- 1Department of Animal Science, College of Coastal Agricultural Sciences, Guangdong Ocean University, Zhanjiang, China
- 2Department of Food Science and Biotechnology, College of Life Science, Sejong University, Seoul, South Korea
- 3Department of Human Nutrition, Food and Animal Sciences, College of Tropical Agriculture and Human Resources, University of Hawaii at Manoa, Honolulu, HI, United States
In the perspective of the global climate change leading to increasing temperature, heat stress (HS) has become a severe issue in broiler production, including the indigenous yellow-feathered broilers. The present study aimed to investigate the effects of HS on jejunal immune response, microbiota structure and their correlation in yellow-feathered broilers. A total of forty female broilers (56-days-old) were randomly and equally divided into normal treatment group (NT group, 21.3 ± 1.2°C, 24 h/day) and HS group (32.5 ± 1.4°C, 8 h/day) with five replicates of each for 4 weeks feeding trial. The results showed that HS exposure increased the contents of TNF-α, IL-1β, and IL-6 in jejunal mucosa (p < 0.05). The HS exposure up-regulated the relative fold changes of NF-κB, TNF-α, IL-1β, and IL-6 (p < 0.01) while down-regulated the relative fold change of IFN-γ in jejunal mucosa (p < 0.05). Meanwhile, HS had no significant impacts on alpha diversity of jejunal microbiota such as Simpson, Chao1 richness estimator (Chao 1), abundance-based coverage estimators (ACE), and Shannon index (p > 0.10). Broilers exposed to HS reduced the jejunal microbial species number at the class and order level (p < 0.05). Moreover, HS decreased the relative abundance of Ruminococcus, Bdellovibrio, and Serratia at the genus level in jejunum (p < 0.05). At the phylum level, four species of bacteria (Bacteroidetes, Cyanobacteria, Thermi, and TM7) were significantly associated with immune-related genes expression (p < 0.05). At the genus level, ten species of bacteria were significantly correlated with the expression of immune-related genes (p < 0.05), including Caulobacteraceae, Actinomyces, Ruminococcaceae, Thermus, Bdellovibrio, Clostridiales, Sediminibacterium, Bacteroides, Sphingomonadales and Ruminococcus. In particular, the microbial with significantly different abundances, Ruminococcus and Bdellovibrio, were negatively associated with pro-inflammatory cytokines expression (p < 0.05). These findings demonstrated that HS exposure promoted the production of pro-inflammatory cytokines in yellow-feathered broilers’ jejunum. The detrimental effects of HS on jejunal immune response might be related to dysbiosis, especially the reduced levels of Ruminococcus and Bdellovibrio.
Introduction
There is an increasing pursuit of flavor in the huge chicken consumer market in China and almost half of Chinese chicken meat is from locally yellow-feathered broilers because of their good meat quality (Gou et al., 2016). South China is the major production area for yellow-feathered broilers (Wang Y. et al., 2021). However, South China is in tropical and subtropical regions, and heat stress (HS) has become a great challenge for yellow-feathered broiler production (Liu et al., 2019). The HS exposure results in detrimental impacts on growth performance and has been well characterized, such as reduced feed intake, poor feed efficiency and decreased growth rate (Chauhan et al., 2021). Along with reduced growth rate, HS induces multiple deleterious consequences on physiological homeostasis, systemic immune function, metabolism status, and gut health (Guo et al., 2021a; Guo et al., 2021b; Liu et al., 2021a; Wasti et al., 2021a). Hence, HS has been one of the most harmful environmental stressors in broiler production, including the indigenous broilers, which causes large economic losses annually (Lara and Rostagno, 2013; Liu et al., 2019).
The gastrointestinal tract (GIT) is particularly vulnerable to HS, because the vasoconstriction in broiler’s GIT is increased to redistribute blood to the peripheral circulation, to maximize heat dissipation under HS situation (Vandana et al., 2021). Thus, HS leads to ischemia-hypoxic injury of the intestinal epithelial barrier and compromises gut integrity (Quinteiro-Filho et al., 2010). Intestinal mucosal immunity has attracted extensive attention for its effectiveness in strengthening the gut barrier function and protecting against pathogenic infection (Kogut and Arsenault, 2016). It has been found that HS-induced gut damage is partly attributed to the inflammatory response, such as an increase of pro-inflammatory cytokines production in broiler’s intestines (Song et al., 2017; Wu et al., 2018). On the other hand, the gut microbiota has a variety of biological functions, which is not only involved in the digestion of nutrients but also plays a vital role in maintaining intestinal barrier integrity and modulating the gut immune function of broilers (Yadav and Jha, 2019). With the development of high-throughput sequencing technology, emerging evidence demonstrated that HS disrupted the community structure and composition of hindgut (ileal and cecal) microbiota in broilers (Wang et al., 2018; Shi et al., 2019; Wang G. et al., 2021); but limited information is available about the effects of HS on fore-midgut microbiota in broilers. As the middle segment of the small intestine, the immune and microbial barrier of jejunum is critical for gut health in broilers (Rajput et al., 2017; Li et al., 2019). Furthermore, compared to the fast-growing chickens, including Ross and Cobb broilers, although the yellow-feathered broilers have better heat tolerance due to their slower growth and lower metabolic rate; it has been confirmed that HS also has negative impacts on gut health in this type of broilers (Gou et al., 2016; Liu et al., 2021a). However, there is a lack of information on changes in jejunal immunity, and the microbiome of yellow-feather broilers under HS. In this context, the present study was done to evaluate the effects of HS on jejunal immune response, microbial community, and their correlation in yellow-feathered broilers. Thus, it can provide novel insight into the interaction between the jejunal microbiome and immune response in slow-growing broilers under HS.
Materials and Methods
Animals, Experimental Design, and Management
A total of forty female Huaixiang chickens (eight-week-old, slow-growing type Chinese yellow-feathered broilers) were used in a 4 weeks HS trial (9–12 weeks of age during the study). The birds with an initial average body weight (BW) of 840.75 ± 20.79 g were sourced from a local supplier (Zhanjiang, Guangdong, China). The age selection of broilers was made considering the indigenous broilers entering the growing-finishing stage after 8-weeks of age, and growing-finishing broilers are more prone to HS for production (Wasti et al., 2020). The female broilers were only used to exclude the influence of gender on the study. It was also because the local people prefer to consume female yellow-feather broilers (Lin, 2008). Chickens were randomly and equally divided into two treatments in a completely randomized design. The treatments included the normal treatment group (NT) (21.3 ± 1.2°C throughout the experimental period, thermoneutral zone) and HS group (32.5 ± 1.4°C, 8 h/day, from 9:00 am to 17:00 pm). The ambient temperature for the rest of the time of the HS group was the same as the NT group. The relative humidity of NT and HS were maintained at 55–70%. Each group had five replications with four broilers per replicate, and one cage was used as one replicate. The chickens were kept in three-layer wire cages (one cage on top, middle, and bottom, with 20 cm gap between each layer for collecting and cleaning excreta). The size of each cage was 90 (length) × 70 (width) × 40 (height) cm. Plastic trays were used under each cage to collect the excreta, and the excreta were manually cleaned twice a day. The birds were ensured that all chickens had free access to water and feed. All broilers were fed corn-soybean meal basal diet to meet the nutrient requirements recommended by the Chinese chicken breeding standard (NY/T33-2004). The environmental control equipment, diet’s composition, and nutrient levels were as reported in our previous study (Guo et al., 2021a).
Sample Collection
At the end of the HS trial, one chicken was randomly selected from each replicate and sacrificed by neck bloodletting (n = 5/group). The jejunum was rapidly separated, and then approximately 2 g of jejunal digesta samples were collected and preserved in the liquid nitrogen and subsequently used for 16S rRNA sequencing. Afterward, the jejunum was opened, and the digesta was washed with PBS at 4°C; the jejunal mucosa was scraped off by glass slides and frozen in liquid nitrogen and then stored at −80°C for further detection of cytokines content and immune-related gene expression.
Determination of Jejunal Cytokines Concentration
The tumor necrosis factor-α (TNF-α), interferon-γ (IFN-γ), interleukin (IL)-1β, IL-2, IL-4, IL-6, and IL-10 concentrations in jejunal mucosa samples, were analyzed using commercial cytokines ELISA kits of chickens (Catalog numbers: TNF-α, MM-0938O2; IFN-γ, MM-0520O1; IL-1β, MM-36910O2; IL-2, MM-0528O2; IL-4, MM-0527O2; IL-6, MM-0521O2; IL-10 MM-1145O2; Jiangsu Enzyme Immunology Co., Ltd., Suzhou, China) following the manufacturer’s instructions.
Detection of Jejunal Immune-Related Genes Expression
Total RNA was extracted from the jejunal mucosa samples using RNA extraction kits (Catalog No. N066, Jiancheng Bioengineering Institute, Nanjing, China). The reverse transcription of cDNA from RNA using RT reagent kits (Catalog No. RR047A, TaKaRa Biotechnology Co., Ltd, Dalian, China). Then the quantitative real-time PCR (qPCR) was performed to detect the mRNA expression of immune-related genes, including NF-κB, TNF-α, IFN-γ, IL-1β, IL-2, IL-4, IL-6, and IL-10. The CFX-96 real-time PCR detection system (BioRad, Irvine, CA, United States) was used for the qPCR reaction. The specific primers, reaction system, and conditions were similar to our previous study (Liu et al., 2021b). β-actin was used as an internal reference gene. The mRNA expression levels of the jejunal immune-related genes were calculated using the 2−ΔΔCt method (Livak and Schmittgen, 2001), and the data were expressed as a relative fold change to the average value of the NT group.
Jejunal Microbiome Analysis
The total microbial DNA of jejunal digesta was extracted using QIAamp DNA Stool Mini Kit (Code No. 51306, QIAGEN, CA, Hamburg, Germany). This study used the Illumina Miseq Platform of Personalbio Technology Co., Ltd. (Shanghai, China) to perform paired-end sequencing of V3-V4 region of 16S rRNA gene. The primer pairs were 338F (5’-ACTCCTACGGGAGGCACAG-3’) and 806R (5’- GGACTACHVGGGTWTCTAAT-3’). The processing and analysis of sequencing data was performed using QIIME2 (Quantitative Insights Into Microbial Ecology 2) 2019.4 and according to the official tutorials (https://docs.qiime2.org/2019.4/tutorials/). Briefly, using cutadapt (v2.3) to excise the primer fragments of the raw sequencing reads and to remove the unmatched sequences with the primers. The fastq_mergepairs module of Vsearch (v2.13.4) was used to assemble the sequences; using the fastq_filter module of Vsearch (v2.13.4) to perform quality control on the assembled sequences; the derep_fulllength module of Vsearch (v2.13.4) was used to remove the duplicates sequences, and the uchime_denovo module of Vsearch (v2.13.4) was conducted to remove the chimeras, thereby resulting in high-quality sequences. Subsequently, the high-quality sequences are clustered at the 97% similarity level using the cluster_size module of Vsearch (v2.13.4), and the operational taxonomic units (OTUs) were obtained accordingly. The bacterial taxonomic information corresponding to each OTU was obtained based on the Greengenes database (Release 13.8, http://greengenes.secondgenome.com/).
Statistical Analysis
The data on jejunal cytokines levels, immune-related genes expression, and the difference in the jejunal microbiota were analyzed using SAS 9.4 (SAS Institute Inc., Cary, NC). Student’s t-test was performed to compare the differences between the two groups. Results are expressed as mean ± standard error. The alpha diversity, beta diversity, and the composition of jejunal microbiota were analyzed using Gene Cloud Analysis Platform (based on the kernel of QIIME 2, https://www.genescloud.cn) of Personalbio Technology Co., Ltd. (Shanghai, China). The Spearman correlation analysis was performed to determine the association between the jejunal microbiota (at the phylum and genus level) and immune-related genes expression using online software (based on the kernel of QIIME 2, https://www.genescloud.cn) of Personalbio Technology Co., Ltd. (Shanghai, China). The probability (P) < 0.05 was considered to be significant, and 0.05 ≤ p < 0.10 was considered to be a trend of significance.
Results
Jejunal Cytokines Content
The jejunal cytokines concentration treatment groups are presented in Table 1. Compared to NT group, broilers in HS group had higher levels of TNF-α, IL-1β, and IL-6 in jejunal mucosa (p < 0.05). There were no significant differences between NT and HS groups in jejunal INF-γ, IL-2, IL-4, and IL-10 contents (p > 0.10).
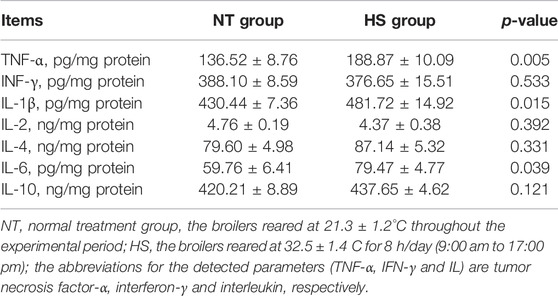
TABLE 1. Effects of heat stress on cytokines levels in jejunal mucosa of yellow-feathered broilers (n = 5).
Jejunal Immune-Related Genes Expression
As illustrated in Figure 1, broilers exposed to HS up-regulated the relative fold changes of NF-κB, TNF-α, IL-1β, and IL-6 (p < 0.01) while down-regulated the relative fold changes of IFN-γ in jejunal mucosa (p < 0.05). In addition, the HS exposure had no significant impact on jejunal relative fold changes of IL-2, IL-4, and IL-10 (p > 0.10).
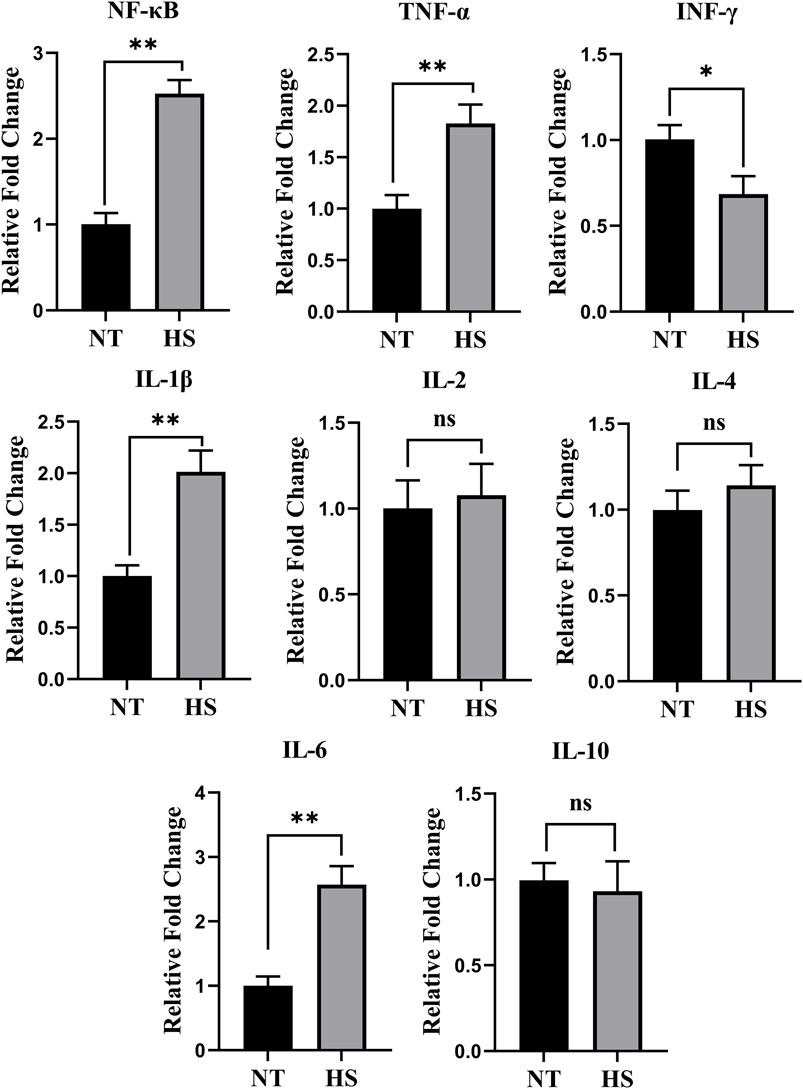
FIGURE 1. Effects of heat stress on relative fold change of jejunal immune-related genes in yellow-feathered broilers (n = 5). NT, normal treatment group (21.3 ± 1.2°C, 24 h/day); HS, heat stress group (32.5 ± 1.4°C, 8 h/day); the abbreviations for the detected parameters (NF-κB, TNF-α, IFN-γ and IL) are nuclear factor-kappa B, tumor necrosis factor-α, interferon-γ and interleukin, respectively. *p < 0.05, **p < 0.01, nsno significant difference.
Microbial Diversity and Composition in Jejunum
As shown in Table 2, the HS exposure had no significant effects on the alpha diversity of jejunal microbiota, including Simpson, Chao1 richness estimator (Chao 1), abundance-based coverage estimators (ACE), and Shannon index (p > 0.10). However, as presented in Table 3 and Figure 2, the HS exposure reduced the jejunal microbial species number at the class and order level (p < 0.05) and had a significant trend to decrease the jejunal microbial species number at the phylum and family level (p < 0.10).
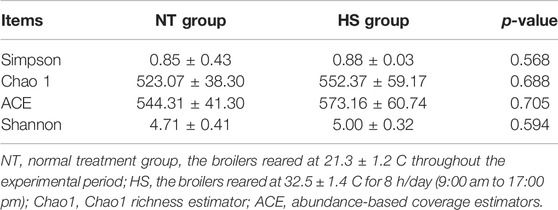
TABLE 2. Effects of heat stress on alpha diversity of jejunal microbiota in yellow-feathered broilers (n = 5).
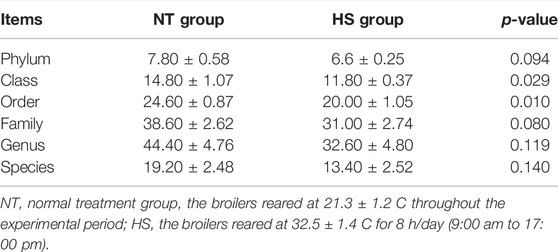
TABLE 3. Effects of heat stress on jejunal microbial species number at each classification level in yellow-feathered broilers (n = 5).
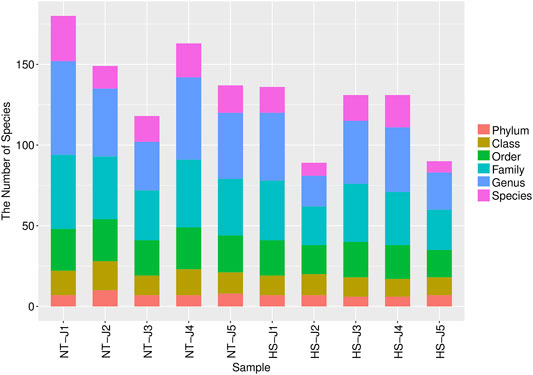
FIGURE 2. Effects of heat stress on distribution of jejunal microbial species number at each classification level in yellow-feathered broilers (n = 5). NT, normal treatment group (21.3 ± 1.2°C, 24 h/day); HS, heat stress group (32.5 ± 1.4°C, 8 h/day).
The results of the jejunal microbial community are presented in Figures 3, 4. There were 884 commonly owned OTUs between the NT and HS groups, 243 OTUs of jejunal microbiota were unique in the NT group, and 270 OTUs of jejunal microbiota were unique in the HS group. The beta diversity revealed that the samples in NT and HS groups have different clusters. Besides, there were no significant differences in the relative abundance of each microbial at the phylum, class, order, and family level (p > 0.10). However, the HS exposure significantly reduced the jejunal relative abundance of Ruminococcus, Bdellovibrio, and Serratia at the genus level (p < 0.05).
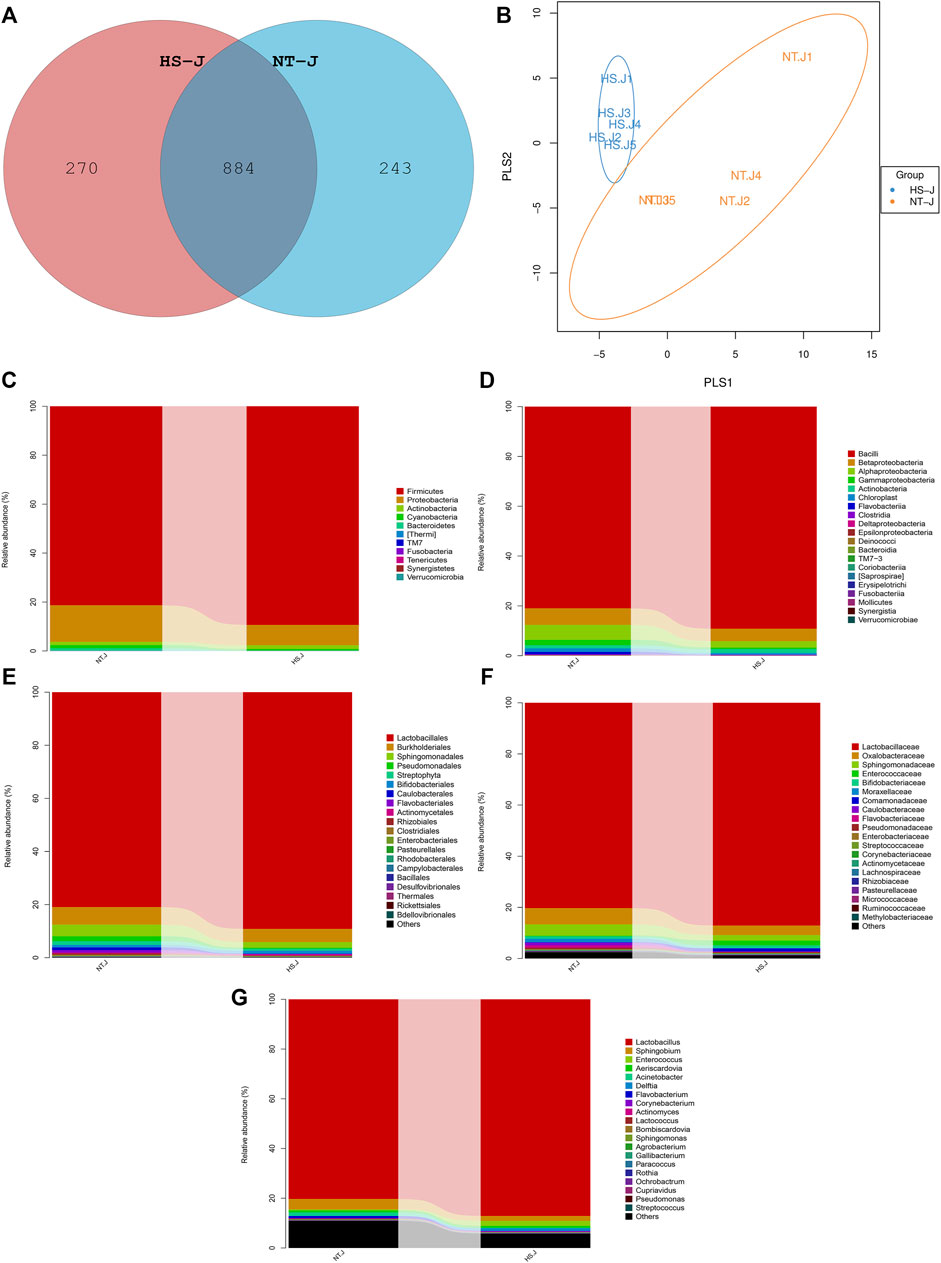
FIGURE 3. Effects of heat stress on jejunal microbial community in yellow-feathered broilers (n = 5). NT, normal treatment group (21.3 ± 1.2°C, 24 h/day); HS, heat stress group (32.5 ± 1.4°C, 8 h/day); (A), operational taxonomic units (OTUs) venn diagram of jejunal microbiota between NT and HS groups; (B), beta diversity of jejunal microbiota between NT and HS groups; (C), microbial compositions at the phylum level; (D), microbial compositions at the class level; (E), microbial compositions at the order level; (F), microbial compositions at the family level; (G), microbial compositions at the genus level.
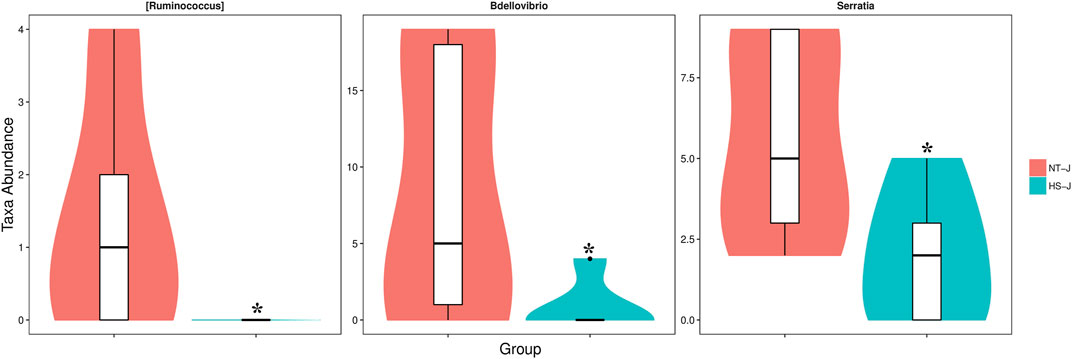
FIGURE 4. Microbials with significantly differential abundance at the genus level as affected by heat stress in yellow-feathered broilers (n = 5). NT, normal treatment group (21.3 ± 1.2°C, 24 h/day); HS, heat stress group (32.5 ± 1.4°C, 8 h/day) *p < 0.05.
Correlation Between Jejunal Microbiome and Immune-Related Genes Expression
The results of Spearman correlation analysis are showed in Figure 5. At the phylum level, Cyanobacteria was positively correlated with IL-10 expression (p < 0.05). Bacteroidetes was negatively correlated with IL-6 expression (p < 0.05). Thermi was negatively correlated with TNF-α and IL-6 expression (p < 0.05), while positively correlated with IL-10 expression (p < 0.01). TM7 was negatively correlated with NF-κB expression (p < 0.05), but positively correlated with IFN-γ expression (p < 0.01). At the genus level, Caulobacteraceae was negatively correlated with TNF-α and IL-6 expression (p < 0.05), whereas positively associated with IL-10 expression (p < 0.05). Actinomyces and Sediminibacterium were positively related with IFN-γ expression (p < 0.01). Ruminococcaceae was negatively correlated with NF-κB, IL-1β and IL-6 expression (p < 0.05). Thermus was negatively correlated with TNF-α and IL-6 expression (p < 0.05), but positively correlated with IL-10 expression (p < 0.01). Bdellovibrio was negatively correlated with TNF-α (p < 0.05) and IL-6 (p < 0.01) expression. Clostridiales was negatively related with NF-κB (p < 0.05), TNF-α (p < 0.01), IL-1β (p < 0.01) and IL-6 (p < 0.01) expression. Bacteroides was negatively associated with IL-1β expression (p < 0.01). Sphingomonadales was negatively correlated with NF-κB expression (p < 0.05), but positively related with IFN-γ expression (p < 0.01). Ruminococcus was negatively related with NF-κB (p < 0.05), TNF-α (p < 0.05), IL-1β (p < 0.05) and IL-6 (p < 0.01) expression.
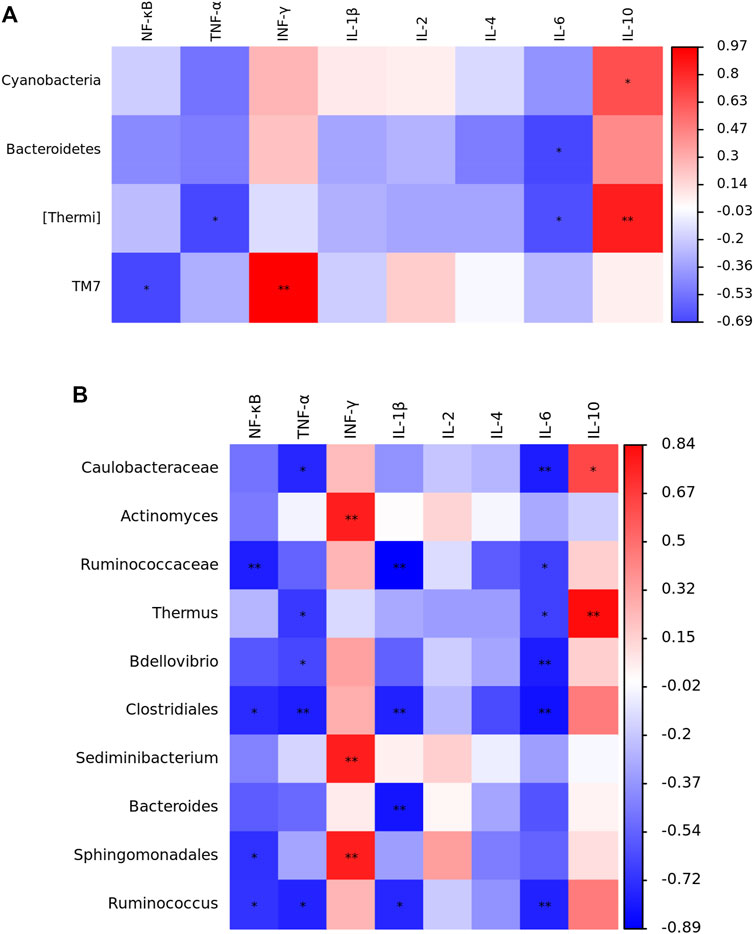
FIGURE 5. Spearman correlation analysis between the jejunal microbials (A), phylum; (B), genus level and immune-related genes expression in yellow-feathered broilers under heat stress (n = 5). NT, normal treatment group (21.3 ± 1.2°C, 24 h/day), HS, heat stress group (32.5 ± 1.4°C, 8 h/day); NF-κB, nuclear factor-kappa B; TNF-α, tumor necrosis factor-α; IFN-γ, interferon-γ; IL, interleukin. *p < 0.05, **p < 0.01.
Discussion
Due to the global climate change, high ambient temperature (around 32°C) often occurs in chicken houses during summer, thus impairing the health and productivity of broilers and resulting in significant economic losses to producers (Wasti et al., 2020; Greene et al., 2021). Although the yellow-feathered broilers have better thermal tolerance than fast-growing broilers due to their slower growth and lower metabolic rate, 32°C is not in the thermoneutral zone of slow-growing broilers, which also causes a series of deleterious consequences for yellow-feathered broilers (Shao et al., 2018; Guo et al., 2021a; Liu et al., 2021b). The gut is considered the main target of HS (Chauhan et al., 2021). The jejunum is the middle intestine and is related to most of the nutrients’ digestion and absorption in broilers; accordingly, the jejunum is more susceptible to HS (Song et al., 2013). Furthermore, as a representative segment of the small intestine, the jejunum is always selected for intestinal research, and jejunal immunity plays a crucial role in maintaining gut health (Song et al., 2014; Abdelli et al., 2021; Wang C. et al., 2021). However, very limited data is available regarding HS exposure’s effects on jejunal immune response in yellow-feathered broilers. In the present research, the ELISA and mRNA results indicated that HS exposure elevated the production of pro-inflammatory cytokines (TNF-α, IL-1β, and IL-6) in jejunal mucosa. In accordance with the studies of fast-growing broilers, Song et al. (2017) found that the HS has increased the mRNA expression levels of pro-inflammatory cytokines such as IL-1β and IL-6 in the jejunum of Arbor Acres broilers. Siddiqui et al. (2020) demonstrated that Ross broilers exposed to HS had high relative mRNA expression levels of jejunal pro-inflammatory cytokine genes (IL-6 and TNF-α). At the same time, Al-Zghoul and Saleh (2020) observed that HS exposure up-regulated the jejunal mRNA expression of TNF-α, IL-1β, and IL-6. Furthermore, this study found that HS promoted the jejunal mRNA expression levels of NF-κB. The NF-κB is widely reported to activate gut inflammatory responses (Sahin, 2015; Liu et al., 2016). Therefore, the findings of this study further confirmed the theory that HS exposure triggers an intestinal inflammatory response in both fast- and slow-growing broilers, which is probably involved in the activation of NF-κB signaling.
Gut microbiota homeostasis is critical for eliminating pathogens, maintaining intestinal epithelial integrity, and regulating mucosal immunity in the GIT (Cao et al., 2021). Existing studies have primarily focused on the effect of HS on the hindgut microbiota of broilers and analyzing the role of microbiota in mediating small intestinal health (Patra and Kar, 2021; Yadav et al., 2021). However, the microbiota colonized in the jejunum can directly crosstalk with the barrier integrity and immune function (Gong et al., 2020). Thus, this study detected the jejunal microbiota structure and community using 16S rRNA sequencing. The alpha diversity of jejunal microbiota was not affected by HS exposure. Similar findings from hindgut microbiota were obtained previously. For instance, Liu et al. (2021a) reported that HS exposure had no significant impact on the alpha diversity index of cecal microbiota in yellow-feathered broilers. Wasti et al. (2021b) suggested that there were no obvious changes in cecal microbial alpha diversity such as Shannon entropy and Simpson’s index of Cobb broilers under HS. Moreover, in this study, the jejunal microbial species number was decreased by HS, suggesting that although HS exposure did not alter the alpha diversity, it reduced the species richness of jejunal microbiota in yellow-feathered broilers. Regarding the microbial composition, HS had no significant effects on the relative abundance of microbiota at the phylum, class, order, and family level. Partly consistent with our results, Shi et al. (2019) found that 28 days of HS did not change the cecal microbial abundance at the phylum level. According to Wang G. et al. (2021), there were no significantly different abundances of cecal bacteria at the phylum level in Arbor Acres broilers under HS. On the contrary, in the study of Liu et al. (2021a), there were cecal bacteria with significantly different abundances at the phylum, class, order, and family level in yellow-feathered broilers exposed to HS. Many factors could contribute to the regulation of gut microbiota composition, such as diet, host genetic background, stress intensity, and intestinal segments (Xia et al., 2022), which might explain the inconsistencies. Notably, HS exposure significantly reduced the relative abundance of Ruminococcus, Bdellovibrio, and Serratia at the genus level. Ruminococcus can break down the cellulose and ferment glucose, xylose, and polysaccharides, producing beneficial metabolites such as acetate, propionate, and butyrate, which have anti-inflammatory functions in the gut (La Reau and Suen, 2018; Jha and Mishra, 2021). It has been found that the abundance of Ruminococcus was reduced in the intestinal inflammatory process of ulcerative colitis and revealed its probiotic and gut health-promoting property (Li et al., 2020). Bdellovibrio has bacteriophage-like action to control harmful bacteria (Sockett and Lambert, 2004) and plays a probiotic role in improving gut health (Dwidar et al., 2012). A previous study reported that Bdellovibrio enters the intestine and releases peptidoglycan and immune substances through glycanase and peptidase, thereby exerting immunomodulatory activity (Chen et al., 2018). Additionally, Serratia is a type of intestinal commensal microbial in animals; the prodigiosin, a metabolite from Serratia, which exhibits anticancer and antibacterial functions (Soenens and Imperial, 2020). However, some strains of Serratia are pathogenic (Abreo and Altier, 2019); the studies on Serratia, including the beneficial and pathogenic strains in poultry intestines, are limited. It is necessary to further isolate and identify the Serratia species in the GIT of broilers and clarify their roles in HS response. Based on the potential immunomodulatory and antibacterial effects of the microbiota with significantly different abundances, it could be speculated that HS-induced jejunal immune dysfunction might be related to the reduced levels of Ruminococcus, Bdellovibrio, and Serratia, but the specific interaction analysis is required.
Emerging evidence linking HS to intestinal damage suggests that the gut microbiota might be an under-appreciated mediator of the inflammatory response (Wen et al., 2021). To delineate the interaction of jejunal microbiota and immunity in yellow-feathered broilers, Spearman correlation analysis was performed in this study. At the phylum level, four species of bacteria were significantly associated with immune genes expression, which were positively correlated with anti-inflammatory cytokines and negatively correlated with pro-inflammatory cytokines. Bacteroidetes is a common parasitic microbial in the gut; it also showed a negative correlation with intestinal pro-inflammatory cytokines in pigs (Xia et al., 2022). There are few studies on the relationship between Cyanobacteria, Thermi, TM7, and immune response; further research is needed to elucidate this correlation (Whitton and Potts, 2012; Rinttilä and Apajalahti, 2013; Chen et al., 2021). At the genus level, ten species of bacteria were significantly related to the expression of immune genes. Specifically, the abundances of Ruminococcus and Bdellovibrio were negatively associated with the pro-inflammatory cytokine expression and NF-κB signaling; this may be due to the anti-inflammatory and immunomodulatory activities of the metabolites produced by Ruminococcus and Bdellovibrio (Chen et al., 2018; Li et al., 2020). Besides, Actinomyces has been reported to regulate the immune system, especially cellular immunity, and positively correlated with the production of INF-γ (Hötte et al., 2019); this is consistent with our results. The correlation result of Ruminococcaceae was similar to the Ruminococcus. A previous study also suggested that the Ruminococcaceae was involved in regulating inflammatory bowel disease through secondary bile acids (Guo et al., 2020). Clostridiales exhibited a significant negative correlation with pro-inflammatory cytokines and NF-κB signaling. The Clostridium butyricum of Clostridiales is a probiotic and has anti-inflammatory properties in broilers (Zhang et al., 2014). Conversely, the Clostridiales also contain some pathogenic bacteria species (Paredes-Sabja et al., 2011). Therefore, the role of Clostridiales in modulating gut immunity is subject to further verification. Furthermore, the Bacteroides showed a similar correlation trend with Bacteroidetes at the phylum level. However, other microbiota related to immune genes expression, such as Caulobacteraceae, Thermus, Sediminibacterium, and Sphingomonadales, their function with immunity is still unclear and needs to be elucidated. Together, integrating the bacterial abundances and correlation analysis, it can be noted that Ruminococcus and Bdellovibrio may be the key microbially mediated elements of the jejunal inflammatory response during HS. And, dietary supplementation of Ruminococcus and Bdellovibrio as potential probiotics may alleviate HS-induced intestinal inflammation in yellow-feathered broilers; further validation of this hypothesis would be valuable.
Conclusion
Four weeks of heat stress at 32.5°C adversely affected jejunal immunity via stimulating the production of pro-inflammatory cytokines such as TNF-α, IL-1β, and IL-6 and activating the NF-κB signaling. Heat stress had no significant impact on alpha diversity. The relative abundance of Ruminococcus, Bdellovibrio, and Serratia in the jejunum was decreased by heat stress. Furthermore, Ruminococcus and Bdellovibrio showed a significant negative correlation with pro-inflammatory cytokines expression. The findings not only provide novel insights into the interaction of jejunal immune response and microbiota, but also contribute to the development of potential nutritional mitigation strategies (probiotics intervention) for the regulation of intestinal immunity in yellow-feathered broilers under heat stress situations.
Data Availability Statement
The datasets presented in this study can be found in online repositories. The names of the repository/repositories and accession number(s) can be found below: https://www.ncbi.nlm.nih.gov/, PRJNA816650.
Ethics Statement
The animal study was reviewed and approved by Department of Animal Science of Guangdong Ocean University (dkx-20181217).
Author Contributions
Conceptualization, W-CL, BB, and RJ. Methodology, W-CL and RJ. Analysis, M-YH. Data curation, W-CL and M-YH. Writing-original draft preparation, W-CL. Writing-review and editing, BB and RJ. Supervision, BB and RJ. Project administration, W-CL. Funding acquisition, W-CL.
Funding
This research was funded by the National Nature Science Foundation of China (32002196).
Conflict of Interest
The authors declare that the research was conducted in the absence of any commercial or financial relationships that could be construed as a potential conflict of interest.
Publisher’s Note
All claims expressed in this article are solely those of the authors and do not necessarily represent those of their affiliated organizations, or those of the publisher, the editors and the reviewers. Any product that may be evaluated in this article, or claim that may be made by its manufacturer, is not guaranteed or endorsed by the publisher.
References
Abdelli N., Ramser A., Greene E. S., Beer L., Tabler T. W., Orlowski S. K., et al. (2021). Effects of Cyclic Chronic Heat Stress on the Expression of Nutrient Transporters in the Jejunum of Modern Broilers and Their Ancestor Wild Jungle Fowl. Front. Physiol. 12, 733134. doi:10.3389/fphys.2021.733134
Abreo E., Altier N. (2019). Pangenome of Serratia marcescens Strains from Nosocomial and Environmental Origins Reveals Different Populations and the Links between Them. Sci. Rep. 9, 46–48. doi:10.1038/s41598-018-37118-0
Al-Zghoul M. B., Mohammad Saleh K. M. (2020). Effects of Thermal Manipulation of Eggs on the Response of Jejunal Mucosae to Posthatch Chronic Heat Stress in Broiler Chickens. Poult. Sci. 99, 2727–2735. doi:10.1016/j.psj.2019.12.038
Cao C., Chowdhury V. S., Cline M. A., Gilbert E. R. (2021). The Microbiota-Gut-Brain axis during Heat Stress in Chickens: A Review. Front. Physiol. 12, 752265. doi:10.3389/fphys.2021.752265
Chauhan S. S., Rashamol V. P., Bagath M., Sejian V., Dunshea F. R. (2021). Impacts of Heat Stress on Immune Responses and Oxidative Stress in Farm Animals and Nutritional Strategies for Amelioration. Int. J. Biometeorol. 65, 1231–1244. doi:10.1007/s00484-021-02083-3
Chen K., Zhong W., Gao Z. (2018). Research Progress on Utilization of Bdellovibrio in Aquaculture. Fish. Sci. 37, 284–290. doi:10.16378/j.cnki.1003-1111.2018.02.024
Chen S., Luo S., Yan C. (2021). Gut Microbiota Implications for Health and Welfare in Farm Animals: A Review. Animals 12, 93. doi:10.3390/ani12010093
Dwidar M., Monnappa A. K., Mitchell R. J. (2012). The Dual Probiotic and Antibiotic Nature of Bdellovibrio Bacteriovorus. BMB Rep. 45, 71–78. doi:10.5483/bmbrep.2012.45.2.71
Gong H. Z., Lang W. Y., Lan H. N., Fan Y. Y., Wang T. P., Chu Q. R., et al. (2020). Effects of Laying Breeder Hens Dietary β-carotene, Curcumin, Allicin, and Sodium Butyrate Supplementation on the Jejunal Microbiota and Immune Response of Their Offspring Chicks. Poult. Sci. 99, 3807–3816. doi:10.1016/j.psj.2020.03.065
Gou Z. Y., Jiang S. Q., Jiang Z. Y., Zheng C. T., Li L., Ruan D., et al. (2016). Effects of High Peanut Meal with Different Crude Protein Level Supplemented with Amino Acids on Performance, Carcass Traits and Nitrogen Retention of Chinese Yellow Broilers. J. Anim. Physiol. Anim. Nutr. 100, 657–664. doi:10.1111/jpn.12420
Greene E. S., Maynard C., Owens C. M., Meullenet J.-F., Dridi S. (2021). Effects of Herbal Adaptogen Feed-Additive on Growth Performance, Carcass Parameters, and Muscle Amino Acid Profile in Heat-Stressed Modern Broilers. Front. Physiol. 12, 784952. doi:10.3389/fphys.2021.784952
Guo X. Y., Liu X. J., Hao J. Y. (2020). Gut Microbiota in Ulcerative Colitis: Insights on Pathogenesis and Treatment. J. Dig. Dis. 21, 147–159. doi:10.1111/1751-2980.12849
Guo Y., Balasubramanian B., Zhao Z.-H., Liu W.-C. (2021a). Heat Stress Alters Serum Lipid Metabolism of Chinese Indigenous Broiler Chickens-A Lipidomics Study. Environ. Sci. Pollut. Res. 28, 10707–10717. doi:10.1007/s11356-020-11348-0
Guo Y., Liao J. H., Liang Z. L., Balasubramanian B., Liu W. C. (2021b). Hepatic Lipid Metabolomics in Response to Heat Stress in Local Broiler Chickens Breed (Huaixiang Chickens). Vet. Med. Sci. 7, 1369–1378. doi:10.1002/vms3.462
Hötte G. J., Koudstaal M. J., Verdijk R. M., Titulaer M. J., Claes J. F. H. M., Strabbing E. M., et al. (2019). Intracranial Actinomycosis of Odontogenic Origin Masquerading as Auto-Immune Orbital Myositis: A Fatal Case and Review of the Literature. BMC Infect. Dis. 19, 763–768. doi:10.1186/s12879-019-4408-2
Jha R., Mishra P. (2021). Dietary Fiber in Poultry Nutrition and Their Effects on Nutrient Utilization, Performance, Gut Health, and on the Environment: A Review. J. Anim. Sci. Biotechnol. 12, 51. doi:10.1186/s40104-021-00576-0
Kogut M. H., Arsenault R. J. (2016). Editorial: Gut Health: The New Paradigm in Food Animal Production. Front. Vet. Sci. 3, 71. doi:10.3389/fvets.2016.00071
La Reau A. J., Suen G. (2018). The Ruminococci: Key Symbionts of the Gut Ecosystem. J. Microbiol. 56, 199–208. doi:10.1007/s12275-018-8024-4
Lara L., Rostagno M. (2013). Impact of Heat Stress on Poultry Production. Animals 3, 356–369. doi:10.3390/ani3020356
Li X., Cao Z., Yang Y., Chen L., Liu J., Lin Q., et al. (2019). Correlation between Jejunal Microbial Diversity and Muscle Fatty Acids Deposition in Broilers Reared at Different Ambient Temperatures. Sci. Rep. 9, 1–12. doi:10.1038/s41598-019-47323-0
Li Q., Ding X., Liu K., Marcella C., Liu X., Zhang T., et al. (2020). Fecal Microbiota Transplantation for Ulcerative Colitis: The Optimum Timing and Gut Microbiota as Predictors for Long-Term Clinical Outcomes. Clin. Transl. Gastroenterol. 11, e00224. doi:10.14309/ctg.0000000000000224
Lin M. (2008). Thoughts on the Industrialization Status of Yellow-Feathered Broilers in Guangdong Province, China. China Poult. Indust. Guide 6, 10–11.
Liu L., Fu C., Yan M., Xie H., Li S., Yu Q., et al. (2016). Resveratrol Modulates Intestinal Morphology and HSP70/90, NF-κB and EGF Expression in the Jejunal Mucosa of Black-Boned Chickens on Exposure to Circular Heat Stress. Food Funct. 7, 1329–1338. doi:10.1039/c5fo01338k
Liu W., Yuan Y., Sun C., Balasubramanian B., Zhao Z., An L. (2019). Effects of Dietary Betaine on Growth Performance, Digestive Function, Carcass Traits, and Meat Quality in Indigenous Yellow-Feathered Broilers under Long-Term Heat Stress. Animals 9, 506. doi:10.3390/ani9080506
Liu W.-C., Guo Y., An L.-L., Zhao Z.-H. (2021a). Protective Effects of Dietary Betaine on Intestinal Barrier Function and Cecal Microbial Community in Indigenous Broiler Chickens Exposed to High Temperature Environment. Environ. Sci. Pollut. Res. 28, 10860–10871. doi:10.1007/s11356-020-11326-6
Liu W.-C., Ou B.-H., Liang Z.-L., Zhang R., Zhao Z.-H. (2021b). Algae-derived Polysaccharides Supplementation Ameliorates Heat Stress-Induced Impairment of Bursa of Fabricius via Modulating NF-κB Signaling Pathway in Broilers. Poult. Sci. 100, 101139. doi:10.1016/j.psj.2021.101139
Livak K. J., Schmittgen T. D. (2001). Analysis of Relative Gene Expression Data Using Real-Time Quantitative PCR and the 2−ΔΔCT Method. Methods 25, 402–408. doi:10.1006/meth.2001.1262
Paredes-Sabja D., Setlow P., Sarker M. R. (2011). Germination of Spores of Bacillales and Clostridiales Species: Mechanisms and Proteins Involved. Trends Microbiol. 19, 85–94. doi:10.1016/j.tim.2010.10.004
Patra A. K., Kar I. (2021). Heat Stress on Microbiota Composition, Barrier Integrity, and Nutrient Transport in Gut, Production Performance, and its Amelioration in Farm Animals. J. Anim. Sci. Technol. 63, 211–247. doi:10.5187/jast.2021.e48
Quinteiro-Filho W. M., Ribeiro A., Ferraz-De-Paula V., Pinheiro M. L., Sakai M., Sá L. R. M., et al. (2010). Heat Stress Impairs Performance Parameters, Induces Intestinal Injury, and Decreases Macrophage Activity in Broiler Chickens. Poult. Sci. 89, 1905–1914. doi:10.3382/ps.2010-00812
Rajput I. R., Ying H., Yajing S., Arain M. A., Weifen L., Ping L., et al. (2017). Saccharomyces Boulardii and Bacillus Subtilis B10 Modulate TLRs and Cytokines Expression Patterns in Jejunum and Ileum of Broilers. PLoS One 12, e0173917. doi:10.1371/journal.pone.0173917
Rinttilä T., Apajalahti J. (2013). Intestinal Microbiota and Metabolites-Implications for Broiler Chicken Health and Performance. J. Appl. Poult. Res. 22, 647–658. doi:10.3382/japr.2013-00742
Sahin K. (2015). Modulation of NF-κB and Nrf2 Pathways by Lycopene Supplementation in Heat-Stressed Poultry. World Poult. Sci. J. 71, 271–284. doi:10.1017/s0043933915000288
Shao D., Wang Q., Hu Y., Shi S., Tong H. (2018). Effects of Cyclic Heat Stress on the Phenotypic Response, Meat Quality and Muscle Glycolysis of Breasts and Thighs of Yellow-Feather Broilers. Ital. J. Anim. Sci. 18, 301–308. doi:10.1080/1828051x.2018.1520051
Shi D., Bai L., Qu Q., Zhou S., Yang M., Guo S., et al. (2019). Impact of Gut Microbiota Structure in Heat-Stressed Broilers. Poult. Sci. 98, 2405–2413. doi:10.3382/ps/pez026
Siddiqui S. H., Kang D., Park J., Khan M., Shim K. (2020). Chronic Heat Stress Regulates the Relation between Heat Shock Protein and Immunity in Broiler Small Intestine. Sci. Rep. 10, 18872–18911. doi:10.1038/s41598-020-75885-x
Sockett R. E., Lambert C. (2004). Bdellovibrio as Therapeutic Agents: A Predatory Renaissance? Nat. Rev. Microbiol. 2, 669–675. doi:10.1038/nrmicro959
Soenens A., Imperial J. (2020). Biocontrol Capabilities of the Genus Serratia. Phytochem. Rev. 19, 577–587. doi:10.1007/s11101-019-09657-5
Song J., Jiao L. F., Xiao K., Luan Z. S., Hu C. H., Shi B., et al. (2013). Cello-oligosaccharide Ameliorates Heat Stress-Induced Impairment of Intestinal Microflora, Morphology and Barrier Integrity in Broilers. Animal Feed Sci. Technol. 185, 175–181. doi:10.1016/j.anifeedsci.2013.08.001
Song J., Xiao K., Ke Y. L., Jiao L. F., Hu C. H., Diao Q. Y., et al. (2014). Effect of a Probiotic Mixture on Intestinal Microflora, Morphology, and Barrier Integrity of Broilers Subjected to Heat Stress. Poult. Sci. 93, 581–588. doi:10.3382/ps.2013-03455
Song Z., Cheng K., Zhang L., Wang T. (2017). Dietary Supplementation of Enzymatically Treated Artemisia Annua Could Alleviate the Intestinal Inflammatory Response in Heat-Stressed Broilers. J. Therm. Biol. 69, 184–190. doi:10.1016/j.jtherbio.2017.07.015
Vandana G. D., Sejian V., Lees A. M., Pragna P., Silpa M. V., Maloney S. K. (2021). Heat Stress and Poultry Production: Impact and Amelioration. Int. J. Biometeorol. 65, 163–179. doi:10.1007/s00484-020-02023-7
Wang X. J., Feng J. H., Zhang M. H., Li X. M., Ma D. D., Chang S. S. (2018). Effects of High Ambient Temperature on the Community Structure and Composition of Ileal Microbiome of Broilers. Poult. Sci. 97, 2153–2158. doi:10.3382/ps/pey032
Wang C., Zhao F., Li Z., Jin X., Chen X., Geng Z., et al. (2021a). Effects of Resveratrol on Growth Performance, Intestinal Development, and Antioxidant Status of Broilers under Heat Stress. Animals 11, 1427. doi:10.3390/ani11051427
Wang G., Li X., Zhou Y., Feng J., Zhang M. (2021b). Effects of Heat Stress on Gut-Microbial Metabolites, Gastrointestinal Peptides, Glycolipid Metabolism, and Performance of Broilers. Animals 11, 1286. doi:10.3390/ani11051286
Wang Y., Gou Z., Lin X., Fan Q., Ye J., Jiang S. (2021c). Optimal Level of Supplemental Manganese for Yellow-Feathered Broilers during the Growth Phase. Animals 11, 1389. doi:10.3390/ani11051389
Wasti S., Sah N., Mishra B. (2020). Impact of Heat Stress on Poultry Health and Performances, and Potential Mitigation Strategies. Animals 10, 1266. doi:10.3390/ani10081266
Wasti S., Sah N., Lee C. N., Jha R., Mishra B. (2021a). Dietary Supplementation of Alpha-Lipoic Acid Mitigates the Negative Effects of Heat Stress in Broilers. PloS one 16, e0254936. doi:10.1371/journal.pone.0254936
Wasti S., Sah N., Singh A. K., Lee C. N., Jha R., Mishra B. (2021b). Dietary Supplementation of Dried Plum: A Novel Strategy to Mitigate Heat Stress in Broiler Chickens. J. Anim. Sci. Biotechno. 12, 1–17. doi:10.1186/s40104-021-00571-5
Wen C., Li S., Wang J., Zhu Y., Zong X., Wang Y., et al. (2021). Heat Stress Alters the Intestinal Microbiota and Metabolomic Profiles in Mice. Front. Microbiol. 12, 706772. doi:10.3389/fmicb.2021.706772
Whitton B. A., Potts M. (2012). “Introduction to the Cyanobacteria,” in Ecology of Cyanobacteria II (German: Springer), 1–13. doi:10.1007/978-94-007-3855-3_1
Wu Q. J., Liu N., Wu X. H., Wang G. Y., Lin L. (2018). Glutamine Alleviates Heat Stress-Induced Impairment of Intestinal Morphology, Intestinal Inflammatory Response, and Barrier Integrity in Broilers. Poult. Sci. 97, 2675–2683. doi:10.3382/ps/pey123
Xia B., Wu W., Fang W., Wen X., Xie J., Zhang H. (2022). Heat Stress-Induced Mucosal Barrier Dysfunction is Potentially Associated with Gut Microbiota Dysbiosis in Pigs. Anim. Nutr. 8, 289–299. doi:10.1016/j.aninu.2021.05.012
Yadav S., Jha R. (2019). Strategies to Modulate the Intestinal Microbiota and Their Effects on Nutrient Utilization, Performance, and Health of Poultry. J. Anim. Sci. Biotechnol. 10, 2. doi:10.1186/s40104-018-0310-9
Yadav S., Caliboso K. D., Nanquil J. E., Zhang J., Kae H., Neupane K., et al. (2021). Cecal Microbiome Profile of Hawaiian Feral Chickens and Pasture-Raised Broiler (Commercial) Chickens Determined Using 16S rRNA Amplicon Sequencing. Poult. Sci. 100, 101181. doi:10.1016/j.psj.2021.101181
Keywords: gene expression, gut microbiota, heat stress, intestinal immunity, yellow-feathered broilers
Citation: Liu W-C, Huang M-Y, Balasubramanian B and Jha R (2022) Heat Stress Affects Jejunal Immunity of Yellow-Feathered Broilers and Is Potentially Mediated by the Microbiome. Front. Physiol. 13:913696. doi: 10.3389/fphys.2022.913696
Received: 06 April 2022; Accepted: 05 May 2022;
Published: 23 May 2022.
Edited by:
Michael Kogut, Agricultural Research Service, United States Department of Agriculture, United StatesReviewed by:
Sharif Hasan Siddiqui, Jeonbuk National University, South KoreaDany Mesa, Instituto de Pesquisa Pelé Pequeno Príncipe, Brazil
Copyright © 2022 Liu, Huang, Balasubramanian and Jha. This is an open-access article distributed under the terms of the Creative Commons Attribution License (CC BY). The use, distribution or reproduction in other forums is permitted, provided the original author(s) and the copyright owner(s) are credited and that the original publication in this journal is cited, in accordance with accepted academic practice. No use, distribution or reproduction is permitted which does not comply with these terms.
*Correspondence: Balamuralikrishnan Balasubramanian, YmFsYS5tLmtAc2Vqb25nLmFjLmty; Rajesh Jha, cmpoYUBoYXdhaWkuZWR1