- 1State Key Laboratory of Cotton Biology, School of Life Sciences, College of Agriculture, Henan University, Kaifeng, China
- 2Institute of Sericulture and Tea Research, Zhejiang Academy of Agricultural Sciences, Hangzhou, China
- 3Key Laboratory of Insect Developmental and Evolutionary Biology, CAS Center for Excellence in Molecular Plant Sciences/Institute of Plant Physiology and Ecology, Shanghai, China
Male fertility is essential for reproduction and population growth in animals. Many factors affect male fertility, such as courtship behavior, sperm quantity, and sperm motility, among others. Seminal Fluid Proteins (SFPs) are vital components of seminal fluid in the male ejaculate, which affect male fertility, sperm activation, and female ovulation. However, the knowledge of SFPs is insufficient; the function of many SFPs remains unknown, and most described functions were mainly characterized in Drosophila or other laboratory models. Here, we focus on the Serine protease 2 (Ser2) gene in the lepidopteran pest Spodoptera litura. The Ser2 gene was specifically expressed in male adults. Disruption of the Ser2 gene mediated by CRISPR/Cas9 induced male sterility but females remained fertile. PCR-based detection of the next-generation mutants showed that male sterility was stably inherited. The qRT-PCR analysis of SlSer2 mutants showed that motor protein family genes and structural protein family genes were down-regulated, while protein modification family genes were up-regulated, suggesting that SlSer2 may be involved in sperm movement and activity. These results demonstrate that Ser2 is an important component of SFPs in seminal fluid and was identified for a useful sterile gene for pest control that may lead to new control strategies for lepidopteran insect pests such as S. litura.
Introduction
The common cutworm, Spodoptera litura (Lepidoptera: Noctuidae) is one of the most destructive phytophagous pests of crops such as tea, tobacco, and vegetables, which causes serious losses of yield and quality of crops in China and other Southeast Asian countries (Rao et al., 1993; Qin et al., 2004; Meagher et al., 2008; Muthusamy et al., 2014). Chemical insecticides are the most commonly used control method for S. litura (Ayyanna et al., 1982). Resistance to chemical insecticides has become a serious problem in insect pests including S. litura (Ahmad et al., 2008; Ahmad et al., 2009; Shad et al., 2010). Moreover, incorrect use of certain pesticides has very serious implications for food security and human health, raising awareness of their eco-environmental and human impacts (Kaur et al., 2014; Rehan et al., 2014). Therefore, alternative species-specific and co-friendly pest management strategies are needed.
The CRISPR/Cas9 system is an effective genome editing tool with potential application in pest management (Wang et al., 2013; Alphey, 2014; Esvelt et al., 2014; Gantz et al., 2015; Alphey, 2016). CRISPR/Cas9 has been used to edit the genomes of numerous eukaryotic organisms (Cong et al., 2013; Hwang et al., 2013), model insect species in the orders Diptera (Bassett et al., 2013; Hall et al., 2015) and Coleoptera (Gillies et al., 2015). In Lepidoptera, CRISPR/Cas9 has been used to analyze gene functions (Liu et al., 2017; Xu et al., 2017; Zeng et al., 2017), to enhance antiviral responses (Chen et al., 2017), to use the silkworm as a bioreactor for important protein products (Xu et al., 2018), and to control female-specific embryonic lethality (Zhang et al., 2018). In S. litura, the CRISPR/Cas9 system has worked efficiently to investigate some gene functions (Bi et al., 2016; Zhu et al., 2016; Bi et al., 2019; Du et al., 2019). But how best to use this powerful biotechnology and select suitable and efficient targeted genes to control S. litura is a significant problem.
The male seminal fluid is a complex medium, that contains many molecules and complex components, such as seminal fluid proteins (SFPs), produced mainly by sex accessory glands (Poiani et al., 2006; Sirot et al., 2014; McGraw et al., 2015). In the mating behavior of insects, SFPs are transferred from males to females through ejaculation, which has possible benefits including sperm capacitation, sperm competition and fertilization, and plays a crucial role in reproductive success (Chapman et al., 2001; Avila et al., 2011; Denis et al., 2017; Taniguchi et al., 2018; Karr et al., 2019). In Drosophila melanogaster, RNAi-mediated knockdown of a type of SFP, Seminase, a predicted serine protease, results in a decrease of eggs and an inability to store sex peptides (LaFlamme et al., 2012). Seminase initiates the protease cascaded signaling pathway by causing proteases to hydrolyze accessory gland proteins (Acps), thus participating in the early regulatory process of the post-mating processes (Laflamme and Wolfner, 2013). Previous studies have shown that serine protease is an important enzyme that promotes sperms to produce energy resources for sperm motility, and its absence affects fertilization success (Nagaoka et al., 2012). Using CRISPR/Cas9 technology to knockout of one of the serine protease gene, Serine protease 2 (Ser2), led to male sterility but did not affect female sterility in Bombyx mori and Plutella xylostella (Xu et al., 2020).
Here, we investigate the function of the Ser2 gene in S. litura. Using the CRISPR/Cas9 genome editing system, we successfully knocked out the Ser2 gene. Ser2 disruption induced male specific sterility in adults with few normal hatched individuals in the next generation. The novel phenotype of male specific sterility showed Ser2 was an important gene in the sperm development process in S. litura. The Sterile insect technology (SIT), which needs to release the sterile insects into the wild and mate with wild type insects that induces the insects sterility, is one of valuable and environmentally friendly pest control approach in lepidopteran and dipteran insects (Tan et al., 2013). Our data indicates that SlSer2, which regulates the fertility of male adults and could decrease the population quantity of pests, through releasing male or female mutants, is a potential male specific sterility gene for using in the control of S. litura and other lepidopteran pests.
Materials and methods
Insect strains and rearing
A laboratory strain of common cutworm, S. litura, was obtained from the College of Plant Protection, Nanjing Agricultural University. Larvae were provided with an artificial diet (Supplementary Table S1) and were kept at 26°C with 80% relative humidity and a 12:12 light:dark photoperiod. Adults were fed 10% honey and kept at 25°C with 80% relative humidity (Bi et al., 2019).
Cloning of SlSer2 and conservative analysis
To identify the SlSer2 gene sequence, based on a homologous gene sequence aligning approach, the B. mori Ser2 sequence (NP_001153675.1, NCBI) was used to search for the S. litura homolog Ser2 sequence using local Protein Basic Local Alignment Search Tool (BLAST) of S. litura protein database. According to the genome sequence of S. litura (Cheng et al., 2017), the related sequence of SlSer2 was found and designed primers to amplify, used the Polymerase Chain Reaction (PCR).
Total RNA was isolated from fifth instar larvae using Trizol Reagent (Invitrogen, Carlsbad, CA, United States) and treated with RNase-free DNase I (Ambion, Austin, TX, United States) according to the manufacturer’s protocol. cDNAs were synthesized with the Omniscript reverse transcriptase kit (Qiagen, Hilden, Germany) in a 20-μl reaction mixture containing 1 μg total RNA per the manufacturer’s instruction. SlSer2 cDNA fragments were amplified by PCR with the following pair of primers (Supplementary Table S2). PCR was carried out using KOD plus polymerase (TOYOBO, Osaka, Japan) under the following conditions: 98°C for 2 min, followed by 30 cycles at 98°C for 30 s, 55°C for 30 s, and 68°C for 1 min, and an elongation phase at 68°C for 10 min. Amplified products were sequenced after cloning into a PJET1.2-T vector (Fermentas, Burlington, ON, Canada).
The multiple alignment and conservative analysis were used DNAMAN 8.0 software, including the putative SER2 protein of S. litura and the other eight lepidopteran SER2 or homologous amino acids sequences (Supplementary Table S2). The GenBank accession numbers of the protein sequence are as follows: B. mori (NP_001153675.1), Agrius convolvuli (BAK52270.1), Samia ricini (BAL04890.1), Pieris rapae (XP_022113521.1), Helicoverpa armigera (XP_021195380.1), Papilio machaon (XP_014359308.1), and P. xylostella (XP_011553524.1), Hyphantria cunea (Li L. et al., 2022).
Expression profile analysis of SlSer2
To investigate the spatio-temporal distribution of SlSer2, total RNA was isolated from each developmental stage, including eggs, the first day of each larval instar, pupae (P), adults (A), and tissues/organs in the male on the third day of the fifth instar larval (L5D3) stage, including head, the epidermis (EPI), fat body (FB), trachea, foregut (FG), midgut (MG), hindgut (HG), and testis (TE), using Trizol reagent (Invitrogen, Carlsbad, CA, United States) and treated with RNase-free DNase I (Ambion, Austin, TX, United States) according to the manufacturer’s protocols. cDNAs were synthesized using the Omniscript reverse transcriptase kit (Qiagen, Hilden, Germany) in a 20-μl reaction mixture containing 1 μg total RNA from a mixture of equal amounts of three RNA samples from each developmental stage. qRT-PCR analysis for Ser2 was performed using a SYBR Green Realtime PCR Master Mix (Thermo Fisher, Waltham, MA, United States) on an Eppendorf Real-time PCR System. The PCR conditions were as follows: initial incubation at 95°C for 5 min, 35 cycles at 95°C for 15 s and 60°C for 1 min qPCR reactions were carried out using gene-specific primers to amplify a 208-bp fragment. Another pair of primers, Actin-qF and Actin-qR (Supplementary Table S2), was used to amplify a 159-bp fragment of SlActin as an internal control.
In vitro transcription of Cas9 mRNA and sgRNA
Two 23-bp sgRNAs were selected to target SlSer2. Each sgRNA was sub-cloned into the 500-bp linearized CloneJet PJET1.2-T vector (Thermo Fisher, Waltham, MA, United States) upstream of the protospacer adjacent motif (PAM) sequence to allow sgRNA expression under the control of the T7 promoter. The sgRNA was synthesized in vitro with a MEGAScript T7 kit (Ambion, Austin, TX, United States) according to the manufacturer’s instructions. Cas9 mRNA was synthesized in vitro using an mMESSAGE T7 Kit (Ambion, Austin, TX, United States) with a PTD1-T7-Cas9 vector as the template (Wang et al., 2013) according to the manufacturer’s instructions.
Microinjection of embryos
Female S. litura moths were allowed to lay eggs on transparent plastic bags. A previously reported microinjection method was employed (Bi et al., 2016). Within 1 h after oviposition, eggs were injected on the lateral side with 10 nl of a mixture containing 300 ng/μl of Cas9 mRNA and 150 ng/μl of each sgRNA. After injection, eggs were incubated in a humidified chamber at 25°C for 4 days until hatching.
Genomic DNA extraction and identification of mutagenesis
The genomic DNA was extracted from newly hatched larvae and adult legs, incubated with proteinase K, and purified via a standard phenol: chloroform extraction and isopropanol precipitation, followed by RNaseA treatment. PCR was carried out to identify SlSer2 mutant alleles using primers F2 and R1 (Supplementary Table S2) spanning the target site in SlSer2. The PCR conditions were as follows: 98°C for 2 min, followed by 35 cycles of 94°C for 10 s, 55°C for 30 s, and 72°C for 1 min, followed by a final extension period of 72°C for 10 min. The PCR products were cloned into pJET1.2-T vectors (Fermentas, Burlington, ON, Canada) and sequenced. The adults and eggs of SlSer2 mutants were photographed with a digital stereoscope (Nikon AZ100, Tokyo, Japan).
Mating behavior analysis and hatchability assay
In order to evaluate mating behavior and hatchability of mutants, the mutants of SlSer2 male and female were crossed with mutant moth and virgin wild type male or female moths. Five pairs of moths were collected for one group. Mating behavior analysis and hatchability assay of each group were repeated three times. The behavioral assays were performed in the transparent plastic bag for one pair. After female moths laid eggs, the eggs of each pair were collected and incubated in a humidified chamber at 25°C for 4 days until hatching. The morphological investigations of mating behavior and egg masses were used the microscope (Nikon AZ100, Tokyo, Japan).
Statistical analysis
A two-tailed Student’s t-test was used to analyze differences between wild-type and mutant individuals. Three independent replicates were used for each treatment and error bars showed the means ± SEM.
Results
Identification and characterization of the SlSer2 gene
The SlSer2 gene was cloned and sequenced by the Sanger sequencing method (Supplementary Figure S1). The 594-bp SlSer2 gene consists of three exons and encodes a putative 197-amino acid protein (Figure 2A). Sequence analysis and multiple alignment showed SlSER2 protein has the trypsin-like serine protease domain, which is high conserved with other lepidopteran insects (Supplementary Figure S2). From qRT-PCR results, SlSer2 gene was hardly expressed in larval stages, but its expression increased from pupal to adult stages (Figure 1A). Remarkably, SlSer2 was most highly expressed in the male adult stage, which was similar to other lepidopteran insects, including B. mori (Xu et al., 2020), P. xylostella (Xu et al., 2020), and H. cunea (Li X. et al., 2022). Moreover, the Ser2 gene was most highly expressed in the trachea, hindgut and testis (Figure 1B). The spatial and temporally specific expression of SlSer2 provided a molecular basis for further functional analysis.
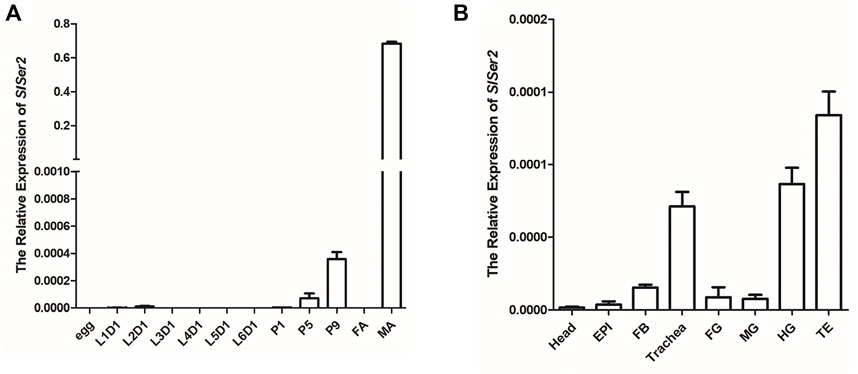
FIGURE 1. The expression patterns of SlSer2 in different developmental stages and various tissues. (A) Relative mRNA expression of SlSer2 in different developmental stages, including egg, the first day of each instar of larva (L1D1, L2D1, L3D1, L4D1, L5D1, and L6D1), pupa (P1, P5, and P9), female adult (FA), and male adult (MA). (B) Relative mRNA expression of SlSer2 in the eight reproductive tissues at the third day of the fifth instar larval (L5D3) stage, including head, epidermis (EPI), fat body (FB), trachea, foregut (FG), midgut (MG), hindgut (HG), and testis (TE).
CRISPR/Cas9 mediated the Ser2 gene mutation in Spodoptera litura
To investigate the function of the SlSer2 gene, we employed the CRISPR/Cas9 system to knockout this gene. Following the single guide RNA (sgRNA) design rule (Wang et al., 2013), we transcribed two sgRNAs in vitro targeting the second and third exons in the Ser2 genome locus (Figure 2A). Using the embryo microinjection system, we injected 150 ng/μl for each sgRNA and 300 ng/μl Cas9 mRNA into eggs, laid less than 1 h before (Table 1). In order to detect the efficient of SlSer2 sgRNAs timely, when these eggs hatched, the genomic DNA of the larvae was extracted and used Sanger sequencing to detect any mutated sequences. Sequencing chromatograms of the PCR product from injected eggs showed that sgRNAs of the SlSer2 gene were effective (Figures 2B,C). Sequencing and mutagenesis analysis revealed that there were diverse deletion mutations of SlSer2 genome sequences (Figure 2D).
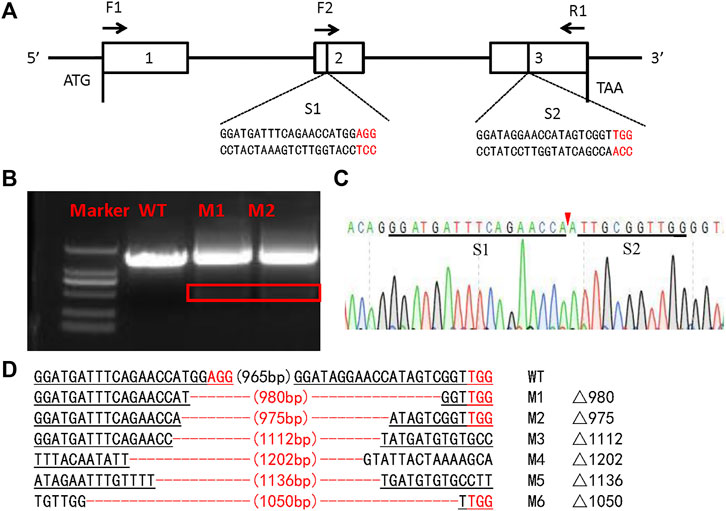
FIGURE 2. The schematic of SlSer2 target sites and CRISPR/Cas9 mediated mutations. (A) Genomic structure of the Ser2 gene in Spodoptera litura (SlSer2). The sgRNA targeting sequences (S1 and S2) are in black text and the protospacer adjacent motif (PAM) sequences are in red. The approximate locations of amplification primers (F2 and R1). (B) Agarose gel electrophoresis of PCR products used for initial detection of the mutations in the SlSer2 gene. The red box represents the deleted sequence fragment of SlSer2 mutants. (C) Sequencing chromatogram of SlSer2 mutants. The black line represents the targeted region. The red wedge indicates position of cleavage by CRISPR/Cas9 genome editing system. (D) Mutations detected by sequencing. The PAM sequence is in red. The black line represents the target sites.
Knockout of SlSer2 results in male adult sterility
Considering the conserved function of Ser2 gene linked to the male reproduction success in other lepidopteran insects (Xu et al., 2020; Li L. et al., 2022), we conducted the mating behavior analysis and hatchability assay. To identify the adult sterility of male mutants, we separated the male and female pupae of wild type and mutants respectively before the adult stage, which can prevent mating with each other and hold the virgin stage. When the hatched larvae grew into adults, a transparent plastic bag kept one pair group to mate with each other and lay eggs inside the plastic bag. The different mating groups included wild-type male mated with wild-type female, wild-type male mated with mutant female, wild-type female mated with mutant male and mutant male mated with mutant female. In both cases, the mating behavior was non-distinctive, and wild type and mutants of either sex could mate with each other successfully and rapidly (Figure 3A). However, the next generation of eggs produced by Ser2 mutants were nearly all unhatched (Figure 3B). In the G0 generation, the wild type males could mate with wild type females and △Ser2 females normally, and whether wild females or △Ser2 females can lay normally hatched egg masses (Figure 3C). But when the △Ser2 males mated with wild type females and △Ser2 females, the hatching rate of egg masses produced by both types of females was lower (Figure 3C).
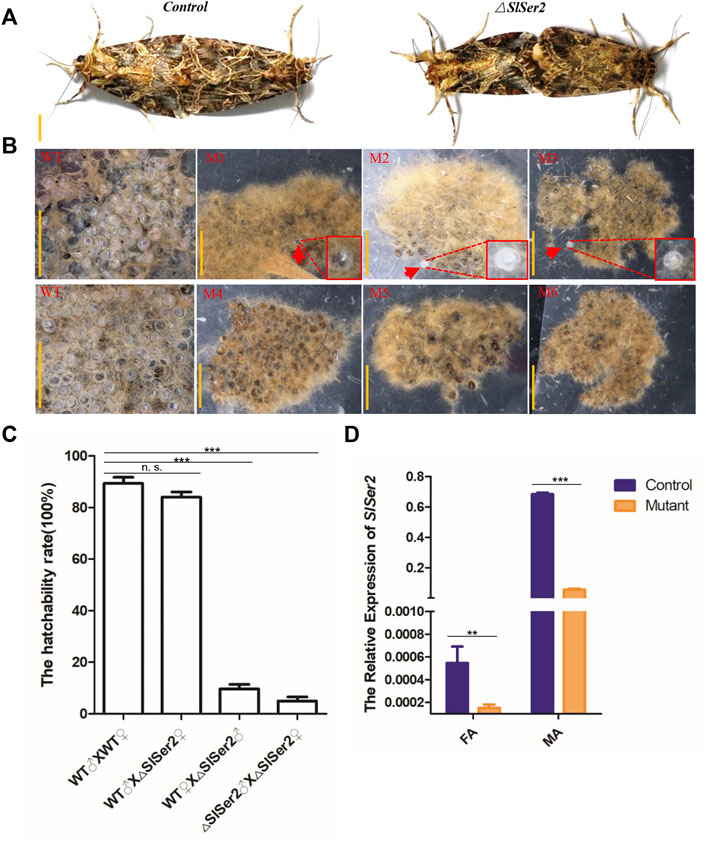
FIGURE 3. Loss of SlSer2 function results in male sterility. (A) The wild type and Ser2 mutants can mate with each other normally. Scale bar: 0.5 cm. (B) CRISPR/Cas9-mediated disruption of Ser2 induced male sterility. WT, wild type egg masses. M1-M6, Ser2 mutant egg masses. Scale bar: 2 mm. (C) The hatchability rate between wild type and mutants. (D) The relative mRNA expression of SlSer2 in wild type and Ser2 mutants. mRNA expression was normalized to SlActin. FA, female adult; MA, male adult. The data shown are means ± S.E.M. Asterisks indicate significant differences with a two-tailed t-test: *p < 0.05; **p < 0.01; ***p < 0.001; n. s. p > 0.05.
To confirm adult genotypes, we used qRT-PCR to detect relative expression of the Ser2 gene in presumptive male and female mutants. These results showed that Ser2 expression was significantly down-regulated in the presumptive mutants, compared with wild type males and females (Figure 3D). Furthermore, using the extracted genomic DNA from legs of mutants, PCR program-based sequencing results of the male and female individuals with the male-specific sterile genotypes, showed different deletion types in the Ser2 loci (Supplementary Figure S3). These data demonstrated that disruption of the SlSer2 gene can cause male-specific sterility in S. litura.
Male sterility is stably heritable in the next generation
In order to investigate the heritability of the phenotype of male sterility, SlSer2 female mutants confirmed by PCR mated with wild type males. We found the progeny of the SlSer2 female mutants could normally hatch and grow. Subsequently, when the next generation individuals developed to the adult stage, we extracted the DNA from each male insect and confirmed the mutant genotype through directed PCR (Figure 4A). Representative sequencing chromatograms of PCR products indicated that the mutants were chimeric. For example, the bottom chromatograms shown in Figure 4B have multiple peaks that indicate the occurrence of more than one nucleotide at a single locus. Ten male mutants mated with the wild type female adults to examine their reproductive status. The statistical result of hatchability showed that only about 10% eggs of female adults could hatch normally, which mated with male mutants and laid eggs (Figure 4C). Thus, these results showed that the male sterility induced by disrupting the SlSer2 gene was stably heritable in the next generation.
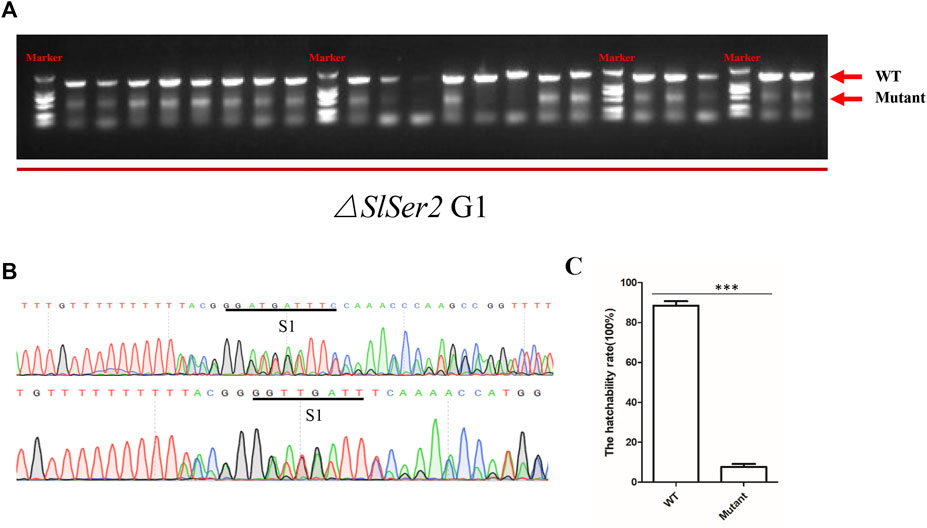
FIGURE 4. Male sterility is heritable stably in the next generation. (A) The PCR detection of mutations in G1 adult males. Arrows indicate band sizes. The upper major band is WT. Different size bands appear in each sample due to induction of different deletions and chimerism. (B) Representative sequencing chromatograms of PCR products of chimeric mutants. The bottom chromatogram shows peaks with multiple nucleotides at some positions (S1), indicating the occurrence of mutant and wild type sequences at the same loci. (C) The offspring hatchability rate of G1 male adult mutants. The data shown are means ± S.E.M. Asterisks indicate significant differences with a two-tailed t-test: ***p < 0.001.
Mutation of the SlSer2 gene affected the relative expression of some other genes
To investigate the underlying reason for the SlSer2 male sterility phenotypes, we used qRT-PCR to analyze the expression of a series of SFP genes involved in protein modification, motor proteins, and structural proteins, which shown to be critical for sperm function (Mcgraw et al., 2004). We selected some important function and potential regulation genes, including defense and immunity genes: attacin-like (XM_022981696.1), cecropin (XM_022971764.1); enzyme genes: lysozyme-like (XM_022959065.1), lysozyme (XM_022981495.1), uricase (XM_022965214.1); motor protein genes: actin muscle (XM_022981497.1), myosin light chain alkali (XM_022970316.1); protease genes: trypsin alkaline C-like (XM_022965904.1), flightin (XM_022976987.1) and calcium binding gene: alpha-amylase 2-like (XM_022958360.1) (Mcgraw et al., 2004). Compared with the wild type, the Myosin light chain alkali (Mlc-A) gene and Actin muscle (Act-M) gene, which are involved in the motor protein family, were down-regulated (Figure 5). The structural protein family gene Flightin was also significantly down-regulated compared with wild-type. In contrast, genes in the protease family, including the Lysozyme-like gene, Uricase gene and Trypsin alkaline C-like gene, were up-regulated in Ser2 mutants. According to these qRT-PCR results, the SlSer2 may regulate the sperm movement and activity to affect the male fertility in S. litura.
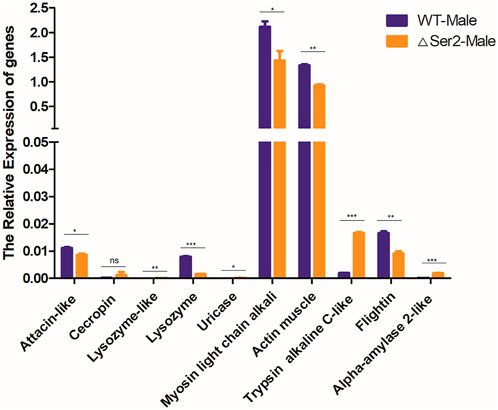
FIGURE 5. Mutation of the SlSer2 gene affects relative expression of genes involved in adult stage. Levels of indicated mRNAs in SlSer2 mutants relative to wild-type levels. Three individual biological replicates of real-time PCR were performed. The asterisks (** or ***) indicate the significant differences (p < 0.01 or p < 0.001, respectively) compared with the wild type adult with a two-tailed t-test.
Discussion
In this study, we investigated the function of the Ser2 gene in the non-model insect S. litura. The Ser2 gene belongs to the SFPs family. The SFPs family is vital to male fertility (Avila et al., 2011; Rodriguezmartinez et al., 2011; Laflamme et al., 2013) and plays a significant role in sperm activation and storage (Neubaum et al., 1999; Sirot et al., 2011; Nagaoka et al., 2012; Zhao et al., 2012); ovulation (Marshall et al., 2009; Xu et al., 2011); female immune function (Guerin et al., 2011), and post-mating behaviors in female insects (Sirot et al., 2009; Laflamme et al., 2012).
The SlSer2 gene is 594-nucleotides long and consists of three exons (Figure 2). It encodes a putative 197-amino acid protein (Supplementary Figure S1). Multiple alignment showed that SlSER2 protein has the conservative Trypsin-like serine protease domain with 85% homology with other SER2 protein sequences (Supplementary Figure S2). We found that SlSer2 was specifically expressed in the testis and adult male stage (Figure 1). As an important and high-efficiency genome editing technique, we successfully used the CRISPR/Cas9 system to knock out the Ser2 gene in S. litura (Figure 2). Loss of function of the SlSer2 gene induced the male sterility (Figure 3). In the progeny of SlSer2 female mutants, we identified that male sterility was inherited stably (Figure 4). Using qRT-PCR, we detected changes in expression of some genes including members of a protease family, motor protein family and structural protein family (Figure 5). These results demonstrate the SlSer2 is one of the most important SFPs involved in energy metabolism and proteolysis in S. litura. CRISPR/Cas9 disruption Ser2 induced the male sterility without affecting female fertility. These phenotypes show Ser2 is a potential target gene for pest control in S. litura and other lepidopteran insects.
The seminal fluid comprises the non-sperm component of the male ejaculate, which contains hundreds of proteins and non-protein components, and affects male fertility (Poiani et al., 2006; Avila et al., 2011; Rodriguezmartinez et al., 2011; Laflamme et al., 2013). Recent studies have shown that the SFPs are ubiquitous and have been identified in many species such as Aedes aegypti (Sirot et al., 2011), Lutzomyia longipalpis (Azevedo et al., 2012), Ceratitis capitata (Davies et al., 2006), Apis mellifera (Baer et al., 2009), Heliconius erato and Heliconius melpomene (Walters et al., 2008; Walters et al., 2010), B. mori (Nagaoka et al., 2012; Xu et al., 2020), Tribolium castaneum (South et al., 2011), Gryllus firmus and Gryllus pennsylvanicus (Andres et al., 2006) and Amblyomma hebraeum (Weiss et al., 2002). These reports show that SFPs are important components of insect male fertility. In SFPs, the serine proteases were the most common class of the protease (Laflamme et al., 2013), with a conserved catalytic triad consisting of a His, Ser, and Asp that coordinate a water molecule (Polgar et al., 1989). The prevalence of serine proteases in the seminal fluid was expected (Page et al., 2008). Moreover, in D. melanogaster, SFPs contribute to many biological processes including immune defense, protein modification, and metabolism (Swanson et al., 2002; Gillott et al., 2003; Mcgraw et al., 2004). The qRT-PCR results of SlSer2 mutations showed the motor protein family gene and structure protein family gene were down-regulated, and the protein modification family gene was up-regulated, suggesting that SlSer2 involved in sperm movement and activity (Figure 5). In B. mori and P. xylostella, Xu et al (2020) used a transgenic CRISPR/Cas9 system to disrupt the Ser2 gene and induce male sterility. In H. cunea, Li X. et al., 2022 used transgenic RNAi and CRISPR/Cas9 technologies to loss of function of HcSer2 gene and demonstrated that Ser2 is an essential and potential target gene for SIT. These results demonstrated that Serine proteases especially the Ser2 gene, including SlSer2, have some conservative domains (Supplementary Figures S1, S2), expression manner (Figure 1) and played a vital function for male reproduction and sterility (Figures 3, 4).
The common cutworm, S. litura (Lepidoptera: Noctuidae) is one of the most destructive phytophagous pests (Tian et al., 2021; Tang et al., 2022). Pest management of lepidopteran insects is becoming increasingly difficult (Richardson et al., 2020; Li L. et al., 2022). SIT is an important approach for pest populations control. But choosing effective target genes is key to pest control by SIT (Harris et al., 2012). Our results suggest that the Ser2 gene regulates the male reproductive capacity and has the potential as a better target gene for pest control, especially in S. litura. In the silkworm, B. mori, the seminal fluid protein genes have been identified as the useful target genes for SIT (Xu et al., 2020; Xu et al., 2022). Sustainability is an important factor in pest control. Disruption of the Ser2 gene induced male sterility in the next generation and did not affect female reproduction. Thus, this study identified a useful sterile gene for pest control that may lead to control strategies in lepidopteran insect pests such as S. litura.
Data availability statement
The original contributions presented in the study are included in the article/Supplementary Materials, further inquiries can be directed to the corresponding authors.
Ethics statement
The animal study was reviewed and approved by Biomedical Research Ethics Committee of Henan University.
Author contributions
YH and SZ conceived and designed the experiments. HB and XX performed experiments. HB, XL, and YW analysed data. HB and XX wrote the manuscript and all the authors approved the final version of the manuscript prior to submission.
Funding
This work was supported by grants from the Key Scientific and Technological Project of Henan Province (222102110108) to B.H.L and the National Science Foundation of China (31530072, 31420103918, and 31572330) to Y.P.H.
Acknowledgments
We appreciate the experimentalist, Ms. Shi Aiqin, who is responsible for insect feeding and material collection.
Conflict of interest
The authors declare that the research was conducted in the absence of any commercial or financial relationships that could be construed as a potential conflict of interest.
Publisher’s note
All claims expressed in this article are solely those of the authors and do not necessarily represent those of their affiliated organizations, or those of the publisher, the editors and the reviewers. Any product that may be evaluated in this article, or claim that may be made by its manufacturer, is not guaranteed or endorsed by the publisher.
Supplementary material
The Supplementary Material for this article can be found online at: https://www.frontiersin.org/articles/10.3389/fphys.2022.931824/full#supplementary-material
References
Ahmad M., Saleem M. A., Sayyed A. H. (2009). Efficacy of insecticide mixtures against pyrethroid- and organophosphate-resistant populations of Spodoptera litura (Lepidoptera: Noctuidae). Pest Manag. Sci. 65 (3), 266–274. doi:10.1002/ps.1681
Ahmad M., Sayyed A. H., Saleem M. A., Ahmad M. (2008). Evidence for field evolved resistance to newer insecticides in Spodoptera litura (Lepidoptera: Noctuidae) from Pakistan. Crop Prot. 27 (10), 1367–1372. doi:10.1016/j.cropro.2008.05.003
Alphey L. (2014). Genetic control of mosquitoes. Annu. Rev. Entomol. 59, 205–224. doi:10.1146/annurev-ento-011613-162002
Alphey L. (2016). Can CRISPR-Cas9 gene drives curb malaria? Nat. Biotechnol. 34 (2), 149–150. doi:10.1038/nbt.3473
Andres J. A., Maroja L. S., Bogdanowicz S. M., Swanson W. J., Harrison R. G. (2006). Molecular evolution of seminal proteins in field crickets. Mol. Biol. Evol. 23 (8), 1574–1584. doi:10.1093/molbev/msl020
Avila F. W., Sirot L. K., Laflamme B. A., Rubinstein C. D., Wolfner M. F. (2011). Insect seminal fluid proteins: Identification and function. Annu. Rev. Entomol. 56 (1), 21–40. doi:10.1146/annurev-ento-120709-144823
Ayyanna T., Arjunarao P., Subbaratanam G. V., Krishna Murthy Rao B. H., Narayana K. L. (1982). Chemical control of Spodoptera litura F. groundnut crop. Peslology 16 (8), 19–20.
Azevedo R. V. D. M., Dias D. B. S., Bretãs J. A. C., Mazzoni C. J., Souza N. A., Albano R. M. (2012). The transcriptome of Lutzomyia longipalpis (diptera: Psychodidae) male reproductive organs. PLOS ONE 7 (4), e34495. doi:10.1371/journal.pone.0034495
Baer B., Heazlewood J. L., Taylor N. L., Eubel H., Millar A. H. (2009). The seminal fluid proteome of the honeybee Apis mellifera. Proteomics 9 (8), 2085–2097. doi:10.1002/pmic.200800708
Bassett A. R., Tibbit C., Ponting C. P., Liu J. (2013). Highly efficient targeted mutagenesis of Drosophila with the CRISPR/Cas9 system. Cell. Rep. 4, 220–228. doi:10.1016/j.celrep.2013.06.020
Bi H., Xu J., He L., Zhang Y., Li K., Huang Y., et al. (2019). CRISPR/Cas9‐mediated ebony knockout results in puparium melanism in Spodoptera litura. Insect Sci. 26, 1011–1019. doi:10.1111/1744-7917.12663
Bi H., Xu J., Tan A., Huang Y. (2016). CRISPR/Cas9-mediated targeted gene mutagenesis in Spodoptera litura. Insect Sci. 23 (3), 469–477. doi:10.1111/1744-7917.12341
Chapman T. (2001). Seminal fluid-mediated fitness traits in Drosophila. Heredity 87 (5), 511–521. doi:10.1046/j.1365-2540.2001.00961.x
Chen S., Hou C., Bi H., Wang Y., Xu J., Li M., et al. (2017). Transgenic clustered regularly interspaced short palindromic repeat/cas9-mediated viral gene targeting for antiviral therapy of Bombyx mori nucleopolyhedrovirus. J. Virol. 91 (8), e02465–16. doi:10.1128/JVI.02465-16
Cheng T., Wu J., Wu Y., Chilukuri R. V., Huang L., Yamamoto K., et al. (2017). Genomic adaptation to polyphagy and insecticides in a major East Asian noctuid pest. Nat. Ecol. Evol. 1 (11), 1747–1756. doi:10.1038/s41559-017-0314-4
Cong L., Ran F. A., Cox D., Lin S., Barretto R., Habib N., et al. (2013). Multiplex genome engineering using CRISPR/Cas systems. Science 339, 819–823. doi:10.1126/science.1231143
Davies S. J., Chapman T. (2006). Identification of genes expressed in the accessory glands of male Mediterranean Fruit Flies (Ceratitis capitata). Insect biochem. Mol. Biol. 36 (11), 846–856. doi:10.1016/j.ibmb.2006.08.009
Denis B., Claisse G., Rouzic A. L., Wickerthomas C., Lepennetier G., Joly D., et al. (2017). Male accessory gland proteins affect differentially female sexual receptivity and remating in closely related Drosophila species. J. Insect Physiol. 99, 67–77. doi:10.1016/j.jinsphys.2017.03.008
Du Q., Wen L., Zheng S., Bi H., Huang Y., Feng Q., et al. (2019). Identification and functional characterization of doublesex gene in the testis of Spodoptera litura. Insect Sci. 26 (6), 1000–1010. doi:10.1111/1744-7917.12608
Esvelt K. M., Smidler A. L., Catteruccia F., Church G. M. (2014). Concerning RNA-guided gene drives for the alteration of wild populations. eLife 3 (3401), e03401. doi:10.7554/eLife.03401
Gantz V. M., Jasinskiene N., Tatarenkova O., Fazekas A., Macias V. M., Bier E., et al. (2015). Highly efficient Cas9-mediated gene drive for population modification of the malaria vector mosquito Aanopheles stephensi. Proc. Natl. Acad. Sci. U. S. A. 112 (49), E6736–E6743. doi:10.1073/pnas.1521077112
Gillies A. F., Schinko J. B., Averof M. (2015). Efficient CRISPR-mediated gene targeting and transgene replacement in the beetle. Tribolium Castaneum. Dev. 142 (16), 2832–2839.
Gillott C. (2003). Male accessory gland secretions: Modulators of female reproductive physiology and behavior. Annu. Rev. Entomol. 48 (1), 163–184. doi:10.1146/annurev.ento.48.091801.112657
Guerin L. R., Moldenhauer L. M., Prins J. R., Bromfield J. J., Hayball J. D., Robertson S. A., et al. (2011). Seminal fluid regulates accumulation of foxp3(+) regulatory t cells in the preimplantation mouse uterus through expanding the foxp3(+) cell pool and ccl19-mediated recruitment. Biol. Reprod. 85 (2), 397–408. doi:10.1095/biolreprod.110.088591
Hall A. B., Basu S., Jiang X., Qi Y., Timoshevskiy V. A., Biedler J. K., et al. (2015). SEX DETERMINATION. A male-determining factor in the mosquito Aedes aegypti. Science 348 (6240), 1268–1270. doi:10.1126/science.aaa2850
Harris A. F., McKemey A. R., Nimmo D., Curtis Z., Black I., Morgan S. A., et al. (2012). Successful suppression of a field mosquito population by sustained release of engineered male mosquitoes. Nat. Biotechnol. 30 (9), 828–830. doi:10.1038/nbt.2350
Hwang W. Y., Fu Y., Reyon D., Maeder M. L., Tsai S. Q., Sander J. D., et al. (2013). Efficient genome editing in zebrafish using a CRISPR-Cas system. Nat. Biotechnol. 31, 227–229. doi:10.1038/nbt.2501
Karr T. L., Southern H., Rosenow M. A., Gossmann T. I., Snook R. R. (2019). The old and the new: Discovery proteomics identifies putative novel seminal fluid proteins in Drosophila. Mol. Cell. Proteomics 18, S23–S33. doi:10.1074/mcp.RA118.001098
Kaur T., Vasudev A., Sohal S. K., Manhas R. K. (2014). Insecticidal and growth inhibitory potential of Streptomyces hydrogenans DH16 on major pest of India, Spodoptera litura (Fab.) (Lepidoptera: Noctuidae). BMC Microbiol. 14 (1), 227. doi:10.1186/s12866-014-0227-1
Laflamme B. A., Ram K. R., Wolfner M. F. (2012). The Drosophila melanogaster seminal fluid protease "seminase" regulates proteolytic and post-mating reproductive processes. PLoS Genet. 8 (1), e1002435. doi:10.1371/journal.pgen.1002435
Laflamme B. A., Wolfner M. F. (2013). Identification and function of proteolysis regulators in seminal fluid. Mol. Reprod. Dev. 80 (2), 80–101. doi:10.1002/mrd.22130
Li L., Zhu B., Sun X., Zheng K., Liang P., Gao X., et al. (2022). miR-34-5p, a novel molecular target against lepidopteran pests. J. Pest Sci. doi:10.1007/s10340-022-01488-2
Li X., Liu Q., Bi H., Wang Y., Xu X., Sun W., et al. (2022). piggyBac-based transgenic RNAi of serine protease 2 results in male sterility in Hyphantria cunea. Insect biochem. Mol. Biol. 143, 103726. doi:10.1016/j.ibmb.2022.103726
Liu Q., Liu W., Zeng B., Wang G., Hao D., Huang Y., et al. (2017). Deletion of the Bombyx mori odorant receptor co-receptor (BmOrco) impairs olfactory sensitivity in silkworms. Insect biochem. Mol. Biol. 86, 58–67. doi:10.1016/j.ibmb.2017.05.007
Marshall J. L., Huestis D. L., Hiromasa Y., Wheeler S., Oppert C., Marshall S. A., et al. (2009). Identification, RNAi knockdown, and functional analysis of an ejaculate protein that mediates a postmating, prezygotic phenotype in a cricket. PloS one 4 (10), e7537. doi:10.1371/journal.pone.0007537
McGraw L. A., Gibson G., Clark A. G., Wolfner M. F. (2004). Genes regulated by mating, sperm, or seminal proteins in mated female Drosophila melanogaster. Curr. Biol. 14 (16), 1509–1514. doi:10.1016/j.cub.2004.08.028
McGraw L. A., Suarez S. S., Wolfner M. F. (2015). On a matter of seminal importance. Bioessays 37 (2), 142–147. doi:10.1002/bies.201400117
Meagher R. L., Brambila J., Hung E. (2008). Monitoring for exotic Spodoptera species (Lepidoptera: Noctuidae) in Florida. Fla. Entomol. 91 (4), 517–522.
Muthusamy R., Vishnupriya M., Shivakumar M. S. (2014). Biochemical mechanism of chlorantraniliprole resistance in Spodoptera litura (Fab) (Lepidoptera: Noctuidae). J. Asia-pacific Entomology 17 (4), 865–869. doi:10.1016/j.aspen.2014.09.001
Nagaoka S., Kato K., Takata Y., Kamei K. (2012). Identification of the sperm-activating factor initiatorin, a prostatic endopeptidase of the silkworm, Bombyx mori. Insect biochem. Mol. Biol. 42 (8), 571–582. doi:10.1016/j.ibmb.2012.04.004
Neubaum D. M., Wolfner M. F. (1999). Mated Drosophila melanogaster females require a seminal fluid protein, Acp36DE, to store sperm efficiently. Genetics 153 (2), 845–857. doi:10.1093/genetics/153.2.845
Page M. J., Cera E. D. (2008). Serine proteases and serine protease inhibitors. Hoboken, NJ, USA: Wiley Encyclopedia of Chemical Biology, 1–29.
Poiani A. (2006). Complexity of seminal fluid: A review. Behav. Ecol. Sociobiol. 60, 289–310. doi:10.1007/s00265-006-0178-0
Qin H., Ye Z., Huang S., Ding J., Luo R. (2004). The correlations of the different host plants with preference level, life duration and survival rate of Spodoptera litura Fabricius. Chin. J. Eco-Agriculture 12, 40–42.
Rao G. V., Wightman J. A., Rao D. V. (1993). World review of the natural enemies and diseases of Spodoptera litura (FabriciusF.) (Lepidoptera: Noctuidae). Int. J. Trop. Insect Sci. 14 (03), 273–284.
Rehan A., Freed S. (2014). Selection, mechanism, cross resistance and stability of spinosad resistance in Spodoptera litura (Fabricius) (Lepidoptera: Noctuidae). Crop Prot. 56, 10–15.
Richardson E. B., Troczka B. J., Gutbrod O., Davies T. G. E., Nauen R. (2020). Diamide resistance: 10 years of lessons from lepidopteran pests. J. Pest Sci. 93 (3), 911–928. doi:10.1007/s10340-020-01220-y
Rodriguezmartinez H., Kvist U., Ernerudh J., Sanz L., Calvete J. J. (2011). Seminal plasma proteins: What role do they play? Am. J. Reprod. Immunol. 66, 11–22. doi:10.1111/j.1600-0897.2011.01033.x
Shad S. A., Sayyed A. H., Saleem M. A. (2010). Cross-resistance, mode of inheritance and stability of resistance to emamectin in Spodoptera litura (Lepidoptera: Noctuidae). Pest Manag. Sci. 66 (8), 839–846. doi:10.1002/ps.1950
Sirot L. K., Hardstone M. C., Helinski M. E. H., Ribeiro José M. C., Kimura M., Deewatthanawong P., et al. (2011). Towards a semen proteome of the dengue vector mosquito: Protein identification and potential functions. PLoS Negl. Trop. Dis. 5 (3), e989. doi:10.1371/journal.pntd.0000989
Sirot L. K., Laflamme B. A., Sitnik J. L., Rubinstein C. D., Avila F. W., Chow C. Y., et al. (2009). Molecular social interactions: Drosophila melanogaster seminal fluid proteins as a case study. Adv. Genet. 68, 23–56. doi:10.1016/S0065-2660(09)68002-0
Sirot L. K., Wong A., Chapman T., Wolfner M. F. (2014). Sexual conflict and seminal fluid proteins: A dynamic landscape of sexual interactions. Cold Spring Harb. Perspect. Biol. 7 (2), a017533. doi:10.1101/cshperspect.a017533
South A., Sirot L. K., Lewis S. M. (2011). Identification of predicted seminal fluid proteins in Tribolium castaneum. Insect Mol. Biol. 20 (4), 447–456. doi:10.1111/j.1365-2583.2011.01083.x
Swanson W. J., Vacquier V. D. (2002). The rapid evolution of reproductive proteins. Nat. Rev. Genet. 3 (2), 137–144. doi:10.1038/nrg733
Tan A., Fu G., Jin L., Guo Q., Li Z., Niu B., et al. (2013). Transgene-based, female-specific lethality system for genetic sexing of the silkworm, Bombyx mori. Proc. Natl. Acad. Sci. U. S. A. 110 (17), 6766–6770. doi:10.1073/pnas.1221700110
Tang R., Liu F., Lan Y., Wang J., Wang L., Li J., et al. (2022). Transcriptomics and metagenomics of common cutworm (Spodoptera litura) and fall armyworm (Spodoptera frugiperda) demonstrate differences in detoxification and development. BMC genomics 23 (1), 388. doi:10.1186/s12864-022-08613-6
Taniguchi K., Kokuryo A., Imano T., Nakagoshi H., Adachiyamada T. (2018). Binucleation of accessory gland lobe contributes to effective ejection of seminal fluid in Drosophila melanogaster. Zool. Sci. 35 (5), 446–458. doi:10.2108/zs170188
Tian L., Gao X., Zhang S., Zhang Y., Ma D., Cui J., et al. (2021). Dynamic changes of transcriptome of fifth-instar Spodoptera litura larvae in response to insecticide. 3 Biotech. 11 (2), 98. doi:10.1007/s13205-021-02651-9
Walters J. R., Harrison R. G. (2010). Combined EST and proteomic analysis identifies rapidly evolving seminal fluid proteins in Heliconius butterflies. Mol. Biol. Evol. 27 (9), 2000–2013. doi:10.1093/molbev/msq092
Walters J. R., Harrison R. G. (2008). EST analysis of male accessory glands from Heliconius butterflies with divergent mating systems. BMC Genomics 9 (1), 592. doi:10.1186/1471-2164-9-592
Wang Y., Li Z., Xu J., Zeng B., Ling L., You L., et al. (2013). The CRISPR/Cas System mediates efficient genome engineering in Bombyx mori. Cell. Res. 23, 1414–1416. doi:10.1038/cr.2013.146
Weiss B. L., Stepczynski J., Wong P., Kaufman W. R. (2002). Identification and characterization of genes differentially expressed in the testis/vas deferens of the fed male tick, Amblyomma hebraeum. Insect biochem. Mol. Biol. 32 (7), 785–793. doi:10.1016/s0965-1748(01)00161-8
Xu J., Chen S., Zeng B., James A. A., Tan A., Huang Y., et al. (2017). Bombyx mori P-element somatic inhibitor (BmPSI) is a key auxiliary factor for silkworm male sex determination. PLoS Genet. 13 (1), e1006576. doi:10.1371/journal.pgen.1006576
Xu J., Dong Q., Yu Y., Niu B., Ji D., Li M., et al. (2018). Mass spider silk production through targeted gene replacement in Bombyx mori. Proc. Natl. Acad. Sci. U. S. A. 115 (35), 8757–8762. doi:10.1073/pnas.1806805115
Xu J., Wang Q. (2011). Seminal fluid reduces female longevity and stimulates egg production and sperm trigger oviposition in a moth. J. Insect Physiol. 57 (3), 385–390. doi:10.1016/j.jinsphys.2010.12.006
Xu X., Wang Y., Bi H., Xu J., Liu Z., Niu C., et al. (2020). Mutation of the seminal protease gene, serine protease 2, results in male sterility in diverse lepidopterans. Insect biochem. Mol. Biol. 116, 103243. doi:10.1016/j.ibmb.2019.103243
Xu X., Wang Y., Chen J., Du X., Yao L., Xu J., et al. (2022). Mutation of Serine protease 1 induces male sterility in Bombyx mori. Front. Physiol. 13, 828859. doi:10.3389/fphys.2022.828859
Zeng B., Huang Y., Xu J., Shiotsuki T., Bai H., Palli S. R., et al. (2017). The FOXO transcription factor controls insect growth and development by regulating juvenile hormone degradation in the silkworm, Bombyx mori. J. Biol. Chem. 292 (28), 11659–11669. doi:10.1074/jbc.M117.777797
Zhang Z., Niu B., Ji D., Li M., Li K., James A. A., et al. (2018). Silkworm genetic sexing through W chromosome-linked, targeted gene integration. Proc. Natl. Acad. Sci. U. S. A. 115 (35), 8752–8756. doi:10.1073/pnas.1810945115
Zhao Y., Sun W., Zhang P., Chi H., Zhang M., Song C., et al. (2012). Nematode sperm maturation triggered by protease involves sperm-secreted serine protease inhibitor (Serpin). Proc. Natl. Acad. Sci. U. S. A. 109 (5), 1542–1547. doi:10.1073/pnas.1109912109
Keywords: Spodoptera litura, Ser2, male sterility, pest control, CRISPR/Cas9
Citation: Bi H, Xu X, Li X, Wang Y, Zhou S and Huang Y (2022) CRISPR/Cas9-mediated Serine protease 2 disruption induces male sterility in Spodoptera litura. Front. Physiol. 13:931824. doi: 10.3389/fphys.2022.931824
Received: 29 April 2022; Accepted: 07 July 2022;
Published: 03 August 2022.
Edited by:
Peng He, Guizhou University, ChinaReviewed by:
Kangxu Wang, Michigan State University, United StatesZhaojiang Guo, Insititute of Vegetables and Flowers (CAAS), China
Copyright © 2022 Bi, Xu, Li, Wang, Zhou and Huang. This is an open-access article distributed under the terms of the Creative Commons Attribution License (CC BY). The use, distribution or reproduction in other forums is permitted, provided the original author(s) and the copyright owner(s) are credited and that the original publication in this journal is cited, in accordance with accepted academic practice. No use, distribution or reproduction is permitted which does not comply with these terms.
*Correspondence: Shutang Zhou, c3pob3VAaGVudS5lZHUuY24=; Yongping Huang, eXBodWFuZ0BzaWJzLmFjLmNu