- 1INRAE, CNRS, IFCE, Université de Tours, PRC, Nouzilly, France
- 2INRAE, CHU de Tours, Université de Tours, PIXANIM, Nouzilly, France
- 3INRAE, Université de Tours, BOA, Nouzilly, France
Storing fertilised eggs prior to incubation is a frequent practice in commercial hatcheries to coordinate activities and synchronise hatchings. However, the conditions used to store eggs can have major impacts on egg quality and the subsequent viability of chicken embryos. While storage temperatures of 16–18°C are classically used in hatcheries, the duration of storage varies from three to more than 10 days. We explored the effect of storage duration (zero, three or 10 days; D0, D3 and D10, respectively) at 16°C, 80% relative humidity (RH) on egg quality (Broiler, Ross 308), using computed tomography (CT) and classical measurements (egg weight, eggshell strength, egg white pH, Haugh units, yolk index and colour). The results revealed that a storage duration of up to 10 days negatively affected some egg quality traits (yolk index and volume, air chamber volume and egg white pH). Eggs stored for three or 10 days were further incubated for 11, 13 or 15 days (37.8°C, 55% RH). Eggs were analysed by magnetic resonance imaging (MRI) and CT to assess the development of the embryo and internal egg changes occurring during incubation. First, data showed that the fertility and sex ratio of eggs were not affected by storage duration. However, the mortality of viable eggs was increased in the D10 group compared to the D3 group. Results of non-invasive imaging technologies revealed that the storage of eggs for 10 days impaired embryo growth as early as 11 days of incubation (decrease in brain and embryo volumes). Collectively, these data provide new evidence that the duration of egg storage negatively affects embryonic growth. They further corroborate that this parameter is likely to be crucial to synchronising embryonic stages and maybe reducing the hatching window, hence limiting the time spent by newborn chicks in hatchers. In addition, our results highlight that CT and MRI imaging technologies are useful non-invasive tools to evaluate egg quality prior to incubation and the impact of storage (or incubation) practices on developmental growth of the embryo.
Introduction
Egg storage prior to incubation is an common practice in the broiler industry (Fasenko, 2007). It allows coordinating hatchery activities, considering the time between laying and the arrival of eggs in hatcheries. It supplies a certain flexibility towards demands and facilitates synchronisation of hatchings.
Egg storage prior to incubation does not negatively affect hatchability when the duration of storage does not exceed 7 days (Fasenko, 2007). It is noteworthy that incubating freshly laid eggs is, unexpectedly, not associated with higher embryo viability compared to that of stored eggs, and eggs incubated the day of laying tend to hatch later compared with eggs stored for one or 2 days (Reis et al., 1997). The freshly laid egg contains a high concentration of carbon dioxide that may be detrimental to initiating the first stages of embryo development, while the thickness of the egg white is assumed to slow vital gas diffusion and limit access to egg nutrients (Benton and Brake, 1996). On the other hand, extended storage can have a dramatic impact on blastoderm reactivation, even if it is conducted at 17–18°C under controlled relative humidity. Storage beyond 7 days is usually associated with decreased hatchability rates compared to short periods of storage (Lapão et al., 1999; Elibol et al., 2002; Hamidu et al., 2011; Goliomytis et al., 2015; Bakst et al., 2016b; Abioja et al., 2021) and egg storage time was evidenced to be the most important factor (among genotype, hen age, setter and hatcher type) associated with early embryonic mortality (Grochowska et al., 2019). Long storage induces an alteration of many egg quality features including a decrease in yolk and albumen quality parameters and water loss, but also impairs the quality of the blastoderm (increased diameter, small shift of its position on the yolk likely due to the progressive disintegration of the chalazae, decreased number of viable cells, increased necrosis and apoptosis, etc.) (Burkhardt et al., 2011; Bakst et al., 2012; Abioja et al., 2021) (Figure 1). These combined alterations result in an increase of early and late embryo mortality. Similar observations have been reported in the literature for other domestic avian species (Fasenko et al., 2001; Hassan et al., 2005; Nowaczewski et al., 2010; Hyánková and Novotná, 2013; Kouame et al., 2019; Taha et al., 2019).
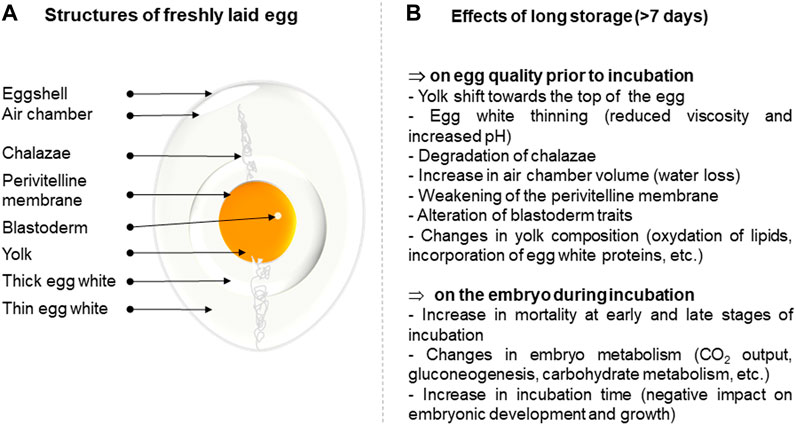
FIGURE 1. Summary of the effect of long-term storage on egg quality and subsequent development of the embryo. (A). Structure of a freshly laid egg. (B). Effects of long-term storage on egg parameters.
Upon egg storage at cooled temperature, the embryo metabolism changes (Christensen et al., 2001) and the embryonic development pauses. The embryo (blastoderm) enters a temperature-induced diapause (Pokhrel et al., 2021), also termed dormancy, which is characterised by reduced cellular activity and suppressed apoptosis (Tona et al., 2003b; Bakst et al., 2016b; Ko et al., 2017). However, lengthening the storage period of eggs irreversibly impairs embryo survival. Prolonged storage (over 10 days) has been shown to activate mechanisms of apoptotic cell death at the blastodermal level (upregulation of pro-apoptotic genes), resulting in decreasing blastodermal cell viability (Hamidu et al., 2011). Long storage also affects the ability of the embryo to resume development once incubated, while embryos that survive long storage treatment undergo delayed hatching by several hours (Christensen et al., 2002; Tona et al., 2003a; Tona et al., 2003b). Long storage of eggs can affect the intestinal morphology of the chicks, the expression of nutrient transporters (Yalcin et al., 2016; Yalcin et al., 2017), chick immunocompetence (Goliomytis et al., 2015) and hormonal metabolism (Tona et al., 2003b). Long storage may also have long-term negative effects on the quality and physiology of hatched chicks (Tona et al., 2003a; Tona et al., 2003b; Reijrink et al., 2010; Yalcin et al., 2017; Mróz et al., 2019).
Many studies have been published on the effects of prolonged storage on egg quality and blastoderm characteristics, but also on the chick after hatching. However, only few articles address the impact of egg storage on embryonic development. Long storage conditions have been shown to affect the development of the embryo, which exhibits lower overall weight (Christensen et al., 2002; Hamidu et al., 2011; Bakst et al., 2016a), lighter heart, liver and thigh muscle (Christensen et al., 2002) and smaller leg bones (Yalcin and Siegel, 2003), compared to those stored for one to 4 days. All these observations strongly support that the development of embryos after long storage is slowed down, which likely explains the delayed hatching observed in several studies (Christensen et al., 2002; Tona et al., 2003a; Tona et al., 2003b) when compared to eggs stored for only few days. It seems that embryos from eggs stored for a long period require more time in the incubator to reach the developmental maturity that is necessary for hatching, compared with eggs stored for a short period.
Based on these data, which were mostly obtained after egg opening, we evaluated whether computed tomography (CT) and magnetic resonance imaging (MRI), as non-invasive technologies, could be used to monitor internal changes that occur in eggs upon short and long storage. CT has been previously applied to localise the germinal disc in ovo (Bartels et al., 2008) and MRI techniques have been used to study the egg yolk structure (Hutchison et al., 1992), the localisation of the germinal disc (Klein et al., 2002) and to monitor the development of the embryo under conventional conditions (Bain et al., 2007; Boss et al., 2008; Zhou et al., 2015; Lindner et al., 2017; Kantarcioglu et al., 2018). However, the use of such techniques to assess the effect of storage time on egg quality and embryo development has not been reported to date.
Materials and Methods
The experimental workflow is illustrated in Figure 2. Using CT, we analysed the quality of eggs collected on the day of laying (D0) or after three and 10 days of storage (D3 and D10, respectively) at 16°C, 80% RH. Eggs were then opened and classical egg quality parameters were measured. Remaining D3 and D10 eggs were incubated (37.8°C, 55% RH) for 11, 13 and 15 days and analysed by MRI and CT, followed by egg opening to weigh the embryo.
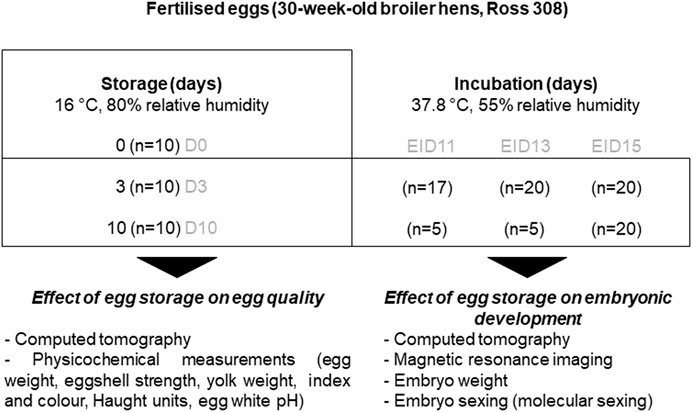
FIGURE 2. Experimental design. The internal quality of freshly laid eggs (day of laying, D0) and eggs stored for three (D3) or 10 days (D10) was measured to evaluate changes occurring during storage (left panel). A series of eggs stored for three or 10 days were further incubated for 11 (EID11), 13 (EID13) or 15 (EID15) days to analyse the impact of storage on embryonic development (right panel).
Incubation Procedures and Sampling
Freshly laid fertilised eggs were obtained from 30-week-old broiler breeder hens (ROSS 308, Boyé Accouvage, La Boissière en Gâtine, France). Eggs were all weighted and ten-egg batches of similar egg weight (56.5 ± 0.52 g) were formed. The egg weight in each batch ranged from 53 to 60.4 g to illustrate natural egg weight heterogeneity. Ten eggs were kept (D0) for analyses, while the remaining eggs were stored in the Poultry Experimental Facility (PEAT) UE1295 (INRAE, F-37380 Nouzilly, France, DOI: 10.15454/1.5572326250887292E12) in a dedicated room at 16°C, 80% RH for three (D3) or 10 days (D10). Ten eggs were collected at D0, D3 and D10 and were analysed by computed tomography, followed by the measurement of some egg quality parameters. The day before incubation 60 D3 and 60 D10 eggs were placed at room temperature (45% hygrometry), and then transferred into a 3900-egg incubator (Bekoto B64-S, Pont-Saint-Martin, France) set at 37.8°C, 55% RH (automatic turning every hour, large end of eggs on top). After 11, 13 and 15 days of incubation (Embryonic incubation day 11, 13 and 15 or EID13, EID13, and EID15, respectively), D3 and D10 eggs containing viable embryos were analysed by computed tomography while others were selected for MRI analyses. After CT and MRI acquisitions, all eggs were weighed and opened to collect embryos that were killed by decapitation. This experimental procedure meets the guidelines approved by the Institutional Animal Care and Use Committee (IACUC). Dead embryos were weighed and a small piece of the liver was collected, kept at −20°C for molecular sexing.
Measurements of Egg Quality Parameters During Egg Storage and Incubation (CT and Physicochemical Measurements)
A series of ten eggs were collected the day of laying, three and 10 days after storage. Eggs were analysed by clinical computed tomography (Siemens Somatom® Definition AS 128, Siemens, Germany). The X-ray source was set at 140 kV and 400 mA/s. The image acquisition mode was 16 cm * 16 cm, 512 pixels matrix size with a slice thickness of 0.4 mm and a resolution of 312.5 µm. Safire U 40 and V80 reconstruction filters were used to characterise the internal components of the egg and the shell, respectively (Table 1). The analysis of egg structures was performed with the Syngo. Via software (Siemens, Germany). The volume of the air chamber and the albumen was estimated automatically using ITK Snap software (Yushkevich et al., 2006).
Following CT acquisition, eggs were characterised for their quality. Egg weight, eggshell strength, Haugh units, yolk colour and index were measured using a Digital Egg Tester 6,000 (Nabel, Kyoto, Japan). The egg yolk was weighed and egg white pH was measured (Eutech pH metre, Thermo Fisher Scientific, Illkirch, France).
MRI Analyses During Incubation
The eggs stored for 3 days (D3) or 10 days (D10) were incubated. After 11, 13 or 15 days (EID11, EID13, EID15), eggs were collected and refrigerated at 4°C for 1 h and 10 min at −20°C prior to analyses with 3 T (T) MRI scanner (Siemens Magnetom®, Verio, Erlangen, Germany). Such an egg cooling was necessary to anesthetise the chick and thus avoid movements of the embryos during MRI acquisition.
We used one radio frequency (RF) ‘loop’ coil, with an inner diameter of 7 cm, to analyse eggs independently. Each egg was inserted in the middle of the loop coil.
Two separate MRI image sequences (T1: spin-lattice or longitudinal relaxation time and T2: spin-spin or transverse relaxation time) were performed on the whole brain, in order to get two distinct image contrasts. The T1 3D and T2 3D were the Magnetisation Prepared Rapid Acquisition Gradient Echo (MPRAGE) and the Sampling Perfection with Application-optimised Contrasts (SPACE), respectively.
The acquisition parameters for these T1 and T2 anatomical analyses were as follows:
-T1 3D: repetition time (TR) = 1970 ms; echo time (TE) = 3.34 ms; inversion time (TI) = 900 ms; flip angle = 9°; field-of-view (FOV): 81 * 81 mm2; matrix: 192 * 1922; and a slice thickness of 0.4 mm resulting in a voxel size of 0.42 * 0.42 * 0.40 mm3. A bandwidth of 150 Hz/Px and two number of excitations (NEX) producing an acquisition time of 9 min 29 s were used.
-T2 3D: TR = 1860 ms; TE = 140 ms; flip angle = 140°; bandwidth of 296 Hz/Px; and a turbo factor of 99. The inter echo space was 7.38 ms. The FOV was 70 * 70 mm2. The matrix was 192 * 1922 and slice thickness was 0.35 mm, which ended with a voxel size of 0.36 * 0.36 * 0.35 mm3. The acquisition time was 8 min 57 s.
Volumes on the MRI images were estimated based on T1 images of the yolk and the albumen, and on the T2 images for the brain, eyes, yolk sac, allantoic fluid and embryo, as T2 contrast clearly allows distinction of water content between egg compartments (Table 1). At EID13 and EID15, the yolk sac and embryo signals were merged, as the tissue aspect of the yolk sac as a very dense and vascularised tissue (Wong and Uni, 2021) resembled the embryo and both structures could not be distinguished.
For volume estimation, the Digital Imaging and Communication in Medicine (DICOM) images were converted into the Neuroimaging Informatics Technology Initiative (NIfTI) format.
The NIfTI images were read with ITK Snap, which is a free, post-processing software generally used to segment 3D medical image structures (Yushkevich et al., 2006). The segmentation of “area growing” type was done automatically and then corrected manually.
After MRI analyses, eggs were weighed, embryos were removed from the eggs and decapitated and embryo weight was determined. Small pieces of the liver were collected and stored at −20°C for further analysis (molecular sexing).
Molecular Sexing
Molecular sexing was performed as previously published with minor adjustments (He et al., 2019). Small pieces of EID11, EID13 and EID15 embryo livers were lysed in 150 μl of lysis buffer containing 10% of chelating beads (Chelex 100), 0.2% SDS, 10 mM Tris pH 8 and 0.2 mg/ml Proteinase K). Samples were incubated for 3 hours at 55°C followed by a 15-min incubation at 95°C. Samples were then centrifuged for 3 min at room temperature at maximum speed with a Mini centrifuge 6K (ExtraGene, Taichung City, Taiwan). Supernatants were recovered and stored at −20°C until use. DNA lysate quantification was assessed by reading the 260 nm absorbance with a micro volume spectrophotometer (Nanodrop One Thermo Scientific, Wilmington United States). Embryo lysates were diluted ten times in nuclease free water and 1 μl of dilution was mixed on ice with primer SWIM (forward: 5′- GAGATCACGAACTCAACCAG -3′/reverse: 5′- CCAGACCTAATACGGTTTTACAG -3′), which is female specific and primer 12S (forward-5′ CTATAATCGATAATCCACGATTCA- 3′, reverse: 5′- CTTGACCTGTCTTATTAGCGAGG -3′) and Dream Taq PCR Master Mix (2X), according to the manufacturer’s recommendations (Thermo Fisher Scientific, Illkirch, France). Amplification by polymerase chain reaction (PCR) was performed using a thermocycler (Eppendorf, Montesson, France), as described previously (He et al., 2019). PCR products were loaded on a 2% agarose gel containing 0.01% gel Red in 1X TAE buffer, and separated by electrophoresis at 100 V. Gels were imaged using a Bio-Print imager (Vilber Lourmat, Marne-la-Vallée, France). Female samples exhibited two amplification products (131 bp for 12S and 212 bp for SWIM gene), while male samples exhibited only one amplification product (131 bp for 12S gene).
Statistical Analyses
All statistical analyses were performed using XLSTAT software (Data Analysis and Statistical Solution for Microsoft Excel, Addinsoft, Paris, France 2017). For most parameters, normality of the samples was not achieved (Shapiro–Wilk test). Thus, all statistical analyses (except for embryo weight) were performed using a Kruskal–Wallis test (p < 0.05), followed by a pair comparison using a Mann–Whitney test (p < 0.05), when required. For embryo weight, we used an ANOVA test.
Results
Impact of Egg Storage for Zero, Three or Ten Days on Egg Quality
CT images that are representative of each egg storage group (D0, D3 and D10) are shown in Figure 3. Analysis of principal components clearly demonstrated that all three groups of eggs, freshly laid (D0) or stored for three or 10 days (D3 and D10, respectively) were distributed distinctly (Figure 4). The D3 group was intermediate between the D0 and D10 groups, as expected. Combined results from CT and egg quality parameter measurements are presented in Table 2. No statistical difference was observed for egg weight, eggshell strength, yolk colour, yolk weight and egg white volume. Yolk index, yolk volume and Haugh units tended to decrease over storage, while egg white pH and the volume of the air chamber increased. Although not statistically significant between days of storage (p = 0.129, ANOVA test), the volume of the “grey zone” (that includes the blastoderm) tended to increase over time. The trend was confirmed when comparisons were performed between two groups (Mann-Whitney test). The difference between D3 and D10 groups was shown to be significant (p-value = 0.031), not significant between D0 and D3 groups (p = 0.297), and close to statistical significance between D0 and D10 groups (p = 0.060). It is noteworthy that volumes of the yolk and the “grey zone” were expected to be negatively correlated: the more pixels attributed to the “grey zone”, the less pixels assigned to the yolk.
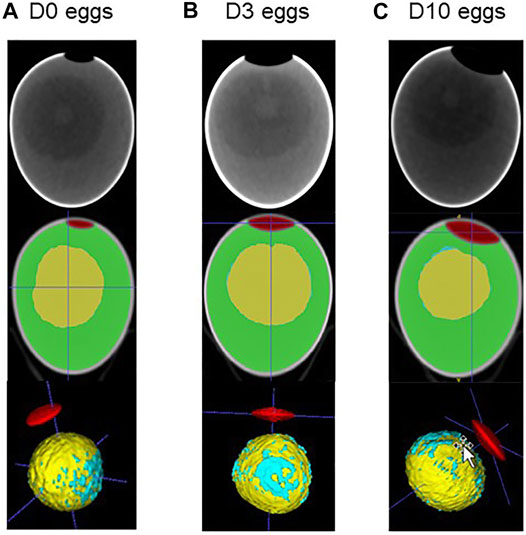
FIGURE 3. CT imaging of fertilised eggs stored for zero, three or 10 days (D0 (A), D3 (B) and D10 (C), respectively). Using ITK. snap tool (Yushkevich et al., 2006), images obtained after CT imaging (upper panel) were analysed for 3D reconstruction, segmentation and coloured for illustration. The air chamber is illustrated in red, the yolk is shown in yellow, the white in green and the blastoderm in blue. It is noteworthy that for the latter parameter, we considered all blue spots distributed on the yolk surface to avoid any bias between groups. A concentration of blue spots that likely corresponds to the blastoderm is clearly visible, while blue spots are also sporadically distributed on the yolk surface.
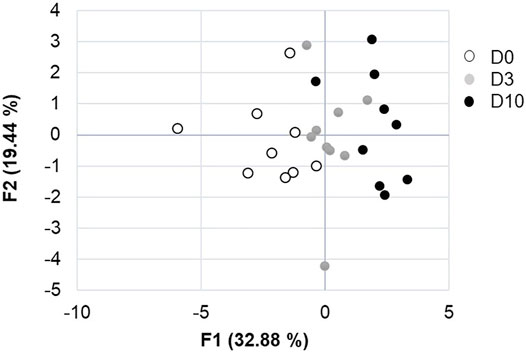
FIGURE 4. Analysis of principal components of the effect of storage duration on egg parameters. F1 and F2 axes explain 52.2% of the variability between D0, D3 and D10 groups.
Impact of Egg Storage for Three or Ten Days on Embryo Viability and Development
The time of storage prior to incubation did not significantly affect fertility (98.1% for the D3 group and 98.5% for the D10 group). However, when considering the whole period of experimentation (from EID0 to EID15), the duration of storage was shown to impair embryo viability: 4.7% mortality on the 65 viable D10-eggs vs. 1% mortality on the 105 viable D3-eggs.
In addition, the sex ratio determined on viable eggs was not equilibrated, especially at EID11 and EID15 after 10 days of incubation (Figure 5A). However, due to the small number of eggs analysed, we could not conclude on the effect of storage on sex ratio at each incubation day. After 15 days of incubation, the sex ratio of the 20 viable eggs was in favour of males (65 and 70% for D3-EID15 and D10-EID15 groups, respectively). Analysis of D3 and D10 groups (after combination of EID11, EID13 and EID15 eggs) indicated that sex ratios were comparable between the two groups, with a slight predominance of female embryos, regardless of the storage time (56/57% females vs. 43/44% males, Figure 5B). Therefore, from this experiment and considering the entire incubation period studied (EID11 to EID15), we concluded that there was no effect of storage duration on the sex ratio of the embryos.
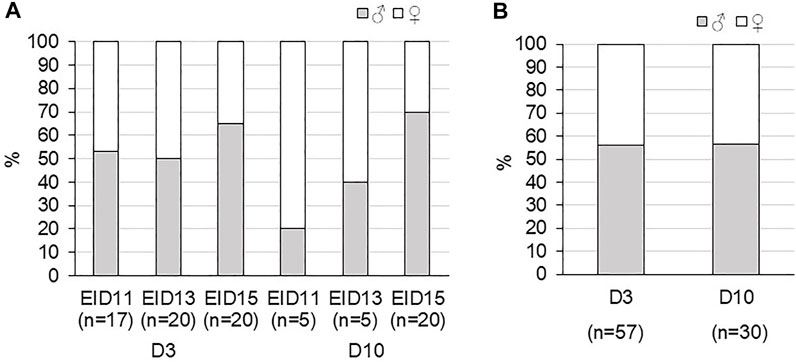
FIGURE 5. Sex ratio of fertilised eggs during incubation after three or 10 days of storage (D3 and D10, respectively) after 11 (EID11), 13 (EID13) and 15 (EID15) days of incubation. (A). Sex ratio at each stage of incubation. (B). Sex ratio in D3 and D10 groups (considering all EID11, EID13 and EID15 eggs).
MRI images were analysed to measure volumes of internal egg components and the embryo (Figure 6).
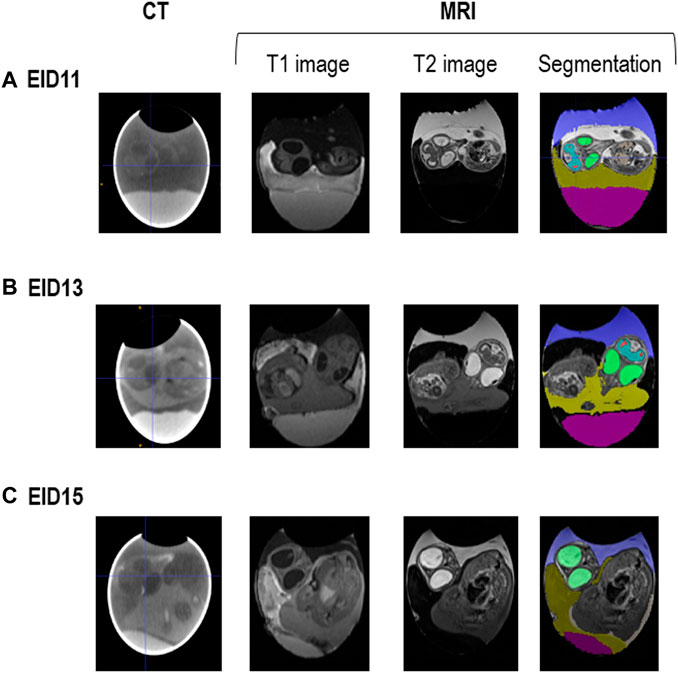
FIGURE 6. CT and MRI representative images of fertilised eggs during incubation. (A). After 11 days of incubation (EID11). (B). After 13 days of incubation (EID13). (C). After 15 days of incubation (EID15). For segmentation (right panel), colours are as follows: in green, eyes; in blue and red spots, brain; in dark blue/purple, allantoic fluid; in pink, egg white; in yellowish colour, yolk. The amniotic fluid corresponds to the white zone between the embryo and the allantoic fluid on A (EID11, right panel) and to the dark zone between the embryo and the allantoic fluid on B (EID13, right panel). The change in the MRI contrast of the amniotic fluid between EID11 and EID13/EID15 (white to black), is due to the transfer of egg white into the amniotic cavity from EID12 onwards.
Independent of storage time, the volumes of egg white, air chamber, allantoic fluid were shown to decrease over time (p < 0.05) during embryonic development, while those of eyes, brain and embryo increased (p < 0.05) (Table 3).
Pair comparisons between D3 and D10 groups at each developmental stage revealed a decrease in eye, brain and embryo (or embryo + yolk for EID13 and EID15 stages) volumes at EID11 and EID15 for D10 eggs, compared with D3 eggs (Table 3). Similarly, these embryo volumes at EID13, tended to be lower in the D10 group compared with the D3 group, although no statistical difference was observed (Table 3). Collectively, these data support that the embryonic development is delayed after a 10-days storage but not altered (absence of visible malformations).
Embryo weight increased similarly between D3 and D10 groups (Figure 7). However, embryos from D10 eggs were significantly lighter than embryos from D3 eggs, especially after 13 (EID13, p < 0.0001) and 15 (EID15, p < 0.0001) days of incubation.
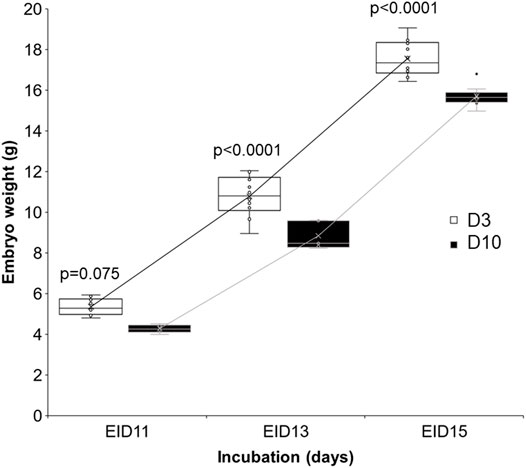
FIGURE 7. Effect of storage time on embryo weight after 11, 13 and 15 days of incubation. D3 and D10 eggs were opened at EID11, EID13 and EID15 after CT or MRI analyses and weighed. Statistical analysis was performed using one-way ANOVA with p < 0.05.
Discussion
In birds, the embryo can pause its development until incubation, when the temperature is too cold or until the clutch size is optimal for brooding. This dormancy is characterised by cell arrest in the G (2) phase and suppression of apoptosis (Ko et al., 2017). However, extended storage impairs hatchability due to its deleterious effects on blastoderm viability. The detrimental effect of extended storage (over 7 days) on egg quality, embryo mortality and hatchability has been extensively reviewed in the literature (Asmundson, 1947; Brake et al., 1997; Fasenko, 2007; Bakst et al., 2012; Bakst et al., 2016a; Branum et al., 2016; Abioja et al., 2021; Melo et al., 2021; Özlü et al., 2021). Such negative effects are very well known to turkey and chicken breeders but they can have difficulties avoiding long storage practices for logistical reasons. Prolonged egg storage alters many egg quality parameters and may deteriorate the blastoderm to the point that it cannot resume upon incubation. Several studies have reported a decrease in viable blastodermal cells after storage over 8 days (Bakst et al., 2012). To mitigate such a negative effect of prolonged storage, strategies have been developed. These include short periods of incubation during egg storage (SPIDES) that reactivate embryo metabolism prior to incubation, thereby increasing the viability of embryos until hatching (Dymond et al., 2013; Damaziak et al., 2018). Some authors also reported a positive hatchability effect of storage at cooled temperatures [15°C, (Bakst and Gupta, 1997); 11.6°C (Guinebretière et al., 2022)], egg turning (Melo et al., 2021) or of in ovo injection of biological buffers to reinforce the buffering capacity of the albumen (Akhlaghi et al., 2013). The mechanisms underlying the reduced viability of blastodermal cells during storage have been partly elucidated: increases in expression of genes associated with apoptosis, oxidative stress and fatty acid metabolism (Hamidu et al., 2011; Bakst et al., 2016b). However, there are only few studies that investigated the impact of prolonged storage on the development of viable embryos. Some authors have described a reduced femur and tibia length being positively correlated with the duration of preincubation storage (Yalcin and Siegel, 2003), while others reported an altered growth of heart and liver (Christensen et al., 2002) and a slower metabolic rate (Christensen et al., 2001; Fasenko, 2007). In this study, we used non-invasive imaging approaches (MRI and CT) to investigate the impact of prolonged storage on egg quality, and on the growth and development of the embryo. Such imaging methods have previously been used to assess egg quality (Hutchison et al., 1992), to identify the localisation of the blastoderm (Bartels et al., 2008) and to monitor brain, liver or eye development (Bain et al., 2007; Boss et al., 2008; Zhou et al., 2015; Lindner et al., 2017).
We first explored how storage duration at 16°C, 80% relative humidity (classical hatchery conditions) for zero, three and 10 days affects egg quality. We used a combination of non-invasive CT on intact eggs and classical measurements on internal egg quality traits after egg breakage. In accordance with numerous articles and reviews (Benton and Brake, 1996; Brake et al., 1997; Abioja et al., 2021), we showed that the length of storage affects several egg parameter traits including egg white pH, Haugh units, yolk index and air chamber volume (Table 2). The volume assigned to the blastoderm tends to increase upon storage, especially when comparing three and 10 days of storage (Mann–Whitney test, p = 0.060), which corroborates previous studies (Bakst et al., 2012; Abioja et al., 2021). The analyses of the 30 eggs (10 eggs at D0, D3 and D10) revealed that the position of the blastoderm is always localised at the surface of the yolk, roughly in the middle (Figure 3), when the large end of the egg is maintained on the top (air chamber located at the top). Only one egg exhibiting a blastoderm oriented towards the bottom was noticed. It would have been interesting to put this egg back into the incubator to assess how this specific orientation impacts embryo development and whether it was still associated with a viable chick or not. Anyway, this observation suggests that CT imaging of eggs before storage to verify the localisation of the blastoderm may help the development of new experiments to determine whether this parameter (blastoderm location) is a predictor of hatchability.
Knowing that the increase in blastoderm volume is unlikely correlated with cell proliferation or the number of viable cells (Bakst et al., 2012), we hypothesised that it may correspond to the dispersion of the blastoderm cells as the vitelline membrane is losing its mechanical strength. Such a hypothesis is in accordance with the decrease of yolk index (Table 2) and the changes in yolk/white physicochemical properties. Further studies on the impact of extended storage (egg white physicochemistry, ultrastructure of the vitelline membrane that supports the embryo) may be useful to identify the determinant parameters that alter embryo survival and restarting. We believe that the quality of the vitelline membrane is crucial in the early stages of incubation as its inner layer contains many proteins that are assumed to support the development of the embryo and the expansion of the yolk sac during embryogenesis (Brégeon et al., 2022). Alteration of the perivitelline membrane is supposed to be a key determinant that can explain very early mortality. Although the duration of storage is detrimental to embryo survival, it is noteworthy that incubation of freshly laid eggs (that are characterised by high viscosity and neutral pH) is not correlated with higher embryo survival (Benton and Brake, 1996). Altogether these data highlight the major role of egg components that surround the blastoderm, on cell survival and hatching success. This observation is partly corroborated by our data, where storage up to 10 days at 17°C, 80% RH did not affect fertility (the number of eggs that restarted was comparable between D3 and D10 eggs) but impaired embryo viability (4.7% mortality for D10 eggs vs. 1% mortality for D3 eggs during incubation). The reason why some eggs resist extended storage more is not known but it probably results from many variables including genetics, egg quality (including composition) and embryo specificities. Christensen et al. reported that embryos from a genetic line that resisted storage mortality maintained greater glycogen concentrations in muscle and heart tissues than those from a line and old hens associated with reduced survival rates (Christensen et al., 2001).
Hence, similar to MRI (Hutchison et al., 1992; Klein et al., 2002; Burkhardt et al., 2011), CT imaging can help to localise the position of the blastoderm, as confirmed in this study and others (Bartels et al., 2008), which might be particularly interesting to develop tools that require information from the embryo. For example, identifying the exact position of the blastoderm on the yolk may help in developing sexing methods, as suggested previously (Burkhardt et al., 2011) and to differentiate an early-dead embryo from an unfertilised germinal disc (Bakst et al., 2016a). In this study, CT has been used to estimate the localisation of the blastoderm during storage but it might be interesting to further explore how the CT signal associated with the blastoderm is changing during the first 3 days of incubation, and whether this method can be used to monitor early stages of embryonic development.
Interestingly, the sex ratio of viable embryos was shown to be comparable between the two experimental (D3 and D10) groups (Figure 5). The verification of this parameter was essential to avoid any bias associated with the sex of the embryo, knowing that the maturation/growth of the embryo may differ between males and females, even during early stages (Tagirov and Golovan, 2015; Hirst et al., 2018). Such information remains important in the context of the development of methods to avoid the culling of male chicks. Indeed, storage or incubation methods that could imbalance the sex ratio in favour of females would diminish the number of male embryos and chicks to eliminate (Gautron et al., 2021).
Data related to embryo weight and brain, embryo and eye volume (Table 3; Figure 7) all support that storing eggs for 10 days negatively affects embryo growth. Similar to our results, body mass of EID15 embryos was previously shown to be significantly affected by a storage duration of up to 3 weeks (p < 0.001) (Branum et al., 2016). It was also reported that the acid-base balance of embryos was modified according to storage duration (Branum et al., 2016). However, storing eggs for 10 days does not seem to affect the kinetics of embryo development (similar growth curves, Figure 7). Further studies on a higher number of eggs are needed to increase the statistical significance of some parameters including egg white and allantoic fluid volumes (Table 3). Notably, at EID15, the egg white volumes tended to be higher in D10 eggs than D3 eggs (2.93 ± 0.96 and 3.19 ± 0.86 in D3 and D10 eggs, respectively). A similar trend was observed for the volume of allantoic fluid, which was higher in D10 than D3 eggs (5.08 ± 0.95 and 5.86 ± 1.67 in D3 and D10 eggs, respectively). These observations corroborate the aforementioned conclusion that the growth/developmental stage of D10 eggs was delayed compared with D3 eggs. Indeed, the decrease in egg white volume, which is located at the bottom of the egg, is concomitant with its transfer into the amniotic cavity between EID11 and EID12 (Da Silva et al., 2019). From EID13 onwards, the amniotic fluid/egg white mix will start to be absorbed orally by the embryo as a source of amino-acids, to accompany its growth. The higher volume of egg white noticed in D10 eggs compared to D3 eggs suggests that the egg white transfer into the amniotic cavity was also delayed in D10 eggs.
Further research should include analyses of other physiological/phenotypical traits including organ growth, embryo positioning within the egg and initiation of skeletal mineralisation, to complete the story and facilitate the identification of indicators of normal or abnormal development/growth. To our knowledge, only a few articles report such experimental studies (Christensen et al., 2001; Christensen et al., 2002; Yalcin and Siegel, 2003; Fasenko, 2007). Our data suggest that the physiological stages of EID11, EID13 and EID15 embryos are more advanced for D3 eggs than D10 eggs and that the development of D10 embryos is delayed compared with D3 embryos. Although our experimental design did not include incubation up to hatching, other studies reported that chicks originating from eggs stored for a long period hatched later than embryos from eggs stored for a short period (Christensen et al., 2002; Tona et al., 2003a; Tona et al., 2003b). However, we have no evidence to date that the duration of storage induces abnormalities.
Additional data on the volume of embryo organs and supporting structures, the orientation of the embryo inside the egg and the movements of egg structures during incubation should also help to revise the atlas of chicken development (Hamburger and Hamilton, 1951). Indeed, most modern chicken lines have been selected for decades on performance, egg or meat quality, and there is an increasing number of articles that alerts on differences in metabolism and health between commercial genotypes (Koenen et al., 2002; Tona et al., 2004; Buzała et al., 2015). Comparisons of the embryonic development (organogenesis, growth, kinetics) between several contrasted phenotypic lines should help to investigate the impact of genetic selection on embryos, whose proper development predetermines health and welfare of chicks and adult chickens. Integrative studies considering the normal development of the embryo using several levels of structural organisation (from the embryo within the egg to the molecular profiling of egg/embryo contents) are lacking. New data from experiments addressing the impact of genetics, age and nutrition of reproductive hens, egg storage and incubation conditions on egg and embryo specificities may be useful for the development of predictive tools (Yimenu et al., 2017) to model egg quality (for both table and fertilised eggs) and developmental kinetics of embryos, upon exposure of eggs to suboptimal conditions.
Conclusion
In this work, we demonstrated: 1) that the storage of fertilised eggs up to 10 days is associated with a decrease in several egg quality parameters; and 2) that the development of embryos exposed to extended egg storage is delayed compared to those stored for a shorter period. These data combined with published works suggest that eggs exposed to different storage durations are likely associated with differences in embryo maturity and hatching time. This observation underlines the necessity to improve the homogeneity of egg batches in terms of storage conditions to narrow the hatching window, thereby limiting the time spent by new hatched chicks in hatchers (without access to water and food). This work also evidences the relevance of imaging techniques to monitor the development of bird embryos during incubation but also to visualise and quantify how egg components (egg white, extraembryonic fluids) are modified throughout incubation. Classical measurements of egg quality parameters and embryo development usually require the egg opening and embryo killing. In this respect, CT and MRI techniques are non-invasive approaches. Although some egg quality parameters cannot be determined using these techniques (egg white pH and viscosity, yolk colour and index), they facilitate the analysis of some egg and embryonic components that are usually difficult to measure/evaluate (volume of the air chamber and extraembryonic fluids, embryo positioning, and movement of egg structures during storage and incubation).
Data Availability Statement
The raw data supporting the conclusions of this article will be made available by the authors, without undue reservation.
Ethics Statement
Ethical review and approval was not required for the embryo study. The experimental procedure meets the guidelines approved by the Institutional Animal Care and Use Committee (IACUC). All experiments were conducted in compliance with the European legislation on the “Protection of Animals Used for Experimental and Other Scientific Purposes” (2010/63/UE) and under the supervision of an authorised scientist (S. Réhault-Godbert, Authorisation no. 37-144).
Author Contributions
HA performed MRI image acquisition and supervised data analyses. VP analysed MRI and CT data, performed segmentation for volume determination and participated in data interpretation. IC contributed to segmentation, statistical analyses and participated in discussions. NB and MH performed experiments on eggs and embryos after egg opening and contributed to data analyses. NB conducted molecular sexing experiments. FL performed CT experiments and contributed to discussions. SR-G conceived, designed, participated in experiments and supervised the entire study. SR-G and HA wrote the first draft of the manuscript. All authors reviewed the manuscript and approved the submitted version.
Funding
The authors thank the Region Centre Val de Loire and the PHASE research department (INRAE) for financing MH's PhD. This study received financial support from the PHASE research department (INRAE, ImEGGging project, 2019, France).
Conflict of Interest
The authors declare that the research was conducted in the absence of any commercial or financial relationships that could be construed as a potential conflict of interest.
Publisher’s Note
All claims expressed in this article are solely those of the authors and do not necessarily represent those of their affiliated organizations, or those of the publisher, the editors and the reviewers. Any product that may be evaluated in this article, or claim that may be made by its manufacturer, is not guaranteed or endorsed by the publisher.
Acknowledgments
The authors are grateful to Joel Delaveau and Christophe Rat (INRAE, PEAT, Centre Val de Loire, DOI: 10.15454/1.5572326250887292E12) for egg handling and incubation.
Abbreviations
CT, computed tomography; D, day; EID, embryonic incubation day; MRI, magnetic resonance imaging; RH, relative humidity.
References
Abioja M. O., Abiona J. A., Akinjute O. F., Ojoawo H. T. (2021). Effect of Storage Duration on Egg Quality, Embryo Mortality and Hatchability in FUNAAB-α Chickens. J. Anim. Physiol. Anim. Nutr. 105 (4), 715–724. doi:10.1111/jpn.13480
Akhlaghi A., Jafari Ahangari Y., Hashemi S. R., Navidshad B., Pirsaraei Z. A., Deldar H., et al. (2013). Prestorage in Ovo Injection of Biological Buffers: An Approach to Improve Hatchability in Long-Term Stored Eggs. Poult. Sci. 92 (4), 874–881. doi:10.3382/ps.2012-02610
Asmundson V. S. (1947). Time Held Prior to Incubation and Hatchability of Turkey Eggs. Poult. Sci. 26 (3), 305–307. doi:10.3382/ps.0260305
Bain M. M., Fagan A. J., Mullin J. M., McNaught I., McLean J., Condon B. (2007). Noninvasive Monitoring of Chick Development in Ovo Using a 7T MRI System from Day 12 of Incubation Through to Hatching. J. Magn. Reson. Imaging 26 (1), 198–201. doi:10.1002/jmri.20963
Bakst M. R., Akuffo V., Nicholson D., French N. (2012). Comparison of Blastoderm Traits From 2 Lines of Broilers Before and After Egg Storage and Incubation. Poult. Sci. 91 (10), 2645–2648. doi:10.3382/ps.2011-02118
Bakst M. R., Gupta S. K. (1997). Preincubation Storage of turkey Eggs: Impact on Rate of Early Embryonic Development. Br. Poult. Sci. 38 (4), 374–377. doi:10.1080/00071669708418005
Bakst M. R., Welch G. R., Camp M. J. (2016a). Observations of Turkey Eggs Stored up to 27 Days and Incubated for 8 Days: Embryo Developmental Stage and Weight Differences and the Differentiation of Fertilized from Unfertilized Germinal Discs. Poult. Sci. 95 (5), 1165–1172. doi:10.3382/ps/pew010
Bakst M. R., Welch G. R., Fetterer R., Miska K. (2016b). Impact of Broiler Egg Storage on the Relative Expression of Selected Blastoderm Genes Associated with Apoptosis, Oxidative Stress, and Fatty Acid Metabolism. Poult. Sci. 95 (6), 1411–1417. doi:10.3382/ps/pew038
Bartels T., Fischer B., Krüger P., Koch E., Ryll M., Krautwald-Junghanns M. E. (2008). 3D-X-Ray Microcomputer Tomography and Optical Coherence Tomography as Methods for the Localization of the Blastoderm in the Newly Laid Unincubated Chicken Egg. Dtsch. Tierarztl Wochenschr 115 (5), 182–188.
Benton C. E., Brake J. (1996). The Effect of Broiler Breeder Flock Age and Length of Egg Storage on Egg Albumen During Early Incubation. Poult. Sci. 75 (9), 1069–1075. doi:10.3382/ps.0751069
Boss A., Oppitz M., Wehrl H. F., Rossi C., Feuerstein M., Claussen C. D., et al. (2008). Measurement of T1, T2, and Magnetization Transfer Properties During Embryonic Development at 7 Tesla Using the Chicken Model. J. Magn. Reson. Imaging 28 (6), 1510–1514. doi:10.1002/jmri.21601
Brake J., Walsh T. J., Benton C. E., Petitte J. N., Meijerhof R., Peñalva G. (1997). Egg Handling and Storage. Poult. Sci. 76 (1), 144–151. doi:10.1093/ps/76.1.144
Branum S. R., Tazawa H., Burggren W. W. (2016). Phenotypic Developmental Plasticity Induced by Preincubation Egg Storage in Chicken Embryos (Gallus gallus domesticus). Physiol. Rep. 4 (4), e12712. doi:10.14814/phy2.12712
Brégeon M., Tomas D., Bernay B., Zatylny-Gaudin C., Georgeault S., Labas V., et al. (2022). Multifaceted Roles of the Egg Perivitelline Layer in Avian Reproduction: Functional Insights from the Proteomes of Chicken Egg Inner and Outer Sublayers. J. Proteomics 258, 104489. doi:10.1016/j.jprot.2022.104489
Burkhardt A., Meister S., Bergmann R., Koch E. (2011). Influence of Storage on the Position of the Germinal Disc in the Fertilized Unincubated Chicken Egg. Poult. Sci. 90 (10), 2169–2173. doi:10.3382/ps.2010-01179
Buzała M., Janicki B., Czarnecki R. (2015). Consequences of Different Growth Rates in Broiler Breeder and Layer Hens on Embryogenesis, Metabolism and Metabolic Rate: A Review. Poult. Sci. 94 (4), 728–733. doi:10.3382/ps/pev015
Christensen V. L., Wineland M. J., Fasenko G. M., Donaldson W. E. (2001). Egg Storage Effects on Plasma Glucose and Supply and Demand Tissue Glycogen Concentrations of Broiler Embryos. Poult. Sci. 80 (12), 1729–1735. doi:10.1093/ps/80.12.1729
Christensen V., Wineland M., Fasenko G., Donaldson W. (2002). Egg Storage Alters Weight of Supply and Demand Organs of Broiler Chicken Embryos. Poult. Sci. 81 (11), 1738–1743. doi:10.1093/ps/81.11.1738
Da Silva M., Dombre C., Brionne A., Monget P., Chessé M., De Pauw M., et al. (2019). The Unique Features of Proteins Depicting the Chicken Amniotic Fluid. Mol. Cell. Proteomics 18 (Suppl. 1), S174–s190. doi:10.1074/mcp.RA117.000459
Damaziak K., Pawęska M., Gozdowski D., Niemiec J. (2018). Short Periods of Incubation, Egg Turning During Storage and Broiler Breeder Hens Age for Early Development of Embryos, Hatching Results, Chicks Quality and Juvenile Growth. Poult. Sci. 97 (9), 3264–3276. doi:10.3382/ps/pey163
Dymond J., Vinyard B., Nicholson A. D., French N. A., Bakst M. R. (2013). Short Periods of Incubation During Egg Storage Increase Hatchability and Chick Quality in Long-Stored Broiler Eggs ,. Poult. Sci. 92 (11), 2977–2987. doi:10.3382/ps.2012-02816
Elibol O., Peak S., Brake J. (2002). Effect of Flock Age, Length of Egg Storage, and Frequency of Turning During Storage on Hatchability of Broiler Hatching Eggs. Poult. Sci. 81 (7), 945–950. doi:10.1093/ps/81.7.945
Fasenko G. M., Christensen V. L., Wineland M. J., Petitte J. N. (2001). Examining the Effects of Prestorage Incubation of Turkey Breeder Eggs on Embryonic Development and Hatchability of Eggs Stored for Four or Fourteen Days. Poult. Sci. 80 (2), 132–138. doi:10.1093/ps/80.2.132
Fasenko G. M. (2007). Egg Storage and the Embryo. Poult. Sci. 86 (5), 1020–1024. doi:10.1093/ps/86.5.1020
Gautron J., Réhault-Godbert S., Van de Braak T. G. H., Dunn I. C. (2021). Review: What Are the Challenges Facing the Table Egg Industry in the Next Decades and What Can Be Done to Address Them? Animal 15 (Suppl. 1), 100282. doi:10.1016/j.animal.2021.100282
Goliomytis M., Tsipouzian T., Hager-Theodorides A. L. (2015). Effects of Egg Storage on Hatchability, Chick Quality, Performance and Immunocompetence Parameters of Broiler Chickens. Poult. Sci. 94 (9), 2257–2265. doi:10.3382/ps/pev200
Grochowska E., Kinal A., Sobek Z., Siatkowski I., Bednarczyk M. (2019). Field Study on the Factors Affecting Egg Weight Loss, Early Embryonic Mortality, Hatchability, and Chick Mortality with the Use of Classification Tree Technique. Poult. Sci. 98 (9), 3626–3636. doi:10.3382/ps/pez180
Guinebretière M., Puterflam J., Keïta A., Réhault-Godbert S., Thomas R., Chartrin P., et al. (2022). Storage Temperature or Thermal Treatments During Long Egg Storage Duration Influences Hatching Performance and Chick Quality. Front. Physiol. 13, 852733. doi:10.3389/fphys.2022.852733
Hamburger V., Hamilton H. L. (1951). A Series of Normal Stages in the Development of the Chick Embryo. J. Morphol. 88 (1), 49–92. doi:10.1002/jmor.1050880104
Hamidu J. A., Uddin Z., Li M., Fasenko G. M., Guan L. L., Barreda D. R. (2011). Broiler Egg Storage Induces Cell Death and Influences Embryo Quality. Poult. Sci. 90 (8), 1749–1757. doi:10.3382/ps.2011-01361
Hassan S. M., Siam A. A., Mady M. E., Cartwright A. L. (2005). Egg Storage Period and Weight Effects on Hatchability of Ostrich (Struthio camelus) Eggs. Poult. Sci. 84 (12), 1908–1912. doi:10.1093/ps/84.12.1908
He L., Martins P., Huguenin J., Van T.-N. -N., Manso T., Galindo T., et al. (2019). Simple, Sensitive and Robust Chicken Specific Sexing Assays, Compliant with Large Scale Analysis. PLoS One 14 (3), e0213033. doi:10.1371/journal.pone.0213033
Hirst C. E., Major A. T., Smith C. A. (2018). Sex Determination and Gonadal Sex Differentiation in the Chicken Model. Int. J. Dev. Biol. 62 (1-2-3), 153–166. doi:10.1387/ijdb.170319cs
Hutchison M. J., Lirette A., Etches R. J., Towner R. A., Janzen E. G. (1992). Research Note: An Assessment of Egg Yolk Structure Using Magnetic Resonance Imaging. Poult. Sci. 71 (12), 2117–2121. doi:10.3382/ps.0712117
Hyánková L., Novotná B. (2013). Divergent Selection for Shape of Growth Curve in Japanese Quail. 7. Effect of Egg Storage at High Temperature on Embryo Development and Hatchability. Br. Poult. Sci. 54 (6), 695–703. doi:10.1080/00071668.2013.853285
Kantarcioglu E., Kahilogullari G., Zaimoglu M., Atmis E. O., Peker E., Yigman Z., et al. (2018). The Effect of Magnetic Resonance Imaging on Neural Tube Development in an Early Chicken Embryo Model. Childs Nerv. Syst. 34 (5), 933–938. doi:10.1007/s00381-018-3734-9
Klein S., Rokitta M., Baulain U., Thielebein J., Haase A., Ellendorff F. (2002). Localization of the Fertilized Germinal Disc in the Chicken Egg Before Incubation. Poult. Sci. 81 (4), 529–536. doi:10.1093/ps/81.4.529
Ko M. H., Hwang Y. S., Rim J. S., Han H. J., Han J. Y. (2017). Avian Blastoderm Dormancy Arrests Cells in G (2) and Suppresses Apoptosis. FASEB J. 31 (8), 3240–3250. doi:10.1096/fj.201601051RR
Koenen M. E., Boonstra-Blom A. G., Jeurissen S. H. (2002). Immunological Differences Between Layer- and Broiler-Type Chickens. Vet. Immunol. Immunopathol. 89 (1-2), 47–56. doi:10.1016/s0165-2427(02)00169-1
Kouame Y. A. E., Nideou D., Kouakou K., Tona K. (2019). Effect of Guinea Fowl Egg Storage Duration on Embryonic and Physiological Parameters, and Keet Juvenile Growth. Poult. Sci. 98 (11), 6046–6052. doi:10.3382/ps/pez264
Lapão C., Gama L., Soares M. (1999). Effects of Broiler Breeder Age and Length of Egg Storage on Albumen Characteristics and Hatchability. Poult. Sci. 78 (5), 640–645. doi:10.1093/ps/78.5.640
Lindner T., Klose R., Streckenbach F., Stahnke T., Hadlich S., Kühn J.-P., et al. (2017). Morphologic and Biometric Evaluation of Chick Embryo Eyes in Ovo Using 7 Tesla MRI. Sci. Rep. 7 (1), 2647. doi:10.1038/s41598-017-02755-4
Melo E. F., Araújo I. C. S., Triginelli M. V., Castro F. L. S., Baião N. C., Lara L. J. C. (2021). Effect of Egg Storage Duration and Egg Turning During Storage on Egg Quality and Hatching of Broiler Hatching Eggs. Animal 15 (2), 100111. doi:10.1016/j.animal.2020.100111
Mróz E., Murawska D., Jankowski J., Stępińska M., Przywitowski M., Otowski K. (2019). The Effects of Hen's Age and Egg Storage Time on the Frequency of Occurrence of Physical Defects in Turkey Poults. Poult. Sci. 98 (12), 7097–7100. doi:10.3382/ps/pez481
Nowaczewski S., Kontecka H., Rosiński A., Koberling S., Koronowski P. (2010). Egg Quality of Japanese Quail Depends on Layer Age and Storage Time. Folia Biol. (Krakow) 58 (3-4), 201–207. doi:10.3409/fb58_3-4.201-207
Özlü S., Uçar A., Erkuş T., Nicholson A. D., Elibol O. (2021). Research Note: Effects of Turning and Short Period of Incubation During Long-Term Egg Storage on Embryonic Development and Hatchability of Eggs From Young and Old Broiler Grandparent Flocks. Poult. Sci. 100 (4), 101026. doi:10.1016/j.psj.2021.101026
Pokhrel N., Sela-Donenfeld D., Cinnamon Y. (2021). The Chick Blastoderm During Diapause, a Landmark for Optimization of Preincubation Storage Conditions. Poult. Sci. 100 (8), 101227. doi:10.1016/j.psj.2021.101227
Reijrink I. A. M., Berghmans D., Meijerhof R., Kemp B., van den Brand H. (2010). Influence of Egg Storage Time and Preincubation Warming Profile on Embryonic Development, Hatchability, and Chick Quality. Poult. Sci. 89 (6), 1225–1238. doi:10.3382/ps.2009-00182
Reis L. H., Gama L., Soares M. (1997). Effects of Short Storage Conditions and Broiler Breeder Age on Hatchability, Hatching Time, and Chick Weights. Poult. Sci. 76 (11), 1459–1466. doi:10.1093/ps/76.11.1459
Tagirov M., Golovan S. (2015). Sexual Dimorphism in the Early Embryogenesis of the Chicken (Gallus Gallus domesticus). Mol. Reprod. Dev. 82 (5), 332–343. doi:10.1002/mrd.22476
Taha A. E., El-Tahawy A. S., Abd El-Hack M. E., Swelum A. A., Saadeldin I. M. (2019). Impacts of Various Storage Periods on Egg Quality, Hatchability, Post-Hatching Performance, and Economic Benefit Analysis of Two Breeds of Quail. Poult. Sci. 98 (2), 777–784. doi:10.3382/ps/pey468
Tona K., Bamelis F., De Ketelaere B., Bruggeman V., Moraes V., Buyse J., et al. (2003a). Effects of Egg Storage Time on Spread of Hatch, Chick Quality, and Chick Juvenile Growth. Poult. Sci. 82 (5), 736–741. doi:10.1093/ps/82.5.736
Tona K., Malheiros R., Bamelis F., Careghi C., Moraes V., Onagbesan O., et al. (2003b). Effects of Storage Time on Incubating Egg Gas Pressure, Thyroid Hormones, and Corticosterone Levels in Embryos and on Their Hatching Parameters. Poult. Sci. 82 (5), 840–845. doi:10.1093/ps/82.5.840
Tona K., Onagbesan O. M., Jego Y., Kamers B., Decuypere E., Bruggeman V. (2004). Comparison of Embryo Physiological Parameters During Incubation, Chick Quality, and Growth Performance of Three Lines of Broiler Breeders Differing in Genetic Composition and Growth Rate. Poult. Sci. 83 (3), 507–513. doi:10.1093/ps/83.3.507
Wong E. A., Uni Z. (2021). Centennial Review: The Chicken Yolk Sac Is a Multifunctional Organ. Poult. Sci. 100 (3), 100821. doi:10.1016/j.psj.2020.11.004
Yalcin S., Gursel I., Bilgen G., Horuluoglu B. H., Gucluer G., Izzetoglu G. T. (2017). Effect of Egg Storage Duration and Brooding Temperatures on Chick Growth, Intestine Morphology and Nutrient Transporters. Animal 11 (10), 1791–1797. doi:10.1017/s1751731117000404
Yalcin S., Gursel I., Bilgen G., Izzetoglu G. T., Horuluoglu B. H., Gucluer G. (2016). Egg Storage Duration and Hatch Window Affect Gene Expression of Nutrient Transporters and Intestine Morphological Parameters of Early Hatched Broiler Chicks. Animal 10 (5), 805–811. doi:10.1017/s175173111500261x
Yalçin S., Siegel P. B. (2003). Developmental Stability of Broiler Embryos in Relation to Length of Egg Storage Prior to Incubation. J. Poult. Sci. 40 (4), 298–308. doi:10.2141/jpsa.40.298
Yimenu S. M., Kim J. Y., Koo J., Kim B. S. (2017). Predictive Modeling for Monitoring Egg Freshness During Variable Temperature Storage Conditions. Poult. Sci. 96 (8), 2811–2819. doi:10.3382/ps/pex038
Yushkevich P. A., Piven J., Hazlett H. C., Smith R. G., Ho S., Gee J. C., et al. (2006). User-Guided 3D Active Contour Segmentation of Anatomical Structures: Significantly Improved Efficiency and Reliability. Neuroimage 31 (3), 1116–1128. doi:10.1016/j.neuroimage.2006.01.015
Keywords: chicken, egg, storage, duration, egg quality, embryonic development, magnetic resonance imaging, computed tomography
Citation: Adriaensen H, Parasote V, Castilla I, Bernardet N, Halgrain M, Lecompte F and Réhault-Godbert S (2022) How Egg Storage Duration Prior to Incubation Impairs Egg Quality and Chicken Embryonic Development: Contribution of Imaging Technologies. Front. Physiol. 13:902154. doi: 10.3389/fphys.2022.902154
Received: 22 March 2022; Accepted: 25 April 2022;
Published: 31 May 2022.
Edited by:
Edgar Orlando Oviedo, North Carolina State University, United StatesReviewed by:
Tatiana Carlesso Santos, State University of Maringa, Brazil Krzysztof Damaziak,Warsaw University of Life Sciences, PolandCopyright © 2022 Adriaensen, Parasote, Castilla, Bernardet, Halgrain, Lecompte and Réhault-Godbert. This is an open-access article distributed under the terms of the Creative Commons Attribution License (CC BY). The use, distribution or reproduction in other forums is permitted, provided the original author(s) and the copyright owner(s) are credited and that the original publication in this journal is cited, in accordance with accepted academic practice. No use, distribution or reproduction is permitted which does not comply with these terms.
*Correspondence: Sophie Réhault-Godbert, c29waGllLnJlaGF1bHQtZ29kYmVydEBpbnJhZS5mcg==