- 1Klinik und Poliklinik für Kardiologie, Universitätsklinikum Leipzig, Leipzig, Germany
- 2Department of Neurology, Universitätsklinikum Würzburg, Würzburg, Germany
- 3Department of Neurology and Center for Stroke Research Berlin, Charité—Universitätsmedizin Berlin, Berlin, Germany
- 4German Center for Neurodegenerative Diseases (DZNE) and German Centre for Cardiovascular Research (DZHK), Berlin, Germany
- 5Institute of Laboratory Medicine, Clinical Chemistry and Molecular Diagnostics, University of Leipzig, Leipzig, Germany
- 6Department for Internal Medicine III, Cardiology, Angiology and Intensive Care Medicine, Saarland University, Saarbrücken, Germany
- 7Rudolf-Boehm-Institut für Pharmakologie und Toxikologie, Medizinische Fakultät, Universität Leipzig, Leipzig, Germany
Objectives: The intracellular NLRP3 inflammasome is an important regulator of sterile inflammation. Recent data suggest that inflammasome particles can be released into circulation. The effects of exercise on circulating extracellular apoptosis-associated speck-like protein (ASC) particles and their effects on endothelial cells are not known.
Methods: We established a flow cytometric method to quantitate extracellular ASC specks in human serum. ASC specks were quantitated in 52 marathon runners 24–72 h before, immediately after, and again 24–58 h after the run. For mechanistic characterization, NLRP3 inflammasome particles were isolated from a stable mutant NLRP3 (p.D303N)-YFP HEK cell line and used to treat primary human coronary artery endothelial cells.
Results: Athletes showed a significant increase in serum concentration of circulating ASC specks immediately after the marathon (+52% compared with the baseline, p < 0.05) and a decrease during the follow-up after 24–58 h (12% reduction compared with immediately after the run, p < 0.01). Confocal microscopy revealed that human endothelial cells can internalize extracellular NLRP3 inflammasome particles. After internalization, endothelial cells showed an inflammatory response with a higher expression of the cell adhesion molecule ICAM1 (6.9-fold, p < 0.05) and increased adhesion of monocytes (1.5-fold, p < 0.05).
Conclusion: These findings identify extracellular inflammasome particles as novel systemic mediators of cell–cell communication that are transiently increased after acute extensive exercise with a high mechanical muscular load.
Introduction
Vascular diseases are the leading cause of mortality and disability worldwide (Roth et al., 2020). In addition to the classic risk factors such as dyslipidemia, diabetes mellitus, and arterial hypertension, vascular inflammation is recognized as a driver of endothelial dysfunction and target of therapy (Daiber et al., 2017). Clinical studies have provided promising data on risk reduction using anti-inflammatory strategies (Ridker et al., 2017; Tardif et al., 2019; Nidorf et al., 2020).
An important cytokine for the inflammatory pathogenesis of cardiovascular diseases is interleukin-1 (IL-1) (Devlin et al., 2002; Bhaskar et al., 2011; van Tassell et al., 2013). IL-1 is processed by caspase-1 after the formation and activation of the NLR family pyrin domain containing 3 (NLRP3) inflammasome complex (Geng and Libby, 1995; Martinon et al., 2002). The intracellular NLRP3 inflammasome has been identified as an important component of the pathology of several inflammatory diseases (Rheinheimer et al., 2017; Zhao et al., 2020). These diseases range from directly inflammasome-associated diseases such as the rare cryopyrin-associated periodic syndrome, the Muckle–Wells syndrome, or the familial cold autoinflammatory syndrome to metabolic disorders with high prevalence such as type 2 diabetes, atherosclerosis, obesity, and gout (Hoffman et al., 2001; Frenkel et al., 2004; Sun et al., 2017). Upon activation, NLRP3 nucleates with apoptosis-associated speck-like protein (ASC) to form an ASC speck, leading to caspase-1-mediated proteolytic activation of interleukin-1β (IL-1β) and interleukin-18 (IL-18), and the induction of inflammatory, pyroptotic cell death. Recent evidence shows that pyroptotic cells can subsequently release ASC specks into the extracellular space where they may perpetuate inflammation (Gaul et al., 2021). Extracellular ASC specks have been described in chronic obstructive pulmonary disease, septic patients, and myelodysplastic syndrome (Franklin et al., 2014; Basiorka et al., 2018; Martínez-García et al., 2019). However, the regulation and importance of circulating ASC specks are incompletely understood.
In cardiovascular diseases, the NLRP3 inflammasome is associated with endothelial dysfunction, the progression of atherosclerosis, and damage caused after reperfusion of ischemic myocardial tissue (Paramel Varghese et al., 2016; Gong et al., 2018). In the vascular wall, the NLRP3 inflammasome can be activated by pathogens such as cholesterol crystals, hypoxia, and hypercholesterolemia (Janoudi et al., 2016; Koka et al., 2017; Jiang et al., 2020). Interestingly, a genetic variant of NLRP3 with an intrinsic higher activity is associated with a higher prevalence of coronary artery disease (Schunk et al., 2021). The effects of other stressors, such as acute exercise with a high mechanical muscular load, on the inflammasome are not known.
Low physical activity and a sedentary lifestyle are risk factors for cardiovascular morbidity and mortality (Gibbs et al., 2015). The positive effects of regular physical activity on the cardiovascular system have been very well documented and are recommended as the basis of vascular prevention (Kyu et al., 2016; Hussain et al., 2018; Pelliccia et al., 2021). In contrast to regular moderate to high-intensity exercise, excessive bouts of physical activities such as marathon running have been associated with an acute upregulation of the parameters of inflammation and oxidative stress (Möhlenkamp et al., 2008; Breuckmann et al., 2009; Armstrong et al., 2015; Lear et al., 2017). Based on these observations, the “extreme exercise hypothesis” was proposed, which states that adverse cardiovascular events may occur more often following high-volume high-intensity exercise (Eijsvogels et al., 2018). The mediators for these potentially negative effects are incompletely understood.
Therefore, the aim of this study was to establish a method to quantitate ASC specks in human blood and to explore the effects of marathon running on circulating ASC specks as mediators of systemic inflammation and inflammasome activation. We hypothesized that inflammasome particles may be taken up by endothelial cells where they may induce a pro-inflammatory phenotype.
Methods
Flow Cytometric Analysis of Apoptosis-Associated Speck-Like Protein Specks in Human Serum
A total of 75 µl of human serum was diluted in 225 µl phosphate-buffered saline (PBS) and centrifuged at 17,000x g for 20 min at 4°C to isolate microparticles. After centrifugation, the supernatant was discarded and the pellet was resuspended in 300 µl PBS. The samples were then stained using ASC-B3-Alexa-647 (sc-514414, Santa Cruz) for 1 h at 37°C. Afterward, 120 µl of the samples was measured using a flow cytometer BDFacsLyric (BDscience). ASC-GFP specks derived from THP1-ASC-GFP cells were labelled with an ASC-B3-Alexa-647 antibody and added to human serum to serve as a positive control for gating the samples. These were detected in the 488-nm channel of the flow cytometer (Figure 1A) and the identified population was then marked accordingly in the 640-nm channel (Figure 1B). The resulting gate was used to identify extracellular ASC specks in the serum of the human samples (Figures 1C, D). Results were then calculated as ASC specks/µl by dividing the amount of ASC specks in the gate by the volume of the probe measured accounting for dilution during the centrifugation steps. The validation of the FACS measurements was based on an established cell line—THP1-ASC-GFP (Invivogen)—for the isolation of ASC specks (Proske and Morgan, 2001). The measured particles in human serum resemble the isolated specks in granularity, size, and fluorescence intensity. Intra- and interassays on the serum of the volunteers without prior exercise yielded coefficients of variation of 21.5 and 17.2%, respectively (Figures 1E,F).
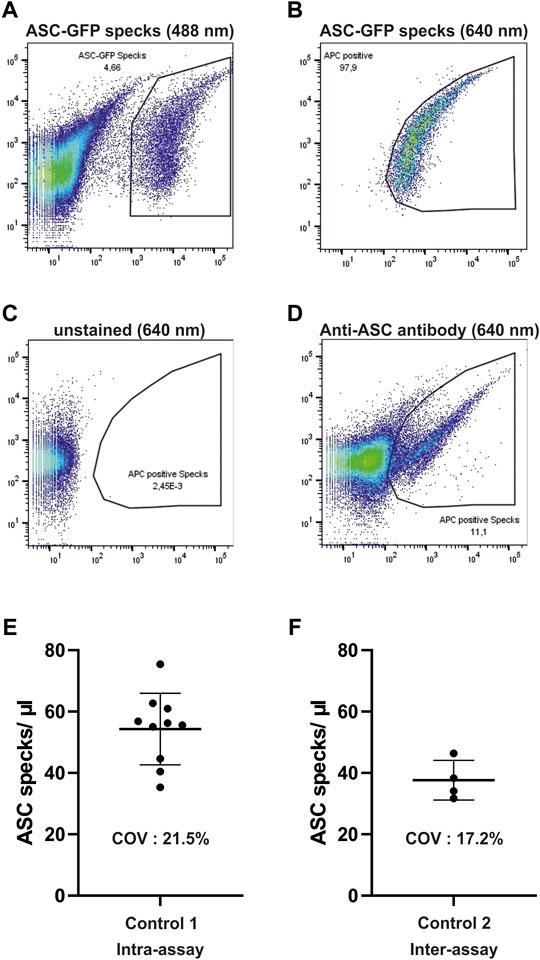
FIGURE 1. Gating strategy and validation of flow cytometric measurements of ASC specks. (A,B) Depicted are dot plots of isolated ASC-GFP particles which are labeled with ASC-B3-Alexa-647 antibodies in human serum in the 488 and 640-nm channels. (C,D) Resulting gate in the Alexa-647 channel is then used for the detection of ASC-specks in human serum after staining with AS-B3-Alexa-647 antibodies. (E,F) One control subject without prior exercise was measured ten times (intra-assay) and another one on four different days (inter-assay) to test for accuracy of measurements. COV, coefficient of variation.
Human Samples
Blood samples were collected from participants of the Berlin Beat of Running study, a prospective, observational, and investigator-initiated study conducted during the 38th Berlin marathon (clinicaltrials.gov, NCT01428778) (Haeusler et al., 2012). Inclusion criteria were completed for registration for the marathon run: age 35–60 years, a marathon history of at least two marathon runs within the last five years and self-reported running for at least 40 km per week. Exclusion criteria included known cardiac diseases or atrial fibrillation, known or newly detected brain disease, contraindications for (brain) magnetic resonance imaging, severe liver or kidney disease, hyperthyroidism, pregnancy, or lactation. The study protocol is in accordance with the Declaration of Helsinki and was approved by the local Ethics Committee of the Charité-Universitätsmedizin Berlin, Germany (EA4/042/ 11). All participants gave their informed consent before their inclusion in the study.
The baseline visit (t0) took place three days before the marathon. Sociodemographics, cardiovascular risk factors, and the level of physical activity were documented. Participants underwent an assessment of vital parameters (heart rate and blood pressure) and venous blood sampling (Sarstedt Monovette). Within 30 min after the end of the marathon run (t1), vital parameters were assessed and the second blood sampling was done. The third blood sampling with the aforementioned parameters and assessment of vital parameters was done up to 58 h after the race (t2) (Haeusler et al., 2012; Herm et al., 2017).
Cell Culture
Primary human coronary artery endothelial cells (HCAEC) were purchased from Promocell (C-12221) and maintained in EGM™ Endothelial Cell Growth Media (CC-3121) and Single Quots™ Supplements (CC-4133). Human umbilical vein cells (EA.hy926) were purchased from ATCC (CRL-2922) and maintained in Dulbecco’s Modified Eagle Medium (DMEM) high glucose (Gibco). Mutant NLRP3-YFP p.D303N human embryonic kidney 293 (HEK) cells were used to isolate overactive YFP-labelled NLRP3-inflammasome oligomers as previously described (Martín-Sánchez et al., 2015). THP1-ASC-GFP cells were maintained in RPMI1640. This cell line was cultured in Dulbecco’s Modified Eagle Medium F-12 (DMEM/F-12) growth media in the presence of Geneticin G418 (2 mg/ml) to maintain mutation (Baroja-Mazo et al., 2014). Internalization of NLRP3-YFP particles in HCAEC was determined by immunofluorescence staining with anti-YFP and anti-rabbit Alexa-488 antibodies. Alexa Fluor™ 555 Phalloidin and DAPI were used for F-actin and nucleus staining, respectively.
Isolation of Inflammasome Particles
Fluorescent p.D303N NLRP3-YFP inflammasome oligomers were isolated and purified from 1 × 107 mutant NLRP3-YFP (p.D303N) HEK cells and resuspended in 100 µl non-denaturating CHAPS buffer following an established protocol (Martín-Sánchez et al., 2015). Fluorescent ASC-GFP specks were isolated and purified from THP1-ASC-GFP cells (Invivogen) and resuspended in 100 µl non-denaturating CHAPS buffer following the same protocol with the addition of a primer with LPS at 1 µg/ ml for 3 h and activation by Nigericin at 5 µM for 1 h before isolation.
Cellular Imaging
HCAEC were treated with NLRP3-YFP particles for 24 h. Cells were fixed with 4% paraformaldehyde, permeabilized and incubated with an anti-YFP (GFP) antibody (#6556, Abcam), anti-YFP Alexa555 antibody (A-31851, Invitrogen), anti-ICAM-1 (#MA5407, Invitrogen), IgG isotype control (#RIgG, #MigG, Dianova), goat anti-mouse Cy3 (#115-165-146, Dianova), donkey anti-rabbit Alexa-488 (A-21206, Invitrogen), or goat anti-rabbit Alexa-647 secondary antibody (A-21245, Invitrogen) to analyze the internalization of extracellular NLRP3-YFP oligomers or ICAM-1 expression. DAPI, Hoechst, and F-actin Phalloidin Alexa 555/ Alexa647 were used for nucleus and F-actin staining, respectively (Gaul et al., 2021). Cells were visualized on a ZEISS Axiovert 200 M fluorescent microscope. Z-stacking (12 subsequently optical slices of 0.8 µm thickness) and 3D reconstruction were performed with AxioVision LE Rel 4.4 software. A minimum of five randomly selected fields were used. To analyze ICAM-1 expression, the evaluation of 8–10 immunofluorescence images per condition and experiment was performed by ImageJR software. First, a gray scale image was used to set a threshold from which the ICAM1 signal was considered positive. This value was applied to all images. Then, the product of the mean intensity and the area of the ICAM1 signal (integrated density) were determined. This determined value was normalized to the cell number, which was counted on the basis of the intact cell nuclei. An ImageStreamX Mk II Imaging Flow Cytometer was also used for internalization studies.
Steroid Hormone Analysis
Steroid hormones in serum, which include cortisol, cortisone, testosterone, androstenedione, 17-hydroxyprogesterone (17-OHP), dehydroepiandrosterone sulfate (DHEAS), progesterone, and estradiol, were simultaneously quantified using liquid chromatography-tandem mass spectrometry (LC–MS/MS) as described (Bae et al., 2019).
Monocyte Adhesion
Monocyte adhesion was assessed by calcein red-orange staining (7.5 µM) of THP1 monocytes and fluorometric analysis (Ex571/Em596) of monocytes on HCAEC. For this purpose, HCAEC were treated with extracellular NLRP3-YFP particles for 24 h and then incubated with stained THP1 monocytes for 4 h. Adherent cells were analyzed fluorometrically and normalized to untreated cells.
Data Analysis
Analyses were performed with the Graph Pad Prism (version 7), ImageJ 1.52a, and FlowJo v10. The significance level was set at p < 0.05. Multiple groups were analyzed using a mixed-effect analysis and the Tukey post-hoc test. Two groups were analyzed by a two-sided Student´s t-test. Data are expressed as mean ± standard error of the mean (SEM) if not stated otherwise. A correlation analysis was performed using JASP 0.14.1 (JASP Team, 2020). CorelDraw2018 was used to create the artwork. Statistical outliers were identified using the ROUT method with Q = 1%.
Results
Marathon Running Increases Extracellular Apoptosis-Associated Speck-Like Protein Specks in Human Serum
Baseline characteristics are shown in Table 1. Performing a marathon run resulted in a significant 1.5-fold increase in circulating ASC specks immediately after the run compared with the baseline assessment (p < 0.05). The absolute number of ASC specks increased from 11 ± 7 ASC specks/µl to 15 ± 9 ASC specks/µl serum. After the marathon, the concentration decreased toward baseline levels to 11 ± 6 ASC specks/µl (p < 0.01) (Figure 2A).
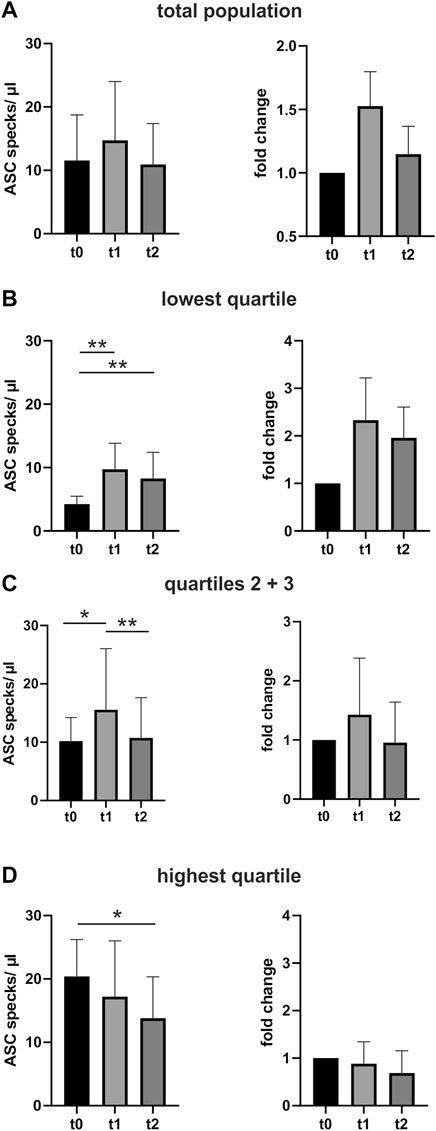
FIGURE 2. Extracellular ASC speck concentration increases after performing a marathon run and decreases again in the days after. (A) Extracellular ASC specks were measured using flow cytometry. Samples were collected 24–48 h before (t0) (N = 52), immediately after (t1) (N = 52), and 2–3 days after the marathon run (t2) (N = 52). Absolute values of ASC specks per microliter serum and the individual fold change to t0 are depicted. (B–D) Subgroup analysis of the quartile with the lowest ASC speck concentration at t0 (B), the middle group (C), and the quartile with the highest (D) ASC speck concentration at t0. Data are expressed as mean +standard deviation *p < 0.05; **p < 0.01. Mixed-effects analysis and the post-hoc Tukey test were used to analyze the data.
An exploratory analysis of quartiles of baseline ASC speck concentrations at time t0 revealed that the exercise-induced regulation correlates with the baseline levels. Athletes with low baseline ASC speck levels showed a marked upregulation at t1 that persisted until t2. The middle group of athletes had a distinct increase in circulating ASC specks after the run that recovered until t2. In contrast, individuals with baseline ASC specks in the highest quartile showed no additional upregulation after the marathon. (Figures 2B–D).
Upregulation of Extracellular Apoptosis-Associated Speck-Like Protein Specks Correlates With Stress Hormones but Not With Microparticles
Marathon running induces the upregulation of stress hormones and cellular microparticles (Bae et al., 2019; Schwarz et al., 2018). The ASC specks showed a significant positive correlation with stress hormones such as cortisone (R = 0.22), cortisol (R = 0.19), aldosterone (R = 0.24), and progesterone (R = 0.24) (Figures 3A,B) (Bae et al., 2019).
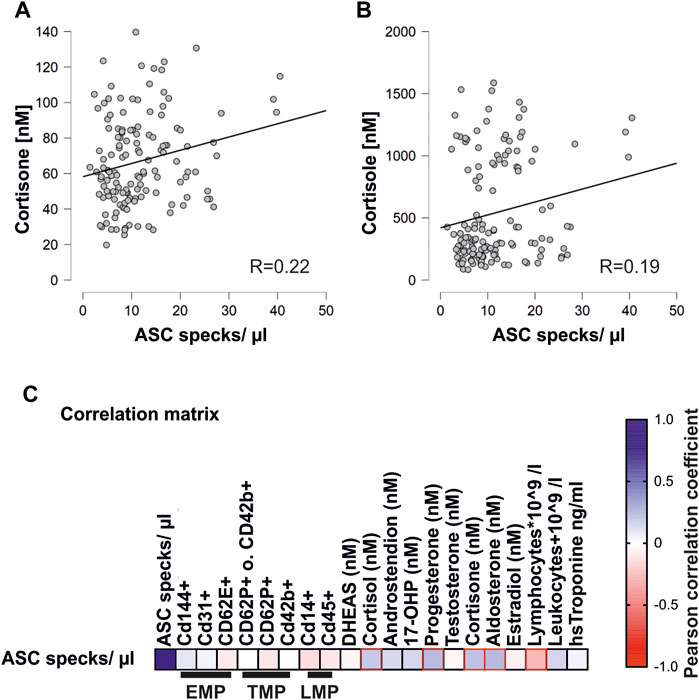
FIGURE 3. Concentrations of circulating ASC specks correlate with steroid hormones and lymphocytes (A) Pearson correlation matrix of extracellular ASC specks with various endothelial (EMP), platelet (TMP), and leukocyte (LMP) microparticles and steroid hormones, lymphocytes, leukocytes, and high-sensitivity troponin. Significant correlations (p < 0.05) are marked in red. (B,1C) Exemplary scatter plots and linear regression of correlations of extracellular ASC specks with cortisone and cortisol. The Pearson correlation coefficient was used to analyze the data.
In addition, there was a significant negative correlation with the number of circulating lymphocytes that are known to decrease after severe endurance exercise (R = 0.27) (Santos et al., 2013).
In contrast to the stress hormones, there was no correlation of the concentration of extracellular ASC specks with circulating microparticles. These included endothelial, platelet, and leukocyte microparticles (Figure 3C) (Schwarz et al., 2018). The lack of correlation between the ASC specks and the different types of microparticles is an indicator of the specificity of the circulating ASC particles as released by inflammasome activation rather than more general cell damage.
Human Coronary Endothelial Cells Internalize Extracellular NLRP3 Inflammasome Particles
To test whether extracellular NLRP3-YFP inflammasome particles are taken up by human endothelial cells, immunofluorescent staining of internalized YFP-tagged NLRP3 inflammasomes and cell organelle staining were performed (Figure 4A). Internalization of extracellular NLRP3-YFP inflammasome particles into HCAEC was analyzed by nucleus (DAPI) and cytoskeleton (F-Actin Phalloidin) staining and the detection of intracellular YFP-positive signal. The intracellular NLRP3-YFP particles are located in the region around the nucleus (Figures 4B–D).
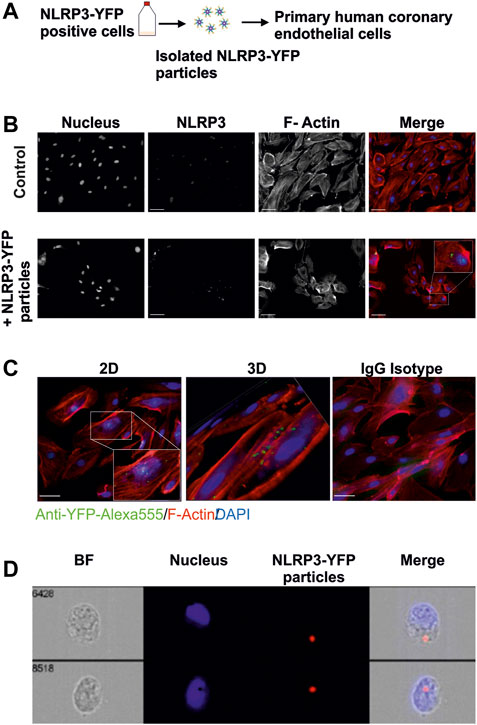
FIGURE 4. Extracellular NLRP3-YFP particles are internalized by primary human coronary artery endothelial cells. (A) Schematic overview. Mutant NLRP3 (p.D303N)-YFP HEK cells are used to isolate oligomeric NLRP3-YFP inflammasome particles and treat primary human coronary artery endothelial cells. (B) Internalization of extracellular NLRP3-YFP particles in primary human coronary artery endothelial cells (HCAEC) after 4 h of incubation and determined by immunofluorescent staining (N = 3) with a primary fluorescently labeled anti-YFP-Alexa555 antibody (green) (scale bar: 50 µm). Alexa Fluor™ 647 Phalloidin (red) and DAPI (blue) were used for F-actin and nucleus staining, respectively. Z-stacks with xz and yz focal planes showing internalized NLRP3-YFP inflammasome particle. (C) Z-stacks were used for 3D reconstruction (scale bar: 50 µm). (D) Two representative ImageStream® analyses of HCAEC including bright field (BF), DAPI (Nucleus), 647/Cy5 Channel (Ex. NLRP3), and a merge of an internalized extracellular NLRP3-YFP inflammasome are shown (scale bar: 10 µm).
Extracellular NLRP3-YFP Particles Induce a Pro-Inflammatory Response in Human Coronary Endothelial Cells.
Treatment of HCAEC with NLRP3-YFP particles (3 NLRP3-YFP particles/cell for 24 h) increased the expression of the cell adhesion molecule ICAM-1 measured by immunofluorescent staining by 6.9-fold (p < 0.05) (Figures 5A,B).
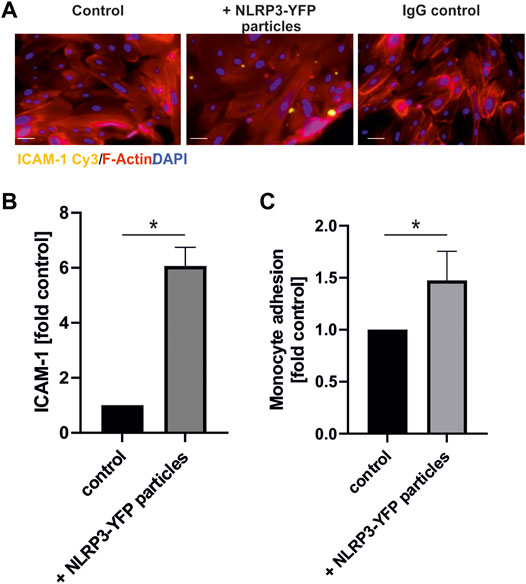
FIGURE 5. Treatment with extracellular NLRP3 inflammasome particles induces inflammation in endothelial cells. (A) Immunofluorescent analysis of ICAM-1 protein expression on coronary artery endothelial cells after 24 h incubation with NLRP3-YFP particles (3 NLRP3-YFP particles/cell) and stained with anti-ICAM1 Cy3 (scale bar: 50 µm). (B) Quantitative analysis of ICAM-1 fluorescence (N = 4). (C) Calcein-stained THP-1 monocyte adhesion on endothelial cells after incubation with NLRP3-YFP particles for 4 h measured by a fluorometric analysis and normalized to untreated cells in the culture medium (control). Two-sided unpaired t-test was used to analyze the data.
Additionally, monocyte adhesion on endothelial cells was increased by the extracellular NLRP3-YFP particles by 1.4-fold (p < 0.05) (Figure 5C).
Discussion
The main findings of the study are the increase of circulating extracellular ASC specks in human serum immediately after a marathon run, the uptake of extracellular inflammasome particles by endothelial cells, and the induction of endothelial cell inflammation. Marathon running induces a marked but transient increase of circulating ASC specks. Mechanistically, the data reveal that cell-free extracellular inflammasome particles can be internalized by human endothelial cells and promote an inflammatory response characterized by the upregulation of ICAM-1 and increased monocyte adhesion. The data show that extracellular ASC specks represent markers of vascular inflammation.
Extracellular Apoptosis-Associated Speck-Like Protein Specks After a Marathon
Extracellular inflammasome particles are generated and released when cells undergo pyroptosis. The number of these particles in the blood reflects pyroptotic cell damage. The upregulation of the ASC specks showed an inverse correlation with baseline levels, the lower the baseline, the higher the marathon-induced upregulation. These data suggest a maximum saturation of the exercise-induced number of circulating inflammasome particles. Further studies are needed to address whether lower baseline ASC speck levels relate to individual predisposition or the amount of exercise performed by different athletes in the days before the marathon run. The variance of baseline ASC speck levels may be attributed to different lifestyles, pre-existing conditions or nutrition, and the microbiome in the gut (Youm et al., 2015; Ding et al., 2019). The number of ASC specks in the serum does not correlate with endothelial, platelet, and leukocyte microparticles as a correlate of cell damage. This observation supports the importance of ASC specks as an independent factor. The mechanical damage of myocytes induces the release of typical inflammasome activators such as extracellular ATP or reactive oxygen species which then results in a cascade of inflammasome activation not only in the surrounding muscles but also vascular cells (Bailey et al., 2004; Bauernfeind et al., 2011; Heid et al., 2013; Panicucci et al., 2020). The cell damage of myocytes is documented by the release of typical proteins, miRNAs, and the clinical presentation of muscle soreness after excessive bouts of endurance exercise (Uhlemann et al., 2014; Bernat-Adell et al., 2021). Therefore, the increased ASC specks after marathon running may originate from skeletal muscle cells that are subjected to high physical strain (Pincheira et al., 2021).
An increase of the circulation of ASC in plasma of cyclists post-exercise using Western blot has already been shown; we see similar results in marathon runners and also a similar variance in our baseline values (Nieman et al., 2020). It is also known that exercise results in an increased expression of NLRP3 indicating that it is the relevant sensor protein to these ASC oligomers (Li et al., 2015; Khakroo Abkenar et al., 2019).
The increase in the circulation of ASC particles after the marathon is in agreement with the observation of upregulation of circulating ASC in the plasma of cyclists (Nieman et al., 2020) and an increased expression of NLRP3 post-exercise (Li et al., 2015; Khakroo Abkenar et al., 2019). We used the flow cytometry method to measure extracellular ASC specks. Compared with Western blot (Nieman et al., 2020), flow cytometry has the advantage that the particles are defined more precisely by spiking experiments and that flow cytometry allows a high turnover with highly standardized, parallel, and quantitative measurements.
Effect of Extracellular Inflammasome Particles on Cardiovascular Cells
The study shows for the first time that endothelial cells internalize inflammasome particles leading to inflammation exemplified by the increase of ICAM-1 expression and the increase of monocyte adhesion. These data identify extracellular inflammasome particles as a mechanism that mediates inflammation from the site of damaged tissue to the endothelium. Previous findings of our group demonstrated that extracellular NLRP3 particles are also ingested by human coronary artery smooth muscle cells and macrophages where they induce pro-inflammatory and atherogenic signaling by increasing the extracellular matrix production, promoting the secretion of pro-atherogenic and inflammatory cytokines and cell migration (Gaul et al., 2021).
Extensive exercise with a high mechanical muscular load can mediate systemic inflammation via the release of extracellular inflammasome complexes. We and others propose that these pyroptosis-derived NLRP3 inflammasome particles exert pro-atherogenic effects (Gaul et al., 2021; Sun et al., 2021).
Inflammation and Extreme Exercise Hypothesis
According to the “extreme exercise hypothesis,” long-term excessive training is associated with an increase in the overall mortality (Eijsvogels et al., 2018). Marathon runners show a higher prevalence of coronary artery calcification scores ≥100 Agatston units compared with the general population (36 vs. 22%) indicating coronary artery atherosclerosis (Möhlenkamp et al., 2008). Champion athletes have more coronary calcifications compared with sedentary men (44.3 vs 22.2%) (Merghani et al., 2017). One critical factor for cardiovascular risk is endothelial cell physiology (Souilhol et al., 2018). Therefore, the internalization of circulating pro-inflammatory inflammasome particles into endothelial cells and smooth muscle cells is likely relevant in the progression of cardiovascular diseases.
Interestingly, athletes have more stable calcified plaque phenotypes than controls and fewer mixed plaques (Merghani et al., 2017). This could indicate different underlying pathomechanisms of atherosclerosis in athletes and the general population. The role of inflammation and immune cells in the development of various plaque types is incompletely understood. Circulating inflammasome particles may be an important component in this process by increasing monocyte adhesion to endothelial cells (Waring et al., 2021).
Limitations
There are limitations to our study. Our study population included only non-professional athletes who completed high-level endurance exercises, so transferring the data to other intensities or forms, such as strength exercise, is not possible. Nevertheless, marathon runs at competitive levels are typical for extremely intensive endurance exercise and are practiced worldwide by an increasing number of individuals. The studied population is large for a marathon study but addresses a selected and homogenous population. Therefore, the results may not apply to the general population. Measurements were performed in human serum. Other tissues are not available. Because the serum was stored for several years, the proteins may have been degraded (Cuhadar et al., 2013). Since we are only measuring one relevant protein and this should be changed in the same way in all samples, this should not change the dynamic of the ASC specks. This is also the reason why cytokines could not be measured in this study, since cytokines are extremely dependent on the storage time and conditions (Jager et al., 2009).
Conclusion
In conclusion, the data show the release of inflammasome particles in response to extensive exercise with high mechanical muscular load, endurance exercise, and their internalization in endothelial cells as a novel mechanism causing vascular inflammation.
Data Availability Statement
The raw data supporting the conclusion of this article will be made available by the authors, without undue reservation.
Ethics Statement
The studies involving human participants were reviewed and approved by the Ethics Committee of the Charité-Universitätsmedizin Berlin, Germany (EA4/042/ 11). The patients/participants provided their written informed consent to participate in this study.
Author Contributions
AK: Investigation, methodology, formal analysis, and writing—original draft and visualization; SF: Conceptualization, formal analysis, and writing—original draft; LU: Investigation; LO: Investigation; JK: Investigation and writing—review and editing; KH: Conceptualization of the Berlin Beats AF study, resources, and writing—review and editing; Juliane Herm: Resources and writing—review and editing; ME: Resources, writing—review and editing; JK: Investigation, writing—review and editing; VS: Investigation and writing—review and editing; CW: Resources and writing—review and editing; HK: Investigation, formal analysis, and writing—review and editing; Pablo Pelegrin: Resources; SG: Conceptualization of the in vitro study, supervision, formal analysis, and writing—review and editing, visualization; UL: Writing—review and editing, supervision, and project administration.
Funding
The investigator-initiated Berlin Beat of Running Study was supported by the SCC EVENTS GmbH, Bayer Healthcare, Sanofi-Aventis, and the GETEMED AG (Teltow, Germany). ME received funding from DFG under Germany´s Excellence Strategy—EXC-2049—390688087, BMBF, DZNE, DZHK, EU, Corona Foundation, and Foundation Leducq. Furthermore, this study was supported by the Leipzig University (research scholarship to LU) and the junior research grant of the Medical Faculty of Leipzig University to SG. The author(s) acknowledge support from the German Research Foundation (DFG) and Universität Leipzig within the program of Open Access Publishing.
Conflict of Interest
KGH reports a study grant by Bayer, lecture fees/advisory board fees from Abbott, AMARIN, AstraZeneca, Bayer, Biotronik, Boehringer Ingelheim, Bristol-Myers-Squibb, Daiichi Sankyo, Edwards Lifesciences, Medtronic, Pfizer, Premier Research, SUN Pharma and W. L. Gore & Associates.
ME reports grants from Bayer and fees paid to the Charité from AstraZeneca, Bayer, Boehringer Ingelheim, BMS, Daiichi Sankyo, Amgen, GSK, Sanofi, Covidien, Novartis, Pfizer, all outside the submitted work.
The remaining authors declare that the research was conducted in the absence of any commercial or financial relationships that could be construed as a potential conflict of interest.
Publisher’s Note
All claims expressed in this article are solely those of the authors and do not necessarily represent those of their affiliated organizations, or those of the publisher, the editors, and the reviewers. Any product that may be evaluated in this article, or claim that may be made by its manufacturer, is not guaranteed or endorsed by the publisher.
Acknowledgments
We would like to thank all researchers and participants of the Berlin Beat of Running study, especially Juliane Herm. In addition, we want to thank Pablo Pelegrin for providing the mutant NLRP3-YFP p.D303N human embryonic kidney 293 cells. We would like to thank Anja Barnikol-Oettler for great technical assistance. Yoon Ju Bae for steroid hormone analysis.
References
Armstrong M. E. G., Green J., Reeves G. K., Beral V., Cairns B. J. (2015). Frequent Physical Activity May Not Reduce Vascular Disease Risk as Much as Moderate Activity. Circulation 131, 721–729. doi:10.1161/CIRCULATIONAHA.114.010296
Bae Y. J., Kratzsch J., Zeidler R., Fikenzer S., Werner C., Herm J., et al. (2019). Unraveling the Steroid Hormone Response in Male marathon Runners: Correlation of Running Time with Aldosterone and Progesterone. J. Steroid Biochem. Mol. Biol. 195, 105473. doi:10.1016/j.jsbmb.2019.105473
Bailey D. M., Young I. S., McEneny J., Lawrenson L., Kim J., Barden J., et al. (2004). Regulation of Free Radical Outflow from an Isolated Muscle Bed in Exercising Humans. Am. J. Physiology-Heart Circulatory Physiol. 287, H1689–H1699. doi:10.1152/ajpheart.00148.2004
Baroja-Mazo A., Martín-Sánchez F., Gomez A. I., Martínez C. M., Amores-Iniesta J., Compan V., et al. (2014). The NLRP3 Inflammasome Is Released as a Particulate Danger Signal that Amplifies the Inflammatory Response. Nat. Immunol. 15, 738–748. doi:10.1038/ni.2919
Basiorka A. A., McGraw K. L., Abbas-Aghababazadeh F., McLemore A. F., Vincelette N. D., Ward G. A., et al. (2018). Assessment of ASC Specks as a Putative Biomarker of Pyroptosis in Myelodysplastic Syndromes: an Observational Cohort Study. Lancet Haematol. 5, e393–e402. doi:10.1016/S2352-3026(18)30109-1
Bauernfeind F., Bartok E., Rieger A., Franchi L., Núñez G., Hornung V. (2011). Cutting Edge: Reactive Oxygen Species Inhibitors Block Priming, but Not Activation, of the NLRP3 Inflammasome. J. Immunol. 187, 613–617. doi:10.4049/jimmunol.1100613
Bernat-Adell M. D., Collado-Boira E. J., Moles-Julio P., Panizo-González N., Martínez-Navarro I., Hernando-Fuster B., et al. (2021). Recovery of Inflammation, Cardiac, and Muscle Damage Biomarkers after Running a Marathon. J. Strength Cond. Res. 35, 626–632. doi:10.1519/JSC.0000000000003167
Bhaskar V., Yin J., Mirza A. M., Phan D., Vanegas S., Issafras H., et al. (2011). Monoclonal Antibodies Targeting IL-1 Beta Reduce Biomarkers of Atherosclerosis In Vitro and Inhibit Atherosclerotic Plaque Formation in Apolipoprotein E-Deficient Mice. Atherosclerosis 216, 313–320. doi:10.1016/j.atherosclerosis.2011.02.026
Breuckmann F., Möhlenkamp S., Nassenstein K., Lehmann N., Ladd S., Schmermund A., et al. (2009). Myocardial Late Gadolinium Enhancement: Prevalence, Pattern, and Prognostic Relevance in Marathon Runners. Radiology 251, 50–57. doi:10.1148/radiol.2511081118
Cuhadar S., Koseoglu M., Atay A., Dirican A. (2013). The Effect of Storage Time and Freeze-Thaw Cycles on the Stability of Serum Samples. Biochem. Med. 23, 70–77. doi:10.11613/bm.2013.009
Daiber A., Steven S., Weber A., Shuvaev V. V., Muzykantov V. R., Laher I., et al. (2017). Targeting Vascular (Endothelial) Dysfunction. Br. J. Pharmacol. 174, 1591–1619. doi:10.1111/bph.13517
de Jager W., Bourcier K., Rijkers G. T., Prakken B. J., Seyfert-Margolis V. (2009). Prerequisites for Cytokine Measurements in Clinical Trials with Multiplex Immunoassays. BMC Immunol. 10, 52. doi:10.1186/1471-2172-10-52
Devlin C. M., Kuriakose G., Hirsch E., Tabas I. (2002). Genetic Alterations of IL-1 Receptor Antagonist in Mice Affect Plasma Cholesterol Level and Foam Cell Lesion Size. Proc. Natl. Acad. Sci. U.S.A. 99, 6280–6285. doi:10.1073/pnas.092324399
Ding S., Xu S., Ma Y., Liu G., Jang H., Fang J. (2019). Modulatory Mechanisms of the NLRP3 Inflammasomes in Diabetes. Biomolecules 9, 850. doi:10.3390/biom9120850
Eijsvogels T. M. H., Thompson P. D., Franklin B. A. (2018). The "Extreme Exercise Hypothesis": Recent Findings and Cardiovascular Health Implications. Curr. Treat. Options. Cardio Med. 20, 84. doi:10.1007/s11936-018-0674-3
Franklin B. S., Bossaller L., De Nardo D., Ratter J. M., Stutz A., Engels G., et al. (2014). The Adaptor ASC Has Extracellular and 'prionoid' Activities that Propagate Inflammation. Nat. Immunol. 15, 727–737. doi:10.1038/ni.2913
Frenkel J., van Kempen M. J. A., Kuis W., van Amstel H. K. P. (2004). Variant Chronic Infantile Neurologic, Cutaneous, Articular Syndrome Due to a Mutation within the Leucine-Rich Repeat Domain ofCIAS1. Arthritis Rheum. 50, 2719–2720. doi:10.1002/art.20295
Gaul S., Schaeffer K. M., Opitz L., Maeder C., Kogel A., Uhlmann L., et al. (2021). Extracellular NLRP3 Inflammasome Particles Are Internalized by Human Coronary Artery Smooth Muscle Cells and Induce Pro-atherogenic Effects. Sci. Rep. 11, 15156. doi:10.1038/s41598-021-94314-1
Geng Y. J., Libby P. (1995). Evidence for Apoptosis in Advanced Human Atheroma. Colocalization with Interleukin-1 Beta-Converting Enzyme. Am. J. Pathol. 147, 251
Gibbs B. B., Hergenroeder A. L., Katzmarzyk P. T., Lee I.-M., Jakicic J. M. (2015). Definition, Measurement, and Health Risks Associated with Sedentary Behavior. Med. Sci. Sports Exerc. 47, 1295–1300. doi:10.1249/MSS.0000000000000517
Gong Z., Pan J., Shen Q., Li M., Peng Y. (2018). Mitochondrial Dysfunction Induces NLRP3 Inflammasome Activation during Cerebral Ischemia/reperfusion Injury. J. Neuroinflammation 15, 242. doi:10.1186/s12974-018-1282-6
Haeusler K. G., Herm J., Kunze C., Krüll M., Brechtel L., Lock J., et al. (2012). Rate of Cardiac Arrhythmias and Silent Brain Lesions in Experienced marathon Runners: Rationale, Design and Baseline Data of the Berlin Beat of Running Study. BMC Cardiovasc. Disord. 12, 69. doi:10.1186/1471-2261-12-69
Heid M. E., Keyel P. A., Kamga C., Shiva S., Watkins S. C., Salter R. D. (2013). Mitochondrial Reactive Oxygen Species Induces NLRP3-dependent Lysosomal Damage and Inflammasome Activation. J. Immunol. 191, 5230–5238. doi:10.4049/jimmunol.1301490
Herm J., Töpper A., Wutzler A., Kunze C., Krüll M., Brechtel L., et al. (2017). Frequency of Exercise-Induced ST-T-Segment Deviations and Cardiac Arrhythmias in Recreational Endurance Athletes during a marathon Race: Results of the Prospective Observational Berlin Beat of Running Study. BMJ open 7, e015798. doi:10.1136/bmjopen-2016-015798
Hoffman H. M., Mueller J. L., Broide D. H., Wanderer A. A., Kolodner R. D. (2001). Mutation of a New Gene Encoding a Putative Pyrin-like Protein Causes Familial Cold Autoinflammatory Syndrome and Muckle-Wells Syndrome. Nat. Genet. 29, 301–305. doi:10.1038/ng756
Hussain N., Gersh B. J., Gonzalez Carta K., Sydó N., Lopez-Jimenez F., Kopecky S. L., et al. (2018). Impact of Cardiorespiratory Fitness on Frequency of Atrial Fibrillation, Stroke, and All-Cause Mortality. Am. J. Cardiol. 121, 41–49. doi:10.1016/j.amjcard.2017.09.021
Janoudi A., Shamoun F. E., Kalavakunta J. K., Abela G. S. (2016). Cholesterol crystal Induced Arterial Inflammation and Destabilization of Atherosclerotic Plaque. Eur. Heart J. 37, 1959–1967. doi:10.1093/eurheartj/ehv653
JASP Team (2020). JASP (Amsterdam: JASP Team). [Computer software]https://jasp-stats.org/.
Jiang Q., Geng X., Warren J., Eugene Paul Cosky E., Kaura S., Stone C., et al. (2020). Hypoxia Inducible Factor-1α (HIF-1α) Mediates NLRP3 Inflammasome-Dependent-Pyroptotic and Apoptotic Cell Death Following Ischemic Stroke. Neuroscience 448, 126–139. doi:10.1016/j.neuroscience.2020.09.036
Khakroo Abkenar I., Rahmani-nia F., Lombardi G. (2019). The Effects of Acute and Chronic Aerobic Activity on the Signaling Pathway of the Inflammasome NLRP3 Complex in Young Men. Medicina 55, 105. doi:10.3390/medicina55040105
Koka S., Xia M., Chen Y., Bhat O. M., Yuan X., Boini K. M., et al. (2017). Endothelial NLRP3 Inflammasome Activation and Arterial Neointima Formation Associated with Acid Sphingomyelinase during Hypercholesterolemia. Redox Biol. 13, 336–344. doi:10.1016/j.redox.2017.06.004
Kyu H. H., Bachman V. F., Alexander L. T., Mumford J. E., Afshin A., Estep K., et al. (2016). Physical Activity and Risk of Breast Cancer, colon Cancer, Diabetes, Ischemic Heart Disease, and Ischemic Stroke Events: Systematic Review and Dose-Response Meta-Analysis for the Global Burden of Disease Study 2013. BMJ 354, i3857. doi:10.1136/bmj.i3857
Lear S. A., Hu W., Rangarajan S., Gasevic D., Leong D., Iqbal R., et al. (2017). The Effect of Physical Activity on Mortality and Cardiovascular Disease in 130 000 People from 17 High-Income, Middle-Income, and Low-Income Countries: the PURE Study. The Lancet 390, 2643–2654. doi:10.1016/S0140-6736(17)31634-3
Li H., Miao W., Ma J., Xv Z., Bo H., Li J., et al. (2015). Acute Exercise-Induced Mitochondrial Stress Triggers an Inflammatory Response in the Myocardium via NLRP3 Inflammasome Activation with Mitophagy. Oxidative Med. Cell Longevity 2016, 1–11. doi:10.1155/2016/1987149
Martin-Sanchez F. t., Gomez A., Pelegren P. (2015). Isolation of Particles of Recombinant ASC and NLRP3. Bio-protocol 5, e1480. doi:10.21769/BioProtoc.1480
Martínez-García J. J., Martínez-Banaclocha H., Angosto-Bazarra D., de Torre-Minguela C., Baroja-Mazo A., Alarcón-Vila C., et al. (2019). P2X7 Receptor Induces Mitochondrial Failure in Monocytes and Compromises NLRP3 Inflammasome Activation during Sepsis. Nat. Commun. 10, 2711. doi:10.1038/s41467-019-10626-x
Martinon F., Burns K., Tschopp J. (2002). The Inflammasome. Mol. Cel 10, 417–426. doi:10.1016/S1097-2765(02)00599-3
Merghani A., Maestrini V., Rosmini S., Cox A. T., Dhutia H., Bastiaenan R., et al. (2017). Prevalence of Subclinical Coronary Artery Disease in Masters Endurance Athletes with a Low Atherosclerotic Risk Profile. Circulation 136, 126–137. doi:10.1161/CIRCULATIONAHA.116.026964
Möhlenkamp S., Lehmann N., Breuckmann F., Bröcker-Preuss M., Nassenstein K., Halle M., et al. (2008). Running: the Risk of Coronary Events : Prevalence and Prognostic Relevance of Coronary Atherosclerosis in marathon Runners. Eur. Heart J. 29, 1903–1910. doi:10.1093/eurheartj/ehn163
Nidorf S. M., Fiolet A. T. L., Mosterd A., Eikelboom J. W., Schut A., Opstal T. S. J., et al. (2020). Colchicine in Patients with Chronic Coronary Disease. N. Engl. J. Med. 383, 1838–1847. doi:10.1056/NEJMoa2021372
Nieman D. C., Ferrara F., Pecorelli A., Woodby B., Hoyle A. T., Simonson A., et al. (2020). Postexercise Inflammasome Activation and IL-1β Production Mitigated by Flavonoid Supplementation in Cyclists. Int. J. Sport Nutr. Exerc. Metab. 30, 396–404. doi:10.1123/ijsnem.2020-0084
Panicucci C., Raffaghello L., Bruzzone S., Baratto S., Principi E., Minetti C., et al. (2020). eATP/P2X7R Axis: An Orchestrated Pathway Triggering Inflammasome Activation in Muscle Diseases. Int. J. Mol. Sci. 21, 5963. doi:10.3390/ijms21175963
Paramel Varghese G., Folkersen L., Strawbridge R. J., Halvorsen B., Yndestad A., Ranheim T., et al. (2016). NLRP3 Inflammasome Expression and Activation in Human Atherosclerosis. JAHA 5, e003031. doi:10.1161/JAHA.115.003031
Pelliccia A., Sharma S., Gati S., Bäck M., Börjesson M., Caselli S., et al. (2021). 2020 ESC Guidelines on Sports Cardiology and Exercise in Patients with Cardiovascular Disease. Eur. Heart J. 42, 17–96. doi:10.1093/eurheartj/ehaa605
Pincheira P. A., Hoffman B. W., Cresswell A. G., Carroll T. J., Brown N. A. T., Lichtwark G. A. (2021). Cyclic Eccentric Stretching Induces More Damage and Improved Subsequent protection Than Stretched Isometric Contractions in the Lower Limb. Eur. J. Appl. Physiol. 121, 3349–3360. doi:10.1007/s00421-021-04787-1
Proske U., Morgan D. L. (2001). Muscle Damage from Eccentric Exercise: Mechanism, Mechanical Signs, Adaptation and Clinical Applications. J. Physiol. 537, 333–345. doi:10.1111/j.1469-7793.2001.00333.x
Rheinheimer J., de Souza B. M., Cardoso A. C., Crispim D. (2017). Current Role of the NLRP3 Inflammasome on Obesity and Insulin Resistance: A Systematic Review. Metabolism 74, 1–9. doi:10.1016/j.metabol.2017.06.002
Ridker P. M., Everett B. M., Thuren T., MacFadyen J. G., Chang W. H., Ballantyne C., et al. (2017). Antiinflammatory Therapy with Canakinumab for Atherosclerotic Disease. N. Engl. J. Med. 377, 1119–1131. doi:10.1056/NEJMoa1707914
Roth G. A., Mensah G. A., Johnson C. O., Addolorato G., Ammirati E., Baddour L. M., et al. (2020). Global Burden of Cardiovascular Diseases and Risk Factors, 1990-2019: Update from the GBD 2019 Study. J. Am. Coll. Cardiol. 76, 2982–3021. doi:10.1016/j.jacc.2020.11.010
Santos V. C., Levada-Pires A. C., Alves S. R., Pithon-Curi T. C., Curi R., Cury-Boaventura M. F. (2013). Changes in Lymphocyte and Neutrophil Function Induced by a marathon Race. Cell Biochem Funct 31, 237–243. doi:10.1002/cbf.2877
Schunk S. J., Kleber M. E., März W., Pang S., Zewinger S., Triem S., et al. (2021). Genetically Determined NLRP3 Inflammasome Activation Associates with Systemic Inflammation and Cardiovascular Mortality. Eur. Heart J. 42, 1742–1756. doi:10.1093/eurheartj/ehab107
Schwarz V., Düsing P., Liman T., Werner C., Herm J., Bachelier K., et al. (2018). Marathon Running Increases Circulating Endothelial- and Thrombocyte-Derived Microparticles. Eur. J. Prev. Cardiolog 25, 317–324. doi:10.1177/2047487317744364
Souilhol C., Harmsen M. C., Evans P. C., Krenning G. (2018). Endothelial-mesenchymal Transition in Atherosclerosis. Cardiovasc. Res. 114, 565–577. doi:10.1093/cvr/cvx253
Sun H.-J., Ren X.-S., Xiong X.-Q., Chen Y.-Z., Zhao M.-X., Wang J.-J., et al. (2017). NLRP3 Inflammasome Activation Contributes to VSMC Phenotypic Transformation and Proliferation in Hypertension. Cell Death Dis 8, e3074. doi:10.1038/cddis.2017.470
Sun S.-W., Tong W.-J., Zheng G.-Q., Tuo Q.-H., Lei X.-Y., Liao D.-F. (2021). Pyroptotic Cell-Derived Microparticle: An Atherogenic Factor in Infectious Diseases. Med. hypotheses 146, 110370. doi:10.1016/j.mehy.2020.110370
Tardif J.-C., Kouz S., Waters D. D., Bertrand O. F., Diaz R., Maggioni A. P., et al. (2019). Efficacy and Safety of Low-Dose Colchicine after Myocardial Infarction. N. Engl. J. Med. 381, 2497–2505. doi:10.1056/NEJMoa1912388
Uhlemann M., Möbius-Winkler S., Fikenzer S., Adam J., Redlich M., Möhlenkamp S., et al. (2014). Circulating microRNA-126 Increases after Different Forms of Endurance Exercise in Healthy Adults. Eur. J. Prev. Cardiolog 21, 484–491. doi:10.1177/2047487312467902
van Tassell B. W., Toldo S., Mezzaroma E., Abbate A. (2013). Targeting Interleukin-1 in Heart Disease. Circulation 128, 1910–1923. doi:10.1161/CIRCULATIONAHA.113.003199
Waring O. J., Skenteris N. T., Biessen E. A. L., Donners M. M. P. C. (2021). Two-faced Janus: The Dual Role of Macrophages in Atherosclerotic Calcification. Cardiovasc. Res., 1–10. doi:10.1093/cvr/cvab301
Youm Y.-H., Nguyen K. Y., Grant R. W., Goldberg E. L., Bodogai M., Kim D., et al. (2015). The Ketone Metabolite β-hydroxybutyrate Blocks NLRP3 Inflammasome-Mediated Inflammatory Disease. Nat. Med. 21, 263–269. doi:10.1038/nm.3804
Zhao Z., Wang Y., Zhou R., Li Y., Gao Y., Tu D., et al. (2020). A Novel Role of NLRP3-Generated IL-1β in the Acute-Chronic Transition of Peripheral Lipopolysaccharide-Elicited Neuroinflammation: Implications for Sepsis-Associated Neurodegeneration. J. Neuroinflammation 17, 64. doi:10.1186/s12974-020-1728-5
Keywords: marathon, NLRP3, inflammasome, cardiovascular disease, endothelial dysfunction, inflammation
Citation: Kogel A, Fikenzer S, Uhlmann L, Opitz L, Kneuer JM, Haeusler KG, Endres M, Kratzsch J, Schwarz V, Werner C, Kalwa H, Gaul S and Laufs U (2022) Extracellular Inflammasome Particles Are Released After Marathon Running and Induce Proinflammatory Effects in Endothelial Cells. Front. Physiol. 13:866938. doi: 10.3389/fphys.2022.866938
Received: 31 January 2022; Accepted: 05 April 2022;
Published: 20 May 2022.
Edited by:
Shawn G Rhind, Defence Research and Development Canada (DRDC), CanadaReviewed by:
David Christopher Nieman, Appalachian State University, United StatesXavier Scott, University of Miami, United States
Debora Da Luz Scheffer, University of São Paulo, Brazil
Copyright © 2022 Kogel, Fikenzer, Uhlmann, Opitz, Kneuer, Haeusler, Endres, Kratzsch, Schwarz, Werner, Kalwa, Gaul and Laufs. This is an open-access article distributed under the terms of the Creative Commons Attribution License (CC BY). The use, distribution or reproduction in other forums is permitted, provided the original author(s) and the copyright owner(s) are credited and that the original publication in this journal is cited, in accordance with accepted academic practice. No use, distribution or reproduction is permitted which does not comply with these terms.
*Correspondence: Alexander Kogel, YWxleGFuZGVyLmtvZ2VsQG1lZGl6aW4udW5pLWxlaXB6aWcuZGU=