% to 120% of the recommended level with all other AA:dLys ratios held constant and observed quadratic responses in average daily gain and FCR up to the 120% dLys level, and no influence on FI. In contrast, the optimal average daily gain and FCR were closer to the 100% recommendation of dLys under thermoneutral conditions. This indicates a potential increase in overall AA requirements under HS, although the authors concluded that the requirement of AA/Mcal was not different in hot or thermoneutral environments, which was also observed by Hruby et al. (1995). Moreover, Alhotan et al. (2021) fed an AA density ranging from 80% to 110% of breeder recommendations to broilers exposed to cyclic HS. In contrast with the results reported by Maharjan et al. (2020), no interactions between environmental temperature and dietary AA density were observed on performance and processing data. However, linear effects of AA density indicated that BWG, feed efficiency, and breast muscle yield responded to increased AA density in both environments. Even though FCR was numerically improved by 10 points with the 110% AA diet relative to the 100% AA diet, this difference was not statistically significant and may indicate that higher AA levels were above the bird’s requirements. In another trial, increasing the density of Met, Lys, and Thr in a reduced CP diet increased production performance of cyclically heat-stressed broilers over the ones obtained with standard CP diet and, in addition, improved intestinal health as indicated by changes in small intestinal morphology and increased mRNA expression of some tight junction proteins (Wang et al., 2022). Therefore, increasing AA density could be beneficial for broilers experiencing HS, especially when achieved with free AA to minimize diet-induced thermogenesis. However, further research is required to better characterize the true AA requirements of birds under HS conditions.
Individual amino acid supplementation
Methionine (Met) is the first limiting AA in avian species and is considered, along with cysteine (Cys), to meet total sulfur AA (TSAA) needs for the bird. Because of its importance in maintenance functions and muscle deposition that are greatly impacted during exposure to HS, defining Met requirements is an important step in optimizing poultry nutrition under HS conditions. Indeed, higher requirements of Met have been found in broilers under high temperatures compared to thermoneutral conditions (Silva Junior et al., 2006; Sahebi-Ala et al., 2021), but this does not appear to be the case in laying hens or pullets (Bunchasak and Silapasorn, 2005; Castro et al., 2019). Several physiological mechanisms have been proposed regarding the importance of Met under HS. First, Met supplementation has been shown to increase the antioxidant capacity of broilers (Del Vesco et al., 2015b; Gasparino et al., 2018; Liu et al., 2019; Santana et al., 2021). Under thermoneutral conditions, the production of reactive oxygen species and the antioxidant systems in chickens are balanced and can adapt to overcome normal challenge. Acute and chronic HS disturb this equilibrium due to an overproduction of reactive oxygen species, which ultimately surpasses the antioxidant capacity and leads to oxidative stress (Lin et al., 2000, 2006a; Azad et al., 2010; Akbarian et al., 2016). Furthermore, Met supplementation affected the inflammation-related gene expression in the liver of broilers placed under high temperature (Liu et al., 2019). Another potential benefit of Met supplementation under HS is its stimulatory effect on protein deposition and inhibition of protein breakdown as indicated by the increased expression of protein synthesis-related genes IGF1, GHR and PI3KR1 in the liver, and decreased expression of protein degradation-related genes atrogin1 and CTSL2 in the breast (Del Vesco et al., 2013; 2015a).
Beneficial effects of increasing the dietary amount of essential AA other than Met are not as well defined. Dietary levels of Lys, the second limiting AA in broiler chicken diets based on corn and soybean meal (Ishii et al., 2019), are closely associated with muscle protein deposition. However, the growth depression under HS does not seem to be ameliorated by supplementing broiler diets with Lys above the thermoneutral requirements (Mendes et al., 1997; Corzo et al., 2003; Attia et al., 2011). Interestingly, when Lys was supplemented in combination with Met in a reduced CP diet, broilers had similar performance and carcass characteristics to those fed a higher CP diet under hot climate conditions (Attia et al., 2020). In this study, additional treatments with supplementation of other essential AA besides Met and Lys did not ameliorate performance reductions caused by HS, emphasizing the potential importance of those two AA under HS conditions.
Threonine is almost invariably the third limiting AA in poultry diets (Kidd, 2000). In broilers, the earliest studies on Thr supplementation above the estimated requirements for birds under hot temperatures showed no or minimal benefits on performance (Dozier et al., 2000; Kidd et al., 2000; Ojano-Dirain and Waldroup, 2002; Shan et al., 2003), whereas more recent studies have shown some performance improvements (Debnath et al., 2019; Miah et al., 2022). In laying hens, increasing the supplementation of dietary Thr to 0.66% instead of 0.43% did not improve performance outcomes, but it decreased HSP70 in the ileum (Azzam et al., 2019) and increased SOD concentration in both serum and liver (Azzam et al., 2012), indicating potential antioxidant effects of Thr under HS condition.
Unlike mammals, poultry are highly dependent on dietary Arg supply because of less active de novo Arg synthesis pathways in birds (Klose et al., 1938; Tamir and Ratner, 1963; Castro and Kim, 2020). In broilers, the determination of Arg requirements under HS conditions have led to inconsistent results among different age periods. Over-supplementation was detrimental from 1 to 3 weeks of age (Chamruspollert et al., 2004), neutral from 3 to 6 weeks of age (Mendes et al., 1997), and beneficial from 6 to 8 weeks of age (Brake, 1998). Arg supplementation also improved FCR of Pekin ducks exposed to cyclic HS (Zhu et al., 2014) and enhanced several welfare indicators and decreased corticosterone plasma concentration in laying hens during the hot summer period (Bozakova et al., 2015). Furthermore, increasing dietary Arg improved performance, reproduction, antioxidant status, immunity, and maternal antibody transmission in quails (Kalvandi et al., 2022). The ability of Arg to reduce physiological stress is likely to be attributed to its antioxidative effects (Gupta et al., 2005). Arg is also the only nitrogen donor in the production of nitric oxide, which is involved in vasodilatation to potentially aid thermoregulation of heat-stressed birds (Uyanga et al., 2021). Interestingly, more focus is being placed on the potential beneficial effects of citrulline (Cit), a compound synthetized during Arg catabolism and the formation of nitric oxide. Recent studies have shown that Cit supplementation can effectively increase systemic Arg levels, even more than direct L-Arg supplementation (Morita et al., 2014; Agarwal et al., 2017). Cit concentration in blood has also been shown to be modulated by hot temperatures (Chowdhury et al., 2014; Chowdhury, 2019) and its supplementation may increase nitric oxide synthesis, provide an anti-inflammatory response, and enhance the central regulation of body temperature (Chowdhury et al., 2017; Uyanga et al., 2021, 2022).
Leu, Ile, and Val are three essential AA collectively known as branched-chain AA (BCAA). Their roles are diverse and include effects on performance, immunity, and intestinal health. They also serve as signaling molecules in the regulation of glucose, lipid, and protein synthesis (Kim et al., 2022). Kop-Bozbay et al. (2021) investigated the effect of increased BCAA density under HS conditions and did not observe any improvement in growth performance. These authors also tested various dietary Val concentrations and did not observe effects on performance. However, high incorporation of Leu in those diets might have triggered the antagonist effect among BCAA (Ospina-Rojas et al., 2020). Interestingly, in ovo Leu injection improved BWG and thermotolerance of birds during subsequent exposure to HS (Han et al., 2017, 2019, 2020; Chowdhury et al., 2021). With the current increasing availability of feed-grade Val and Ile, further work is needed to define the potential for BCAA to combat HS in poultry.
Trp is an essential AA in poultry diets due to its need for protein synthesis, as well as serotonin and niacin production (Le Floc’h et al., 2011). Few studies have been published on the requirements of Trp under HS conditions, although high dietary concentrations did not improve performance in broilers (Tabiri et al., 2002; Shan et al., 2003; Badakhshan et al., 2021) or layers (Dong et al., 2012). However, Trp supplementation did decrease rectal temperature and abated corticosterone responses caused by HS in broilers (Badakhshan et al., 2021). Trp supplementation also increased eggshell quality and decreased SOD serum concentration in laying hens during HS (Dong et al., 2012). To our knowledge, no studies on the effect of dietary supplementation of less-limiting essential AA beyond Trp, such as His and Phe, have been conducted in poultry subjected to HS.
The remaining AA are non-essential AA and can be synthesized from other precursors. Besides altering essential AA needs, the reduced FI caused by HS limits the amount of nitrogen consumed by birds, which could potentially lead to a lack of sufficient nitrogen quantity for non-essential AA synthesis (Awad et al., 2014). Feeding low CP diets during hot temperatures could also worsen this nitrogen deficiency. Birds fed a diet with increased essential and non-essential AA concentrations under HS had a better performance than when a diet with only increased essential AA concentrations was fed (Awad et al., 2014, 2015). However, when comparing individual supplementation of several non-essential AA in low CP diets, only Gly improved broiler FCR under both normal and acute HS conditions (Awad et al., 2015, 2018). Recent research also suggests that Gly and Ser, which are normally evaluated together as Gly equivalents, are co-limiting or limiting before some BCAA in low CP diets under thermoneutral conditions (Chrystal et al., 2020; Maynard et al., 2022), which could make Gly equivalents important AA to consider during reduced FI caused by HS.
Therefore, for the essential AA, it seems possible that supplementation of Met and potentially Arg above current requirements could be beneficial under HS condition. However, further research is required to elucidate the effects of other essential AA, as well as Gly, non-essential AA, and overall nitrogen supply.
Conclusion
Adaptating to rising global temperatures while maintaining production efficiency is an important emerging challenge for the poultry industry. Under hot temperatures, birds reduce their FI to lower HP, and this is the main factor explaining the degradation of bird performance (Figure 3). Mitigation of those negative effects requires a holistic approach, and adjusting feeding practices and nutritional programs have a critical role to play. Even though some feeding strategies are difficult to implement in the field, especially with intensive rearing systems, several practices discussed in this review have shown beneficial effects to reduce the heat load on poultry. Increasing dietary lipid concentration and maintaining a standard CP level are also recommended to compensate for the FI reduction and better fit the birds’ requirements under elevated temperatures. Considering an increase in the density of some AA, like methionine and arginine, to meet the increased AA requirements for maintenance functions could also be advantageous. Therefore, further research is required to characterize nutrient partitioning and requirements of birds under HS conditions to ensure efficient and cost-effective solutions for the poultry industry.
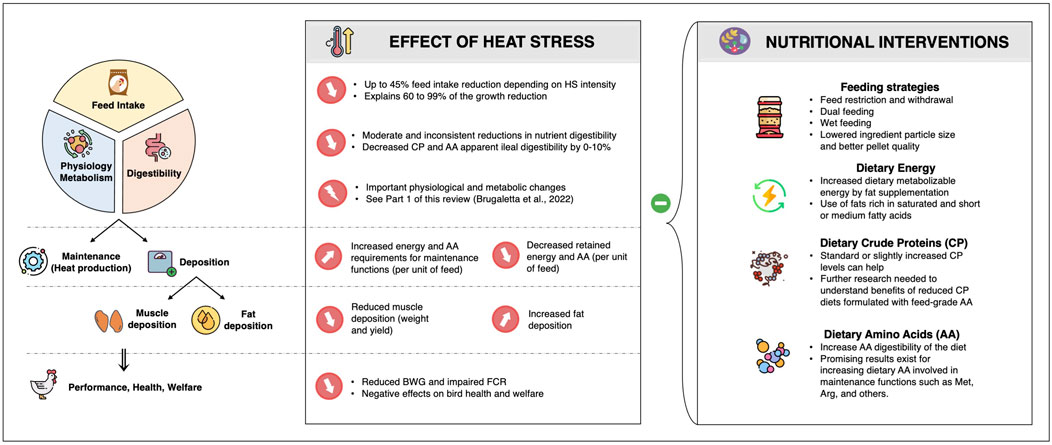
FIGURE 3. Conclusive scheme of the beneficial nutritional interventions on broilers exposed to heat stress conditions.
Author contributions
JRT and SR conceived this review section (Part II) with input from GB and FS. JRT wrote Part II of the review under the supervision of SR. GB, FS, SD, and SR revised this manuscript. All authors read and approved the submitted version of this manuscript.
Funding
The authors appreciate funding from Adisseo to support a graduate research assistantship at the University of Arkansas for JRT.
Conflict of interest
The authors declare that the research was conducted in the absence of any commercial or financial relationships that could be construed as a potential conflict of interest.
Publisher’s note
All claims expressed in this article are solely those of the authors and do not necessarily represent those of their affiliated organizations, or those of the publisher, the editors and the reviewers. Any product that may be evaluated in this article, or claim that may be made by its manufacturer, is not guaranteed or endorsed by the publisher.
Abbreviation
AA, amino acid; AME, apparent metabolizable energy; AMEn, nitrogen-corrected apparent metabolizable energy; BCAA, branched-chain AA; BW, body weight; BWG, body weight gain; Cit, citrulline; CP, crude protein; DM, dry matter; dLys, digestible Lysine; FCR, feed conversion ratio; FI, feed intake; HP, heat production; HS, heat stress; ME, metabolizable energy; TSAA, total sulfur AA.
References
Aardsma M. P., Mitchell R. D., Parsons C. M. (2017). Relative metabolizable energy values for fats and oils in young broilers and adult roosters. Poult. Sci. 96, 2320–2329. doi:10.3382/ps/pex028
Abdel-Moneim A. M. E., Shehata A. M., Khidr R. E., Paswan V. K., Ibrahim N. S., El-Ghoul A. A., et al. (2021). Nutritional manipulation to combat heat stress in poultry – a comprehensive review. J. Therm. Biol. 98, 102915. doi:10.1016/j.jtherbio.2021.102915
Abdelli N., Ramser A., Greene E. S., Beer L., Tabler T. W., Orlowski S. K., et al. (2021). Effects of cyclic chronic heat stress on the expression of nutrient transporters in the jejunum of modern broilers and their ancestor wild jungle fowl. Front. Physiol. 12, 733134. doi:10.3389/fphys.2021.733134
Abdollahi M. R., Ravindran V., Wester T. J., Ravindran G., Thomas D. V. (2011). Influence of feed form and conditioning temperature on performance, apparent metabolisable energy and ileal digestibility of starch and nitrogen in broiler starters fed wheat-based diet. Anim. Feed Sci. Technol. 168, 88–99. doi:10.1016/j.anifeedsci.2011.03.014
Abu-Dieyeh Z. H. M. (2006). Effect of chronic heat stress and long-term feed restriction on broiler performance. Int. J. Poult. Sci. 5, 185–190. doi:10.3923/ijps.2006.185.190
Agarwal U., Didelija I. C., Yuan Y., Wang X., Marini J. C. (2017). Supplemental citrulline is more efficient than arginine in increasing systemic arginine availability in mice. J. Nutr. 147, 596–602. doi:10.3945/jn.116.240382
Akbarian A., Michiels J., Degroote J., Majdeddin M., Golian A., De Smet S. (2016). Association between heat stress and oxidative stress in poultry; mitochondrial dysfunction and dietary interventions with phytochemicals. J. Anim. Sci. Biotechnol. 7, 37. doi:10.1186/s40104-016-0097-5
Al-Harthi M. A., El-Deek A. A., Al-Harbi B. L. (2002). Interrelation ships among triiodothyronine (T3), energy and sex on nutritional and physiological responses of heat stressed broilers. Egypt. Poult. Sci. 22, 349–385.
Al-Zghoul M. B., Alliftawi A. R. S., Saleh K. M. M., Jaradat Z. W. (2019). Expression of digestive enzyme and intestinal transporter genes during chronic heat stress in the thermally manipulated broiler chicken. Poult. Sci. 98, 4113–4122. doi:10.3382/ps/pez249
Alhotan R. A., Al-Sagan A. A., Al-Abdullatif A. A., Hussein E. O. S., Saadeldin I. M., Azzam M. M., et al. (2021). Interactive effects of dietary amino acid density and environmental temperature on growth performance and expression of selected amino acid transporters, water channels, and stress-related transcripts. Poult. Sci. 100, 101333. doi:10.1016/j.psj.2021.101333
Alleman F., Leclercq B. (1997). Effect of dietary protein and environmental temperature on growth performance and water consumption of male broiler chickens. Br. Poult. Sci. 38, 607–610. doi:10.1080/00071669708418044
Almirall M., Cos R., Esteve‐Garcia E., Brufau J. (1997). Effect of inclusion of sugar beet pulp, pelleting and season on laying hen performance. Br. Poult. Sci. 38, 530–536. doi:10.1080/00071669708418033
Amerah A. M., Ravindran V., Lentle R. G., Thomas D. G. (2008). Influence of feed particle size on the performance, energy utilization, digestive tract development, and digesta parameters of broiler starters fed wheat- and corn-based diets. Poult. Sci. 87, 2320–2328. doi:10.3382/ps.2008-00149
Amiri M., Ghasemi H. A., Hajkhodadadi I., Farahani A. H. K. (2019). Efficacy of guanidinoacetic acid at different dietary crude protein levels on growth performance, stress indicators, antioxidant status, and intestinal morphology in broiler chickens subjected to cyclic heat stressficacy of guanidinoacetic acid at different dietary crude protein levels on growth performance, stress indicators, antioxidant status, and intestinal morphology in broiler chickens subjected to cyclic heat stress. Anim. Feed Sci. Technol. 254, 114208. doi:10.1016/j.anifeedsci.2019.114208
Attia Y. A., Abd El-Hamid A. E.-H. E., Abedalla A. A., Berika M. A., Al-Harthi M. A., Kucuk O., et al. (2016). Laying performance, digestibility and plasma hormones in laying hens exposed to chronic heat stress as affected by betaine, vitamin C, and/or vitamin E supplementation. SpringerPlus 5, 1619. doi:10.1186/s40064-016-3304-0
Attia Y. A., Al-Harthi M. A., El-Shafey A. S., Rehab Y. A., Kim W. K. (2017). Enhancing tolerance of broiler chickens to heat stress by supplementation with vitamin E, vitamin C and/or probiotics. Ann. Anim. Sci. 17, 1155–1169. doi:10.1515/aoas-2017-0012
Attia Y. A., Al-Harthi M. A., Elnaggar Sh.A. (2018). Productive, physiological and immunological responses of two broiler strains fed different dietary regimens and exposed to heat stress. Ital. J. Anim. Sci. 17, 686–697. doi:10.1080/1828051X.2017.1416961
Attia Y. A., Böhmer B. M., Roth-Maier D. A. (2006). Responses of broiler chicks raised under constant relatively high am-bient temperature to enzymes, amino acid supplementations, or a high-nutrient diet. Arch. für Geflugelkd. 70.
Attia Y. A., Bovera F., Wang J., Al-Harthi M. A., Kim W. K. (2020). Multiple amino acid supplementations to low-protein diets: Effect on performance, carcass yield, meat quality and nitrogen excretion of finishing broilers under hot climate conditions. Animals. 10, 973. doi:10.3390/ani10060973
Attia Y. A., Hassan R. A., Tag El-Din A. E., Abou-Shehema B. M. (2011). Effect of ascorbic acid or increasing metabolizable energy level with or without supplementation of some essential amino acids on productive and physiological traits of slow-growing chicks exposed to chronic heat stress. J. Anim. Physiol. Anim. Nutr. 95, 744–755. doi:10.1111/j.1439-0396.2010.01104.x
Attia Y. A., Hassan S. S. (2017). Broiler tolerance to heat stress at various dietary protein/energy levels. Eur. Poult. Sci. 81, 1–15. doi:10.1399/eps.2017.171
Awad E. A., Fadlullah M., Zulkifli I., Farjam A. S., Chwen L. T. (2014). Amino acids fortification of low-protein diet for broilers under tropical climate: Ideal essential amino acids profile. Ital. J. Anim. Sci. 13, 3166. doi:10.4081/ijas.2014.3166
Awad E. A., Idrus Z., Soleimani Farjam A., Bello A. U., Jahromi M. F. (2018). Growth performance, duodenal morphology and the caecal microbial population in female broiler chickens fed glycine-fortified low protein diets under heat stress conditions. Br. Poult. Sci. 59, 340–348. doi:10.1080/00071668.2018.1440377
Awad E. A., Zulkifli I., Soleimani A. F., Aljuobori A. (2017). Effects of feeding male and female broiler chickens on low-protein diets fortified with different dietary glycine levels under the hot and humid tropical climate. Ital. J. Anim. Sci. 16, 453–461. doi:10.1080/1828051X.2017.1291288
Awad E. A., Zulkifli I., Soleimani A. F., Loh T. C. (2015). Individual non-essential amino acids fortification of a low-protein diet for broilers under the hot and humid tropical climate. Poult. Sci. 94, 2772–2777. doi:10.3382/ps/pev258
Awojobi H. A., Oluwole B. O., Adekunmisi A. A., Buraimo R. A. (2009). Performance of finisher broilers fed wet mash with or without drinking water during wet season in the tropics. Int. J. Poult. Sci. 8, 592–594. doi:10.3923/ijps.2009.592.594
Azad M. A. K., Kikusato M., Maekawa T., Shirakawa H., Toyomizu M. (2010). Metabolic characteristics and oxidative damage to skeletal muscle in broiler chickens exposed to chronic heat stress. Comp. Biochem. Physiol. A Mol. Integr. Physiol. 155, 401–406. doi:10.1016/j.cbpa.2009.12.011
Azzam M. M., Alhotan R., Al-Abdullatif A., Al-Mufarrej S., Mabkhot M., Alhidary I. A., et al. (2019). Threonine requirements in dietary low crude protein for laying hens under high-temperature environmental climate. Animals. 9, 586. doi:10.3390/ani9090586
Azzam M. M. M., Dong X. Y., Xie P., Zou X. T. (2012). Influence of L–threonine supplementation on goblet cell numbers, histological structure and antioxidant enzyme activities of laying hens reared in a hot and humid climate. Br. Poult. Sci. 53, 640–645. doi:10.1080/00071668.2012.726707
Badakhshan Y., Emadi L., Esmaeili-Mahani S., Nazifi S. (2021). The effect of L-tryptophan on the food intake, rectal temperature, and blood metabolic parameters of 7-day-old chicks during feeding, fasting, and acute heat stress. Iran. J. Vet. Res. 22, 55–64. doi:10.22099/ijvr.2020.37266.5428
Barboza P. S., Parker K. L., and Hume I. D. (Editors) (2009). “Metabolic constituents: Water, minerals and vitamins,” Integrative wildlife nutrition (Berlin, Heidelberg: Springer Berlin Heidelberg), 157–206. doi:10.1007/978-3-540-87885-8_9
Baziz H. A., Geraert P. A., Padilha J. C. F., Guillaumin S. (1996). Chronic heat exposure enhances fat deposition and modifies muscle and fat partition in broiler carcasses. Poult. Sci. 75, 505–513. doi:10.3382/ps.0750505
Bonnet S., Geraert P. A., Lessire M., Carré B., Guillaumin S. (1997). Effect of high ambient temperature on feed digestibility in broilers. Poult. Sci. 76, 857–863. doi:10.1093/ps/76.6.857
Bozakova N., Sotirov L., Sasakova N., Veszelits Lakticova K. (2015). Welfare improvement in laying hens during the hot period under a semi-open rearing system through dietary arginine and vitamin C supplementation. Bulg. J. Vet. Med. 18, 216–226. doi:10.15547/bjvm.869
Brake J., Balnave D., Dibner J. J. (1998). Optimum dietary arginine: Lysine ratio for broiler chickens is altered during heat stress in association with changes in intestinal uptake and dietary sodium chloride. Br. Poult. Sci. 39, 639–647. doi:10.1080/00071669888511
Brugaletta G., Teyssier J. R., Rochell S. J., Dridi S., Sirri F. (2022). A review of heat stress in chickens. Part I: Insight into gut health and physiology. SUBMITTED FOR PEER-REVIEW IN 2022. Front. Physiol.
Bruno L. D. G., Maiorka A., Macari M., Furlan R. L., Givisiez P. E. N. (2011). Water intake behavior of broiler chickens exposed to heat stress and drinking from bell or and nipple drinkers. Rev. Bras. Cienc. Avic. 13, 147–152. doi:10.1590/s1516-635x2011000200009
Bunchasak C., Silapasorn T. (2005). Effects of adding methionine in low-protein diet on production performance, reproductive organs and chemical liver composition of laying hens under tropical conditions. Int. J. Poult. Sci. 4, 301–308. doi:10.3923/ijps.2005.301.308
Buyse J., Decuypere E., Berghman L., Kuhn E. R., Vandesande F. (1992). Effect of dietary protein content on episodic growth hormone secretion and on heat production of male broiler chickens. Br. Poult. Sci. 33, 1101–1109. doi:10.1080/00071669208417552
Cardoso D. M., Cardeal P. C., Soares K. R., Sousa L. S., Castro F. L. S., Araújo I. C. S., et al. (2022). Feed form and nutritional level for rearing growing broilers in thermoneutral or heat stress environments. J. Therm. Biol. 103, 103159. doi:10.1016/j.jtherbio.2021.103159
Carew L. B., Alster F. A., Foss D. C., Scanes C. G. (1983). Effect of a tryptophan deficiency on thyroid gland, growth hormone and testicular functions in chickens. J. Nutr. 113, 1756–1765. doi:10.1093/jn/113.9.1756
Carew L. B., Evarts K. G., Alster F. A. (1997). Growth and plasma thyroid hormone concentrations of chicks fed diets deficient in essential amino acids. Poult. Sci. 76, 1398–1404. doi:10.1093/ps/76.10.1398
Castro F. L. S., Kim H. Y., Hong Y. G., Kim W. K. (2019). The effect of total sulfur amino acid levels on growth performance, egg quality, and bone metabolism in laying hens subjected to high environmental temperature. Poult. Sci. 98, 4982–4993. doi:10.3382/ps/pez275
Castro F. L. S., Kim W. K. (2020). Secondary functions of arginine and sulfur amino acids in poultry health: Review. Animals. 10, 2106. doi:10.3390/ani10112106
Chamruspollert M., Pesti G. M., Bakalli R. I. (2004). Influence of temperature on the arginine and methionine requirements of young broiler chicks. J. Appl. Poult. Res. 13, 628–638. doi:10.1093/japr/13.4.628
Chegini S., Kiani A., Rokni H. (2018). Alleviation of thermal and overcrowding stress in finishing broilers by dietary propolis supplementation. Ital. J. Anim. Sci. 17, 377–385. doi:10.1080/1828051X.2017.1360753
Cheng T. K., Hamre M. L., Coon C. N. (1999). Effect of constant and cyclic environmental temperatures, dietary protein, and amino acid levels on broiler performance. J. Appl. Poult. Res. 8, 426–439. doi:10.1093/japr/8.4.426
Chowdhury V. S., Tomonaga S., Ikegami T., Erwan E., Ito K., Cockrem J. F., et al. (2014). Oxidative damage and brain concentrations of free amino acid in chicks exposed to high ambient temperature. Comp. Biochem. Physiol. A Mol. Integr. Physiol. 169, 70–76. doi:10.1016/j.cbpa.2013.12.020
Chowdhury V. S., Han G., Bahry M. A., Tran P. V., Do P. H., Yang H., et al. (2017). L-Citrulline acts as potential hypothermic agent to afford thermotolerance in chicks. J. Therm. Biol. 69, 163–170. doi:10.1016/j.jtherbio.2017.07.007
Chowdhury V. S., Han G., Eltahan H. M., Haraguchi S., Gilbert E. R., Cline M. A., et al. (2021). Potential role of amino acids in the adaptation of chicks and market-age broilers to heat stress. Front. Vet. Sci. 7, 610541. doi:10.3389/fvets.2020.610541
Chowdhury V. S. (2019). Heat Stress biomarker amino acids and neuropeptide afford thermotolerance in chicks. J. Poult. Sci. 56, 1–11. doi:10.2141/jpsa.0180024
Chrystal P. V., Moss A. F., Yin D., Khoddami A., Naranjo V. D., Selle P. H., et al. (2020). Glycine equivalent and threonine inclusions in reduced-crude protein, maize-based diets impact on growth performance, fat deposition, starch-protein digestive dynamics and amino acid metabolism in broiler chickens. Anim. Feed Sci. Technol. 261, 114387. doi:10.1016/j.anifeedsci.2019.114387
Collin A., Buyse J., van As P., Darras V. M., Malheiros R. D., Moraes V. M. B., et al. (2003). Cold-induced enhancement of avian uncoupling protein expression, heat production, and triiodothyronine concentrations in broiler chicks. Gen. Comp. Endocrinol. 130, 70–77. doi:10.1016/S0016-6480(02)00571-3
Corzo A., Moran E. T., Hoehler D. (2003). Lysine needs of summer-reared male broilers from six to eight weeks of age. Poult. Sci. 82, 1602–1607. doi:10.1093/ps/82.10.1602
Costa-Pinto R., Gantner D. (2020). Macronutrients, minerals, vitamins and energy. Anaesth. Intensive Care Med. 21, 157–161. doi:10.1016/j.mpaic.2019.12.006
Dale N. M., Fuller H. L. (1980). Effect of diet composition on feed intake and growth of chicks under heat stress. II. Constant vs. cycling temperatures. Poult. Sci. 59, 1434–1441. doi:10.3382/ps.0591434
Dale N. M., Fuller H. L. (1979). Effects of diet composition on feed intake and growth of chicks under heat stress. Poult. Sci. 58, 1529–1534. doi:10.3382/ps.0581529
De Antonio J., Fernandez-Alarcon M. F., Lunedo R., Squassoni G. H., Ferraz A. L. J., Macari M., et al. (2017). Chronic heat stress and feed restriction affects carcass composition and the expression of genes involved in the control of fat deposition in broilers. J. Agric. Sci. 155, 1487–1496. doi:10.1017/S0021859617000624
De Basilio V., Vilariño M., Yahav S., Picard M. (2001). Early age thermal conditioning and a dual feeding program for male broilers challenged by heat stress. Poult. Sci. 80, 29–36. doi:10.1093/ps/80.1.29
De Souza J. B. F., De Morais Oliveira V. R., De Arruda A. M. V., De Melo Silva A., De Macedo Costa L. L. (2015). The relationship between corn particle size and thermoregulation of laying hens in an equatorial semi-arid environment. Int. J. Biometeorol. 59, 121–125. doi:10.1007/s00484-014-0827-3
De Souza L. F. A., Espinha L. P., De Almeida E. A., Lunedo R., Furlan R. L., Macari M. (2016). How heat stress (continuous or cyclical) interferes with nutrient digestibility, energy and nitrogen balances and performance in broilers. Livest. Sci. 192, 39–43. doi:10.1016/j.livsci.2016.08.014
Debnath B. C., Biswas P., Roy B. (2019). The effects of supplemental threonine on performance, carcass characteristics, immune response and gut health of broilers in subtropics during pre starter and starter period. J. Anim. Physiol. Anim. Nutr. 103, 29–40. doi:10.1111/jpn.12991
Dei H. K., Bumbie G. Z. (2011). Effect of wet feeding on growth performance of broiler chickens in a hot climate. Br. Poult. Sci. 52, 82–85. doi:10.1080/00071668.2010.540230
Del Vesco A. P., Gasparino E., Grieser D. O., Zancanela V., Voltolini D. M., Khatlab A. S., et al. (2015a). Effects of methionine supplementation on the expression of protein deposition-related genes in acute heat stress-exposed broilers. PLoS ONE 10, e0115821. doi:10.1371/journal.pone.0115821
Del Vesco A. P., Gasparino E., Grieser D., Zancanela V., Soares M. A. M., De Oliveira Neto A. R. (2015b). Effects of methionine supplementation on the expression of oxidative stress-related genes in acute heat stress-exposed broilers. Br. J. Nutr. 113, 549–559. doi:10.1017/S0007114514003535
Del Vesco A. P., Gasparino E., Oliveira Neto A. R., Guimarães S. E. F., Marcato S. M. M., Voltolini D. M. (2013). Dietary methionine effects on IGF-I and GHR mRNA expression in broilers. Genet. Mol. Res. 12, 6414–6423. doi:10.4238/2013.December.10.2
Dong X. Y., Azzam M. M. M., Rao W., Yu D. Y., Zou X. T. (2012). Evaluating the impact of excess dietary tryptophan on laying performance and immune function of laying hens reared under hot and humid summer conditions. Br. Poult. Sci. 53, 491–496. doi:10.1080/00071668.2012.719149
Dozier W. A., Moran E. T., Kidd M. T. (2000). Threonine requirement of broiler males from 42 to 56 days in a summer environment. J. Appl. Poult. Res. 9, 496–500. doi:10.1093/japr/9.4.496
Emami N. K., Greene E. S., Kogut M. H., Dridi S. (2021). Heat stress and feed restriction distinctly affect performance, carcass and meat yield, intestinal integrity, and inflammatory (chemo) cytokines in broiler chickens. Front. Physiol. 12, 707757. doi:10.3389/fphys.2021.707757
Emami N. K., Jung U., Voy B., Dridi S. (2020). Radical response: Effects of heat stress-induced oxidative stress on lipid metabolism in the avian liver. Antioxidants 10, 35. doi:10.3390/antiox10010035
FAO (2022). Data from: Crops and livestock products dataset. License: CC BY-NC-SA 3.0 igo. Available at: http://www.fao.org/faostat/en/#data/QC (Accessed April 01, 2022).
Faria Filho D. E., Campos D. M. B., Torres K. A., Vieira B. S., Rosa P. S., Vaz A. M., et al. (2007). Protein levels for heat-exposed broilers: Performance, nutrients digestibility, and energy and protein metabolism. Int. J. Poult. Sci. 6, 187–194. doi:10.3923/ijps.2007.187.194
Faria Filho D. E., Rosa P. S., Vieira B. S., Macari M., Furlan R. L. (2005). Protein levels and environmental temperature effects on carcass characteristics, performance, and nitrogen excretion of broiler chickens from 7 to 21 days of age. Rev. Bras. Cienc. Avic. 7, 247–253. doi:10.1590/S1516-635X2005000400009
Flees J., Rajaei-Sharifabadi H., Greene E., Beer L., Hargis B. M., Ellestad L., et al. (2017). Effect of Morinda citrifolia (noni)-enriched diet on hepatic heat shock protein and lipid metabolism-related genes in heat stressed broiler chickens. Front. Physiol. 8, 919. doi:10.3389/fphys.2017.00919
Fondevila G., Archs J. L., Cámara L., de Juan A. F., Mateos G. G. (2020). The length of the feed restriction period affects eating behavior, growth performance, and the development of the proximal part of the gastrointestinal tract of young broilers. Poult. Sci. 99, 1010–1018. doi:10.1016/j.psj.2019.10.011
Fouad A. M., Chen W., Ruan D., Wang S., Xia W. G., Zheng C. T., et al. (2016). Impact of heat stress on meat, egg quality, immunity and fertility in poultry and nutritional factors that overcome these effects: A review. Int. J. Poult. Sci. 15, 81–95. doi:10.3923/ijps.2016.81.95
Fuller H. L., Rendon M. (1977). Energetic efficiency of different dietary fats for growth of young chicks. Poult. Sci. 56, 549–557. doi:10.3382/ps.0560549
Furlan R. L., Faria Filho D. E., Rosa P. S., Macari M. (2004). Does low-protein diet improve broiler performance under heat stress conditions? Rev. Bras. Cienc. Avic. 6, 71–79. doi:10.1590/S1516-635X2004000200001
Gadzirayi C. T., Mutandwa E., Chihiya J., Mlambo R. (2006). A comparative economic analysis of mash and pelleted feed in broiler production under deep litter housing system. Int. J. Poult. Sci. 5, 629–631. doi:10.3923/ijps.2006.629.631
Garriga C., Hunter R. R., Amat C., Planas J. M., Mitchell M. A., Moretó M., et al. (2006). Heat stress increases apical glucose transport in the chicken jejunum. Am. J. Physiol. Regul. Integr. Comp. Physiol. 290, R195–R201. doi:10.1152/ajpregu.00393.2005
Gasparino E., Del Vesco A. P., Khatlab A. S., Zancanela V., Grieser D. O., Silva S. C. C., et al. (2018). Effects of methionine hydroxy analogue supplementation on the expression of antioxidant-related genes of acute heat stress-exposed broilers. Animal 12, 931–939. doi:10.1017/S1751731117002439
Geraert P. A. (1991). Métabolisme énergétique du poulet de chair en climat chaud. INRA. Prod. Anim. 4, 257–267. doi:10.20870/productions-animales.1991.4.3.4340
Geraert P. A., Guillaumin S., Zuprizal (1992). Research note: Effect of high ambient temperature on dietary metabolizable energy values in genetically lean and fat chickens. Poult. Sci. 71, 2113–2116. doi:10.3382/ps.0712113
Geraert P. A., Padilha J. C. F., Guillaumin S. (1996). Metabolic and endocrine changes induced by chronic heatexposure in broiler chickens: Growth performance, body composition and energy retention. Br. J. Nutr. 75, 195–204. doi:10.1079/bjn19960124
Ghazalah A. A., Abd-Elsamee M. O., Ali A. M. (2008). Influence of dietary energy and poultry fat on the response of broiler chicks to heat therm. Int. J. Poult. Sci. 7, 355–359. doi:10.3923/ijps.2008.355.359
Goel A., Ncho C. M., Choi Y. H. (2021). Regulation of gene expression in chickens by heat stress. J. Anim. Sci. Biotechnol. 12, 11. doi:10.1186/s40104-020-00523-5
Gonzalez-Esquerra R., Leeson S. (2006). Physiological and metabolic responses of broilers to heat stress - implications for protein and amino acid nutrition. World's. Poult. Sci. J. 62, 282–295. doi:10.1079/WPS200597
Gonzalez-Esquerra R., Leeson S. (2005). Effects of acute versus chronic heat stress on broiler response to dietary protein. Poult. Sci. 84, 1562–1569. doi:10.1093/ps/84.10.1562
Greene E. S., Cauble R., Kadhim H., De Almeida Mallmann B., Gu I., Lee S. O., et al. (2021). Protective effects of the phytogenic feed additive “comfort” on growth performance via modulation of hypothalamic feeding- and drinking-related neuropeptides in cyclic heat-stressed broilers. Domest. Anim. Endocrinol. 74, 106487. doi:10.1016/j.domaniend.2020.106487
Guillot E., Vaugelade P., Lemarchali P., Re Rat A. (1993). Intestinal absorption and liver uptake of medium-chain fatty acids in non-anaesthetized pigs. Br. J. Nutr. 69, 431–442. doi:10.1079/BJN19930045
Gupta V., Gupta A., Saggu S., Divekar H. M., Grover K., Kumar R. (2005). Anti-stress and adaptogenic activity of L-arginine supplementation. Evid. Based. Complement. Altern. Med. 2, 93–97. doi:10.1093/ecam/neh054
Habashy W. S., Milfort M. C., Adomako K., Attia Y. A., Rekaya R., Aggrey S. E. (2017a). Effect of heat stress on amino acid digestibility and transporters in meat-type chickens. Poult. Sci. 96, 2312–2319. doi:10.3382/ps/pex027
Habashy W. S., Milfort M. C., Fuller A. L., Attia Y. A., Rekaya R., Aggrey S. E. (2017b). Effect of heat stress on protein utilization and nutrient transporters in meat-type chickens. Int. J. Biometeorol. 61, 2111–2118. doi:10.1007/s00484-017-1414-1
Hai L., Rong D., Zhang Z. Y. (2000). The effect of thermal environment on the digestion of broilers. J. Anim. Physiol. Anim. Nutr. 83, 57–64. doi:10.1046/j.1439-0396.2000.00223.x
Han G., Ouchi Y., Hirota T., Haraguchi S., Miyazaki T., Arakawa T., et al. (2020). Effects of l-leucine in ovo feeding on thermotolerance, growth and amino acid metabolism under heat stress in broilers. Animal 14, 1701–1709. doi:10.1017/S1751731120000464
Han G., Yang H., Bahry M. A., Tran P. V., Do P. H., Ikeda H., et al. (2017). L-Leucine acts as a potential agent in reducing body temperature at hatching and affords thermotolerance in broiler chicks. Comp. Biochem. Physiol. A Mol. Integr. Physiol. 204, 48–56. doi:10.1016/j.cbpa.2016.10.013
Han G., Yang H., Wang Y., Haraguchi S., Miyazaki T., Bungo T., et al. (2019). L-Leucine increases the daily body temperature and affords thermotolerance in broiler chicks. Asian-australas. J. Anim. Sci. 32, 842–848. doi:10.5713/ajas.18.0677
He X., Lu Z., Ma B., Zhang L., Li J., Jiang Y., et al. (2019). Effects of dietary taurine supplementation on growth performance, jejunal morphology, appetite-related hormones, and genes expression in broilers subjected to chronic heat stress. Poult. Sci. 98, 2719–2728. doi:10.3382/ps/pez054
Hecker M., Sommer N., Mayer K. (2021). “Assessment of short- and medium-chain fatty acids on mitochondrial function in severe inflammation,” in Mitochondrial medicine methods in molecular biology. Editors V. Weissig, M. Edeas, and Humana (New York, NY, 125–132. doi:10.1007/978-1-0716-1270-5_8
Hosseini S. M., Afshar M. (2017a). Effect of diet form and enzyme supplementation on stress indicators and bone mineralisation in heat-challenged broilers fed wheat-soybean diet. Ital. J. Anim. Sci. 16, 616–623. doi:10.1080/1828051X.2017.1321973
Hosseini S. M., Afshar M. (2017b). Effects of feed form and xylanase supplementation on performance and ileal nutrients digestibility of heat-stressed broilers fed wheat–soybean diet. J. Appl. Anim. Res. 45, 550–556. doi:10.1080/09712119.2016.1224765
Hruby M., Hamre M. L., Coon C. N. (1995). Predicting amino acid requirements for broilers at 21.1°C and 32.2°C. J. Appl. Poult. Res. 4, 395–401. doi:10.1093/japr/4.4.395
Ishii T., Shibata K., Kai S., Noguchi K., Hendawy A. O., Fujimura S., et al. (2019). Dietary supplementation with lysine and threonine modulates the performance and plasma metabolites of broiler chicken. J. Poult. Sci. 56, 204–211. doi:10.2141/jpsa.0180104
IPCC (2021). “Climate change 2021,” in The physical science basis. Contribution of working group I to the sixth assessment report of the intergovernmental Panel on climate change [Masson-Delmotte. Editors V. Masson-Delmotte, P. Zhai, A. Pirani, S. L. Connors, C. Péan, S. Bergeret al. (Cambridge University Press).
Iyasere O. S., Bateson M., Beard A. P., Guy J. H. (2021). Provision of additional cup drinkers mildly alleviated moderate heat stress conditions in broiler chickens. J. Appl. Anim. Welf. Sci. 24, 188–199. doi:10.1080/10888705.2020.1846534
Kalvandi O., Sadeghi A., Karimi A. (2022). Arginine supplementation improves reproductive performance, antioxidant status, immunity and maternal antibody transmission in breeder Japanese quail under heat stress conditions. Ital. J. Anim. Sci. 21, 8–17. doi:10.1080/1828051X.2021.2013136
Keshavarz K., Fuller H. L. (1980). The influence of widely fluctuating temperatures on heat production and energetic efficiency of broilers. Poult. Sci. 59, 2121–2128. doi:10.3382/ps.0592121
Khoa M. A. (2007). Wet and coarse diets in broiler nutrition: Development of the GI tract and performance. Wageningen: Doctoral dissertation, Wageningen University and Research.
Kidd M. T., Kerr B. J., Allard J. P., Rao S. K., Halley J. T. (2000). Limiting amino acid responses in commercial broilers. J. Appl. Poult. Res. 9, 223–233. doi:10.1093/japr/9.2.223
Kidd M. T. (2000). Nutritional considerations concerning threonine in broilers. World's. Poult. Sci. J. 56, 139–151. doi:10.1079/WPS20000011
Kim D. H., Lee Y. K., Lee S. D., Kim S. H., Lee S. R., Lee H. G., et al. (2020). Changes in production parameters, egg qualities, fecal volatile fatty acids, nutrient digestibility, and plasma parameters in laying hens exposed to ambient temperature. Front. Vet. Sci. 7, 412. doi:10.3389/fvets.2020.00412
Kim W. K., Singh A. K., Wang J., Applegate T. (2022). Functional role of branched chain amino acids in poultry: A review. Poult. Sci. 101, 101715. doi:10.1016/j.psj.2022.101715
Klose A. A., Stokstad E. L. R., Almquist H. J. (1938). The essential nature of arginine in the diet of the chick. J. Biol. Chem. 123, 691–698. doi:10.1016/S0021-9258(18)74114-8
Kop-Bozbay C., Akdag A., Atan H., Ocak N. (2021). Response of broilers to supplementation of branched-chain amino acids blends with different valine contents in the starter period under summer conditions. Anim. Biosci. 34, 295–305. doi:10.5713/ajas.19.0828
Kumar M., Ratwan P., Dahiya S. P., Nehra A. K. (2021). Climate change and heat stress: Impact on production, reproduction and growth performance of poultry and its mitigation using genetic strategies. J. Therm. Biol. 97, 102867. doi:10.1016/j.jtherbio.2021.102867
Kutlu H. R. (2001). Influences of wet feeding and supplementation with ascorbic acid on performance and carcass composition of broiler chicks exposed to a high ambient temperature. Arch. Tierernahr. 54, 127–139. doi:10.1080/17450390109381972
Lara L. J., Rostagno M. H. (2013). Impact of heat stress on poultry production. Animals 3, 356–369. doi:10.3390/ani3020356
Latshaw J. D., Moritz J. S. (2009). The partitioning of metabolizable energy by broiler chickens. Poult. Sci. 88, 98–105. doi:10.3382/ps.2008-00161
Laudadio V., Dambrosio A., Normanno G., Khan R. U., Naz S., Rowghani E., et al. (2012). Effect of reducing dietary protein level on performance responses and some microbiological aspects of broiler chickens under summer environmental conditions. Avian Biol. Res. 5, 88–92. doi:10.3184/175815512X13350180713553
Le Floc’h N., Otten W., Merlot E. (2011). Tryptophan metabolism, from nutrition to potential therapeutic applications. Amino Acids 41, 1195–1205. doi:10.1007/s00726-010-0752-7
Lin H., Decuypere E., Buyse J. (2006a). Acute heat stress induces oxidative stress in broiler chickens. Comp. Biochem. Physiol. A Mol. Integr. Physiol. 144, 11–17. doi:10.1016/j.cbpa.2006.01.032
Lin H., Du R., Zhang Z. Y. (2000). Peroxide status in tissues of heat-stressed broilers. Asian-Australas. J. Anim. Sci. 13, 1373–1376. doi:10.5713/ajas.2000.1373
Lin H., Jiao H. C., Buyse J., Decuypere E. (2006b). Strategies for preventing heat stress in poultry. World's. Poult. Sci. J. 62, 71–86. doi:10.1079/WPS200585
Lin Law F., Idrus Z., Soleimani Farjam A., Juan Boo L., Awad E. A. (2019). Effects of protease supplementation of low protein and/or energy diets on growth performance and blood parameters in broiler chickens under heat stress condition. Ital. J. Anim. Sci. 18, 679–689. doi:10.1080/1828051X.2018.1557019
Liu G., Magnuson A. D., Sun T., Tolba S. A., Starkey C., Whelan R., et al. (2019). Supplemental methionine exerted chemical form-dependent effects on antioxidant status, inflammation-related gene expression, and fatty acid profiles of broiler chicks raised at high ambient temperature. J. Anim. Sci. 97, 4883–4894. doi:10.1093/jas/skz348
Liu L., Ren M., Ren K., Jin Y., Yan M. (2020). Heat stress impacts on broiler performance: A systematic review and meta-analysis. Poult. Sci. 99, 6205–6211. doi:10.1016/j.psj.2020.08.019
Liu Q. W., Feng J. H., Chao Z., Chen Y., Wei L. M., Wang F., et al. (2016). The influences of ambient temperature and crude protein levels on performance and serum biochemical parameters in broilers. J. Anim. Physiol. Anim. Nutr. 100, 301–308. doi:10.1111/jpn.12368
Lozano C., De Basilio V., Oliveros I., Alvarez R., Colina I., Bastianelli D., et al. (2006). Is sequential feeding a suitable technique to compensate for the negative effects of a tropical climate in finishing broilers? Anim. Res. 55, 71–76. doi:10.1051/animres:2005047
Lu Q., Wen J., Zhang H. (2007). Effect of chronic heat exposure on fat deposition and meat quality in two genetic types of chicken. Poult. Sci. 86, 1059–1064. doi:10.1093/ps/86.6.1059
Ma B., Zhang L., Li J., Xing T., Jiang Y., Gao F., et al. (2021). Heat stress alters muscle protein and amino acid metabolism and accelerates liver gluconeogenesis for energy supply in broilers. Poult. Sci. 100, 215–223. doi:10.1016/j.psj.2020.09.090
MacLeod M. G., Hocking P. M. (1993). Thermoregulation at high ambient temperature in genetically fat and lean broiler hens fed ad libitum or on a controlled‐feeding regime. Br. Poult. Sci. 34, 589–596. doi:10.1080/00071669308417614
Maharjan P., Mullenix G., Hilton K., Caldas J., Beitia A., Weil J., et al. (2020). Effect of digestible amino acids to energy ratios on performance and yield of two broiler lines housed in different grow-out environmental temperatures. Poult. Sci. 99, 6884–6898. doi:10.1016/j.psj.2020.09.019
Massuquetto A., Durau J. F., Schramm V. G., Netto M. V. T., Krabbe E. L., Maiorka A. (2018). Influence of feed form and conditioning time on pellet quality, performance and ileal nutrient digestibility in broilers. J. Appl. Poult. Res. 27, 51–58. doi:10.3382/japr/pfx039
Massuquetto A., Panisson J. C., Marx F. O., Surek D., Krabbe E. L., Maiorka A. (2019). Effect of pelleting and different feeding programs on growth performance, carcass yield, and nutrient digestibility in broiler chickens. Poult. Sci. 98, 5497–5503. doi:10.3382/ps/pez176
Mateos G. G., Sell J. L., Eastwood J. A. (1982). Rate of food passage (transit time) as influenced by level of supplemental fat. Poult. Sci. 61, 94–100. doi:10.3382/ps.0610094
Maynard C. W., Kidd M. T., Chrystal P. V., McQuade L. R., McInerney B. V., Selle P. H., et al. (2022). Assessment of limiting dietary amino acids in broiler chickens offered reduced crude protein diets. Anim. Nutr. 10, 1–11. doi:10.1016/j.aninu.2021.11.010
Mendes A. A., Watkins S. E., England J. A., Saleh E. A., Waldroup A. L., Waldroup P. W. (1997). Influence of dietary lysine levels and arginine:lysine ratios on performance of broilers exposed to heat or cold stress during the period of three to six weeks of age. Poult. Sci. 76, 472–481. doi:10.1093/ps/76.3.472
Miah M. Y., Saha S., Koiri N., Mahbub A., Islam M. A., Channarayapatna G., et al. (2022). Effects of dietary methionine and threonine on growth performance, carcass traits and blood metabolites of broilers in a hot environment. Eur. Poult. Sci. 86, 1–13. doi:10.1399/eps.2022.348
Mignon-Grasteau S., Moreri U., Narcy A., Rousseau X., Rodenburg T. B., Tixier-Boichard M., et al. (2015). Robustness to chronic heat stress in laying hens: A meta-analysis. Poult. Sci. 94, 586–600. doi:10.3382/ps/pev028
Mohamed A. S. A., Lozovskiy A. R., Ali A. M. A. (2019). Strategies to combat the deleterious impacts of heat stress through feed restrictions and dietary supplementation (vitamins, minerals) in broilers. J. Indones. Trop. Anim. Agric. 44, 155–166. doi:10.14710/jitaa.44.2.155-166
Morales A., Gómez T., Villalobos Y. D., Bernal H., Htoo J. K., González-Vega J. C., et al. (2020). Dietary protein-bound or free amino acids differently affect intestinal morphology, gene expression of amino acid transporters, and serum amino acids of pigs exposed to heat stress. J. Anim. Sci. 98, skaa056. doi:10.1093/jas/skaa056
Morita M., Hayashi T., Ochiai M., Maeda M., Yamaguchi T., Ina K., et al. (2014). Oral supplementation with a combination of L-citrulline and L-arginine rapidly increases plasma L-arginine concentration and enhances NO bioavailability. Biochem. Biophys. Res. Commun. 454, 53–57. doi:10.1016/j.bbrc.2014.10.029
Moritz J. S., Beyer R. S., Wilson K. J., Cramer K. R., Mckinney L. J., Fairchild F. J., et al. (2001). Effect of moisture addition at the mixer to a corn-soybean-based diet on broiler performance. J. Appl. Poult. Res. 10, 347–353. doi:10.1093/japr/10.4.347
Musharaf N. A., Latshaw J. D. (1999). Heat increment as affected by protein and amino acid nutrition. World's. Poult. Sci. J. 55, 233–240. doi:10.1079/WPS19990017
Naderinejad S., Zaefarian F., Abdollahi M. R., Hassanabadi A., Kermanshahi H., Ravindran V. (2016). Influence of feed form and particle size on performance, nutrient utilisation, and gastrointestinal tract development and morphometry in broiler starters fed maize-based diets. Anim. Feed Sci. Technol. 215, 92–104. doi:10.1016/j.anifeedsci.2016.02.012
Nardone A., Ronchi B., Lacetera N., Ranieri M. S., Bernabucci U. (2010). Effects of climate changes on animal production and sustainability of livestock systems. Livest. Sci. 130, 57–69. doi:10.1016/j.livsci.2010.02.011
Nawab A., Ibtisham F., Li G., Kieser B., Wu J., Liu W., et al. (2018). Heat stress in poultry production: Mitigation strategies to overcome the future challenges facing the global poultry industry. J. Therm. Biol. 78, 131–139. doi:10.1016/j.jtherbio.2018.08.010
Noblet J., Dubois S., Van Milgen J., Warpechowski M., Carre B. (2007). Heat production in broilers is not affected by dietary crude protein, 124. Wageningen: Publication-European Association for animal production, 479.
Noblet J., Van Milgen J., Carré B., Dimon P., Dubois S., Rademacher M., et al. (2003). Effect of body weight and dietary crude protein on energy utilisation in growing pigs and broilers, 109. Wageningen: Publication-European Association for animal production, 205–208.
NRC (1994). Nutrient requirements of poultry: Ninth revised edition. Washington, D.C.: National Academies Press. doi:10.17226/2114
Ojano-Dirain C. P., Waldroup P. W. (2002). Evaluation of lysine, methionine and threonine needs of broilers three to six week of age under moderate temperature stress. Int. J. Poult. Sci. 1, 16–21. doi:10.3923/ijps.2002.16.21
Okan F., Kutlu H. R., Baykal L., Canogullari S. (1996a). Effect of wet feeding on laying performance of Japanese quail maintained under high environmental temperature. Br. Poult. Sci. 37, S70.
Okan F., Kutlu H. R., Canogullari S., Baykal L. (1996b). Influence of dietary supplemental ascorbic acid on laying performance of Japanese quail reared under high environmental temperature. Br. Poult. Sci. 37, S71.
Oloyo A. (2018). The use of housing system in the management of heat stress in poultry production in hot and humid climate: A review. Poult. Sci. J. 6. doi:10.22069/psj.2018.13880.1284
Orhan C., Kucuk O., Sahin N., Tuzcu M., Sahin K. (2020). Effects of taurine supplementation on productive performance, nutrient digestibility and gene expression of nutrient transporters in quails reared under heat stress. J. Therm. Biol. 92, 102668. doi:10.1016/j.jtherbio.2020.102668
Ospina-Rojas I. C., Pozza P. C., Rodrigueiro R. J. B., Gasparino E., Khatlab A. S., Murakami A. E. (2020). High leucine levels affecting valine and isoleucine recommendations in low-protein diets for broiler chickens. Poult. Sci. 99, 5946–5959. doi:10.1016/j.psj.2020.08.053
Özkan S., Akbaş Y., Altan Ö., Altan A., Ayhan V., ÖZkan K. (2003). The effect of short-term fasting on performance traits and rectal temperature of broilers during the summer season. Br. Poult. Sci. 44, 88–95. doi:10.1080/0007166031000085292
Pawar S. S., Sajjanar B., Lonkar L. V., Kurade N. P., Kadam A. S., Nirmale A. V., et al. (2016). Assessing and mitigating the impact of heat stress in poultry. Adv. Anim. Vet. Sci. 4, 332–341. doi:10.14737/journal.aavs/2016/4.6.332.341
Plavnik I., Yahav S. (1998). Research notes: Effect of environmental temperature on broiler chickens subjected to growth restriction at an early age. Poult. Sci. 77, 870–872. doi:10.1093/ps/77.6.870
Raju M. V. L. N., Shyam Sunder G., Chawak M. M., Rama Rao S. V., Sadagopan V. R. (2004). Response of naked neck (Nana) and normal (nana) broiler chickens to dietary energy levels in a subtropical climate. Br. Poult. Sci. 45, 186–193. doi:10.1080/00071660410001715786
Renaudeau D., Collin A., Yahav S., De Basilio V., Gourdine J. L., Collier R. J. (2012). Adaptation to hot climate and strategies to alleviate heat stress in livestock production. Animal 6, 707–728. doi:10.1017/S1751731111002448
Richards S. A. (1970). Physiology of thermal panting in birds. Ann. Biol. Anim. Bioch. Biophys. 10, 151–168. doi:10.1051/rnd:19700614
Saeed M., Abbas G., Alagawany M., Kamboh A. A., Abd El-Hack M. E., Khafaga A. F., et al. (2019). Heat stress management in poultry farms: A comprehensive overview. J. Therm. Biol. 84, 414–425. doi:10.1016/j.jtherbio.2019.07.025
Sahebi-Ala F., Hassanabadi A., Golian A. (2021). Effect of replacement different methionine levels and sources with betaine on blood metabolites, breast muscle morphology and immune response in heat-stressed broiler chickens. Ital. J. Anim. Sci. 20, 33–45. doi:10.1080/1828051X.2020.1868358
Sakomura N. K., Longo F. A., Oviedo-Rondon E. O., Boa-Viagem C., Ferraudo A. (2005). Modeling energy utilization and growth parameter description for broiler chickens. Poult. Sci. 84, 1363–1369. doi:10.1093/ps/84.9.1363
Santana T. P., Gasparino E., de Sousa F. C. B., Khatlab A. S., Zancanela V., Brito C. O., et al. (2021). Effects of free and dipeptide forms of methionine supplementation on oxidative metabolism of broilers under high temperature. Animal 15, 100173. doi:10.1016/j.animal.2021.100173
Santos M. M., Souza-Junior J. B. F., Queiroz J. P. A. F., Costa M. K. O., Lima H. F. F., Arruda A. M. V., et al. (2019). Broilers’ behavioural adjustments when submitted to natural heat stress and fed different maize particle sizes in the diet. J. Agric. Sci. 157, 743–748. doi:10.1017/S0021859620000131
Schönfeld P., Wojtczak L. (2016). Short- and medium-chain fatty acids in energy metabolism: The cellular perspective. J. Lipid Res. 57, 943–954. doi:10.1194/jlr.R067629
Searchinger T., Waite R., Hanson C., Ranganathan J., Dumas P., Matthews E., et al. (2019). Creating a sustainable food future: A menu of solutions to feed nearly 10 billion people by 2050. Washington: WRI. Final report.
Seifi K., Rezaei M., Yansari A. T., Riazi G. H., Zamiri M. J., Heidari R. (2018). Saturated fatty acids may ameliorate environmental heat stress in broiler birds by affecting mitochondrial energetics and related genes. J. Therm. Biol. 78, 1–9. doi:10.1016/j.jtherbio.2018.08.018
Seifi K., Rezaei M., Yansari A. T., Zamiri M. J., Riazi G. H., Heidari R. (2020). Short chain fatty acids may improve hepatic mitochondrial energy efficiency in heat stressed-broilers. J. Therm. Biol. 89, 102520. doi:10.1016/j.jtherbio.2020.102520
Sekiz S. S., Scott M. L., Nesheim M. C. (1975). The effect of methionine deficiency on body weight, food and energy utilization in the chick. Poult. Sci. 54, 1184–1188. doi:10.3382/ps.0541184
Serrano M. P., Frikha M., Corchero J., Mateos G. G. (2013). Influence of feed form and source of soybean meal on growth performance, nutrient retention, and digestive organ size of broilers. 2. Battery study. Poult. Sci. 92, 693–708. doi:10.3382/ps.2012-02372
Shan A. S., Sterling K. G., Pesti G. M., Bakalli R. I., Driver J. P., Tejedor A. A. (2003). The influence of temperature on the threonine and tryptophan requirements of young broiler chicks. Poult. Sci. 82, 1154–1162. doi:10.1093/ps/82.7.1154
Shariatmadari F., Forbes J. M. (2005). Performance of broiler chickens given whey in the food and/or drinking water. Br. Poult. Sci. 46, 498–505. doi:10.1080/00071660500190900
Silva Junior R. G. C., Lana G. R. Q., Rabello C. B. V., Lana S. R. V., Barboza W. A. (2006). Exigências de metionina + cistina para frangos de corte fêmeas de 1 a 21 e de 22 a 42 dias de idade criados em região de clima tropical. R. Bras. Zootec. 35, 497–503. doi:10.1590/s1516-35982006000200023
Skinner-Noble D. O., McKinney L. J., Teeter R. G. (2005). Predicting effective caloric value of nonnutritive factors: III. Feed form affects broiler performance by modifying behavior patterns. Poult. Sci. 84, 403–411. doi:10.1093/ps/84.3.403
Smith R. R., Rumsey G. L., Scott M. L. (1978). Heat increment associated with dietary protein, fat, carbohydrate and complete diets in salmonids comparative energetic efficiency. J. Nutr. 108, 1025–1032. doi:10.1093/jn/108.6.1025
Soares K. R., Lara L. J. C., da Silva Martins N. R., e Silva R. R., Pereira L. F. P., Cardeal P. C., et al. (2020). Protein diets for growing broilers created under a thermoneutral environment or heat stress. Anim. Feed Sci. Technol. 259, 114332. doi:10.1016/j.anifeedsci.2019.114332
Soleimani A. F., Meimandipour A., Azhar K., Ebrahimi M., Zulkifli I. (2010). Effects of heat exposure and sex on ileal digestibility of amino acids of soybean meal in broiler chickens. Arch. Geflügelk. 74, 249–255.
Song J., Xiao K., Ke Y. L., Jiao L. F., Hu C. H., Diao Q. Y., et al. (2014). Effect of a probiotic mixture on intestinal microflora, morphology, and barrier integrity of broilers subjected to heat stress. Poult. Sci. 93, 581–588. doi:10.3382/ps.2013-03455
Song Z. H., Cheng K., Zheng X. C., Ahmad H., Zhang L. L., Wang T. (2018). Effects of dietary supplementation with enzymatically treated Artemisia annua on growth performance, intestinal morphology, digestive enzyme activities, immunity, and antioxidant capacity of heat-stressed broilers. Poult. Sci. 97, 430–437. doi:10.3382/ps/pex312
St-Pierre N. R., Cobanov B., Schnitkey G. (2003). Economic losses from heat stress by US livestock industries. J. Dairy Sci. 86, E52–E77. doi:10.3168/jds.S0022-0302(03)74040-5
Sugiharto S., Yudiarti T., Isroli I., Widiastuti E., Kusumanti E. (2017). Dietary supplementation of probiotics in poultry exposed to heat stress – A review. Ann. Anim. Sci. 17, 591–604. doi:10.1515/aoas-2016-0062
Sun X., Zhang H., Sheikhahmadi A., Wang Y., Jiao H., Lin H., et al. (2015). Effects of heat stress on the gene expression of nutrient transporters in the jejunum of broiler chickens (Gallus gallus domesticus). Int. J. Biometeorol. 59, 127–135. doi:10.1007/s00484-014-0829-1
Swennen Q., Janssens G. P. J., Decuypere E., Buyse J. (2004). Effects of substitution between fat and protein on feed intake and its regulatory mechanisms in broiler chickens: Energy and protein metabolism and diet-induced thermogenesis. Poult. Sci. 83, 1997–2004. doi:10.1093/ps/83.12.1997
Swick R. A., Wu S.-B., Zuo J., Rodgers N., Barekatain M. R., Choct M., et al. (2013). Implications and development of a net energy system for broilers. Anim. Prod. Sci. 53, 1231. doi:10.1071/AN13204
Syafwan S., Kwakkel R. P., Verstegen M. W. A. (2011). Heat stress and feeding strategies in meat-type chickens. World's. Poult. Sci. J. 67, 653–674. doi:10.1017/S0043933911000742
Syafwan S., Wermink G. J. D., Kwakkel R. P., Verstegen M. W. A. (2012). Dietary self-selection by broilers at normal and high temperature changes feed intake behavior, nutrient intake, and performance. Poult. Sci. 91, 537–549. doi:10.3382/ps.2011-01559
Tabiri H. Y., Sato K., Takahashi K., Toyomizu M., Akiba Y. (2002). Effects of heat stress and dietary tryptophan on performance and plasma amino acid concentrations of broiler chickens. Asian-Australas. J. Anim. Sci. 15, 247–253. doi:10.5713/ajas.2002.247
Tadtiyanant C., Lyons J. J., Vandepopuliere J. M. (1991). Influence of wet and dry feed on laying hens under heat stress. Poult. Sci. 70, 44–52. doi:10.3382/ps.0700044
Tamir H., Ratner S. (1963). Enzymes of arginine metabolism in chicks. Arch. Biochem. Biophys. 102, 249–258. doi:10.1016/0003-9861(63)90178-4
Teyssier J. R., Preynat A., Cozannet P., Briens M., Mauromoustakos A., Greene E. S., et al. (2022). Constant and cyclic chronic heat stress models differentially influence growth performance, carcass traits and meat quality of broilers. Poult. Sci. 10196. doi:10.1016/j.psj.2022.101963
Uyanga V. A., Liu L., Zhao J., Wang X., Jiao H., Lin H. (2022). Central and peripheral effects of L-citrulline on thermal physiology and nitric oxide regeneration in broilers. Poult. Sci. 101, 101669. doi:10.1016/j.psj.2021.101669
Uyanga V. A., Wang M., Tong T., Zhao J., Wang X., Jiao H., et al. (2021). L-Citrulline influences the body temperature, heat shock response and nitric oxide regeneration of broilers under thermoneutral and heat stress condition. Front. Physiol. 12, 671691. doi:10.3389/fphys.2021.671691
Vandana G. D., Sejian V., Lees A. M., Pragna P., Silpa M. V., Maloney S. K. (2021). Heat stress and poultry production: Impact and amelioration. Int. J. Biometeorol. 65, 163–179. doi:10.1007/s00484-020-02023-7
Wallis I. R., Balnave D. (1984). The influence of environmental temperature, age and sex on the digestibility of amino acids in growing broiler chickens. Br. Poult. Sci. 25, 401–407. doi:10.1080/00071668408454880
Wang Z., Shao D., Kang K., Wu S., Zhong G., Song Z., et al. (2022). Low protein with high amino acid diets improves the growth performance of yellow feather broilers by improving intestinal health under cyclic heat stress. J. Therm. Biol. 105, 103219. doi:10.1016/j.jtherbio.2022.103219
Wasti S., Sah N., Mishra B. (2020). Impact of heat stress on poultry health and performances, and potential mitigation strategies. Animals. 10, 1266. doi:10.3390/ani10081266
Yalçin S., Özkan S., Çabuk M., Siegel P. B. (2003). Criteria for evaluating husbandry practices to alleviate heat stress in broilers. J. Appl. Poult. Res. 12, 382–388. doi:10.1093/japr/12.3.382
Yalçin S., Özkan S., Türkmut L., Siegel P. B. (2001). Responses to heat stress in commercial and local broiler stocks. 1. Performance traits. Br. Poult. Sci. 42, 149–152. doi:10.1080/00071660120048375
Yamazaki M., Zi‐Yi Z. (1982). A note on the effect of temperature on true and apparent metabolisable energy values of a layer diet. Br. Poult. Sci. 23, 447–450. doi:10.1080/00071688208447977
Zaman Q. U., Mushtaq T., Nawaz H., Mirza M. A., Mahmood S., Ahmad T., et al. (2008). Effect of varying dietary energy and protein on broiler performance in hot climate. Anim. Feed Sci. Technol. 146, 302–312. doi:10.1016/j.anifeedsci.2008.01.006
Zeferino C. P., Komiyama C. M., Pelícia V. C., Fascina V. B., Aoyagi M. M., Coutinho L. L., et al. (2016). Carcass and meat quality traits of chickens fed diets concurrently supplemented with vitamins C and E under constant heat stress. Animal 10, 163–171. doi:10.1017/S1751731115001998
Zhu W., Jiang W., Wu L. Y. (2014). Dietary L-arginine supplement alleviates hepatic heat stress and improves feed conversion ratio of Pekin ducks exposed to high environmental temperature. J. Anim. Physiol. Anim. Nutr. 98, 1124–1131. doi:10.1111/jpn.12195
Zulkifli I., Akmal A. F., Soleimani A. F., Hossain M. A., Awad E. A. (2018). Effects of low-protein diets on acute phase proteins and heat shock protein 70 responses, and growth performance in broiler chickens under heat stress condition. Poult. Sci. 97, 1306–1314. doi:10.3382/ps/pex436
Zulkifli I., Htin N. N., Alimon A. R., Loh T. C., Hair-Bejo M. (2006). Dietary selection of fat by heat-stressed broiler chickens. Asian-Australas. J. Anim. Sci. 20, 245–251. doi:10.5713/ajas.2007.245
Zuo J., Xu M., Abdullahi Y. A., Ma L., Zhang Z., Feng D. (2015). Constant heat stress reduces skeletal muscle protein deposition in broilers. J. Sci. Food Agric. 95, 429–436. doi:10.1002/jsfa.6749
Keywords: heat stress, chicken, feed intake, digestibility, feeding strategies, amino acids, energy, broiler
Citation: Teyssier J-R, Brugaletta G, Sirri F, Dridi S and Rochell SJ (2022) A review of heat stress in chickens. Part II: Insights into protein and energy utilization and feeding. Front. Physiol. 13:943612. doi: 10.3389/fphys.2022.943612
Received: 13 May 2022; Accepted: 05 July 2022;
Published: 08 August 2022.
Edited by:
Gale Strasburg, Michigan State University, United StatesReviewed by:
Paramjit S. Tappia, I. H. Asper Clinical Research Institute, CanadaMarkus Rodehutscord, University of Hohenheim, Germany
Birger Svihus, Norwegian University of Life Sciences, Norway
Copyright © 2022 Teyssier, Brugaletta, Sirri, Dridi and Rochell. This is an open-access article distributed under the terms of the Creative Commons Attribution License (CC BY). The use, distribution or reproduction in other forums is permitted, provided the original author(s) and the copyright owner(s) are credited and that the original publication in this journal is cited, in accordance with accepted academic practice. No use, distribution or reproduction is permitted which does not comply with these terms.
*Correspondence: Jean-Rémi Teyssier, jteyssie@uark.edu; Samuel J. Rochell, rochell@uark.edu