- 1Univ Angers, CHU Angers, CRC, INSERM, CNRS, MITOVASC, Equipe CarMe, SFR ICAT, Angers, France
- 2State Key Laboratory of Space Medicine Fundamentals and Application, China Astronaut Research and Training Center, Beijing, China
- 3Beijing Tiantan Hospital, Medical Capital University, Beijing, China
- 4CNES, Toulouse, France
- 5CNES, Paris, France
Spaceflight is associated with enhanced inactivity, resulting in muscular and cardiovascular deconditioning. Although physical exercise is commonly used as a countermeasure, separate applications of running and resistive exercise modalities have never been directly compared during long-term bedrest. This study aimed to compare the effectiveness of two exercise countermeasure programs, running and resistance training, applied separately, for counteracting cardiovascular deconditioning induced by 90-day head-down bedrest (HDBR). Maximal oxygen uptake (
1 Introduction
Exposure to space environment and its ground-based analogs causes several deleterious effects (Wang et al., 2019; Pavy-Le-Traon et al., 2007). Neuromuscular function is systematically affected, with motor disturbances and decreases in muscle tone, force, and mass (Pavy-Le-Traon et al., 2007; Mounier et al., 2009). This muscle deconditioning may be related to inactivity, gravitational unloading, and metabolic changes (Rynders et al., 2018). The cardiovascular system undergoes deconditioning with a decrease in maximal oxygen uptake (
Maintaining the health and fitness of astronauts is essential, particularly with a view toward deep space missions. Several countermeasures have been tested or used empirically to limit cardiovascular deconditioning and preserve physical performance. Thus, salt and water ingestion prior to landing (Wang et al., 2019; Kozlovskaya et al., 2015) is used to expand plasma volume. A lower body gradient compression garment (Wang et al., 2019; Kozlovskaya et al., 2015) is used to create a cephalad fluid shift during landing and postflight adaptation. Onboard, a lower body negative pressure device (LBNP) (Wang et al., 2019; Kozlovskaya et al., 2015) is used, mainly by Russian crew members, to create a downward fluid shift, simulating the “cardiovascular” effects of orthostasis. A key element in the countermeasures system in long-term flights is physical training and, especially, locomotor training (Grigoriev and Kozlovskaya 2018). Astronauts exercise daily to limit the decrease in cardiorespiratory and muscular fitness (Wang et al., 2019; Kozlovskaya et al., 2015).
Several head-down bedrest (HDBR) investigations have already been conducted to test exercise countermeasures against cardiovascular deconditioning. Resistance exercise alone (flywheel horizontal leg press) did not counteract the decrease in orthostatic tolerance following 90-day HDBR (Belin de Chantemele et al., 2004). Aerobic exercise of moderate intensity and duration seems insufficient to preserve maximal aerobic capacity (Lee et al., 2010). Indeed, mild supine exercise (cycling at 40%
Separate applications of running and resistive exercise modalities have never been directly compared during long-term HDBR. It is important to understand the separate effects of locomotor and resistive exercise to optimize countermeasure prescriptions, to individually adapt the proportion of modalities for different moments of missions and different aspects of deconditioning, and, in case of difficulties, to use a combination of countermeasures because of crew time, spacecraft volume, technology, equipment issues, etc.
1.1 Objective
We aimed to compare the effectiveness of two exercise countermeasure programs, running and resistance, applied separately, for counteracting cardiovascular deconditioning in healthy young men during a 90-day HDBR.
2 Materials and methods
2.1 Subjects
A total of 22 healthy Asian nonathletic men (with maximal oxygen uptake,
The protocol was approved by the ethics committee of the Astronaut Center of China (ACC). All the procedures and risks associated with this experiment were explained to the subjects, and written consent was obtained from each participant.
2.2 Study design
The study was conducted at the Space Institute of Southern China (SISC, Shenzhen, China) in 2019. Baseline measurements (Pre) were started 15 days before bedrest (ambulatory subjects freely moving in the facility), and recovery measurements (R) ended 33 days after bedrest (Figure 1). Strict bedrest lasted 90 days. Coffee, tea, and nicotine were prohibited during the experiment. The lights-off period was set from 22:30 to 06:30. All routine activities (toilet, meals, etc.) and height and weight measurements were performed in a 6° head-down posture. Subjects were allowed to change position but were required to keep at least one shoulder in contact with the bed. Muscle activity of the legs was not allowed outside of the specific countermeasures and protocols. Subjects were observed continuously by video to ensure compliance. For operational reasons, daily caloric intake was fixed independently of body mass at 2,700 kcal for CON and 2,800 kcal for RUN and RES, with 50%–65% covered by carbohydrates, 20%–35% covered by fat, and 12%–15% covered by protein (with 60% of animal source and 40% of vegetable source). Water intake was ad libitum. Sodium intake was 1.5–3 g/day.
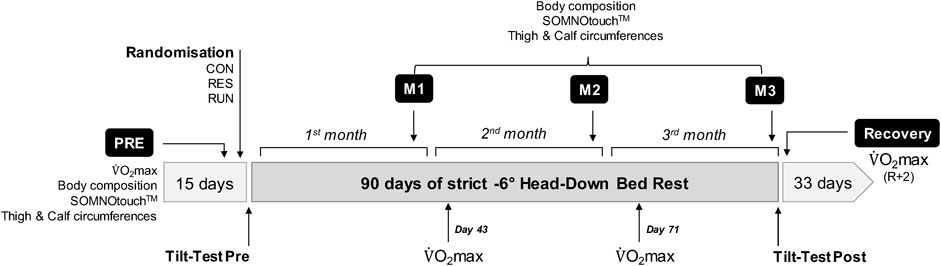
FIGURE 1. Study chronology with performed measurements. The experiment consisted of 15 days of baseline measurements (Pre), followed by 90 days of strict head-down bedrest where the subjects remained in a 6° head-down posture full time and 33 days of recovery (R + x).
2.3 Exercise countermeasure protocol
2.3.1 Running exercise countermeasure
The RUN group used a vertical treadmill, which allowed exercise to be performed while lying down horizontally (Figure 2A). A shoulder-and-waist harness was used to load the exercising subjects. The subjects ran continuously at about 70%–80% of their
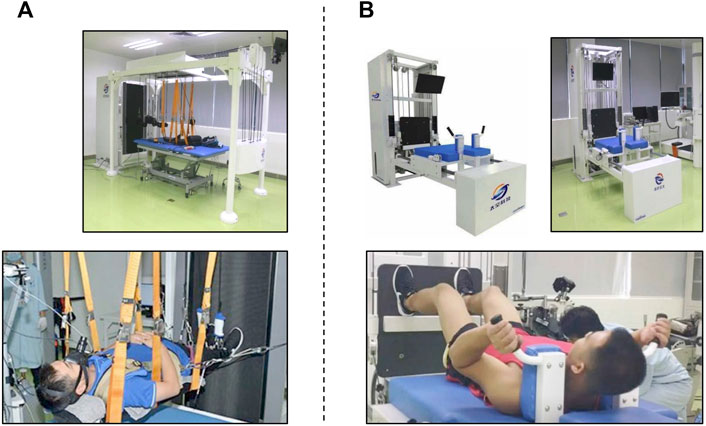
FIGURE 2. Exercise countermeasures. Treadmill training (A). A vertically tilted treadmill allows the subject to perform moderate- and high-intensity running while remaining supine. Exercise protocol: moderate running (60%–80%
Continuous running and high-intensity run sets were performed in the motor-powered treadmill mode. Heart rate and running speed were controlled. Exercise levels were adjusted to the desired percentage of maximal HR by modifying running speed at a scale of 0.5 km/h.
2.3.2 Resistance exercise countermeasure
The RES group used a flywheel exercise device, which was mounted on a supine resistance exercise platform to allow exercising while lying down (Figure 2B). This supine resistance exercise platform was developed to simulate the exercise mode in orbit without the action of body weight. We wanted this platform to provide free weight or flywheel resistance. This platform has two kinds of resistance force. One comes from the constant resistance block. Another resistance force comes from the flywheel. Its force is transmitted by a tension band. The output resistance splits by a fixed pulley and then each end connects to a moving pulley. Through this pulley assembly, we can have the bilateral resistance output. In fact, using a flywheel can complete exercise in the supine posture. As we have established the platform and the posture can be easily controlled in this platform, we have chosen to use the platform to complete this experiment.
Subjects were positioned on a moveable platform with the shoulder pads and hand grips. The subjects exercised for 45 min three times a week (Monday, Wednesday, and Friday). They performed squats, heel raises, shoulder shrugs, and curls at 60%–80% of maximal voluntary contraction (MVC) in 3 sets of 10–12 repetitions. For squats, the knee flexion angle was from ∼90° to ∼170°, and repetition of concentric to eccentric contraction was about 2–3 s. For heel raises, the subject placed his forefoot on the platform, did ankle plantar flexion, and maintained it for about 2 s, letting the heel drop when he felt obvious stretching of the gastrocnemius. For shrugs, the subject lifted the shoulders as high as he could and held contraction for 1 s and then slowly returned to the starting position. For curls, the subject lifted up the holding bar in about 2 s and then returned to the starting position in about 2 s. Subjects were given visual feedback, ensuring that the subjects were performing the exercise in the desired range of motion and speed. As the load of the flywheel is tightly related to the speed of action performance, the exercise intensity could not be finely controlled. The primary training load was set at 60%–80% of pre-HDBR MVC, and then the maximal loads were rising across daily training. When the maximal load was greater than 80% MVC, the loads were controlled at the range of 75%–125% of the actual maximal loads.
During bedrest, the loads were adjusted monthly according to MVC assessment results. MVC was determined for squats, as mean maximal contraction for three repetitions. It was planned to adjust resistance exercise intensity if MVC dropped below 75% of the pre-HDBR baseline, but this did not happen.
2.4 Maximal oxygen uptake test
An incremental dynamic leg exercise test on a cycle ergometer (Shanxi Orient Health Industry Co., Ltd., China) was performed in the -6° position at Pre, D43, D71, and R + 2 to determine maximal oxygen uptake (
The subjects rested for 5 min and then cycled for 2 min at 50 W, followed by an increase of 50 W every 2 min until 150 W, which was then changed to an increase of 25 W every 2 min until the subject reached the maximal heart rate (HR) (220—age) or maximal power of 300 W or 230 mmHg of systolic blood pressure (SBP) or peak exertion or wanted to stop.
2.5 Tilt test
A tilt test was performed before and immediately after the end of bedrest (first return to upright), in the morning or in the afternoon (the same sequence of subjects was used at Pre and Post), at least 1 h after the meal, using an automatic tilt table (Beijing Juchi Pharmaceutical Technology Co., Ltd., China). The subject remained in a horizontal position for 10 min, and then supine data were acquired for 10 min. The subject was then tilted up to 75° for a maximum of 30 min. Criteria for stopping the test earlier were symptoms such as excessive sweating, pallor, vertigo, nausea, or a sudden drop in BP (SBP drop > 25 mmHg/min, or DBP drop > 15 mmHg/min, or SBP < 70 mmHg) or HR drop > 15 beats/min. Subjects completing the full 30 min of tilt were considered finishers.
During the test, orthostatic tolerance time (OTT) was measured. Subjects were monitored in real time using a task-force monitor (CN-system). Hemodynamic indices—blood pressure (SBP and DBP), HR, stroke volume (SV; assessed by impedance cardiography), and total peripheral resistance (TPR)—were noted every 5 min; the ECG was recorded.
Autonomic cardiac modulation was assessed from the ECG recording via spectral heart rate variability (HRV) analysis markers—normalized low-frequency (LF) and high-frequency (HF) spectrum power and the LF-to-HF ratio [for details see Coupé et al. (2011)]. The last 5 min of stable supine and tilt recordings (before the onset of intolerance if it occurred) were selected for this analysis.
2.6 Continuous monitoring of electrocardiogram, blood pressure, and actimetry
Measurements were performed using the SOMNOtouch™ (SOMNOmedics®, Randersacker, Germany) at four time points: once at Pre and once at the end of each month of HDBR (M1, M2, and M3). This system allows a beat-by-beat estimation of blood pressure based on the measurement of the pulse transit time (Bilo et al., 2015).
The SOMNOtouch™ was placed a few hours (0–2 h) before bedtime and was removed the following evening. Brachial blood pressure for calibration was measured using an Omron automatic blood pressure monitor. Cardiovascular variables (R-R interval, SBP, and DBP) and activity (wrist monitor) were recorded continuously for 21 h, from 21:00 to 18:00. The average values per hour were extracted.
To calculate “day” and “night” mean values, the 23:00–05:00 period was considered “night,” and 07:00–17:00 was considered “day.” The 22:00 and 06:00 points were excluded from the averages to avoid artifacts during these transition periods.
2.7 Body mass, body composition, and fluid compartments
Bioimpedance measurements using a multifrequency impedance device (ImpediMed SFB7, Carlsbad) were performed at Pre, D30, D57, and D85 in the morning before breakfast and after voiding. The subjects were weighed prior to measurements, and body mass data were used for calculations. Total body water (TBW), extracellular fluid (ECF), intracellular fluid (ICF), fat mass (FM), and lean mass (LM) were estimated.
2.8 Thigh and calf circumferences
Thigh and calf circumferences were measured in the −6° position using inelastic tapeline with the same topography, respectively, to the distal apex of the patella at Pre, D28, D56, and D84. Measurements for the two legs were averaged.
2.9 Statistical analysis
Data are presented as the mean ± SEM. Three-way ANOVA or 2-way ANOVA for repeated measures was used, with bedrest (measurement time points) and position (supine and tilt) as the within-subject factors and the countermeasure (CON, RES, and RUN) as the between-subject factor. Statistically significant differences were further analyzed by two-sided pairwise multiple comparisons with Sidak correction. Relationships between variables were examined using the Pearson correlation coefficient (r). Orthostatic tolerance (finisher and nonfinisher numbers across groups) was analyzed using the chi-square test. Differences were considered statistically significant when the adjusted p ≤ 0.05. Analyses were performed using Prism GraphPad 9.0.2.
3 Results
3.1 General data
All subjects completed the entire protocol without significant medical or psychological issues. All subjects completed all exercise sessions. HR and BP remained within normal limits.
3.2 Maximal oxygen uptake
With HDBR,
In RES,
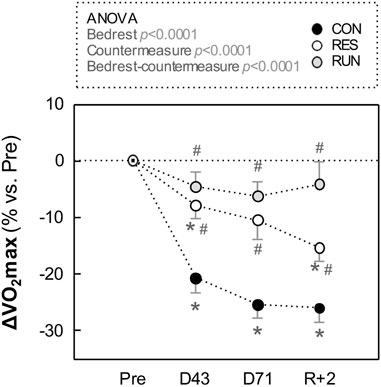
FIGURE 3. Evolution in maximal oxygen uptake (V̇O2max) during HDBR. Measurements were performed in the –6° position using a bicycle ergometer test before (Pre), during (at day 43 and day 71), and after (R + 2: 2nd day of recovery) HDBR. The sample size was n = 8/CON, n = 7/RES, n = 7/RUN. Data are presented as the mean ± SEM. *p < 0.05 vs. Pre; #p < 0.05 vs. CON.
3.3 Tilt test
3.3.1 Orthostatic tolerance
Pre-HDBR, all subjects finished a 30-min upright period, except one in the CON group who became intolerant at the 27th min. Post-HDBR, there were 2 non-finishers out of 8 in CON, 2 out of 7 in RES, and 4 out of 7 in RUN (Figure 4A). The number of nonfinishers post-HDBR did not differ statistically significantly across the groups (chi-square test p = 0.38). The post-HDBR orthostatic tolerance time statistically significantly decreased only for RUN and was 24 ± 4 min for CON, 25 ± 3 min for RES, and 16 ± 5 min for RUN, without statistically significant difference between groups. Irrespective of the group, post-HDBR nonfinishers (n = 8, height 172 ± 1 cm) were significantly taller than finishers (n = 14, height 168 ± 1 cm) (unpaired t-test p = 0.01; Figure 4B).
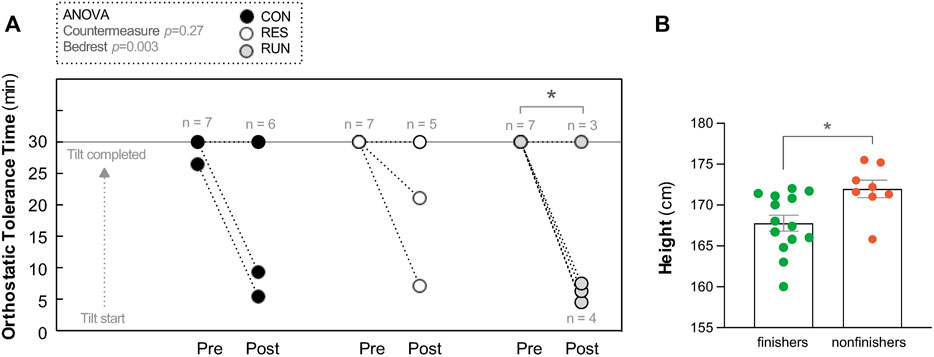
FIGURE 4. Orthostatic tolerance during a 30-min 75° head-up tilt test. Orthostatic tolerance time (OTT) (A). Tilt was performed before (Pre) and immediately after (Post) 90-day head-down bedrest. Each circle represents the individual OTT. *p < 0.05 vs. OTT Pre. Body height of finishers vs. non-finishers post-HDBR (B) (all groups pooled together). Data are presented as the mean ± SEM, *p < 0.05 vs. finishers.
3.3.2 Hemodynamic and autonomic responses to tilt
Before bedrest, upright positioning induced the expected changes in central hemodynamics (increased HR and decreased SV), as well as in HRV spectral power (decreased HF, increased LF, and increased LF/HF ratio). SBP and DBP showed a slight increase of 7–8 mmHg, without statistical significance (Figures 5C,E; Table 2).
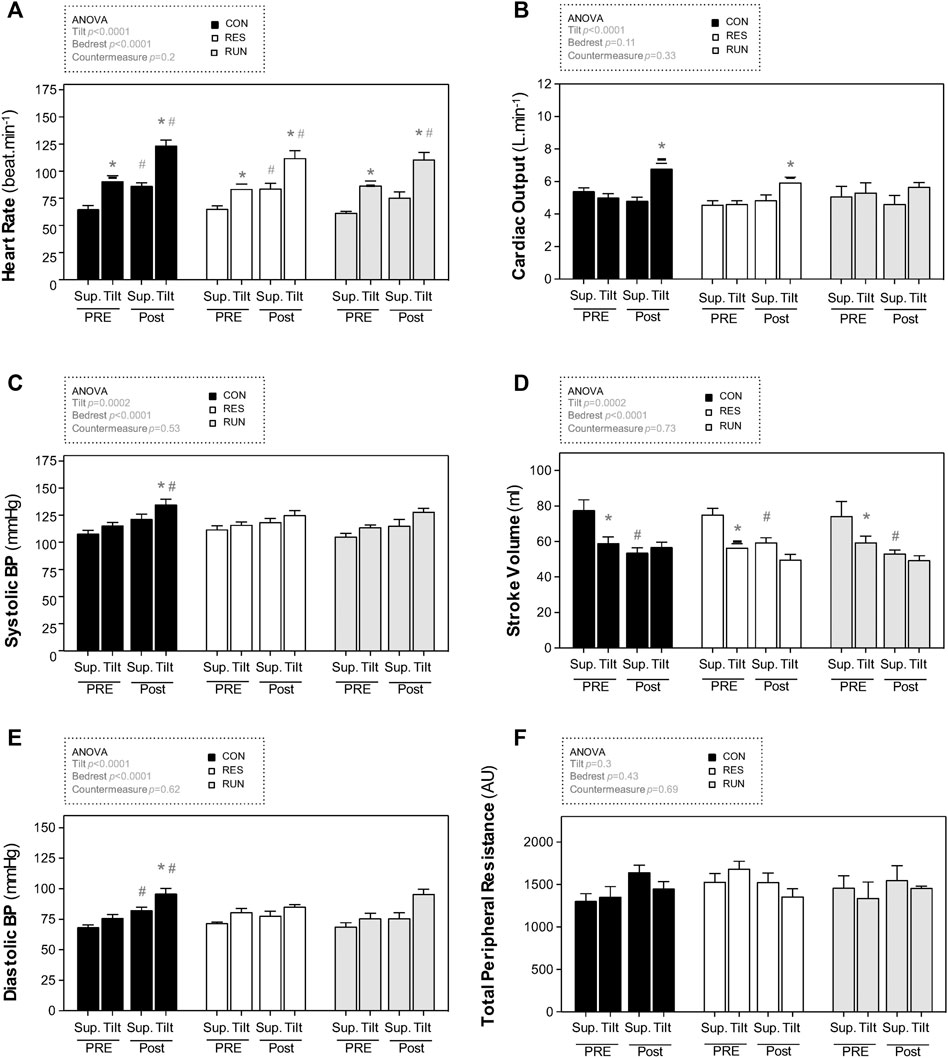
FIGURE 5. Hemodynamic responses to a 30-min 75° head-up tilt test. The tilt test was performed before (Pre) and immediately after (Post) 90-day head-down bedrest. End-supine (Sup) and end-tilt (Tilt) heart rate (A), cardiac output (B), systolic blood pressure (C), stroke volume (D), diastolic blood pressure (E), and total peripheral resistance (F) are shown. The sample size was n = 8/CON, n = 7/RES, n = 7/RUN. Data are presented as the mean ± SEM. *p < 0.05 vs. Supine; #p < 0.05 vs. Pre.
Postbedrest (R0), supine measurements showed a ∼34% increase in HR (from 65 ± 3 to 87 ± 3 bpm in controls), a ∼31% decrease in SV (from 78 ± 6 ml to 54 ± 3 ml in controls), and a 3-fold increase in the LF/HF ratio. Supine BP slightly increased (by 8–15 mmHg), significantly for DBP; supine TPR and CO were not significantly modified. Countermeasures had no significant effect (Figure 5; Table 2).
Postbedrest upright measurements showed a ∼36% increase in upright HR compared to Pre (from 91 ± 5 to 124 ± 5 bpm in controls). Upright BP increased (SBP from 116 ± 3 to 135 ± 5 mmHg and DBP from 76 ± 3 to 96 ± 4 mmHg in controls). Unlike that at prebedrest, postbedrest upright SV (already low when supine) did not further decrease with orthostasis (from 59 ± 3 to 57 ± 3 ml in controls). However, we observed an increase in post-BR upright CO. The LF/HF ratio post bedrest was already increased when supine, and its further increase in response to orthostasis was blunted post-HDBR. Countermeasures had no significant effect on hemodynamic and autonomic responses to tilt (Figure 5; Table 2).
3.4 Continuous monitoring of electrocardiogram, blood pressure, and actimetry
Circadian profiles of BP (shown for SBP, Figure 6) and HR (not shown) demonstrated the expected circadian variance, seemingly not much affected by HDBR. For SBP, day–night difference was preserved (nocturnal SBP dipping of 10 ± 1% or ∼12 mmHg throughout the protocol). For the R-R interval, the day–night difference was somewhat flattened under HDBR (from 225 ± 23 ms at Pre to 161 ± 17 ms at M3 in CON, corresponding to decrease in nocturnal HR dipping from 22 ± 1% to 17 ± 1%) (Figures 6, 7A,B).
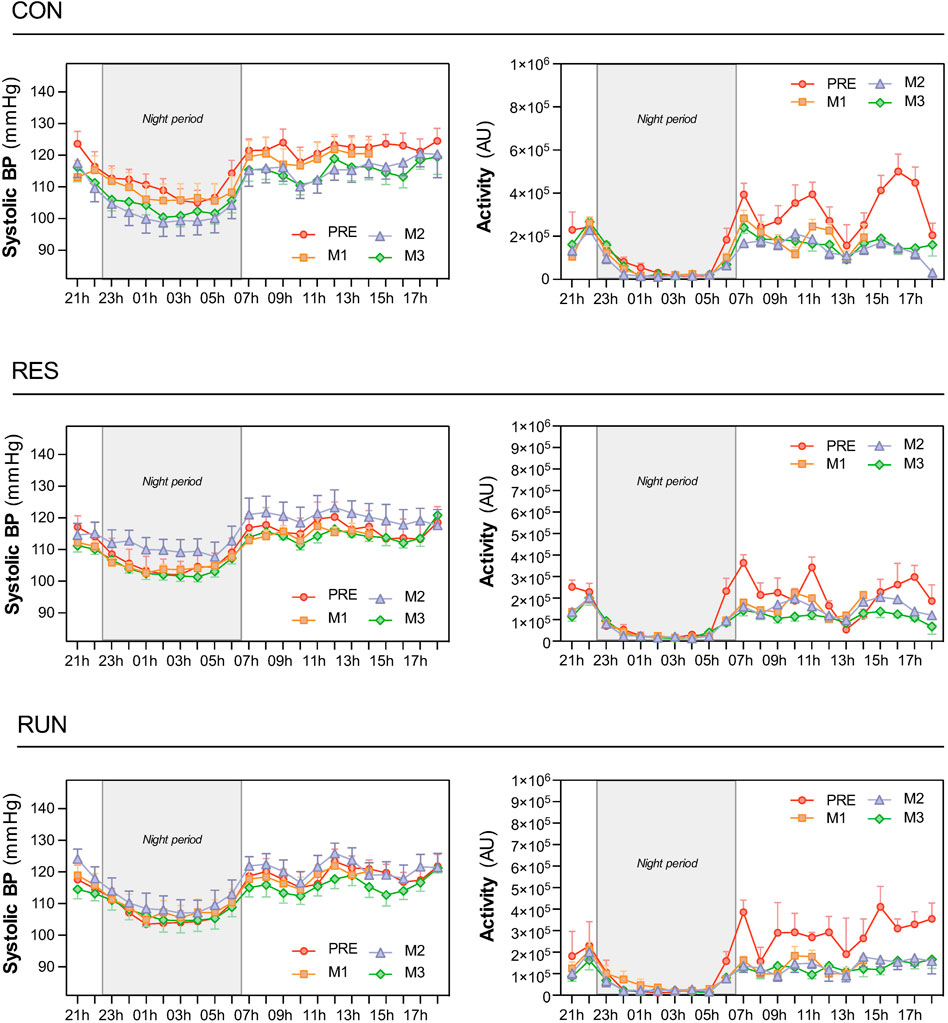
FIGURE 6. Circadian patterns of systolic blood pressure and physical activity. Recordings were performed using the SOMNOtouch™ device over 21 h, before HDBR and at the end of each month (M1, M2, and M3). The night period was 22:30–06:30. The sample size was n = 8/CON, n = 7/RES, n = 7/RUN. Data are presented as the mean ± SEM.
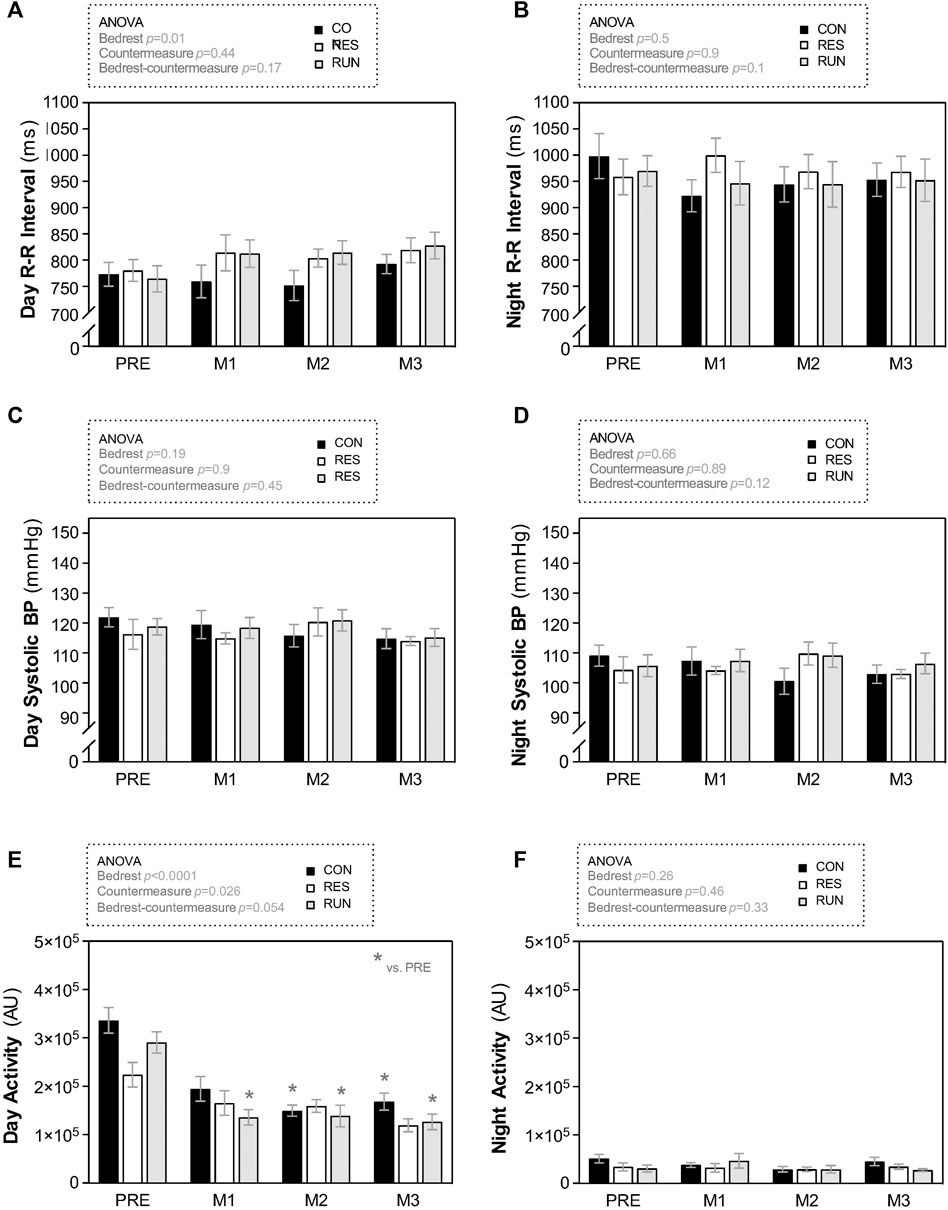
FIGURE 7. Evolution in fluid compartments, body mass, and body composition during HDBR. Fluid compartments (A,C,D) body mass used for bioimpedance measurements (B), and body composition (E,F) were estimated by bioimpedance measurements using a multifrequency impedance device. The subjects were weighed prior to measurement, and body mass data used for calculations. The sample size was n = 8/CON, n = 7/RES, n = 7/RUN. Data are presented as the mean ± SEM. *p < 0.05 vs. Pre; #p < 0.05 vs. CON.
Circadian BP decreased during HDBR in CON (reduction of 6–7 mmHg for systolic), although without reaching statistical significance for “day” and “night” mean values. In the countermeasure groups, this decreasing pattern was less evident, although the SBP curve still seemed to be lowest at M3.
The activity level dropped as expected during bedrest and was extremely reduced at night (Figures 6, 7E,F).
The R-R interval and SBP, as estimated by “day” and “night” mean values, did not differ between groups during bedrest (Figures 7A–D).
3.5 Body mass, body composition, and fluid compartments
Results are presented as percentage changes in Figure 8 and as changes in native scales in Supplementary Data S2. The body mass of the CON tended to progressively decrease throughout the HDBR (∼2 kg loss at D85), although without reaching statistical significance. Body mass in the countermeasure groups remained rather stable (Figure 8B).
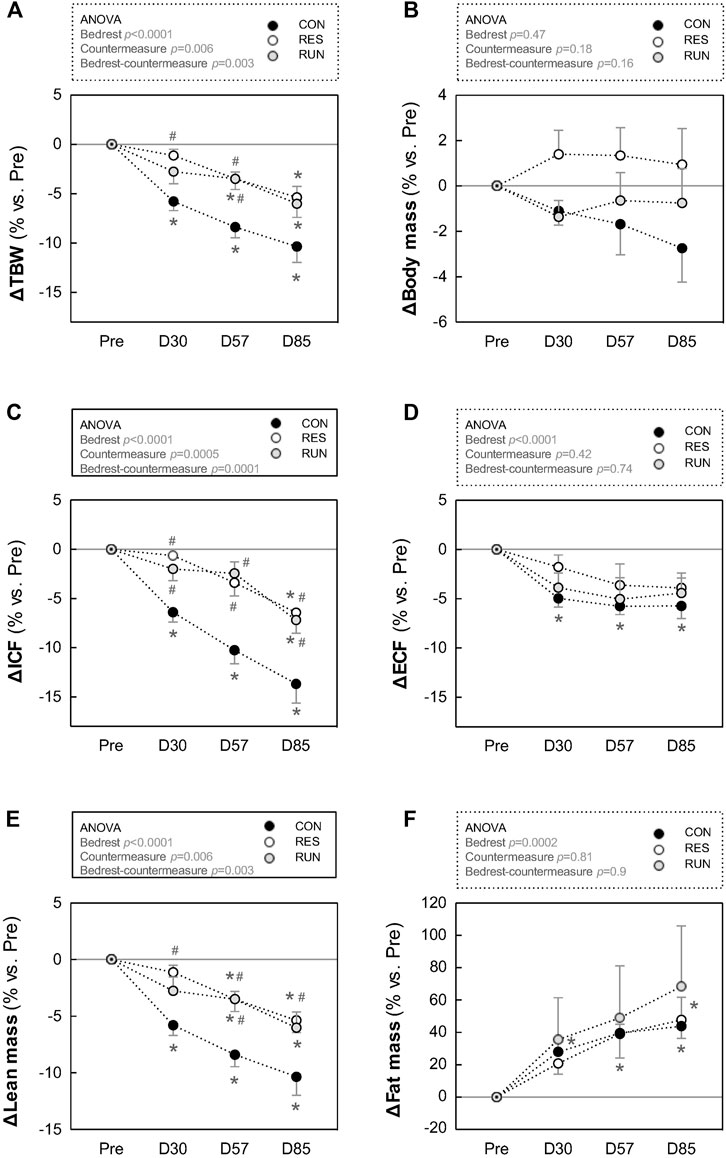
FIGURE 8. Average day and night R-R interval (A,B), systolic blood pressure (C,D), and physical activity (E,F). To calculate mean “day” and “night” values, 23:00–05:00 was considered “night,” and 07:00–17:00 was considered “day.” The 22:00 and 06:00 points were excluded from the averages to avoid artifacts during these transition periods. Recordings were performed before HDBR and at the end of each month (M1, M2, and M3). The sample size was n = 8/CON. Data are presented as the mean ± SEM. *p < 0.05 vs. Pre.
LM in CON gradually decreased during HDBR. LM loss was 2–4 kg in the first month and 5–7 kg at the end of HDBR. This loss in LM was reduced by half in both countermeasure groups (10% loss in CON vs. 5%–6% loss in RES and RUN at the end of HDBR) (Figure 8E).
FM gradually increased during HDBR without a significant difference between groups. By the end of HDBR, subjects gained 3–4 kg in FM (∼9 kg of estimated FM at baseline vs. ∼12–13 kg at D85) (Figure 8F).
TBW in CON gradually decreased, by ∼6% at M1 and ∼10% at M3. This decrease occurred initially (at M1) at the expense of both ECF and ICF compartments and then (at M2–M3) mostly at the expense of the ICF compartment (Figure 8A).
Both countermeasures reduced the losses in TBW and ICF by half (TBW: 10% loss in CON vs. 5%–6% loss in RES and RUN at the end of HDBR; ICF: 14% loss in CON vs. 6%–7% loss in RES and RUN at the end of HDBR). The loss in ECF (4–6% at M3) was not significantly different between groups (Figures 8C,D).
3.6 Thigh and calf circumferences
Results are presented as percentage changes in Figure 9 and as changes in native scales in Supplementary Data S3. Thigh and calf circumferences progressively decreased during HDBR. Most of the changes occurred in the first 2 months and reached 7.4 ± 1.0% loss for the thigh and 10.2 ± 0.5% loss for the calf in the CON group at the end of bedrest. Exercise countermeasures counteracted this decrease, more efficiently at the thigh than at the calf (loss in thigh circumference at M3 was nonsignificant for both countermeasure groups), and more efficiently in RUN than in RES (average loss in calf circumference at M3 was −10.2% in CON, −7.2% in RES, and −5.1% in RUN) (Figure 9).
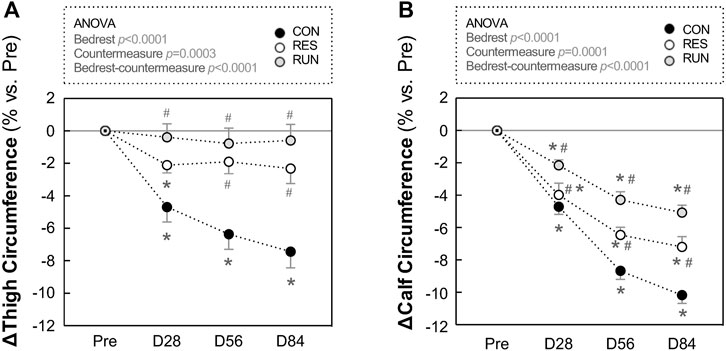
FIGURE 9. Evolution in thigh and calf circumferences during HDBR. Thigh (A) and calf (B) circumferences were measured in the –6° position. The sample size was n = 8/CON, n = 7/RES, n = 7/RUN. Data are presented as the mean ± SEM. *p < 0.05 vs. Pre; #p < 0.05 vs. CON.
3.7 Correlations between changes in maximal oxygen uptake and muscular and cardiovascular variables
To gain insight into parameters that could be related to the pre-to-post changes in
To explore relations between changes in
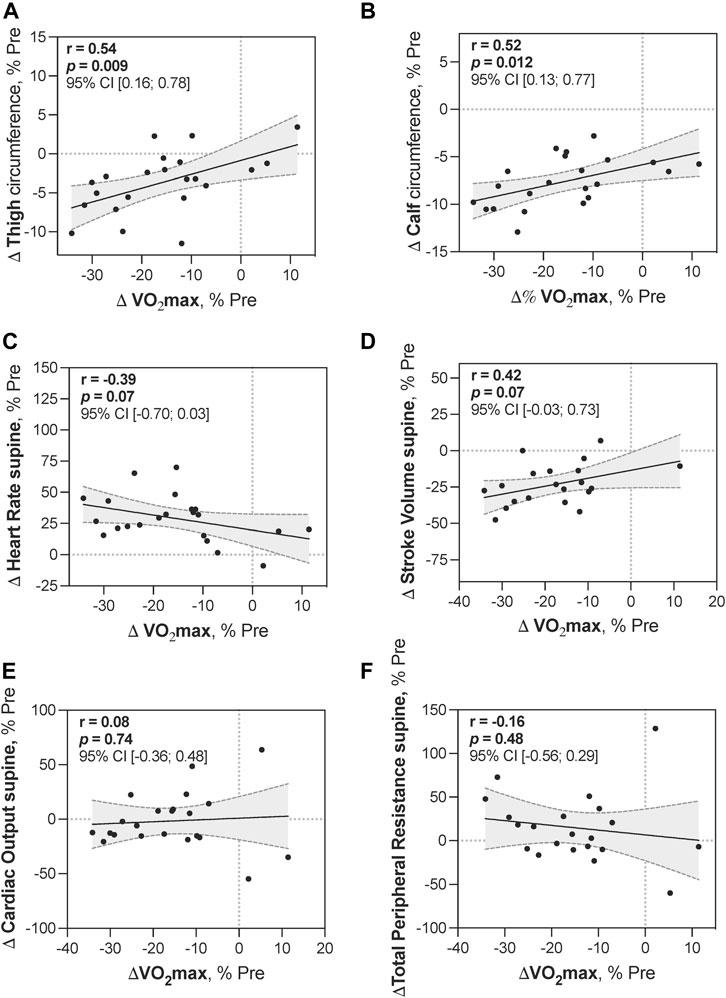
FIGURE 10. Correlations between changes in V̇O2max and muscular and cardiovascular variables. Correlations between relative changes in
Reductions in leg circumferences at D84 showed relatively strong positive correlations with a decrease in
4 Discussion
For the first time, resistance exercise and running countermeasures were tested separately and compared directly during the same long-term HDBR. The main finding is that running countermeasure preserved
Our discussion will mainly focus on comparison with the results of other long-term bedrest protocols (>1 month). Some of them have tested combined physical exercise countermeasures (Trappe et al., 2007; Schneider et al., 2009; Coupé et al., 2011; Lee et al., 2014; Ploutz-Snyder et al., 2018).
4.1 Effects of prolonged strict HDBR
4.1.1 Maximal oxygen uptake
We aimed to explore
In long-term spaceflights, a maximal decrease in
4.1.2 Orthostatic tolerance during tilt test
In our study, 90 days of strict bedrest did not significantly reduce the tolerance time, although two subjects in the control group did not reach 10 min in the orthostatic position during the tilt post-HDBR (one of them was nonfinisher pre-HDBR). Interestingly, in a previous 60-day HDBR with Chinese subjects, tolerance was also preserved (Coupé et al., 2011). That 60-day HDBR was not strict—subjects were allowed to stand briefly in the morning and in the evening for hygiene. Our 90-day study was a strict HDBR, so a short period of orthostatic stimulation could not be evoked. As orthostatic tolerance is dependent on head-to-heart distance, shorter subjects might better tolerate orthostasis (Ludwig and Convertino, 1994; Pavy-Le-Traon et al., 1999). In line with this, Table 4 summarizes the characteristics of the subjects in different studies who participated in long-term bedrest, suggesting that shorter subjects are more tolerant of tilt.
4.1.3 Body composition: Muscle atrophy and gain in fat
Body mass did not change significantly, but mass repartition shifted from lean to fat, together with a decrease in leg circumferences, presumably due to enhanced inactivity.
Considering leg muscle loss, previous HDBR studies using magnetic resonance imaging (MRI) found, in no-exercise groups, a volume loss for quadriceps of −18% (90-d HDBR in men) (Alkner and Tesch, 2004) and −21% (60-d HDBR in women) (Trappe et al., 2007); for triceps surae, both studies found a loss of −29%. In our study, as change in volume for the leg segment (roughly cylindrical) is proportional to the squared change in circumference, in the control group, estimated volume loss consisted of −14% for the thigh and −19% for the calf. Taken together, this suggests that calf muscles are more influenced by long-term HDBR than thigh ones. Other studies also noted a hierarchy of greater atrophy in the extensors of the calf, thigh, and then hip musculature (Belavý et al., 2009). Lesser values for our study might be explained by difference in methods (direct MRI measurement vs. approximation via circumference measurement), and also by difference in muscles taken into account (targeted measure of extensors which are more affected by unloading vs. the total cross-section).
4.1.4 Cardiovascular response during tilt test
An observed increase in resting and upright HR post-HDBR is commonly reported after spaceflight and microgravity simulations as a part of cardiovascular deconditioning syndrome. The post-HDBR increase in resting BP before orthostatic testing, frequently seen in bedrest protocols, could be associated with the stress of the first orthostatic challenge after prolonged bedrest (Traon et al., 1998). Decrease in resting SV (of ∼30%) reflects bedrest-induced hypovolemia.
4.1.5 Day–night heart rate and blood pressure
Day–night rhythms of HR and BP were visibly preserved. Of note, for technical reasons, we were limited to continuous recordings of 21 h. We did not perform specific circadian analysis. However, a recent study specifically evaluating circadian rhythms of R-R intervals in 5-, 21-, and 60-day HDBR using Cosinor analysis (Solbiati et al., 2021) revealed flattening of day–night oscillations for R-R intervals progressing with the duration of bedrest. These results are in agreement with our findings.
Interestingly, circadian blood pressure in the present study exhibited the same decreasing pattern in the control group during HDBR (drop of 6–7 mmHg for systolic blood pressure) as that observed in long-duration spaceflight [8–10 mmHg reported by Norsk et al. (2015)] (Figure 11). The healthy night “dip” in systolic and diastolic blood pressure is preserved in bedrest, as was also observed by Norsk et al. (2015) in real microgravity. This reinforces our belief that long-term ground-based microgravity simulations are useful in the context of preparing for missions to the Moon and Mars.
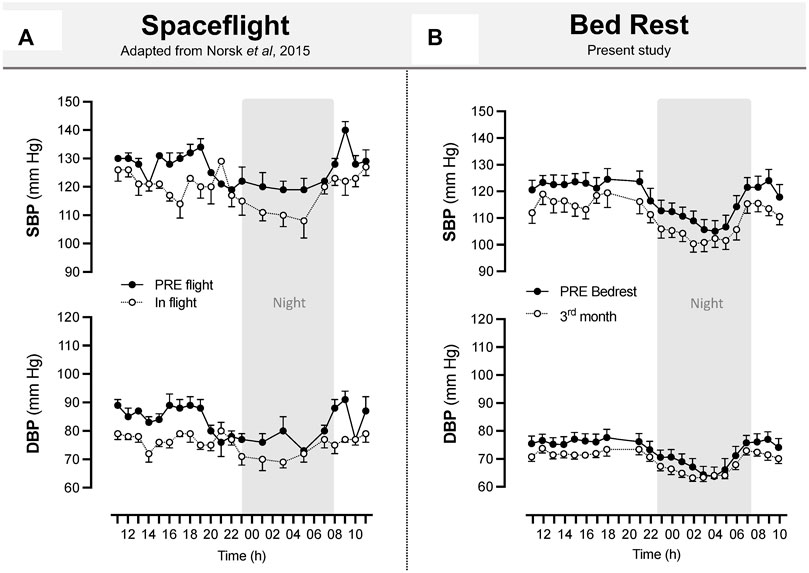
FIGURE 11. Space flight and long-term bedrest similarly induced a decrease in systolic and diastolic blood pressure. Systolic and diastolic blood pressure during spaceflight (A). Upper arm artery blood pressure was measured between 85 and 192 days of flight. Recordings are hourly during the day and bihourly during the night over 11:00–11:00. The sample size was n = 8, and data are presented as the mean ± SEM. Adapted from Norsk et al., 2015. Systolic and diastolic blood pressure during 90-day bedrest (B) estimated from Pulse Transit Time using the SOMNOtouch™ device. Recordings are beat by beat over 21:00–18:00. The sample size was n = 8 (CON), and data are presented as the mean ± SEM from the present study.
4.2 Effects of physical exercise countermeasures
4.2.1 Muscular system and body composition
In space, training is seen as a holistic method and used in an integrative way, with aerobic, mainly locomotor, modality to maintain the cardiorespiratory fitness and resistance modality focusing on strength and muscle size. With future goals of reaching the Moon and Mars, solutions to apply both aerobic and resistance exercise modalities inevitably present issues. These primarily relate to reducing the mass, size, energy use, heat production, and cost of devices (Steele et al., 2019).
Leg muscles are more strongly affected in microgravity, as astronauts still use their arms and hands to conduct daily routines and navigate across the ISS. In this study, RUN and RES countermeasures halved LM loss and limited lower limb circumferences loss. Consistently, after 60 days of bedrest in women, DEXA measurements revealed significantly greater leg lean mass in the running + resistance exercise group (supine treadmill within LBNP 3–4 days/wk and supine leg- and calf-press exercises 2–4 days/wk) than in no-exercise controls (12.5 vs. 11.6 kg) (Lee et al., 2014), and whole-body lean tissue mass loss was limited (∼5% loss in controls vs. ∼2% loss in exercise group) (Schneider et al., 2009).
4.2.2 O2max
Changes in the muscular
The present study is the first to directly compare running alone (without being coupled to nutritional, pharmacological, or other exercise countermeasures) and resistance exercise alone. We showed that the RUN succeeded in maintaining the baseline
4.2.3 Orthostatic tolerance, muscle mass, indices of CV, and autonomic fitness
The RUN group had four intolerant subjects post-HDBR compared with 2 in CON and 2 in RES. Neither countermeasure counteracted orthostatic intolerance. Of note, exercise training might increase limb compliance and thus reduce orthostatic tolerance; on the other hand, if initial tolerance is low, moderate exercise might also improve it (Pavy-Le-Traon et al., 2007). In any case, the subjects of all 3 groups maintained rather good orthostatic tolerance after 90 days of strict bedrest.
Both countermeasure groups lost less global lean mass and leg circumferences than the control group, so these types of exercise could limit muscle wasting.
In our study, both resistance and running countermeasures had no effect on volemia (SV) and the hemodynamic and autonomic indices associated with cardiovascular fitness. Consistently, previous 90-day bedrest with the same resistance exercise device (flywheel) also failed to improve these cardiovascular parameters (Belin de Chantemele et al., 2004). In another study (60-day bedrest), resistance exercise combined with vibration prevented an increase in the sympathetic index and limited a drop in baroreflex sensitivity (Coupé et al., 2011), although it had no effect on orthostatic tolerance. Furthermore, aerobic exercise coupled with restoration of plasma volume maintained orthostatic tolerance after 18 days of bedrest (Shibata et al., 2010).
Exercise intensity in our protocol might appear insufficient in terms of time and/or power. Thus, on the ISS, astronauts perform 1 h of resistance and 30–45 min of locomotor aerobic exercises 6 days/wk (Wang et al., 2019). In addition, a combination of countermeasures is conceivable. According to Wang et al. (2019), artificial gravity is considered the first option for the novel concept of countermeasures for Chinese long-term spaceflight. In fact, artificial gravity protocols were able to limit orthostatic tolerance loss after short bedrest (Stenger et al., 2012; Li et al., 2017). However, they seemed less efficient in abolishing cardiovascular deconditioning (in terms of tachycardia, reduction in LVEDV, CO, and SV) after long-term HDBR (Hoffmann et al., 2021).
In the current bedrest, a novel combined resistance exercise platform has been tested. Another flywheel exercise device, developed in Sweden by Alkner and Tesch almost 20 years ago, has been tested in bedrest studies and appeared very efficient in conserving the knee extensor but not the knee flexor muscles during 90-day HDBR (LTBR study, France, 2001–2002, n = 9 in Flywheel exercise group and n = 16 in inactive group, Belavý et al., 2017).
Comparison between these two systems is clearly limited by the differences in protocols as well as by the absence of direct results on muscles in the current study. However, in terms of time spent on resistive exercise, LTBR subjects exercised for 20 min every third day, that is, 2-3 times/wk, which seems less than our RES subjects (for 45 min 3 times/wk). In terms of efficiency, reducing muscle atrophy seems comparable. Indeed, for calf muscles, LTBR resistive exercise reduced soleus loss by ∼31% (20% volume loss for flywheel group vs. 29% loss in inactive group), whereas in the current bedrest, RES exercise reduced calf volume loss by ∼26% (14% estimated loss in RES vs. 19%—in Controls). For thigh muscles, LTBR, resistive exercise reduced vasti loss by ∼80% (4% volume loss for flywheel group vs. 20% loss in inactives). In our bedrest, RES exercise reduced thigh volume loss by ∼71% (4% estimated loss in RES vs. 14%—in Controls).
It should be recognized that the effect of any training depends not only on its modality but also on the specific parameters of the exercises used (mode, intensity, and volume). For example, as shown in a 370-day 4.5° HDBR with 10 healthy men, intense treadmill training can overshadow the effect of weak resistance training (Grigoriev and Kozlovskaya 2018). So we can compare for the chosen modes of running and resistance training. But all specific parameters of exercise are extremely important, and this makes for very delicate comparison of exercise countermeasures in general.
4.3 Variables potentially related to maximal oxygen uptake decrease
The decrease in
Thus, higher correlation coefficients observed with muscular parameters indicate a stronger dependency of
During bedrest, the early
4.4 Limitations
Several limitations should be noted in this study. The main concern is the lack of direct measures for muscle outcomes (using MRI, DEXA, etc.). Indeed, leg circumferences are not a strong measure of regional muscle mass, cross-sectional area, or architecture. The sample size was limited to eight subjects in the control group and seven in the exercise groups. This small n has an impact on statistical power. Additionally, plasma volume was not measured directly, so we used resting stroke volume changes as an indirect reflection of plasma volume changes, but this remains a simple estimation and not an actual measurement. Moreover, we did not perform cardiac structure tests (especially assessments of left ventricular mass evolution) and thus did not study the role of eventual cardiac muscle atrophy in
5 Conclusion
During this 90-day strict head-down bedrest, running exercise countermeasure preserved
Data availability statement
The raw data supporting the conclusion of this article will be made available by the authors, without undue reservation.
Ethics statement
The studies involving human participants were reviewed and approved by the Ethics Committee of the Astronaut Center of China (ACC). The patients/participants provided their written informed consent to participate in this study.
Author contributions
YL, M-AC, GG-K, and J-CL took part in the study design. LW conducted the tilt test and data processing; JL conducted the tilt test and data check; YM and AR conducted SOMNOtouch™ data recording; ZL and HW conducted the cardiopulmonary test; XD conducted oxygen uptake data processing; KL conducted body composition measurement; WL conducted leg circumference measurement; SL, JZ, KL, and XL managed exercise countermeasures; LQ performed data monitoring. AR, NN, and M-AC performed data processing and analysis and interpreted the results. AR, NN, M-AC, and LW drafted the manuscript. GG-K, J-CL, and YL performed manuscript revision.
Funding
This work was funded by the China Manned Space Advanced Research Project (HY-5), the Key Project of the State Key Laboratory of Space Medicine Fundamentals and Application (SMFA18A01), and the Space Medical Experimental Project (HYZHXM01001). AR received a PhD grant from CNES and Région des Pays de la Loire, France. This work was also funded by the French Space Agency (CNES—DAR n 2019/4300001021).
Acknowledgments
We thank the participants and the personnel of the Space Institute of Southern China who assisted with the experiment, Fanfan Cui for assistance with the SOMNOtouch™ data recording, and Dong Xu, Miaohong Chen, and Hongzhi Shi for medical support during the tilt test.
Conflict of interest
The authors declare that the research was conducted in the absence of any commercial or financial relationships that could be construed as a potential conflict of interest.
Publisher’s note
All claims expressed in this article are solely those of the authors and do not necessarily represent those of their affiliated organizations, or those of the publisher, the editors, and the reviewers. Any product that may be evaluated in this article, or claim that may be made by its manufacturer, is not guaranteed or endorsed by the publisher.
Supplementary material
The Supplementary Material for this article can be found online at: https://www.frontiersin.org/articles/10.3389/fphys.2022.902983/full#supplementary-material
Abbreviations
ACC, Astronaut Center of China; BP, blood pressure; CM, countermeasure; CO, cardiac output; CON, control group; DBP, diastolic blood pressure; ECF, extracellular fluid; ECG, electrocardiogram; FM, fat mass; HDBR, head-down bedrest; HF, high frequency; HR, heart rate; HRV, heart rate variability; ICF, intracellular fluid; ISS, International Space Station; LBNP, lower body negative pressure; LM, lean mass; LF, low frequency; OTT, orthostatic tolerance time; RES, resistance exercise group; RUN, running exercise group; SBP, systolic blood pressure; SEM, standard error of the mean; Sup, supine; SV, stroke volume; TBW, total body water; TPR, total peripheral resistance.
References
Adami A., Pizzinelli P., Bringard A., Capelli C., Malacarne M., Lucini D., et al. (2013). Cardiovascular re-adjustments and baroreflex response during clinical reambulation procedure at the end of 35-day bed rest in humans. Appl. Physiol. Nutr. Metab. 38, 673–680. doi:10.1139/apnm-2012-0396
Alkner B. A., Tesch P. A. (2004). Knee extensor and plantar flexor muscle size and function following 90 days of bed rest with or without resistance exercise. Eur. J. Appl. Physiol. 93, 294–305. doi:10.1007/s00421-004-1172-8
Belavý D. L., Miokovic T., Armbrecht G., Richardson C. A., Rittweger J., Felsenberg D., et al. (2009). Differential atrophy of the lower-limb musculature during prolonged bed-rest. Eur. J. Appl. Physiol. 107, 489–499. doi:10.1007/s00421-009-1136-0
Belavý D. L., Ohshima H., Rittweger J., Felsenberg D. (2017). High-intensity flywheel exercise and recovery of atrophy after 90 days bed--rest. BMJ Open Sport Exerc. Med. 3 (1), e000196. doi:10.1136/bmjsem-2016-000196
Belin de Chantemele E., Blanc S., Pellet N., Duvareille M., Ferretti G., Gauquelin-Koch G., et al. (2004). Does resistance exercise prevent body fluid changes after a 90-day bed rest? Eur. J. Appl. Physiol. 92, 555–564. doi:10.1007/s00421-004-1121-6
Bilo G., Zorzi C., Ochoa Munera J. E., Torlasco C., Giuli V., Parati G. (2015). Validation of the Somnotouch-NIBP noninvasive continuous blood pressure monitor according to the European Society of Hypertension International Protocol revision 2010, Blood Press Monit. 20, 291–294. doi:10.1097/MBP.0000000000000124
Capelli C., Antonutto G., Kenfack M. A., Cautero M., Lador F., Moia C., et al. (2006). Factors determining the time course of VO2(max) decay during bedrest: Implications for VO2(max) limitation. Eur. J. Appl. Physiol. 98, 152–160. doi:10.1007/s00421-006-0252-3
Coupé M., Yuan M., Demiot C., Bai Y. Q., Jiang S. Z., Li Y. Z., et al. (2011). Low-magnitude whole body vibration with resistive exercise as a countermeasure against cardiovascular deconditioning after 60 days of head-down bed rest. Am. J. Physiol. Regul. Integr. Comp. Physiol. 301, R1748–R1754. doi:10.1152/ajpregu.00234.2011
Fu Q., Shibata S., Hastings J. L., Platts S. H., Hamilton D. M., Bungo M. W., et al. (2019). Impact of prolonged spaceflight on orthostatic tolerance during ambulation and blood pressure profiles in astronauts. Circulation 140, 729–738. doi:10.1161/CIRCULATIONAHA.119.041050
Grigoriev A. I., Kozlovskaya I. B., One-year antiorthostatic hypokinesia (ANOG) – a physiological model of interplanetary space flight (in Russian), (2018). (Monograph) (Moscow: Russian academy of sciences). 978-5-907036-40-6.
Hoffmann F., Rabineau J., Mehrkens D., Gerlach D. A., Moestl S., Johannes B. W., et al. (2021). Cardiac adaptations to 60 day head-down-tilt bed rest deconditioning. Findings from the AGBRESA study. Esc. Heart Fail. 8, 729–744. doi:10.1002/ehf2.13103
Hoffmann U., Moore A. D., Koschate J., Drescher U. (2016). V̇O2 and HR kinetics before and after international space station missions. Eur. J. Appl. Physiol. 116, 503–511. doi:10.1007/s00421-015-3298-2
Kozlovskaya I. B., Yarmanova E. N., Yegorov A. D., Stepantsov V. I., Fomina E. V., Tomilovaskaya E. S., et al. (2015). Russian countermeasure systems for adverse effects of microgravity on long-duration ISS flights. Aerosp. Med. Hum. Perform. 86, A24-A31. doi:10.3357/AMHP.EC04.2015
Kramer A., Gollhofer A., Armbrecht G., Felsenberg D., Gruber M. (2017). How to prevent the detrimental effects of two months of bed-rest on muscle, bone and cardiovascular system: An RCT. Sci. Rep. 7, 13177. doi:10.1038/s41598-017-13659-8
Lee S. M., Schneider S. M., Boda W. L., Watenpaugh D. E., Macias B. R., Meyer R. S., et al. (2007). Supine LBNP exercise maintains exercise capacity in male twins during 30-d bed rest. Med. Sci. Sports Exerc. 39, 1315–1326. doi:10.1249/mss.0b013e31806463d9
Lee S. M., Moore A. D., Everett M. E., Stenger M. B., Platts S. H. (2010). Aerobic exercise deconditioning and countermeasures during bed rest. Aviat. Space Environ. Med. 81, 52–63. doi:10.3357/asem.2474.2010
Lee S. M., Schneider S. M., Boda W. L., Watenpaugh D. E., Macias B. R., Meyer R. S., et al. (20091985). LBNP exercise protects aerobic capacity and sprint speed of female twins during 30 days of bed rest. J. Appl. Physiol. 106, 919–928. doi:10.1152/japplphysiol.91502.2008
Lee S. M., Schneider S. M., Feiveson A. H., Macias B. R., Smith S. M., Watenpaugh D. E., et al. (20141985). WISE-2005: Countermeasures to prevent muscle deconditioning during bed rest in women. J. Appl. Physiol. 116, 654–667. doi:10.1152/japplphysiol.00590.2013
Li X. T., Yang C. B., Zhu Y. S., Sun J., Shi F., Wang Y. C., et al. (2017). Moderate exercise based on artificial gravity preserves orthostatic tolerance and exercise capacity during short-term head-down bed rest. Physiol. Res. 66 (4), 567–580. doi:10.33549/physiolres.933493
Ludwig D. A., Convertino V. A. (1994). Predicting orthostatic intolerance: Physics or physiology? Aviat. Space Environ. Med. 65, 404–411.
Meck J. V., Reyes C. J., Perez S. A., Goldberger A. L., Ziegler M. G. (2001). Marked exacerbation of orthostatic intolerance after long- vs. short-duration spaceflight in veteran astronauts. Psychosom. Med. 63, 865–873. doi:10.1097/00006842-200111000-00003
Moore A. D., Downs M. E., Lee S. M., Feiveson A. H., Knudsen P., Ploutz-Snyder L., et al. (20141985). Peak exercise oxygen uptake during and following long-duration spaceflight. J. Appl. Physiol. 117, 231–238. doi:10.1152/japplphysiol.01251.2013
Mounier Y., Tiffreau V., Montel V., Bastide B., Stevens L. (20091985). Phenotypical transitions and Ca2+ activation properties in human muscle fibers: Effects of a 60-day bed rest and countermeasures. J. Appl. Physiol. 106, 1086–1099. doi:10.1152/japplphysiol.90695.2008
Norsk P., Asmar A., Damgaard M., Christensen N. J. (2015). Fluid shifts, vasodilatation and ambulatory blood pressure reduction during long duration spaceflight. J. Physiol. 593, 573–584. doi:10.1113/jphysiol.2014.284869
Pavy-Le Traon A., Heer M., Narici M. V., Rittweger J., Vernikos J., PAvy-Le TrAon A. (2007). From space to earth: Advances in human physiology from 20 years of bed rest studies (1986-2006). Eur. J. Appl. Physiol. 101, 143–194. doi:10.1007/s00421-007-0474-z
Pavy-Le Traon A., Louisy F., Vasseur-Clausen P., Güell A., Gharib C., PAvy-Le TrAon A., et al. (1999). Contributory factors to orthostatic intolerance after simulated weightlessness. Clin. Physiol. 19, 360–368. doi:10.1046/j.1365-2281.1999.00189.x
Ploutz-Snyder L. L., Downs M., Goetchius E., Crowell B., English K. L., Ploutz-Snyder R., et al. (2018). Exercise training mitigates multisystem deconditioning during bed rest. Med. Sci. Sports Exerc. 50, 1920–1928. doi:10.1249/MSS.0000000000001618
Ried-Larsen M., Aarts H. M., Joyner M. J. (20171985). Effects of strict prolonged bed rest on cardiorespiratory fitness: Systematic review and meta-analysis. J. Appl. Physiol. 123, 790–799. doi:10.1152/japplphysiol.00415.2017
Rynders C. A., Blanc S., DeJong N., Bessesen D. H., Bergouignan A. (2018). Sedentary behaviour is a key determinant of metabolic inflexibility. J. Physiol. 596, 1319–1330. doi:10.1113/JP273282
Schneider S. M., Lee S. M. C., Macias B. R., Watenpaugh D. E., Hargens A. R. (2009). WISE-2005: Exercise and nutrition countermeasures for upright VO2pk during bed rest. Med. Sci. Sports Exerc. 41, 2165–2176. doi:10.1249/MSS.0b013e3181aa04e5
Shibata S., Perhonen M., Levine B. D. (20101985). Supine cycling plus volume loading prevent cardiovascular deconditioning during bed rest. J. Appl. Physiol. 108, 1177–1186. doi:10.1152/japplphysiol.01408.2009
Solbiati S., Martin-Yebra A., Vaïda P., Caiani E. G. (2021). Evaluation of cardiac circadian rhythm deconditioning induced by 5-to-60 Days of head-down bed rest. Front. Physiol. 11, 612188. doi:10.3389/fphys.2020.612188
Steele J., Androulakis-Korakakis P., Perrin C., Fisher J. P., Gentil P., Scott C., et al. (2019). Comparisons of resistance training and "cardio" exercise modalities as countermeasures to microgravity-induced physical deconditioning: New perspectives and lessons learned from terrestrial studies. Front. Physiol. 10, 1150. doi:10.3389/fphys.2019.01150
Stenger M. B., Evans J. M., Knapp C. F., Lee S. M., Phillips T. R., Perez S. A., et al. (2012). Artificial gravity training reduces bed rest-induced cardiovascular deconditioning. Eur. J. Appl. Physiol. 112, 605–616. doi:10.1007/s00421-011-2005-1
Suzuki Y., Kashihara H., Takenaka K., Kawakubo K., Makita Y., Goto S., et al. (1994). Effects of daily mild supine exercise on physical performance after 20 days bed rest in young persons. Acta Astronaut. 33, 101–111. doi:10.1016/0094-5765(94)90114-7
Tank J., Baevsky R. M., Funtova I. I., Diedrich A., Slepchenkova I. N., Jordan J., et al. (2011). Orthostatic heart rate responses after prolonged space flights. Clin. Auton. Res. 21, 121–124. doi:10.1007/s10286-010-0106-2
Traon A. P., Sigaudo D., Vasseur P., Maillet A., Fortrat J. O., Hughson R. L., et al. (1998). Cardiovascular responses to orthostatic tests after a 42-day head-down bed-rest. Eur. J. Appl. Physiol. Occup. Physiol. 77, 50–59. doi:10.1007/s004210050299
Trappe T. A., Burd N. A., Louis E. S., Lee G. A., Trappe S. W. (2007). Influence of concurrent exercise or nutrition countermeasures on thigh and calf muscle size and function during 60 days of bed rest in women. Acta Physiol. (Oxf). 191, 147–159. doi:10.1111/j.1748-1716.2007.01728.x
Vernice N. A., Meydan C., Afshinnekoo E., Mason C. E. (2020). Long-term spaceflight and the cardiovascular system. Precis. Clin. Med. 3, 284–291. doi:10.1093/pcmedi/pbaa022
Wang L., Li Z., Tan C., Liu S., Zhang J., He S., et al. (2019). Physiological effects of weightlessness: Countermeasure system development for a long-term Chinese manned spaceflight. Front. Med. 13, 202–212. doi:10.1007/s11684-017-0587-7
Keywords: maximal working capacity, countermeasure, microgravity, HDBR, cardiovascular deconditioning, orthostatic tolerance, V̇O2max, oxygen uptake
Citation: Robin A, Wang L, Custaud M-A, Liu J, Yuan M, Li Z, Lloret J-C, Liu S, Dai X, Zhang J, Lv K, Li W, Gauquelin-Koch G, Wang H, Li K, Li X, Qu L, Navasiolava N and Li Y (2022) Running vs. resistance exercise to counteract deconditioning induced by 90-day head-down bedrest. Front. Physiol. 13:902983. doi: 10.3389/fphys.2022.902983
Received: 23 March 2022; Accepted: 29 June 2022;
Published: 31 August 2022.
Edited by:
Karen R. Kelly, Naval Health Research Center, United StatesReviewed by:
Jochen Zange, German Aerospace Center (DLR), GermanyOlga Vinogradova, Institute of Biomedical Problems (RAS), Russia
Copyright © 2022 Robin, Wang, Custaud, Liu, Yuan, Li, Lloret, Liu, Dai, Zhang, Lv, Li, Gauquelin-Koch, Wang, Li, Li, Qu, Navasiolava and Li. This is an open-access article distributed under the terms of the Creative Commons Attribution License (CC BY). The use, distribution or reproduction in other forums is permitted, provided the original author(s) and the copyright owner(s) are credited and that the original publication in this journal is cited, in accordance with accepted academic practice. No use, distribution or reproduction is permitted which does not comply with these terms.
*Correspondence: Nastassia Navasiolava, TmFzdGFzc2lhLk5hdmFzaW9sYXZhQGNodS1hbmdlcnMuZnI=; Yinghui Li, eWluZ2h1aWRkQHZpcC5zaW5hLmNvbQ==
†These authors have contributed equally to this work