- Laboratory of Intestinal Neuro-immune Interaction, Translational Research Center for Gastrointestinal Disorders, Department of Chronic Diseases, Metabolism and Ageing, KU Leuven, Leuven, Belgium
Serine proteases are heavily present in the gastrointestinal tract where they are essential in numerous physiological processes. An imbalance in the proteolytic activity is a central mechanism underlying abdominal pain in irritable bowel syndrome (IBS). Therefore, protease inhibitors are emerging as a promising therapeutic tool to manage abdominal pain in this functional gastrointestinal disorder. With this review, we provide an up-to-date overview of the implications of serine proteases in the development of abdominal pain in IBS, along with a critical assessment of the current developments and prospects of protease inhibitors as a therapeutic tool. In particular, we highlight the current knowledge gap concerning the identity of dysregulated serine proteases that are released by the rectal mucosa of IBS patients. Finally, we suggest a workflow with state-of-the-art techniques that will help address the knowledge gap, guiding future research towards the development of more effective and selective protease inhibitors to manage abdominal pain in IBS.
Introduction
Irritable bowel syndrome (IBS) is a highly prevalent disorder of the gut-brain axis affecting 4% of the world population (Sperber et al., 2021). Also according to the Rome IV criteria, IBS is diagnosed when a patient has recurrent abdominal pain that is associated with a change in stool frequency and/or form (Drossman, 2016). Also bloating, gas and cramping are common symptoms, but their presence is not necessary for diagnosis. IBS prevalence is higher in women than in men; female-to-male odds ratio of 1.7 (1.5–1.9), while geographical location does not seem to have an impact (Sperber et al., 2021). Effective therapy to manage the debilitating symptoms is however still lacking and the costs associated with diagnosis and work absenteeism place a heavy burden on both the patient and the health care systems (Drossman et al., 2009; Flacco et al., 2019).
The symptoms of IBS are likely a manifestation of several contributing mechanisms, as depicted in Figure 1A. Visceral hypersensitivity (VHS), an increased pain perception in the gastrointestinal tract, is a major contributing factor to abdominal pain in IBS (Farzaei et al., 2016). Mechanical, thermal, or chemical information in the gut wall is sensed by extrinsic, primary afferent neurons with cell bodies in the dorsal root ganglia (DRG). The signal is transduced to different somatosensory areas in the brain via secondary neurons in the spinal cord, where it is subject to central processing to be perceived as noxious or not (Vermeulen et al., 2014). Peripheral sensitization of extrinsic, primary afferents is determined by the expression of specific channels that are involved in sensing noxious stimuli (Anand et al., 2007). In particular, the transient receptor potential (TRP) channels, more specifically TRPA1, TRPV1, and TRPV4, were shown to be sensitized in submucosal neurons of rectal biopsies from IBS patients, thereby contributing to VHS (Wouters et al., 2016; Balemans et al., 2017, 2019). Besides histamine, bradykinin, and serotonin, also proteases have emerged as mediators that can sensitize these channels and consequently contribute to VHS in IBS (Farzaei et al., 2016; Brizuela et al., 2021).
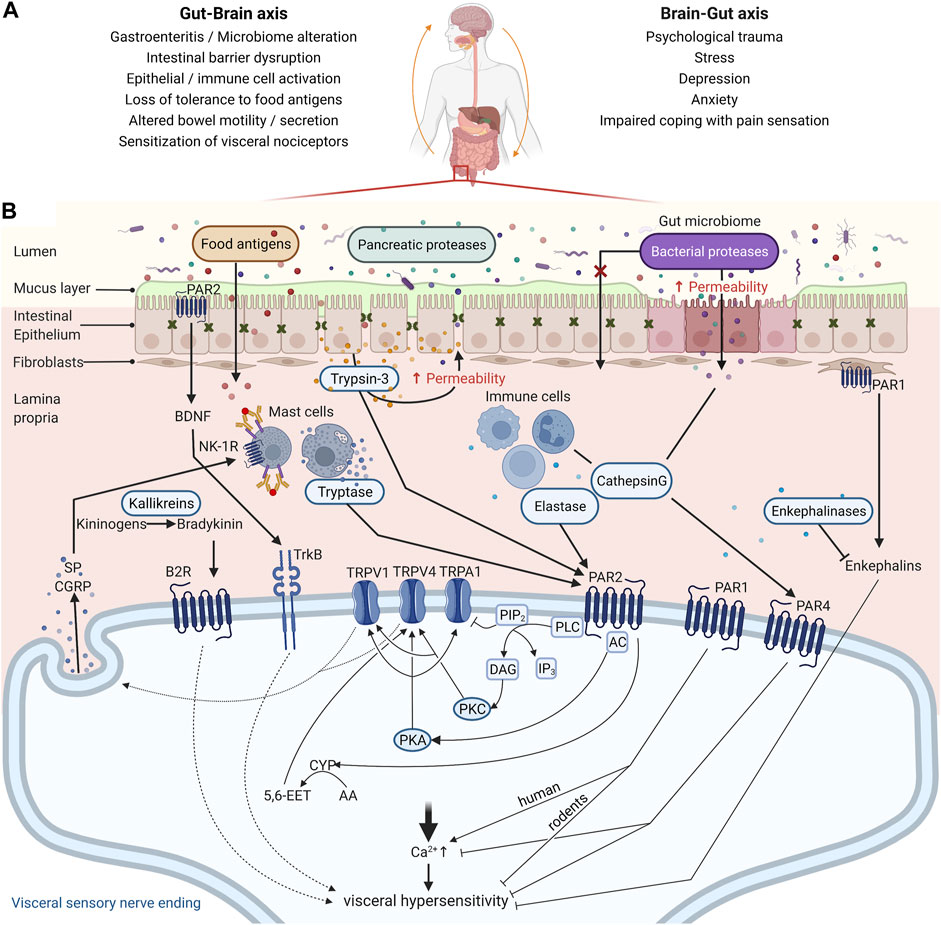
FIGURE 1. Mechanisms contributing to abdominal pain associated with irritable bowel syndrome (IBS). (A) The pathophysiology of IBS is tightly associated with perturbations in the Gut-Brain Axis, which encompasses a bi-directional communication between these organs. Increasing reports indicate that gastroenteritis may promote intestinal barrier disruption, activation of epithelial and immune cells in the gut mucosa, and loss of tolerance to dietary antigens. Upon re-exposure to those dietary antigens, mast cells degranulate and their mediators sensitize nociceptors leading to visceral hypersensitivity. Conversely, IBS is also associated with a higher prevalence of psychiatric disorders such as depression and anxiety, factors that affect coping with pain sensation. A history of psychological trauma and stress are also risk factors for IBS, although the mechanisms involved remain unclear. (B) Peripheral sensitization of the gut innervation is a central mechanism underlying abdominal pain in IBS, and proteases are emerging as central mediators in this process. Proteases from the lumen (pancreatic or microbial), epithelial cells (trypsin-3), and immune cells (tryptase, elastase, and cathepsin (G) participate in the disruption of the intestinal barrier and can signal to visceral nociceptors and mediate VHS via protease-activated receptor (PAR) activation. PAR2 activation on the nerve endings of visceral nociceptors induces VHS through TRPV1, TRPV4, and/or TRPA1 sensitization after Gɑs or Gɑq recruitment, depending on the protease. TRPV1 and TRPV4 on sensory neurons also mediate the release of substance P (SP) and Calcitonin gene-related peptide (CGRP), further contributing to the pain signal. Additionally, proteases modulate synthesis and secretion of respectively bradykinin and brain-derived neurotrophic factor (BDNF), compounds found to be involved in inflammatory pain. On the other hand, activation of PAR1 and PAR4 has an analgesic effect in mice, while in humans PAR1 is emerging as pro-nociceptive. For PAR4 this is likely mediated through reducing intracellular calcium levels. Whether PAR1 mediates VHS due to activation of the receptor in the sensory nerve endings or fibroblasts remains to be investigated in animal models of IBS and patient samples. Unlike the other PARs, PAR3 seems to rather act as a cofactor for PAR1 and PAR4 signaling by potentiating the cleavage by proteases like thrombin, but its role still warrants further investigation. Transient Receptor Potential Cation Channel Subfamily V Member 1 (TRPV1) and 4 (TRPV4); Transient Receptor Potential Cation Channel Subfamily A Member 1 (TRPA1); Adenylyl Cyclase (AC); Protein Kinase A (PKA); Phospholipase C (PLC); Protein Kinase C (PKC); Cytochrome P450 Epoxygenase (CYP); Arachidonic Acid (AA); 5,6-epoxy-8Z,11Z, 14Z-eicosatrienoic acid (5,6-EET); Neurokinin-1 Receptor (NK1-R); Bradykinin Receptor B2 (B2R); Neurotrophic Receptor Tyrosine Kinase 2 (TrkB); Phosphatidylinositol 4,5-bisphosphate (PIP2); Inositol 1,4,5-trisphosphate (IP3); Diacyl Glycerol (DAG). Created with BioRender.com.
Proteases are enzymes that hydrolyze peptide bonds, which can result not only in the inactivation of the target but also in the activation of signaling molecules and receptors. In the gastrointestinal tract, while proteases are best known for their role in food digestion, they are now emerging as pivotal mediators of increased intestinal epithelial permeability and visceral pain (Cenac, 2013; Van Spaendonk et al., 2017). Among the different classes of proteases, serine proteases are most likely to mediate pain since they are predominantly released to the extracellular milieu (Vergnolle, 2016). This family of proteases is characterized by the presence of a nucleophilic serine in the reactive site and is further subdivided into catalytic groups according to substrate preference as chymotrypsin-, trypsin- and elastase-like serine proteases (Ovaere et al., 2009). This review focuses on the role of serine proteases in the pathophysiology of VHS in IBS, as well as the therapeutic potential of their inhibition.
How Proteases Mediate Visceral Pain in IBS
Proteases can modulate visceral pain through several mechanisms as illustrated in Figure 1B, which are discussed in the following paragraphs. The most extensively studied mechanism to date, is via activation of protease-activated receptors (PARs). PARs belong to a family of cell-surface signaling proteins called G protein-coupled receptors and the four members of the PAR family, PAR1-4, are expressed throughout the body, including various cell types in the intestines, such as epithelial cells, mast cells, and neurons (Vergnolle, 2005). Different proteases can cleave the receptors at different sites and thereby induce different signaling pathways or even inactivate the receptor (Gottesman, 2021). Trypsin and tryptase activate canonical signaling after cleavage of PAR2, leading to G-protein mediated signaling via downstream messengers such as PKC (Dai et al., 2004; Amadesi et al., 2006; Grant et al., 2007). Cathepsin S and elastase cleave elsewhere and activate biased signaling, which includes G-protein mediated activation of cAMP and PKA (Zhao et al., 2015). Hence, the profile of active proteases within a tissue microenvironment is a major determinant of the downstream PAR signaling taking place, with direct consequences for nociception.
Evidence from pre-clinical studies has indicated that proteases can promote VHS in mice via PAR2-mediated sensitization of TRPA1 and TRPV4. Intracolonic administration of PAR2 activating peptide causes visceral hyperalgesia in wild-type mice, but not in TRPA1 knock-out mice, suggesting that TRPA1 is needed to mediate PAR2-induced hyperalgesia (Cattaruzza et al., 2010). Additionally, using TRPV4-targeted siRNA and PAR2 knock-out mice, Cenac and others show that colonic biopsy supernatants from IBS-D patients induce VHS when administered in the colon, in a TRPV4-dependant mechanism by activating PAR2 in sensory neurons (Cenac et al., 2015). A link between PAR2 activation and TRPV1 sensitization has also been shown in murine DRG neurons and models of somatic pain, but this remains to be explored further in visceral pain (Amadesi et al., 2006). PAR1 activation induces antinociceptive effects and even appears to prevent visceral hyperalgesia in mice (Asfaha et al., 2002; Kawao et al., 2004; Martin et al., 2009). However, studies on human enteric and DRG neurons showed that colonic biopsy supernatant from IBS patients could activate the neurons via PAR1, but not PAR2 (Mueller et al., 2011; Kugler et al., 2012; Buhner et al., 2018; Desormeaux et al., 2018). This might suggest very distinct roles for PAR1 and PAR2 in humans versus rodents and might be explained in part by the fact that the class of serine proteases has many lineage-specific differences between humans and mice (Puente et al., 2003). PARs also have other ways to signal than calcium mobilization that would be of interest to investigate in regard to TRP sensitization (Zhao et al.,2014a; 2014b; Sostegni et al., 2015). Interestingly, expression of both PAR1 and PAR4, also suggested to be antinociceptive, was lowered in IBS patients compared to healthy controls, while PAR2 levels were unchanged (Bian et al., 2009; Zhao et al., 2012). To date, there is no data showing co-expression of TRP channels and PARs on human DRGs or sensory nerve endings in human colonic biopsies. Nevertheless, both TRP channels and PARs are broadly expressed in nociceptive neurons; therefore, their co-expression on nerve endings innervating the gut is quite certain (Cenac, 2013; Balemans et al., 2017; Desormeaux et al., 2018).
Proteases can also convert molecules that are active on pain pathways. Bradykinin for example, a potent proinflammatory mediator, is generated through cleavage of kininogens by kallikreins, which are serine proteases, and can induce hyperalgesia via TRPV1 and TRPA1 sensitization (Mizumura et al., 2009). The contribution of the kallikrein-kinin pathway in experimental acute colitis is without controversy, however, there is a lack of studies investigating the role of this mechanism in VHS (Stadnicki, 2011). Endogenous opioids are modulators of nociceptive signaling promoting analgesia of which the activity is also regulated by serine proteases. Enkephalins, one of the endogenous opioid families, are known to promote ion and water absorption in the gut, as well as play a role in visceral nociception (Mosinska et al., 2016). Aminopeptidase N (ANPEP), a metallopeptidase, is an important enzyme involved in the degradation of enkephalins. In colonic biopsies taken from inflamed regions of patients with inflammatory bowel disease (IBD), increased levels of enkephalins were described but protease activity levels were not assessed (Owczarek et al., 2011). To date, reports regarding levels of ANPEP or endogenous opioids in IBS patients are lacking, but levels of opioid receptor expression were significantly lower in the colon of diarrhea-predominant IBS patients compared to healthy controls (Zielińska et al., 2015).
Proteases in Visceral Hypersensitivity
An increase in serine protease activity, mainly trypsin-like, in colonic tissue or tissue supernatants of IBS patients compared to healthy controls has been reported by several groups (Barbara et al., 2004; Cenac et al., 2007; Buhner et al., 2009; Rolland-Fourcade et al., 2017; Aguilera-Lizarraga et al., 2021). Accordingly, elevated tryptase and trypsin mRNA and protein levels were shown (Zhao et al., 2012; Liang et al., 2016). The increase in tryptase levels was correlated with a higher number of mast cells in the mucosa by some groups (Barbara et al., 2004; Bian et al., 2009; Buhner et al., 2009) although others reported no increase in mast cell count (Cenac et al., 2007; Wouters et al., 2016). Most recently, we provided compelling evidence that mast cells in the rectal mucosa of IBS patients are more sensitized with IgE, thereby more prone to degranulation and tryptase release upon antigen stimulation (Aguilera-Lizarraga et al., 2021). Trypsin-3, as revealed by in situ zymography, is expressed by the intestinal epithelium and upregulated in IBS (Rolland-Fourcade et al., 2017). Thrombin, recently identified as upregulated in IBD patients (Denadai-Souza et al., 2018), did not differ, at least in expression levels, between IBS patients and controls (Bian et al., 2009). Interestingly, proteome analysis with LC-MS/MS (liquid chromatography with tandem mass spectrometry) revealed upregulation of elastase-like and not trypsin-like serine proteases in colonic biopsy supernatants from IBS patients (Buhner et al., 2018). However, no complementary experiments were done to confirm this finding. Also, in feces of IBS patients, researchers found an increase in serine protease activity from either the patient or the intestinal bacteria (Annaházi et al., 2009; Tooth et al., 2014; Edogawa et al., 2020). Although these enzymes are less likely to play a direct role in the sensitization of intestinal nociceptors since they would first have to cross the epithelial barrier, they might be involved in barrier dysfunction (Steck et al., 2012; Lomax et al., 2019).
Protease Inhibitors in Visceral Hypersensitivity
With increasing evidence that serine proteases contribute to the pathophysiology of IBS, also the search for effective protease inhibitors as therapeutic agents has started. Targeting proteases has resulted in several great success stories in other conditions such as HIV and cardiovascular diseases but it remains a challenging endeavor overall (Turk, 2006; Drag, 2010). To date, no protease inhibitors are marketed or in clinical trials for IBS and only few studies have explored the effects of serine protease inhibitors in experimental models of VHS.
Nafamostat mesylate, also called FUT-175, is a short-acting, synthetic inhibitor of serine proteases with poor oral bioavailability that has been approved in Japan as a treatment for acute pancreatitis and disseminated intravascular coagulation (Cao et al., 2008). FUT-175 could prevent development of VHS in WT mice when co-administered intracolonic with IBS supernatants (Cenac et al., 2007). Additionally, a single intraperitoneal injection of FUT-175 normalized visceral sensitivity in post-colitis rats (Ceuleers et al., 2018). Camostat mesylate, or FOY-305, has similar properties and indications as FUT-175. Intragastric pretreatment with FOY-305 could prevent acute stress-induced VHS in rats (Zhao et al., 2011). Both inhibitors target a broad-spectrum of serine proteases, have anticoagulation activity and are associated with rare, but important side effects such as hyperkalemia, as well as mild complications like headache (Sawada et al., 2016; Quinn et al., 2022). It is important to emphasize that the broad range of targets and intravenous administration (for FUT-175) are crucial determinants of the risk for side effects. Improved targeting of the inhibitors is definitely needed when considering these compounds as treatment options for a chronic and not life-threatening condition such as IBS. Alternatively, oral compounds that act locally and are not systemically absorbed are most likely the safest approach with minimal risk of side effects.
Two newly developed serine protease inhibitors, UAMC-00050 and UAMC-01162, have a more favorable inhibitory profile with a limited inhibition of proteases related to the blood coagulation cascade, but a good inhibition of tryptase (Joossens et al., 2007). Neither had an effect on visceral sensitivity in healthy control animals but successfully reversed VHS in post-colitis rats in the highest dose used (1 mg/kg UAM-00050; 2.5 mg/kg UAMC-01162) (Ceuleers et al., 2018). Plant-derived Bowman-Birk inhibitors are specific toward trypsin and chymotrypsin and are present in vegetables like soybeans. Oral administration of soy germ extract for 15 days resulted in a decrease in visceral sensitivity, fecal protease activity, and PAR2 expression in a stress-induced rat model for VHS (Moussa et al., 2013). However, soy germ extract also contains phytoestrogens and an estrogen-receptor antagonist reversed all effects, except the decrease in fecal proteolytic activity (Moussa et al., 2013). The positive effect of soy germ extract is therefore likely a result of the combination of estrogen-receptor and PAR2 mediated pathways. A randomized, double-blind, placebo-controlled trial done in patients with ulcerative colitis indicated that soy extract is safe although not very effective (Lichtenstein et al., 2008).
Another therapeutic strategy is to increase the bioactive life-span of endogenous opioids in the gut and thereby relieve abdominal pain. Agonists of the µ-opioid receptor are already on the market for diarrhea-predominant IBS, e.g. loperamide, but these drugs can cause severe secondary constipation and do not adequately resolve abdominal pain (Moayyedi et al., 2019). Inhibiting opioid degrading enzymes would have an effect that is restricted in time and space, allowing better control of pharmacological activity. Additionally, repeated administration of RB101, the first dual enkephalinase inhibitor, did not induce tolerance or physical dependence in early animal studies, unlike opioid receptor agonists such as morphine (Noble et al.,1992a; 1992b). Sialorphin, a natural enkephalinase inhibitor, was recently studied for its analgesic potential in a mouse model of visceral pain induced by colorectal distension (Fabisiak et al., 2018). They showed that subcutaneous administration of sialorphin increased the pain threshold comparable to the opioid Leu-enkephalin. Nevertheless, further and more targeted research investigating the potency and side effects of these inhibitors is necessary.
Protease inhibitors with greater specificity have not been tested for their effects on VHS, mainly because the exact targets still need to be identified. However, the following inhibitors might be of interest to explore further. Leupeptin, an organic compound produced by many Actinomycetales bacteria in the human intestinal tract, targets serine proteases (trypsin, plasmin, and kallikrein) and cysteine proteases (papain and cathepsin B), but not thrombin, elastase, or chymotrypsin. This inhibitor was used by Rolland-Fourcade et al. to demonstrate that lipopolysaccharide-stimulation of intestinal epithelial cells results in basolateral release of trypsin-like proteases (Rolland-Fourcade et al., 2017). APC-366 is a tryptase-specific inhibitor and was employed recently to discriminate tryptase activity from total trypsin-like activity (Aguilera-Lizarraga et al., 2021). Initially, it was developed to treat asthma, allergy, and colitis, but it lacked potency and selectivity. This inhibitor also has high affinity for thrombin, a pivotal enzyme in blood coagulation, thus when not applied locally, APC-366 could lead to bleeding. APC-2059 had improved specificity and seemed to be useful as adjuvant therapy in ulcerative colitis in an open-label, phase two clinical study (Tremaine et al., 2002). However, it needed to be injected and was not developed further.
This indicates that drugability is a critical aspect that requires a lot of attention. For compound UAMC-00050 the efficacy decreased when given locally via an enema versus intraperitoneal injection (Ceuleers et al., 2018; Hanning et al., 2021). Plasma concentrations were very low after intracolonic administration and on average only 5.4% of the plasma concentration after intravenous administration although a five times larger dose was used for local application. This suggests that the inhibitor mainly stays in the lumen or intestinal mucosa. The animals were observed for 3 h after administration of the drug and no side effects were noted. Nonetheless, it would be more relevant for clinical trials if the compounds can be taken orally. Giardina et al. developed a novel inhibitor of human b-tryptase with good oral bioavailability (Giardina et al., 2018). As a dimeric inhibitor, it bridges two active sites resulting in increased affinity and specificity compared to monomeric controls. But unlike previously reported polyvalent inhibitors, this one contains a disiloxane linker, which greatly improves the bioavailability after oral administration. This compound would be of great interest to explore in the context of IBS. The same applies for the use of recombinant lactic acid bacteria to deliver serine protease inhibitors to the intestinal mucosa (Bermúdez-Humarán et al., 2015). However, only biologicals can be delivered via this system and these tend to have a broader selectivity.
Towards More Selective Protease Targeting
The two major factors hampering the development of specific protease inhibitors are the superficial identification of overactive serine proteases in this disorder, as well as the limited mapping of substrate preferences of relevant proteases. Activity-based probes (ABPs) provide a solution for the first obstacle. The reactive group of the probe binds specifically and irreversibly to the proteases of interest. This allows identification of the bound proteases by mass spectrometry after affinity-based purification. The use of these probes is slowly increasing in the field of inflammatory and functional bowel diseases, which can only be encouraged further (Mayers et al., 2017; Denadai-Souza et al., 2018; Anderson et al., 2019; Solà-Tapias et al., 2020). To tackle the second obstacle; the hybrid combinatorial substrate library (HyCoSuL) using natural and unnatural amino acids, is an efficient way to find highly selective protease substrates (Kasperkiewicz et al., 2014). A HyCoSuL for serine proteases would consist of three sublibraries of tetrapeptides with a fixed amino acid at P1 and amino acid mixtures at the P4-P2 positions as well as an ACC (7-aminocoumarin-4-acetic acid) fluorescent tag occupying the P1’ position. Once the tetrapeptide is cleaved, the ACC produces a fluorescent signal.
HyCoSuL has proven to be a valuable tool to study protease substrate preference, however, it is limited to the amino acid residues N-terminal to the cleavage site (Poreba et al., 2018; Anderson et al., 2019). Internally quenched fluorescent substrates are an excellent way to study the specificity of both the prime and non-prime pockets but are inefficient for large screenings. A very good tool for screening a large (up to 1010) and diverse pool of substrates is phage display technology (Kehoe, 2005; Caberoy et al., 2011). Random peptides are expressed on the phage surface and subjected to protease cleavage followed by phage amplification if successfully cleaved. Thus, optimal substrates are enriched over multiple rounds of selection. A major drawback is that the substrates are label-free, so they need to be resynthesized with an appropriate reporter group to compare the kinetics of the best substrates. Label-free substrates are not per se a bad thing; not having a label right next to the cleavage site means it also cannot influence the enzyme-substrate binding interactions. Other approaches to study substrate specificity without the use of reporter tags utilize mass spectrometry (O’Donoghue et al., 2012; Wood et al., 2017; Lapek et al., 2019). A beautiful example by De Bruyn et al. in an acute- and a post-colitis rat model illustrates this is a valuable way to investigate the identity and cleavage patterns of proteases in biological samples (De Bruyn et al., 2021). By applying this method, the authors demonstrated that tryptase and trypsin-3 also unmask PAR1. Nevertheless, also this technique is somewhat biased and requires prior knowledge of the proteases that are being studied. It is important to note that these methods do not exclude each other, on the contrary, they are complementary and provide extra strength where there is overlap.
Combining target identification via ABPs and inhibitor design via HyCoSuL should provide a reliable and accessible toolbox to design highly specific protease inhibitors for the management of abdominal pain in IBS (Figure 2A). Testing the effectiveness and safety of promising serine protease inhibitors should be done in animal models of IBS to maximize translational value (Figure 2B). Although these models inherently are limited in reflecting the complex disease mechanisms underlying IBS, they are able to induce VHS, an important factor in IBS and the target of these protease inhibitors (Accarie, 2020). It must be noted that the research investigating the role of serine proteases in VHS in these models is in its infancy and the translational value of the results requires further exploration.
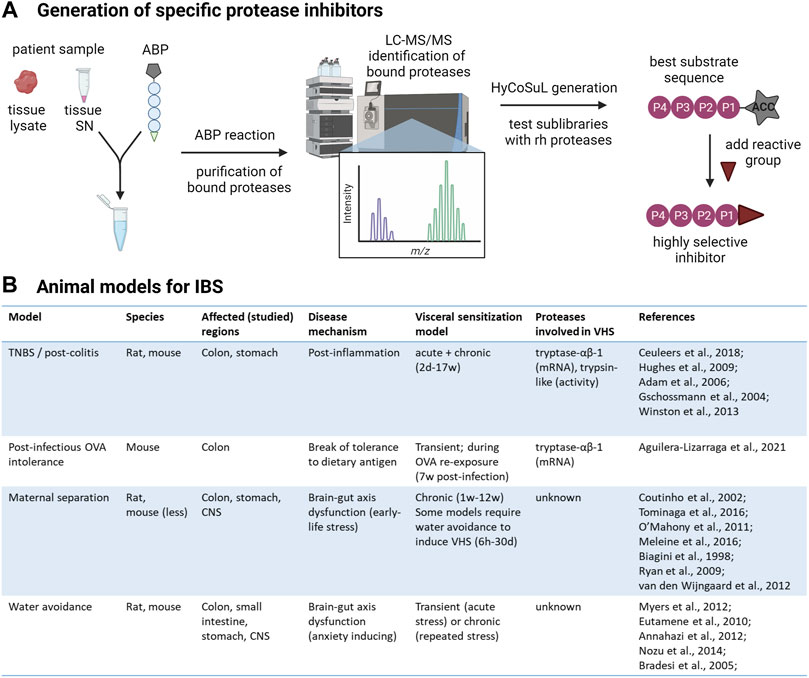
FIGURE 2. From protease identification to inhibitor design. (A) Workflow to develop highly selective protease inhibitors. First, serine proteases present in patient samples, whole tissue lysate or supernatant (SN) containing secreted mediators, will be labeled with desthiobiotin-tagged activity-based probes (ABPs) specific for this class. The desthiobiotin-tag of the ABP is represented as a pentagon, the circles represent the recognition element, and the reactive group that will bind the attacking protease is represented as a triangle. The bound proteases will be isolated with paramagnetic beads conjugated to streptavidin and analyzed by LC-MS/MS. This will unambiguously inform the researcher about the identity of all active serine proteases in the sample and which ones are increased in IBS patients compared to controls. Next, hybrid combinatorial substrate libraries (HyCoSuL) will be developed to determine the substrate specificities of the identified protease(s). For serine proteases, three tetrapeptide sublibraries are made with a fixed amino acid at the P1 position, according to the serine protease of interest. The protease will attack the bond between the amino acid at P1 and the fluorogenic reporter (7-aminocoumarin-4-acetic acid, ACC) at the P1′ position so that hydrolysis can be measured in a plate reader. In each sublibrary, one of the three remaining positions in the tetrapeptide (P2-P4) has a fixed amino acid while the remaining positions contain an equimolar mixture of amino acids. Ultimately, the optimal substrates can be determined using recombinant human (rh) proteases by combining the results from all sublibraries. With prior knowledge about the specificity of the protease, the palette of amino acids can be customized to minimize the number of sublibraries. The best substrate sequence will be used as a scaffold to synthesize highly specific protease inhibitors. (B) Animal models of IBS to test the effectiveness of serine protease inhibitors on abdominal pain. TNBS: Trinitrobenzene sulfonic acid; OVA: ovalbumin; CNS: central nervous system (Adam et al., 2006; Annaházi et al., 2012; Biagini et al., 1998; Bradesi et al., 2005; Coutinho et al., 2002; Eutamene et al., 2010; Gschossmann et al., 2004; Hughes et al., 2009; Meleine et al., 2016; Myers and Greenwood-Van Meerveld, 2012; Nozu et al., 2014; O' Mahony et al., 2011; Ryan et al., 2009; Tominaga et al., 2016; van den Wijngaard et al., 2012; Winston and Sarna, 2013). Created with BioRender.com.
Conclusion
The investigation of protease dysregulation in gastrointestinal diseases, and more specifically in VHS and IBS, has definitely increased in recent years, bringing protease inhibition to the forefront of one of the most promising novel therapeutic strategies to explore. However, three important factors need to be addressed to advance serine protease inhibitors as a treatment for VHS in IBS. The first is the limited knowledge of the targets. The dysregulated proteases need to be characterized unambiguously and on a patient level, an endeavor that can be achieved with activity-based probes. Secondly, as underlined in this review, proteases have many important physiological roles and any inhibition will have to be carefully targeted to limit side effects. Accurate mapping of substrate specificities of the proteases of interest with a technique like HyCoSuL will facilitate and accelerate the development of highly specific serine protease inhibitors. The third and final limiting factor is that the current oral bioavailability of serine protease inhibitors is poor and improvement of formulations favoring local delivery should be prioritized to give the inhibitors a higher chance to succeed in clinical trials for IBS.
Author Contributions
LD prepared the figures and drafted the manuscript; LD and AD-S edited the manuscript; LD, AD-S, and GB revised and approved the final version of the manuscript.
Funding
This work was funded by the FWO PhD fellowship no. 11B8920N (to L.D.) and the FWO-SBO grant no. S001017N.
Conflict of Interest
The authors declare that the research was conducted in the absence of any commercial or financial relationships that could be construed as a potential conflict of interest.
Publisher’s Note
All claims expressed in this article are solely those of the authors and do not necessarily represent those of their affiliated organizations, or those of the publisher, the editors and the reviewers. Any product that may be evaluated in this article, or claim that may be made by its manufacturer, is not guaranteed or endorsed by the publisher.
References
Accarie A., Vanuytsel T. (2020). Animal Models for Functional Gastrointestinal Disorders. Front. Psychiatry 11, 509681. doi:10.3389/fpsyt.2020.509681
Adam B., Liebregts T., Gschossmann J. M., Krippner C., Scholl F., Ruwe M., et al. (2006). Severity of Mucosal Inflammation as a Predictor for Alterations of Visceral Sensory Function in a Rat Model. Pain 123, 179–186. doi:10.1016/j.pain.2006.02.029
Aguilera-Lizarraga J., Florens M. V., Viola M. F., Jain P., Decraecker L., Appeltans I., et al. (2021). Local Immune Response to Food Antigens Drives Meal-Induced Abdominal Pain. Nature 590, 151–156. doi:10.1038/s41586-020-03118-2
Amadesi S., Cottrell G. S., Divino L., Chapman K., Grady E. F., Bautista F., et al. (2006). Protease-Activated Receptor 2 Sensitizes TRPV1 by Protein Kinase Cɛ- and A-Dependent Mechanisms in Rats and Mice. J. Physiol. 575, 555–571. doi:10.1113/jphysiol.2006.111534
Anand P., Aziz Q., Willert R., Van Oudenhove L. (2007). Peripheral and Central Mechanisms of Visceral Sensitization in Man. Neurogastroenterol. Motil. 19, 29–46. doi:10.1111/j.1365-2982.2006.00873.x
Anderson B. M., Poole D. P., Aurelio L., Ng G. Z., Fleischmann M., Kasperkiewicz P., et al. (2019). Application of a Chemical Probe to Detect Neutrophil Elastase Activation During Inflammatory Bowel Disease. Sci. Rep. 9, 13295. doi:10.1038/s41598-019-49840-4
Annaházi A., Dabek M., Gecse K., Salvador-Cartier C., Polizzi A., Rosztóczy A., et al. (2012). Proteinase-Activated Receptor-4 Evoked Colorectal Analgesia in Mice: An Endogenously Activated Feed-Back Loop in Visceral Inflammatory Pain. Neurogastroenterol. Motil. 24, 76–e13. doi:10.1111/j.1365-2982.2011.01805.x
Annaházi A., Gecse K., Dabek M., Ait-Belgnaoui A., Rosztóczy A., Róka R., et al. (2009). Fecal Proteases from Diarrheic-IBS and Ulcerative Colitis Patients Exert Opposite Effect on Visceral Sensitivity in Mice. Pain 144, 209–217. doi:10.1016/j.pain.2009.04.017
Asfaha S., Brussee V., Chapman K., Zochodne D. W., Vergnolle N. (2002). Proteinase-Activated Receptor-1 Agonists Attenuate Nociception in Response to Noxious Stimuli. Br. J. Pharmacol. 135, 1101–1106. doi:10.1038/sj.bjp.0704568
Balemans D., Aguilera-Lizarraga J., Florens M. V., Jain P., Denadai-Souza A., Viola M. F., et al. (2019). Histamine-Mediated Potentiation of Transient Receptor Potential (TRP) Ankyrin 1 and TRP Vanilloid 4 Signaling in Submucosal Neurons in Patients with Irritable Bowel Syndrome. Am. J. Physiology-Gastrointestinal Liver Physiology 316, G338–G349. doi:10.1152/ajpgi.00116.2018
Balemans D., Boeckxstaens G. E., Talavera K., Wouters M. M. (2017). Transient Receptor Potential Ion Channel Function in Sensory Transduction and Cellular Signaling Cascades Underlying Visceral Hypersensitivity. Am. J. Physiology-Gastrointestinal Liver Physiology 312, G635–G648. doi:10.1152/ajpgi.00401.2016
Barbara G., Stanghellini V., De Giorgio R., Cremon C., Cottrell G. S., Santini D., et al. (2004). Activated Mast Cells in Proximity to Colonic Nerves Correlate with Abdominal Pain in Irritable Bowel Syndrome. Gastroenterology 126, 693–702. doi:10.1053/j.gastro.2003.11.055
Bermúdez-Humarán L. G., Motta J.-P., Aubry C., Kharrat P., Rous-Martin L., Sallenave J.-M., et al. (2015). Serine Protease Inhibitors Protect Better Than IL-10 and TGF-β Anti-Inflammatory Cytokines Against Mouse Colitis When Delivered by Recombinant Lactococci. Microb. Cell Fact. 14, 26. doi:10.1186/s12934-015-0198-4
Biagini G., Pich E. M., Carani C., Marrama P., Agnati L. F. (1998). Postnatal Maternal Separation During the Stress Hyporesponsive Period Enhances the Adrenocortical Response to Novelty in Adult Rats by Affecting Feedback Regulation in the CA1 Hippocampal Field. Int. J. Dev. Neurosci. 16, 187–197. doi:10.1016/s0736-5748(98)00019-7
Bian Z. X., Li Z., Huang Z. X., Zhang M., Chen H. L., Xu H. X., et al. (2009). Unbalanced Expression of Protease-Activated Receptors-1 and -2 in the Colon of Diarrhea-Predominant Irritable Bowel Syndrome Patients. J. Gastroenterol. 44, 666–674. doi:10.1007/s00535-009-0058-2
Bradesi S., Schwetz I., Ennes H. S., Lamy C. M. R., Ohning G., Fanselow M., et al. (2005). Repeated Exposure to Water Avoidance Stress in Rats: A New Model for Sustained Visceral Hyperalgesia. Am. J. Physiology-Gastrointestinal Liver Physiology 289, G42–G53. doi:10.1152/ajpgi.00500.2004
Brizuela M., Castro J., Harrington A. M., Brierley S. M. (2021). Pruritogenic Mechanisms and Gut Sensation: Putting the “Irritant” into Irritable Bowel Syndrome. Am. J. Physiology-Gastrointestinal Liver Physiology 320, G1131–G1141. doi:10.1152/ajpgi.00331.2020
Buhner S., Hahne H., Hartwig K., Li Q., Vignali S., Ostertag D., et al. (2018). Protease Signaling Through Protease Activated Receptor 1 Mediate Nerve Activation by Mucosal Supernatants from Irritable Bowel Syndrome but Not from Ulcerative Colitis Patients. PLoS One 13, e0193943–16. doi:10.1371/journal.pone.0193943
Buhner S., Li Q., Vignali S., Barbara G., De Giorgio R., Stanghellini V., et al. (2009). Activation of Human Enteric Neurons by Supernatants of Colonic Biopsy Specimens from Patients with Irritable Bowel Syndrome. Gastroenterology 137, 1425–1434. doi:10.1053/j.gastro.2009.07.005
Caberoy N., Alvarado G., Li W. (2011). Identification of Calpain Substrates by ORF Phage Display. Molecules 16, 1739–1748. doi:10.3390/molecules16021739
Cao Y.-g., Zhang M., Yu D., Shao J.-p., Chen Y.-c., Liu X.-q. (2008). A Method for Quantifying the Unstable and Highly Polar Drug Nafamostat Mesilate in Human Plasma with Optimized Solid-Phase Extraction and ESI-MS Detection: More Accurate Evaluation for Pharmacokinetic Study. Anal. Bioanal. Chem. 391, 1063–1071. doi:10.1007/s00216-008-2054-4
Cattaruzza F., Spreadbury I., Miranda-Morales M., Grady E. F., Vanner S., Bunnett N. W. (2010). Transient Receptor Potential Ankyrin-1 Has a Major Role in Mediating Visceral Pain in Mice. Am. J. Physiology-Gastrointestinal Liver Physiology 298, G81–G91. doi:10.1152/ajpgi.00221.2009
Cenac N., Andrews C. N., Holzhausen M., Chapman K., Cottrell G., Andrade-Gordon P., et al. (2007). Role for Protease Activity in Visceral Pain in Irritable Bowel Syndrome. J. Clin. Investig. 117, 636–647. doi:10.1172/JCI29255.636
Cenac N., Bautzova T., Le Faouder P., Veldhuis N. A., Poole D. P., Rolland C., et al. (2015). Quantification and Potential Functions of Endogenous Agonists of Transient Receptor Potential Channels in Patients with Irritable Bowel Syndrome. Gastroenterology 149, 433–444. e7. doi:10.1053/j.gastro.2015.04.011
Cenac N. (2013). Protease-Activated Receptors as Therapeutic Targets in Visceral Pain. Cn 11, 598–605. doi:10.2174/1570159X113119990039
Ceuleers H., Hanning N., Heirbaut J., Van Remoortel S., Joossens J., Van Der Veken P., et al. (2018). Newly Developed Serine Protease Inhibitors Decrease Visceral Hypersensitivity in a Post-Inflammatory Rat Model for Irritable Bowel Syndrome. Br. J. Pharmacol. 175, 3516–3533. doi:10.1111/bph.14396
Coutinho S. V., Plotsky P. M., Sablad M., Miller J. C., Zhou H., Bayati A. I., et al. (2002). Neonatal Maternal Separation Alters Stress-Induced Responses to Viscerosomatic Nociceptive Stimuli in Rat. Am. J. Physiology-Gastrointestinal Liver Physiology 282, G307–G316. doi:10.1152/ajpgi.00240.2001
Dai Y., Moriyama T., Higashi T., Togashi K., Kobayashi K., Yamanaka H., et al. (2004). Proteinase-Activated Receptor 2-Mediated Potentiation of Transient Receptor Potential Vanilloid Subfamily 1 Activity Reveals a Mechanism for Proteinase-Induced Inflammatory Pain. J. Neurosci. 24, 4293–4299. doi:10.1523/JNEUROSCI.0454-04.2004
De Bruyn M., Ceuleers H., Hanning N., Berg M., De Man J. G., Hulpiau P., et al. (2021). Proteolytic Cleavage of Bioactive Peptides and Protease-Activated Receptors in Acute and Post-Colitis. Ijms 22, 10711. doi:10.3390/ijms221910711
Denadai-Souza A., Bonnart C., Tapias N. S., Marcellin M., Gilmore B., Alric L., et al. (2018). Functional Proteomic Profiling of Secreted Serine Proteases in Health and Inflammatory Bowel Disease. Sci. Rep. 8, 1–9. doi:10.1038/s41598-018-26282-y
Desormeaux C., Bautzova T., Garcia-Caraballo S., Rolland C., Barbaro M. R., Brierley S. M., et al. (2018a). Protease-Activated Receptor 1 Is Implicated in Irritable Bowel Syndrome Mediators-Induced Signaling to Thoracic Human Sensory Neurons. Pain 159, 1257–1267. doi:10.1097/j.pain.0000000000001208
Drag M., Salvesen G. S. (2010). Emerging Principles in Protease-Based Drug Discovery. Nat. Rev. Drug Discov. 9, 690–701. doi:10.1038/nrd3053
Drossman D. A., Chang L., Schneck S., Blackman C., Norton W. F., Norton N. J. (2009). A Focus Group Assessment of Patient Perspectives on Irritable Bowel Syndrome and Illness Severity. Dig. Dis. Sci. 54, 1532–1541. doi:10.1007/s10620-009-0792-6
Drossman D. A. (2016). Functional Gastrointestinal Disorders: History, Pathophysiology, Clinical Features, and Rome IV. Gastroenterology 150, 1262–1279. doi:10.1053/J.GASTRO.2016.02.032
Edogawa S., Edwinson A. L., Peters S. A., Chikkamenahalli L. L., Sundt W., Graves S., et al. (2020). Serine Proteases as Luminal Mediators of Intestinal Barrier Dysfunction and Symptom Severity in IBS. Gut 69, 62–73. doi:10.1136/gutjnl-2018-317416
Eutamene H., Bradesi S., Larauche M., Theodorou V., Beaufrand C., Ohning G., et al. (2010). Guanylate Cyclase C-Mediated Antinociceptive Effects of Linaclotide in Rodent Models of Visceral Pain. Neurogastroenterol. Motil. 22, 312–e84. doi:10.1111/j.1365-2982.2009.01385.x
Fabisiak A., Sobocińska M., Kamysz E., Fichna J., Zielińska M. (2018). Antinociceptive Potency of Enkephalins and Enkephalinase Inhibitors in the Mouse Model of Colorectal Distension-Proof-Of-Concept. Chem. Biol. Drug Des. 92, 1387–1392. doi:10.1111/cbdd.13186
Farzaei M. H., Bahramsoltani R., Abdollahi M., Rahimi R. (2016). The Role of Visceral Hypersensitivity in Irritable Bowel Syndrome: Pharmacological Targets and Novel Treatments. J. Neurogastroenterol. Motil. 22, 558–574. doi:10.5056/jnm16001
Flacco M. E., Manzoli L., De Giorgio R., Gasbarrini A., Cicchetti A., Bravi F., et al. (2019). Costs of Irritable Bowel Syndrome in European Countries with Universal Healthcare Coverage: A Meta-Analysis. Eur. Rev. Med. Pharmacol. Sci. 23, 2986–3000. doi:10.26355/eurrev_201904_17580
Giardina S. F., Werner D. S., Pingle M., Bergstrom D. E., Arnold L. D., Barany F. (2018). A Novel, Nonpeptidic, Orally Active Bivalent Inhibitor of Human β-Tryptase. Pharmacology 102, 233–243. doi:10.1159/000492078
Gottesman-Katz L., Latorre R., Vanner S., Schmidt B. L., Bunnett N. W. (2021). Targeting G Protein-Coupled Receptors for the Treatment of Chronic Pain in the Digestive System. Gut 70, 970–981. doi:10.1136/gutjnl-2020-321193
Grant A. D., Cottrell G. S., Amadesi S., Trevisani M., Nicoletti P., Materazzi S., et al. (2007). Protease-Activated Receptor 2 Sensitizes the Transient Receptor Potential Vanilloid 4 Ion Channel to Cause Mechanical Hyperalgesia in Mice. J. Physiol. 578, 715–733. doi:10.1113/jphysiol.2006.121111
Gschossmann J. M., Liebregts T., Adam B., Buenger L., Ruwe M., Gerken G., et al. (2004). Long-Term Effects of Transient Chemically Induced Colitis on the Visceromotor Response to Mechanical Colorectal Distension. Dig. Dis. Sci. 49, 96–101. doi:10.1023/B:DDAS.0000011609.68882.3a
Hanning N., De bruyn M., Ceuleers H., Boogaerts T., Berg M., Smet A., et al. (2021). Local Colonic Administration of a Serine Protease Inhibitor Improves Post-Inflammatory Visceral Hypersensitivity in Rats. Pharmaceutics 13, 811. doi:10.3390/pharmaceutics13060811
Hughes P. A., Brierley S. M., Martin C. M., Brookes S. J. H., Linden D. R., Blackshaw L. A. (2009). Post-Inflammatory Colonic Afferent Sensitisation: Different Subtypes, Different Pathways and Different Time Courses. Gut 58, 1333–1341. doi:10.1136/gut.2008.170811
Joossens J., Ali O. M., El-Sayed I., Surpateanu G., Van der Veken P., Lambeir A.-M., et al. (2007). Small, Potent, and Selective Diaryl Phosphonate Inhibitors for Urokinase-Type Plasminogen Activator with In Vivo Antimetastatic Properties. J. Med. Chem. 50, 6638–6646. doi:10.1021/jm700962j
Kasperkiewicz P., Poreba M., Snipas S. J., Parker H., Winterbourn C. C., Salvesen G. S., et al. (2014). Design of Ultrasensitive Probes for Human Neutrophil Elastase through Hybrid Combinatorial Substrate Library Profiling. Proc. Natl. Acad. Sci. U.S.A. 111, 2518–2523. doi:10.1073/pnas.1318548111
Kawao N., Ikeda H., Kitano T., Kuroda R., Sekiguchi F., Kataoka K., et al. (2004). Modulation of Capsaicin-Evoked Visceral Pain and Referred Hyperalgesia by Protease-Activated Receptors 1 and 2. J. Pharmacol. Sci. 94, 277–285. doi:10.1254/jphs.94.277
Kehoe J. W., Kay B. K. (2005). Filamentous Phage Display in the New Millennium. Chem. Rev. 105, 4056–4072. doi:10.1021/cr000261r
Kugler E. M., Mazzuoli G., Demir I. E., Ceyhan G. O., Zeller F., Schemann M. (2012). Activity of Protease-Activated Receptors in Primary Cultured Human Myenteric Neurons. Front. Neurosci. 6, 1–8. doi:10.3389/fnins.2012.00133
Lapek J. D., Jiang Z., Wozniak J. M., Arutyunova E., Wang S. C., Lemieux M. J., et al. (2019). Quantitative Multiplex Substrate Profiling of Peptidases by Mass Spectrometry, Mol. Cell. Proteomics, 18. ZIP, 968–981. doi:10.1074/MCP.TIR118.001099/ATTACHMENT/FC3E83B2-FE0F-46D3-8599-4AC07943FBAC/MMC1
Liang W.-J., Zhang G., Luo H.-S., Liang L.-X., Huang D., Zhang F.-C. (2016). Tryptase and Protease-Activated Receptor 2 Expression Levels in Irritable Bowel Syndrome. Gut Liver 10, 382–390. doi:10.5009/gnl14319
Lichtenstein G. R., Deren J. J., Katz S., Lewis J. D., Kennedy A. R., Ware J. H. (2008). Bowman-Birk Inhibitor Concentrate: A Novel Therapeutic Agent for Patients with Active Ulcerative Colitis. Dig. Dis. Sci. 53, 175–180. doi:10.1007/s10620-007-9840-2
Lomax A. E., Pradhananga S., Sessenwein J. L., O’Malley D. (2019). Bacterial Modulation of Visceral Sensation: Mediators and Mechanisms. Am. J. Physiology-Gastrointestinal Liver Physiology 317, G363–G372. doi:10.1152/ajpgi.00052.2019
Martin L., Augé C., Boué J., Buresi M. C., Chapman K., Asfaha S., et al. (2009). Thrombin Receptor: An Endogenous Inhibitor of Inflammatory Pain, Activating Opioid Pathways. Pain 146, 121–129. doi:10.1016/j.pain.2009.07.016
Mayers M. D., Moon C., Stupp G. S., Su A. I., Wolan D. W. (2017). Quantitative Metaproteomics and Activity-Based Probe Enrichment Reveals Significant Alterations in Protein Expression from a Mouse Model of Inflammatory Bowel Disease. J. Proteome Res. 16, 1014–1026. doi:10.1021/acs.jproteome.6b00938
Meleine M., Boudieu L., Gelot A., Muller E., Lashermes A., Matricon J., et al. (2016). Comparative Effects of α2δ-1 Ligands in Mouse Models of Colonic Hypersensitivity. Wjg 22, 7111–7123. doi:10.3748/wjg.v22.i31.7111
Mizumura K., Sugiura T., Katanosaka K., Banik R. K., Kozaki Y. (2009). Excitation and Sensitization of Nociceptors by Bradykinin: What Do We Know? Exp. Brain Res. 196, 53–65. doi:10.1007/s00221-009-1814-5
Moayyedi P., Andrews C. N., MacQueen G., Korownyk C., Marsiglio M., Graff L., et al. (2019). Canadian Association of Gastroenterology Clinical Practice Guideline for the Management of Irritable Bowel Syndrome (IBS). J. Can. Assoc. Gastroenterol. 2, 6–29. doi:10.1093/jcag/gwy071
Mosinska P., Zielinska M., Fichna J. (2016). Expression and Physiology of Opioid Receptors in the Gastrointestinal Tract. Curr. Opin. Endocrinol. Diabetes Obes. 23:(1):3-10. doi:10.1097/MED.0000000000000219
Moussa L., Bézirard V., Salvador-Cartier C., Bacquié V., Houdeau E., Théodorou V. (2013). A New Soy Germ Fermented Ingredient Displays Estrogenic and Protease Inhibitor Activities Able to Prevent Irritable Bowel Syndrome-Like Symptoms in Stressed Female Rats. Clin. Nutr. 32, 51–58. doi:10.1016/j.clnu.2012.05.021
Mueller K., Michel K., Krueger D., Demir I. E., Ceyhan G. O., Zeller F., et al. (2011). Activity of Protease-Activated Receptors in the Human Submucous Plexus. Gastroenterology 141, 2088–2097. doi:10.1053/j.gastro.2011.08.034
Myers B., Greenwood-Van Meerveld B. (2012). Differential Involvement of Amygdala Corticosteroid Receptors in Visceral Hyperalgesia Following Acute or Repeated Stress. Am. J. Physiology-Gastrointestinal Liver Physiology 302, G260–G266. doi:10.1152/ajpgi.00353.2011
Noble F., Soleilhac J. M., Soroca-Lucas E., Turcaud S., Fournie-Zaluski M. C., Roques B. P. (1992a). Inhibition of the Enkephalin-Metabolizing Enzymes by the First Systemically Active Mixed Inhibitor Prodrug RB 101 Induces Potent Analgesic Responses in Mice and Rats. J. Pharmacol. Exp. Ther. 261, 181–190.
Noble F., Turcaud S., Fournié-Zaluski M.-C., Roques B. P. (1992b). Repeated Systemic Administration of the Mixed Inhibitor of Enkephalin-Degrading Enzymes, RB101, Does Not Induce Either Antinociceptive Tolerance or Cross-Tolerance with Morphine. Eur. J. Pharmacol. 223, 83–89. doi:10.1016/0014-2999(92)90821-k
Nozu T., Kumei S., Takakusaki K., Okumura T. (2014). Water-Avoidance Stress Enhances Gastric Contractions in Freely Moving Conscious Rats: Role of Peripheral CRF Receptors. J. Gastroenterol. 49, 799–805. doi:10.1007/s00535-013-0828-8
O' Mahony S. M., Coelho A.-M., Fitzgerald P., Lee K., Winchester W., Dinan T. G., et al. (2011). The Effects of Gabapentin in Two Animal Models of Co-Morbid Anxiety and Visceral Hypersensitivity. Eur. J. Pharmacol. 667, 169–174. doi:10.1016/j.ejphar.2011.05.055
O'Donoghue A. J., Eroy-Reveles A. A., Knudsen G. M., Ingram J., Zhou M., Statnekov J. B., et al. (2012). Global Identification of Peptidase Specificity by Multiplex Substrate Profiling. Nat. Methods 9, 1095–1100. doi:10.1038/nmeth.2182
Ovaere P., Lippens S., Vandenabeele P., Declercq W. (2009). The Emerging Roles of Serine Protease Cascades in the Epidermis. Trends Biochem. Sci. 34, 453–463. doi:10.1016/J.TIBS.2009.08.001
Owczarek D., Cibor D., Mach T., Cieśla A., Pierzchała-Koziec K., Sałapa K., et al. (2011). Met-Enkephalins in Patients with Inflammatory Bowel Diseases. Adv. Med. Sci. 56, 158–164. doi:10.2478/v10039-011-0051-x
Poreba M., Rut W., Vizovisek M., Groborz K., Kasperkiewicz P., Finlay D., et al. (2018). Selective Imaging of Cathepsin L in Breast Cancer by Fluorescent Activity-Based Probes. Chem. Sci. 9, 2113–2129. doi:10.1039/C7SC04303A
Puente X. S., Sánchez L. M., Overall C. M., López-Otín C. (2003). Human and Mouse Proteases: A Comparative Genomic Approach. Nat. Rev. Genet. 4, 544–558. doi:10.1038/nrg1111
Quinn T. M., Gaughan E. E., Bruce A., Antonelli J., O'Connor R., Li F., et al. (2022). Randomised Controlled Trial of Intravenous Nafamostat Mesylate in COVID Pneumonitis: Phase 1b/2a Experimental Study to Investigate Safety, Pharmacokinetics and Pharmacodynamics. eBioMedicine 76, 103856. doi:10.1016/j.ebiom.2022.103856
Rolland-Fourcade C., Denadai-Souza A., Cirillo C., Lopez C., Jaramillo J. O., Desormeaux C., et al. (2017). Epithelial Expression and Function of Trypsin-3 in Irritable Bowel Syndrome. Gut 66, 1767–1778. doi:10.1136/gutjnl-2016-312094
Ryan B., Musazzi L., Mallei A., Tardito D., Gruber S. H. M., El Khoury A., et al. (2009). Remodelling by Early-Life Stress of NMDA Receptor-Dependent Synaptic Plasticity in a Gene-Environment Rat Model of Depression. Int. J. Neuropsychopharm. 12, 553–559. doi:10.1017/S1461145708009607
Sawada K., Ohdo M., Ino T., Nakamura T., Numata T., Shibata H., et al. (2016). Safety and Tolerability of Nafamostat Mesilate and Heparin as Anticoagulants in Leukocytapheresis for Ulcerative Colitis: Post Hoc Analysis of a Large‐Scale, Prospective, Observational Study. Ther. Apher. Dial. 20, 197–204. doi:10.1111/1744-9987.12357
Solà-Tapias N., Vergnolle N., Denadai-Souza A., Barreau F. (2020). The Interplay Between Genetic Risk Factors and Proteolytic Dysregulation in the Pathophysiology of Inflammatory Bowel Disease. J. Crohns. Colitis 14, 1149–1161. doi:10.1093/ecco-jcc/jjaa033
Sostegni S., Diakov A., McIntyre P., Bunnett N., Korbmacher C., Haerteis S. (2015). Sensitisation of TRPV4 by PAR2 Is Independent of Intracellular Calcium Signalling and Can Be Mediated by the Biased Agonist Neutrophil Elastase. Pflugers Arch. - Eur. J. Physiol. 467, 687–701. doi:10.1007/s00424-014-1539-6
Sperber A. D., Bangdiwala S. I., Drossman D. A., Ghoshal U. C., Simren M., Tack J., et al. (2021). Worldwide Prevalence and Burden of Functional Gastrointestinal Disorders, Results of Rome Foundation Global Study. Gastroenterology 160, 99–114. e3. doi:10.1053/J.GASTRO.2020.04.014
Stadnicki A. (2011). Intestinal Tissue Kallikrein-Kinin System in Inflammatory Bowel Disease. Inflamm. Bowel Dis. 17, 645–654. doi:10.1002/ibd.21337
Steck N., Mueller K., Schemann M., Haller D. (2012). Bacterial Proteases in IBD and IBS. Gut 61, 1610–1618. doi:10.1136/gutjnl-2011-300775
Tominaga K., Fujikawa Y., Tanaka F., Kamata N., Yamagami H., Tanigawa T., et al. (2016). Structural Changes in Gastric Glial Cells and Delayed Gastric Emptying as Responses to Early Life Stress and Acute Adulthood Stress in Rats. Life Sci. 148, 254–259. doi:10.1016/j.lfs.2016.02.025
Tooth D., Garsed K., Singh G., Marciani L., Lam C., Fordham I., et al. (2014). Characterisation of Faecal Protease Activity in Irritable Bowel Syndrome with Diarrhoea: Origin and Effect of Gut Transit. Gut 63, 753–760. doi:10.1136/gutjnl-2012-304042
Tremaine W. J., Brzezinski A., Katz J. A., Wolf D. C., Fleming T. J., Mordenti J., et al. (2002). Treatment of Mildly to Moderately Active Ulcerative Colitis with a Tryptase Inhibitor (APC 2059): An Open-Label Pilot Study. Aliment. Pharmacol. Ther. 16, 407–413. doi:10.1046/j.1365-2036.2002.01194.x
Turk B. (2006). Targeting Proteases: Successes, Failures and Future Prospects. Nat. Rev. Drug Discov. 5, 785–799. doi:10.1038/nrd2092
van den Wijngaard R. M., Stanisor O. I., van Diest S. A., Welting O., Wouters M. M., de Jonge W. J., et al. (2012). Peripheral α-helical CRF (9-41) Does Not Reverse Stress-Induced Mast Cell Dependent Visceral Hypersensitivity in Maternally Separated Rats. Neurogastroenterol. Motil. Off. J. Eur. Gastrointest. Motil. Soc. 24, 274–e111. doi:10.1111/j.1365-2982.2011.01840.x
Van Spaendonk H., Ceuleers H., Witters L., Patteet E., Joossens J., Augustyns K., et al. (2017). Regulation of Intestinal Permeability: The Role of Proteases. Wjg 23, 2106–2123. doi:10.3748/wjg.v23.i12.2106
Vergnolle N. (2005). Clinical Relevance of Proteinase Activated Receptors (Pars) in the Gut. Gut 54, 867–874. doi:10.1136/gut.2004.048876
Vergnolle N. (2016). Protease Inhibition as New Therapeutic Strategy for GI Diseases. Gut 65, 1215–1224. doi:10.1136/gutjnl-2015-309147
Vermeulen W., De Man J. G., Pelckmans P. A., De Winter B. Y. (2014). Neuroanatomy of Lower Gastrointestinal Pain Disorders. World J. Gastroenterol. 20, http://www.wjgnet.com/20, 1005–1020. doi:10.3748/WJG.V20.I4.1005
Winston J. H., Sarna S. K. (2013). Developmental Origins of Functional Dyspepsia-Like Gastric Hypersensitivity in Rats. Gastroenterology 144, 570–579. doi:10.1053/J.GASTRO.2012.11.001
Wood S. E., Sinsinbar G., Gudlur S., Nallani M., Huang C.-F., Liedberg B., et al. (2017). A Bottom-Up Proteomic Approach to Identify Substrate Specificity of Outer-Membrane Protease OmpT. Angew. Chem. Int. Ed. 56, 16531–16535. doi:10.1002/anie.201707535
Wouters M. M., Balemans D., Van Wanrooy S., Dooley J., Cibert-Goton V., Alpizar Y. A., et al. (2016). Histamine Receptor H1-Mediated Sensitization of TRPV1 Mediates Visceral Hypersensitivity and Symptoms in Patients with Irritable Bowel Syndrome. Gastroenterology 150, 875–887. doi:10.1053/j.gastro.2015.12.034
Zhao J.-h., Dong L., Shi H.-t., Wang Z.-y., Shi H.-y., Ding H. (2012). The Expression of Protease-Activated Receptor 2 and 4 in the Colon of Irritable Bowel Syndrome Patients. Dig. Dis. Sci. 57, 58–64. doi:10.1007/s10620-011-1827-3
Zhao J., Wang J., Dong L., Shi H., Wang Z., Ding H., et al. (2011). A Protease Inhibitor Against Acute Stress-Induced Visceral Hypersensitivity and Paracellular Permeability in Rats. Eur. J. Pharmacol. 654, 289–294. doi:10.1016/j.ejphar.2010.12.032
Zhao P., Lieu T., Barlow N., Metcalf M., Veldhuis N. A., Jensen D. D., et al. (2014a). Cathepsin S Causes Inflammatory Pain via Biased Agonism of PAR2 and TRPV4. J. Biol. Chem. 289, 27215–27234. doi:10.1074/jbc.M114.599712
Zhao P., Lieu T., Barlow N., Sostegni S., Haerteis S., Korbmacher C., et al. (2015). Neutrophil Elastase Activates Protease-Activated Receptor-2 (PAR2) and Transient Receptor Potential Vanilloid 4 (TRPV4) to Cause Inflammation and Pain. J. Biol. Chem. 290, 13875–13887. doi:10.1074/jbc.M115.642736
Zhao P., Metcalf M., Bunnett N. W. (2014b). Biased Signaling of Protease-Activated Receptors. Front. Endocrinol. 5, 1–16. doi:10.3389/fendo.2014.00067
Keywords: irritable bowel syndrome, visceral hypersensitivity, proteases, protease-activated receptors, protease inhibitors
Citation: Decraecker L, Boeckxstaens G and Denadai-Souza A (2022) Inhibition of Serine Proteases as a Novel Therapeutic Strategy for Abdominal Pain in IBS. Front. Physiol. 13:880422. doi: 10.3389/fphys.2022.880422
Received: 21 February 2022; Accepted: 26 April 2022;
Published: 19 May 2022.
Edited by:
Elizabeth S. Fernandes, Pelé Pequeno Príncipe Research Institute, BrazilReviewed by:
AisahAniisahAubdool, Queen Mary University of London, United KingdomDaniele Maria-Ferreira, Pelé Pequeno Príncipe Research Institute, Brazil
Copyright © 2022 Decraecker, Boeckxstaens and Denadai-Souza. This is an open-access article distributed under the terms of the Creative Commons Attribution License (CC BY). The use, distribution or reproduction in other forums is permitted, provided the original author(s) and the copyright owner(s) are credited and that the original publication in this journal is cited, in accordance with accepted academic practice. No use, distribution or reproduction is permitted which does not comply with these terms.
*Correspondence: Alexandre Denadai-Souza, alexandre.denadai-souza@kuleuven.be