- 1Department of Biology, Faculty of Science, Burapha University, Chon Buri, Thailand
- 2Wildlife Reproductive Innovation Center, Research Department, Bureau of Conservation and Research, Zoological Park Organization of Thailand Under the Royal Patronage of H.M. the King, Bangkok, Thailand
- 3Department of Anatomy, Faculty of Science, Mahidol University, Bangkok, Thailand
The avian embryos growing outside the natural eggshell (ex ovo) were observed since the early 19th century, and since then chick embryonic structures have revealed reaching an in-depth view of external and internal anatomy, enabling us to understand conserved vertebrate development. However, the internal environment within an eggshell (in ovo) would still be the ideal place to perform various experiments to understand the nature of avian development and to apply other biotechnology techniques. With the advent of genetic manipulation and cell culture techniques, avian embryonic parts were dissected for explant culture to eventually generate expandable cell lines (in vitro cell culture). The expansion of embryonic cells allowed us to unravel the transcriptional network for understanding pluripotency and differentiation mechanism in the embryos and in combination with stem cell technology facilitated the applications of avian culture to the next levels in transgenesis and wildlife conservation. In this review, we provide a panoramic view of the relationship among different cultivation platforms from in ovo studies to ex ovo as well as in vitro culture of cell lines with recent advances in the stem cell fields.
Introduction
In mammals, it is infeasible to observe the development of embryos outside the womb from fertilization to birth. Possibly, aves as a mammalian counterpart provide us an excellent model to be able to observe such a thing. Their advantages are low-cost without the need of feeding the embryos, ease of handling the eggs, and easy visualization of the embryonic development (Wolpert, 2004; Stern, 2005). Several studies have observed morphological changes in the development of the embryo within the eggshell by windowing or injecting compounds into the egg to observe changes in hatchability or physiological response in in ovo studies, or by removing the embryos from natural eggshells to new surrogate eggshells in semi-shell-less studies; however, there is a limitation in each method (Silver, 1960; Fisher and Schoenwolf, 1983; Andacht et al., 2004). Alternatively, completely removing embryos from their natural eggshells to various types of recently developed artificial vessels for further observation of developmental changes and genetic manipulation is called ex ovo studies. To provide in-depth studies into avian embryogenesis, parts of embryos were taken to derive cell lines in in vitro culture including embryonic stem cells (ESCs) and primordial germ cells (PGCs), and become an essential tool to understand pluripotency network regulating early development (van de Lavoir et al., 2006; Choi et al., 2010; Aubel and Pain, 2013; Whyte et al., 2015; Altgilbers et al., 2021). In vitro culture with genetic manipulation also enables us to study the gain/loss of functions and perform cellular reprogramming to produce induced pluripotent stem cells (iPSCs) capable of differentiation into all cell types, similar to ESCs (Dai et al., 2014; Katayama et al., 2018). Thus, in this review, we highlight the recent development of techniques used to study avian embryos in both in ovo and ex ovo cultivation as well as environmental parameters affecting hatchability and also the roles of in vitro culture to understand avian early development; in particular, pluripotency and germline-competent stem cells are also discussed.
In Ovo Cultivation: Essential Tool for Avian Development Insight
The avian embryo developed within a calcium carbonate-containing eggshell to provide protection and to be part of normal development. This enclosing environment of the eggshell provides sufficient nutrients from the yolk and osmotic pressure from albumen to generate a new live young chick, unlike receiving continuing support within the mother’s body in eutherian mammals. The isolated and complete system of avian eggs to progress into full development without further needs of materials from the mother, and high availability with low cost in poultry markets enables us to easily study the development of avian species, in particular the domestic chick (Gallus gallus domesticus). In recent years, studies have shown the great advantages of using developing chicken eggs in biotechnology including in ovo delivery of biological supplements and vaccination via amniotic inoculation or embryo body inoculation (reviewed in Saeed et al., 2019). In ovo feeding also plays an important role to improve the health of gastrointestinal tracts and immunity, increasing hatchability and resistance to pathogens (reviewed in Das et al., 2021). From day 4 to 5 post laid egg, the chorioallantoic membrane (CAM), a vascularized membrane responsible for gas exchanges that attaches directly to the eggshell, develops and this in ovo CAM structure provides a great platform to study diverse fields (reviewed in Nowak-Sliwinska et al., 2014) including tissue engineering [e.g., biomaterial and biosensors (Borges et al., 2003; Valdes et al., 2003; Azzarello et al., 2007)], testing of various compounds in angiogenesis (Nowak-Sliwinska et al., 2014), drug screening, and tumor growth treatment (Dupertuis et al., 2015).
To address the mechanism of development in avian embryos, chick embryos in in ovo cultivation have been used to understand the changes in embryonic morphology over time through the open windowed eggshell (windowing) method (Speksnijder and Ivarie, 2000; Andacht et al., 2004; Korn and Cramer, 2007), Figure 1. The key question in development is to understand pluripotency networks orchestrating early development and how these networks are conserved across species, in particular mammalian versus avian models. The timeframe to study early events in pluripotency acquisition [indicated by the presence of Pou5f3 (PouV), Sox2, and Nanog] in avian species is limited due to the onset of development that has already occurred since intrauterine development (Han et al., 2018) requires the sacrifice of hens. At the freshly laid egg stage, pluripotency has been shown to differ between finch and chick embryos, as the finch blastoderm in the laid egg (EGK V–VIII) expresses more naive-like state genes similar to naïve mouse embryonic stem cells (mESCs) while the chick blastoderm at the laid egg (EGK IX–XI) has primed bias similar to the mouse epiblast stem cells (EpiSC). This suggests that the pluripotency mechanism in different avian species is diverse at the early stage of freshly laid eggs and cannot be relied only on a chick model. A recent study of in ovo interspecies chimerism also shows the striking result to highlight the pluripotent state at equivalent points between human versus chick at an early embryonic development in that the EGK X blastodermal cells matched well with naïve human pluripotent stem cells (hPSCs), while the primed hPSCs can incorporate into the gastrulating epiblasts of chick embryos (Akhlaghpour et al., 2021) (Figure 1). In addition, CAM is used to understand pluripotency properties in an interspecies manner. Traditionally, the mouse model has been used as a platform for tumor formation from ESCs or induced pluripotent stem cells (iPSCs) established from various species of mammals to ensure the true nature of cells possessing “pluripotency”; the method is called teratoma assay. To replace mouse as an animal model for teratoma assay, CAM in the in ovo cultivation was used as a platform for seeding human iPSCs, which can grow on CAM and form a three-germ layer containing tumors within 9 days at 37°C (Weber et al., 2021). Taken together, in ovo study is still an essential tool for an ideal environment to unravel the developmental state and can be used to understand mammalian development mechanisms to reduce the use of animals, in particular mice, in the 3R model.
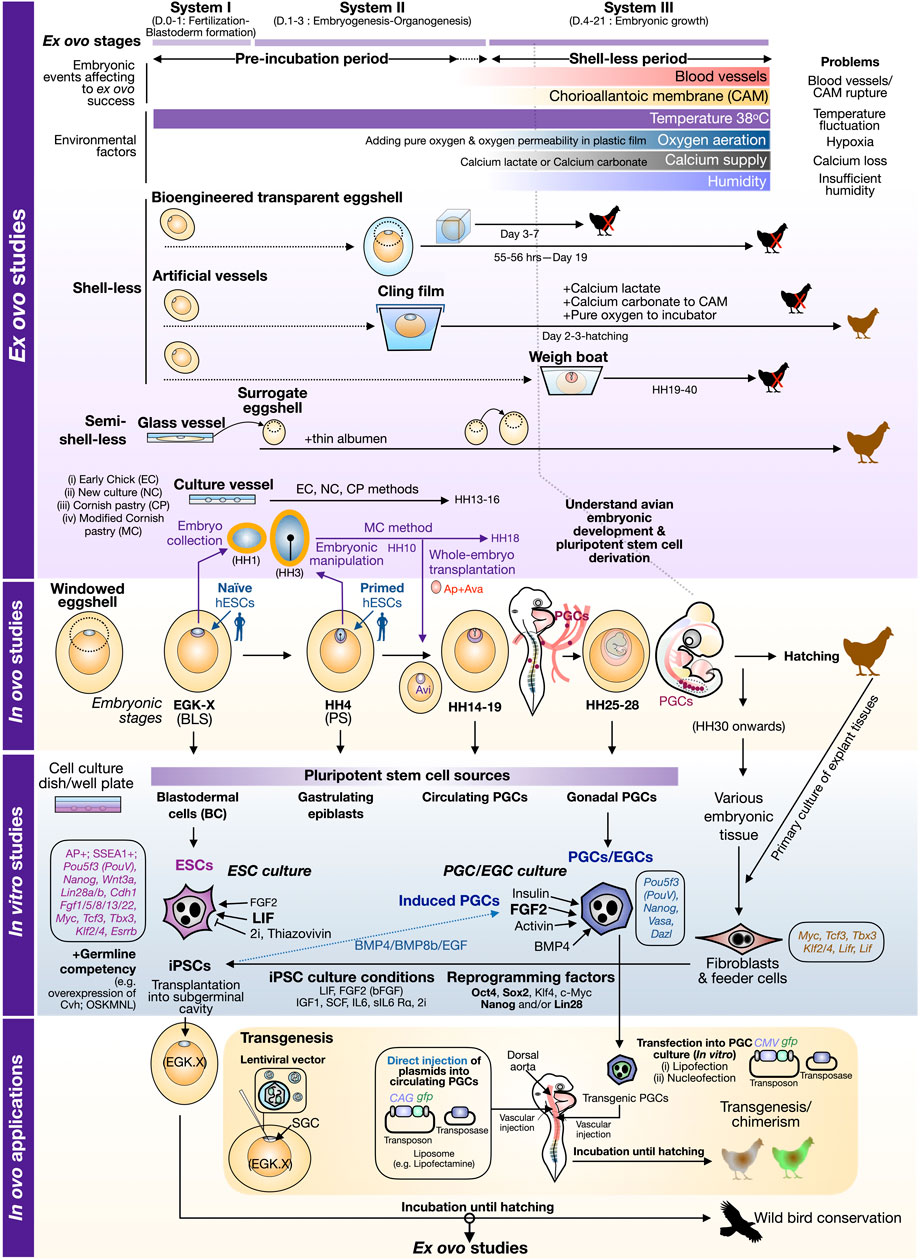
FIGURE 1. Perspective view of avian embryonic culture with ex ovo–in ovo cultivation and in vitro cell culture. In the schematic illustration of ex ovo studies, avian embryos at early stages can be collected into culture vessels (e.g., Petri dish) and cultured under chemically defined solutions (as listed in Table 1). In “shell-less” culture, experiments aimed to nourish embryos without natural eggshells until hatching. Specific types of shell-less cultures are described in Table 1. In “semi-shell-less” culture, early embryos are temporarily cultured in the culture vessels and later transferred to surrogate eggshells with the addition of thin albumen. A bigger eggshell is also required at the transition from embryonic system II to III. Environmental factors and potential problems affecting the success of ex ovo culture are noted in relation to embryonic systems I–III. In ovo culture depicts the early stages (EGK X to HH28, day 0–5) of avian embryos using a chick as a model. The early embryonic stage of a chick embryo at the freshly laid egg and stage HH4 can be incorporated well with naive and primed ESCs, respectively (Akhlaghpour et al., 2021). Dark magenta dots in the embryo stages HH14–19 and HH25–28 represent primordial germ cells (PGCs) circulating in blood vessels and migrating to the genital ridges which later become gonads (Nakamura et al., 2013). The embryos described in in ovo are also used to establish pluripotent stem cells in in vitro culture. Specific culture conditions to support avian ESCs/EGCs are also noted with a brief list of gene expression profiles from Katayama et al. (2018). Fibroblasts are a common source for cellular reprogramming. The list of reprogramming factors, Oct4 (O), Sox2 (S), Klf4 (K), c-Myc (M), Nanog (N), and Lin28 (L), are listed as well as cytokines and inhibitors used for avian iPSC induction. The bottom panel shows the connection from in vitro studies to in ovo works, in particular how to produce transgenic birds via injection of lentiviral vectors in the subgerminal cavity (SGC), transgenic PGCs, and direct transfection of plasmids into the dorsal aorta of early embryos. Brown chicks indicate embryonic progression into hatching. Black chicks with red cross indicate embryos incapable of hatching or terminated before hatching. Brown-white/brown-green chicks indicate the current success of chimera and transgenic birds. Abbreviation: AP+, positive alkaline phosphatase staining; Ap, area pellucida; Ava, area vasculosa; Avi, area opaca vitellina; D., embryonic day; EGC, embryonic germ cells; EGK, Eyal-Giladi and Kochav (1976) Chick Embryonic Stages; HH, Hamburger and Hamilton (1951) Chick Embryonic Stages; hESCs, human embryonic stem cells; GC, germline competency.
Ex Ovo Cultivation of Avian Embryos: Environmental Parameters for Successful Hatching
Ex ovo culture is a system in which the original eggshell is removed, and the embryos are transferred to the new culture milieus, including a surrogate eggshell, Petri dishes, and artificial eggshell-like vessels (Ono, 2000; Borwompinyo et al., 2005; Liu et al., 2012; Tahara and Obara, 2014). By removing the eggshells, the ex ovo culture allows us to manipulate developing embryos at certain stages for surgical methods (Cloney and Franz-Odendaal, 2015). Of course, it also paves the way to seek how the embryo develops without a protective layer (Buskohl et al., 2012). Many studies have used this technique in several aspects such as angiogenesis (Hockel et al., 1987), intravasation assays (Uchibayashi et al., 1992), grafting, and tumor formation (Dohle et al., 2009; Villanueva and Sikora, 2022). Moreover, this technique can be applied to the lessons in high school and advanced developmental biology research fields (Buskohl et al., 2012; Dorrell et al., 2012; Cloney and Franz-Odendaal, 2015).
Since a previous study has shown that metabolism and internal environment within eggshells change dramatically at different stages (Givisiez et al., 2020), ex ovo cultivation aimed to follow embryonic development until hatching needs to find the optimal balance of several factors, in particular oxygen demand, humidity, and calcium requirement. Generally, the development of chick embryos can be divided into three phases: fertilization to blastoderm formation (day 0–day 1), embryogenesis (day 1–day 3), and embryonic growth (day 4–day 21) (Perry, 1988). Hitherto, three different aforementioned systems can be categorized into three systems with roman numerals: system I (for day 0–day 1), system II (day 1–day 3), and system III (day 4–day 21). The details of different techniques or modified methods described in previous studies are summarized in Table 1. Here, we also provide insights into each parameter (i–v) required for successful ex ovo studies and hatchability in relation to three phases of avian development (Figure 1).
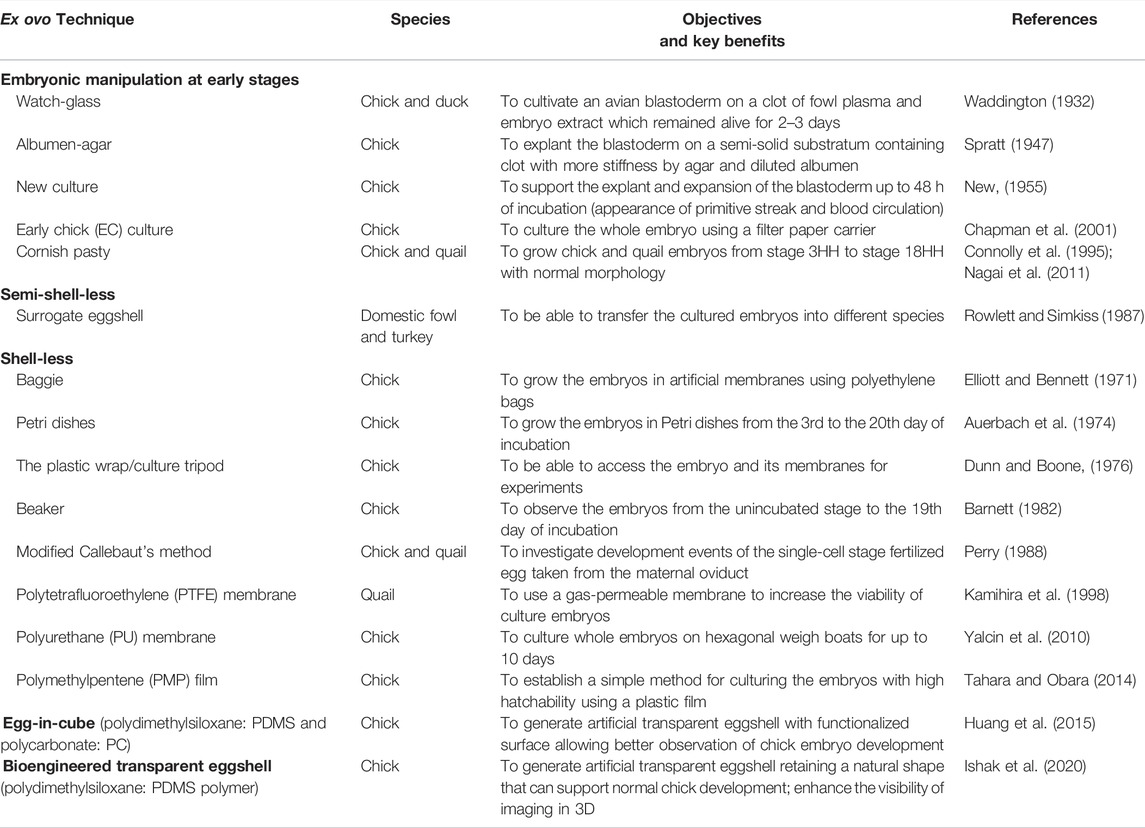
TABLE 1. Timeline of ex ovo techniques developed to understand the embryonic development in avian species.
Incubation Period and Embryonic Stage
Significantly, the embryonic age is the key factor for manipulating ex ovo cultured embryos. To increase viability during culture, transferring the embryos to the new culture vessels after stage HH15-16 (Hamburger and Hamilton, 1951) is recommended (Tahara and Obara, 2014). Those embryos developing in a polymethylpentene film and supplemented with calcium lactate and distilled water resulted in a 90% survival rate (Tahara and Obara, 2014). Tahara and Obara (2014) also showed that the embryos transferred to the culture vessel after stage HH16 showed viability on day 8 of incubation. Hence, the timing of the incubation period before transferring to the new environment plays a major role in enhancing the viability. Ideally, the embryos should be transferred to the new culture vessel at stage HH19 (Rowlett and Simkiss, 1989; Borwompinyo et al., 2005). Even though different egg preincubation periods between stages HH13 and 16 affected embryonic survival, higher viability was still observed before stage HH15 (Tahara and Obara, 2014). In addition to the embryonic age, it was also reported that care had to be taken with the vitelline membrane as it could be easily damaged while transferring to the new culture vessel (Tahara and Obara, 2014). This can be seen particularly in manipulating after-stage HH17 embryos. Dohle et al. (2009) reported that the stage HH35 (at around 9 days of incubation) embryos were less sensitive to agitation when compared to those at earlier stages. In addition, the survival rate of ex ovo cultured embryos can be higher up to 90–100% when they reached stage HH35, but then declined at the later stages, that is at stages HH40–41 (Cloney and Franz-Odendaal, 2015). Altogether, handling the right time of incubation period to acquire the right embryonic stage to proceed with hatching is essential for the ex ovo culture technique.
Temperature and Humidity
Practically, the temperature inside the incubator should not be lower than 38°C, which could cause a developmental delay (Dohle et al., 2009), and the ideal humidity was reported at 40% (Cloney and Franz-Odendaal, 2015). However, it was reported that covering the top of culture vessels with a polystyrene plastic lid could maintain 100% humidity and could be varied to 38°C with 80% humidity (Tahara and Obara, 2014). When the CAM is formed, which can be recognized on day 3 of incubation, the humidity is vital to preventing the desiccation of embryonic structures (Dohle et al., 2009). Importantly, the humidity of the system at the stage of the yolk sac formation is crucial to decreasing the humidity, to lower than 60%, which caused the CAM rupture by sticking to the eggshells (Dohle et al., 2009). Regarding the concern with water circulation systems, Yalcin et al. (2010) recommended that the culture should be kept at an optimal temperature. The design of the vessel structure is not only to prevent water loss but to facilitate oxygen supply (Tahara and Obara, 2014). The installation of a circulating water bath within the culture system helps to maintain the incubation temperature for developing embryos (Yalcin et al., 2010). In addition, a resistance heater was also used to regulate the water temperature (Yalcin et al., 2010).
Calcium Supplementation
Calcium, primarily calcium carbonate, is one of the major constituents of the eggshells (Scanes and Christensen, 2020) that serves as a tertiary envelope to protect developing embryos (Bellairs and Osmond, 2014). The majority of calcium provided to the embryos until hatching derives from the eggshell (Rowlett and Simkiss, 1987; Kamihira et al., 1998). Furthermore, calcium from the eggshells is also needed for ossification, and its deficit results in limb deformity (Cloney and Franz-Odendaal, 2015); therefore, supplying calcium to developing embryos is required (Elliott and Bennett, 1971; Rowlett and Simkiss, 1987). During the ex ovo culture, the embryos develop with no eggshells as the protective structure leading to a high mortality rate (Tahara and Obara, 2021). Therefore, adding calcium carbonate to the CAM in shell-less cultured embryos helped to increase more than 40% hatchability (Tahara et al., 2021). Kamihira et al. (1998) reported that various forms of calcium including eggshell powder and calcium lactate could also be added to the albumen as a calcium source following the eggshell removal. Although calcium carbonate is the main substance found in the eggshells, it failed to increase the hatchability rate for the shell-less system (Tahara et al., 2021). Alternatively, calcium lactate was shown to improve the hatchability rate with less toxicity to the cultured embryos (Kamihira et al., 1998; Tahara and Obara, 2014). However, supplementing calcium carbonate could increase hatchability up to 40% if it was added to the CAM (Tahara et al., 2021). These indicate that calcium supplementation with the minimum requirement is necessary for developing embryos to hatch in the shell-less system. Furthermore, information to understand the details of calcium availability and dynamics during embryonic development in this system still needs functional and molecular studies.
Oxygen Supply
Aeration in the culture vessels is mandatory for the survival of the embryos due to the loss of moisture by embryonic transpiration (Dohle et al., 2009). Importantly, the efficiency of the hatching rate can be improved by adding pure oxygen to culture vessels (Kamihira et al., 1998; Tahara and Obara, 2014). Previous studies reported that the number of embryos survived at the later stages in the pure oxygen culture system is higher than 50%, indicating the achievement of a high hatchability by culturing in such an artificial vessel (Kamihira et al., 1998; Tahara and Obara, 2014). Practically, the aeration in this culture system can be made to the ventilation holes on the top of the sealing film (Tahara and Obara, 2014). Ideally, the rate of aeration with pure oxygen installed plastic tube in the vessel should be 500 ml/h, and it should be well prepared, as the embryos do not survive to hatch without an oxygen supply (Tahara and Obara, 2014). Rowlett and Simkiss (1989) reported that chick embryos cultured in an artificial vessel made of a “cling-film” were susceptible to hypoxia and hypocapnia. Moreover, oxygen aeration at the early stages of culture should be a concern due to its toxic effect that can reduce embryonic viability (Tahara and Obara, 2014). Considerably, for the later stages (stage HH43–44), at around 17 days of incubation, oxygen insufficiency can be noticed by changing the color of the CAM vessels (Dorrell et al., 2012; Tahara and Obara, 2014; Tahara et al., 2021), indicating that oxygen aeration to the later stages is indispensable for the ex ovo culture.
Sealing Film
Favorably, in the ex ovo culture, the use of a transparent plastic film allows the embryos to reside as a cradle, namely, a hammock (Kamihira et al., 1998; Yalcin et al., 2010; Tahara and Obara, 2014) as mentioned in Table 1. The adhesive property of this material with the culture vessels is relatively low. A rubber band was carefully used to tighten the film with culture vessels firmly averting embryonic mortality during manipulation (Cloney and Franz-Odendaal, 2015). A previous study reported that the positioning of the eggs and the sealing material in culture vessels affect the hatchability rate (Borwompinyo et al., 2005); the sealing materials including Handi and Saran plastic films were able to increase the percentage of hatchability (Borwompinyo et al., 2005). There are different types of plastic packaging such as polyethylene, polyvinylidene chloride, and polymethylpentene. Tahara and Obara (2014) demonstrated that these materials should possess oxygen permeability. One important point that also increases viability and hatchability is smoothening of film surface since film wrinkles caused a low survival rate of culture embryos (Tahara and Obara, 2014, 2021). Although using transparent films would help to facilitate whole-embryo observation, some disadvantages of their properties should be ameliorated to increase the hatchability rate.
In Vitro Culture: Essential Toolkit for Avian Pluripotency Studies, Transgenesis, and Conservation
As pluripotent cells in embryos are restricted in some periods and in some embryonic tissues, the understanding of the pluripotency mechanism in embryos is limited. The advents of in vitro culture from avian embryos enable us to examine the pluripotency network and possible use of germline-competent stem cells (GCSCs) in avian biotechnology, in particular gene editing, transgenesis, and wild bird conservation (Han et al., 2015). There are four main types of GCSCs that can be derived from the in vitro culture of avian embryonic tissues: blastodermal cells/embryonic stem cells (ESCs), embryonic germ cells (EGCs), primordial germ cells (PGCs), and spermatogonial stem cells (SSCs) (Han et al., 2015). The discovery of chick ESCs and blastodermal cell culture emphasizes the conserved network regulating pluripotency required at least leukemia inhibitory factor (LIF) (Etches et al., 1997; Pain et al., 1996), a cytokine used to culture naïve mouse ESCs and iPSCs (Niwa et al., 1998; Takahashi and Yamanaka, 2006). In addition, basic fibroblast growth factor (bFGF), a cytokine used to cultivate primed human ESCs/iPSCs (Thomson et al., 1998; Takahashi et al., 2007), is required in several avian ESCs/iPSCs studies (Pain et al., 1996; van de Lavoir et al., 2006; Whyte et al., 2015; Choi et al., 2016; Katayama et al., 2018). Thus, this suggests that pluripotent stem cells from avian species exhibit some bias in a naïve-primed direction which could depend on other culture supplements. Several studies have used (Aubel and Pain, 2013) protocol to culture chick iPSCs in DMEM/F12 containing other several cytokines in addition to LIF and/or bFGF (FGF2) including IGF1, SCF, IL6, and sIL6 Rα (Dai et al., 2014; Fuet and Pain, 2017; Fuet et al., 2018). To force pluripotent cells to bias toward a naïve state, two inhibitors’ (2i) cocktail including CHIR99021 (GSK3 inhibitor) and PD0325901 (MEK inhibitor), generally used in naïve mouse ESCs (Ying et al., 2008) can also support the establishment of chick iPSCs (Dai et al., 2014; Katayama et al., 2018; Yuan et al., 2021), as shown in Figure 1. In avian cellular reprogramming, choices of delivery and expression system and reprogramming factors are crucial for induction success of iPSCs. Exogenous gene delivery can be done via using a virus (retrovirus, lentivirus, and Sendai virus) (Dai et al., 2014; Fuet et al., 2018) and liposome-based transfection of a piggyBac transposon carrying a single polycistronic reprogramming cassette (Modified Oct4, Sox2, Klf4, c-Myc, Lin28, and Nanog) (Katayama et al., 2018) (Figure 1). Reprogramming factors used for induction of avian iPSCs varied in several studies but at least Oct4 and Sox2 were used in all studies (Dai et al., 2014; Fuet and Pain, 2017; Fuet et al., 2018; Katayama et al., 2018), while Klf4 and c-Myc were replaced with Nanog and Lin28 in Yuan et al.( 2021) and Zhao et al. (2021). Fuet et al. (2018) also showed that Nanog is essential for long-term iPSC culture. In addition to pluripotent stem cells, in vitro culture allows the exploration of the primordial germ cell (PGCs) specification mechanism. It has been shown that avian PGCs do not require LIF for self-renewal (Whyte et al., 2015) while mammalian PGCs need it (Leitch et al., 2013). But, instead, bird PGCs, which resemble more the mammalian gastrulating epiblast (or in vitro mouse EpiSCs), can be supported under FGF2, insulin, and activin-BMP4 to induce SMAD signaling (Whyte et al., 2015). Also, chick iPSCs can differentiate into induced PGCs (iPGCs) which can be transplanted into another strain of a chick embryo to produce viable offspring (Zhao et al., 2021).
In vitro culture also supports the study of transcriptomic analysis of established ESCs/iPSC cell lines and sheds light on the conservation and uniqueness of pluripotency-related transcriptional networks in the avian species (Jean et al., 2015; Katayama et al., 2018). The list of ESCs/PGCs versus fibroblast markers is also shown in Figure 1. Finally, the key advantage of in vitro culture is the application of established cell lines including GCSCs back into in ovo cultivation for producing interspecific avian chimeras (within avian species or even mammalian to avian species), as shown in Figure 1. At the early stage of transgenic avian technology, transgenesis in chick embryo was done using a virus-dependent approach, e.g., injection of lentiviral vectors into the subgerminal cavity of the early embryo (Chapman et al., 2005). The advent of PGC culture allows easy manipulation of gene delivery via transfection (lipofection or nucleofection) as shown in the success of stable integration of gfp transgene into the host genome and later transgenic PGCs can be transplanted back to the embryos to eventually generate chimera and transgenic chicks (Park and Han, 2012). Recently, the direct injection of plasmids carrying the gene of interest, together with a liposome-based reagent, into the dorsal aorta of chick embryo containing circulating PGCs was performed with the successful production of transgenic quail offspring (Serralbo et al., 2020). Overall, the achievement of in vitro culture with in ovo application and avian embryo plasticity to accept xenograft cells provide a hope of using chick embryos as a platform to propagate endangered wild birds under stem cell-based conservation.
Future Recommendations and Conclusion
For ex ovo milieu, exploration of possible avenues on how to culture avian embryo without a natural shell continues with the further development of imaging, chemistry, and nano-biomaterial technology, which can provide better-engineered eggshells resembling natural ones and fit to in-demand experiments such as in-depth imaging of embryos or transgenic embryos with artificial eggshells intact. In addition to in vitro culture, research on cellular reprogramming and ESCs derivation of various avian species is still required to understand the nature of naïve-primed pluripotency in relation to germline competency-the benefit of this understanding is that one day it can provide long-term security and prevent the extinction of avian species.
Author Contributions
WS and SI conceived the idea. WS, AT, and SI contributed to the writing of the manuscript. All authors contributed to the articles and approved the submitted manuscript.
Funding
This work was supported by the e-ASIA Joint Research Program (e-ASIA JRP) as administered by National Research Council of Thailand (grant no. N33A640448).
Conflict of Interest
The authors declare that the research was conducted in the absence of any commercial or financial relationships that could be construed as a potential conflict of interest.
Publisher’s Note
All claims expressed in this article are solely those of the authors and do not necessarily represent those of their affiliated organizations, or those of the publisher, the editors and the reviewers. Any product that may be evaluated in this article, or claim that may be made by its manufacturer, is not guaranteed or endorsed by the publisher.
References
Akhlaghpour A., Taei A., Ghadami S. A., Bahadori Z., Yakhkeshi S., Molamohammadi S., et al. (2021). Chicken Interspecies Chimerism Unveils Human Pluripotency. Stem Cel Rep. 16, 39–55. doi:10.1016/j.stemcr.2020.11.014
Altgilbers S., Klein S., Dierks C., Weigend S., Kues W. A. (2021). Cultivation and Characterization of Primordial Germ Cells from Blue Layer Hybrids (Araucana Crossbreeds) and Generation of Germline Chimeric Chickens. Sci. Rep. 11, 12923. doi:10.1038/s41598-021-91490-y
Andacht T., Hu W., Ivarie R. (2004). Rapid and Improved Method for Windowing Eggs Accessing the Stage X Chicken Embryo. Mol. Reprod. Dev. 69, 31–34. doi:10.1002/mrd.20155
Aubel P., Pain B. (2013). Chicken Embryonic Stem Cells: Establishment and Characterization. Methods Mol. Biol. 1074, 137–150. doi:10.1007/978-1-62703-628-3_11
Auerbach R., Kubai L., Knighton D., Folkman J. (1974). A Simple Procedure for the Long-Term Cultivation of Chicken Embryos. Dev. Biol. 41, 391–394. doi:10.1016/0012-1606(74)90316-9
Azzarello J., Ihnat M. A., Kropp B. P., Warnke L. A., Lin H.-K. (2007). Assessment of Angiogenic Properties of Biomaterials Using the Chicken Embryo Chorioallantoic Membrane Assay. Biomed. Mater. 2, 55–61. doi:10.1088/1748-6041/2/2/001
Barnett S. B. (1982). A Method for the Observation of Long Term Development in Chick Embryos. Poult. Sci. 61, 172–174. doi:10.3382/ps.0610172
Borges J., Tegtmeier F. T., Padron N. T., Mueller M. C., Lang E. M., Stark G. B. (2003). Chorioallantoic Membrane Angiogenesis Model for Tissue Engineering: a New Twist on a Classic Model. Tissue Eng. 9, 441–450. doi:10.1089/107632703322066624
Borwompinyo S., Brake J., Mozdziak P. E., Petitte J. N. (2005). Culture of Chicken Embryos in Surrogate Eggshells. Poult. Sci. 84, 1477–1482. doi:10.1093/ps/84.9.1477
Buskohl P. R., Gould R. A., Curran S., Archer S. D., Butcher J. T. (2012). Multidisciplinary Inquiry-Based Investigation Learning Using an Ex Ovo Chicken Culture Platform: Role of Vitamin A on Embryonic Morphogenesis. Am. Biol. Teach. 74, 636–643. doi:10.1525/abt.2012.74.9.7
Chapman S. C., Collignon J. r. m., Schoenwolf G. C., Lumsden A. (2001). Improved Method for Chick Whole-Embryo Culture Using a Filter Paper Carrier. Dev. Dyn. 220, 2842–2895. doi:10.1002/1097-0177(20010301)220:3<284::AID-DVDY1102>3.0.CO;2-5
Chapman S. C., Lawson A., Macarthur W. C., Wiese R. J., Loechel R. H., Burgos-Trinidad M., et al. (2005). Ubiquitous GFP Expression in Transgenic Chickens Using a Lentiviral Vector. Development 132, 935–940. doi:10.1242/dev.01652
Choi J. W., Kim S., Kim T. M., Kim Y. M., Seo H. W., Park T. S., et al. (2010). Basic Fibroblast Growth Factor Activates MEK/ERK Cell Signaling Pathway and Stimulates the Proliferation of Chicken Primordial Germ Cells. PLoS ONE 5, e12968. doi:10.1371/journal.pone.0012968
Choi H. W., Kim J. S., Choi S., Ju Hong Y., Byun S. J., Seo H. G., et al. (2016). Mitochondrial Remodeling in Chicken Induced Pluripotent Stem-like Cells. Stem Cell Dev. 25, 472–476. doi:10.1089/scd.2015.0299
Cloney K., Franz-Odendaal T. A. (2015). Optimized Ex-Ovo Culturing of Chick Embryos to Advanced Stages of Development. JoVE 95, 52129. doi:10.3791/52129
Connolly D., Mcnaugbton L., Krumlauf R., Cooke J. (1995). Improved Development of the Chick Embryo Using Roller-Tube Culture. Trends Genet. 11, 259–260. doi:10.1016/s0168-9525(00)89070-8
Dai R., Rossello R., Chen C.-c., Kessler J., Davison I., Hochgeschwender U., et al. (2014). Maintenance and Neuronal Differentiation of Chicken Induced Pluripotent Stem-like Cells. Stem Cell Int. 2014, 1–14. doi:10.1155/2014/182737
Das R., Mishra P., Jha R. (2021). In Ovo Feeding as a Tool for Improving Performance and Gut Health of Poultry: A Review. Front. Vet. Sci. 8, 754246. doi:10.3389/fvets.2021.754246
Dohle D. S., Pasa S. D., Gustmann S., Laub M., Wissler J. H., Jennissen H. P., et al. (2009). Chick Ex Ovo Culture and Ex Ovo CAM Assay: How it Really Works. JoVE 33, 1620. doi:10.3791/1620
Dorrell M. I., Marcacci M., Bravo S., Kurz T., Tremblay J., Rusing J. C. (2012). Ex Ovo Model for Directly Visualizing Chick Embryo Development. Am. Biol. Teach. 74, 628–634. doi:10.1525/abt.2012.74.9.6
Dunn B. E., Boone M. A. (1976). Growth of the Chick Embryo In Vitro. Poult. Sci. 55, 1067–1071. doi:10.3382/ps.0551067
Dupertuis Y. M., Delie F., Cohen M., Pichard C. (2015). In Ovo Method for Evaluating the Effect of Nutritional Therapies on Tumor Development, Growth and Vascularization. Clin. Nutr. Exp. 2, 9–17. doi:10.1016/j.yclnex.2015.08.001
Elliott J. H., Bennett J. (1971). Growth of Chick Embryos in Polyethylene Bags. Poult. Sci. 50, 974–975. doi:10.3382/ps.0500974
Etches R., Clark M., Zajchowski L., Speksnijder G., Verrinder Gibbins A., Kino K., et al. (1997). Manipulation of Blastodermal Cells. Poult. Sci. 76, 1075–1083. doi:10.1093/ps/76.8.1075
Eyal-Giladi H., Kochav S. (1976). From Cleavage to Primitive Streak Formation: A Complementary Normal Table and a New Look at the First Stages of the Development of the Chick. I. General Morphology. Dev. Biol. 49, 321–337. doi:10.1016/0012-1606(76)90178-0
Fisher M., Schoenwolf G. C. (1983). The Use of Early Chick Embryos in Experimental Embryology and Teratology: Improvements in Standard Procedures. Teratology 27, 65–72. doi:10.1002/tera.1420270110
Fuet A., Pain B. (2017). Chicken Induced Pluripotent Stem Cells: Establishment and Characterization. Methods Mol. Biol. 1650, 211–228. doi:10.1007/978-1-4939-7216-6_14
Fuet A., Montillet G., Jean C., Aubel P., Kress C., Rival-Gervier S., et al. (2018). NANOG Is Required for the Long-Term Establishment of Avian Somatic Reprogrammed Cells. Stem Cel Rep. 11, 1272–1286. doi:10.1016/j.stemcr.2018.09.005
Givisiez P. E. N., Moreira Filho A. L. B., Santos M. R. B., Oliveira H. B., Ferket P. R., Oliveira C. J. B., et al. (2020). Chicken Embryo Development: Metabolic and Morphological Basis for In Ovo Feeding Technology. Poult. Sci. 99, 6774–6782. doi:10.1016/j.psj.2020.09.074
Hamburger V., Hamilton H. L. (1951). A Series of normal Stages in the Development of the Chick Embryo. J. Morphol. 88, 49–92. doi:10.1002/jmor.1050880104
Han J., Lee H., Park T. (2015). Germline-competent Stem Cell in Avian Species and its Application. Asian J. Androl. 17, 421–426. doi:10.4103/1008-682X.148073
Han J. Y., Lee H. G., Park Y. H., Hwang Y. S., Kim S. K., Rengaraj D., et al. (2018). Acquisition of Pluripotency in the Chick Embryo Occurs during Intrauterine Embryonic Development via a Unique Transcriptional Network. J. Anim. Sci Biotechnol. 9, 31. doi:10.1186/s40104-018-0246-0
Höckel M., Sasse J., Wissler J. H. (1987). Purified Monocyte-Derived Angiogenic Substance (Angiotropin) Stimulates Migration, Phenotypic Changes, and "Tube Formation" but Not Proliferation of Capillary Endothelial Cells In Vitro. J. Cel. Physiol. 133, 1–13. doi:10.1002/jcp.1041330102
Huang W., Arai F., Kawahara T. (2015). Egg-in-Cube: Design and Fabrication of a Novel Artificial Eggshell with Functionalized Surface. PLoS ONE 10, e0118624. doi:10.1371/journal.pone.0118624
Ishak N., Chan M., Ang S. C., Cheung C., Teoh S. H. (2020). Bioengineered Three‐dimensional Transparent Eggshell as a Chicken Embryo Experimentation Platform for Biomedical Research. Eng. Rep. 2, e12092. doi:10.1002/eng2.12092
Jean C., Oliveira N. M. M., Intarapat S., Fuet A., Mazoyer C., De Almeida I., et al. (2015). Transcriptome Analysis of Chicken ES, Blastodermal and Germ Cells Reveals that Chick ES Cells Are Equivalent to Mouse ES Cells rather Than EpiSC. Stem Cel Res. 14, 54–67. doi:10.1016/j.scr.2014.11.005
Kamihira M., Oguchi S., Tachibana A., Kitagawa Y., Iijima S. (1998). Improved Hatching for In Vitro Quail Embryo Culture Using Surrogate Eggshell and Artificial Vessel. Dev. Growth Differ. 40, 449–455. doi:10.1046/j.1440-169x.1998.t01-2-00010.x
Katayama M., Hirayama T., Tani T., Nishimori K., Onuma M., Fukuda T. (2018). Chick Derived Induced Pluripotent Stem Cells by the Poly‐cistronic Transposon with Enhanced Transcriptional Activity. J. Cel Physiol. 233, 990–1004. doi:10.1002/jcp.25947
Korn M. J., Cramer K. S. (2007). Windowing Chicken Eggs for Developmental Studies. JoVE 8, 306. doi:10.3791/306
Leitch H. G., Nichols J., Humphreys P., Mulas C., Martello G., Lee C., et al. (2013). Rebuilding Pluripotency from Primordial Germ Cells. Stem Cel Rep. 1, 66–78. doi:10.1016/j.stemcr.2013.03.004
Liu C., Zu J., Baskar V., Wernery U., Chang I. K. (2012). Culture of Chicken Embryo in Interspecific Surrogate Egg white. Poult. Sci. 91, 2866–2871. doi:10.3382/ps.2012-02403
Nagai H., Lin M.-C., Sheng G. (2011). A Modified Cornish Pasty Method for Ex Ovo Culture of the Chick Embryo. Genesis 49, 46–52. doi:10.1002/dvg.20690
Nakamura Y., Kagami H., Tagami T. (2013). Development, Differentiation and Manipulation of Chicken Germ Cells. Dev. Growth Differ. 55 (1), 20–40. doi:10.1111/dgd.12026
New D. A. T. (1955). A New Technique for the Cultivation of the Chick Embryo In Vitro. Development 3, 326–331. doi:10.1242/dev.3.4.326
Niwa H., Burdon T., Chambers I., Smith A. (1998). Self-renewal of Pluripotent Embryonic Stem Cells Is Mediated via Activation of STAT3. Genes Dev. 12, 2048–2060. doi:10.1101/gad.12.13.2048
Nowak-Sliwinska P., Segura T., Iruela-Arispe M. L. (2014). The Chicken Chorioallantoic Membrane Model in Biology, Medicine and Bioengineering. Angiogenesis 17, 779–804. doi:10.1007/s10456-014-9440-7
Ono T. (2000). Exo Ovo Culture of Avian Embryos. Methods Mol. Biol. 135, 39–46. doi:10.1385/1-59259-685-1:39
Pain B., Clark M. E., Shen M., Nakazawa H., Sakurai M., Samarut J., et al. (1996). Long-term In Vitro Culture and Characterisation of Avian Embryonic Stem Cells with Multiple Morphogenetic Potentialities. Development 122, 2339–2348. doi:10.1242/dev.122.8.2339
Park T. S., Han J. Y. (2012). piggyBac Transposition into Primordial Germ Cells Is an Efficient Tool for Transgenesis in Chickens. Proc. Natl. Acad. Sci. U.S.A. 109, 9337–9341. doi:10.1073/pnas.1203823109
Perry M. M. (1988). A Complete Culture System for the Chick Embryo. Nature 331, 70–72. doi:10.1038/331070a0
Rowlett K., Simkiss K. (1987). Explanted Embryo Culture: In Vitro and In Ovo Techniques for Domestic Fowl. Br. Poult. Sci. 28, 91–101. doi:10.1080/00071668708416940
Rowlett K., Simkiss K. (1989). Respiratory Gases and Acid-Base Balance in Shell-Less Avian Embryos. J. Exp. Biol. 143, 529–536. doi:10.1242/jeb.143.1.529
Saeed M., Babazadeh D., Naveed M., Alagawany M., Abd El‐Hack M. E., Arain M. A., et al. (2019). In Ovo Delivery of Various Biological Supplements, Vaccines and Drugs in Poultry: Current Knowledge. J. Sci. Food Agric. 99, 3727–3739. doi:10.1002/jsfa.9593
Scanes C. G., Christensen K. D. (2020). Poultry Science. 5th Edn. Long Grove, IL: Waveland Press, Inc.
Serralbo O., Salgado D., Véron N., Cooper C., Dejardin M.-J., Doran T., et al. (2020). Transgenesis and Web Resources in Quail. Elife 9, e56312. doi:10.7554/eLife.56312
Silver P. H. S. (1960). Special Problems of Experimenting In Ovo on the Early Chick Embryo, and a Solution. Development 8, 369–375. doi:10.1242/dev.8.4.369
Speksnijder G., Ivarie R. (2000). A Modified Method of Shell Windowing for Producing Somatic or Germline Chimeras in Fertilized Chicken Eggs. Poult. Sci. 79, 1430–1433. doi:10.1093/ps/79.10.1430
Spratt N. T. (1947). Development In Vitro of the Early Chick Blastoderm Explanted on Yolk and Albumen Extract saline-agar Substrata. J. Exp. Zool. 106, 345–365. doi:10.1002/jez.1401060308
Stern C. (2005). The Chick: A Great Model System Becomes Even Greater. Dev. Cell. 8, 9–17. doi:10.1016/j.devcel.2004.11.018
Tahara Y., Obara K. (2014). A Novel Shell-Less Culture System for Chick Embryos Using a Plastic Film as Culture Vessels. J. Poult. Sci. 51, 307–312. doi:10.2141/jpsa.0130043
Tahara Y., Obara K. (2021). Ex Ovo Culture System for Avian Embryos and its Application. J. Poult. Sci. 58, 1–4. doi:10.2141/jpsa.0200016
Tahara Y., Obara K., Kamihira M. (2021). Calcium Carbonate Supplementation to Chorioallantoic Membranes Improves Hatchability in Shell-Less Chick Embryo Culture. J. Biosci. Bioeng. 131, 314–319. doi:10.1016/j.jbiosc.2020.11.001
Takahashi K., Yamanaka S. (2006). Induction of Pluripotent Stem Cells from Mouse Embryonic and Adult Fibroblast Cultures by Defined Factors. Cell 126, 663–676. doi:10.1016/j.cell.2006.07.024
Takahashi K., Tanabe K., Ohnuki M., Narita M., Ichisaka T., Tomoda K., et al. (2007). Induction of Pluripotent Stem Cells from Adult Human Fibroblasts by Defined Factors. Cell 131, 861–872. doi:10.1016/j.cell.2007.11.019
Thomson J. A., Itskovitz-Eldor J., Shapiro S. S., Waknitz M. A., Swiergiel J. J., Marshall V. S., et al. (1998). Embryonic Stem Cell Lines Derived from Human Blastocysts. Science 282, 1145–1147. doi:10.1126/science.282.5391.1145
Uchibayashi T., Egawa M., Nakajima K., Hisazumi H., Tanaka M., Endo Y., et al. (1992). Responses of Tumour Cell Lines Implanted onto the Chorioallantoic Membrane of Chick Embryo to Anticancer Agents in Combination with Hyperthermia. Urol. Res. 20, 237–239. doi:10.1007/BF00299724
Valdes T. I., Klueh U., Kreutzer D., Moussy F. (2003). Ex Ova Chick Chorioallantoic Membrane as a Novel In Vivo Model for Testing Biosensors. J. Biomed. Mater. Res. 67A, 215–223. doi:10.1002/jbm.a.10055
van de Lavoir M.-C., Diamond J. H., Leighton P. A., Mather-Love C., Heyer B. S., Bradshaw R., et al. (2006). Germline Transmission of Genetically Modified Primordial Germ Cells. Nature 441, 766–769. doi:10.1038/nature04831
Villanueva H., Sikora A. G. (2022). The Chicken Embryo Chorioallantoic Membrane (CAM): A Versatile Tool for the Study of Patient-Derived Xenografts. Methods Mol. Biol. 2471, 209–220. doi:10.1007/978-1-0716-2193-6_11
Waddington C. H. (1932). III. Experiments on the Development of Chick and Duck Embryos, Cultivated In Vitro. Phil. Trans. R. Soc. Lond. B 221, 179–230. doi:10.1098/rstb.1932.0003
Weber J., Weber M., Steinle H., Schlensak C., Wendel H.-P., Avci-Adali M. (2021). An Alternative In Vivo Model to Evaluate Pluripotency of Patient-Specific iPSCs. Altex 38, 442–450. doi:10.14573/altex.2005221
Whyte J., Glover J. D., Woodcock M., Brzeszczynska J., Taylor L., Sherman A., et al. (2015). FGF, Insulin, and SMAD Signaling Cooperate for Avian Primordial Germ Cell Self-Renewal. Stem Cel Rep. 5, 1171–1182. doi:10.1016/j.stemcr.2015.10.008
Wolpert L. (2004). Much More from the Chicken's Egg Than Breakfast - a Wonderful Model System. Mech. Dev. 121, 1015–1017. doi:10.1016/j.mod.2004.04.021
Yalcin H. C., Shekhar A., Rane A. A., Butcher J. T. (2010). An Ex-Ovo Chicken Embryo Culture System Suitable for Imaging and Microsurgery Applications. JoVE 44, 2154. doi:10.3791/2154
Ying Q.-L., Wray J., Nichols J., Batlle-Morera L., Doble B., Woodgett J., et al. (2008). The Ground State of Embryonic Stem Cell Self-Renewal. Nature 453, 519–523. doi:10.1038/nature06968
Yuan X., Zhang C., Zhao R., Jiang J., Shi X., Zhang M., et al. (2021). Glycolysis Combined with Core Pluripotency Factors to Promote the Formation of Chicken Induced Pluripotent Stem Cells. Animals 11, 425. doi:10.3390/ani11020425
Keywords: avian embryo, embryonic development, ex ovo cultivation, in ovo cultivation, in vitro culture, pluripotency
Citation: Sukparangsi W, Thongphakdee A and Intarapat S (2022) Avian Embryonic Culture: A Perspective of In Ovo to Ex Ovo and In Vitro Studies. Front. Physiol. 13:903491. doi: 10.3389/fphys.2022.903491
Received: 24 March 2022; Accepted: 12 April 2022;
Published: 16 May 2022.
Edited by:
Servet Yalcin, Ege University, TurkeyReviewed by:
Paul Mozdziak, North Carolina State University, United StatesTadashi Nomura, Kyoto Prefectural University of Medicine, Japan
Copyright © 2022 Sukparangsi, Thongphakdee and Intarapat. This is an open-access article distributed under the terms of the Creative Commons Attribution License (CC BY). The use, distribution or reproduction in other forums is permitted, provided the original author(s) and the copyright owner(s) are credited and that the original publication in this journal is cited, in accordance with accepted academic practice. No use, distribution or reproduction is permitted which does not comply with these terms.
*Correspondence: Sittipon Intarapat, c2l0dGlwb24uaW50QG1haGlkb2wuYWMudGg=