- 1Institute of Heart Diseases, Wroclaw Medical University, Wroclaw, Poland
- 2Institute of Heart Diseases, University Hospital, Wroclaw, Poland
From a physiological point of view, peripheral chemoreceptors (PCh) are the main sensors of hypoxia in mammals and are responsible for adaptation to hypoxic conditions. Their stimulation causes hyperventilation—to increase oxygen uptake and increases sympathetic output in order to counteract hypoxia-induced vasodilatation and redistribute the oxygenated blood to critical organs. While this reaction promotes survival in acute settings it may be devastating when long-lasting. The permanent overfunctionality of PCh is one of the etiologic factors and is responsible for the progression of sympathetically-mediated diseases. Thus, the deactivation of PCh has been proposed as a treatment method for these disorders. We review here physiological background and current knowledge regarding the influence of widely prescribed medications on PCh acute and tonic activities.
Introduction
The clusters of chemoreceptor cells in the human body are localized in the central nervous system—central chemoreceptors (CCh) and in the carotid (CB) and aortic bodies - peripheral chemoreceptors (PCh) (O'Regan and Majcherczyk, 1982). The former are mainly hypercapnia sensors while the latter are activated by hypoxia, hypercapnia and acidosis (O'Regan and Majcherczyk, 1982). Activation of both areas leads to hyperventilation, sympathetically-mediated vasoconstriction and usually tachycardia (Kara et al., 2003; Tubek et al., 2018; Zera et al., 2019). The magnitude of the acute (provoked) physiological response (most commonly ventilatory response) to the stimulation of PCh is called peripheral chemoreceptors sensitivity (PChS) and is used as the measure of PCh function (Tubek et al., 2018). However, emerging experimental evidence indicates that PCh exert also tonic (independent of the stimuli) influence on cardio-respiratory centers, leading to a chronic increase in sympathetic tone (Sinski et al., 2012; Tubek et al., 2018; Tubek et al., 2021). This influence is neglectable in healthy individuals; however, it is significantly increased in patients with hypertension (HT), heart failure (HF) or obstructive sleep apnoea and was recognized as an important factor in the pathophysiology and progression of these diseases (Schultz et al., 2007; Sinski et al., 2012; Paton et al., 2013a; Schultz et al., 2013; Xing et al., 2014). The most widely used measurement employed to assess the PCh tonic activity (PChT) is the magnitude of the ventilatory or sympathetic nerve activity (SNA) drop following PCh inhibition (Schultz et al., 2007; Tubek et al., 2018).
Several molecular mechanisms have been identified as potential PChS and PChT sensitizers including upregulation of angiotensin II/nicotinamide adenine dinucleotide phosphate (NADPH) oxidase and serotonin/NADPH oxidase; downregulation of nitric oxide synthases (NOS) and glucagon-like peptide-1 receptor or increase in leptin, endothelin, or proinflammatory cytokines concentrations (Chen et al., 2002; Li et al., 2005; Li et al., 2007; Schultz et al., 2007; Yuan et al., 2018; Shin et al., 2019; Iturriaga et al., 2022; Pauza et al., 2022). Moreover, numerous modulatory neurotransmitters have been identified in CB, such as adrenaline, noradrenaline, dopamine, adenosine and acetylcholine, which adjust PChs’ activity to current needs (Pérez-García et al., 1993; Prabhakar, 1994).
CB has been proposed as a therapeutic target in sympathetically mediated disease based on animal studies (Abdala et al., 2012; Del Rio et al., 2013; McBryde et al., 2013; Schultz et al., 2013) and even some encouraging data from humans, regarding interventional CB deactivation, has been published (Narkiewicz et al., 2016; Niewinski et al., 2017). However, such procedures, particularly bilateral, may be complicated with sleep apnoea exaggeration or inappropriate adaptation to hypoxic environmental conditions e.g. during plane flights (Tubek et al., 2018; Niewinski et al., 2021). This along with invasive and irreversible character of CB excision encourages to look for pharmacological methods of PCh modulation.
Bearing in mind that at least some of the mechanisms leading to PCh overfunctionality are covered with guidelines-recommended cardiovascular medications we review the available literature to: 1) find out proved and potential interactions between these drugs and PCh function to present possible ways of pharmacological modulation of chemoreflex, 2) expose gaps in the evidence of such interaction to stimulate further studies in the field, 3) stress the role of the therapy individualization—drugs decreasing PCh function should be preferred in a patient with known PCh overfunctionality.
Adrenergic Drugs
Dopaminergic and both alpha- and beta-adrenergic receptors are present in chemosensory (type I) glomus cells of most mammals species (Kou et al., 1991; Pérez-García et al., 1993; Prabhakar, 1994). Moreover, the release of dopamine and adrenaline from chemosensory cells in the response to hypoxia is well described in rodents (Fidone et al., 1982; Gonzalez et al., 1992; Kato et al., 2013). According to animal studies, alpha2-receptors agonists seems to have an inhibitory influence on CB, when beta-receptors agonists (βRA), most likely β2RA, are enhancing the CB-mediated response (Mir et al., 1983; Kou et al., 1991). On the other hand, the response to dopamine is dose-dependent. Low-dose dopamine infusion (LDDI) affects mostly type 2 dopamine receptors (D2R) on chemosensory cells, which in consequence limits the release of neurotransmitters (Lehmann et al., 1983; Prabhakar, 1994; González et al., 1995). High doses of dopamine activate both D1R and D2R in CB, but the stimulating effect of postsynaptic D1R dominates and leads to increased stimulation of nerve endings surrounding CB (González et al., 1995). According to the published evidence, it seems that the crucial physiological role of catecholamines in CB is self-limitation of the amplitude and persistence of the response to acute stimulation (PChS modulation) (Prabhakar, 1994). Nevertheless, it was shown recently, that the pre-treatment with propranolol (non-selective beta-blocker) prevents the development of chronic intermittent hypoxia (IH) induced increase in PChT (Alzahrani et al., 2021). It should be finally mentioned, that adrenergic drugs exerts also direct vasomotor effects modulating the perfusion of CB, which may influence the organ functionality (Iturriaga et al., 2016). Since, the net effect of systemically administered adrenergic drug on PCh would be dependent on the pattern of adreno-receptor interactions on chemosensory cells and chemosensory organs perfusion changes.
Beta-Receptors Agonists
In humans, the influence of βRA on PCh is well documented. Similarly as in animals oral, single dose, administration of fenoterol (β2RA) increased baseline ventilation by 15%, as well as, augmented ventilatory response to both hypoxia and hyperoxic hypercapnia in healthy subjects (Yoshiike et al., 1995). This finding indicates that β2RA modulates both central and peripheral chemoperception. Intravenous infusion of another β2RA—salbutamol—increased ventilatory responses to hypoxic hypercapnia (stimulation of both PCh and CCh) and hyperoxic hypercapnia (isolated CCh stimulation) (Leitch et al., 1976).
Effects of β1RA—isoprenaline—were described by Heistad et al. in healthy individuals. The authors revealed a stimulating effect of this catecholamine on resting ventilation, which was reversed with a non-selective beta-receptors blocker (βRB)—propranolol and well known PCh inhibitor—100% hyperoxia (Heistad et al., 1972). However, isoprenaline did not exaggerate the ventilatory response to hypoxia. Due to these difficult to interpret results, which were in contrast to the animal data (Folgering et al., 1982), an elegant study in goats was designed by Hudgel et al. Goats with intact and denervated CB underwent the same protocol consisting of isoprenaline administration and isocapnic, hypoxic exposures (Hudgel et al., 1986). The study showed, that isoprenaline caused the same resting hyperventilation in both groups. This response was reversed by propranolol. Further, despite an increase in minute ventilation, isoprenaline did not alter the carotid sinus nerve chemoreceptor discharge rate during hypoxia. Therefore, despite β1RA increases resting ventilation the influence of these molecules on PChS is scarce both in animals and humans.
Intravenous administration of non-selective β1-, β2- and α1-adrenergic agonist—dobutamine—caused a dose-dependent increase in resting minute ventilation and increased ventilatory and sympathetic responses to hypoxia (PChS), but not to hyperoxic hypercapnia (CChS) in healthy (Velez-Roa et al., 2003; Pathak et al., 2006). The effects of dobutamine were blunted in five subjects with atenolol—a moderately selective β1RA, which despite higher affinity to β1R binds also to β2R (Smith and Teitler, 1999; Pathak et al., 2006). Taking into account the neglectable effects of isoprenaline (β1RA) on hypoxia-induced responses it seems that β2RA and α1RA components are responsible for dobutamine’s ventilatory actions.
Beta-Receptors Blockers
Beloka et al. found that 7 days of oral beta-blocked with selective β1-receptor blocker (β1RB) bisoprolol did not change baseline ventilation; ventilatory and sympathetic nervous system (measured with muscle SNA) responses to hypoxia or hypercapnia in healthy (subjects with generally low PChS and PChT) (Beloka et al., 2008).
However, in the study in HF patients (subjects with generally high PChS and PChT) treatment with carvedilol (non-selective β1RB, β2RB, α1RB), reduced resting ventilation (during normoxia and hypoxia) and exercise hyperventilation compared to placebo (Agostoni et al., 2006). Unfortunately, PChS was not assessed in the study. But in another study in HF population—CARNEBI trial—2-months long treatment with carvedilol caused a significant decrease in PChS and central chemosensitivity compering to the treatment with bisoprolol (Contini et al., 2013). Moreover, PChS but not CCh sensitivity was also diminished with nebivolol compared to bisoprolol (Contini et al., 2013). The actions of bisoprolol on PCh were not assessed against placebo.
Hence, it seems that both peripheral and central chemoperception in humans are modulated by β2R but not β1R. Otherwise, nebivolol β1RB, which is also β3-agonist, seems to decrease PChS via the nitric oxide (NO) pathway. Therefore non-selective or NO-active beta-blockers should be preferred, when PChS modulation is aimed. This observation is particularly interesting in the contest of the recently described protective mechanism of propranolol (non-selective β-blocker) on chronic IH induced hypertension in rats (Alzahrani et al., 2021).
Alfa-Receptors
Otherwise, data regarding the influence of αR stimulation or inhibition on PCh is scarce. Heistad et al. administered phenylephrine (α1RA) in healthy individuals, which decreased resting ventilation (Heistad et al., 1972). However, the influence of the drug on hypoxic ventilatory response was not tested, since the role of PCh or CCh in this change remains questionable.
Dopamine
The influence of dopamine on PCh is well described and is known to be dose-dependent (Iturriaga and Alcayaga, 2004). In healthy humans, high-dose infusion increased and LDDI decreased hypoxic minute ventilation (Welsh et al., 1978). Apart from diminishing the ventilatory response to acute hypoxia (PChS), LDDI diminished also arterial pressure response to the stimuli (Niewinski et al., 2014). Some evidence has also been found for the influence of LDDI on PChT—namely, the infusion decreased normoxic minute ventilation in HFrEF (patients with presumed high PChT), but the data in healthy is inconsistent (with presumed low PChT) (Welsh et al., 1978; Van De Borne et al., 1998; Niewinski et al., 2014). Moreover, withdrawal of LDDI causes temporary hyperventilation probably due to the sudden release of accumulated neurotransmitters following PCh re-activation (Niewinski et al., 2014). LDDI seems to be a good inhibitor of both PChS and PChT for human experimental use; however, one has to be aware, that: 1) high interindividual variability in the inhibitory effect magnitude is observed (Limberg et al., 2016) and 2) the medication itself exerts some hemodynamic effects including slight tachycardia and vasodilatation. The latter are most likely secondary to D2R and adrenergic β1R activation, which may mask the hemodynamic effects of PChT inhibition (Van De Borne et al., 1998; Overgaard and Dzavík, 2008; Niewinski et al., 2014).
Renin-Angiotensin-Aldosterone Axis Modulators
The presence of angiotensinogen and expression of both angiotensin receptors (ATR1 and ATR2) has been confirmed in CB (Lam and Leung, 2002; Leung et al., 2003). Generally, the activation of the ATR1 and ATR2 generates opposite physiological effects at CB level; however, the stimulatory effects of ATR1 dominate the final response (Schultz, 2011). Overexpression of these receptors, leading to augmentation of PChS, has been identified in animal models of IH and HF (Li et al., 2006; Fung, 2014; Lam et al., 2014). Moreover, administration of ATR1 inhibitor in HF rabbits decreased PChS and renal SNA response to hypoxia; however, neither resting ventilation, nor resting renal SNA were changed—lack of evidence on PChT modulation (Li et al., 2006). The similar manoeuvre did not change PChS in healthy animals. Otherwise, administration of angiotensin II analogs increased ventilatory and renal SNA responses to hypoxia (acute PChS) in healthy animals, but not in HF ones (Li et al., 2006). These data suggest that angiotensin signalling has a modulatory role in PChS and depends on the expression and saturation of ATR1. It should be noticed, that angiotensin II acts also directly on central nervous system leading to systemic sympathoexcitation, which via efferent nerve fibers may further modulate PCh functionalities (Ye et al., 2002b).
The influence of ATR1 inhibition on PCh is poorly studied in humans. Among a few studies in the topic, all tested selective ATR1 blocker—losartan. Consistently with animal data administration of the drug did not affect resting ventilation (PChT) in healthy individuals (Foster et al., 2010) and in patients with obstructive sleep apnoea (OSA) (Morgan et al., 2018). Otherwise, the study conducted in hypertensive patients with OSA (with presumed high PChS) revealed a lack of the influence of losartan on the hemodynamic, muscle SNA and ventilatory responses to hypoxia (PChS) (Morgan et al., 2018). However, patients included were optimally treated (majority were prescribed with continuous positive airway pressure—CPAP) and had generally low PChS and muscle SNA response to hypoxia at the study entry, what finally influenced on negative results of the study (Morgan et al., 2018). Further, Foster et al. (2010) found that treatment with losartan prevents IH-induced increase in mean arterial pressure in healthy individuals; however, were unable to prove the influence of the drug on PChS since IH in their model did not increase PChS. Unfortunately, the influence of ATR antagonists in other populations of patients with presumed high PChS and PChT, like HF or HT, has not been tested. Solaiman et al. (2014) tested the effects of intravenous infusion of low and high doses of angiotensin II in healthy volunteers, but in contrast to animal studies neither peripheral nor central chemosensitivity was influenced with the intervention. The influence of angiotensin-converting enzyme inhibitors and aldosterone antagonists on PChS has not been tested in animal and human subjects.
Nitric Oxide
Both enzymes responsible for nitric oxide (NO) synthesis namely neuronal and endothelial synthases are present in CB (Wang et al., 1993). Two modes of NO synthesis have been distinguished in rodents’ CBs: 1) basal production, which is responsible for limiting CB tonic discharges during normoxia (Ding et al., 2008; Ding et al., 2011) and 2) hypoxia-dependent acute/provoked, which is self-limiting the acute response (Ye et al., 2002a; Yamamoto et al., 2006; Fung, 2014). The intensity of the basal/chronic NO synthesis acts on PChT—taking an important role e.g. in the upregulation of this parameter in HF (Ding et al., 2008) when the provoked production modulates PChS diminishing the acute response to hypoxia, e.g. in chronic IH model (Ye et al., 2002a).
The influence of NO concentrations on PChS in humans was tested by Bock et al. Researchers revealed that long-term dietary nitrate supplementation with beetroot powder in older healthy subjects caused a significant reduction in PChS compared to placebo (Bock et al., 2017a; Bock et al., 2017b). Further, Notay et al. found a drop in muscle SNA following acute beetroot oral supplementation in healthy humans, which may be hypothetically the consequence of PChT inhibition; however this needs to be confirmed in future studies (Notay et al., 2017). Our group is currently investigating the influence of low-dose intravenous NO (which is used to treat patients with acute HF decompensation), on PChS in stable HF patients and the results will be available soon.
Antiplatelets—P2Y12 Adenosine Receptors Antagonists
Storage of adenine nucleotides was described in CB cells in animals and adenosine, via activation of postsynaptic P2X and A2s receptors in the CBs, is one of the neurotransmitters responsible for excitatory response to hypoxia (Böck, 1980; Leonard et al., 2018). However, adenosine acts also on the presynaptic P2Y receptors group, including P2Y12 receptors, which inhibit type I cells by negative feedback mechanisms and limits the response (Agarwal et al., 2011; Leonard et al., 2018). Intracarotid injections of adenosine in cats caused a dose-dependent increase in the discharge rate of CB neural afferents (McQueen and Ribeiro, 1983). The physiological role of adenosine in CB has also been confirmed in conscious hypertensive humans, where unilateral intracarotid administration of adenosine caused dose-dependent hyperventilation and hypertension (Tubek et al., 2016). Pharmacological modification of CB has been tested by Pijacka et al. in spontaneously hypertensive rats. Administration of P2X3 antagonist normalized tonic sympathetic activity to the extent seen in healthy animals and reduced chemoreflex-mediated sympathetic activity and additionally decreased blood pressure and heart rate (Pijacka et al., 2016). Overexpression of P2X3 receptors was also found in hypertensive humans cadavers, which suggests a possible role of these structures in the pathogenesis of human hypertension (Pijacka et al., 2016). This, so far hypothetical role, may get investigatable soon, as gefapixant - P2X3 receptors antagonist - is currently tested in humans as a drug for chronic cough (Muccino et al., 2020).
PCh functionality may be theoretically influenced by antiplatelet drugs widely prescribed in humans—clopidogrel, tikagrelor or prasugrel—via adenosine P2Y12 receptor inhibition. Moreover, one of the widely prescribed P2Y12 inhibitors—ticagrelor—has proven, both in animal and human models, inhibitory influence on adenosine cellular uptake, leading to increased tissue adenosine concentrations (Cattaneo and Faioni, 2012). Further, some clinical evidence may indicate possible effects of ticagrelor on chemoreflex. First of all, the most common side effect of ticagrelor treatment is dyspnea, which was hypothesised to be mediated by chemoreceptors (Cattaneo and Faioni, 2012). Secondly, exaggeration/new onset of disordered breathing (including Cheyne-Stokes respiration) was observed in patients following ticagrelor administration (Giannoni et al., 2016; Giannoni et al., 2021). Giannoni et al. tested chemoreflex response in the group of patients with such a complications and found higher ventilatory response to hypercapnia (central chemosensitivity) compared to patients without these side effects (Giannoni et al., 2021). Similarly, higher central chemosensitivity was found in the whole group of patients treated with ticagrelor compared to patients on prasugrel (Giannoni et al., 2021). The study aiming to reveal the influence of ticagrelor on PChS is being currently conducted in our center.
Diuretics
The only diuretic drug with a confirmed influence on chemoreflex is acetazolamide. This carbonic anhydrase inhibitor leads to mild metabolic acidosis with a consequent: 1) ventilatory compensation (drop in CO2 arterial levels) and 2) increase in cerebral blood flow. Net effects of these changes are: desensitization of PChS and augmentation of central chemoreflex (the ventilatory response to carbon dioxide) (Adams and Johnson, 1990; Wagenaar et al., 1998; Vovk et al., 2000; Fontana et al., 2011). Moreover, it has been recently demonstrated that administration of acetazolamide diminishes periodic breathing and improves haemoglobin saturation in HF patients (Fontana et al., 2011; Javaheri et al., 2014). The effects of loop, thiazide and thiazide-like diuretics on peripheral chemoreceptors have not been studied.
Calcium Channel Blockers
The transient influx of calcium ions into glomus type I cells of CB is crucial for the release of neurotransmitters in hypoxic conditions (Shirahata and Fitzgerald, 1991). In an animal model, verapamil administration attenuated resting ventilation (PChT) and PChS in anesthetized rats but did not influence central chemosensitivity (Chapman, 1985). However, this was not confirmed in healthy humans by Long et al. (1989). Another study in healthy humans by Watt et al. revealed no changes in resting minute ventilatory in normoxic and hypoxic hypocapnic conditions following amlodipine vs placebo administration (Watt et al., 2000). These data suggest that calcium-blockers have no influence on PChS in healthy subjects, but further studies in patients with presumed high PCh activity have to be performed.
Digitalis Glycosides
Digitalis glycosides affect intracellular calcium level indirectly by inhibiting Na+/K+ ATPase leading to hypernatremia and enhanced calcium ions influx (Levi et al., 1994). Animal data revealed that intravenous infusion of oubain during normoxia causes a transient increase in discharge rate in the carotid sinus nerve innervating the cat’s CB (McQueen and Ribeiro, 1983). In the same experiment, oubain led also to increased hypoxic CB activity. In healthy humans, digoxin and cedilanid were found to increase PChS and did not affect hypercapnic response which suggest the influence only on PCh (Schobel et al., 1994; Janssen et al., 2010). Some indirect evidence may also indicate an influence of digoxine on PChT. Pagenelli et al. found that the administration of the drug abolishes hyperoxia induced decrease in HR and cardiac output in HF patients. These effects were linked with PChT inhibition by the authors; however, this interaction has to be verified in the future trials (Paganelli et al., 2001).
Anti-Inflamatory Medications and Anti-Oxidants
The modulatory role of proinflammatory cytokines on CB functionality was revealed in animal models (Iturriaga et al., 2022). Both local inflammation (within CB) and systemic inflammatory response are increasing PChT and PChS in rats (Iturriaga et al., 2022; Katayama et al., 2022). Ibuprofen, which is well-known cyclooxygenase 1 and 2 inhibitor suppresses proinflammatory cytokines production (Scheuren et al., 1998) and prevents chronic hypoxia aftereffects such as: 1)increased interleukin-1 and tumour necrosis factor alfa production within CB, 2) hypertension, 3) enhanced hypoxic ventilatory response (PChS) and carotid body discharges (PChT) (Liu et al., 2009; Del Rio et al., 2012).
Another mechanism enhancing CB functionality following IH is oxidative stress. In rats, ascorbic acid added to drinking water reduced the malondialdehyde and 3-nitrotyriosine plasma (oxidative stress markers) levels and prevented IH-induced augmentation of PChS (Del Rio et al., 2010).
The data regarding the influence of anti-inflammatory and antioxidants on PChS and PChT in humans is missing.
Discussion
Molecules with confirmed or suspected modulatory influence on PCh are listed in Table 1. However, despite the treatment the prevalence of elevated PCh functionality in sympathetically mediated diseases is still high - e.g. in HF patients PCh oversensitivity can be identified in more than every third patient despite guidelines-recommended treatment including beta-blockers and renin-angiotensin-aldosterone axis modulators (Niewinski et al., 2013). Thus, the development of the novel methods for chemoreflex modulation, preferably these reversible and titerable, is required. As it was presented above the complexity of PCh function, numerous, probably not completely discovered, pathophysiological pathways leading to PCh overfunctionality and numerous modulatory factors has to be considered when designing future research in the field. First of all, PChT rather than PChS or both should be aimed with the treatment to limit permanently increased SNA (Paton et al., 2013b; Paton et al., 2013a; 61). Secondly, the effects of PChT modulation can be found usually only in subjects with excessive PCh output (in healthy the input from PChT is neglectable), thus the subjects with presumed high PChT should be included in the trials. Thirdly, the pathomechanism that triggers PCh overactivity may differ across diseases and, therefore, potential treatments to reduce PChT and/or PChS are likely to be disease-specific (Schultz et al., 2007). Fourthly, vasodilatory agents will increase SNA via baroreceptors unload, hence PChT (with SNA dynamics) should be assessed after the restoration of baseline blood pressure or should be expressed as changes in minute ventilation (Ding et al., 2008). Fifthly, PChS or PChT are dynamic parameters, which change within particular individuals allowing adaptation to environmental or functional changes, e.g. during physical activity (Weil et al., 1972); since, the effects of novel PCh modulators should be tested both at rest and during physiological challenges relevant to patients’ daily life. Finally, the novel treatment techniques developed in animal models (medication-naïve subjects), may not work in human patients, because the mechanisms addressed may be already covered with prescribed drugs.
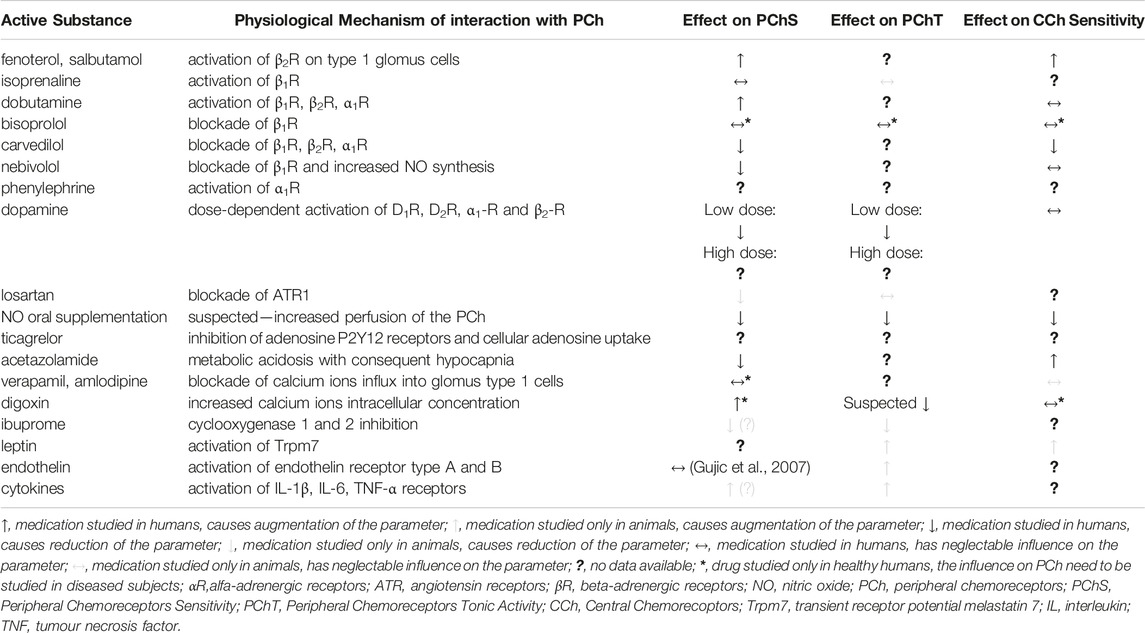
TABLE 1. Confirmed and potential (based on animal data) effect of medications on chemoreceptors functionality.
Author Contributions
All authors listed have made a substantial, direct, and intellectual contribution to the work and approved it for publication.
Funding
This research was financially supported from the National Science Center Poland (NCN) grant no 2017/27/N/NZ4/02863.
Conflict of Interest
The authors declare that the research was conducted in the absence of any commercial or financial relationships that could be construed as a potential conflict of interest.
Publisher’s Note
All claims expressed in this article are solely those of the authors and do not necessarily represent those of their affiliated organizations, or those of the publisher, the editors and the reviewers. Any product that may be evaluated in this article, or claim that may be made by its manufacturer, is not guaranteed or endorsed by the publisher.
References
Abdala, A. P., Mcbryde, F. D., Marina, N., Hendy, E. B., Engelman, Z. J., Fudim, M., et al. (2012). Hypertension Is Critically Dependent on the Carotid Body Input in the Spontaneously Hypertensive Rat. J. physiology 590, 4269–4277. doi:10.1113/jphysiol.2012.237800
Adams, J. M., and Johnson, N. L. (1990). Inhibiting Carbonic Anhydrase in Brain Tissue Increases the Respiratory Response to Rebreathing CO2. Brain Res. 519, 23–28. doi:10.1016/0006-8993(90)90056-h
Agarwal, A., Kim, I., and Carroll, J. L. (2011). Atp Inhibits Carotid Body Glomus Cell Hypoxia Response via P2y12 Receptors, Am. Thorac. Soc. A109. HYPOXIA. doi:10.1164/ajrccm-conference.2011.183.1_meetingabstracts.a2484
Agostoni, P., Contini, M., Magini, A., Apostolo, A., Cattadori, G., Bussotti, M., et al. (2006). Carvedilol Reduces Exercise-Induced Hyperventilation: A Benefit in Normoxia and a Problem with Hypoxia. Eur. J. heart Fail. 8, 729–735. doi:10.1016/j.ejheart.2006.02.001
Alzahrani, A. A., Cao, L. L., Aldossary, H. S., Nathanael, D., Fu, J., Ray, C. J., et al. (2021). β-Adrenoceptor Blockade Prevents Carotid Body Hyperactivity and Elevated Vascular Sympathetic Nerve Density Induced by Chronic Intermittent Hypoxia. Pflugers Arch. - Eur. J. Physiol. 473, 37–51. doi:10.1007/s00424-020-02492-0
Beloka, S., Gujic, M., Deboeck, G., Niset, G., Ciarka, A., Argacha, J.-F., et al. (2008). β-Adrenergic Blockade and Metabo-Chemoreflex Contributions to ExerciseCapacity. Med. Sci. Sports Exerc 40, 1932–1938. doi:10.1249/mss.0b013e31817fbe11
Bock, J., Limberg, J., Hughes, W., Kruse, N., Ueda, K.-I., and Casey, D. (2017a). The Impact of Nitrate Supplementation on the Peripheral Chemoreceptor Reflex to Hypoxia in Older Adults. FASEB J. 31, 867.1.
Bock, J. M., Ueda, K., Schneider, A. C., Hughes, W. E., Limberg, J. K., Bryan, N. S., et al. (2017b). Inorganic Nitrate Supplementation Attenuates Peripheral Chemoreflex Sensitivity but Does Not Improve Cardiovagal Baroreflex Sensitivity in Older Adults. Am. J. Physiology - Heart Circulatory Physiology. 314(1)
Böck, P. (1980). Adenine Nucleotides in the Carotid Body. Cell Tissue Res. 206, 279–290. doi:10.1007/BF00232772
Cattaneo, M., and Faioni, E. M. (2012). Why Does Ticagrelor Induce Dyspnea? Thromb. Haemost. 108, 1031–1036. doi:10.1160/TH12-08-0547
Chapman, R. W. (1985). Effect of Verapamil on Ventilation and Chemical Control of Breathing in Anesthetized Rats. Can. J. Physiol. Pharmacol. 63, 1608–1611. doi:10.1139/y85-265
Chen, J., He, L., Dinger, B., Stensaas, L., and Fidone, S. (2002). Role of Endothelin and Endothelin A-type Receptor in Adaptation of the Carotid Body to Chronic Hypoxia. Am. J. Physiology-Lung Cell. Mol. Physiology 282, L1314–L1323. doi:10.1152/ajplung.00454.2001
Contini, M., Apostolo, A., Cattadori, G., Paolillo, S., Iorio, A., Bertella, E., et al. (2013). Multiparametric Comparison of CARvedilol, vs. NEbivolol, vs. BIsoprolol in Moderate Heart Failure: the CARNEBI Trial. Int. J. Cardiol. 168, 2134–2140. doi:10.1016/j.ijcard.2013.01.277
Del Rio, R., Marcus, N. J., and Schultz, H. D. (2013). Carotid Chemoreceptor Ablation Improves Survival in Heart Failure. J. Am. Coll. Cardiol. 62, 2422–2430. doi:10.1016/j.jacc.2013.07.079
Del Rio, R., Moya, E. A., and Iturriaga, R. (2010). Carotid Body and Cardiorespiratory Alterations in Intermittent Hypoxia: the Oxidative Link. Eur. Respir. J. 36, 143–150. doi:10.1183/09031936.00158109
Del Rio, R., Moya, E. A., Parga, M. J., Madrid, C., and Iturriaga, R. (2012). Carotid Body Inflammation and Cardiorespiratory Alterations in Intermittent Hypoxia. Eur. Respir. J. 39, 1492–1500. doi:10.1183/09031936.00141511
Ding, Y., Li, Y.-L., and Schultz, H. D. (2008). Downregulation of Carbon Monoxide as Well as Nitric Oxide Contributes to Peripheral Chemoreflex Hypersensitivity in Heart Failure Rabbits. J. Appl. physiology 105, 14–23. doi:10.1152/japplphysiol.01345.2007
Ding, Y., Li, Y.-L., and Schultz, H. D. (2011). Role of Blood Flow in Carotid Body Chemoreflex Function in Heart Failure. J. Physiology 589, 245–258. doi:10.1113/jphysiol.2010.200584
Fidone, S., Gonzalez, C., and Yoshizaki, K. (1982). Effects of Low Oxygen on the Release of Dopamine from the Rabbit Carotid Bodyin Vitro. J. Physiology 333, 93–110. doi:10.1113/jphysiol.1982.sp014441
Folgering, H., Ponte, J., and Sadig, T. (1982). Adrenergic Mechanisms and Chemoreception in the Carotid Body of the Cat and Rabbit. J. physiology 325, 1–21. doi:10.1113/jphysiol.1982.sp014131
Fontana, M., Emdin, M., Giannoni, A., Iudice, G., Baruah, R., and Passino, C. (2011). Effect of Acetazolamide on Chemosensitivity, Cheyne-Stokes Respiration, and Response to Effort in Patients with Heart Failure. Am. J. Cardiol. 107, 1675–1680. doi:10.1016/j.amjcard.2011.01.060
Foster, G. E., Hanly, P. J., Ahmed, S. B., Beaudin, A. E., Pialoux, V., and Poulin, M. J. (2010). Intermittent Hypoxia Increases Arterial Blood Pressure in Humans through a Renin-Angiotensin System-dependent Mechanism. Hypertension 56, 369–377. doi:10.1161/hypertensionaha.110.152108
Fung, M. L. (2014). The Role of Local Renin-Angiotensin System in Arterial Chemoreceptors in Sleep-Breathing Disorders. Front. Physiol. 5, 336. doi:10.3389/fphys.2014.00336
Giannoni, A., Borrelli, C., Gentile, F., Mirizzi, G., Coceani, M., Paradossi, U., et al. (2021). Central Apnoeas and Ticagrelor-Related Dyspnoea in Patients with Acute Coronary Syndrome. Eur. Heart Journal-Cardiovascular Pharmacother. 7, 180–188. doi:10.1093/ehjcvp/pvaa089
Giannoni, A., Emdin, M., and Passino, C. (2016). Cheyne-Stokes Respiration, Chemoreflex, and Ticagrelor-Related Dyspnea. N. Engl. J. Med. 375, 1004–1006. doi:10.1056/nejmc1601662
González, C., Almaraz, L., Obeso, A., and Rigual, R. (1992). Oxygen and Acid Chemoreception in the Carotid Body Chemoreceptors. Trends Neurosci. 15, 146–153. doi:10.1016/0166-2236(92)90357-e
González, C., López-López, J., Obeso, A., Pérez-García, M. T., and Rocher, A. (1995). Cellular Mechanisms of Oxygen Chemoreception in the Carotid Body. Respir. Physiol. 102, 137–147. doi:10.1016/0034-5687(95)00069-0
Gujic, M., Houssière, A., Xhaët, O., Argacha, J.-F., Denewet, N., Noseda, A., et al. (2007). Does Endothelin Play a Role in Chemoreception during Acute Hypoxia in Normal Men? Chest 131, 1467–1472. doi:10.1378/chest.06-1775
Heistad, D. D., Wheeler, R. C., Mark, A. L., Schmid, P. G., and Abboud, F. M. (1972). Effects of Adrenergic Stimulation on Ventilation in Man. J. Clin. Invest. 51, 1469–1475. doi:10.1172/jci106943
Hudgel, D. W., Kressin, N. A., Nielsen, A. M., and Bisgard, G. E. (1986). Role of Beta Adrenergic Receptors in Carotid Body Function of the Goat. Respir. Physiol. 64, 203–211. doi:10.1016/0034-5687(86)90042-3
Iturriaga, R., and Alcayaga, J. (2004). Neurotransmission in the Carotid Body: Transmitters and Modulators between Glomus Cells and Petrosal Ganglion Nerve Terminals. Brain Res. Rev. 47, 46–53. doi:10.1016/j.brainresrev.2004.05.007
Iturriaga, R., Del Rio, R., and Alcayaga, J. (2022). Carotid Body Inflammation: Role in Hypoxia and in the Anti-inflammatory Reflex. Physiology 37, 128–140. doi:10.1152/physiol.00031.2021
Iturriaga, R., Del Rio, R., Idiaquez, J., and Somers, V. K. (2016). Carotid Body Chemoreceptors, Sympathetic Neural Activation, and Cardiometabolic Disease. Biol. Res. 49, 13. doi:10.1186/s40659-016-0073-8
Janssen, C., Lheureux, O., Beloka, S., Deboeck, G., Adamopoulos, D., Naeije, R., et al. (2010). Digoxin Increases Peripheral Chemosensitivity and the Ventilatory Response to Exercise in Normal Subjects. Clin. Exp. Pharmacol. Physiol. 37, 303–308. doi:10.1111/j.1440-1681.2009.05287.x
Javaheri, S., Sands, S. A., and Edwards, B. A. (2014). Acetazolamide Attenuates Hunter-Cheyne-Stokes Breathing but Augments the Hypercapnic Ventilatory Response in Patients with Heart Failure. Ann. ATS 11, 80–86. doi:10.1513/annalsats.201306-201oc
Kara, T., Narkiewicz, K., and Somers, V. K. (2003). Chemoreflexes - Physiology and Clinical Implications. Acta physiol. Scand. 177, 377–384. doi:10.1046/j.1365-201x.2003.01083.x
Katayama, P. L., Leirão, I. P., Kanashiro, A., Luiz, J. P. M., Cunha, F. Q., Navegantes, L. C. C., et al. (2022). The Carotid Body Detects Circulating Tumor Necrosis Factor-Alpha to Activate a Sympathetic Anti-inflammatory Reflex. Brain, Behav. Immun. 102, 370–386. doi:10.1016/j.bbi.2022.03.014
Kato, K., Yokoyama, T., Yamaguchi-Yamada, M., and Yamamoto, Y. (2013). Short-term Hypoxia Transiently Increases Dopamine β-Hydroxylase Immunoreactivity in Glomus Cells of the Rat Carotid Body. J. Histochem Cytochem. 61, 55–62. doi:10.1369/0022155412464639
Kou, Y. R., Ernsberger, P., Cragg, P. A., Cherniack, N. S., and Prabhakar, N. R. (1991). Role of α2-adrenergic Receptors in the Carotid Body Response to Isocapnic Hypoxia. Respir. Physiol. 83, 353–364. doi:10.1016/0034-5687(91)90054-m
Lam, S.-Y., Liu, Y., Ng, K.-M., Liong, E. C., Tipoe, G. L., Leung, P. S., et al. (2014). Upregulation of a Local Renin-Angiotensin System in the Rat Carotid Body during Chronic Intermittent Hypoxia. Exp. Physiol. 99, 220–231. doi:10.1113/expphysiol.2013.074591
Lam, S. Y., and Leung, P. S. (2002). A Locally Generated Angiotensin System in Rat Carotid Body. Regul. Pept. 107, 97–103. doi:10.1016/s0167-0115(02)00068-x
Lehmann, J., Briley, M., and Langer, S. Z. (1983). Characterization of Dopamine Autoreceptor and [3H]spiperone Binding Sites In Vitro with Classical and Novel Dopamine Receptor Agonists. Eur. J. Pharmacol. 88, 11–26. doi:10.1016/0014-2999(83)90387-4
Leitch, A. G., Clancy, L. J., Costello, J. F., and Flenley, D. C. (1976). Effect of Intravenous Infusion of Salbutamol on Ventilatory Response to Carbon Dioxide and Hypoxia and on Heart Rate and Plasma Potassium in Normal Men. Bmj 1, 365–367. doi:10.1136/bmj.1.6006.365
Leonard, E. M., Salman, S., and Nurse, C. A. (2018). Sensory Processing and Integration at the Carotid Body Tripartite Synapse: Neurotransmitter Functions and Effects of Chronic Hypoxia. Front. Physiol. 9, 225. doi:10.3389/fphys.2018.00225
Leung, P. S., Fung, M. L., and Tam, M. S. C. (2003). Renin-angiotensin System in the Carotid Body. Int. J. Biochem. Cell Biol. 35, 847–854. doi:10.1016/s1357-2725(02)00180-2
Levi, A. J., Boyett, M. R., and Lee, C. O. (1994). The Cellular Actions of Digitalis Glycosides on the Heart. Prog. biophysics Mol. Biol. 62, 1–54. doi:10.1016/0079-6107(94)90005-1
Li, Y.-L., Li, Y.-F., Liu, D., Cornish, K. G., Patel, K. P., Zucker, I. H., et al. (2005). Gene Transfer of Neuronal Nitric Oxide Synthase to Carotid Body Reverses Enhanced Chemoreceptor Function in Heart Failure Rabbits. Circulation Res. 97, 260–267. doi:10.1161/01.res.0000175722.21555.55
Li, Y., Gao, L., Zucker, I., and Schultz, H. (2007). NADPH Oxidase-Derived Superoxide Anion Mediates Angiotensin II-Enhanced Carotid Body Chemoreceptor Sensitivity in Heart Failure Rabbits. Cardiovasc. Res. 75, 546–554. doi:10.1016/j.cardiores.2007.04.006
Li, Y., Xia, X., Zheng, H., Gao, L., Li, Y., Liu, D., et al. (2006). Angiotensin II Enhances Carotid Body Chemoreflex Control of Sympathetic Outflow in Chronic Heart Failure Rabbits. Cardiovasc. Res. 71, 129–138. doi:10.1016/j.cardiores.2006.03.017
Limberg, J. K., Johnson, B. D., Holbein, W. W., Ranadive, S. M., Mozer, M. T., and Joyner, M. J. (2016). Interindividual Variability in the Dose-specific Effect of Dopamine on Carotid Chemoreceptor Sensitivity to Hypoxia. J. Appl. Physiology 120, 138–147. doi:10.1152/japplphysiol.00723.2015
Liu, X., He, L., Stensaas, L., Dinger, B., and Fidone, S. (2009). Adaptation to Chronic Hypoxia Involves Immune Cell Invasion and Increased Expression of Inflammatory Cytokines in Rat Carotid Body. Am. J. Physiology-Lung Cell. Mol. Physiology 296, L158–L166. doi:10.1152/ajplung.90383.2008
Long, G. R., Filuk, R., Balakumar, M., Easton, P. A., and Anthonisen, N. R. (1989). Ventilatory Response to Sustained Hypoxia: Effect of Methysergide and Verapamil. Respir. Physiol. 75, 173–182. doi:10.1016/0034-5687(89)90061-3
Mcbryde, F. D., Abdala, A. P., Hendy, E. B., Pijacka, W., Marvar, P., Moraes, D. J., et al. (2013). The Carotid Body as a Putative Therapeutic Target for the Treatment of Neurogenic Hypertension. Nat. Commun. 4, 2395. doi:10.1038/ncomms3395
Mcqueen, D. S., and Ribeiro, J. A. (1983). Effects of Ouabain on Carotid Body Chemoreceptor Activity in the Cat. J. Physiology 335, 221–235. doi:10.1113/jphysiol.1983.sp014530
Mir, A. K., Pallot, D. J., and Nahorski, S. R. (1983). Biogenic Amine-Stimulated Cyclic Adenosine-3',5'-Monophosphate Formation in the Rat Carotid Body. J. Neurochem. 41, 663–669. doi:10.1111/j.1471-4159.1983.tb04792.x
Morgan, B. J., Teodorescu, M., Pegelow, D. F., Jackson, E. R., Schneider, D. L., Plante, D. T., et al. (2018). Effects of Losartan and Allopurinol on Cardiorespiratory Regulation in Obstructive Sleep Apnoea. Exp. Physiol. 103, 941–955. doi:10.1113/ep087006
Muccino, D. R., Morice, A. H., Birring, S. S., Dicpinigaitis, P. V., Pavord, I. D., Assaid, C., et al. (2020). Design and Rationale of Two Phase 3 Randomised Controlled Trials (COUGH-1 and COUGH-2) of Gefapixant, a P2X3 Receptor Antagonist, in Refractory or Unexplained Chronic Cough. ERJ Open Res. 6. doi:10.1183/23120541.00284-2020
Narkiewicz, K., Ratcliffe, L. E. K., Hart, E. C., Briant, L. J. B., Chrostowska, M., Wolf, J., et al. (2016). Unilateral Carotid Body Resection in Resistant Hypertension. JACC Basic Transl. Sci. 1, 313–324. doi:10.1016/j.jacbts.2016.06.004
Niewinski, P., Engelman, Z. J., Fudim, M., Tubek, S., Paleczny, B., Jankowska, E. A., et al. (2013). Clinical Predictors and Hemodynamic Consequences of Elevated Peripheral Chemosensitivity in Optimally Treated Men with Chronic Systolic Heart Failure. J. cardiac Fail. 19, 408–415. doi:10.1016/j.cardfail.2013.03.013
Niewinski, P., Janczak, D., Rucinski, A., Tubek, S., Engelman, Z. J., Piesiak, P., et al. (2017). Carotid Body Resection for Sympathetic Modulation in Systolic Heart Failure: Results from First-In-Man Study. Eur. J. Heart Fail 19, 391–400. doi:10.1002/ejhf.641
Niewinski, P., Tubek, S., Banasiak, W., Paton, J. F. R., and Ponikowski, P. (2014). Consequences of Peripheral Chemoreflex Inhibition with Low-Dose Dopamine in Humans. J. physiology 592, 1295–1308. doi:10.1113/jphysiol.2013.266858
Niewinski, P., Tubek, S., Paton, J. F. R., Banasiak, W., and Ponikowski, P. (2021). Oxygenation Pattern and Compensatory Responses to Hypoxia and Hypercapnia Following Bilateral Carotid Body Resection in Humans. J. Physiol. 599, 2323–2340. doi:10.1113/jp281319
Notay, K., Incognito, A. V., and Millar, P. J. (2017). Acute Beetroot Juice Supplementation on Sympathetic Nerve Activity: a Randomized, Double-Blind, Placebo-Controlled Proof-Of-Concept Study. Am. J. Physiology-Heart Circulatory Physiology 313, H59–H65. doi:10.1152/ajpheart.00163.2017
O'Regan, R. G., and Majcherczyk, S. (1982). Role of Peripheral Chemoreceptors and Central Chemosensitivity in the Regulation of Respiration and Circulation. J. Exp. Biol. 100, 23–40. doi:10.1242/jeb.100.1.23
Overgaard, C. B., and Dzavik, V. (2008). Inotropes and Vasopressors. Circulation 118, 1047–1056. doi:10.1161/circulationaha.107.728840
Paganelli, F., Maixent, J. M., Gélisse, R., Barnay, P., Dodero, F., Francheschi, F., et al. (2001). Effects of Digoxin on Chemoreflex in Patients with Chronic Heart Failure. Cell Mol. Biol. (Noisy-le-grand) 47, 335–340.
Pathak, A., Velez-Roa, S., Xhaët, O., Najem, B., and Van De Borne, P. (2006). Dose-dependent Effect of Dobutamine on Chemoreflex Activity in Healthy Volunteers. Br. J. Clin. Pharmacol. 62, 272–279. doi:10.1111/j.1365-2125.2006.02657.x
Paton, J. F. R., Ratcliffe, L., Hering, D., Wolf, J., Sobotka, P. A., and Narkiewicz, K. (2013a). Revelations about Carotid Body Function through its Pathological Role in Resistant Hypertension. Curr. Hypertens. Rep. 15, 273–280. doi:10.1007/s11906-013-0366-z
Paton, J. F., Sobotka, P. A., Fudim, M., Engelman, Z. J., Hart, E. C., Mcbryde, F. D., et al. (2013b). The Carotid Body as a Therapeutic Target for the Treatment of Sympathetically Mediated Diseases. Hypertension 61. doi:10.1161/hypertensionaha.111.00064
Pauza, A. G., Thakkar, P., Tasic, T., Felippe, I., Bishop, P., Greenwood, M. P., et al. (2022). GLP1R Attenuates Sympathetic Response to High Glucose via Carotid Body Inhibition. Circ. Res. 130, 694–707. doi:10.1161/circresaha.121.319874
Pérez-García, M., Gómez-Niño, A., Almaraz, L., and González, C. (1993). Neurotransmitters and Second Messenger Systems in the Carotid Body. Neurobiol. Cell Physiology Chemorecept. Springer. doi:10.1007/978-1-4615-2966-8_39
Pijacka, W., Moraes, D. J. A., Ratcliffe, L. E. K., Nightingale, A. K., Hart, E. C., Da Silva, M. P., et al. (2016). Purinergic Receptors in the Carotid Body as a New Drug Target for Controlling Hypertension. Nat. Med. 22, 1151–1159. doi:10.1038/nm.4173
Prabhakar, N. R. (1994). Neurotransmitters in the Carotid Body. Arter. Chemorecept., 57–69. doi:10.1007/978-1-4615-2572-1_6
Scheuren, N., Bang, H., Münster, T., Brune, K., and Pahl, A. (1998). Modulation of Transcription Factor NF-Κb by Enantiomers of the Nonsteroidal Drug Ibuprofen. Br. J. Pharmacol. 123, 645–652. doi:10.1038/sj.bjp.0701652
Schobel, H. P., Ferguson, D. W., Clary, M. P., and Somers, V. K. (1994). Differential Effects of Digitalis on Chemoreflex Responses in Humans. Hypertension 23, 302–307. doi:10.1161/01.hyp.23.3.302
Schultz, H. D. (2011). Angiotensin and Carotid Body Chemoreception in Heart Failure. Curr. Opin. Pharmacol. 11, 144–149. doi:10.1016/j.coph.2010.12.004
Schultz, H. D., Li, Y. L., and Ding, Y. (2007). Arterial Chemoreceptors and Sympathetic Nerve Activity. Hypertension 50, 6–13. doi:10.1161/hypertensionaha.106.076083
Schultz, H. D., Marcus, N. J., and Del Rio, R. (2013). Role of the Carotid Body in the Pathophysiology of Heart Failure. Curr. Hypertens. Rep. 15, 356–362. doi:10.1007/s11906-013-0368-x
Shin, M.-K., Eraso, C. C., Mu, Y.-P., Gu, C., Yeung, B. H. Y., Kim, L. J., et al. (2019). Leptin Induces Hypertension Acting on Transient Receptor Potential Melastatin 7 Channel in the Carotid Body. Circ. Res. 125, 989–1002. doi:10.1161/circresaha.119.315338
Shirahata, M., and Fitzgerald, R. S. (1991). Dependency of Hypoxic Chemotransduction in Cat Carotid Body on Voltage-Gated Calcium Channels. J. Appl. physiology 71, 1062–1069. doi:10.1152/jappl.1991.71.3.1062
Siński, M., Lewandowski, J., Przybylski, J., Bidiuk, J., Abramczyk, P., Ciarka, A., et al. (2012). Tonic Activity of Carotid Body Chemoreceptors Contributes to the Increased Sympathetic Drive in Essential Hypertension. Hypertens. Res. 35, 487–491. doi:10.1038/hr.2011.209
Smith, C., and Teitler, M. (1999). Beta-blocker Selectivity at Cloned Human Beta1-And Beta2-Adrenergic Receptors. Cardiovasc. drugs Ther. 13, 123–126. doi:10.1023/a:1007784109255
Solaiman, A. Z., Feehan, R. P., Chabitnoy, A. M., Leuenberger, U. A., and Monahan, K. D. (2014). Ventilatory Responses to Chemoreflex Stimulation Are Not Enhanced by Angiotensin II in Healthy Humans. Aut. Neurosci. 183, 72–79. doi:10.1016/j.autneu.2014.01.010
Tubek, S., Niewinski, P., Paleczny, B., Langner-Hetmanczuk, A., Banasiak, W., and Ponikowski, P. (2021). Acute Hyperoxia Reveals Tonic Influence of Peripheral Chemoreceptors on Systemic Vascular Resistance in Heart Failure Patients. Sci. Rep. 11, 20823–20829. doi:10.1038/s41598-021-99159-2
Tubek, S., Niewiński, P., Paleczny, B., Langner, A., Banasiak, W., and Ponikowski, P. (2018). Human Carotid Bodies as a Therapeutic Target: New Insights from Clinician Perspective. Kardiologia Pol. Pol. Heart J. 76(10)
Tubek, S., Niewinski, P., Reczuch, K., Janczak, D., Rucinski, A., Paleczny, B., et al. (2016). Effects of Selective Carotid Body Stimulation with Adenosine in Conscious Humans. J. Physiol. 594, 6225–6240. doi:10.1113/jp272109
Van De Borne, P., Oren, R., and Somers, V. K. (1998). Dopamine Depresses Minute Ventilation in Patients with Heart Failure. Circulation 98, 126–131. doi:10.1161/01.cir.98.2.126
Velez-Roa, S., Kojonazarov, B., Ciarka, A., Godart, P., Naeije, R., Somers, V. K., et al. (2003). Dobutamine Potentiates Arterial Chemoreflex Sensitivity in Healthy Normal Humans. Am. J. Physiology-Heart Circulatory Physiology 285, H1356–H1361. doi:10.1152/ajpheart.01126.2002
Vovk, A., Duffin, J., Kowalchuk, J. M., Paterson, D. H., and Cunningham, D. A. (2000). Changes in Chemoreflex Characteristics Following Acute Carbonic Anhydrase Inhibition in Humans a Rest. Exp. Physiol. 85, 847–856. doi:10.1111/j.1469-445x.2000.02016.x
Wagenaar, M., Teppema, L., Berkenbosch, A., Olievier, C., and Folgering, H. (1998). Effect of Low-Dose Acetazolamide on the Ventilatory CO2 Response during Hypoxia in the Anaesthetized Cat. Eur. Respir. J. 12, 1271–1277. doi:10.1183/09031936.98.12061271
Wang, Z.-Z., Bredt, D. S., Fidone, S. J., and Stensaas, L. J. (1993). Neurons Synthesizing Nitric Oxide Innervate the Mammalian Carotid Body. J. Comp. Neurol. 336, 419–432. doi:10.1002/cne.903360308
Watt, M., Peacock, A. j., Newell, J., Mcdonagh, T., and Grant, S. (2000). The Effect of Amlodipine on Respiratory and Pulmonary Vascular Responses to Hypoxia in Mountaineers. Eur. Respir. J. 15, 459–463. doi:10.1034/j.1399-3003.2000.15.06.x
Weil, J. V., Byrne-Quinn, E., Sodal, I. E., Kline, J. S., Mccullough, R. E., and Filley, G. F. (1972). Augmentation of Chemosensitivity during Mild Exercise in Normal Man. J. Appl. Physiology 33, 813–819. doi:10.1152/jappl.1972.33.6.813
Welsh, M. J., Heistad, D. D., and Abboud, F. M. (1978). Depression of Ventilation by Dopamine in Man. J. Clin. Invest. 61, 708–713. doi:10.1172/jci108983
Xing, D. T., May, C. N., Booth, L. C., and Ramchandra, R. (2014). Tonic Arterial Chemoreceptor Activity Contributes to Cardiac Sympathetic Activation in Mild Ovine Heart Failure. Exp. Physiol. 99, 1031–1041. doi:10.1113/expphysiol.2014.079491
Yamamoto, Y., König, P., Henrich, M., Dedio, J., and Kummer, W. (2006). Hypoxia Induces Production of Nitric Oxide and Reactive Oxygen Species in Glomus Cells of Rat Carotid Body. Cell Tissue Res. 325, 3–11. doi:10.1007/s00441-006-0178-4
Ye, J.-S., Tipoe, G. L., Fung, P. C., and Fung, M.-L. (2002a). Augmentation of Hypoxia-Induced Nitric Oxide Generation in the Rat Carotid Body Adapted to Chronic Hypoxia: an Involvement of Constitutive and Inducible Nitric Oxide Synthases. Pflügers Arch. - Eur. J. Physiol. 444, 178–185. doi:10.1007/s00424-002-0785-1
Ye, S., Zhong, H., Duong, V. N., and Campese, V. M. (2002b). Losartan Reduces Central and Peripheral Sympathetic Nerve Activity in a Rat Model of Neurogenic Hypertension. Hypertension 39, 1101–1106. doi:10.1161/01.hyp.0000018590.26853.c7
Yoshiike, Y., Suzuki, S., Watanuki, Y., and Okubo, T. (1995). Effects of Fenoterol on Ventilatory Responses to Hypoxia and Hypercapnia in Normal Subjects. Thorax 50, 139–142. doi:10.1136/thx.50.2.139
Yuan, F., Wang, H., Feng, J., Wei, Z., Yu, H., Zhang, X., et al. (2018). Leptin Signaling in the Carotid Body Regulates a Hypoxic Ventilatory Response through Altering TASK Channel Expression. Front. Physiol. 9, 249. doi:10.3389/fphys.2018.00249
Keywords: chemoreflex, peripheral chemoreceptors, chemoreceptors sensitivity, chemoreceptors tonicity, pharmacotherapy, medications
Citation: Langner-Hetmańczuk A, Tubek S, Niewiński P and Ponikowski P (2022) The Role of Pharmacological Treatment in the Chemoreflex Modulation. Front. Physiol. 13:912616. doi: 10.3389/fphys.2022.912616
Received: 04 April 2022; Accepted: 19 May 2022;
Published: 14 June 2022.
Edited by:
Eduardo Colombari, Universidade Estadual Paulista, BrazilReviewed by:
Bruno Moreira Silva, Federal University of São Paulo, BrazilPedro L. Katayama, Boston Children’s Hospital and Harvard Medical School, United States
Copyright © 2022 Langner-Hetmańczuk, Tubek, Niewiński and Ponikowski. This is an open-access article distributed under the terms of the Creative Commons Attribution License (CC BY). The use, distribution or reproduction in other forums is permitted, provided the original author(s) and the copyright owner(s) are credited and that the original publication in this journal is cited, in accordance with accepted academic practice. No use, distribution or reproduction is permitted which does not comply with these terms.
*Correspondence: Stanisław Tubek, stanislaw.tubek@umw.edu.pl
†These authors have contributed equally to this work and share first authorship