- 1Programa de Doctorado en Ciencias de Recursos Naturales, Universidad de La Frontera, Temuco, Chile
- 2Laboratorio de Química Ecológica, Departamento de Ciencias Químicas y Recursos Naturales, Facultad de Ingeniería y Ciencias, Universidad de La Frontera, Temuco, Chile
Nowadays, insect chemosensation represents a key aspect of integrated pest management in the Anthropocene epoch. Olfaction-related proteins have been the focus of studies due to their function in vital processes, such ashost finding and reproduction behavior. Hence, most research has been based on the study of model insects, namely Drosophila melanogaster, Bombyx mori or Tribolium castaneum. Over the passage of time and the advance of new molecular techniques, insects considered non-models have been studied, contributing greatly to the knowledge of insect olfactory systems and enhanced pest control methods. In this review, a reference point for non-model insects is proposed and the concept of model and non-model insects is discussed. Likewise, it summarizes and discusses the progress and contribution in the olfaction field of both model and non-model insects considered pests in agriculture.
Introduction
Among living species, insects are considered the major class with an estimated more than five million species, and an important role in the ecosystem (Stork, 2018). However, with an ever-growing global population and a subsequent intensive agriculture at a global scale, an increasing number of insect species have become a threat to the food supply. Thus, the impact of insects pests on agriculture has caused serious damage to the global economy, causing losses of 20%–40% of world food production (FAO, 2017). In that sense, it has been reported that the greatest damage caused by insects lies in direct feeding on plant parts, such as chewing leaves, piercing stems and roots (Cranshaw, 2004; Oerke, 2006).
Since insecticides emerged, chemical control has been the main strategy to fight insect pests. However, their indiscriminate use as a consequence of a high demand for food has resulted in long residual on plants as well as leaching into soil, reaching groundwater and affecting soil nutritional quality, threatening the environment and living beings (Peralta et al., 1994; Arora and Sahni, 2016; Md Meftaul et al., 2020). It is believed that the inherent toxicity of insecticides has generated serious issues for human health. This is manifest in neurological symptoms and some related diseases, such as Parkinson’s disease, based on direct or exposure to these chemicals (Hertzman et al., 1994; Kamel et al., 2005; Keifer and Firestone, 2007). Over the years, insects have managed to adapt to different classes insecticides resulted in developing resistance (Heisey and Norton, 2007; Bass et al., 2014; Khan and Ahmad, 2019; Mohammed et al., 2019; Attia et al., 2020; Khan et al., 2020). For instance, the intensive use of transgenic corn with the insecticidal protein Cry1F has provoked resistance in some major corn pests, the codling moth Spodoptera frugiperda, affecting the economy and agriculture of Puerto Rico, Brazil and the United States (Storer et al., 2010; Storer et al., 2012; Farias et al., 2014; Huang et al., 2014; Yang et al., 2016).
Alternatively, volatile chemicals already present in nature have been extensively studied for their potential role in manipulating insect behavior (i.e., semiochemicals). Particular emphasis has been placed on sex pheromones, which are volatiles usually released by a female, that can elicit strong attraction in conspecific males. Additionally, an approach called “push-pull” that interferes with the ability of insects to find hosts by using plants capable of producing attractants and repellents has been used (Hassanali et al., 2008). Thus, these behavior-based techniques are related to olfaction and employed as effective control methods in pest management, being non-toxic nor invasive for crops (Smart et al., 2014; Soroker et al., 2015).
To understand how insects smell and their resulting behaviors, the study of their olfactory physiology has arisen as a groundbreaking field of research. Insects smell mainly through their antennae, which houses specialized units called sensilla. Inside these, odorant-binding proteins (OBPs) and chemosensory proteins (CSPs) are small extracellular proteins which thought to aid in capture and transport of odorants and pheromones to the receptors (Leal, 2005; Zhou, 2010; Leal, 2013). The OBPs and CSPs play a fundamental role in the mechanism of olfaction and in the evolutionary adaptation of the olfactory system (Pelosi and Maida, 1990; Vogt et al., 1991; Vieira and Rozas, 2011; Pelosi et al., 2018; D'Onofrio et al., 2020). On the other hand, different types of transmembrane proteins are present in olfactory receptor neurons (ORNs), such as odorant receptors (ORs), gustatory receptors (GRs), ionotropic receptors (IRs) and sensory neuron membrane proteins (SNMPs) (Croset et al., 2010; Abuin et al., 2011). Altogether, these allow the transduction of chemical signals into electrical signals (Kaissling, 2013). Currently, ORs, CSPs and OBPs are being studied comprehensively to clarify their binding specificities and mechanisms. Their use as targets to identify novel potent semiochemicals can be argued in terms of failures and successes (Venthur and Zhou, 2018). Ultimately, these approaches assumed to play an important role in integrated pest management and broadening the limited options available today.
Over the last few decades, the advance in our understanding of how insects smell was based on a few species. An important milestone included the identification and synthesis of the first sex pheromone, bombykol, from the silkmoth Bombyx mori (Butenandt, 1959; Butenandt, 1963; Mansurova et al., 2009). Then came the advance in molecular techniques to identify OBPs as key odorant carriers (Vogt and Riddiford, 1981) and, years later, the first complete genome of an insect that was from Drosophila melanogaster (Adams et al., 2000). Such events made B. mori, Antheraea polyphemus and D. melanogaster the first identifiable model insects for research. Later, other insect species with complete genomes, such as the malaria mosquito Anopheles gambiae (Holt et al., 2002) and the honeybee Apis mellifera (Weinstock et al., 2006), have also been considered model insects for olfaction research. Nowadays, moths, namely Manduca sexta and Spodoptera littoralis, have outstanding studies related to olfactory proteins through next generation sequencing and functional assays (Grosse-Wilde et al., 2011; de Fouchier et al., 2017).
Considering the significant advance in insect research, we believe that there is no clear definition of what makes an insect either a model or non-model. Likewise, we believe that there are insects that have not been sufficiently considered, which have outstanding features at molecular, physiological and behavioral levels that can provide key evidence for the understanding of nature, from agricultural questions to broader fields. Therefore, the present review aims to discuss the impact of studying insects with agricultural importance in the last decade as well as to identify patterns that can allow to propose a benchmark for model and non-model insects, including the nuances between both concepts. In addition, this revision provides a general perspective of the importance of insect olfaction research and hints of what future could bring for the field.
In order to retrieve information to build our datasets, a literature search was performed in the Web of Science (WOS), Scopus, PLoS and Scielo databases, mainly using the following keywords: pest control, sustainability, insect olfaction, model insects, non-model insects and chemosensory proteins. In addition, some phrases such as olfactory physiology, control methods, agriculture and chemoreception were used, thus collecting more than 300 scientific articles. These datasets can be found in Supplementary Tables S1, S2.
What are Model and Non-Model Insects?
Although no clear definition of what either a model or non-model insect is, a few factors can be established that are common among the first identifiable model insects. For instance, B. mori is considered a model system for entomological studies due to its large body size, ease of rearing in laboratory, and economic importance in sericulture (Mita et al., 2004). D. melanogaster, on the other hand, began to be a study model because of its short generation times, ease of breeding and tolerance to relatively high population densities (Ashburner, 1989). Studies on the red flour beetle (Tribolium castaneum) arose because of its importance as a pest of stored grains and grain products, with a worldwide distribution, in addition to its ease of rearing, relatively short generation interval, ability to be reared in field and low maintenance (Chu et al., 2014; Kumar et al., 2018). Finally, A. polyphemus (similar to B. mori) has been extensively studied because of ease of breeding, large size and molecular mechanisms of sex pheromone action (Collins and Weast, 1961; Hall, 2015).
Considering the above, it is possible to establish common characteristics that model insects have, such as ease of rearing and size, which could have helped scientists perform early molecular biology-related methods. By contrast, non-model insects can be considered little-known species that are often specific to a geographic region. For instance, Neotetranychus lek, a species of the red mite family first reported in 2013 in Thailand, is believed to be endemic to Southeast Asia and a pest of cassava of agricultural importance (Flechtmann, 2013). Another example is the Bactrocera jarvisi fly, endemic to Australia, which is considered a pest in the territory due to the damage it causes to commercially important crops, such as mango (Peng et al., 2007; Fay, 2012). Many times, however, it depends on the study area whether an insect is considered a model or not. For example, the greater wax moth, Galleria mellonella, a harmful pest of beehives, can be considered a non-model insect in terms of its understanding of olfactory physiology, with some recent advances in the field (Aburto et al., 2019; Zhao et al., 2019; Lizana et al., 2020). Nevertheless, it can be a model insect due to its ease of rearing and use as hosts for the study of pathogens (Killiny, 2018; Ménard et al., 2021). Another example is the butterfly Araschnia levana, which can be considered a model for the study of the molecular basis and evolutionary ecology of seasonal polyphenism due to its characteristic seasonal dimorphism (Baudach and Vilcinskas, 2021). In recent years, the order Coleoptera has taken on great importance in the identification of little-known species, which can be considered models for their particularities. An example is the canola beetle Meligethes aeneus, considered a model to determine how agricultural practices affect pest population dynamics as well as the impact of different control strategies on the risk and speed of resistance development (Stratonovitch, et al., 2014). Another case is the potato beetle Leptinotarsa decemlineata, which due to its special ability to adapt to plants, environmental conditions, with particular resistance to more than 50 pesticides, can be considered a model species for agricultural pest genomics (Schoville et al., 2018). Furthermore, the number of studies of a particular species over the years may result in a species initially considered as non-model now being classified as a model species. The study of non-model insects can bring multiple advantages for researchers, such as new and original research questions and greater knowledge of pest insects of agricultural importance. This would enable the establishment of both species-specific control methods and less harmful to the environment. Moreover, the increased knowledge through research on non-model insects endemic to different geographic areas would allow the respective economic development of these areas. An example are beetles endemic to Chile, namely Hylamorpha elegans and Brachysternus prasinus. These insects are distributed in the same geographic area (central and southern part of the country) and are considered pests of economic importance due to the serious damage they cause to crops, such as wheat (Triticum aestivum) and red clover (Trifolium pratense) (Gonzalez, 1989; Artigas, 1994; Aguilera, 1995; Palma, 2004). Therefore, the study of these coleopterans would allow us to understand the sympatry present in them as well as speciation. Another example is eggplant borer Leucinodes orbonalis in Bangladesh, where it has been possible to establish control using pheromonal traps, thus minimizing the use of pesticides (Mazumder and Khalequzzaman, 2010).
Overall, we believe that a non-model insect has limited information on genomics and biological mechanisms, and is generally distributed in specific geographic areas.
Role of Non-Model Insects in the Study of Olfaction
For insects, there are some key aspects for the development of their respective life cycles, such as searching for food, mate finding, a place to oviposit and even detecting a nearby predator. Interestingly, all of them depend on the olfactory system (De Bruyne and Baker, 2008). Thus, insect olfaction at perireceptor level has several types of proteins for such purposes. Soluble proteins, namely OBPs and CSPs, transport semiochemicals through the sensillar lymph to reach receptors in ORNs. These carriers function as the first filter of olfactory information for insects (Leal, 2005). Receptors (e.g., ORs, IRs and GRs), on the other hand, recognize semiochemicals, allowing signal transduction followed by a cascade of events that end in the central nervous system of insects, unleashing behavioral responses (Wicher and Marion-Poll, 2018). In particular, IRs and GRs have been a matter of interest in recent years due to their involvement in hygroreception or sugar/bitter perception, respectively (Slone et al., 2007; Jiao et al., 2008; Wanner and Robertson, 2008; Enjin et al., 2016; Kim and Wang, 2016; Knecht et al., 2017). Conversely, SNMPs have received less attention. However, these types of transmembrane proteins appear to have a key role in signal transduction, likely involved in the heteromeric complex of OR/Orco (Zhang et al., 2020). Besides these, OBPs, CSPs and ORs stand out as the most studied to date. Hence, this review will focus on these.
Odorant Binding Proteins and Chemosensory Proteins: Key Transporters for Chemoreception
OBPs play a fundamental role in binding hydrophobic odorants, such as pheromones, plant volatiles, among others, in the pores of the sensilla and transporting them through the sensillar lymph to facilitate their solubilization (Prestwich, 1996). Thus, it is proposed that they participate in the first biochemical stage of perireceptor events in odorant capture (Jacquin-Joly and Merlin, 2004; Pelosi et al., 2005; Zhou, 2010). Defined as molecules capable of recognizing a variety of volatile compounds (Venthur et al., 2014), it has been established that OBPs act as a semiochemical solubilizers, transporters and ligand filters, mediating the activation of ORs (Fan et al., 2011). For in-depth reviews around OBPs (and CSPs too), we suggest readers revise Zhou (2010), Venthur et al. (2014), and Pelosi et al. (2018).
Insect OBPs were first identified in A. polyphemus (Vogt and Riddiford, 1981), adopting the nomenclature of pheromone-binding protein (PBP) for those OBPs that were male-biased in expression and that showed affinity to sex pheromones. Nowadays, this classification has remained only for Lepidopterans. An outstanding example is the PBP1 of B. mori (BmorPBP1), which became a study model to understand the binding mechanism of pheromone components and to expand the knowledge about how these proteins function in the olfactory system of insects (Wojtasek and Leal, 1999; Damberger et al., 2000; Horst et al., 2001; Lee et al., 2002). Outstandingly, the first three-dimensional (3D) structure for an insect OBP was BmorPBP1, showing that residue Ser59 was important for establishing hydrogen bonds with bombykol (i.e., B. mori sex pheromone) (Sandler et al., 2000). Later, Lautenschlager et al. (2005; 2007) were able to show that BmorPBP1 undergoes important conformational changes that depend on pH, suggesting ligand binding under neutral conditions, and release under acidic conditions (i.e., near the dendritic membrane of ORNs). Furthermore, BmorPBP1 binds and holds bombykol across different pH values, contrary to other types of odorants (Damberger et al., 2013). This certainly opened up the field to study other insect species. Currently, the 3D structure of OBPs for a diverse range of insects, such as navel orangeworm Amyelois transitella (Di Luccio et al., 2013), medfly Ceratitis capitata (Falchetto et al., 2019), aphids Megoura viciae and Nasonovia ribisnigri (Northey et al., 2016). For instance, these last structures have been used as templates to build the 3D structure of homologues OBPs for other insect species, allowing their study at bioinformatics and biochemical level. Thus, aphid Rhopalosiphum padi, for which OBP7 strongly bound (E)-β-farnesene, a repellent for R. padi, was supported by the use of crystal structures of M. viciae and N. ribisnigri OBPs. More recently, the crystal structure for Epiphyas postvittana PBP3 was reported, representing the first 3D structure of an OBP for tortricid moths, which have an enormous economic impact for agriculture worldwide (Hamiaux et al., 2020). This represents an important advance in the study of other tortricids of economic importance, such as Lobesia botrana, Grapholita molesta and Cydia pomonella, allowing a more precise construction of 3D models for OBPs.
CSPs, as well as OBPs, are involved in peripheral olfactory processing, and together they are the most important proteins for chemoreception due to their functions as carriers of semiochemicals (Breer et al., 1994; Calvello et al., 2003). Remarkably, early evidence not only suggested CSPs as odorant transporters, but that they were also involved in non-chemosensory functions (Qiao et al., 2013), as they have been identified in both chemosensory and non-chemosensory organs. An example of this is Locusta migratoria, where 17 CSPs were identified in reproductive organs (Zhou et al., 2013). Unlike OBPs that are considered to be more antenna-specific, CSPs are distributed in various insect tissues, namely legs, proboscis, thorax, etc. (Picimbon et al., 2001; Wanner et al., 2005; Foret et al., 2007). However, recent reports strongly suggest their role as carriers of semiochemicals (Li et al., 2016; Peng et al., 2017). For example, CSP1, CSP2 and CSP3 of rice leaf folder Cnaphalocrocis medinalis (major pest of rice plants) showed high binding affinity to host-related semiochemicals, such as terpenoids, and even sex pheromones (Z)-11-hexadecenyl acetate and (Z)-11-hexadecenal (Zeng et al., 2018). More recently, silencing of CSP4 and CSP5 in aphid R. padi (pest of Poaceae plants) resulted in significant reduction of octanal detection, a host plant attractant (Peng et al., 2020). In addition to the role of CSPs binding semiochemicals, their involvement in insecticide resistance has been recently reported for cotton aphid Aphis gossypii, a polyphagous pest with such resistance (Li et al., 2021). The authors found that CSP5 of A. gossypii is upregulated under insecticide treatments (imidacloprid and cypermethrin), potentially helping to decrease mortality.
The first 3D structure to be solved for CSPs was cabbage moth Mamestra brassicae CSP (Lartigue et al., 2002), a serious pest for Brassica plants. On the one hand, 3D structures for Schistocerca gregaria and M. brassicae CSPs, which have economic importance as pests, have been solved and deposited in databases (https://www.rcsb.org/). In the case of OBPs, more 3D structures are available; for example, there are 28 structures available for Lepidoptera, two structures for both Coleoptera and Hemiptera and in the case of Hemiptera, there are more than 30, though only 3 are strongly related to agricultural pests. Consequently, more structural studies can be performed for OBPs than CSPs, being a key factor to study non-model insect pests.
Odorant Receptors and Their Importance for Understanding Insect Olfaction
In the family of membrane chemosensory receptors in insects, the so-called ORs and IRs are found. Although both receptors are responsable for chemical detection, ORs have received significant attention due to their role in capturing secondary metabolites as odorants, emitted by either host plants or conspecific insects. The latter was identified in D. melanogaster using its assembled genome (Adams et al., 2000), which encodes the particular 7TM for insects (Clyne et al., 1999; Gao and Chess, 1999; Vosshall et al., 1999). Then, with the identification of D. melanogaster Orco, initially coded as Or83b (Vosshall and Hansson, 2011), new questions arose about the role of this receptor, since it was both highly conserved across insect species and expressed in all ORN types (Vosshall et al., 2000). Nowadays, it is known that Orco and ORs are a functional heteromeric complex as odorant-sensing cation channels, which ultimately provide the rapid odorant signaling of insects, which is transient, sensitive and prolonged in nature (Neuhaus et al., 2004; Wicher et al., 2008). For comprehensive reviews around insect ORs, we recommend Yan et al. (2020) and Wicher and Miazzi (2021).
Besides the identification of Orco, the role of ORs as specific subunits was first tested in B. mori OR1 (BmorOR1) through electrophysiological recordings using Xenopus oocytes (Sakurai et al., 2004). The authors reported outstanding specificity of BmorOR1, responding to bombykol but not bombykal. Using D. melanogaster OR/Orco, Wicher et al. (2008) and Sato et al. (2008) were able to answer how signal transduction functions upon OR activation. Their results suggested that ionotropic responses are followed by membrane depolarization and, ultimately, metabotropic responses are unleashed. More recently, the use of CRISPR-Cas9 to disrupt the expression of Orco in the hawkmoth M. sexta showed that Orco is crucial for sexual communication but not for host location (Fandino et al., 2019). Despite all these advances based on model insects, the study of a non-model insect established a milestone in insect olfaction. The first three-dimensional (3D) structure of the Orco was from the fig parasitic wasp, Apocrypta bakeri (Butterwick et al., 2018). This has provided key knowledge on structure for these receptors. Consequently, the A. bakeri Orco structure has been used as a template for homology modeling on OR46 and OR49 of bark beetle Ips typographus, a serious threat to forest ecosystems (Yuvaraj et al., 2021).
Furthermore, the development of techniques, such as Calcium imaging through HEK293 cells (Corcoran et al., 2014), electrophysiology through Xenopus oocytes (Choo et al., 2018) and single-sensillum recordings (SSRs) from Drosophila (de Fouchier et al., 2017), have allowed functional studies for non-model insects. For instance, by using the moth E. postvittana the utilization and capabilities of HEK293 cells in insects was demonstrated (Corcoran et al., 2014). Another example is aphid Acyrthosiphon pisum, where functional assays with ORs enabled the identification of new candidate volatiles for aphid control strategies and demonstrated that these assays are suitable for aphid ORs (Zhang et al., 2017).
Relevant Non-Model Insects for the Advance of Olfactory Physiology
In the last decade, different insect species have contributed to the understanding of peripheral olfactory systems, which today are known for being important economic pests, such as S. littoralis or Helicoverpa armigera. In our analyses, groundbreaking findings have been reported from early non-model insects, which are summarized in Table 1. As shown, the order Lepidoptera has contributed the most to olfactory physiology and, consistent with the agricultural importance that these have, we propose that these species can be considered study models. This summary supports the fact that new discoveries are difficult to be achieved based on a few insect species (e.g., study models). Thus, the particularities presented by these insects such as the inverse sexual communication of G. mellonella and the predatory behavior of H. convergens that allows the control of pests, such as aphids generate new study opportunities that model insects, such as D. melanogaster and B. mori, cannot provide. Also, the study of non-model insects shed lights to new patterns of insect biology that had not been studied before.
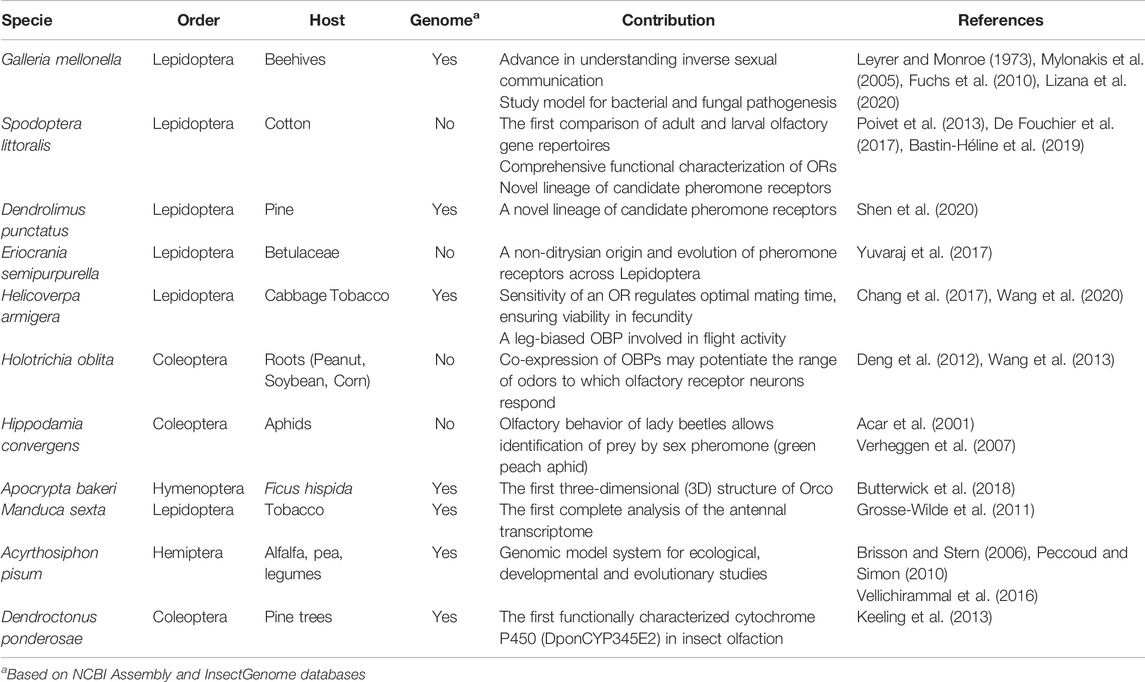
TABLE 1. Summary of early non-model insects and their contribution to olfactory physiology in the last decade.
A decade ago, advances in olfactory knowledge set a precedent in the understanding of molecular mechanisms related to insect olfaction. An example of this is the first complete analysis of the antennal transcriptome involved in olfaction in what could had been considered a non-model insect, M. sexta (Grosse-Wilde et al., 2011). Another example is the first comparison of adult and larval olfactory gene repertoires in the then non-model moth S. littoralis (Poivet et al., 2013). Currently, both Lepidopterans can be considered model insects according to the contributions in olfactory physiology and number of studies on each moth. The same happens with other insects that today can be considered study models, but a decade ago were still incipient case studies, such as Holotrichia oblita, H. armigera and G. mellonella.
Besides Lepidopterans, it is observed that Hemipterans appear in a large number of studies, explained by their condition as agricultural pests (Sorensen, 2009; Dedryver et al., 2010). Thus, species such as Adelphocoris lineolatus, Myzus persicae, Sitobion avenae, among others, can be found (Supplementary Table S2). By contrast, Hemipterans appear under-represented. An important number of studies around olfaction (i.e., OBPs, CSPs and ORs) have been performed on different species of Hemipterans, and besides A. pisum, we were unable to find studies that represent a breakthrough in the research field. On the other hand, the order Orthoptera includes insects that cause serious damage to crops and tree seedlings, such as leafhoppers (Joshi et al., 1999; Akhtar et al., 2012). However, no studies were found in comparison with those included in Table 1. Without underestimating evidence on Hempiterans and Orthopterans, we believe it is important to advance in future studies that can enhance our knowledge on their olfactory physiology, behavior and likely control methods.
Role of Sequencing Techniques on Non-Model Insect Research
The development of next generation sequencing (NGS) has increased both genomic and transcriptomic studies of species with limited information (Ekblom and Galindo, 2010; McCormack et al., 2013; Wachi et al., 2018). These techniques have contributed to the discovery of levels and patterns of genetic diversity, phylogenetic relationships and adaptations (Nadeau and Jiggins, 2010; Stapley et al., 2010). Examples are D. melanogaster, B. mori, A. gambiae and T. castaneum (Adams et al., 2000; Holt et al., 2002; Mita et al., 2004; Richards et al., 2008), for which genomes were deposited in the early 2000s. Furthermore, more precise and broad phylogenetic analyses have been performed. For example, the first phylogenomic tree, performed in 2014, had 2,696 genes from Lepidopterans, establishing an evolutionary framework in relation to butterflies and moths (Kawahara and Breinholt, 2014). It is worth mentioning that during the last decade, extraordinary efforts have resulted in hundreds of olfactory proteins identified through both genome and transcriptome sequencing projects. By 2019, Li et al. (2019) reported that 1,219 insect genome-sequencing projects were registered in the National Center for Biotechnology Information (NCBI). Likewise, Venthur and Zhou (2018) showed that 54 antennal or head transcriptomes (RNA-seq technology) were published. The advance in RNA-seq has allowed the expansion in type and number of samples to be sequenced. For instance, 67 RNA-seq datasets have been reported for M. sexta (a recent model insect), including different tissues and developmental stages (Cao and Jiang, 2017). Despite this, there are still other insect species, considered non-models as discussed above, with lack of sequencing data (Oppenheim et al., 2015). It is noteworthy that they are of great importance as agricultural pests or because they have special features that allow advances in scientific research. Nevertheless, an important increase in genomic and transcriptomic studies in specific insect species (i.e., non-model insects) has occurred in recent years (Figure 1.). Thus, it can be seen that the ratio of studies on model:non-model insects has changed from 1:1 to 1:14. Therefore, lower costs in sequencing projects, the rapid spread of insects worldwide and the search for control/monitoring methods are among those factors that can explain these findings.
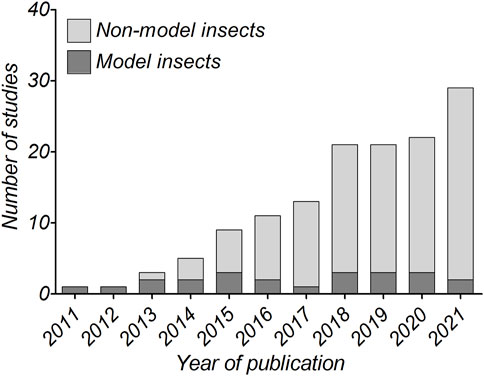
FIGURE 1. Number of studies published in the last 10 years on olfactory protein identification from antennal transcriptome (detailed data available in Supplementary Table S1).
Examples of how useful these techniques have become is the development of a computational acetyl cholinesterase that, together with RNA-seq, determined insecticide resistance mutations in insects like Plutella xylostella, Chilo suppressalis and Bemisia tabaci (Guo et al., 2017). Interestingly, CSPs and OBPs have been found to be involved in insecticide contact in P. xylostella, where CSP4, CSP8 and OBP13 are reported to be up-regulated after permethrin treatment (Bautista et al., 2015). Furthermore, it has been shown that the use of NGS can provide larger and more efficient large-scale RNA interference (RNAi) targets to knock-down gene of interests, allowing their application along with more conventional pest control strategies (Wang et al., 2011). As shown in Figure 1, over the years antennal transcriptome studies have steadily increased, and the identification of chemosensory proteins has gained great scope for researchers, with emphasis on non-model insects. It is believed that in addition to the large amount of data from transcriptomics, the next challenge is genomic sequencing and the subsequent collection of massive datasets for non-model species.
Spread of Non-Model Insects: Road to be Study Models of Economic Importance
The growing and uncontrollable demographic trends have caused great concern because of an increased demand for food. Estimates have been made that by the year 2050 this demand will be up to 60% higher (Alexandratos and Bruinsma, 2012). In that sense, agriculture plays a significant role in food production (Tilman et al., 2011). Thus, it is estimated that with globalization more than one-tenth of all known pests have reached other countries where their hosts grow (Bebber et al., 2014). More discouraging is the fact that climate change can affect insect pest establishment, with increased invasion by insects due to a mixture of factors, such as changes in precipitation and increases in both atmospheric CO2 and temperature (Skendžić et al., 2021). On the other hand, it is proposed that a decline in flying insects has occurred in recent years due to intensive agriculture as well as urbanization and overuse of insecticides (Eggleton, 2020). However, we as well as Eggleton (2020) argue that this is a preliminary statement, and that anthropological activity (e.g., agriculture) can selectively support some insect species, such as polyphagous pests, to settle in new geographic areas. Consequently, it is crucial that in the face of a changing world, basic research on a diversity of insects can be performed. In particular, chemosensation (i.e., study of OBPs, CSPs or ORs) can provide relevant information considering it is the main driving force for insect behavior. Our literature analyses suggest a significant increase in studies related to chemosensation of non-model insects during the last decade (Table 2), from 16 to more than 80 published research articles every year. Remarkably, China is positioned as a highly productive country with 435 studies in the last decade followed by the US and France with 27 and 19, respectively. This finding could be related to the diversity of insects that have been studied. For instance, 11 different insect species were studied by 2011, whereas it scaled up to 71 in 2020 and 52 in 2021. Despite this diversity, there are some insects that were not initially considered as models and that have been systematically studied in the last 10 years, such as S. littoralis, A. lineolatus and H. armigera. It is worth mentioning that about 20 studies related to olfaction of each of these species have been published. Hence, these insects can be considered as models for the number of studies in the area. Finally, this summary evidences the impact of agricultural pests in the last few years and how research teams have had to address their control and monitoring.
Among those insects as study models, D. melanogaster can be considered the most famous “workhorse” for bioinformatics, experimental biology and genetics, with 6 Nobel prizes to its credit. However, D. melanogaster is a small representative of a large lineage of Drosophila species. An interesting subgroup of species from melanogaster is the suzukii subgroup, where D. suzukii appears. This fly is a serious pest of berries, laying eggs in healthy unwounded fruits (Sasaki and Sato, 1995). Originally linked to Southeast Asia, particularly Japan, China, Taiwan, North and South Korea, and even the Russian Far East, D. suzukii has spread rapidly across the European and American continents (Cini et al., 2012). Nowadays, it can be found in South America and Africa (Deprá et al., 2014; Hassani et al., 2020). Interestingly, studies around chemosensation in D. suzukii are only recent compared with other insect species mentioned in this review. For instance, 71 OR genes were identified in 2016 by comparative genomics, including D. suzukii, D. biarmipes and D. takahashii (Hickner et al., 2016). More recently, the differential expression of ORs was evaluated, which appeared to be modulated by post-mating status in D. suzukii females (Crava et al., 2019). Likewise, 27 chemosensory genes, such as ORs, OBPs and CSPs, were found to be sex-biased in expression by 2020 (Ahn et al., 2020). Recent evidence in 2021 reported the role of OBP69a and OBP76a in D. suzukii, being functional against floral compounds, such as β-ionone (Zhan et al., 2021). These advances represent an important first step towards likely big discoveries around the chemical ecology of D. suzukii, which could become a study model in the near future.
Another example of a non-model insect that has spread in recent years is the grapevine moth Lobesia botrana. This moth is reported as the most harmful pest in grapevine production in Southern and Central European countries (Moschos et al., 2004), and currently, including South and North American countries, such as the wine-growing regions of Chile, California and Argentina. The first report of L. botrana was in Austria in 1776 by Denis and Schiffermüller; however, its geographic origin remains uncertain. Although this moth is linked to grapes and originally to berries from Daphne gnidium, it is considered a polyphagous insect (Maher and Thiéry, 2006). Given its rapid spread and economic importance, it has been extensively studied in terms of its chemical ecology and pest management (Tasin et al., 2010; Chelef et al., 2020). In relation to chemosensation, recent studies have addressed the identification and functional characterization of olfactory proteins. Thus, a profile of 61 ORs, 35 OBPs and 18 CSPs was reported for L. botrana in 2018 (Rojas et al., 2018), and an OBP tuned to pheromone components was reported in 2019 (Venthur and Xhou, 2018). It is believed that L. botrana, like other Tortricids of economic importance, such as C. pomonella and G. molesta, will likely become a study model in the future.
Besides Dipterans and Lepidopterans, Coleopterans have been a matter of research in recent years. An example is the red palm weevil Rhynchophorus ferrugineus, which attacks palm trees in a mass coordinated process (Oehlschlager, 2016). Originally related to South and Southeast Asia, this insect has reached worldwide distribution (El-Mergawy and Al-Ajlan, 2011; Fouda et al., 2022). In terms of olfaction, important advances have been made in recent years. For example, the OR, RferOR1, has been identified as active against the pheromone ferrugineol ((4RS,5RS)-4-methylnonan-5-ol) and ferrugineone ((4RS)-methylnonan-5-one) (Antony et al., 2016). Likewise, the profile of olfactory proteins (e.g., 37 OBPs, 10 CSPs and 63 ORs, among others) has been reported (Gonzalez et al., 2021). In fact, the genome of R. ferrugineus has been published, suggesting that this insect will become a study model (Hazzouri et al., 2020). Another example are bark beetles, such as Ips typographus, an important forest pest, where olfactory proteins (15 OBPs, 6 CSPs, 3 SNMPs, 43 ORs, 6 GRs and 7 IRs) have been identified and analyzed (Andersson et al., 2013). Recently, putative ligand binding sites have been identified in ORs of this bark beetle that affect the responses of these insect receptors (Yuvaraj et al., 2021).
Other insect species, such as aphids M. persicae and S. avenae as well as Lepidopterans related to stored food pests, such as Plodia interpunctella, are already being studied according to our literature analysis (Supplementary Table S2). Thus, depending on their contribution to insect chemosensation, we argue that these insects (as well as others) can become study models. Nevertheless, we believe that each contribution strengthens the study of olfactory physiology, and pushes the limits towards more in-depth research. Thus, in addition to the economic importance of these insects, over the years the gap between model and non-model insects has been narrowing, leading to an increase in knowledge at the chemosensory level. However, there are still insects that have not been studied or have been little studied. An example is Euborellia annulipes, an important predator of pest insects, whose olfactory system has not yet been studied (da Silva Nunes et al., 2020; Tangkawanit et al., 2021).
Control of Non-Model Insects Still Led by Conventional Integrated Pest Management Strategies
With industrial revolution pushing the limits of productive areas, agriculture was no exception. By 1940 insect pest control strategies were needed to mitigate negative impacts; therefore, insects were controlled using man-made chemicals, called pesticides (Jones, 1998). However, concerns were raised in the ensuing decades because of pesticide persistence, groundwater contamination and appearance of resistance in insects (Hou and Wu, 2010; Hillocks, 2012; Garrigou et al., 2020). Therefore, alternative approaches emerged, such as the use of semiochemicals, which served for integrated pest management (IPM) strategies. This term is defined as a pest management strategy that employs methods consistent with economic, ecological and toxicological requirements in order to maintain pests below the economic threshold, giving priority to natural limiting factors (Katsoyannos, 1992; Jones, 1998). Consequently, it was necessary to understand the biology and life cycles of insect pests to apply IPM strategies. In that sense, several olfaction-dependent techniques raised in the context of IPM strategies, such as mating disruption, mass trapping, lure and kill and push-pull (Jones, 1998). For a more in-depth review of these techniques, we suggest readers to check Nesreen and Abd El-Ghany (2019), and Morrison et al. (2021).
During the 2000s, the first steps in understanding insect olfactory systems allowed some advances in the area of insect pest control, where OBPs were used as targets, despite being discovered years before by Vogt and Riddiford (1981). Thus, OBPs were the first target to use to clarify behaviorally active chemicals as a response to issues in semiochemically-based IPM strategies, such as time-consuming experiments for semiochemical identification, extensive bioassays at laboratory and field level, and related costs. Nowadays, OBPs and ORs have been proposed to be key targets for pest control, thus ligand binding to these proteins could interfere with specific insect behaviors (Venthur and Zhou, 2018). Therefore, the last decade has been marked by the development of reverse chemical ecology approaches, which take advantage of olfactory proteins (i.e., OBPs or ORs) to identify novel potent semiochemicals for pest control (Leal, 2005). The first successful study was on the OBP1 of mosquito C. quinquefasciatus, where trimethylamine and nonanal exhibited potent attraction (Leal et al., 2008). More recently, OR36 of C. quinquefasciatus has been studied, which from a panel of 230 odorants, acetaldehyde was the strongest in activating OR36 (Choo et al., 2018). Likewise, 4-methylcyclohexanol was found as a specific activator of OR80 in Chagas vector Rhodnius prolixus, being repellent in behavioral assays (Franco et al., 2018). In addition, with scientific advances in the area, reverse chemical ecology has been implemented in non-model insects of agricultural importance. In the oriental fruit moth G. molesta, it was demonstrated through behavioral and field trapping assays that the compound codlemone ((E,E)-8,10-dodecadienol) is an excellent pheromone synergist that can be detected by GmolPBP2 and also provides an optimization of commercial sexual attractants used in the control of this pest (Liu et al., 2022).
It is worth noting that in recent years reverse chemical ecology has evolved into a genetically-based approach. For example, in the cotton leafworm S. littoralis, the CRISPR-Cas9 technique was used to edit the genome by eliminating the Orco gene (Koutroumpa et al., 2016). Results showed that more than 80% of the individuals had mutations in the Orco gene, passing them on to the next generation, which caused problems in the olfactory detection of pheromones by this moth. This appears to be the first step into new, ethically debatable, control techniques for specific species.
Despite the progress in olfactory physiology in both model and non-model insects, the jump towards IPM strategies based on OBPs, CSPs and/or ORs, remains elusive. Currently, many insect pests are still monitored and/or controlled by conventional IPM strategies. An example is the use of pheromones, which have proven to be a successful method (i.e., mating disruption) for several pest species, mainly in Lepidoptera (Conchou et al., 2019), by releasing high concentrations of pheromones in crop fields (Reddy and Guerrero, 2010; Benelli et al., 2019). Recently, sex pheromone-baited traps ((Z,Z)-7,10-hexadecadienal) were designed for monitoring the apple orchard pest Chilecomadia valdiviana, certainly a non-model insect (Barros-Parada et al., 2021). The mating disruption technique has also been used against the olive moth Prays oleae to suppress damage in olives (Ortiz et al., 2021). The authors’ findings suggest that aerosol-type traps could yield >75% of male captures and 20% less damage in olives.
Other types of conventional IPM techniques are also still used. For example, the push-pull strategy is a technique that repels an insect pest from a crop of interest, while attracting it towards an external location. A reduction in population of leafhopper Empoasca flavescens has been reported by using repellents and attractants to control the insect (Niu et al., 2022). Likewise, mass trapping has been useful in controlling medfly Ceratitis capitata, which functions as powerful trap full of attractants (Hafsi et al., 2019). For C. capitata, two food attractants (amine and organic acids) were released from baited sprays, which resulted in low damage (2.2%–3.9%) to citrus fruits.
To date, it is clear that IPM strategies are pivotal in insect pest control and monitoring in order to pursue environmentally friendly methods. However, with the enormous number of olfactory proteins identified year by year in response to the spread of non-model insects, we strongly believe that significant discoveries and technological advances are yet to come.
Concluding Remarks
The study of insect olfaction is no longer a novel approach for integrated pest management. It has evolved from the study of a few insect species and their olfactory proteins to dozens of insect species and several type of proteins. This might be led by such factors as insecticide resistance, intensive agriculture and climate change that have affected spread and establishment of pests worldwide. Consequently, sequencing technologies are becoming increasingly feasible for studying insect olfaction. This has resulted in groundbreaking evolutionary studies as well as improved integrated pest management strategies. Particularly, non-model insects have provided us with a profound knowledge of olfaction, from structural features of receptors (A. bakeri Orco) to tissue-biased expression with special functions (H. armigera OBP3). Furthermore, we believe that the study of non-model insects, in addition to providing multiple benefits for agriculture, can pave the way to face future challenges in a changing ecosystem.
Author Contributions
PL wrote all sections. PL and HV developed the tables and figures. HV, AM, and AQ conceived the idea for the review article. All authors contributed to the article and approved the submitted version.
Conflict of Interest
The authors declare that the research was conducted in the absence of any commercial or financial relationships that could be construed as a potential conflict of interest.
Publisher’s Note
All claims expressed in this article are solely those of the authors and do not necessarily represent those of their affiliated organizations, or those of the publisher, the editors and the reviewers. Any product that may be evaluated in this article, or claim that may be made by its manufacturer, is not guaranteed or endorsed by the publisher.
Acknowledgments
We thank ANID scholarship N° 21201517 and Fomento publicaciones científicas en áreas temáticas para estudiantes de postgrado, Universidad de La Frontera.
Supplementary Material
The Supplementary Material for this article can be found online at: https://www.frontiersin.org/articles/10.3389/fphys.2022.924750/full#supplementary-material
References
Abuin L., Bargeton B., Ulbrich M. H., Isacoff E. Y., Kellenberger S., Benton R., et al. (2011). Functional architecture of olfactory ionotropic glutamate receptors. Neuron 69 (1), 44–60. doi:10.1016/j.neuron.2010.11.042
Aburto C., Godoy R., Bardehle L., Quiroz A., Mutis A., Venthur H., et al. (2019). Antennal morphology and localization of a general odorant-binding protein in the great wax moth, Galleria mellonella (Lepidoptera: Pyralidae). J. Apic. Res. 59 (4), 472–485. doi:10.1080/00218839.2019.1702325
Acar E. B., Medina J. C., Lee M. L., Booth G. M. (2001). Olfactory behavior of convergent lady beetles (Coleoptera: Coccinellidae) to alarm pheromone of green peach aphid (Hemiptera: Aphididae). Can. Entomol. 133 (3), 389–397. doi:10.4039/ENT133389-3
Adams M. D., Celniker S. E., Holt R. A., Evans C. A., Gocayne J. D., Amanatides P. G., et al. (2000). The genome sequence of Drosophila melanogaster. Science 287 (5461), 2185–2195. American Association for the Advancement of Science. doi:10.1126/science.287.5461.2185
Aguilera A. (1995). Plagas subterráneas, págs. 85-140 En: Centro de Investigación INIA-Carillanca. Seminario de protección vegetal. Serie Carillanca. Temuco, Chile.
Ahn S. J., Oh H. W., Corcoran J., Kim J. A., Park K. C., Park C. G., et al. (2020). Sex-biased gene expression in antennae of Drosophila suzukii. Arch. Insect Biochem. Physiol. 104 (1), e21660. doi:10.1002/ARCH.21660
Akhtar M. H., Usmani M. K., Nayeem M. R., Kumar H. (2012). Species Diversity and abundance of Grasshopper fauna (Orthoptera) in rice ecosystem. Ann. Biol. Res. 3 (5), 2190–2193.
Alexandratos N., Bruinsma J. (2012). World agriculture towards 2030/2050: The 2012 revision. Rome: FAO, Agricultural Development Economics Division. WORLD AGRICULTURE. doi:10.22004/AG.ECON.288998
Andersson M. N., Grosse-Wilde E., Keeling C., Bengtsson J., Yuen M., Li M., et al. (2013). Análisis del transcriptoma antenal de las familias de genes quimiosensoriales en los escarabajos descortezadores que matan árboles, Ips typographus y Dendroctonus ponderosae (Coleoptera: Curculionidae: Scolytinae)Purification, structural characterization, cloning and immunocytochemical localization of chemoreception proteins from Schistocerca gregaria. Eur. J. Biochem.European J. Biochem. 14262 (3), 198745–198754. doi:10.1186/1471-2164-14-198Angeli10.1046/j.1432-1327.1999.00438.x
Antony B., Soffan A., Jakše J., Abdelazim M. M., Aldosari S. A., Aldawood A. S., et al. (2016). Identification of the genes involved in odorant reception and detection in the palm weevil Rhynchophorus ferrugineus, an important quarantine pest, by antennal transcriptome analysis. BMC Genomics 17, 69. doi:10.1186/s12864-016-2362-6
Arora S., Sahni D. (2016). Pesticides effect on soil microbial ecology and enzyme activity- an overview. J. Appl. Nat. Sci. 8 (2), 1126–1132. doi:10.31018/JANS.V8I2.929
Artigas J. (1994). “Hylamorpha elegans (Burmeister). Pololo San Juan verde (San juan green beetle),” in Economic Entomology. Insects of agricultural, forestry, medical and veterinary interest (Concepción, Chile: University of Concepción Editorial). Universidad de Concepción.
Attia M. A., Wahba T. F., Shaarawy N., Moustafa F. I., Guedes R. N. C., Dewer Y., et al. (2020). Stored grain pest prevalence and insecticide resistance in Egyptian populations of the red flour beetle Tribolium castaneum (Herbst) and the rice weevil Sitophilus oryzae (L.). J. Stored Prod. Res. 87, 101611. doi:10.1016/J.JSPR.2020.101611
Barros-Parada W., Fuentes-Contreras E., Bergmann J., Herrera H., Kinsho T., Miyake Y., et al. (2021). Monitoring Chilecomadia valdiviana (Lepidoptera: Cossidae) using sex pheromone-baited traps in Apple orchards in Chile. Insects 12, 511. doi:10.3390/insects12060511
Bass C., Puinean A. M., Zimmer C. T., Denholm I., Field L. M., Foster S. P., et al. (2014). The evolution of insecticide resistance in the peach potato aphid, Myzus persicae. Insect biochem. Mol. Biol. 51 (1), 41–51. doi:10.1016/J.IBMB.2014.05.003
Bastin-Héline L., de Fouchier A., Cao S., Koutroumpa F., Caballero-Vidal G., Robakiewicz S., et al. (2019). A novel lineage of candidate pheromone receptors for sex communication in moths. ELife 8, e49826. doi:10.7554/eLife.49826
Baudach A., Vilcinskas A. (2021). The European map butterfly Araschnia levana as a model to study the molecular basis and evolutionary ecology of seasonal polyphenism. Insects 12 (4), 325. doi:10.3390/insects12040325
Bautista M. A. M., Bhandary B., Wijeratne A. J., Michel A. P., Hoy C. W., Mittapalli O., et al. (2015). Evidence for trade-offs in detoxification and chemosensation gene signatures in Plutella xylostella. Pest Manag. Sci. 71 (3), 423–432. doi:10.1002/PS.3822
Bebber D. P., Holmes T., Gurr S. J. (2014). The global spread of crop pests and pathogens. Glob. Ecol. Biogeogr. 23 (12), 1398–1407. doi:10.1111/GEB.12214
Benelli G., Lucchi A., Thomson D., Ioriatti C. (2019). Sex pheromone aerosol devices for mating disruption: Challenges for a brighter future. Insects 1010 (10), 308. Page 308. doi:10.3390/INSECTS10100308
Bengtsson J. M., Trona F., Montagné N., Anfora G., Ignell R., Witzgall P., et al. (2012). Putative chemosensory receptors of the codling moth, Cydia pomonella, identified by antennal transcriptome analysis. PLOS ONE 7 (2), e31620. doi:10.1371/JOURNAL.PONE.0031620
Bian L., Li Z. Q., Ma L., Cai X. M., Luo Z. X., Chen Z. M., et al. (2018). Identification of the genes in tea leafhopper, Empoasca onukii (Hemiptera: Cicadellidae), that encode odorant-binding proteins and chemosensory proteins using transcriptome analyses of insect heads. Appl. Entomol. Zool. 53, 93–105. doi:10.1007/s13355-017-0533-9
Bin S. Y., Qu M. Q., Pu X. H., Wu Z. Z., Lin J. T. (2017). Antennal transcriptome and expression analyses of olfactory genes in the sweetpotato weevil Cylas formicarius. Sci. Rep. 7, 11073. doi:10.1038/s41598-017-11456-x
Breer H., Raming K., Krieger J. (1994). Signal recognition and transduction in olfactory neurons. Biochim. Biophys. Acta 1224, 277–287. doi:10.1016/0167-4889(94)90201-1
Brisson J. A., Stern D. L. (2006). The pea aphid, Acyrthosiphon pisum: An emerging genomic model system for ecological, developmental and evolutionary studies. BioEssays 28 (7), 747–755. doi:10.1002/BIES.20436
Butenandt A. (1963). Bombykol, the sex-attractive substance of the silk worm, Bombyx mori. Annual Lecture 1962 before the Society of Endocrinology. J. Endocrinol. 27, IX–XVI.
Butenandt A. (1959). Geschlechtsspezifische lockstoffe der Schmetterlinge. Max-Planck-Gesellschaft Jhb., 23–32.
Butterwick J. A., del Mármol J., Kim K. H., Kahlson M. A., Rogow J. A., Walz T., et al. (2018). Cryo-EM structure of the insect olfactory receptor Orco. Nature 560 (7719), 447–452. doi:10.1038/s41586-018-0420-8
Calvello M., Guerra N., Brandazza A., D’Ambrosio C., Scaloni A., Dani F. R., et al. (2003). Soluble proteins of chemical communication in the social wasp Polistes dominulus. Cell. Mol. Life Sci. 60 (9), 1933–1943. doi:10.1007/s00018-003-3186-5
Cao D., Liu Y., Wei J., Liao X., Walker W. B., Li J., et al. (2014). Identification of candidate olfactory genes in Chilo suppressalis by antennal transcriptome analysis. Int. J. Biol. Sci. 10, 846–860. doi:10.7150/ijbs.9297
Cao X., Jiang H. (2017). An analysis of 67 RNA-seq datasets from various tissues at different stages of a model insect, Manduca sexta. BMC Genomics 18 (1), 7961. doi:10.1186/S12864-017-4147-Y
Chang H., Liu Y., Ai D., Jiang X., Dong S., Wang G., et al. (2017). A pheromone antagonist regulates optimal mating time in the moth Helicoverpa armigera. Curr. Biol. 27 (11), 1610–1615. e3. doi:10.1016/j.cub.2017.04.035
Chelef M., Hemida H., Hassani A., Mazrou K. (2020). Efficacy of three vegetal powders against Sitophilus granarius in stored wheat and the evaluation of their effect on grain parameters. Int. J. Environ. Stud. 78 (4), 1–17. doi:10.1080/00207233.2020.184555610.1080/00207233.2020.1845556
Chen H., Lin L., Xie M., Zhang G., Su W. (2014). De novo sequencing, assembly and characterization of antennal transcriptome of Anomala corpulenta Motschulsky (Coleoptera: Rutelidae). PLoS ONE 9 (12), e114238. doi:10.1371/journal.pone.0114238
Choo Y. M., Xu P., Hwang J. K., Zeng F., Tan K., Bhagavathy G., et al. (2018). Reverse chemical ecology approach for the identification of an oviposition attractant for Culex quinquefasciatus. Proc. Natl. Acad. Sci. U. S. A. 115, 714–719. doi:10.1073/pnas.1718284115
Chu F., Klobasa W. A., Lorenzen M. (2014). Genetics and genomics of Tribolium Medea elements. J. TSAE. doi:10.14455/DOA.res.2014.14
Cilia G., Nanetti A. (2021). Identification of odorant-binding proteins (OBPs) in Aethina tumida A Novel TaqMan ® assay for Nosema ceranae quantification in honey bee, based on the protein coding gene Hsp70 View project Leptospirosis in wild animals View project. from https://www.researchgate.net/publication/350384082 (Retrieved July 22, 2021).
Cini A., Ioriatti C., Anfora G. (2012). A review of the invasion of Drosophila suzukii in Europe and a draft research agenda for integrated pest management. Bull. Insectology 65 (1), 149–160. www.eppo.org.
Clyne P. J., Warr C. G., Freeman M. R., Lessing D., Kim J., Carlson J. R., et al. (1999). A novel family of divergent seven-transmembrane proteins: Candidate odorant receptors in Drosophila. Neuron 22 (2), 327–338. doi:10.1016/S0896-6273(00)81093-4
Collins M., Weast R. (1961). Wild silk moths of the United States: Saturniinae. Cedar Rapids, Iowa: Collins Radio Company, 138.
Conchou L., Lucas P., Meslin C., Proffit M., Staudt M., Renou M., et al. (2019). Insect odorscapes: From plant volatiles to natural olfactory scenes. Front. Physiol. 10 (JUL), 972. doi:10.3389/fphys.2019.00972
Corcoran J. A., Jordan M. D., Carraher C., Newcomb R. D. (2014). A novel method to study insect olfactory receptor function using HEK293 cells. Insect biochem. Mol. Biol. 54, 22–32. doi:10.1016/J.IBMB.2014.08.005
Cranshaw W. (2004). Garden insects of North America : The ultimate guide to backyard bugs. Princeton University Press, 656.
Crava C. M., Sassù F., Tait G., Becher P. G., Anfora G. (2019). Functional transcriptome analyses of Drosophila suzukii antennae reveal mating-dependent olfaction plasticity in females. Insect biochem. Mol. Biol. 105, 51–59. doi:10.1016/J.IBMB.2018.12.012
Croset V., Rytz R., Cummins S. F., Budd A., Brawand D., Kaessmann H., et al. (2010). Ancient protostome origin of chemosensory ionotropic glutamate receptors and the evolution of insect taste and olfaction. PLoS Genet. 6 (8), e1001064. doi:10.1371/journal.pgen.1001064
Cui H. H., Gu S. H., Zhu X. Q., Wei Y., Liu H. W., Khalid H. D., et al. (2017). Odorant-binding and chemosensory proteins identified in the antennal transcriptome of Adelphocoris suturalis Jakovlev. Comp. Biochem. Physiol. Part D. Genomics Proteomics 24, 139–145. doi:10.1016/j.cbd.2016.03.001
Cui Y., Kang C., Wu Z., Lin J. (2019). Identification and expression analyses of olfactory gene families in the rice grasshopper, Oxya chinensis, from antennal transcriptomes. Front. Physiol. 0 (SEP), 1223. doi:10.3389/FPHYS.2019.01223
da Silva Nunes G., Truzi C. C., Cardoso C. P., Vieira N. F., Ramalho D. G., de Souza J. M., et al. (2020). Temperature-dependent functional response of Euborellia annulipes (Dermaptera: Anisolabididae) preying on Plutella xylostella (Lepidoptera: Plutellidae) larvae. J. Therm. Biol. 93, 102686. doi:10.1016/J.JTHERBIO.2020.102686
Damberger F. F., Michel E., Ishida Y., Leal W. S., Wüthrich K. (2013). Pheromone discrimination by a pH-tuned polymorphism of the Bombyx mori pheromone-binding protein. Proc. Natl. Acad. Sci. U. S. A. 110 (46), 18680–18685. doi:10.1073/PNAS.1317706110
Damberger F., Horst R., Wüthrich K., Peng G., Nikonova L., Leal W. S., et al. (2000). NMR characterization of a pH-dependent equilibrium between two folded solution conformations of the pheromone-binding protein from Bombyx mori. Protein Sci. 9 (5), 1038–1041. doi:10.1110/PS.9.5.1038
De Bruyne M., Baker T. C. (2008). Odor detection in insects: Volatile codesJ. Chem. Ecol.7, 34. Springer, 882–897. doi:10.1007/s10886-008-9485-4
de Fouchier A., Walker W. B., Montagné N., Steiner C., Binyameen M., Schlyter F., et al. (2017). Functional evolution of Lepidoptera olfactory receptors revealed by deorphanization of a moth repertoire. Nat. Commun. 8, 15709. doi:10.1038/ncomms15709
Dedryver C. A., Le Ralec A., Fabre F. (2010). The conflicting relationships between aphids and men: A review of aphid damage and control strategies. C. R. Biol. 333 (6–7), 539–553. doi:10.1016/J.CRVI.2010.03.009
Deng S., Yin J., Zhong T., Cao Y., Li K. (2012). Function and immunocytochemical localization of two novel odorant- binding proteins in olfactory sensilla of the scarab beetle Holotrichia oblita Faldermann (Coleoptera: Scarabaeidae). Chem. Senses 37 (2), 141–150. doi:10.1093/chemse/bjr084
Deprá M., Poppe J. L., Schmitz H. J., de Toni D. C., Valente V. L. S. (2014). The first records of the invasive pest Drosophila suzukii in the South American continent. J. Pest Sci. (2004). 87 (3), 379–383. doi:10.1007/s10340-014-0591-5
Di Luccio E. di, Ishida Y., Leal W. S., Wilson D. K. (2013). Crystallographic observation of pH-induced conformational changes in the Amyelois transitella pheromone-binding protein AtraPBP1. PLOS ONE 8 (2), e53840. doi:10.1371/JOURNAL.PONE.0053840
D’Onofrio C., Zaremska V., Zhu J., Knoll W., Pelosi P. (2020). Ligand-binding assays with OBPs and CSPs. Methods Enzymol. 642, 229–258. doi:10.1016/BS.MIE.2020.05.006
Eggleton P. (2020). The State of the World’s Insects, 45, 61–82. doi:10.1146/Annurev-Environ-012420-05003510.1146/ANNUREV-ENVIRON-012420-050035
Ekblom R., Galindo J. (2010). Applications of next generation sequencing in molecular ecology of non-model organisms. Heredity 1107 (1), 1–15. doi:10.1038/hdy.2010.152
El-Mergawy R. A. A. M., Al-Ajlan A. M. (2011). Red palm weevil, Rhynchophorus ferrugineus (olivier): Economic importance, biology, biogeography and integrated pest management. J. Agric. Sci. Technol. A 1, 1–23.
Enjin A., Zaharieva E. E., Frank D. D., Mansourian S., Suh G. S. B., Gallio M., et al. (2016). Humidity sensing in Drosophila. Curr. Biol. 26 (10), 1352–1358. doi:10.1016/J.CUB.2016.03.049
Falchetto M., Ciossani G., Scolari F., Cosimo A. Di, Nenci S., Field L. M., et al. (2019). Structural and biochemical evaluation of Ceratitis capitata odorant-binding protein 22 affinity for odorants involved in intersex communication. Insect Mol. Biol. 28 (3), 431–443. doi:10.1111/IMB.12559
Fan J., Francis F., Liu Y., Chen J., Cheng D., Mol G. (2011). An overview of odorant-binding protein functions in insect peripheral olfactory reception. Genet. Mol. Res. 10 (4), 3056–3069. doi:10.4238/2011.December.8.2
Fandino R. A., Haverkamp A., Bisch-Knaden S., Zhang J., Bucks S., Thi T.-A. N., et al. (2019). Mutagenesis of orco impairs foraging but not oviposition in the hawkmoth Manduca sexta. BioRxiv, 541201. doi:10.1101/541201
FAO (2017). Plant health and food security. International plant protection convention. Available at: http://www.fao.org/3/i7829e/i7829e.pdf.
Farias J. R., andow D. A., Horikoshi R. J., Sorgatto R. J., Fresia P., dos Santos A. C., et al. (2014). Field-evolved resistance to Cry1F maize by Spodoptera frugiperda (Lepidoptera: Noctuidae) in Brazil. Crop Prot. 64, 150–158. doi:10.1016/J.CROPRO.2014.06.019
Fay H. A. C. (2012). A highly effective and selective male lure for Bactrocera jarvisi (Tryon) (Diptera: Tephritidae). Aust. J. Entomol. 51 (3), 189–197. doi:10.1111/J.1440-6055.2011.00847.X
Feng B., Guo Q., Zheng K., Qin Y., Du Y. (2017). Antennal transcriptome analysis of the piercing moth Oraesia emarginata (Lepidoptera: Noctuidae). PLoS One 12, e0179433. doi:10.1371/journal.pone.0179433
Feng B., Lin X., Zheng K., Qian K., Chang Y., Du Y., et al. (2015). Transcriptome and expression profiling analysis link patterns of gene expression to antennal responses in Spodoptera litura. BMC Genomics 16, 269. doi:10.1186/s12864-015-1375-x
Flechtmann C. H. W. (2013). A new species of Neotetranychus Trägårdh (Acari, Prostigmata, Tetranychidae) from Thailand with a key to world species. Persian J. Acarol. 2 (1), 35–40. doi:10.22073/pja.v2i1.9947
Foret S., Wanner K. W., Maleszka R. (2007). Chemosensory proteins in the honey bee: Insights from the annotated genome, comparative analyses and expressional profiling. Insect biochem. Mol. Biol. 37 (1), 19–28. doi:10.1016/J.IBMB.2006.09.009
Fouda M. M. A., Tufail M., Takeda M., Mahmoud S. H. (2022). DNA barcoding and population genetic structure of the red palm weevil, Rhynchophorus ferrugineus (Coleoptera: Curculionidae) in Egypt based on mtDNA sequencing. Biologia 77 (44), 1017–1025. doi:10.1007/S11756-022-01033-7
Franco T. A., Xu P., Brito N. F., Oliveira D. S., Wen X., Moreira M. F., et al. (2018). Reverse chemical ecology-based approach leading to the accidental discovery of repellents for Rhodnius prolixus, a vector of Chagas diseases refractory to DEET. Insect biochem. Mol. Biol. 103, 46–52. doi:10.1016/J.IBMB.2018.10.004
Fuchs B. B., O’Brien E., Khoury J. B. E., Mylonakis E. (2010). Methods for using Galleria mellonella as a model host to study fungal pathogenesis. Virulence 1 (6), 475–482. doi:10.4161/viru.1.6.12985
Gao Q., Chess A. (1999). Identification of candidate Drosophila olfactory receptors from genomic DNA sequence. Genomics 60 (1), 31–39. doi:10.1006/geno.1999.5894
Garrigou A., Laurent C., Berthet A., Colosio C., Jas N., Daubas-Letourneux V., et al. (2020). Critical review of the role of PPE in the prevention of risks related to agricultural pesticide use. Saf. Sci. 123, 104527. doi:10.1016/j.ssci.2019.104527
Gong X., Xie S., Yang P., Guo L., Chen D., Che X., et al. (2020). Analysis of the antennal transcriptome and olfaction-related genes of Agrilus zanthoxylumi (Coleoptera: Buprestidae). Acta Entomol. Sin. 63. https://www.cabdirect.org/cabdirect/abstract/20210022226.
Gonzalez F., Johny J., Walker W. B., Guan Q., Mfarrej S., Jakše J., et al. (2021). Antennal transcriptome sequencing and identification of candidate chemoreceptor proteins from an invasive pest, the American palm weevil, Rhynchophorus palmarum. Sci. Rep. 11 (1), 83341. doi:10.1038/s41598-021-87348-y
Gonzalez F., Witzgall P., Walker W. B. (2017). Antennal transcriptomes of three tortricid moths reveal putative conserved chemosensory receptors for social and habitat olfactory cues. Sci. Rep. 7, 41829. doi:10.1038/srep41829
Gonzalez R. (1989). Insectos y ácaros de importancia agrícola y Cuarentenaria en Chile. Santiago de Chile: Ograma.
González-González A., Rubio-Meléndez M. E., Ballesteros G. I., Ramírez C. C., Palma-Millanao R. (2019). Sex- and tissue-specific expression of odorant-binding proteins and chemosensory proteins in adults of the scarab beetle Hylamorpha elegans (Burmeister) (Coleoptera: Scarabaeidae). PeerJ 7 (6), e7054. doi:10.7717/PEERJ.7054
Grosse-Wilde E., Kuebler L. S., Bucks S., Vogel H., Wicher D., Hansson B. S., et al. (2011). Antennal transcriptome of Manduca sexta. Proc. Natl. Acad. Sci. U. S. A. 108 (18), 7449–7454. doi:10.1073/PNAS.1017963108
Gu S. H., Sun L., Yang R. N., Wu K. M., Guo Y. Y., Li X. C., et al. (2014). Molecular characterization and differential expression of olfactory genes in the antennae of the black cutworm moth Agrotis ipsilon. PLoS One 9, e103420. doi:10.1371/journal.pone.0103420
Gu T., Huang K., Tian S., Sun Y., Li H., Chen C., et al. (2019). Antennal transcriptome analysis and expression profiles of odorant binding proteins in Clostera restitura. Comp. Biochem. Physiol. Part D. Genomics Proteomics 29, 211–220. doi:10.1016/J.CBD.2018.12.002
Gu X. C., Zhang Y. N., Kang K., Dong S. L., Zhang L. W. (2015). Antennal transcriptome analysis of odorant reception genes in the red turpentine beetle (RTB), Dendroctonus valens. PLoS One 10, e0125159. doi:10.1371/journal.pone.0125159
Guo B., Hao E., Qiao H., Wang J., Wu W., Zhou J., et al. (2021). Antennal transcriptome analysis of olfactory genes and characterizations of odorant binding proteins in two woodwasps, Sirex noctilio and Sirex nitobei (Hymenoptera: Siricidae). BMC Genomics (1221), 172. doi:10.1186/S12864--021-07452-1
Guo D., Luo J., Zhou Y., Xiao H., He K., Yin C., et al. (2017). Ace: An efficient and sensitive tool to detect insecticide resistance-associated mutations in insect acetylcholinesterase from RNA-Seq data. BMC Bioinforma. 18 (1), 330. doi:10.1186/s12859-017-1741-6
Hafsi A., Rahmouni R., Othman S. B., Abbes K., Elimem M., Chermiti B., et al. (2019). Mass trapping and bait station techniques as alternative methods for IPM of Ceratitis capitata Wiedmann (Diptera: Tephritidae) in citrus orchards. Orient. Insects 54, 285–298. doi:10.1080/00305316.2019.1623133
Hall D. W. (2015). Polyphemus Moth Antheraea polyphemus (Cramer) (Insecta: Lepidoptera: Saturniidae: Saturniinae), 9. Available at: https://edis.ifas.ufl.edu/pdffiles/IN/IN94500.pdf.
Hamiaux C., Carraher C., Löfstedt C., Corcoran J. A. (2020). Crystal structure of Epiphyas postvittana pheromone binding protein 3. Sci. Rep. 10 (11), 16366. doi:10.1038/s41598-020-73294-8
Han H., Liu Z., Meng F., Jiang Y., Cai J. (2020). Identification of olfactory genes of a forensically important blow fly, Aldrichina grahami (Diptera: Calliphoridae). PeerJ 8, e9581. doi:10.7717/PEERJ.9581
Hassanali A., Herren H., Khan Z. R., Pickett J. A., Woodcock C. M. (2008). Integrated pest management: The push-pull approach for controlling insect pests and weeds of cereals, and its potential for other agricultural systems including animal husbandry. Philos. Trans. R. Soc. Lond. B Biol. Sci. 363 (1491), 611–621. doi:10.1098/rstb.2007.2173
Hassani I. M., Behrman E. L., Prigent S. R., Gidaszewski N., Raveloson Ravaomanarivo L. H., Suwalski A., et al. (2020). First occurrence of the pest Drosophila suzukii (Diptera: Drosophilidae) in the Comoros archipelago (western Indian ocean). Afr. Entomol. 28 (1), 78. doi:10.4001/003.028.0078
Hazzouri K. M., Sudalaimuthuasari N., Kundu B., Nelson D., Al-Deeb M. A., le Mansour A., et al. (2020). The genome of pest Rhynchophorus ferrugineus reveals gene families important at the plant-beetle interface. Commun. Biol. 3 (11), 323. doi:10.1038/s42003-020-1060-8
He Y., Wang K., Zeng Y., Guo Z., Zhang Y., Wu Q., et al. (2020). Analysis of the antennal transcriptome and odorant-binding protein expression profiles of the parasitoid wasp Encarsia formosa. Genomics 112 (3), 2291–2301. doi:10.1016/J.YGENO.2019.12.025
Heisey P. W., Norton G. W. (2007). Chapter 53 Fertilizers and other farm chemicalsHandb. Agric. Econ., 3. Elsevier, 2741–2777. doi:10.1016/S1574-0072(06)03053-2
Hertzman C., Wiens M., Snow B., Kelly S., Calne D. (1994). A case‐control study of Parkinson’s disease in a horticultural region of British Columbia. Mov. Disord. 9 (1), 69–75. doi:10.1002/mds.870090111
Hickner P. v., Rivaldi C. L., Johnson C. M., Siddappaji M., Raster G. J., Syed Z., et al. (2016). The making of a pest: Insights from the evolution of chemosensory receptor families in a pestiferous and invasive fly, Drosophila suzukii. BMC Genomics 17 (1), 648. doi:10.1186/s12864-016-2983-9
Hillocks R. L. (2012). Farming with fewer pesticides: EU pesticide review and resulting challenges for UK agriculture. Crop Prot. 31, 85–93. doi:10.1016/j.cropro.2011.08.008
Holt R. A., Mani Subramanian G., Halpern A., Sutton G. G., Charlab R., Nusskern D. R., et al. (2002). The genome sequence of the malaria mosquito Anopheles gambiae. Science 298 (5591), 129–149. doi:10.1126/science.1076181
Horst R., Damberger F., Luginbühl P., Güntert P., Peng G., Nikonova L., et al. (2001). NMR structure reveals intramolecular regulation mechanism for pheromone binding and release. Proc. Natl. Acad. Sci. U. S. A. 98 (25), 14374–14379. doi:10.1073/PNAS.251532998
Hou B., Wu L. (2010). Safety impact and farmer awareness of pesticide residues. Food Agric. Immunol. 21, 191–200. doi:10.1080/09540105.2010.484858
Huang F., Qureshi J. A., Meagher R. L., Reisig D. D., Head G. P., andow D. A., et al. (2014). Cry1F resistance in fall armyworm Spodoptera frugiperda: Single gene versus pyramided bt maize. PLOS ONE 9 (11), e112958. doi:10.1371/JOURNAL.PONE.0112958
Jacquin-joly E., Merlin C. (20042004). Insect olfactory receptors: Contributions of molecular biology to chemical ecology. J. Chem. Ecol. 30 (12), 122359–122397. doi:10.1007/S10886-004-7941-3
Jia X. J., Wang H. X., Yan Z. G., Zhang M. Z., Wei C. H., Qin X. C., et al. (2016). Antennal transcriptome and differential expression of olfactory genes in the yellow peach moth, Conogethes punctiferalis (Lepidoptera: Crambidae). Sci. Rep. 6, 29067. doi:10.1038/srep29067
Jia X., Zhang X., Liu H., Wang R., Zhang T. (2018). Identification of chemosensory genes from the antennal transcriptome of Indian meal moth Plodia interpunctella. PLoS One 13, e0189889. doi:10.1371/journal.pone.0189889
Jiao Y., Moon S. J., Wang X., Ren Q., Montell C. (2008). Gr64f is required in combination with other gustatory receptors for sugar detection in Drosophila. Curr. Biol. 18 (22), 1797–1801. doi:10.1016/J.CUB.2008.10.009
Jing D., Zhang T., Bai S., He K., Prabu S., Luan J., et al. (2020). Sexual-biased gene expression of olfactory-related genes in the antennae of Conogethes pinicolalis (Lepidoptera: Crambidae). BMC Genomics 21 (1), 244. doi:10.1186/s12864-020-6648-3
Jones O. T. (1998). “Practical applications of pheromones and other semiochemicals,” in Insect pheromones and their use in pest management. Editors P. E. Howse, O. T. Jones, and I. D. R. Stevens (Londo, UK: Champan & Hall), 260–276.
Joshi P. C., Lockwood J. A., Vashishth N., Singh A. (1999). Grasshopper (orthoptera: Acridoidea) community dynamics in a moist deciduous forest in India. J. Orthoptera Res. 8, 17. doi:10.2307/3503420
Kaissling K.-E. (2013). Kinetics of olfactory responses might largely depend on the odorant–receptor interaction and the odorant deactivation postulated for flux detectors. J. Comp. Physiol. A Neuroethol. Sens. Neural Behav. Physiol. 11199 (11), 879–896. doi:10.1007/S00359-013-0812-Z
Kamel F., Engel L. S., Gladen B. C., Hoppin J. A., Alavanja M. C. R., Sandler D. P., et al. (2005). Neurologic symptoms in licensed private pesticide applicators in the agricultural health study. Environ. Health Perspect. 113 (7), 877–882. doi:10.1289/ehp.7645
Kang Z.-W., Liu F.-H., Pang R.-P., Yu W.-B., Tan X.-L., Zheng Z.-Q., et al. (2018). The identification and expression analysis of candidate chemosensory genes in the bird cherry-oat aphid Rhopalosiphum padi (L.). Bull. Entomol. Res. 108 (5), 645–657. doi:10.1017/S0007485317001171
Kang Z. W., Liu F. H., Xu Y. Y., Cheng J. H., Lin X. L., Jing X. F., et al. (2021). Identification of candidate odorant-degrading enzyme genes in the antennal transcriptome of Aphidius gifuensis. Entomological Res. 51 (1), 36–54. doi:10.1111/1748-5967.12489
Katsoyannos P. (1992). Olive pest problems and their control in the near East. Rome: FAO Plant Production and Protection Paper XXXFAO.
Kawahara A. Y., Breinholt J. W. (2014). Phylogenomics provides strong evidence for relationships of butterflies and moths. Proc. Biol. Sci., 20140970. doi:10.1098/rspb.2014.0970
Keeling C., Henderson H., Li M., Dullat H., Ohnishi T., Bohlmann J., et al. (2013). CYP345E2, an antenna-specific cytochrome P450 from the mountain pine beetle, Dendroctonus ponderosae Hopkins, catalyses the oxidation of pine host monoterpene volatiles. Insect biochem. Mol. Biol. 43 (12), 1142–1151. doi:10.1016/J.IBMB.2013.10.001
Keifer M., Firestone J. (2007). Neurotoxicity of pesticides. J. Agromedicine 12 (1), 17–25. doi:10.1300/J096v12n01_03
Khan M. A., Ahmad W. (2019). Synthetic chemical insecticides: Environmental and agro contaminants. Springer, 1–22. doi:10.1007/978-3-030-23045-6_1
Khan S., Uddin M. N., Rizwan M., Khan W., Farooq M., Shah A. S., et al. (2020). Mechanism of insecticide resistance in insects/pests. Pol. J. Environ. Stud. 29 (3), 2023–2030. doi:10.15244/PJOES/108513
Killiny N. (2018). Generous hosts: Why the larvae of greater wax moth, Galleria mellonella is a perfect infectious host model? Virulence 9 (1), 860–865. doi:10.1080/21505594.2018.1454172
Kim S. M., Wang J. W. (2016). Hygrosensation: Feeling wet and cold. Curr. Biol. 26 (10), R408–R410. doi:10.1016/J.CUB.2016.04.040
Knecht Z. A., Silbering A. F., Cruz J., Yang L., Croset V., Benton R., et al. (2017). Ionotropic receptor-dependent moist and dry cells control hygrosensation in Drosophila. ELife 6, e26654. doi:10.7554/ELIFE.26654
Koutroumpa F. A., Monsempes C., François M. C., de Cian A., Royer C., Concordet J. P., et al. (2016). Heritable genome editing with CRISPR/Cas9 induces anosmia in a crop pest moth. Sci. Rep. 6, 29620. doi:10.1038/SREP29620
Kumar H., Panigrahi M., Chhotaray S., Bhanuprakash V., Shandilya R., Sonwane A., et al. (2018)., 11. Veterinary World, 1043–1046. doi:10.14202/vetworld.2018.1043-1046Red flour beetle (Tribolium castaneum): From population genetics to functional genomicsVeterinary World8
Lartigue A., Campanacci V., Roussel A., Larsson A. M., Jones T. A., Tegoni M., et al. (2002). X-Ray structure and ligand binding study of a moth chemosensory protein. J. Biol. Chem. 277 (35), 32094–32098. doi:10.1074/JBC.M204371200
Lautenschlager C., Leal W. S., Clardy J. (2007). Bombyx mori pheromone-binding protein binding non-pheromone ligands: Implications for pheromone recognition. Structure 15 (9), 1148–1154. doi:10.1016/J.STR.2007.07.013
Lautenschlager C., Leal W. S., Clardy J. (2005). Coil-to-helix transition and ligand release of Bombyx mori pheromone-binding protein. Biochem. Biophys. Res. Commun. 335 (4), 1044–1050. doi:10.1016/J.BBRC.2005.07.176
Leal W. S., Barbosa R. M. R., Xu W., Ishida Y., Syed Z., Latte N., et al. (2008). Reverse and conventional chemical ecology approaches for the development of oviposition attractants for Culex mosquitoes. PLOS ONE 3 (8), e3045. doi:10.1371/JOURNAL.PONE.0003045
Leal W. S. (2013). Odorant reception in insects: Roles of receptors, binding proteins, and degrading enzymes. Annu. Rev. Entomol. 58 (1), 373–391. doi:10.1146/annurev-ento-120811-153635
Leal W. S. (2005). “Pheromone reception,” in Topics in current chemistry (Berlin, Heidelberg: Springer), 1–36. doi:10.1007/B98314
Lee D., Damberger F. F., Peng G., Horst R., Güntert P., Nikonova L., et al. (2002). NMR structure of the unliganded Bombyx mori pheromone-binding protein at physiological pH. FEBS Lett. 531 (2), 314–318. doi:10.1016/S0014-5793(02)03548-2
Leitch O., Papanicolaou A., Lennard C., Kirkbride K. P., Anderson A. (2015). Chemosensory genes identified in the antennal transcriptome of the blowfly Calliphora stygia. BMC Genomics 16, 255. doi:10.1186/s12864-015-1466-8
Leyrer R. L., Monroe R. E. (1973). Isolation and identification of the scent of the moth, Galleria mellonella, and a revaluation of its sex pheromone. J. Insect Physiology 19 (11), 2267–2271. doi:10.1016/0022-1910(73)90143-1
Li F., Venthur H., Wang S., Homem R. A., Zhou J.-J. (2021). Evidence for the involvement of the chemosensory protein AgosCSP5 in resistance to insecticides in the cotton aphid, Aphis gossypii. Insects 1212 (4), 335. Page 335. doi:10.3390/INSECTS12040335
Li F., Zhao X., Li M., He K., Huang C., Zhou Y., et al. (2019). Insect genomes: Progress and challenges. Insect Mol. Biol. 28 (6), 739–758. doi:10.1111/IMB.12599
Li G., Du J., Li Y., Wu J. (2015b). Identification of putative olfactory genes from the oriental fruit moth Grapholita molesta via an antennal transcriptome analysis. 1–30. doi:10.1371/journal.pone.0142193
Li H. L., Ni C. X., Tan J., Zhang L. Y., Hu F. L. (2016). Chemosensory proteins of the eastern honeybee, Apis cerana: Identification, tissue distribution and olfactory related functional characterization. Comp. Biochem. Physiol. B Biochem. Mol. Biol. 194-195, 11–19. doi:10.1016/J.CBPB.2015.11.014
Li X. M., Zhu X. Y., Wang Z. Q., Wang Y., He P., Chen G., et al. (2015). Candidate chemosensory genes identified in Colaphellus bowringi by antennal transcriptome analysis. BMC Genomics 16, 1028. doi:10.1186/s12864-015-2236-3
Liu H., Zhang X., Liu C., Liu Y., Mei X., Zhang T., et al. (2019). Identification and expression of candidate chemosensory receptors in the white-spotted flower chafer, Protaetia brevitarsis. Sci. Rep. 9 (11), 3339. doi:10.1038/s41598-019-38896-x
Liu J., Zhou T., Li C., Li R., Ye X., Tian Z., et al. (2022). Reverse chemical ecology guides the screening for Grapholita molesta pheromone synergists. Pest Manag. Sci. 78 (2), 643–652. doi:10.1002/PS.6674
Liu S., Rao X. J., Li M. Y., Feng M. F., He M. Z., Li S. G., et al. (2015). Identification of candidate chemosensory genes in the antennal transcriptome of Tenebrio molitor (Coleoptera: Tenebrionidae). Comp. Biochem. Physiol. Part D. Genomics Proteomics 13, 44–51. doi:10.1016/j.cbd.2015.01.004
Lizana P., Machuca J., Larama G., Quiroz A., Mutis A., Venthur H. (2020). Mating-based regulation and ligand binding of an odorant-binding protein support the inverse sexual communication of the greater wax moth, Galleria mellonella (Lepidoptera: Pyralidae). Insect Mol. Biol. 29 (3), 337–351. doi:10.1111/imb.12638
Ma C., Zhao C., Cui S., Zhang Y., Chen G., Chen H., et al. (2019). Identification of candidate chemosensory genes of Ophraella communa LeSage (Coleoptera: Chrysomelidae) based on antennal transcriptome analysis. Sci. Rep. 9 (11), 15551. doi:10.1038/s41598-019-52149-x
Maher N., Thiéry D. (2006). Daphne gnidium, a possible native host plant of the European grapevine moth Lobesia botrana, stimulates its oviposition. Is a host shift relevant? CHEMOECOLOGY 16 (33), 135–144. doi:10.1007/S00049-006-0339-7
Mansurova M., Klusák V., Nešněrová P., Muck A., Doubský J., Svatoš A., et al. (2009). Design and synthesis of bombykol analogues for probing pheromone-binding protein-ligand interactions. Tetrahedron 65 (5), 1069–1076. doi:10.1016/j.tet.2008.10.106
Mazumder F., Khalequzzaman M. (2010). Eggplant shoot and fruit borer Leucinodes orbonalis guénee male moth catch in sex pheromone trap with special reference of lure elevation and IPM. J. Biosci. (Rajshari). 18 (1), 9–15. doi:10.3329/jbs.v18i0.8768
McCormack J., Hird S., Zellmer A., Carstens B., Brumfield R. (2013). Applications of next-generation sequencing to phylogeography and phylogenetics. Mol. Phylogenet. Evol. 66 (2), 526–538. doi:10.1016/J.YMPEV.2011.12.007
Md Meftaul I., Venkateswarlu K., Dharmarajan R., Annamalai P., Megharaj M. (2020). Pesticides in the urban environment: A potential threat that knocks at the door. Sci. Total Environ. 711, 134612. doi:10.1016/J.SCITOTENV.2019.134612
Ménard G., Rouillon A., Ghukasyan G., Emily M., Felden B., Donnio P.-Y., et al. (2021). Galleria mellonella larvae as an infection model to investigate sRNA-mediated pathogenesis in Staphylococcus aureus. Front. Cell. Infect. Microbiol. 0, 631710. doi:10.3389/FCIMB.2021.631710
Mita K., Kasahara M., Sasaki S., Nagayasu Y., Yamada T., Kanamori H., et al. (2004). The genome sequence of silkworm, Bombyx mori. DNA Res. 11 (1), 27–35. doi:10.1093/dnares/11.1.27
Mohammed S., Lamoree M., Ansa-Asare O. D., de Boer J. (2019). Review of the analysis of insecticide residues and their levels in different matrices in Ghana. Ecotoxicol. Environ. Saf. 171, 361–372. doi:10.1016/j.ecoenv.2018.12.049
Morrison W. R., Scully E. D., Campbell J. F. (2021). Towards developing areawide semiochemical-mediated, behaviorally-based integrated pest management programs for stored product insects. Pest Manag. Sci. 77, 2667–2682. doi:10.1002/ps.6289
Moschos T., Souliotis C., Broumas T., Kapothanassi V. (2004). Control of the European grapevine moth Lobesia botrana in Greece by the mating disruption technique: A three-year survey. Phytoparasitica 32132 (1), 83–96. doi:10.1007/BF02980864
Mylonakis E., Moreno R., El Khoury J. B., Idnurm A., Heitman J., Calderwood S. B., et al. (2005). Galleria mellonella as a model system to study Cryptococcus neoformans pathogenesis. Infect. Immun. 73 (7), 3842–3850. doi:10.1128/IAI.73.7.3842-3850.2005
Nadeau N. J., Jiggins C. D. (2010). A golden age for evolutionary genetics? Genomic studies of adaptation in natural populations. Trends Genet. 26 (11), 484–492. doi:10.1016/J.TIG.2010.08.004
Nesreen M., Abd El-Ghany N. (2019). Semiochemicals for controlling insect pests. J. Plant Prot. Res. 59, 1–11. doi:10.24425/jppr.2019.126036
Neuhaus E. M., Gisselmann G., Zhang W., Dooley R., Störtkuhl K., Hatt H., et al. (2004). Odorant receptor heterodimerization in the olfactory system of Drosophila melanogaster. Nat. Neurosci. 8 (11), 15–17. doi:10.1038/nn1371
Niu D. J., Liu Y., Dong X. T., Dong S. L. (2016). Transcriptome based identification and tissue expression profiles of chemosensory genes in Blattella germanica (Blattaria: Blattidae). Comp. Biochem. Physiol. Part D. Genomics Proteomics 18, 30–43. doi:10.1016/j.cbd.2016.03.002
Niu Y., Han S., Wu Z., Pan C., Wang M., Tang Y., et al. (2022). A push–pull strategy for controlling the tea green leafhopper (Empoasca flavescens F.) using semiochemicals from Tagetes erecta and Flemingia macrophylla. Pest Manag. Sci. 78, 2161–2172. doi:10.1002/PS.6840
Northey T., Venthur H., De Biasio F., Chauviac F.-X., Cole A., Ribeiro K. A. L., et al. (2016). Crystal structures and binding dynamics of odorant-binding protein 3 from two aphid species Megoura viciae and Nasonovia ribisnigri. Sci. Rep. 6 (11), 24739. doi:10.1038/srep24739
Oehlschlager A. C. (2016). Palm weevil pheromones – discovery and use. J. Chem. Ecol. 42 (7), 617–630. doi:10.1007/s10886-016-0720-0
Oerke E. C. (2006). Crop losses to pests. J. Agric. Sci.Issue 1, 144. Cambridge University Press, 31–43. doi:10.1017/S0021859605005708
Oppenheim S. J., Baker R. H., Simon S., Desalle R. (2015). We can’t all be supermodels: The value of comparative transcriptomics to the study of non-model insects. Insect Mol. Biol. 24 (2), 139–154. doi:10.1111/imb.12154
Ortiz A., Porras A., Marti J., Tudela A., Rodríguez-González A., Sambado P., et al. (2021). Mating disruption of the olive moth Prays oleae (Bernard) in olive groves using aerosol dispensers. Insects 12, 1113. doi:10.3390/insects12121113
Palma R. (2004). Relaciones entre densidades larvales de Hylamorpha elegans (Burmeister) (Coleoptera: Scarabaeidae), y el establecimiento y desarrollo de la especie pratense Lolium perenne L. Tesis de Licenciado en Agronomía. Facultad de Ciencias Agrarias, Universidad Austral de Chile.
Paula D. P., Togawa R. C., Costa M. M. C., Grynberg P., Martins N. F., Andow D. A., et al. (2016). Identification and expression profile of odorant-binding proteins in Halyomorpha halys (Hemiptera: Pentatomidae). Insect Mol. Biol. 25, 580–594. doi:10.1111/imb.12243
Peccoud J., Simon J.-C. (2010). The pea aphid complex as a model of ecological speciation. Ecol. Entomol. 35 (Suppl. 1), 119–130. doi:10.1111/J.1365-2311.2009.01147.X
Pelosi P., Calvello M., Ban L. (2005). Diversity of odorant-binding proteins and chemosensory proteins in insects. Chem. Senses 30 (Suppl. 11), 291–292. doi:10.1093/chemse/bjh229
Pelosi P., Iovinella I., Zhu J., Wang G., Dani F. R. (2018). Beyond chemoreception: Diverse tasks of soluble olfactory proteins in insects. Biol. Rev. Camb. Philos. Soc. 93 (1), 184–200. doi:10.1111/BRV.12339
Pelosi P., Maida R. (1990). Odorant-binding proteins in vertebrates and insects: Similarities and possible common function. Chem. Senses 15 (2), 205–215. doi:10.1093/CHEMSE/15.2.205
Peng R. K., Christian K., Doi Dx. (2007). Effective control of Jarvis’s fruit fly, Bactrocera jarvisi (Diptera: Tephritidae), by the weaver ant, Oecophylla smaragdina (Hymenoptera: Formicidae), in mango orchards in the Northern Territory of Australia. Int. J. Pest Manag. 52 (4), 275–282. doi:10.1080/09670870600795989
Peng X., Liu L., Huang Y.-X., Wang S.-J., Li D.-X., Chen S.-T., et al. (2020). Involvement of chemosensory proteins in host plant searching in the bird cherry-oat aphid. Insect Sci. 28, 1338–1353. doi:10.1111/1744-7917.12865
Peng Y., Wang S.-N., Li K.-M., Liu J.-T., Zheng Y., Shan S., et al. (2017). Identification of odorant binding proteins and chemosensory proteins in Microplitis mediator as well as functional characterization of chemosensory protein 3. PLOS ONE 12 (7), e0180775. doi:10.1371/JOURNAL.PONE.0180775
Peralta R. C., Hegazy M. A., Musharrafieh G. R. (1994). Preventing pesticide contamination of groundwater while maximizing irrigated crop yield. Water Resour. Res. 30 (Issue 11), 3183–3193. doi:10.1029/94wr01724
Picimbon J. F., Dietrich K., Krieger J., Breer H. (2001). Identity and expression pattern of chemosensory proteins in Heliothis virescens (Lepidoptera, Noctuidae). Insect biochem. Mol. Biol. 31 (12), 1173–1181. doi:10.1016/S0965-1748(01)00063-7
Poivet E., Gallot A., Montagné N., Glaser N., Legeai F., Jacquin-Joly E., et al. (2013). A comparison of the olfactory gene repertoires of adults and larvae in the noctuid moth Spodoptera littoralis. PLOS ONE 8 (4), e60263. doi:10.1371/JOURNAL.PONE.0060263
Pregitzer P., Jiang X., Grosse-Wilde E., Breer H., Krieger J., Fleischer J., et al. (2017). In search for pheromone receptors: Certain members of the odorant receptor family in the desert locust Schistocerca gregaria (orthoptera: Acrididae) are co-expressed with SNMP1. Int. J. Biol. Sci. 13, 911–922. doi:10.7150/ijbs.18402
Prestwich G. D. (1996). Proteins that smell: Pheromone recognition and signal transduction. Bioorg. Med. Chem. 4 (3), 505–513. doi:10.1016/0968-0896(96)00033-8
Qi M., Wei S., Wei C. (2018). Identification of candidate olfactory genes in cicada Subpsaltria yangi by antennal transcriptome analysis. Comp. Biochem. Physiol. Part D. Genomics Proteomics 28, 122–133. doi:10.1016/J.CBD.2018.08.001
Qian J.-L., Mang D.-Z., Lv G.-C., Ye J., Li Z.-Q., Chu B., et al. (2020). Identification and expression profile of olfactory receptor genes based on Apriona germari (hope) antennal transcriptome. Front. Physiol. 0, 807. doi:10.3389/FPHYS.2020.00807
Qiao H. L., Deng P. Y., Li D. D., Chen M., Jiao Z. J., Liu Z. C., et al. (2013). Expression analysis and binding experiments of chemosensory proteins indicate multiple roles in Bombyx mori. J. Insect Physiol. 59 (7), 667–675. doi:10.1016/J.JINSPHYS.2013.04.004
Qiu L., He L., Tan X., Zhang Z., Wang Y., Li X., et al. (2020). Identification and phylogenetics of Spodoptera frugiperda chemosensory proteins based on antennal transcriptome data. Comp. Biochem. Physiol. Part D. Genomics Proteomics 34, 100680. doi:10.1016/J.CBD.2020.100680
Qu M. Q., Cui Y., Zou Y., Wu Z. Z., Lin J. T. (2020). Identification and expression analysis of odorant binding proteins and chemosensory proteins from dissected antennae and mouthparts of the rice bug Leptocorisa acuta. Comp. Biochem. Physiol. Part D. Genomics Proteomics 33, 100631. doi:10.1016/J.CBD.2019.100631
Rasool K. G., Mehmood K., Husain M., Tufail M., Alwaneen W. S., Aldawood A. S., et al. (2021). De novo transcriptome analysis and identification of reproduction control genes from the red palm weevil Rhynchophorus ferrugineus. PLOS ONE 16 (5), e0251278. doi:10.1371/JOURNAL.PONE.0251278
Reddy G. V. P., Guerrero A. (2010). New pheromones and insect control strategies. Vitam. Horm. 83 (C), 493–519. doi:10.1016/S0083-6729(10)83020-1
Richards S., Gibbs R., Weinstock G., Brown S. J., Denell R., Beeman R. W., et al. (2008). The genome of the model beetle and pest Tribolium castaneum. Nature 452 (7190), 949–955. doi:10.1038/NATURE06784
Rinker D. C., Zhou X., Pitts R. J., Rokas A., Zwiebel L. J., Neafsey D. E., et al. (2013). Antennal transcriptome profiles of anopheline mosquitoes reveal human host olfactory specialization in Anopheles gambiae. BMC Genomics 14, 749. doi:10.1186/1471-2164-14-749
Rojas V., Jiménez H., Palma-Millanao R., González-González A., Machuca J., Godoy R., et al. (2018). Analysis of the grapevine moth Lobesia botrana antennal transcriptome and expression of odorant-binding and chemosensory proteins. Comp. Biochem. Physiol. Part D. Genomics Proteomics 27, 1–12. doi:10.1016/j.cbd.2018.04.003
Rondoni G., Roman A., Meslin C., Montagné N., Conti E., Jacquin-Joly E., et al. (2021). Antennal transcriptome analysis and identification of candidate chemosensory genes of the harlequin ladybird beetle, Harmonia axyridis (pallas) (Coleoptera: Coccinellidae). Insects 12, 209. doi:10.3390/insects12030209
Sakurai T., Nakagawa T., Mitsuno H., Mori H., Endo Y., Tanoue S., et al. (2004). Identification and functional characterization of a sex pheromone receptor in the silkmoth Bombyx mori. Proc. Natl. Acad. Sci. U. S. A. 101 (47), 16653–16658. doi:10.1073/PNAS.0407596101
Sandler B., Nikonova L., Leal W., Clardy J. (2000). Sexual attraction in the silkworm moth: Structure of the pheromone-binding-protein-bombykol complex. Chem. Biol. 7 (2), 143–151. doi:10.1016/S1074-5521(00)00078-8
Sasaki M., Sato R. (1995). Bionomics of the cherry drosophila, Drosophila suzukii matsumura (Diptera: Drosophilidae) in fukushima prefecture [Japan], 1: Drosophila injured on cherry [prunus avium] fruit.
Sato K., Pellegrino M., Nakagawa T., Nakagawa T., Vosshall L. B., Touhara K., et al. (2008). Insect olfactory receptors are heteromeric ligand-gated ion channels. Nature 452, 71904521002–71904521006. doi:10.1038/nature06850
Schoville S. D., Chen Y. H., andersson M. N., Benoit J. B., Bhandari A., Bowsher J. H., et al. (2018). A model species for agricultural pest genomics: The genome of the Colorado potato beetle, Leptinotarsa decemlineata (Coleoptera: Chrysomelidae). Sci. Rep. 8 (1), 1931. doi:10.1038/s41598-018-20154-1
Shen S., Cao S., Zhang Z., Kong X., Liu F., Wang G., et al. (2020). Evolution of sex pheromone receptors in Dendrolimus punctatus Walker (lepidoptera: Lasiocampidae) is divergent from other moth species. Insect biochem. Mol. Biol. 122, 103375. doi:10.1016/j.ibmb.2020.103375
Shiao M.-S., Fan W.-L., Fang S., Lu M.-Y. J., Kondo R., Li W.-H., et al. (2013). Transcriptional profiling of adult Drosophila antennae by high-throughput sequencing. Zool. Stud. (11), 421. doi:10.1186/1810-522X-52-42
Skendžić S., Zovko M., Živković I. P., Lešić V., Lemić D. (2021). The impact of climate change on agricultural insect pests. Insects 12 (5), 440. Page 440. doi:10.3390/INSECTS12050440
Slone J., Daniels J., Amrein H. (2007). Sugar receptors in Drosophila. Curr. Biol. 17 (20), 1809–1816. doi:10.1016/J.CUB.2007.09.027
Smart L. E., Aradottir G. I., Bruce T. J. A. (2014). “Role of semiochemicals in integrated pest management,” in Integrated pest management: Current concepts and ecological perspective (Elsevier), 93–109. doi:10.1016/B978-0-12-398529-3.00007-5
Song Y.-Q., Sun H.-Z., Du J. (2018). Identification and tissue distribution of chemosensory protein and odorant binding protein genes in Tropidothorax elegans Distant (Hemiptera: Lygaeidae). Sci. Rep. 8, 7803. doi:10.1038/s41598-018-26137-6
Song Y., Song Z., Gu H., Sun H., Zhao J. (2021). Identification and expression analysis of odorant-binding proteins and chemosensory proteins in the antennal transcriptome of Yemma signatus (Hsiao, 1974). Phytoparasitica 49, 917–933. doi:10.1007/S12600-021-00917-9
Soroker V., Harari A., Faleiro J. R. (2015). “The role of semiochemicals in date pest management,” in Sustainable pest management in date palm: Current status and emerging challenges (Springer International Publishing), 315–346. doi:10.1007/978-3-319-24397-9_11
Stapley J., Reger J., Feulner P. G. D., Smadja C., Galindo J., Ekblom R., et al. (2010). Adaptation genomics: The next generation. Trends Ecol. Evol. 25 (12), 705–712. doi:10.1016/J.TREE.2010.09.002
Storer N. P., Babcock J. M., Schlenz M., Meade T., Thompson G. D., Bing J. W., et al. (2010). Discovery and characterization of field resistance to bt maize: Spodoptera frugiperda (Lepidoptera: Noctuidae) in Puerto Rico. J. Econ. Entomol. 103 (4), 1031–1038. doi:10.1603/EC10040
Storer N. P., Kubiszak M. E., King J., Thompson G. D., Santos A. C. (2012). Status of resistance to bt maize in Spodoptera frugiperda: Lessons from Puerto Rico. J. Invertebr. Pathol. 110 (3), 294–300. doi:10.1016/J.JIP.2012.04.007
Stork N. E. (2018). How many species of insects and other terrestrial arthropods are there on earth? Annu. Rev. Entomol. 63 (1), 31–45. doi:10.1146/annurev-ento-020117-043348
Stratonovitch P., Elias J., Denholm I., Slater R., Semenov M. A., Guedes R. N. C. (2014). An individual-based model of the evolution of pesticide resistance in heterogeneous environments: Control of meligethes aeneus population in oilseed rape Crops. PLoS ONE 9 (12), e115631. doi:10.1371/journal.pone.0115631
Tang Q.-F., Shen C., Zhang Y., Yang Z.-P., Han R.-R., Wang J., et al. (2019). Antennal transcriptome analysis of the maize weevil Sitophilus zeamais: Identification and tissue expression profiling of candidate odorant-binding protein genes. Arch. Insect Biochem. Physiol. 101 (1), e21542. doi:10.1002/ARCH.21542
Tangkawanit U., Seehavet S., Siri N. (2021). The potential of Labidura riparia and Euborellia annulipes (Dermaptera) as predators of house fly in livestock. Songklanakarin J. Sci. Technol. 43 (3), 603–607.
Tasin M., Bäckman A. C., Anfora G., Carlin S., Ioriatti C., Witzgall P., et al. (2010). Attraction of female grapevine moth to common and specific olfactory cues from 2 host plants. Chem. Senses 35 (1), 57–64. doi:10.1093/CHEMSE/BJP082
Tian Z., Sun L., Li Y., Quan L., Zhang H., Yan W., et al. (2018). Antennal transcriptome analysis of the chemosensory gene families in Carposina sasakii (Lepidoptera: Carposinidae). BMC Genomics 19 (1), 5441. doi:10.1186/S12864-018-4900-X
Tilman D., Balzer C., Hill J., Befort B. L. (2011). Global food demand and the sustainable intensification of agriculture. Proc. Natl. Acad. Sci. U. S. A. 108 (50), 20260–20264. doi:10.1073/pnas.1116437108
Tran H. (2020). Identification of odorant receptor genes in the antennal transcriptome of the common eastern fireflies, Honors Theses. Photinus pyralis. Available at: https://digitalcommons.bucknell.edu/honors_theses/532.
Vellichirammal N. N., Madayiputhiya N., Brisson J. A. (2016). The genomewide transcriptional response underlying the pea aphid wing polyphenism. Mol. Ecol. 25 (17), 4146–4160. doi:10.1111/mec.13749
Venthur H., Mutis A., Zhou J. J., Quiroz A. (2014). Ligand binding and homology modelling of insect odorant-binding proteins. Physiol. Entomol.Issue 3, 39. Saint Albans: Blackwell Publishing Ltd, 183–198. doi:10.1111/phen.12066
Venthur H., Zhou J. J. (2018). Odorant receptors and odorant-binding proteins as insect pest control targets: A comparative analysisFront. PhysiologyIssue AUG, 9. Lausanne: Frontiers Media S.A, 1163. doi:10.3389/fphys.2018.01163
Verheggen F. J., Fagel Q., Heuskin S., Lognay G., Francis F., Haubruge E., et al. (2007). Electrophysiological and behavioral responses of the multicolored asian lady beetle, Harmonia axyridis pallas, to sesquiterpene semiochemicals. J. Chem. Ecol. 3333 (11), 112148–112155. doi:10.1007/S10886-007-9370-6
Vieira F. G., Rozas J. (2011). Comparative genomics of the odorant-binding and chemosensory protein gene families across the arthropoda: Origin and evolutionary history of the chemosensory system. Genome Biol. Evol. 3 (1), 476–490. doi:10.1093/GBE/EVR033
Vogt R. G., Prestwich G. D., Lerner M. R. (1991). Odorant-binding-protein subfamilies associate with distinct classes of olfactory receptor neurons in insects. J. Neurobiol. 22 (1), 74–84. doi:10.1002/NEU.480220108
Vogt R. G., Riddiford L. M. (1981). Pheromone binding and inactivation by moth antennae. Nature 293293 (5828), 5828161–5828163. doi:10.1038/293161a0
Vosshall L. B., Amrein H., Morozov P. S., Rzhetsky A., Axel R. (1999). A spatial map of olfactory receptor expression in the Drosophila antenna. Cell. 96 (5), 725–736. doi:10.1016/S0092-8674(00)80582-6
Vosshall L. B., Hansson B. S. (2011). A unified nomenclature system for the insect olfactory coreceptor. Chem. Senses 36 (Issue 6), 497–498. Oxford University Press. doi:10.1093/chemse/bjr022
Vosshall L. B., Wong A. M., Axel R. (2000). An olfactory sensory map in the fly brain. Cell. 102 (2), 147–159. doi:10.1016/S0092-8674(00)00021-0
Wachi N., Matsubayashi K. W., Maeto K. (2018). Application of next-generation sequencing to the study of non-model insects. Entomological Sci. 21 (1), 3–11. doi:10.1111/ENS.12281
Walker W. B., Roy A., anderson P., Schlyter F., Hansson B. S., Larsson M. C., et al. (20192019). Transcriptome analysis of gene families involved in chemosensory function in Spodoptera littoralis (Lepidoptera: Noctuidae). BMC Genomics 20 (11), 428. doi:10.1186/S12864-019-5815-X
Wang B., Guan L., Zhong T., Li K., Yin J., Cao Y., et al. (2013). Potential cooperations between odorant-binding proteins of the scarab beetle Holotrichia oblita Faldermann (Coleoptera: Scarabaeidae). PLoS ONE 8 (12), 84795. doi:10.1371/journal.pone.0084795
Wang R., Li F., Zhang W., Zhang X., Qu C., Tetreau G., et al. (2017). Identification and expression profile analysis of odorant binding protein and chemosensory protein genes in Bemisia tabaci MED by head transcriptome. PLoS One 12, e0171739. doi:10.1371/journal.pone.0171739
Wang S., Minter M., Homem R. A., Michaelson L. V., Venthur H., Lim K. S., et al. (2020). Odorant binding proteins promote flight activity in the migratory insect, Helicoverpa armigera. Mol. Ecol. 29 (19), 3795–3808. doi:10.1111/MEC.15556
Wang Y., Zhang H., Li H., Miao X. (2011). Second-generation sequencing supply an effective way to screen RNAi targets in large scale for potential application in pest insect control. PLoS ONE 6 (4), 18644. doi:10.1371/journal.pone.0018644
Wanner K. W., Isman M. B., Feng Q., Plettner E., Theilmann D. A. (2005). Developmental expression patterns of four chemosensory protein genes from the Eastern spruce budworm, Chroistoneura fumiferana. Insect Mol. Biol. 14 (3), 289–300. doi:10.1111/j.1365-2583.2005.00559.x
Wanner K. W., Robertson H. M. (2008). The gustatory receptor family in the silkworm moth Bombyx mori is characterized by a large expansion of a single lineage of putative bitter receptors. Insect Mol. Biol. 17 (6), 621–629. doi:10.1111/J.1365-2583.2008.00836.X
Weinstock G. M., Robinson G. E., Gibbs R. A., Worley K. C., Evans J. D., Maleszka R., et al. (2006). Insights into social insects from the genome of the honeybee Apis mellifera. Nature 443 (7114), 931–949. doi:10.1038/nature05260
Wen X., Wang Q., Gao P., Wen J. (2018). Identification and comparison of chemosensory genes in the antennal transcriptomes of Eucryptorrhynchus scrobiculatus and E. brandti fed on ailanthus altissima. Front. Physiol. 0 (NOV), 1652. doi:10.3389/FPHYS.2018.01652
Wicher D., Marion-Poll F. (2018). Editorial: Function and regulation of chemoreceptors. Front. Cell. Neurosci. 12, 496. doi:10.3389/FNCEL.2018.00496
Wicher D., Miazzi F. (2021). Functional properties of insect olfactory receptors: Ionotropic receptors and odorant receptors. Cell. Tissue Res. 383 (11), 7–19. doi:10.1007/S00441-020-03363-X
Wicher D., Schäfer R., Bauernfeind R., Stensmyr M. C., Heller R., Heinemann S. H., et al. (2008). Drosophila odorant receptors are both ligand-gated and cyclic-nucleotide-activated cation channels. Nature 452, 71904521007–71904521011. doi:10.1038/nature06861
Wojtasek H., Leal W. S. (1999). Conformational change in the pheromone-binding protein from Bombyx mori induced by pH and by interaction with membranes. J. Biol. Chem. 274 (43), 30950–30956. doi:10.1074/JBC.274.43.30950
Wu S., Deng W., Li M., Xiao Y., Li J., Teng K., et al. (2020). Analysis of chemosensory genes in full and hungry adults of Arma chinensis (pentatomidae) through antennal transcriptome. Front. Physiol. 11, 588291. doi:10.3389/FPHYS.2020.588291
Wu Z. Z., Cui Y., Qu M. Q., Lin J. H., Chen M. S., Bin S. Y., et al. (2019). Candidate genes coding for odorant binding proteins and chemosensory proteins identified from dissected antennae and mouthparts of the southern green stink bug Nezara viridula. Comp. Biochem. Physiol. Part D. Genomics Proteomics 31, 100594. doi:10.1016/J.CBD.2019.100594
Xiao Y., Sun L., Ma X.-Y., Dong K., Liu H.-W., Wang Q., et al. (2017). Identification and characterization of the distinct expression profiles of candidate chemosensory membrane proteins in the antennal transcriptome of Adelphocoris lineolatus (Goeze). Insect Mol. Biol. 26 (1), 74–91. doi:10.1111/IMB.12272
Xing Y., Thanasirungkul W., Adeel M. M., Yu J., Aslam A., Chi D. F., et al. (2021). Identification and analysis of olfactory genes in Dioryctria abietella based on the antennal transcriptome. Comp. Biochem. Physiol. Part D. Genomics Proteomics 38, 100814. doi:10.1016/J.CBD.2021.100814
Xu J.-W., Zhu X.-Y., Chao Q.-J., Zhang Y.-J., Yang Y.-X., Wang R.-R., et al. (2019). Chemosensory gene families in the oligophagous pear pest Cacopsylla chinensis (Hemiptera: Psyllidae). Insects 10 (6), 175. Page 175. doi:10.3390/INSECTS10060175
Xu Q., Wu Z., Zeng X., An X. (2020). Identification and expression profiling of chemosensory genes in Hermetia illucens via a transcriptomic analysis. Front. Physiol. 0, 720. doi:10.3389/FPHYS.2020.00720
Xue W., Fan J., Zhang Y., Xu Q., Han Z., Sun J., et al. (2016). Identification and expression analysis of candidate odorant-binding protein and chemosensory protein genes by antennal transcriptome of sitobion avenae. PLoS One 11, e0161839. doi:10.1371/journal.pone.0161839
Yan C., Sun X., Cao W., Li R., Zhao C., Sun Z., et al. (2020). Identification and expression pattern of chemosensory genes in the transcriptome of Propsilocerus akamusi. PeerJ 8, e9584. doi:10.7717/PEERJ.9584
Yang F., Kerns D. L., Brown S., Kurtz R., Dennehy T., Braxton B., et al. (2016). Performance and cross-crop resistance of Cry1F-maize selected Spodoptera frugiperda on transgenic bt cotton: Implications for resistance management. Sci. Rep. 6 (11), 28059. doi:10.1038/srep28059
Yang J., Guo H., Id N. J., Id R. T., Id G. L. (2021). Identification of a gustatory receptor tuned to sinigrin in the cabbage butterfly Pieris rapae, 1–28. doi:10.1371/journal.pgen.1009527
Yang X., Zhang M., Jiang Y., Tang Q., Zhang Q., Lin L., et al. (2020). Analysis of the antennal transcriptome and olfaction-related genes of Coccinella septempunctata (Coleoptera: Coccinellidae). Acta Entomologica Sinica. Available at: https://www.cabdirect.org/cabdirect/abstract/20203465943.
Yang Y., Li W., Tao J., Zong S. (2019). Antennal transcriptome analyses and olfactory protein identification in an important wood-boring moth pest, Streltzoviella insularis (Lepidoptera: Cossidae). Sci. Rep. 9 (11), 17951. doi:10.1038/s41598-019-54455-w
Yi J. K., Yang S., Wang S., Wang J., Zhang X. X., Liu Y., et al. (2018). Identification of candidate chemosensory receptors in the antennal transcriptome of the large black chafer Holotrichia parallela Motschulsky (Coleoptera: Scarabaeidae). Comp. Biochem. Physiol. Part D. Genomics Proteomics 28, 63–71. doi:10.1016/J.CBD.2018.06.005
Yin X. W., Iovinella I., Marangoni R., Cattonaro F., Flamini G., Sagona S., et al. (2013). Odorant-binding proteins and olfactory coding in the solitary bee Osmia cornuta. Cell. Mol. Life Sci. 70, 3029–3039. doi:10.1007/s00018-013-1308-2
Yuan H., Chang H., Zhao L., Yang C., Huang Y. (20192019). Sex- and tissue-specific transcriptome analyses and expression profiling of olfactory-related genes in Ceracris nigricornis Walker (Orthoptera: Acrididae). BMC Genomics 120 (1), 808. doi:10.1186/S12864-019-6208-X
Yuvaraj J. K., Corcoran J. A., andersson M. N., Newcomb R. D., anderbrant O., Löfstedt C., et al. (2017). Characterization of odorant receptors from a non-ditrysian moth, Eriocrania semipurpurella sheds light on the origin of sex pheromone receptors in lepidoptera. Mol. Biol. Evol. 34 (11), 2733–2746. doi:10.1093/molbev/msx215
Yuvaraj J. K., Roberts R. E., Sonntag Y., Hou X.-Q., Grosse-Wilde E., Machara A., et al. (2021). Putative ligand binding sites of two functionally characterized bark beetle odorant receptors. BMC Biol. 19 (11), 16. doi:10.1186/S12915-020-00946-6
Zeng F. F., Liu H., Zhang A., Lu Z. X., Leal W. S., Abdelnabby H., et al. (2018). Three chemosensory proteins from the rice leaf folder Cnaphalocrocis medinalis involved in host volatile and sex pheromone reception. Insect Mol. Biol. 27 (6), 710–723. doi:10.1111/IMB.12503
Zhan H., Li D., Dewer Y., Niu C., Li F., Luo C., et al. (2021). Identification and functional characterization of odorant-binding proteins 69a and 76a of Drosophila suzukii. Heliyon 7 (3), e06427. doi:10.1016/J.HELIYON.2021.E06427
Zhang H. J., Xu W., Chen Q. M., Sun L. N., anderson A., Xia Q. Y., et al. (2020). A phylogenomics approach to characterizing sensory neuron membrane proteins (SNMPs) in Lepidoptera. Insect biochem. Mol. Biol. 118, 103313. doi:10.1016/J.IBMB.2020.103313
Zhang J., Wang B., Dong S., Cao D., Dong J., Walker W. B., et al. (2015a). Antennal transcriptome analysis and comparison of chemosensory gene families in two closely related noctuidae moths, Helicoverpa armigera and H. assulta. PLoS One 10, e0117054. doi:10.1371/journal.pone.0117054
Zhang L. W., Kang K., Jiang S. C., Zhang Y. N., Wang T. T., Zhang J., et al. (2016). Analysis of the antennal transcriptome and insights into olfactory genes in Hyphantria Cunea (Drury). PLoS One 11, e0164729. doi:10.1371/journal.pone.0164729
Zhang R., Wang B., Grossi G., Falabella P., Liu Y., Yan S., et al. (2017). Molecular basis of alarm pheromone detection in aphids. Curr. Biol. 27 (1), 55–61. doi:10.1016/J.CUB.2016.10.013
Zhang S., Zhang Z., Wang H., Kong X. (2014). Antennal transcriptome analysis and comparison of olfactory genes in two sympatric defoliators, Dendrolimus houi and Dendrolimus kikuchii (lepidoptera: Lasiocampidae). Insect biochem. Mol. Biol. 52, 69–81. doi:10.1016/j.ibmb.2014.06.006
Zhang T., Coates B. S., Ge X., Bai S., He K., Wang Z., et al. (2015b). Male- and female-biased gene expression of olfactory-related genes in the antennae of Asian corn borer, Ostrinia furnacalis (Guenée) (Lepidoptera: Crambidae). PLoS One 10, e0128550. doi:10.1371/journal.pone.0128550
Zhang X., Yang S., Zhang J., Wang X., Wang S., Liu M., et al. (2019). Identification and expression analysis of candidate chemosensory receptors based on the antennal transcriptome of Lissorhoptrus oryzophilus. Comp. Biochem. Physiol. Part D. Genomics Proteomics 30, 133–142. doi:10.1016/J.CBD.2019.02.007
Zhao H. X., Xiao W. Y., Ji C. H., Ren Q., Xia X. S., Zhang X. F., et al. (2019). Candidate chemosensory genes identified from the greater wax moth, Galleria mellonella, through a transcriptomic analysis. Sci. Rep. 9 (1), 10032. doi:10.1038/s41598-019-46532-x
Zhao Y., Ding J., Zhang Z., Liu F., Zhou C., Mu W., et al. (2018). Sex- and tissue-specific expression profiles of odorant binding protein and chemosensory protein genes in Bradysia odoriphaga (Diptera: Sciaridae). Front. Physiol. 9, 107. doi:10.3389/fphys.2018.00107
Zhao Y., Wang F., Zhang X., Zhang S., Guo S., Zhu G., et al. (2016). Transcriptome and expression patterns of chemosensory genes in antennae of the parasitoid wasp Chouioia cunea. PLoS One 11, e0148159. doi:10.1371/journal.pone.0148159
Zhou J. J. (2010). “Odorant-binding proteins in insects,” in Vitamins and hormones (Academic Press), 83. Issue C. doi:10.1016/S0083-6729(10)83010-9
Zhou ., Li L., Pang B., Shan Y., Zhang Z. (20192019). Antennal transcriptome analysis and expression profiling of chemosensory protein genes in Oedaleus asiaticus (Orthopera: Acrididae). Acta Entomol. Sin. 62 (1), 21–32.
Zhou S. S., Sun Z., Ma W., Chen W., Wang M. Q. (2014). De novo analysis of the Nilaparvata lugens (Stål) antenna transcriptome and expression patterns of olfactory genes. Comp. Biochem. Physiol. Part D. Genomics Proteomics 9, 31–39. doi:10.1016/j.cbd.2013.12.002
Zhou X.-H., Ban L.-P., Iovinella I., Zhao L.-J., Gao Q., Felicioli A., et al. (2013). Diversity, abundance, and sex-specific expression of chemosensory proteins in the reproductive organs of the locust Locusta migratoria manilensis. Biol. Chem. 394 (1), 43–54. doi:10.1515/HSZ-2012-0114
Keywords: pest control, sustainability, insect olfaction, model insects, non-model insects, chemosensory proteins
Citation: Lizana P, Mutis A, Quiroz A and Venthur H (2022) Insights Into Chemosensory Proteins From Non-Model Insects: Advances and Perspectives in the Context of Pest Management. Front. Physiol. 13:924750. doi: 10.3389/fphys.2022.924750
Received: 20 April 2022; Accepted: 13 June 2022;
Published: 22 August 2022.
Edited by:
Robert Huber, Bowling Green State University, United StatesReviewed by:
Youssef Dewer, Agricultural Research Center, EgyptSufang Zhang, Chinese Academy of Forestry, China
Copyright © 2022 Lizana, Mutis, Quiroz and Venthur. This is an open-access article distributed under the terms of the Creative Commons Attribution License (CC BY). The use, distribution or reproduction in other forums is permitted, provided the original author(s) and the copyright owner(s) are credited and that the original publication in this journal is cited, in accordance with accepted academic practice. No use, distribution or reproduction is permitted which does not comply with these terms.
*Correspondence: Herbert Venthur, aGVyYmVydC52ZW50aHVyQHVmcm9udGVyYS5jbA==