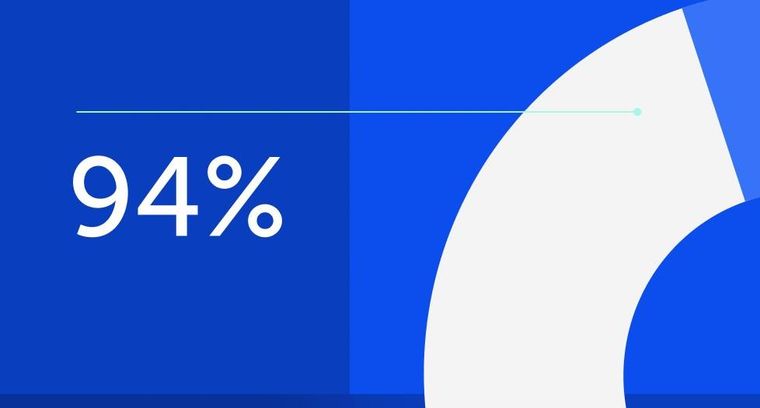
94% of researchers rate our articles as excellent or good
Learn more about the work of our research integrity team to safeguard the quality of each article we publish.
Find out more
ORIGINAL RESEARCH article
Front. Physiol., 23 June 2022
Sec. Integrative Physiology
Volume 13 - 2022 | https://doi.org/10.3389/fphys.2022.934597
This article is part of the Research TopicMolecular Physiology of Tissue Adaptation to Acute Ischemic InjuryView all 5 articles
We used nicorandil, a K-ATP channel opener, to study the role of these channels in the amelioration of renal ischemia/reperfusion (I/R)-induced pancreatic injury, and the possible involvement of PI3K/Akt/mTOR signaling pathway. Forty-two male Wistar rats were included in this study, six were sacrificed for extraction of bone marrow mesenchymal stem cells (BM-MSCs) and conducting the in-vitro work, the others were included in vivo study and equally divided into six groups. Group 1 (sham control), but groups 2–6 were subjected to bilateral renal I/R: Group 2 (I/R); Group 3 (I/R-NC), treated with nicorandil; Group 4 (I/R-MSCs), treated with BM-MSCs; Group 5 (I/R-MSCC), treated with nicorandil-preconditioned BM-MSCs; Group 6 (I/R-NC-MSCC), treated with both systemic nicorandil and preconditioned BM-MSCC. Renal injury and subsequent pancreatic damage were detected in the I/R group by a significant increase in serum urea, creatinine, fasting glucose, and pancreatic enzymes. The pancreatic tissues showed a reduction in cellularity and a significant decrease in the expression of the cell survival pathway, PI3K/Akt/mTOR, in the I/R group compared to the control. Preconditioning MSCs with nicorandil significantly enhanced the proliferation assay and decreased their apoptotic markers. Indeed, combined systemic nicorandil and nicorandil-preconditioning maintained survival of MSC in the pancreatic tissue and amelioration of apoptotic markers and pancreatic TNF-α production. Histologically, all treated groups revealed better pancreatic architecture, and increased area % of anti-insulin antibody and CD31, which were all best observed in the NC-MSCC group. Thus, using K-ATP channel opener was efficient to enhance PI3K/Akt/mTOR expression levels (in vivo and in vitro).
Renal ischemia/reperfusion injury (I/R) is one of the unavoidable co-incidences during kidney transplantation or vascular surgery. Tissue ischemia causes multiple series events that progress to cell injury, dysfunction, necrosis, and death (Kocaturk et al., 2020). Moreover, reperfusion exacerbates this damage via enhancing the production of reactive oxygen species (ROS) and inflammatory response (Yapca et al., 2013). Renal ischemia/reperfusion induces multi-organ damage including pancreatic injury (Hussein et al., 2014). Abogresha and colleagues (2016) depicted the contribution of ROS as an important cause of pancreatic cell death following renal I/R injury. In this context, M El Agaty and Ahmed (2020) reported a significant decrease in area % of insulin immune reactivity denoting a declined β-cell number in rats exposed to renal I/R injury for 45 min versus sham-operated group.
K-ATP channels are found in beta cells and in other extra-pancreatic tissues. In pancreatic tissues, K-ATP channels regulate insulin secretion. By inhibiting these channels, the beta cells depolarize and Ca2+ influx occurs, on the contrary opening K-ATP channels leads to hyperpolarization of the beta cells and prevents insulin release (Ashcroft and Gribble. 1999). Worthy, the activation of K-ATP channels in cardiac muscles protects the heart during ischemia by limiting Ca2+ entry. Even more, activation of these channels in blood vessels causes membrane hyperpolarization, thus it could inhibit voltage-sensitive Ca2+-channels eventually causing vasodilation (Nichols, 2016). In brain tissues, the K-ATP opener exerts neuroprotective anti-neuroinflammatory effects against trauma and ischemia (Zhou et al., 2008). Previous data showed inhibition of rotenone-induced production of tumor necrosis factor-alpha (TNF-α) and ROS in brain tissues of rats treated with K-ATP opener (Zhou et al., 2008). One of the K-ATP openers is a 2-nicotiamidoethyl-nitrate ester (Nicorandil), it is the only mitochondrial K-ATP opener and also acts as nitric oxide (NO) donor (Zhang et al., 2015). Indeed; Horinaka et al, (2001) reported that nicorandil enhanced the cardiac eNOS levels in rats, secondary to its K-ATP opening effect. In a dose-dependent manner, nicorandil succeeded in amelioration of acute tubular damage observed in rats exposed to unilateral renal I/R for 30 min (Shimizu et al., 2011), however, its therapeutic efficacy in the remote effects of prolonged bilateral I/R injury of the kidneys wasn’t investigated.
Mesenchymal stem cells (MSCs) have been widely studied in regenerative medicine. With its impressive paracrine ability, anti-inflammatory, and differentiation capacity, MSCs represent one of the most ideal lines for tissue regeneration in several diseases such as ischemic conditions (Hu and Zou. 2017). However, the major challenge for the therapeutic potential of MSCs is the poor survival rate following transplantation. In Zhang and colleagues’ study (2015), nicorandil enhanced PI3K/Akt pathway in stem cells exposed to hypoxia, therefore protecting them from apoptosis and decreased ROS production in culture media. The PI3K/Akt is a pro-survival pathway that controls cell apoptosis. Akt control pancreatic cell plasticity, consequently Akt/mTOR inhibition induces both autophagy and apoptotic cell death (Yu et al., 2018). Thus, it is likely to target the mitochondrial K-ATP role as a novel regulator against ischemia-reperfusion hazards. By administration of the mitochondrial K-ATP opener (nicorandil), that was used in vitro for MSCs preconditioning or in vivo in male rats exposed to bilateral renal I/R injury. We speculated that nicorandil may enhance the survival of MSCs in vitro and together with its in vivo intake could optimize pancreatic protection from the remote effect of bilateral renal ischemia for 45 min and reperfusion for 7 days.
A total of forty-two male Wistar rats (aged 10–12 months and of body weight 180–200 g) were included in this study. Six of them were used for isolation and expansion of MSCs and the others were included in the in vivo study. All experimental work and animal procedures were done in accordance with the international guidelines of Helsinki. Part of this assurance includes the establishment of an appropriated constituted Institutional Animal Care and Use Committee that had the responsibility to review and monitor research involving experimental animals. Animals were purchased from and inbred in the Experimental Animal Unit, Faculty of Medicine, Cairo University. Male rats were maintained in specific pathogen-free conditions, fed normal animal chow, and were subjected to a 12:12-h daylight/darkness. Rats were housed in standard cages at room temperature of 25 ± 2°C and left to acclimatize for a few days before starting the experimental procedures.
The experimental design and all animal procedures were carried out in accordance with the U.K. Animals (Scientific Procedures) Act, 1986 and associated guidelines, EU Directive 2010/63/EU for animal experiments. This study was conducted in and approved by the Animal House, Physiology-Faculty of Medicine, Cairo University according to the guidelines for the use of experimental animals of Cairo University.
Thirty-six rats were involved in the in vivo study, divided into six groups (6 rats in each). Animals were randomly allocated into; Group 1 (control), as sham-operated rats, the rats in this group were anesthetized and the abdominal wall was opened then the kidneys were exposed. Animals were observed for the same duration equivalent to that of renal ischemia in the other I/R groups but no further surgical manipulations were done. A well-moistened gauze was applied over the abdominal viscera, thus keeping organs moist; Group 2 (I/R), as a group of bilateral renal I/R, rats in the group were subjected to bilateral renal ischemia for 45 min and reperfusion for 7 days; Group 3 (I/R-NC), a group of bilateral renal I/R treated with systemic nicorandil 30 min before and 24 h after the operation (3 mg·kg-1 I.P) (Shimizu et al., 2011); Group 4 (I/R-MSCs), bilateral renal I/R group treated with BM-MSCs; Group 5 (I/R-MSCC), bilateral renal I/R group treated with BM-MSCs preconditioned with nicorandil; Group 6 (I/R-NC-MSCC), presenting bilateral renal I/R group that was treated with a combined systemic nicorandil and conditioned BM-MSCC. Both conditioned and non-conditioned BM-MSCs were given once at a dose of 1.5 × 106 cells for each rat (Erpicum et al., 2017) and injected to rat tail veins immediately after reperfusion. The total duration of the experiment was 7 days and early at the eighth-day blood samples were collected from the animals and all were sacrificed using a high dose of phenobarbital (800 mg/kg IP) (Zatroch et al., 2017).
Rats were anesthetized with ketamine (50 mg/kg) and xylazine (5 mg/kg) that were injected intraperitoneal (Struck et al., 2011). The animals were fixed in the supine position on a thermoregulated heating board to maintain their body temperature at 37°C. After hair shaving, the skin of the abdominal wall was sterilized. A midline abdominal incision was done to open all layers of the anterior abdominal wall and fascia, thus allowing exposure of kidneys. Then, renal pedicles on both sides were exposed and clamped using a vascular clamp to induce renal ischemia. After 45 min, the vascular clamps were removed and the abdominal cavity was closed with double sutures (for peritoneum and facia) (Hussein et al., 2014).
Blood samples were collected at the end of the study; serum was separated after centrifugation and stored at -80°C for biochemical analysis. Pancreatic tissues were collected (extracted) and prepared for histological examinations, immunohistochemical analysis of CD31, and biochemical measurement of apoptotic markers.
- Nicorandil: obtained from Sigma-Aldrich was dissolved in saline (0.9% NaCl and pH 7.4)
- Ketamine HCl obtained from the pharmacy: a product of Sigma-Tec (Egypt) in the form of vials, each one containing 50 mg/ml.
- Xylazine HCl obtained from the veterinary pharmacy: a product of Kepro veterinary solutions (Holland) in the form of vials, each one containing 20 mg/ml.
- Phenobarbital obtained from the veterinary pharmacy was given for euthanasia once at the end of the study at a dose of 800 mg/kg
Six male Wistar rats were sacrificed and femur bones were dissected and used for extraction of bone marrow samples. The bone marrow samples were obtained by flushing the bone marrow cavity with phosphate-buffered saline [PBS, Sigma Chemical Co. (United States)]. A preparation of 15 ml from flushed bone marrow cells was carefully layered for 35 min on 15 ml Ficoll-Paque (Gibco-Invitrogen, Grand Island, NY). Then the preparation was centrifuged at 400×g rpm. The mononuclear layer of cells was carefully aspirated and washed twice in PBS containing 2 mM Ethylene diamine tetra-acetic acid (EDTA) and centrifuged for 10 min at 200×g rpm at 5°C.
On 25 ml flasks, the isolated bone marrow MSCs were expanded. Cells were cultured in Roswell Park Memorial Institute (RPMI)-1640 medium containing 10% fetal bovine serum (FBS product of Sigma, United States), 0.5% penicillin, and streptomycin. The prepared flasks were incubated at 37°C and 5% CO2 until reaching 80%–90% allowing their confluence within 7 days. Expansion of MSCs was done till reaching the third passage. MSCs were identified by morphology and Fluorescent Analysis Cell Sorting technique (FACS) to be CD 29+, CD 90+, and CD 105+ positive and CD 34+ negative before preconditioning. (Figure 1).
FIGURE 1. Immunophenotyping of the isolated bone marrow mesenchymal stem cells. Isolated MSCs were identified by flow cytometry for detection of CD 29+, CD 90+, and CD 105+ cell surface proteins and negativity of CD 34+.
Obtained mesenchymal stem cells were divided into non-conditioned (MSCs) and nicorandil-conditioned (MSCs-Conditioned) stem cells.
The conditioned MSCs were incubated with nicorandil (100 µM) for a time period of 90 min. This time was selected according to Zhang and colleagues’ study (2015).
The proliferation of both non-conditioned and nicorandil-conditioned stem cells was determined using the 3-(4,5-dimethylthiazol-2-yl) −2.5-diphenyltetrazolium bromide (MTT) cell proliferation kit (Trevigen Inc., Gaithersburg, MD). All procedures were done according to the manufacturer’s instructions. After culturing the cells and overnight incubation to permit cell attachment, an MTT reagent was added to allow the reduction of the soluble intracellular MTT to an insoluble purple formazan dye. Viable cells with active metabolism convert MTT into a purple-colored formazan product. The formazan is then solubilized prior to recording absorbance. Absorbance was measured at 550–600 nm using a plate reading spectrophotometer to evaluate cell proliferation.
All MSCs were washed with DMEM then were labeled with PKH 26 Red Fluorescent Cell Linker Kit for General Cell Membrane Labeling (Sigma-Aldrich) and injected into the rat tail vein immediately after reperfusion. A fresh solution of PKH 26 ethanolic dye was prepared that contained a final concentration of 2 × 10–6 PKH 26. A mix of 2 μl of working dye and 1 × 107 cell/ml were centrifuged then dilution was done according to manufacturer instructions. For detection of stem cells in the pancreas, after sacrifice pancreatic paraffin blocks were prepared and MSCs were detected under Leica immunofluorescence microscopy.
Transplanted stem cells (either non-conditioned or pre-conditioned) were directly injected into rat tail veins of assigned groups at a dose of 1.5 × 106 cells for each rat (Erpicum et al., 2017).
Measurements of serum urea and creatinine were carried out by colorimetry using reagent kits (Urea Colorimetric Assay Kit Cat. No. E-BC-K329-S Houston, Texas) & Creatinine Assay Kit (Colorimetric) (ab204537) Waltham, United States) to assess renal function.
Fasting serum glucose was measured by colorimetry using (Glucose Colorimetric Assay Kit (ab282922), Waltham, United States) kits.
Serum amylase was measured using Colorimetric assay kits obtained from Abcam (ab102523) following the manufacturer’s instructions. Lactate dehydrogenase Enzyme (LDH) Activity Assay: Tissue damage following ischemia-reperfusion was assessed using serum LDH activity. According to kit instruction [LDH Assay Kit/Lactate Dehydrogenase Assay Kit (Colorimetric) (ab102526)]. Determination of myeloperoxidase (MPO) activity: Evidence of inflammation and pancreatic neutrophil infiltration were detected by quantifying tissue MPO activity. According to kit instruction; MPO Activity Assay Kit (Colorimetric) (ab105136).
Determination of TNF- α concentrations in pancreatic tissues by the sandwich Enzyme-Linked Immuno-Sorbent Assay (ELISA) kits according to the manufacturer’s instruction; TNF-alpha ELISA Kit, RAB1089-1 KT (Sigma-Aldrich Chemie GmbH Eschenstrasse 5 D-82024 TAUFKIRCHEN).
After tissue homogenization, the formed component was mixed with PBS, the formed homogenate was centrifugated and the supernatant was collected. Then using quantitative Sandwich Rat Cyclic Guanosine Monophosphate (c-GMP) ELISA Kit (MyBioSource, Catalog #MBS007871, the level of c-GMP was estimated and expressed per ml.
Phosphorylated PI3K/Akt/mTOR were detected by western blot analysis (Mahmood and Yang. 2012) using anti-phospho-PI3K (Tyr458, 1:1000; Cell Signaling), anti-phospho-AKT (Ser473, 1:2000; Cell Signaling) (#4060), anti-phospho-mTOR (Ser2448, 1:1000; Cell Signaling) antibodies (#2971) and were normalized to β-actin antibodies (Cell signaling technology), Proteins from pancreatic tissues and MSCs pellets were extracted by RIPA lysis buffer which was provided by Bio Basic Inc. (Markham, Ontario L3R 8T4, Canada). 20 μg protein were separated by SDS/PAGE on 4%–20% polyacrylamide gradient gels, then added in each lane. After incubation in 5% non-fat dry milk, Tris/HCl, 0.1% Tween 20 for 1 h; collagen-I monoclonal antibody was added to one of the membranes containing specimen samples and incubated at 4°C overnight. The blots were incubated with the peroxidase-conjugated secondary antibody (Novus Biologicals) solution for 2 h at room temperature. After being washed twice with 1× TBST, densitometric analyses of the immunoblots were performed to quantitate the amount of PI3K, Akt, and mTOR against the control sample by total protein normalization using image analysis software on the ChemiDocMP imaging system (version 3) produced by Bio-Rad (Hercules, CA).
The pancreatic tissues and MSCs of all the studied groups were lysed and total RNA was isolated with Gene JET Kit (Thermo Fisher Scientific Inc., Germany, #K0732). The one-step qRT-PCR reaction was done; for reverse transcription; about 5 µl from the total RNA from each sample was used with subsequent amplification with Bioline, amedian life science company, U.K. (SensiFASTTM SYBR R Hi-ROX) One-step Kit (catalog number PI-50217 V) in a 48-well plate using the Step-one instrument (Applied Biosystems, United States of America). The thermal profile was as follows: 45°C for 15min in one cycle (for cDNA synthesis), 10 min at 95°C for reverse transcriptase enzyme inactivation, followed by 40 cycles of PCR amplification. 10 s at 95°C, 30 s at 60°C, and 30 s at 72°C. were adjusted for each cycle. The expression levels of studied genes were normalized relative to the mean critical threshold (CT) values of ß actin as the housekeeping gene by the Ct method. The qPCR assay was done with primers specific for Bax (forward: 5′- GTT TCA TCC AGG ATC GAG CAG-3′; and reverse: 5′- CAT CTT CTT CCA GAT GGT GA-3′, for Bcl2 (forward: 5′- CCT GTG GAT GAC TGA GTA CC-3′ and reverse: 5′- GAG ACA GCC AGG AGA AAT CA-3′ and the housekeeping gene ß-actin (forward: 5′- GCA CCA CAC CTT CTA CAA TG-3′ and reverse: 5′- TGC TTG CTG ATC CAC ATC TG-3′).
Pancreatic tissues were carefully-dissected and kept in 10% formol saline solution. After routine histological preparation and paraffin embedding, 3–5 μm sections were stained with hematoxylin and eosin (H&E) and were subjected to immunohistochemical evaluation. Histological assessment of the pancreas was performed in six non-over-lapping fields as previously described (Yang et al., 2020). Grading of the pancreas was evaluated based on a 0 to 4 score of edema, inflammatory cellular infiltration, and acinar necrosis where 0 indicates no lesion, 1 indicates focal edema, ductal inflammation, or peri-ductal necrosis, 2 is given to diffuse edema, <20% of the lobules with inflammation or focal parenchymal necrosis, 3 is scored for acinar disruption, 20%–50% of the lobules with inflammation or diffuse parenchymal necrosis, score 4 is given to separated acini, inflammation in >50% of the lobules or diffuse parenchymal necrosis (>50%).
Immunohistochemical staining was performed according to the manufacturer’s instructions using the following antibodies; rabbit polyclonal anti-cleaved caspase-3 antibody (Cat. # ab2302, 1:100), rabbit polyclonal anti-CD31antibody (ab124432, 1:100), rabbit polyclonal anti-insulin antibody (ab63820, 1:100) (IHC-P, species reactivity including rats, Abcam R, Cambridge, United States). The 3 μm sections were deparaffinized, rehydrated, and subjected to microwave mediated antigen retrieval, quenching of endogenous peroxidase activity, and staining with the avidin-biotin method as previously described (Wanas et al., 2022). The sections were counterstained with Meyer’s hematoxylin for nuclear visualization. Negative controls were prepared using the same procedure after the omission of the primary antibodies.
The area percentage of insulin immune-reaction in the pancreatic islets, the count of CD31 positive micro-capillaries, the area percentage of caspase 3 immunohistochemical expression, and the count of PKH26-labeled MSCs, were all performed in six non-overlapping fields under magnification 400 inside a standard measuring frame of 85,550 mm2 using Leica Qwin-500 LTD-software image analysis computer system (Cambridge, England) and ImageJ analysis computer system (J Image Pro Plus6.0, Media Cybernetics, Silver Spring, MD, United States).
Data were expressed as mean ± SD and analyzed using SPSS computer software version 22. For in vivo study; comparisons between groups were done using ANOVA and Bonferroni post hoc tests for multiple comparisons. Paired sample t-test was done to compare between MSCs groups in the in vitro study. p-value ≤ 0.05 was considered significant.
A significant increase in serum urea and creatinine levels was observed in I/R group compared to the sham control group (p-value <0.001). The level of urea and creatinine showed a significant decrease in all treated groups compared to the I/R group (p-value <0.001).
The results showed no significant difference in urea and creatinine levels between I/R-NC, I/R-MSCs, and I/R-MSCC groups (p-value >0.05), while the combined systemic intake of NC and MSC conditioned with NC resulted in a significant decrease in urea and creatinine compared to I/R-NC, I/R-MSCs and I/R-MSCC groups (p-value <0.001). (Table 1).
TABLE 1. Serum urea and creatinine levels in all studied groups (n = 6 in each group). p-value ≤0.05 is considered significant.
Bilateral I/R injury resulted in pancreatic damage that was detected by a significant increase in fasting glucose level in rats subjected to I/R injury compared to control (p-value <0.001) (Table 2). Whereas, a significant decrease in glucose level was documented in all treated groups compared to the I/R group (p-value <0.001). A significant decrease in glucose level was observed in I/R-MSCC, and I/R-NC-MSCC compared to both I/R -NC, and I/R-MSCs (p-value 0.019, <0.001) (0.032, <0.001 respectively). Moreover, the combined systemic NC and MSC-conditioned stem cells induced a significant decrease in glucose level in I/R-NC-MSCC compared to I/R-MSCC, (p-value <0.001) (Table 2).
TABLE 2. Estimated levels of blood glucose, LDH, amylase, MPO, and TNF-α in all studied groups (n = 6 in each group). p-value ≤0.05 is considered significant.
The remote effect of I/R injury was also detected by the significant increase in LDH, amylase, and MPO (markers for pancreatic injury) in the I/R group compared to the control sham-operated group (p-value <0.001). In addition, all treated groups showed a significant decrease in LDH, amylase, and MPO levels compared to the I/R group (p-value <0.001), however, the level of LDH was still significantly higher in all treated groups except I/R-NC-MSCC compared to the control sham-operated group (p-value <0.001 for all and 0.219 for I/R-NC-MSCC), and no significant difference observed in amylase levels between I/R-NC-MSCC, I/R-MSCC, and control group (p-value >0.05). The combined treatment with systemic NC and MSCC produced a significant decrease in MPO compared to I/R-NC, I/R-MSCs, I/R-MSCC (p-value <0.001) (Table 2). Ischemic/reperfusion injury was associated with significant decrease in c-GMP level compared to control group. Whereas, nicorandil treatment yielded significant increase in tissue cGMP levels compared to I/R and I/R-MSC and I/R-MSCs.
Bilateral renal I/R injury enhanced the production of pancreatic TNF-α compared to the control group (p-value <0.001). However, in all treated groups, our results showed a significant decrease in TNF-α that was observed compared to the I/R group (p-value <0.001). The combined intake of nicorandil and MSCs that were conditioned with NC successfully decreased the tissue level of TNF-α, indeed the level of this marker was significantly decreased in I/R-NC-MSCC (p-value <0.001) compared to I/R-NC, I/R-MSCs, and I/R-MSCC groups (Table 2).
Activation of the PI3K/Akt pathway supports and promotes cell survival and proliferation. Currently, the results showed a significant decrease in PI3K/Akt/mTOR expression in the I/R group compared to the control sham-operated group (p-value <0.001), while a significant increase in their expression levels was detected in all treated groups compared to the I/R group (p-value <0.05). The intake of NC systemically together with MSCC in the I/R-NC-MSCC group caused a significant increase in immunoblotting of PI3K/Akt levels compared to I/R–NC (p-value <0.001), I/R-MSCs group (p-value <0.001), and I/R-MSCC (p-value <0.001). mTOR is downstream signaling of PI3K/Akt was estimated also. Our results showed a significant increase in immunoblotting of mTOR in I/R-NC-MSCC group compared to I/R–NC (p-value = 0.004), I/R-MSCs group (p-value = 0.004), and I/R-MSCC (p-value = 0.047) (Figure 2).
FIGURE 2. Western blot analysis of PI3K/Akt/mTOR in pancreatic tissues of the in vivo study. (A) Protein bands and (B) Quantitative measurement of mean and SD of all data. a: significant versus control group, b: significant versus I/R group, c: significant versus I/R -NC group, d: significant versus I/R-MSCs group, and e: denotes significant difference versus I/R-MSCC group at p-value ≤ 0.05 using ANOVA, Bonferroni post hoc test, n = 6).
Bcl2 gives rise to cellular protection via exerting anti-apoptosis, it could bind to Bax preventing the pore formation and subsequent molecular changes that end in cell death. For instance, the expression level of Bax was significantly increased (p-value <0.001) in the I/R group compared to the control sham-operated one. The results showed a significant decrease in Bax in all treated groups compared to the I/R group (p-value <0.001), however, the maximum inhibition of Bax, the death-promoting factor, was observed in combined systemic administration of NC and pre-conditioned MSCs. In this context, the level of Bax was significantly decreased in the I/R-NC-MSCC group compared to I/R-NC, I/R-MSCs, and I/R-MSCC groups (p-value <0.001).
Compared to Bax, the expressions levels of Bcl2 were significantly decreased in the I/R group compared to the control group (p-value <0.001). Over-expression of Bcl-2 enhances cell survival by suppressing apoptosis, thus our results showed a significant increase in Bcl2 in all treated groups compared to the I/R group (p-value <0.001). There was also a significant rise in I/R-MSCC, I/R-NC-MSCC groups compared to the I/R-MSCs group (p-value <0.001). Accordingly, pre-conditioning of MSCs augmented their anti-apoptotic effects (Table 3).
TABLE 3. Changes in Bax and Bcl2 in all studied groups (n = 6 in each group). p-value ≤0.05 is considered significant.
Comparable results have been obtained from the immunohistochemistry of caspase 3 (the effector of apoptosis) where the area percent of caspase 3 immunohistochemical expression showed a significant increase in the I/R group (p-value <0.001), and a significant decrease in all the treated groups with best results obtained in the combined NC-MSCC group. A noticeable result was the statistically-significant difference between the MSCs group and the conditioned MSC on one side and the combined NC-MSCC group on the other side, which further confirms the role of nicorandil in augmenting the anti-apoptotic effect of MSCs (Figure 3).
FIGURE 3. Immunohistochemical staining of caspase 3 in the endocrine and exocrine portion of the pancreatic tissue of (A) Control group, (B) Ischemia/reperfusion (I/R), (C) Ischemia/reperfusion-Nicorandil group (I/R-NC), (D) Ischemia/reperfusion-mesenchymal stem cell group (I/R-MSCs), (E) Ischemia/reperfusion-conditioned mesenchymal stem cell group (I/R-MSCC), (F) Ischemia/reperfusion-conditioned mesenchymal stem cell + nicorandil group (I/R-NC-MSCC) displaying strong positive caspase 3 expression in both the pancreatic islets and acini of the I/R group, moderate immunoreactivity in the I/R-NC group, mild expression in the I/R-MSCs and I/R-MSCC groups and minimal immunoreactivity in the I/R-NC-MSCC group. (G,H) Area percentage of caspase 3 expression in the endocrine (G) and exocrine (H) portion of the pancreas in the different study groups a: significant versus control group, b: significant versus I/R group, c: significant versus I/R -NC group, d: significant versus I/R-MSCs group and e: denotes significant difference versus I/R-MSCC group at p-value ≤ 0.05 using ANOVA, Bonferroni post hoc test, n = 6).
Using MTT%, the proliferation of both non-conditioned and mesenchymal stem cells-conditioned with NC was determined. Our results showed increased MTT% of MSC-conditioned that was statistically significant to MSCs (P-value <0.001) (Table 4).
TABLE 4. Enhanced cell proliferation assay (MTT%) and decreased apoptotic markers of MSC incubated with nicorandil. p-value ≤0.05 is considered significant.
In culture media, the expression levels of Bax were significantly decreased in the MSC-Conditioned group compared to MSCs (p-value <0.001), whereas Bcl2 gene expression, as well as the immunoblotting of PI3K/Akt/mTOR, were significantly increased in the MSC-Conditioned group compared to MSCs (p-values 0.004, 0.001, <0.001 and <0.001 respectively) (Table 4; Figure 4).
FIGURE 4. Western blot analysis of PI3K/Akt/mTOR in vitro study. Incubation with nicorandil enhanced the pro-survival PI3K/Akt/mTOR signaling pathway of MSCs. (A) Protein bands and (B) Quantitative measurement of mean and SD of all data. a: significant versus non-conditioned MSCs.
Evaluation of delivered MSCs in pancreatic tissues under the Leica immunofluorescence microscope revealed a statistically-significant increase in the count of PKH26-labeled MSC, in the nicorandil-preconditioned MSC group relative to the non-conditioned MSCs. The combination between systemic nicorandil and nicorandil-preconditioning caused better survival of MSC in the pancreatic tissue with results superior to both the NC-MSCs and the NC-MSCC groups (Figure 5).
FIGURE 5. PKH26-labeled immunofluorescent images showing enhanced survival of the MSCs into the pancreatic tissue of the combined systemic nicorandil and nicorandil-conditioned MSC group (I/R-NC-MSCC) relative to the other groups, (I/R-MSCs: Ischemia/reperfusion mesenchymal stem cell group, I/R-MSCC: Ischemia/reperfusion-conditioned mesenchymal stem cell group) (d: significant versus I/R-MSCs group and e: denotes significant difference versus I/R-MSCC group at p-value ≤ 0.05 using ANOVA, Bonferroni post hoc test, n = 6).
Histological scoring of H&E-stained sections was carried out for evaluating the pathological alterations in the different study groups. Renal I/R caused significant pathological changes in both the endocrine and exocrine portion of the pancreas, where the islets of Langerhans appeared atrophied with pyknotic nuclei and exhibited a marked reduction in cellularity. The pancreatic lobules appear shrunken and were seen separated by wide interlobular septae. The pancreatic acini appeared degenerated and were invaded by mononuclear inflammatory cellular infiltration. Treatment with nicorandil and/or MSC caused improvement in pancreatic architecture. This improvement was unsurpassed observed in the combined NC-MSCC group where the islets of Langerhans displayed dark rounded nuclei and pale acidophilic cytoplasm with healthy capillaries in-between and the serous acini showed well-defined boundaries and were seen lined by intact pyramidal cells with basal rounded nuclei and apical acidophilic cytoplasm comparable to the control group (Figure 6).
FIGURE 6. H&E-stained sections of the pancreatic tissue of (A) Control group, (B) Ischemia/reperfusion (I/R) showing small atrophied islet of Langerhans with a marked reduction in cellularity and pyknotic nuclei (IL). The pancreatic lobules appear shrunken and separated by wide interlobular septae (S). Degenerated acini (A), ductal hyperplasia (D), and vascular angiopathy are also observed. A mononuclear inflammatory cellular infiltration (I) and blood extravasation (curved arrows) are also noticed between pancreatic lobules, (C) Ischemia/reperfusion-Nicorandil group (I/R-NC) showing mild irregularity and vacuolations (V) of the pancreatic acini, (D) Ischemia/reperfusion-mesenchymal stem cell group (I/R-MSCs) showing improved islet and acinar structure and normal interlobular duct with only mild vascular congestion (C) and focal acinar degeneration (spiral arrow), (E) Ischemia/reperfusion-conditioned mesenchymal stem cell group (I/R-MSCC) displaying preserved islet and acinar architecture, (F) Ischemia/reperfusion-conditioned mesenchymal stem cell + nicorandil group (I/R-NC-MSCC) illustrating intact cells of islets of Langerhans with dark rounded nuclei and pale acidophilic cytoplasm with blood capillaries in-between. The serous acini show well-defined boundaries, appear lined by pyramidal cells with basal rounded nuclei and apical acidophilic cytoplasm comparable to the control group, (G) pathological injury scoring of the different study groups.
The area percentage of insulin immunohistochemistry showed a significant decrease in the I/R group compared with the control group. The treatment groups showed a significant increase in insulin expression compared to the I/R group (p-value <0.001) with the maximum induction observed in the combined systemic NC and nicorandil pre-conditioned MSCs (Figure 7).
FIGURE 7. Immunohistochemical staining of insulin in the pancreatic islets of (A) Control group, (B) Ischemia/reperfusion (I/R), (C) Ischemia/reperfusion-Nicorandil group (I/R-NC), (D) Ischemia/reperfusion-mesenchymal stem cell group (I/R-MSCs), (E) Ischemia/reperfusion-conditioned mesenchymal stem cell group (I/R-MSCC), and (F) Ischemia/reperfusion-conditioned mesenchymal stem cell + nicorandil group (I/R-NC-MSCC). Notice: restoration of the pancreatic β-cells insulin immune-stained granules in the treatment groups with Strong positive immunoreactivity in the I/R-NC-MSCC group, (G) Area percentage of insulin immune-expression in the different study groups (a: significant versus control group, b: significant versus I/R group, c: significant versus I/R-NC group, d: significant versus I/R-MSCs group, and e: denotes significant difference versus I/R-MSCC group at p-value ≤ 0.05 using ANOVA, Bonferroni post hoc test, n = 6).
Owing to the importance of CD31 as well-defined markers of angiogenesis highly expressed on the surface of endothelial cells, the current study has evaluated the changes in immunohistochemical expression of CD31 in ischemia/reperfusion with and without supplementation of nicorandil and mesenchymal stem cells.
Our results have revealed a statistically significant decrease in the CD31 immune-histochemical expression in the I/R group. Supplementation with nicorandil and nicorandil-preconditioned mesenchymal stem cells resulted in a significant increase in CD31 immunoreactivity which essentially denotes improvement in angiogenesis and microcirculation (Figure 8).
FIGURE 8. Immunohistochemical staining of CD31 reactive micro-vessels in the pancreas of (A) Control group, (B) Ischemia/reperfusion (I/R), (C) Ischemia/reperfusion-Nicorandil group (I/R-NC), (D) Ischemia/reperfusion-mesenchymal stem cell group (I/R-MSCs), (E) Ischemia/reperfusion-conditioned mesenchymal stem cell group (I/R-MSCC), (F) Ischemia/reperfusion-conditioned mesenchymal stem cell + nicorandil group (I/R-NC-MSCC) demonstrating marked reduction in CD31 immunoreactivity in the I/R group and numerous CD31 positive microcapillaries in both the pancreatic acini and islets of Langerhans in the combined I/R-NC-MSCC group, and (G) Count of CD31 immuno-reactive micro-capillaries in the different study groups (a: significant versus control group, b: significant versus I/R group, c: significant versus I/R -NC group, d: significant versus I/R-MSCs group and e: denotes significant difference versus I/R-MSCC group, at p-value ≤ 0.05 using ANOVA, Bonferroni post hoc test, n = 6).
In the last few decades, many efforts are expended to alleviate the damaging effect of ischemia-reperfusion injury (Frangogiannis. 2015). From this concept, we investigated in this study the effect of opening the K-ATP channel in modulating the deleterious hazards associated with the renal I/R injury in rat pancreas and the underlying molecular mechanism related to the PI3K/Akt/mTOR signaling pathway. To evaluate the role of the channel, we used nicorandil, a well-known K-ATP channel opener. In addition, we hypothesized that engaging several protocols by applying nicorandil, as a pharmacological therapeutic approach as well as a preconditioning therapy for mesenchymal stem cells may be so promising. Our findings revealed activated K-ATP channel may provide new insights in rescuing pancreatic damage following renal I/R injury through its anti-inflammatory, anti-apoptotic and pro-survival effect on stem cells.
It is well known that prolonged periods of renal ischemia over 35 min can result in irreversible acute tubular injury and kidney dysfunction (Holderied et al., 2020). In accordance, our results showed significantly impaired kidney function, manifested by elevated serum urea and creatinine in I/R rats.
The remote effect of renal I/R injury has been considerably investigated in various organs like the liver, heart, brain, and lungs. Here, we reported significant elevation in markers of pancreatic tissue injury (LDH, amylase, and MPO) after 7 days of I/R associated with high serum glucose levels. Such changes indicated that the pancreatic structure and function were significantly damaged after I/R, a finding which is consistent with previous studies (Hussein et al., 2014; Abogresha et al., 2016).
Besides the biochemical findings, these studies are also consistent with our histopathological results, which demonstrated marked deterioration of both the endocrine and exocrine portion of the pancreas in the form of atrophy of islets of Langerhans, shrinkage of pancreatic lobules, acinar necrosis, ductal hyperplasia, vascular angiopathy, blood extravasation, and mononuclear inflammatory cellular infiltration.
It was generally believed that I/R and associated distant organ failure is a complex process with multiple underlying mechanisms (Yuan et al., 2020). The release of inflammatory factors, such as TNF-α has been found to have a crucial role in mediating and exacerbating ischemia/reperfusion injury. In the current study, TNF-α was investigated as a proinflammatory cytokine that can mediate apoptosis via increasing caspase levels. It was significantly elevated in pancreatic tissue in ischemic rats compared to sham-operated rats. Consistent with excess TNF-α, elevated Bax/Bcl2 ratio denoting apoptotic cascades stimulation was significantly observed in injured pancreatic tissues. This agrees with previous works, which demonstrated that I/R was associated with excessive production of ROS leading to acute pancreatitis and apoptosis (Abogresha et al., 2016; Tong et al., 2019). The released TNF-α evoked in situ and systemic changes affecting distant organs leading eventually to the recruitment of neutrophils. Neutrophil infiltration to ischemic tissues leads to increased MPO enzymatic activity, that produces massive damage to vascular and parenchymal cells (Kvietys and Granger. 2012; Frangogiannis, 2015). This supports our results which indicates an association between TNF-α level in pancreatic tissues and elevated MPO levels, which goes in line with the correlation studies.
K-ATP channel is a special type of K channels activated by intracellular ATP depletion that occurs during pathological states of hypoxia or ischemia (Szeto et al., 2018). The previous meta-analysis demonstrated its protective effect on the ischemic heart (Zhao et al., 2019), and others proved that activation of K-ATP channels prevents neurodegeneration following cerebral ischemia in rats (Mayanagi et al, 2007; Wang et al., 2011).
Nicorandil has been recognized to have clinical importance as a K-ATP channel activator (Ahmed, 2019). There is growing evidence about its potential therapeutic role against ischemia-reperfusion injury in the heart, in skeletal muscle as well as its role in ischemic stroke (Cahoon et al., 2013; Yang et al., 2015; Sánchez-Duarte et al., 2017; Zhao et al., 2021). Meanwhile, treatment with nicorandil significantly suppresses renal damage after I/R with remarkable improvement in kidney functions.
To date, there have been no reports in the literature exploring the role of nicorandil in pancreatic tissue damage following bilateral renal I/R injury. The cardioprotective effect of nicorandil against ischemic injury has been previously attributed to its anti-inflammatory effects with neutrophil modulation in addition to anti-apoptotic function (Zhao et al., 2014; Li et al., 2015). More specifically, nicorandil could reduce hypoxia-induced apoptosis by activating mitochondrial K-ATP channels, lowering mitochondrial dysfunction and the associated oxidative damage (Ahmed and El-Maraghy. 2013; Afzal et al., 2016).
In the current study, we investigated the protective effect of nicorandil on the pancreas after renal ischemia-reperfusion injury, which to the best of our knowledge is the first time to be investigated. Our results aid further support as nicorandil-treated rats showed a significant reduction in TNF-α expression compared to untreated rats. Additionally, downregulation of Bax and upregulation of Bcl2 expression, were also recorded, indicating the antiapoptotic role of nicorandil. These observations are concordant with the work of Wang and colleagues (Wang et al., 2012) who found that nicorandil injection regulated apoptosis-related proteins before/during ischemia or at the onset of reperfusion. According to previous studies conducted on myocytes, nicorandil can influence cellular proliferation and apoptosis in tissues exposed to hypoxic conditions, these effects are attributed to its ability to activate mitochondrial KATP channels and cGMP-dependent mechanisms (Nagata et al., 2003; Carreira et al., 2008).
Thus, we measured the level of c-GMP in all studied groups, and we found significant increase in c-GMP in NC-treated groups.
In another study conducted in isolated rat lungs exposed to interrupted (ischemia) for 60 min, and then resumed for 60 min nicorandil pretreatment ameliorated increases in pulmonary microvascular permeability secondary I/R. Moreover, the protective effects of nicorandil against I/R lung injury was blocked by glibenclamide as a KATP channel blocker and 1H-[1,2,4] oxadiazolo [4,3-a]quinoxaline-1-one (ODQ) as inhibitors of guanylate cyclase (Abe et al., 2020). These protective effects may indicate the involvement of least two mechanisms, KATP channel opener and the cGMP pathway.
In other treated groups we used MSCs unconditioned and conditioned with nicorandil, in a trial to improve the survival rate of donor MSCs with a multipotential differentiation capacity, that have been widely used in regenerative medicine. In vivo, poor blood supply with limited nutrients in the ischemic area is suggested to be the leading cause for the massive MSCs death (Zhu et al., 2006).
Zhang and co-workers (2015) reported that nicorandil may exert protective effects on MSCs under ischemic conditions by activating mitochondrial K-ATP channels. It has been previously reported that nicorandil has a beneficial effect in dilating micro-vessels and improving myocardial perfusion through its effect as a K-ATP channels opener (Horinaka, 2011). In this study, Using MMT assay, MSCs proliferation was significantly increased after incubation with nicorandil.
Consistent with promoted MSCs proliferation, our findings showed significant improvement in renal function tests after MSCs treatment. Notably, combined treatment with systemic nicorandil and conditioned MSCs may induce better results.
The degree of pancreatic tissue injury detected by estimation of LDH, amylase, and MPO levels in all treated rats was significantly lower compared to untreated rats. Preconditioning of MSCs with nicorandil separately or combined with systemic nicorandil decreased both LDH, amylase levels near control levels. This agrees with Zhang and colleagues (2015) who reported pro-survival effects for nicorandil on MSCs under conditions resembling myocardial ischemia. Injection of bone marrow stem cells in a mouse model of remote liver injury, secondary to renal I/R, has been reported to decrease the inflammatory response and apoptotic marker levels, suggesting a remote regenerative and protective effect on injured organs by releasing factors in the bloodstream (El-Tahawy and Ali. 2017).
The beneficial effect was also observed as a preconditioning therapy, as preconditioned MSCs with nicorandil augmented the MSCs antiapoptotic effect, an observation which is consistent with Zhang and his colleague’s study (2015).
Interestingly, maximum protection occurs with combined treatment. These results suggested that nicorandil can suppress the inflammatory and apoptotic response caused by renal I/R injury.
A special focus has been placed in this study on the signaling pathways of nicorandil during pancreatic protection in I/R. Previous reports showed that mTOR-atypical serine/threonine-protein kinase, which belongs to the phosphoinositide 3 kinase (PI3K)-related kinase family-, plays a central role in regulating many essential cellular processes as cell growth, cell survival, and autophagy. Previous reports indicate that mTOR is involved in ischemic injury, and it was rapidly activated and exerts protective effects during the reperfusion phase (Matsui et al., 2007; Wei et al., 2016; Fan et al., 2017). Accumulating evidence indicates that stimulating PI3K/Akt/mTORC1 pathway may suppress apoptosis in brain tissues, causing neuroprotection against IR injury (Yan et al., 2016; Zhang et al., 2016).
The PI3K/Akt signaling pathway is one of the most essential signaling pathways regulating cell response to various stresses, especially anti-apoptosis, and pro-survival responses (Sun et al., 2017; Handa et al., 2019).
In different ischemia-reperfusion injury mouse models applied on hepatic and cardiac tissues, increased levels of p-PI3K, p-Akt have been recorded, indicating that I/R may activate the PI3K/Akt signaling pathway to initiate tissue repair following injury (Zhang et al., 2014; Thokala et al., 2017). Targeting PI3K/Akt signaling pathway by different pharmacological therapies as a potential protective pathway against I/R injury has attracted great attention in many studies (Yapca et al., 2013; Guan et al., 2020; Chen et al., 2021). In the current study, Western blot analysis showed significant upregulation in PI3K/Akt/mTOR expression in pancreatic tissue after nicorandil injection as a single treatment or combined with preconditioned MSCs. In accordance with our results, Wang’s study (2012) verified that nicorandil can alleviate apoptosis in diabetic cardiomyopathy through PI3K/Akt pathway activation. Moreover, in our in vitro studies, incubation with nicorandil decreased apoptotic markers and enhanced the pro-survival PI3K/Akt/mTOR signaling pathway of MSCs. Therefore, our results give further support to a previous work suggesting a fundamental role for the PI3K/Akt signaling pathway in the nicorandil-mediated protection of MSCs exposed to ischemic conditions (Zhang et al., 2015).
Limitations of the current study are to be considered and future experiments are recommended. Our key findings are that the pancreatic protective effects of nicorandil in bilateral I/R injury were associated with enhancement of both PI3K/AKT/mTOR and c-GMP in I/R groups treated with NC, which suggests their probable involvement in these effects. In addition, NC could enhance MSCs therapeutic potentials via attenuating stem cell apoptosis that was indicated by Bax/Bcl2 levels. Thus, our future experiments should employ knockout models and/or blocker of the PI3K/AKT/mTOR genes with or without 1H-[1,2,4] oxadiazolo [4,3-a] quinoxaline-1-one (ODQ) as an inhibitor of guanylate cyclase, that would more conclusively determine the role of these crucial pathways in bilateral renal I/R-induced pancreatic damage. Additionally, it will provide a more efficient link about the direct involvement of PI3K/AKT/mTOR and/or c-GMP in NC induced pancreatic protection.
In conclusion, bilateral renal I/R in rats resulted in pancreatic injury. This study showed that preconditioning MSCs with nicorandil and using combined nicorandil with MSCC ameliorates renal parameters (serum urea and creatinine) and the accompanied pancreatic damage. As it improved blood glucose level, LDH, serum amylase, and MPO. In addition, MSCs enhance PI3K/Akt/mTORC1 pathway, suppress apoptosis, and increase CD31 expression which essentially denotes improvement in angiogenesis and microcirculation (Figure 9). Our in vitro study showed that nicorandil enhanced MSCs proliferation, augmented pro-survival signaling pathway, and decreased apoptotic markers which strength our results in vivo study. This work supports the view that MSCs pre-conditioning with nicorandil, may introduce a novel treatment in IR injury-induced pancreatic damage which occurs after renal transplantation.
FIGURE 9. Schematic presentation demonstrating pancreatic protection from the remote effect of renal ischemia/reperfusion injury by engaging several protocols. Applying systemic nicorandil, as a pharmacological therapeutic approach, as well as a preconditioning therapy for mesenchymal stem cells, maximizes this protection.
The original contributions presented in the study are included in the article/Supplementary Materials, further inquiries can be directed to the corresponding author.
The animal study was reviewed and approved by Ethics and Scientific Committee, Department of Physiology, Kasr Al Ainy Faculty of Medicine, Cairo University, Egypt.
Conceptualization: AS and NS. Methodology and validation: LR, AM, FH, SE-A, MM, HA, and BA. Data curation: NS, SG, AM, SA, FH, and AS. Writing—original draft preparation: AS, BA, HA, and NS Writing—review and editing: AS, MM, FH, and MG. Supervision, NS. Project administration: AS.
This research was supported and funded by Kasr Alainy, Faculty of Medicine, Cairo University.
The authors declare that the research was conducted in the absence of any commercial or financial relationships that could be construed as a potential conflict of interest.
All claims expressed in this article are solely those of the authors and do not necessarily represent those of their affiliated organizations, or those of the publisher, the editors and the reviewers. Any product that may be evaluated in this article, or claim that may be made by its manufacturer, is not guaranteed or endorsed by the publisher.
All authors offer sincere appreciation for the experimental animal unit, Faculty of medicine-Cairo University for providing rats.
The Supplementary Material for this article can be found online at: https://www.frontiersin.org/articles/10.3389/fphys.2022.934597/full#supplementary-material
Abe K., Horiguchi T., Enzan K., Masaki Y., Nishikawa T., Kimura T. (2020). Nicorandil, a KATP Channel Opener, Attenuates Ischemia-Reperfusion Injury in Isolated Rat Lungs. Lung 198 (2), 315–321. doi:10.1007/s00408-020-00339-0
Abogresha N. M., Greish S. M., Abdelaziz E. Z., Khalil W. F. (2016). Remote Effect of Kidney Ischemia-Reperfusion Injury on Pancreas: Role of Oxidative Stress and Mitochondrial Apoptosis. aoms 2, 252–262. doi:10.5114/aoms.2015.48130
Afzal M. Z., Reiter M., Gastonguay C., McGivern J. V., Guan X., Ge Z.-D., et al. (2016). Nicorandil, a Nitric Oxide Donor and ATP-Sensitive Potassium Channel Opener, Protects Against Dystrophin-Deficient Cardiomyopathy. J. Cardiovasc. Pharmacol. Ther. 21, 549–562. doi:10.1177/1074248416636477
Ahmed L. A., El-Maraghy S. A. (2013). Nicorandil Ameliorates Mitochondrial Dysfunction in Doxorubicin-Induced Heart Failure in Rats: Possible Mechanism of Cardioprotection. Biochem. Pharmacol. 86, 1301–1310. doi:10.1016/j.bcp.2013.07.005
Ahmed L. (2019). Nicorandil: A Drug with Ongoing Benefits and Different Mechanisms in Various Diseased Conditions. Indian J. Pharmacol. 51, 296–301. doi:10.4103/ijp.ijp_298_19
Ashcroft F. M., Gribble F. M. (1999). ATP-Sensitive K + Channels and Insulin Secretion: Their Role in Health and Disease. Diabetologia 42, 903–919. doi:10.1007/s001250051247
Cahoon N. J., Naparus A., Ashrafpour H., Hofer S. O. P., Huang N., Lipa J. E., et al. (2013). Pharmacologic Prophylactic Treatment for Perioperative Protection of Skeletal Muscle from Ischemia-Reperfusion Injury in Reconstructive Surgery. Plastic Reconstr. Surg. 131, 473–485. doi:10.1097/prs.0b013e31827c6e0b
Carreira R. S., Monteiro P., Kowaltowski A. J., Gonçalves L. M., Providência L. A. (2008). Nicorandil Protects Cardiac Mitochondria Against Permeability Transition Induced by Ischemia-Reperfusion. J. Bioenerg. Biomembr. 40 (2), 95–102. doi:10.1007/s10863-008-9133-2
Chen X., Zhang J., Xia L., Wang L., Li H., Liu H., et al. (2021). β-Arrestin-2 Attenuates Hepatic Ischemia-Reperfusion Injury by Activating PI3K/Akt Signaling. Aging (Albany NY) 31, 2251. doi:10.18632/aging.202246
El-Tahawy A., Ali A. (2017). Possible Protective Effect of Bone Marrow-Mesenchymal Stem Cells (BM-MSCs) Against the Remote Liver Injury Induced by Renal Ischemia-Reperfusion in Male Albino Rats. J. Cytol. Histol. 8, 1–14. doi:10.4172/2157-7099.1000484
Erpicum P., Rowart P., Poma L., Krzesinski J. M., Detry O., Jouret F. (2017). Administration of Mesenchymal Stromal Cells Before Renal Ischemia/Reperfusion Attenuates Kidney Injury and May Modulate Renal Lipid Metabolism in Rats. Sci. Rep. 17, 1–3. doi:10.1038/s41598-017-08726-z
Fan W., Han D., Sun Z., Ma S., Gao L., Chen J., et al. (2017). Endothelial Deletion of mTORC1 Protects Against Hindlimb Ischemia in Diabetic Mice via Activation of Autophagy, Attenuation of Oxidative Stress and Alleviation of Inflammation. Free Radic. Biol. Med. 108, 725–740. doi:10.1016/j.freeradbiomed.2017.05.001
Frangogiannis N. G. (2015). Inflammation in Cardiac Injury, Repair, and Regeneration. Curr. Opin. Cardiol. 30, 240–245. doi:10.1097/hco.0000000000000158
Guan B. F., Dai X. F., Huang Q. B., Zhao D., Shi J. L., Chen C., et al. (2020). Icariside II Ameliorates Myocardial Ischemia and Reperfusion Injury by Attenuating Inflammation and Apoptosis Through the Regulation of the PI3K/AKT Signaling Pathway. Mol. Med. Rep. 22, 3151–3160. doi:10.3892/mmr.2020.11396
Handa T., Katayama A., Yokobori T., Yamane A., Fujii T., Obayashi S., et al. (2019). Carboxypeptidase A4 Accumulation Is Associated with an Aggressive Phenotype and Poor Prognosis in Triple-Negative Breast Cancer. Int. J. Oncol. 54, 833–844. doi:10.3892/ijo.2019.4675
Holderied A., Kraft F., Marschner J. A., Weidenbusch M., Anders H. J. (2020). “Point of No Return” in Unilateral Renal Ischemia-Reperfusion Injury in Mice. J. Biomed. Sci. 27, 1–15. doi:10.1186/s12929-020-0623-9
Horinaka S., Kobayashi N., Higashi T., Hara K., Hara S., Matsuoka H. (2001). Nicorandil Enhances Cardiac Endothelial Nitric Oxide Synthase Expression via Activation of Adenosine Triphosphate-Sensitive K Channel in Rat. J. Cardiovasc. Pharmacol. 38, 200–210. doi:10.1097/00005344-200108000-00005
Horinaka S. (2011). Use of Nicorandil in Cardiovascular Disease and its Optimization. Drugs 71, 1105–1119. doi:10.2165/11592300-000000000-00000
Hu H., Zou C. (2017). Mesenchymal Stem Cells in Renal Ischemia-Reperfusion Injury: Biological and Therapeutic Perspectives. Curr. Stem Cell Res. Ther. 12, 183–187. doi:10.2174/1574888x11666161024143640
Hussein A. M., Abd-Elkhabir A., Abozahra A., Baiomy A., Ashamallah S. A., Sheashaa H. A., et al. (2014). Pancreatic Injury Secondary to Renal Ischemia/Reperfusion (I/R) Injury: Possible Role of Oxidative Stress. Physiol. Res. 63, 47–55. doi:10.33549/physiolres.932497
Kocaturk H., Bedir F., Altay M. S., Bakan E., Suleyman B., Yazici G. N., et al. (2020). The Effect of Desloratadine on Ischemia Reperfusion-Induced Oxidative and Inflammatory Renal Injury in Rats. Ren. Fail 42, 531–538. doi:10.1080/0886022x.2020.1769656
Kvietys P. R., Granger D. N. (2012). Role of Reactive Oxygen and Nitrogen Species in the Vascular Responses to Inflammation. Free Radic. Biol. Med. 52, 556–592. doi:10.1016/j.freeradbiomed.2011.11.002
Li W., Wu N., Shu W., Jia D., Jia P. (2015). Pharmacological Preconditioning and Postconditioning with Nicorandil Attenuates Ischemia/Reperfusion-Induced Myocardial Necrosis and Apoptosis in Hypercholesterolemic Rats. Exp. Ther. Med. 10, 2197–2205. doi:10.3892/etm.2015.2782
M El Agaty S., Ahmed A. I. (2020). Pathophysiological and Immunohistochemical Analysis of Pancreas After Renal Ischemia/Reperfusion Injury: Protective Role of Melatonin. Arch. Physiol. Biochem. 126, 264–275. doi:10.1080/13813455.2018.1517182
Mahmood T., Yang P. C. (2012). Western Blot: Technique, Theory, and Troubleshooting. N. Am. J. Med. Sci. 4, 429–434. doi:10.4103/1947-2714.100998
Matsui Y., Takagi H., Qu X., Abdellatif M., Sakoda H., Asano T., et al. (2007). Distinct Roles of Autophagy in the Heart During Ischemia and Reperfusion: Roles of AMP-Activated Protein Kinase and Beclin 1 in Mediating Autophagy. Circulation Res. 100, 914–922. doi:10.1161/01.res.0000261924.76669.36
Mayanagi K., Gáspár T., Katakam P., Kis B., Busija D. (2007). The Mitochondrial K(ATP) Channel Opener BMS- 191095 Reduces Neuronal Damage After Transient Focal Cerebral Ischemia in Rats. J. Cereb. Blood Flow Metabolismofficial J. Int. Soc. Cereb. Blood Flow Metabolism 27, 348–355. doi:10.1038/sj.jcbfm.9600345
Nagata K., Obata K., Odashima M., Yamada A., Somura F., Nishizawa T., et al. (2003). Nicorandil Inhibits Oxidative Stress-Induced Apoptosis in Cardiac Myocytes Through Activation of Mitochondrial ATP-Sensitive Potassium Channels and a Nitrate-Like Effect. J. Mol. Cell. Cardiol. 35 (12), 1505–1512. doi:10.1016/j.yjmcc.2003.09.018
Nichols C. G. (2016). Adenosine Triphosphate-Sensitive Potassium Currents in Heart Disease and Cardioprotection. Card. Electrophysiol. Clin. 8, 323–335. doi:10.1016/j.ccep.2016.01.005
Sánchez-Duarte E., Trujillo X., Cortés-Rojo C., Saavedra-Molina A., Camargo G., Hernández L., et al. (2017). Nicorandil Improves Post-fatigue Tension in Slow Skeletal Muscle Fibers by Modulating Glutathione Redox State. J. Bioenergetics Biomembr. 49, 159–170. doi:10.1007/s10863-016-9692-6
Shimizu S., Saito M., Kinoshita Y., Ohmasa F., Dimitriadis F., Shomori K., et al. (2011). Nicorandil Ameliorates Ischemia-Reperfusion Injury in the Rat Kidney. Br. J. Pharmacol. 163, 272–282. doi:10.1111/j.1476-5381.2011.01231.x
Struck M. B., Andrutis K. A., Ramirez H. E., Battles A. H. (2011). Effect of a Short-Term Fast on Ketamine-Xylazine Anesthesia in Rats. J. Am. Assoc. Lab. Anim. Sci. 50, 344–348.
Sun L., Guo C., Burnett J., Pan J., Yang Z., Ran Y., et al. (2017). Association Between Expression of Carboxypeptidase 4 and Stem Cell Markers and Their Clinical Significance in Liver Cancer Development. J. Cancer 8, 111. doi:10.7150/jca.17060
Szeto V., Chen N. H., Sun H. S., Feng Z. P. (2018). The Role of KATP Channels in Cerebral Ischemic Stroke and Diabetes. Acta Pharmacol. Sin. 39, 683–694. doi:10.1038/aps.2018.10
Thokala S., Inapurapu S., Bodega V. L., Vemuri P. K., Bodiga S. (2017). Loss of ErbB2-PI3K/Akt Signaling Prevents Zinc Pyrithione-Induced Cardioprotection During Ischemia/Reperfusion. Biomed. Pharmacother. 88, 309–324. doi:10.1016/j.biopha.2017.01.065
Tong F., Tang X., Liu D. (2019). Phycocyanin/PEG-b-(PG-g-PEI) Attenuated Hepatic Ischemia/Reperfusion-Induced Pancreatic Islet Injury and Enlarged Islet Functionality. Int. J. Nanomedicine 14, 339–351. doi:10.2147/ijn.s190938
Wanas H., El Shereef Z., Rashed L., Aboulhoda B. E. (2022). Ticagrelor Ameliorates Bleomycin-Induced Pulmonary Fibrosis in Rats by Inhibition of TGF-β1/Smad3 and PI3K/AKT/mTOR Pathways. Curr. Mol. Pharmacol. 15, 227–238. doi:10.2174/1874467214666210204212533
Wang A., Chen F., Xie Y., Guo Z., Yu Y. (2012). Protective Mechanism of Nicorandil on Rat Myocardial Ischemia-Reperfusion. J. Cardiovasc Med. Hagerst. 13, 511–515. doi:10.2459/jcm.0b013e3283542031
Wang L., Zhu Q. L., Wang G. Z., Deng T. Z., Chen R., Liu M. H., et al. (2011). The Protective Roles of Mitochondrial ATP-Sensitive Potassium Channels During Hypoxia–Ischemia-Reperfusion in Brain. Neurosci. Lett. 491, 63–67. doi:10.1016/j.neulet.2010.12.065
Wei H., Li Y., Han S., Liu S., Zhang N., Zhao L., et al. (2016). cPKCγ-Modulated Autophagy in Neurons Alleviates Ischemic Injury in Brain of Mice with Ischemic Stroke Through Akt-mTOR Pathway. Transl. stroke Res. 7, 497–511. doi:10.1007/s12975-016-0484-4
Yan W., Chen Z., Chen J., Chen H. (2016). Isoflurane Preconditioning Protects Rat Brain from Ischemia-Reperfusion Injury via Up-Regulating the HIF-1alpha Expression Through Akt/mTOR/s6K Activation. Cell Mol. Biol. (Noisy-le-grand) 62, 38–44.
Yang J., Zhang J., Cui W., Liu F., Xie R., Yang X., et al. (2015). Cardioprotective Effects of Single Oral Dose of Nicorandil Before Selective Percutaneous Coronary Intervention. Anatol. J. Cardiol. 15, 125. doi:10.5152/akd.2014.5207
Yang X., Zhang X., Lin Z., Guo J., Yang X., Yao L., et al. (2020). Chaiqin Chengqi Decoction Alleviates Severe Acute Pancreatitis Associated Acute Kidney Injury by Inhibiting Endoplasmic Reticulum Stress and Subsequent Apoptosis. Biomed. Pharmacother. 125, 110024. doi:10.1016/j.biopha.2020.110024
Yapca O. E., Borekci B., Suleyman H. (2013). Ischemia-Reperfusion Damage. Eurasian J. Med. 45, 126–127. doi:10.5152/eajm.2013.24
Yu C., Li W. B., Liu J. B., Lu J. W., Feng J. F. (2018). Autophagy: Novel Applications of Nonsteroidal Anti-Inflammatory Drugs for Primary Cancer. Cancer Med. 7, 471–484. doi:10.1002/cam4.1287
Yuan X., Juan Z., Zhang R., Sun X., Yan R., Yue F., et al. (2020). Clemastine Fumarate Protects Against Myocardial Ischemia Reperfusion Injury by Activating the TLR4/PI3K/Akt Signaling Pathway. Front. Pharmacol. 11, 28. doi:10.3389/fphar.2020.00028
Zatroch K. K., Knight C. G., Reimer J. N., Pang D. S. (2017). Refinement of Intraperitoneal Injection of Sodium Pentobarbital for Euthanasia in Laboratory Rats (Rattus norvegicus). BMC Vet. Res. 13, 60. doi:10.1186/s12917-017-0982-y
Zhang F., Cui J., Lv B., Yu B. (2015). Nicorandil Protects Mesenchymal Stem Cells Against Hypoxia and Serum Deprivation-Induced Apoptosis. Int. J. Mol. Med. 36, 415–423. doi:10.3892/ijmm.2015.2229
Zhang P., Guo Z. F., Xu Y. M., Li Y. S., Song J. G. (2016). N-Butylphthalide (NBP) Ameliorated Cerebral Ischemia Reperfusion-Induced Brain Injury via HGF-Regulated TLR4/NF-Kappa B Signaling Pathway. Biomed. Pharmacother. 83, 658–666. doi:10.1016/j.biopha.2016.07.040
Zhang R., Zhang L., Manaenko A., Ye Z., Liu W., Sun X. (2014). Helium Preconditioning Protects Mouse Liver Against Ischemia and Reperfusion Injury Through the PI3K/Akt Pathway. J. Hepatol. 61, 1048–1055. doi:10.1016/j.jhep.2014.06.020
Zhao A. P., Dong Y. F., Liu W., Gu J., Sun X. L. (2014). Nicorandil Inhibits Inflammasome Activation and Toll-Like Receptor-4 Signal Transduction to Protect Against Oxygen-Glucose Deprivation-Induced Inflammation in BV-2 Cells. CNS Neurosci. Ther. 20, 147–153. doi:10.1111/cns.12178
Zhao X. T., Zhang C. F., Liu Q. J. (2019). Meta-Analysis of Nicorandil Effectiveness on Myocardial Protection After Percutaneous Coronary Intervention. BMC Cardiovasc. Disord. 19, 144. doi:10.1186/s12872-019-1071-x
Zhao Y., Yang Z., He Y., Sun R., Yuan H. (2021). The KATP Channel Opener, Nicorandil, Ameliorates Brain Damage by Modulating Synaptogenesis After Ischemic Stroke. PLoS ONE 16, e0246019. doi:10.1371/journal.pone.0246019
Zhou F., Yao H. H., Wu J. Y., Ding J. H., Sun T., Hu G. (2008). Opening of Microglial K(ATP) Channels Inhibits Rotenone-Induced Neuroinflammation. J. Cell Mol. Med. 12, 1559–1570. doi:10.1111/j.1582-4934.2007.00144.x
Keywords: MSc, nicorandil, bilateral renal I/R, PI3K/AKT/mTOR, pancreatic damage
Citation: ShamsEldeen AM, El-Aal SAA, Aboulhoda BE, AbdAllah H, Gamal SM, Hassan FE, Mehesen MN, Rashed LA, Mostafa A and Sadek NB (2022) Combined Systemic Intake of K-ATP Opener (Nicorandil) and Mesenchymal Stem Cells Preconditioned With Nicorandil Alleviates Pancreatic Insufficiency in a Model of Bilateral Renal Ischemia/Reperfusion Injury. Front. Physiol. 13:934597. doi: 10.3389/fphys.2022.934597
Received: 02 May 2022; Accepted: 31 May 2022;
Published: 23 June 2022.
Edited by:
Andrei Krivtsov, Dana–Farber Cancer Institute, United StatesReviewed by:
Jorge Sanchez, Instituto Politécnico Nacional de México (CINVESTAV), MexicoCopyright © 2022 ShamsEldeen, El-Aal, Aboulhoda, AbdAllah, Gamal, Hassan, Mehesen, Rashed, Mostafa and Sadek. This is an open-access article distributed under the terms of the Creative Commons Attribution License (CC BY). The use, distribution or reproduction in other forums is permitted, provided the original author(s) and the copyright owner(s) are credited and that the original publication in this journal is cited, in accordance with accepted academic practice. No use, distribution or reproduction is permitted which does not comply with these terms.
*Correspondence: Asmaa Mohammed ShamsEldeen, YXNtYWE4MnNoYW1zZWxkZWVuQGdtYWlsLmNvbQ==, QXNtYWEuQWJkdWxXYWhhYkBrYXNyYWxhaW55LmVkdS5lZw==, ZHJfYXNtYWFzaGFtczgyQGN1LmVkdS5lZA==, b3JjaWQub3JnLzAwMDAtMDAwMi00MjUwLTA4MTg=
Disclaimer: All claims expressed in this article are solely those of the authors and do not necessarily represent those of their affiliated organizations, or those of the publisher, the editors and the reviewers. Any product that may be evaluated in this article or claim that may be made by its manufacturer is not guaranteed or endorsed by the publisher.
Research integrity at Frontiers
Learn more about the work of our research integrity team to safeguard the quality of each article we publish.