- 1Marine Biological Station, Sado Island Center for Ecological Sustainability, Niigata University, Sado, Japan
- 2Department of Fish Biology and Biotechnology, Faculty of Fisheries, Chattogram Veterinary and Animal Sciences University, Chattogram, Bangladesh
- 3Department of Genetics and Fish Breeding, Faculty of Fisheries, Bangabandhu Sheikh Mujibur Rahman Agricultural University, Gazipur, Bangladesh
Fish are poikilotherm and small changes in water temperature can greatly affect physiological processes including reproduction, which is regulated by complex neuroendocrine mechanisms that respond to climatic events. This review provides evidence that anomalous high and low temperature may directly affect reproduction in fish by suppressing the expression of genes in the reproductive neuroendocrine system. The grass puffer, Takifugu alboplumbeus, is an excellent animal model for studying the thermal regulation of reproduction, for they exhibit periodic spawning activities, which are synchronized with seasonal, lunar and daily cycles. In the grass puffer, the expression of the genes encoding gonadotropin-releasing hormone (GnRH) 1, kisspeptin, gonadotropin-inhibitory hormone (GnIH) and their receptors were markedly suppressed in the diencephalon of fish exposed to high temperature (28°C) when compared to normal temperature (21°C), followed by the decrease in the pituitary mRNA levels for follicle-stimulating hormone (FSH), luteinizing hormone (LH) and growth hormone (GH). On the other hand, the exposure to low temperature (14°C) also inhibited the expression of gnrh1, kiss2, gnih and their receptor genes in the brain and fshb, lhb, gh and prl in the pituitary. Taken together, it is plausible that anomalous high and low temperature may be a proximate driver of termination of reproduction by suppressing the activity of the reproductive GnRH/kisspeptin/GnIH system, possibly through direct action of temperature signals at transcription level.
Introduction
The world’s climate has changed drastically over the last few centuries and considered as a crucial challenge to the humanity in the 21st century. At present, climate change is contributing to extreme climatic events that include heat waves, heavy rainfall and drought in a more frequent and severe fashion. While climate change is a global phenomenon, its negative effect falls upon in all living being, especially in the wild environment. Changes in the environmental factors (temperature, photoperiod, humidity, etc.) greatly influence on the physiology, behavior and ecology of a wide spectrum of organisms (Wuethrich, 2001; Walther et al., 2002). Indeed, an important biological function that has been affected by the climate change is reproduction; changes in the ambient temperature most likely leads to the advancement or delay in the reproductive events of seasonal breeders, particularly in temperate zone and this may cause a decrease in the wild resources because there is only a short period in the annual cycle when conditions are most suitable for reproduction of many seasonal breeders including fish (Wang et al., 2010).
Intragovernmental Panel on Climate Change (IPCC) is now devastatingly convincing that climate change is unpredicted, widespread, rapid and intensifying in upcoming days. IPCC also predicted that global surface temperature will increase 1.5°C above pre-industrial levels by 2030–2050 (Global Warming of 1.5°C, IPCC, 2021) and this may have a great impact on the biodiversity, ecosystems and livelihood, unless massive reduction in the CO2 and other greenhouse gases. So, it is high time to focus on the effect of climate change and the possible impact on the biodiversity of the planet.
The impact of climate change on the aquatic life broadly classified as direct and indirect. Rising water temperature, salinity, water acidification and transformed hydrological regimes are all direct effects. The indirect effects include the potential loss of estuarine habitat due to rising sea level as well as construction of dams and water abstraction for other purposes which limits the diversity and distribution of the wild fisheries resources. The effect of such environmental changes has a great impact of reproduction in fish (Servili et al., 2020). Among these factors, water temperature is a fundamental physical regulatory factor in the lives of fish and its effect is expressed strongly in the control of all reproductive and developmental processes including gamete development and maturation, ovulation and spermiation, spawning, embryogenesis, hatching, larval development and juvenile survival (Alix et al., 2020). Warmer waters decreased the biomass production (van Dorst et al., 2019), reducing the population structure which ultimately leads to the extinction of the species. For the protection, enhancement and restoration of the wild fisheries resources, it is necessary to understand the adaptive mechanism of wild species for water temperature and appropriate management strategies should be implemented for reducing the impact of global warming on individual species, ecosystems and society. As fish are poikilotherm, small changes in water temperature can greatly affect their physiological process which makes them an ideal animal model for studying the thermal regulation of reproduction.
The Hypothalamo-Pituitary-Gonadal Axis in the Regulation of Reproduction
Reproduction in fish is controlled by the action of multiple endocrine and neuroendocrine factors in the hypothalamo-pituitary-gonadal (HPG) axis. The timing and success of reproduction is under the control of complex interaction between various environmental factors (temperature, photoperiod, moon cycle, tides, etc.) and multiple hypothalamic neurohormones including gonadotropin-releasing hormone (GnRH), kisspeptin, and gonadotropin-inhibitory hormone (GnIH) (Simonneaux et al., 2013; Dufour et al., 2020). Among these factors, GnRH is the principal regulator of the secretion of gonadotropins (GTHs), namely follicle-stimulating hormone (FSH) and luteinizing hormone (LH), from the pituitary in many vertebrate species including fish (Ando and Urano, 2005; Zohar et al., 2010; Muñoz-Cueto et al., 2020). Kisspeptin plays an important role in the neuroendocrine regulation of reproduction through stimulating the GnRH secretion in mammals (Oakley et al., 2009). In fish, there are two paralogous genes for kisspeptin (kiss1 and kiss2) and kisspeptin receptor (kissr1, kissr2, kissr3 and kissr4) and this increases the complexity of the kisspeptin system in fish (Ogawa and Parhar, 2018; Dufour et al., 2020). Although kisspeptin has also been shown to play an important role in fish reproduction, some authors described as stimulatory, inhibitory or having no effect on reproduction depending on the species (Felip et al., 2009; Kitahashi et al., 2009; Pasquier et al., 2011; Kanda et al., 2013; Nakajo et al., 2017; Ohga et al., 2018). The role of GnIH is to inhibit the GTH secretion by antagonistic interaction with GnRH in mammals and birds (Tsutsui et al., 2012; Ubuka et al., 2016). However, in fish, both stimulatory and inhibitory effects of GnIH on the secretion of GTH has been found depending on species and gonadal stage (Muñoz-Cueto et al., 2017; Di Yorio et al., 2019).
These hormonal inputs from the hypothalamus stimulate the synthesis and release of FSH and LH, which are pituitary glycoprotein hormones stimulating the gonadal maturation and the production of sex steroid hormones (Yaron et al., 2003). In addition, growth hormone (GH) and prolactin (PRL) have been shown to be involved in teleost’s gonadal development, gametogenesis, and steroidogenesis (Singh et al., 1988; Canosa et al., 2007; Whittington and Wilson, 2013). Previous studies in temperate fish species have shown that neuroendocrine components of the HPG axis exhibit substantial seasonality in their expression levels in association with changes in the environmental factors, especially water temperature. Here in this review, we focused on the effect of anomalous temperature on fish reproduction and the possible neuroendocrine mechanism in response to variable water temperature.
Effects of Water Temperature on the Reproductive Neuroendocrine System in Fish
Water temperature has a remodelling role for fine tuning the reproductive cycle, that is, the precise timing of gamete formation, maturation and spawning, in particular environment (Van Der Kraak and Pankhurst, 1997; Pankhurst and King, 2010). To evaluate the effect of fluctuating water temperature on the reproductive events, a large number of experiments have been conducted in various teleost fishes. The effects of anomalous high and low temperature on the expression of the genes for various endocrine and neuroendocrine factors in the HPG axis have been examined in some teleost fish species and those on the hypothalamic neuropeptides and their receptors are summarized in Table 1 and those for the pituitary hormones and neurohormone receptors are summarized in Table 2. In the brain, it is evident that fluctuating water temperature reduced the expression levels of the genes for GnRH, kisspeptin, GnIH and their receptors, with the exception of GnRH3 and GnIH in sheepshead minnow and Kiss1 in zebrafish (Table 1). There are three GnRH isoforms in teleosts, namely GnRH1, GnRH2 and GnRH3, and their brain distribution and functional roles are different (Zohar et al., 2010; Muñoz-Cueto et al., 2020). Among them, preoptic GnRH1 or GnRH3 neurons, depending on fish species that possess two or three GnRH isoforms in their brains, directly innervate the pituitary and stimulate GTH secretion, and the gene expression of these GnRH isoforms were suppressed by anomalous high and low temperature in most cases (Table 1). In sheepshead minnow, the increased gnrh3 expression of the fish exposed to high temperature conditions with reduced pituitary fshb and lhb expressions suggests that GnRH3 does not have the hypophysiotropic role in this fish (Bock et al., 2021). On the other hand, GnIH, which is also stimulated in the high temperature conditions, is considered to have a negative role in GTH secretion in sheepshead minnow (Bock et al., 2021). However, GnIH has been shown to have a stimulatory role in GTH synthesis in some fish species including the grass puffer, Takifugu alboplumbeus, in which the gnih expression was suppressed in the fish exposed to high temperature conditions (Table 1). In zebrafish, Kiss1 neurons are exclusively localized in the habenula and implicated in the modulation of the serotonergic system (Ogawa et al., 2012; Ogawa and Parhar, 2018), while Kiss2 neurons in hypothalamic nuclei are implicated in reproduction (Servili et al., 2011). Therefore, kiss1/kissr1 expressions stimulated by low temperature was considered to be involved in the alteration of behavior under low temperature conditions in zebrafish (Shahjahan et al., 2013).
In the pituitary, the expression of the genes for GTH subunits, GH, PRL as well as receptors for GnRH and GnIH was significantly suppressed by anomalous high and low temperature in all cases examined (Table 2). These changes have been shown to be accompanied with the inhibition of oogenesis and steroidogenesis and termination of reproduction. Taken together, these results suggest that anomalous temperature mostly has an inhibitory effect on fish reproduction by suppressing the activity of the reproductive GnRH/kisspeptin/GnIH system, possibly through direct action of temperature signals at transcription level.
Reproductive Periodicity and Changes in the Expression of the Reproductive Neuroendocrine System in the Grass Puffer
To address the molecular neuroendocrine regulation of reproductive periodicity and temperature regulation of reproduction, the grass puffer provides an excellent animal model because grass puffer displays a strong reproductive periodicity. The grass puffer is a common intertidal puffer species in the Northwest Pacific Ocean, ranging from as far north as Japan down to the Philippines (Shao et al., 2014). In Japan, grass puffer usually inhibits environments between 10 and 29°C and spawning occurs during spring to early summer with ambient water temperature ranging from about 17 to 25°C. Interestingly, the timing of spawning is tightly connected with the lunar and diurnal cycles during the spawning season (Motohashi et al., 2010; Ando et al., 2013, 2018). During spring tide, on the new and full moon days, the fish aggregate at certain seashore places for spawning and spawning starts 1.5–2.0 h before high tide and continues for about 1 h during the rising tidal phase. Therefore, the spawning of grass puffer is tightly connected to the seasonal, lunar, daily and tidal rhythms where the environmental factors, such as water temperature, light (daylight and moonlight) and tidal cycle may interplay in the control of reproduction.
We previously examined the expression of the genes for the hypothalamic neurohormones and their receptors in the brain of grass puffer over several months during the reproductive cycle. We developed a quantitative real-time PCR assay for each mRNA (Motohashi et al., 2008; Shahjahan et al., 2010a, 2010b, 2011), which is so reliable to generate the sensitivity of 100 copies of particular mRNA with satisfactory range of variation. In the brain of grass puffer, three forms of GnRHs displayed differential expression patterns throughout the spawning season. The expression levels of gnrh1 and gnrh3 were significantly elevated during spawning season, while gnrh2 does not show any variations (Shahjahan et al., 2010a). The genes encoding kisspeptin (kiss2) and its receptor (kiss2r) was predominantly expressed over the course of sexual maturation from the pre-spawning to post-spawning stages (Shahjahan et al., 2010b). On the other hand, mRNA levels for GnIH (gnih) and its receptor (gnihr) gradually increased during the spawning period and significantly decreased at the post-spawning stage (Shahjahan et al., 2011).
In the pituitary, the expression levels of GTH subunit genes (gpa, fshb and lhb) were extensively increased in parallel with gnrh1 and gnrh3 during the spawning season (Shahjahan et al., 2010a). The expression of gh was also significantly higher in the spawning and post-spawning stages, while the expression of prl was significantly higher in the pre-spawning and spawning stages (Shahjahan et al., 2016). Moreover, the plasma concentration of estradiol-17β (E2) and testosterone (T) were higher in the mature fish at the spawning stage compared to other reproductive stages (Shahjahan et al., 2010a). In addition, these genes demonstrated diurnal and circadian oscillations in their expression levels during the spawning period (Shahjahan et al., 2011; Ando et al., 2014).
Tiger puffer, Takifugu rubripes, is closely related to grass puffer, also displayed reproductive periodicity in the expression of the HPG axis genes. The mRNA levels of three gnrhs, gnih, gnihr, fshb and lhb were extensively higher in the mature fish compared to the immature fish, especially in females, and the expression of these genes was significantly decreased in the post-ovulatory females (Zahangir et al., 2021). So, grass puffer as well as the closely related tiger puffer displayed a strong reproductive periodicity with the dynamic variation in the expression of the hypothalamic neurohormone and pituitary hormone genes.
Effects of Anomalous High Temperature on the Expression of the Reproductive Neuroendocrine System in the Grass Puffer
In our previous studies, the mature grass puffer was exposed to a normal temperature (21°C), low temperature (14°C) and a high temperature (28°C) for 7 days, and the expression levels of the HPG axis genes were measured. The mRNA levels of gnrh1, kiss2 and gnih in the diencephalon were significantly decreased in the fish exposed to high temperature compared to normal temperature (Figure 1). In addition, the expression levels of kiss2r and gnihr were significantly decreased in the high temperature-exposed fish (Shahjahan et al., 2017; Rahman et al., 2019). In the pituitary, the exposure to high temperature significantly decreased the levels of gnihr mRNA as well as fshb, lhb and gh mRNAs (Shahjahan et al., 2017). In the grass puffer, GnIH has been shown to be a multifunctional hypophysiotropic factor that stimulates the expression of not only fshb and lhb but also gh and prl (Shahjahan et al., 2011, 2016; Ando et al., 2018). Taken together, the findings on the grass puffer indicate that anomalous high temperature (28°C) has an inhibitory effect on the GnRH1/Kiss2/GnIH/GTH/GH system and this may lead to termination of reproduction at the end of breeding season in July in nature.
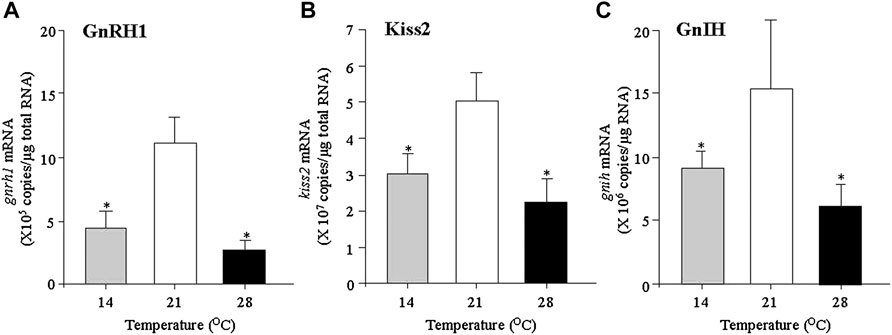
FIGURE 1. Changes in the expression levels of gnrh1 (A), kiss2 (B) and gnih (C) in the diencephalon of grass puffer exposed to low temperature (14°C), normal temperature (21°C) and high temperature (28°C) conditions for 7 days. Values are presented as mean ± standard error of the mean (SEM) (n = 8 in each group). Asterisk indicates a significant difference in the low and high temperature groups compared to the normal temperature group (*, p < 0.05).
Effects of Anomalous Low Temperature on the Expression of the Reproductive Neuroendocrine System in the Grass Puffer
Our previous studies revealed that the mRNA levels of gnrh1, kiss2, kiss2r, gnih and gnihr in the diencephalon were significantly decreased in the low temperature (14°C) conditions when compared to the normal temperature (21°C) conditions (Shahjahan et al., 2017; Rahman et al., 2019). At the pituitary level, the mRNA levels of fshb, lhb, gh and prl were significantly decreased in the low temperature conditions.
Since fish are poikilotherm, they cannot circulate the heated blood throughout the body, hence low temperature may be highly susceptible as stress (Donaldson et al., 2008). The response to stress is delineated by the incitement from the brain and the release of stress hormone like cortisol and catecholamine results in the activation of the neuroendocrine system and a consequential cascade for the metabolic and physiological changes (Zahangir et al., 2015). In the grass puffer exposed to the anomalous low temperature (14°C) conditions, the plasma levels of cortisol were significantly elevated compared to the normal temperature (21°C) conditions, whereas no noticeable change was observed in the high temperature (28°C) conditions, suggesting that the high temperature conditions are within the physiologically acceptable level (Shahjahan et al., 2017). It is therefore considered that the inhibition of prl expression observed only in the low temperature conditions was most probably due to stress response. The inhibitory action of cortisol on the prl expression in the tilapia pituitary supports this notion (Uchida et al., 2004).
Perspectives: Transient Receptor Potential Channels as Possible Molecular Signals of Temperature on the Reproductive Neuroendocrine System
The molecular mechanism of thermal signal transduction in the reproductive neuroendocrine system in response to variable water temperature is largely unknown. Teleost fish have been suggested to sense water temperature using surface thermal receptors located in the trunk lateral line (Sullivan, 1954), and those in trigeminal ganglia have been shown to be involved in thermoregulatory behavior (Haesemeyer, 2020). Although there is a paucity of information concerning on the neural circuits involved in the temperature-dependent regulation of the HPG-axis, temperature is primarily detected by thermo-gated ion channels in somatosensory neurons (Gracheva and Bagriantsev, 2015). It is well known in vertebrates that thermosensitive transient receptor potential (TRP) ion channels called thermoTRP channels play an important role in transducing temperature signals to neural signals (Patapoutian et al., 2003; Diaz-Franulic et al., 2016). Among thermoTRP family members such as ankyrin TRP (TRPA), metastatin TRP (TRPM) and vanilloid TRP (TRPV), TRPV1 and TRPA1 sense noxious ranges of hot and cold stimuli, respectively (Saito and Tominaga, 2017). Although thermoTRPs are mainly expressed in primary sensory neurons, TRPV has also been shown to express in the central nerve system in mammals, with widespread and discrete brain regions including the hypothalamus (Cavanaugh et al., 2011; Nedungadi et al., 2012). Regarding the HPG axis, canonical TRP (TRPC) is expressed in GnRH and kisspeptin neurons (Götz et al., 2017). TRPC channels in mouse GnRH neurons are involved in the kisspeptin-induced depolarization (Zhang et al., 2008) and the expression of TRPC channel subunits are upregulated by estrogen, suggesting that TRPC plays a role in reproductive stage-dependent stimulation of GnRH neurons by kisspeptin (Bosch et al., 2013).
In fish, TRPV members (V1-V4) has been shown to be activated by noxious high temperature (Dhaka et al., 2006). In salmonids, TRPV1 and TRPV4 are expressed in the pineal photoreceptor cells and may mediate the effects of temperature on melatonin production (Nisembaum et al., 2022). On the other hand, the thermal sensitivity of TRPA1 is variable among vertebrate species (Saito and Tominaga, 2017). The zebrafish TRPA1 was shown to respond to both heat and cold stimuli when expressed in Xenopus oocytes (Oda et al., 2016). Since fish are poikilotherm, it is possible that thermoTRP channels in the brain may serve as a thermal sensor that directly senses variable water temperature and its signals may be transmitted to the hypothalamus. In fact, temperature-sensitive neurons have been identified in the hypothalamus of fish (Greer and Gardner, 1974; Nelson and Prosser, 1981). Further studies on the function and expression of thermoTRP channels in the peripheral somatosensory neurons and also in the hypothalamus would provide valuable information on the molecular mechanisms of thermosensitive regulation of reproduction in fish (Figure 2).
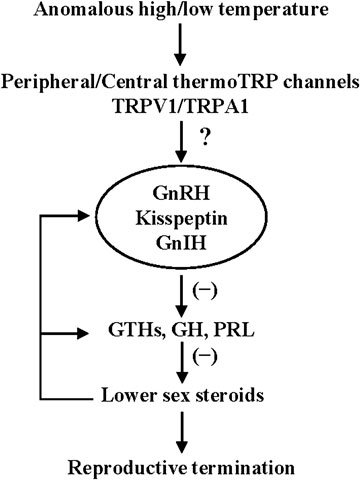
FIGURE 2. Schematic representation of the proposed neuroendocrine mechanism of thermosensitive regulation of reproduction in fish. See the text for explanation.
Conclusion
The evidence summarized in this review revealed that anomalous temperature could directly affect the activity of the reproductive neuroendocrine system, inhibiting the expression of the genes for the hypothalamic neurohormones and their receptors and the pituitary hormones, i.e., the expression of gnrh1/3, kiss2, kiss2r, gnih and gnihr in the diencephalon and fshb, lhb, gh and prl in the pituitary. Grass puffer shows a strong reproductive periodicity where high water temperature (28°C) transmits environmental signals to inhibit the activity of the reproductive neuroendocrine system for the termination of breeding season. On the other hand, anomalous low temperature during the breeding season also transmits environmental signals to inhibit the activity of reproductive neuroendocrine system as, in part, a response to stress in the grass puffer. Taken as a whole, the reproductive neuroendocrine system is most probably the primary target of anomalous changes in water temperature and their effects are attributed to the transcriptional regulation of the genes for the hypothalamic neurohormones and their receptors and the pituitary hormones.
Author Contributions
MMZ, MLR wrote the article. HA reviewed and edited the article. All authors contributed to the article and approved the submitted version.
Funding
This work was supported by MEXT/JSPS KAKENHI grants (245700659, 16H04812 and 17K19385 to HA).
Conflict of Interest
The authors declare that the research was conducted in the absence of any commercial or financial relationships that could be construed as a potential conflict of interest.
Publisher’s Note
All claims expressed in this article are solely those of the authors and do not necessarily represent those of their affiliated organizations, or those of the publisher, the editors and the reviewers. Any product that may be evaluated in this article, or claim that may be made by its manufacturer, is not guaranteed or endorsed by the publisher.
Acknowledgments
We thank Marine Biological Station, Sado Island Center of Ecological Sustainability, Niigata University for the resources.
References
Alix M., Kjesbu O. S., Anderson K. C. (2020). From Gametogenesis to Spawning: How Climate‐driven Warming Affects Teleost Reproductive Biology. J. Fish. Biol. 97, 607–632. doi:10.1111/jfb.14439
Ando H., Ogawa S., Shahjahan M., Ikegami T., Doi H., Hattori A., et al. (2014). Diurnal and Circadian Oscillations in Expression of Kisspeptin, Kisspeptin Receptor and Gonadotrophin-Releasing Hormone 2 Genes in the Grass Puffer, a Semilunar-Synchronised Spawner. J. Neuroendocrinol. 26, 459–467. doi:10.1111/jne.12165
Ando H., Shahjahan M., Hattori A. (2013). Molecular Neuroendocrine Basis of Lunar-Related Spawning in Grass Puffer. Gen. Comp. Endocrinol. 181, 211–214. doi:10.1016/j.ygcen.2012.07.027
Ando H., Shahjahan M., Kitahashi T. (2018). Periodic Regulation of Expression of Genes for Kisspeptin, Gonadotropin-Inhibitory Hormone and Their Receptors in the Grass Puffer: Implications in Seasonal, Daily and Lunar Rhythms of Reproduction. Gen. Comp. Endocrinol. 265, 149–153. doi:10.1016/j.ygcen.2018.04.006
Ando H., Urano A. (2005). Molecular Regulation of Gonadotropin Secretion by Gonadotropin Releasing Hormone in Salmonid Fishes. Zool. Sci. 22, 379–389. doi:10.2108/zsj.22.379
Bock S. L., Chow M. I., Forsgren K. L., Lema S. C. (2021). Widespread Alterations to Hypothalamic-Pituitary-Gonadal (HPG) axis Signaling Underlie High Temperature Reproductive Inhibition in the Eurythermal Sheepshead Minnow (Cyprinodon variegatus). Mol. Cell. Endocrinol. 537, 111447. doi:10.1016/j.mce.2021.111447
Bosch M. A., Tonsfeldt K. J., Rønnekleiv O. K. (2013). mRNA Expression of Ion Channels in GnRH Neurons: Subtype-specific Regulation by 17β-Estradiolfic Regulation by 17ß-Estradiol. Mol. Cell. Endocrinol. 367, 85–97. doi:10.1016/j.mce.2012.12.021
Canosa L. F., Chang J. P., Peter R. E. (2007). Neuroendocrine Control of Growth Hormone in Fish. Gen. Comp. Endocrinol. 151, 1–26. doi:10.1016/j.ygcen.2006.12.010
Cavanaugh D. J., Chesler A. T., Jackson A. C., Sigal Y. M., Yamanaka H., Grant R., et al. (2011). Trpv1 Reporter Mice Reveal Highly Restricted Brain Distribution and Functional Expression in Arteriolar Smooth Muscle Cells. J. Neurosci. 31, 5067–5077. doi:10.1523/JNEUROSCI.6451-10.2011
David D., Degani G. (2011). Temperature Affects Brain and Pituitary Gene Expression Related to Reproduction and Growth in the Male Blue Gouramis, Trichogaster trichopterus. J. Exp. Zool. 315A, 203–214. doi:10.1002/jez.663
Dhaka A., Viswanath V., Patapoutian A. (2006). Trp Ion Channels and Temperature Sensation. Annu. Rev. Neurosci. 29, 135–161. doi:10.1146/annurev.neuro.29.051605.112958
Di Yorio M. P., Muñoz-Cueto J. A., Paullada-Salmerón J. A., Somoza G. M., Tsutsui K., Vissio P. G. (2019). The Gonadotropin-Inhibitory Hormone: what We Know and what We Still Have to Learn from Fish. Front. Endocrinol. 10, 78. doi:10.3389/fendo.2019.00078
Diaz-Franulic I., Poblete H., Miño-Galaz G., González C., Latorre R. (2016). Allosterism and Structure in Thermally Activated Transient Receptor Potential Channels. Annu. Rev. Biophys. 45, 371–398. doi:10.1146/annurev-biophys-062215-011034.x
Donaldson M. R., Cooke S. J., Patterson D. A., Macdonald J. S. (2008). Cold Shock and Fish. J. Fish. Biol. 73, 1491–1530. doi:10.1111/j.1095-8649.2008.0206110.1111/j.1095-8649.2008.02061.x
Dufour S., Quérat B., Tostivint H., Pasqualini C., Vaudry H., Rousseau K. (2020). Origin and Evolution of the Neuroendocrine Control of Reproduction in Vertebrates, with Special Focus on Genome and Gene Duplications. Physiol. Rev. 100, 869–943. doi:10.1152/physrev.00009.2019
Elisio M., Chalde T., Miranda L. A. (2012). Effects of Short Periods of Warm Water Fluctuations on Reproductive Endocrine axis of the Pejerrey (Odontesthes Bonariensis) Spawning. Comp. Biochem. Physiol. Part A Mol. Integr. Physiol. 163, 47–55. doi:10.1016/j.cbpa.2012.05.178
Felip A., Zanuy S., Pineda R., Pinilla L., Carrillo M., Tena-Sempere M., et al. (2009). Evidence for Two Distinct KiSS Genes in Non-placental Vertebrates that Encode Kisspeptins with Different Gonadotropin-Releasing Activities in Fish and Mammals. Mol. Cell. Endocrinol. 312, 61–71. doi:10.1016/j.mce.2008.11.017
Götz V., Qiao S., Beck A., Boehm U. (2017). Transient Receptor Potential (TRP) Channel Function in the Reproductive axis. Cell Calcium 67, 138–147. doi:10.1016/j.ceca.2017.04.004
Gracheva E. O., Bagriantsev S. N. (2015). Evolutionary Adaptation to Thermosensation. Curr. Opin. Neurobiol. 34, 67–73. doi:10.1016/j.conb.2015.01.021
Greer G. L., Gardner D. R. (1974). Characterization of Responses from Temperature-Sensitive Units in Trout Brain. Comp. Biochem. Physiol. Part A Physiol. 48, 189–203. doi:10.1016/0300-9629(74)90866-4
Haesemeyer M. (2020). Thermoregulation in Fish. Mol. Cell. Endocrinol. 518, 110986. doi:10.1016/j.mce.2020.110986
IPCC (2021). Intragovernmental Panel on Climate Change: AR6 Climate Change 2021: The Physical Science Basis. Sixth Assessment Report, Working Group I. Geneva, Switzerland: Subsidiary Body for Scientific and Technological Advice.
Kanda S., Akazome Y., Mitani Y., Okubo K., Oka Y. (2013). Neuroanatomical Evidence that Kisspeptin Directly Regulates Isotocin and Vasotocin Neurons. PLoS One 8, e62776. doi:10.1371/journal.pone.0062776
Kitahashi T., Ogawa S., Parhar I. S. (2009). Cloning and Expression of Kiss2 in the Zebrafish and Medakafish and Medaka. Endocrinology 150, 821–831. doi:10.1210/en.2008-0940
Levy G., David D., Degani G. (2011). Effect of Environmental Temperature on Growth- and Reproduction-Related Hormones Gene Expression in the Female Blue Gourami (Trichogaster trichopterus). Comp. Biochem. Physiol. Part A Mol. Integr. Physiol. 160, 381–389. doi:10.1016/j.cbpa.2011.07.007
Motohashi E., Hamabata T., Ando H. (2008). Structure of Neurohypophysial Hormone Genes and Changes in the Levels of Expression during Spawning Season in Grass Puffer (Takifugu niphobles). Gen. Comp. Endocrinol. 155, 456–463. doi:10.1016/j.ygcen.2007.07.009
Motohashi E., Yoshihara T., Doi H., Ando H. (2010). Aggregating Behavior of the Grass Puffer,Takifugu niphobles, Observed in Aquarium during the Spawning Period. Zool. Sci. 27, 559–564. doi:10.2108/zsj.27.559
Muñoz-Cueto J. A., Paullada-Salmerón J. A., Aliaga-Guerrero M., Cowan M. E., Parhar I. S., Ubuka T. (2017). A Journey through the Gonadotropin-Inhibitory Hormone System of Fish. Front. Endocrinol. 8, 285. doi:10.3389/fendo.2017.00285
Muñoz-Cueto J. A., Zmora N., Paullada-Salmerón J. A., Marvel M., Mañanos E., Zohar Y. (2020). The Gonadotropin-Releasing Hormones: Lessons from Fish. Gen. Comp. Endocrinol. 291, 113422. doi:10.1016/j.ygcen.2020.113422
Nakajo M., Kanda S., Karigo T., Takahashi A., Akazome Y., Uenoyama Y., et al. (2017). Evolutionally Conserved Function of Kisspeptin Neuronal System Is Nonreproductive Regulation as Revealed by Nonmammalian Study. Endocrinology 159, 163–183. doi:10.1210/en.2017-00808
Nedungadi T. P., Dutta M., Bathina C. S., Caterina M. J., Cunningham J. T. (2012). Expression and Distribution of TRPV2 in Rat Brain. Exp. Neurol. 237, 223–237. doi:10.1016/j.expneurol.2012.06.017
Nelson D. O., Prosser C. L. (1981). Intracellular Recordings from Thermosensitive Preoptic Neurons. Science 213, 787–789. doi:10.1126/science.7256280
Nisembaum L. G., Loentgen G., L’Honoré T., Martin P., Paulin C.-H., Fuentès M., et al. (2022). Transient Receptor Potential-Vanilloid (TRPV1-TRPV4) Channels in the Atlantic Salmon, Salmo salar. A Focus on the Pineal Gland and Melatonin Production. Front. Physiol. 12, 784416. doi:10.3389/fphys.2021.784416
Oakley A. E., Clifton D. K., Steiner R. A. (2009). Kisspeptin Signaling in the Brain. Endocr. Rev. 30, 713–743. doi:10.1210/er.2009-0005
Oda M., Kurogi M., Kubo Y., Saitoh O. (2016). Sensitivities of Two Zebrafish TRPA1 Paralogs to Chemical and Thermal Stimuli Analyzed in Heterologous Expression Systemsfish TRPA1 Paralogs to Chemical and Thermal Stimuli Analyzed in Heterologous Expression Systems. Chemse 41, 261–272. doi:10.1093/chemse/bjv091
Ogawa S., Ng K. W., Ramadasan P. N., Nathan F. M., Parhar I. S. (2012). Habenular Kiss1 Neurons Modulate the Serotonergic System in the Brain of Zebrafishfish. Endocrinology 153, 2398–2407. doi:10.1210/en.2012-1062
Ogawa S., Parhar I. S. (2018). Biological Significance of Kisspeptin-Kiss 1 Receptor Signaling in the Habenula of Teleost Species. Front. Endocrinol. 9, 222. doi:10.3389/fendo.2018.00222
Ohga H., Selvaraj S., Matsuyama M. (2018). The Roles of Kisspeptin System in the Reproductive Physiology of Fish with Special Reference to Chub Mackerel Studies as Main Axis. Front. Endocrinol. 9, 147. doi:10.3389/fendo.2018.00147
Okuzawa K., Gen K. (2013). High Water Temperature Impairs Ovarian Activity and Gene Expression in the Brain-Pituitary-Gonadal axis in Female Red Seabream during the Spawning Season. Gen. Comp. Endocrinol. 194, 24–30. doi:10.1016/j.ygcen.2013.08.015
Okuzawa K., Kumakura N., Gen K., Yamaguchi S., Lim B.-S., Kagawa H. (2003). Effect of High Water Temperature on Brain-Pituitary-Gonad axis of the Red Seabream during its Spawning Season. Fish Physiol. Biochem. 28, 439–440. doi:10.1023/b:fish.0000030621.31336.91
Pankhurst N. W., King H. R. (2010). Temperature and Salmonid Reproduction: Implications for Aquaculture. J. Fish. Biol. 76, 69–85. doi:10.1111/J.1095-8649.2009.02484.X
Pasquier J., Lafont A.-G., Leprince J., Vaudry H., Rousseau K., Dufour S. (2011). First Evidence for a Direct Inhibitory Effect of Kisspeptins on LH Expression in the Eel, Anguilla anguilla. Gen. Comp. Endocrinol. 173, 216–225. doi:10.1016/j.ygcen.2011.05.019
Patapoutian A., Peier A. M., Story G. M., Viswanath V. (2003). ThermoTRP Channels and beyond: Mechanisms of Temperature Sensation. Nat. Rev. Neurosci. 4, 529–539. doi:10.1038/nrn1141
Pérez L., Peñaranda D. S., Dufour S., Baloche S., Palstra A. P., Van Den Thillart G. E. E. J. M., et al. (2011). Influence of Temperature Regime on Endocrine Parameters and Vitellogenesis during Experimental Maturation of European Eel (Anguilla anguilla) Females. Gen. Comp. Endocrinol. 174, 51–59. doi:10.1016/j.ygcen.2011.08.009
Rahman M. L., Zahangir M. M., Kitahashi T., Shahjahan M., Ando H. (2019). Effects of High and Low Temperature on Expression of GnIH, GnIH Receptor, GH and PRL Genes in the Male Grass Puffer during Breeding Season. Gen. Comp. Endocrinol. 282, 113200. doi:10.1016/j.ygcen.2019.06.004
Saito S., Tominaga M. (2017). Evolutionary Tuning of TRPA1 and TRPV1 Thermal and Chemical Sensitivity in Vertebrates. Temperature 4, 141–152. doi:10.1080/23328940.2017.1315478
Servili A., Canario A. V. M., Mouchel O., Muñoz-Cueto J. A. (2020). Climate Change Impacts on Fish Reproduction Are Mediated at Multiple Levels of the Brain-Pituitary-Gonad axis. Gen. Comp. Endocrinol. 291, 113439. doi:10.1016/j.ygcen.2020.113439
Servili A., Le Page Y., Leprince J., Caraty A., Escobar S., Parhar I. S., et al. (2011). Organization of Two Independent Kisspeptin Systems Derived from Evolutionary-Ancient Kiss Genes in the Brain of Zebrafishfish. Endocrinology 152, 1527–1540. doi:10.1210/en.2010-0948
Shahjahan M., Doi H., Ando H. (2016). LPXRFamide Peptide Stimulates Growth Hormone and Prolactin Gene Expression during the Spawning Period in the Grass Puffer, a Semi-lunar Synchronized Spawner. Gen. Comp. Endocrinol. 227, 77–83. doi:10.1016/j.ygcen.2015.09.008
Shahjahan M., Hamabata T., Motohashi E., Doi H., Ando H. (2010a). Differential Expression of Three Types of Gonadotropin-Releasing Hormone Genes during the Spawning Season in Grass Puffer, Takifugu niphobles. Gen. Comp. Endocrinol. 167, 153–163. doi:10.1016/j.ygcen.2010.01.018
Shahjahan M., Ikegami T., Osugi T., Ukena K., Doi H., Hattori A., et al. (2011). Synchronised Expressions of LPXRFamide Peptide and its Receptor Genes: Seasonal, Diurnal and Circadian Changes during Spawning Period in Grass Puffer. J. Neuroendocrinol. 23, 39–51. doi:10.1111/j.1365-2826.2010.02081.x
Shahjahan M., Kitahashi T., Ando H. (2017). Temperature Affects Sexual Maturation through the Control of Kisspeptin, Kisspeptin Receptor, GnRH and GTH Subunit Gene Expression in the Grass Puffer during the Spawning Season. Gen. Comp. Endocrinol. 243, 138–145. doi:10.1016/j.ygcen.2016.11.012
Shahjahan M., Kitahashi T., Ogawa S., Parhar I. S. (2013). Temperature Differentially Regulates the Two Kisspeptin Systems in the Brain of Zebrafish. Gen. Comp. Endocrinol. 193, 79–85. doi:10.1016/j.ygcen.2013.07.015
Shahjahan M., Motohashi E., Doi H., Ando H. (2010b). Elevation of Kiss2 and its Receptor Gene Expression in the Brain and Pituitary of Grass Puffer during the Spawning Season. Gen. Comp. Endocrinol. 169, 48–57. doi:10.1016/j.ygcen.2010.07.008
Shao K., Liu M., Hardy G., Leis J. L., Matsuura K., Jing L. (2014). Takifugu niphobles. United Kingdom: IUCN Red List of Threatened Species 2014: e.T21341A2775256. doi:10.2305/IUCN.UK.2014-3.RLTS.T21341A2775256.en
Simonneaux V., Ancel C., Poirel V. J., Gauer F. (2013). Kisspeptins and RFRP-3 Act in Concert to Synchronize Rodent Reproduction with Seasons. Front. Neurosci. 7, 22. doi:10.3389/fnins.2013.00022
Singh H., Griffith R. W., Takahashi A., Kawauchi H., Thomas P., Stegeman J. J. (1988). Regulation of Gonadal Steroidogenesis in Fundulus heteroclitus by Recombinant Salmon Growth Hormone and Purified Salmon Prolactin. Gen. Comp. Endocrinol. 72, 144–153. doi:10.1016/0016-6480(88)90190-6
Soria F. N., Strüssmann C. A., Miranda L. A. (2008). High Water Temperatures Impair the Reproductive Ability of the Pejerrey Fish Odontesthes Bonariensis: Effects on the Hypophyseal‐Gonadal Axis. Physiol. Biochem. Zool. 81, 898–905. doi:10.1086/588178
Sullivan C. M. (1954). Temperature Reception and Responses in Fish. J. Fish. Res. Bd. Can. 11, 153–170. doi:10.1139/f54-013
Tsutsui K., Ubuka T., Bentley G. E., Kriegsfeld L. J. (2012). Gonadotropin-inhibitory Hormone (GnIH): Discovery, Progress and Prospect. Gen. Comp. Endocrinol. 177, 305–314. doi:10.1016/j.ygcen.2012.02.013
Ubuka T., Son Y. L., Tsutsui K. (2016). Molecular, Cellular, Morphological, Physiological and Behavioral Aspects of Gonadotropin-Inhibitory Hormone. Gen. Comp. Endocrinol. 227, 27–50. doi:10.1016/j.ygcen.2015.09.009
Uchida K., Yoshikawa-Ebesu J. S. M., Kajimura S., Yada T., Hirano T., Gordon Grau E. (2004). In Vitro effects of Cortisol on the Release and Gene Expression of Prolactin and Growth Hormone in the tilapia, Oreochromis mossambicus. Gen. Comp. Endocrinol. 135, 116–125. doi:10.1016/j.ygcen.2003.08.010
Van Der Kraak G., Pankhurst N. W. (1997). “Temperature Effects on the Reproductive Performance of Fish,” in Global Warming: Implications for Freshwater and Marine Fish. Editors C. M. Wood,, and D. G. McDonald (Cambridge: Cambridge University Press), 159–176.
van Dorst R. M., Gårdmark A., Svanbäck R., Beier U., Weyhenmeyer G. A., Huss M. (2019). Warmer and Browner Waters Decrease Fish Biomass Production. Glob. Change. Biol. 25, 1395–1408. doi:10.1111/gcb.14551
Walther G.-R., Post E., Convey P., Menzel A., Parmesan C., Beebee T. J. C., et al. (2002). Ecological Responses to Recent Climate Change. Nature 416, 389–395. doi:10.1038/416389a
Wang N., Teletchea F., Kestemont P., Milla S., Fontaine P. (2010). Photothermal Control of the Reproductive Cycle in Temperate Fishes. Rev. Aquacult. 2, 209–222. doi:10.1111/j.1753-5131.2010.01037.x
Whittington C. M., Wilson A. B. (2013). The Role of Prolactin in Fish Reproduction. Gen. Comp. Endocrinol. 191, 123–136. doi:10.1016/j.ygcen.2013.05.027
Wuethrich B. (2000). How Climate Change Alters Rhythms of the Wild. Science 287, 793–795. doi:10.1126/science.287.5454.793
Yaron Z., Gur G., Melamed P., Rosenfeld H., Elizur A., Levavi-Sivan B. (2003). Regulation of Fish Gonadotropins. Int. Rev. Cytol. 225, 131–185. doi:10.1016/s0074-7696(05)25004-0
Zahangir M. M., Haque F., Mostakim G. M., Islam M. S. (2015). Secondary Stress Responses of Zebrafish to Different pH: Evaluation in a Seasonal Manner. Aquac. Rep. 2, 91–96. doi:10.1016/j.aqrep.2015.08.008
Zahangir M. M., Matsubara H., Ogiso S., Suzuki N., Ueda H., Ando H. (2021). Expression Dynamics of the Genes for the Hypothalamo-Pituitary-Gonadal axis in Tiger Puffer (Takifugu rubripes) at Different Reproductive Stages. Gen. Comp. Endocrinol. 301, 113660. doi:10.1016/j.ygcen.2020.113660
Zhang C., Roepke T. A., Kelly M. J., Ronnekleiv O. K. (2008). Kisspeptin Depolarizes Gonadotropin-Releasing Hormone Neurons through Activation of TRPC-like Cationic Channels. J. Neurosci. 28, 4423–4434. doi:10.1523/JNEUROSCI.5352-07.2008
Keywords: GnIH, GnRH, grass puffer, hypothalamus, kisspeptin, reproduction, transient receptor potential, pituitary
Citation: Zahangir MM, Rahman ML and Ando H (2022) Anomalous Temperature Interdicts the Reproductive Activity in Fish: Neuroendocrine Mechanisms of Reproductive Function in Response to Water Temperature. Front. Physiol. 13:902257. doi: 10.3389/fphys.2022.902257
Received: 22 March 2022; Accepted: 21 April 2022;
Published: 24 May 2022.
Edited by:
Md Shahjahan, Bangladesh Agricultural University, BangladeshReviewed by:
Radha Chaube, Banaras Hindu University, IndiaTakayoshi Ubuka, Cancer Medical Service, Japan
Copyright © 2022 Zahangir, Rahman and Ando. This is an open-access article distributed under the terms of the Creative Commons Attribution License (CC BY). The use, distribution or reproduction in other forums is permitted, provided the original author(s) and the copyright owner(s) are credited and that the original publication in this journal is cited, in accordance with accepted academic practice. No use, distribution or reproduction is permitted which does not comply with these terms.
*Correspondence: Hironori Ando, aGFuZG8zMTFAY2MubmlpZ2F0YS11LmFjLmpw