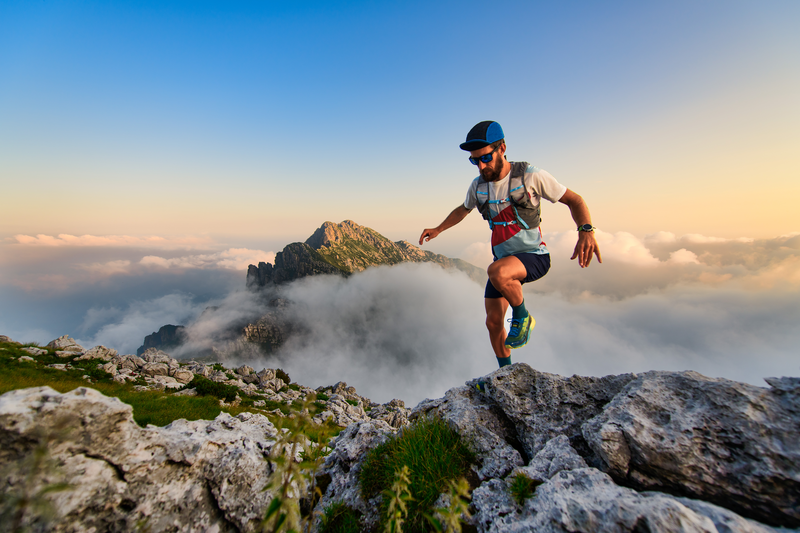
95% of researchers rate our articles as excellent or good
Learn more about the work of our research integrity team to safeguard the quality of each article we publish.
Find out more
ORIGINAL RESEARCH article
Front. Physiol. , 31 March 2022
Sec. Integrative Physiology
Volume 13 - 2022 | https://doi.org/10.3389/fphys.2022.841181
This article is part of the Research Topic Novel Mechanisms Involved in Urinary Bladder Control: Advances in Neural, Humoral and Local Factors Underlying Function and Disease, Volume II View all 12 articles
With many common bladder diseases arising due to abnormal contractions, a greater understanding of the receptor systems involved may aid the development of future treatments. The aim of this study was to identify any difference in the involvement of extracellular calcium (Ca2+) across prominent contractile-mediating receptors within cells lining the bladder. Strips of porcine urothelium and lamina propria were isolated from the urinary bladder dome and mounted in isolated tissue baths containing Krebs-bicarbonate solution, perfused with carbogen gas at 37°C. Tissue contractions, as well as changes to the frequency and amplitude of spontaneous activity were recorded after the addition of muscarinic, histamine, 5-hydroxytryptamine, neurokinin-A, prostaglandin E2, and angiotensin II receptor agonists in the absence and presence of 1 µM nifedipine or nominally zero Ca2+ solution. The absence of extracellular Ca2+ influx after immersion into nominally zero Ca2+ solution, or the addition of nifedipine, significantly inhibited the contractile responses (p < 0.05 for all) after stimulation with carbachol (1 µM), histamine (100 µM), 5-hydroxytryptamine (100 µM), neurokinin-A (300 nM), prostaglandin E2 (10 µM), and angiotensin II (100 nM). On average, Ca2+ influx from extracellular sources was responsible for between 20–50% of receptor-mediated contractions. This suggests that although the specific requirement of Ca2+ on contractile responses varies depending on the receptor, extracellular Ca2+ plays a key role in mediating G protein-coupled receptor contractions of the urothelium and lamina propria.
While strong and sustained bladder contractions are vital for urinary voiding, during the filling stage abnormal and spontaneous contractions can result in bladder dysfunction. One common presentation is underactive bladder, characterized by a slow urinary stream, hesitancy and straining to void, with or without a feeling of incomplete bladder emptying, and sometimes with storage symptoms (Chapple et al., 2018). Normally, voiding commences when M3 muscarinic receptors in the smooth muscle of the bladder wall are stimulated by neuronally derived acetylcholine. However, recent research has identified a number of other receptor systems on cells within the bladder wall which may also modulate bladder contractions (Stromberga et al., 2019; Stromberga et al., 2020b). Of particular interest are those linked to Gq/11 proteins, with their stimulation often resulting in strong tissue contractions. With many common bladder diseases arising as a result of abnormal contractions, an understanding of the potential receptor systems involved in this response is vital for the development of new and upcoming treatments for those affiliated with bladder dysfunctions.
A continuing interest has developed into the potential role of histamine (Stromberga et al., 2019), prostaglandins (Stromberga et al., 2020b), angiotensin (Lim et al., 2021), adrenergic (Moro et al., 2013), neurokinin (Grundy et al., 2018), muscarinic (Moro et al., 2011), and 5-hydroxytryptamine (Moro et al., 2016) receptors in influencing contractions of the urothelium and lamina propria (U&LP) layers. This layer has been observed to modulate overall bladder activity through the release of chemical mediators (Moro et al., 2011), as well as other pacemaking functions via the presence of the muscularis mucosae in the underlying connective tissue layer (Fry et al., 2007). The U&LP (also referred to as the bladder mucosa) may also play a key role in the mechanisms of action for current therapeutics (Moro et al., 2011), such as the parasympathomimetic bethanechol, which is one of the more commonly prescribed first-line treatments in the pharmaceutical management of underactive bladder (Kim, 2017). However, aside from this focus on the M3 muscarinic receptor, there is also a growing interest in combination therapies where, for example, administering both muscarinic agonists and alpha-adrenoceptor antagonists concurrently demonstrated greater success when compared to monotherapy in the management of underactive bladder (Yamanishi et al., 2004). This potential for alternative therapeutic options is important, as many patients who are administered muscarinic-acting pharmaceuticals in the management of bladder contractile disorders cease the regimen due to adverse side effects or lower than expected treatment outcomes (Yeowell et al., 2018). This is a growing area of research, with more work to be done regarding the potential benefits or harms from using parasympathomimetics in the management of underactive bladder (Moro et al., 2021).
Although the detrusor smooth muscle layer of the bladder has been the traditional target for research and therapeutic development, in recent years the importance of the U&LP in the maintenance of normal bladder function has been highlighted. The bladder generates spontaneous contractions, which can be altered in cases such as outlet obstruction or nerve injury (Kushida and Fry, 2016). The role of this spontaneous activity in the intact bladder is unclear (Fry and McCloskey, 2019), but may be mediated by signals originating in the U&LP, suggesting mechanisms where this tissue might underlie bladder contractile disorders (Fry and Vahabi, 2016). Calcium (Ca2+) and membrane potential transients commence in the lamina propria and spread towards the detrusor. In addition, the U&LP can release mediators, such as acetylcholine during stretching (Moro et al., 2011), which can induce increased spontaneous contractions in the detrusor (Kanai, 2007). A number of cells within the tissue may induce this spontaneous activity, as well as generate spontaneous Ca2+ transients, such as myofibroblasts (Sui et al., 2008), pericytes (Lee et al., 2016; Hashitani et al., 2018), interstitial-like cells (Fry and McCloskey, 2019) or muscularis mucosae (Heppner et al., 2011; Kushida and Fry, 2016). These cells remain highly sensitive to Ca2+, and there is value in identifying sources of Ca2+ influx into the tissue from extracellular fluids. Of note, however, is the potential for species differences in the generation and activity of phasic contractions. For example, the spontaneous activity of the bladder urothelium and lamina propria in the pig appears to predominantly arise from the muscularis mucosae, although there is evidence that this is not the case across all species (Mitsui et al., 2020).
With stimulation of the G protein-coupled receptors (GPCRs) resulting in contractions of the urinary bladder U&LP, identifying any common mechanisms of action between them is important. One primary function of the GPCRs in the urinary bladder may be the modulation of Ca2+ channels in the cell membranes, accommodating an influx of Ca2+ from extracellular fluids, and mediating a variety of physiological responses, from bladder contractions to increased pacemaker activity (Wuest et al., 2007). This study aims to identify similarities in extracellular Ca2+ requirements between muscarinic, histamine, 5-hydroxytryptamine (5-HT), neurokinin-A (NKA), prostaglandin E2 (PGE2), and angiotensin II (ATII) receptors for mediating contractile activity of the urinary bladder U&LP.
Urinary bladders from Large White-Landrace-Duroc pigs (6 months old, 80 kg live weight) were used as the tissue in this study. All bladders were obtained from the local abattoir after slaughter for the routine commercial provision of food with no animals bred, harmed, culled, interfered, or interacted with as part of this research project. As such, animal ethics approval was not required (Business Queensland, 2015). Only female bladders were used in this study. After collection from the abattoir, tissues were transported in a portable cooler in cold Krebs-Henseleit bicarbonate solution (NaCl 118.4 mM, NaHCO3 24.9 mM, D-glucose 11.7 mM, KCl 4.6 mM, MgSO4 2.41 mM, CaCl2 1.9 mM, and KH2PO4 1.18 mM) maintained at 4 °C to the University research facilities and used within 3 hours of the animal’s slaughter.
A single 4 cm strip was taken longitudinally from the bladder wall at the midpoint between the trigone and the bladder apex. Tissue strips were continually washed with a cold Krebs-Henseleit bicarbonate solution during the preparation and dissection stage. The white-coloured detrusor smooth muscle was dissected away from the pink-coloured urothelium and lamina propria using fine scissors. Histological assessment is known to effectively separate the two layers (Moro et al., 2012). After dissection and preparation, this single strip was cut in the middle, resulting in 2 × 2 cm strips which were mounted and suspended in 10 ml isolated tissue baths (Labglass, Brisbane, Australia) containing warmed Krebs solution at 37°C and perfused with carbogen gas (95% oxygen and 5% carbon dioxide). A maximum of two 4 cm strips were taken from each unique porcine bladder across the experiments. Throughout this manuscript, n values quoted are from paired tissue strips, and as such, the number of animals (N) used can be calculated using
Krebs-Henseleit bicarbonate solution ingredients were obtained from Sigma-Aldrich (Missouri, U.S.). Carbamylcholine chloride (carbachol) and histamine dihydrochloride (histamine) were obtained from Sigma-Aldrich (Missouri, U.S.), nifedipine and NKA were from Tocris Bioscience (Bristol, U.K.), 5-HT was from Toronto Research Centre (Toronto, CA), and ATII and PGE2 were obtained from Cayman Chemicals (Michigan, U.S.). Nifedipine and PGE2 were dissolved in 100% ethanol, while all other pharmaceutical agents were soluble in distilled water. Concentrations chosen for the agonists and antagonists were selected based on their selectivity at each receptor and consistent with concentrations used in previous studies. In each case, the agonist concentration used induced a submaximal contraction (with an aim to achieve around 80% of peak receptor-induced contraction).
In the nifedipine studies, a vehicle control of 100% ethanol was added to control tissues or nifedipine (1 µM) was added to the experimental tissues and left to equilibrate for 30 min. Nifedipine was kept in darkness until the final application in the organ bath and experiments were concluded within 30 min to ensure no adverse light impacts. In the Ca2+-free studies, control tissues were washed with Krebs solution as normal, whereas the experimental tissues were washed three times with a nominally Ca2+-free Krebs solution. This also ensured that any excess Ca2+ on the tissue, or Ca2+ leaking out from intracellular sources, was cleared from the bath. Tissues were then left to equilibrate for 3 min in nominally zero Ca2+ solution before agonists were added. A single dose of a select GPCR agonist was added to both the control and experimental tissues after equilibration. Tension, frequency, and amplitude of spontaneous contractions were measured with an isometric force transducer (MCT050/D, ADInstruments, Castle Hill, Australia) and recorded on a Powerlab system using Labchart v7 software (ADInstruments). Although a threshold was not applied, in all cases each spontaneous contraction exceeded 0.4 g.
Changes in tension and amplitude were measured in grams (g), where amplitude was measured from the lowest point of spontaneous phasic contraction to peak. Frequency was expressed as the number of spontaneous phasic contractions per minute (cpm). All data was analysed using GraphPad Prism version 9 (San Diego, CA), and results were presented as mean ± standard error of the mean (SEM). A paired t-test was used to analyse tissue responses before and after the addition of agonist. A paired Student’s two-tailed t-test was used to analyse the significance of results when comparing tissues with direct controls, as per previous studies (Stromberga et al., 2020a). A one-way ANOVA with Tukey post-test was also undertaken to compare the means where more than two variables were assessed. For all statistical analysis, p < 0.05 was considered statistically significant.
There is variation in the literature surrounding methods to remove extracellular Ca2+ from an isolated tissue bath. Firstly, this may involve the omission of calcium chloride (CaCl) from Krebs-Henseleit bicarbonate solution (Poyser, 1984). Secondly, the addition of Ca2+ chelating agents ethylenediaminetetraacetic acid (EDTA, 1–5 mM) (Maggi et al., 1989) or glycoletherdiaminetetraacetic acid (EGTA, 0.5 mM) solution (Yoshimura and Yamaguchi, 1997) have been proposed. However, EGTA may have impacts on other ions (Wheeler and Weiss, 1979) or other mechanisms within the tissue (Poyser, 1984), as well as potentially destabilise cell membranes, leading to increased Ca2+ permeability (Guan et al., 1988). To assess this feasibility of using Ca2+ chelators, we observed that compared to the simple omission of CaCl, the addition of 1 mM EDTA or 0.5 mM EGTA had no effect on contractile responses to our agonists (e.g., carbachol 1 µM). Thirdly, in some studies, the removal of Ca2+ appeared to result in a hypovolemic solution, and in order to rectify this, the concentration of other ions was altered. In our pilot studies, when incorporating additional Mg2+ to substitute for omitted Ca2+, there was no significant effect on contractions (n = 8). As such, no additional magnesium was added to replace the omission of the Ca2+ divalent cation. Fourthly, there is the potential for Ca2+ to enter the Ca2+-free bath solution from the cytoplasm, or via the activation of other cellular pumps/exchangers. To minimise the impact of this, after mounting in the bath, tissues were washed three times over 5 min in warmed Ca2+-free Krebs. As a final check, before and after contractions, the extracellular buffer was collected and checked with spectrophotometric assessments (Pacific Laboratory Products, Victoria, Australia), with no Ca2+ detected on this assay. This provided confidence in a nominally zero Ca2+ solution. As there were no observable or significant impacts to any contractions from adjusting for the methods listed above, the decision was made to henceforth solely remove Ca2+ from the Krebs solution and not alter anything else, as this was the path of least manipulation.
In the absence of stimulation from any agonist, U&LP tissue strips developed spontaneous phasic contractions at a frequency of 3.65 ± 0.08 cpm with an amplitude of 0.73 ± 0.04 g (n = 104). When receptor agonists carbachol (1 µM), histamine (100 µM), 5-HT (100 µM), NKA (300 nM), PGE2 (10 µM), and ATII (100 nM) were added to the tissues, U&LP baseline tension increased significantly for all activated receptors (p < 0.001, Table 1). In addition, the frequency of spontaneous phasic contractions increased for carbachol (p < 0.001), histamine (p < 0.05), 5-HT (p < 0.01), and ATII (p < 0.05), but not NKA or PGE2. Amplitude was reduced by all the agonists, but the changes were statistically significant for only carbachol (p < 0.05), 5-HT (p < 0.001), and ATII (p < 0.01).
TABLE 1. Summary of U&LP tissue spontaneous phasic activity. Tension, frequency, and amplitude recorded in response to receptor agonists. Data presented as mean ± SEM.
When nifedipine (1 µM) or vehicle control (totaling 0.035% ethanol) were added to tissues at the start of the 30 min equilibration period, there were no immediate changes to baseline tension, frequency, or amplitude for any of the agonists.
After activation of the muscarinic, histamine, 5-HT, NKA, PGE2 and ATII receptors, the baseline tension of the U&LP tissue increased, and the increases were similar for all the agonists (Figure 1). In the presence of nifedipine (1 µM), the contractions were inhibited as follows (paired Student’s two-tailed t-tests): carbachol by 54% (1µM, n = 11, p < 0.01); histamine by 45% (100µM, n = 8, p < 0.05); 5-HT by 28% (100µM, n = 8, p < 0.01); NKA by 49% (300nM, n = 8, p < 0.001); PGE2 by 29% (10µM, n = 8, p < 0.05); and ATII by 47% (100nM, n = 8, p < 0.05). The impact of nifedipine was relatively consistent after the activation of each agonist, with no significant differences found between any of the responses (p = NSD, ANOVA with Tukey post-test for this final statistical assessment only).
FIGURE 1. U&LP baseline tension responses to receptor agonists carbachol (1 µM), histamine (100 µM), 5-HT (100 µM), NKA (300 nM), PGE2 (10 µM), and ATII (100 nM) in the absence of (left) and the presence of (right) nifedipine (1 µM). *p < 0.05, **p < 0.01, ***p < 0.001 (paired Student’s two-tailed t-test).
The frequencies and amplitudes of spontaneous phasic contractions produced by the U&LP tissues were investigated in the absence and presence of nifedipine for each of the receptor agonists (Table 2). No significant differences in the contractile frequency or amplitude were observed between the presence and absence of nifedipine (1 µM) for responses to carbachol, histamine, 5-HT, or NKA. Contractions for PGE2 (10µM, n = 8, p < 0.01) and ATII (100nM, n = 8, p < 0.05) exhibited a reduction in the amplitude of spontaneous phasic contractions in the presence of nifedipine, and when compared to the response in the absence of nifedipine, was a statistically significant difference.
TABLE 2. U&LP change in frequency and amplitude responses to receptor agonists in the absence (control) and presence of nifedipine (1 µM). There were no significant differences between the average frequency changes between the absence and presence of nifedipine for any of the agonists. Data presented as mean ± SEM.
U&LP contractions for all receptor agonists in the presence of nominally zero Ca2+ solution were significantly inhibited compared to the control group in normal Krebs solution. In the absence of any extracellular Ca2+ sources, the contractions of the U&LP were impaired (paired Student’s two-tailed t-test, Figure 2). Contractions were inhibited as follows: carbachol by 39% (1µM, n = 11, p < 0.01); histamine by 46% (100 µM, n = 8, p < 0.05); 5-HT by 28% (100 µM, n = 8, p < 0.05); NKA by 22% (300 nM, n = 9, p < 0.05); PGE2 by 32% (10 µM, n = 8, p < 0.05); and ATII by 43% (100 nM, n = 8, p < 0.01). Across all receptors, there were no significant differences between the averaged responses (p = NSD, ANOVA with Tukey post-test).
FIGURE 2. U&LP baseline tension responses to receptor agonists carbachol (1 µM), histamine (100 µM), 5-HT (100 µM), NKA (300 nM), PGE2 (10 µM), and ATII (100 nM) as controls in the normal Krebs (left) and in nominally zero Ca2+ solution (right). *p < 0.05, **p < 0.01, ***p < 0.001 (paired Student’s two-tailed t-test).
Tissues exposed to a Ca2+-free extracellular environment had no alterations to their frequency or amplitude of spontaneous phasic contractions during responses to receptor agonists carbachol (1 µM), histamine (100 µM), 5-HT (100 µM), NKA (300 nM) or PGE2 (10 µM). Although the activation of all receptors induced increases to baseline tensions, only ATII (100 nM) also resulted in the significant decrease in the frequency of spontaneous phasic contractions in the presence of nominally zero Ca2+ solution (Table 3).
TABLE 3. U&LP change in frequency and amplitude responses to receptor agonists in the absence (control) and presence of nominally zero Ca2+ solution. No significant differences between the average amplitude changes between the absence and presence of nominally zero Ca2+ solution for any of the agonists. Data presented as mean ± SEM.
In this study, two different methods were applied to assess the impact of extracellular Ca2+: the immersion of the tissue in nominally zero Ca2+ solution; or through Ca2+ channel antagonism with nifedipine (1 µM). Both methods significantly inhibited contractile activity changes in response to the assessed agonists. Overall, when looking at the impact of either nifedipine or nominally zero Ca2+ solution, there were no significant differences (Student’s two-tailed unpaired t-tests for each) between the effectiveness of inhibitions of tension, frequency, or amplitude after the addition of carbachol (1 µM), histamine (100 µM), 5-HT (100 µM), NKA (300 nM), PGE2 (10 µM), and ATII (100nM, p = NSD for all).
The extent of influence that either extracellular or intracellular Ca2+ has on smooth muscle contraction varies throughout the body, promoting a specific interest towards identifying the prominent sources of Ca2+ influx across different organs. Ca2+ has a clear role in mediating the contractile activity of the bladder (Ikeda and Kanai, 2008), but the specific source of the Ca2+ in the urothelium and lamina propria tissue layer has never been investigated. Extracellular Ca2+ is of particular interest as it plays an essential role in many physiologic functions throughout the human body, and stimulates not only contraction, but also key underlying Ca2+ -dependent systems, which could be altered in bladder disorders (de Groat, 2004). As such, identifying the potential mechanisms involved in receptor-mediated contractions can support the development of new and novel pharmaceuticals, as well as develop a greater understanding of potential mechanisms underlying bladder dysfunction.
This study has identified prominent extracellular Ca2+ influences on muscarinic, histamine, 5-HT, neurokinin-A, prostaglandin E2 and angiotensin-II receptor mechanisms of action. Each of these receptor systems are G protein-coupled receptors of the Gq11 class. Particular subtypes of interest have been previously identified as the M3 muscarinic (Moro et al., 2011), H1 histamine (Stromberga et al., 2019), 5-HT2A serotonergic (Moro et al., 2016), neurokinin (Grundy et al., 2018), EP1 prostaglandin (Stromberga et al., 2020b), and AT1 angiotensin II (Lim et al., 2021) receptors. This broad presence of GPCRs in the U&LP constitutes the majority of surface receptors in the bladder, and many can be activated through neurotransmitters, hormones, and external stimuli that elicit a variety of cellular responses to stimulate downstream signalling activities (Mizuno and Itoh, 2009). Both inhibition of the L-type Ca2+ channels with nifedipine or inhibiting Ca2+ influx from extracellular fluids showed similar influences towards impairing GPCR contractions, inhibiting 20–50% of the contractile responses. These results are consistent with the finding by Heppner et al. (2011) where the inhibition of L-type voltage-gated Ca2+ channels reduced carbachol-induced contractions of human tissue by 74%, 18% in pig and 27% in mouse tissue. The additional finding that spontaneous phasic activity is not affected by the addition of nifedipine suggests that the overall conductance which generates the spontaneous contractions does not appear to have a relation with the L-type Ca2+ channels.
In this study, the response of each of the GPCRs to nifedipine has supported the presence of L-type voltage-gated Ca2+ channels in the U&LP of the urinary bladder. Lining the bladder lumen, the urothelium not only has an integral role in acting as the highly resistant physical barrier between urine and the underlying tissues, but also responds to stimuli and can transfer information to underlying cells (Birder and Andersson, 2013). Heppner et al. (2011) also observed the bladder U&LP of guinea pig tissue to exhibit dependence on Ca2+ influx through L-type Ca2+ channels, with spontaneous contractions significantly inhibited by nifedipine. However, clear evidence is lacking on the location of the L-type Ca2+ channels within these tissue layers. The lamina propria, a layer of highly innervated connective tissue located between the basement membrane of the urothelium and luminal surface of the detrusor, has demonstrated an essential role in signalling functions (Andersson and McCloskey, 2014; Heppner et al., 2017). In particular, its role in Ca2+ signalling may be of importance to the maintenance of normal bladder function and may present as a core site of extracellular Ca2+ influence. This could be through myofibroblasts, which have been identified to contribute to spontaneous activity through extracellular sources, and also express muscarinic and purinergic receptors that could assist in propagating signals to the urothelium (Heppner et al., 2011). Moreover, it has been suggested that the muscularis mucosae found within the underlying lamina propria of some species may also be impacted by the entry of extracellular Ca2+ (Heppner et al., 2011). In addition, interstitial cells of Cajal-like cells, located within the lamina propria layers, have also demonstrated firing activity of Ca2+ transients (Hashitani et al., 2004; Johnston et al., 2008), and there is some influence on the activity of pericytes surrounding blood vessels within the tissue (Mitsui and Hashitani, 2020).
The partial reduction (20–50%) in GPCR-mediated contractions of the U&LP by nifedipine may indicate additional sources of Ca2+ entry. This could include other voltage-gated channels such as T-type or P-type Ca2+ channels, previously identified in the urinary bladder (Deng et al., 2012). In addition, this partial reduction which was maintained in a Ca2+ -free environment suggests internal stores of Ca2+, such as from the sarcoplasmic reticulum, or other signalling pathways activated by Gq/11 receptor proteins, may play a role in mediating contractile activity of the U&LP.
Antimuscarinics and parasympathomimetics have shown success for the management of bladder contractile disorders, and currently sit as first-line pharmaceutical treatments for overactive bladder and underactive bladder, respectively. However, most patients cease treatment regimens due to lower than expected benefits and adverse side effects. Recent success has been found with combination therapies and there is increasing interest in the identification of alternative receptors systems that may be involved in contraction, and hence future targets for therapeutic treatments. This study’s identification of similarities between receptors mediating and modulating contraction in the urinary bladder may present future therapeutic targets or provide insights into mechanisms that may be dysfunctional in overactive or underactive bladder. Of particular interest was this study’s finding that histamine, neurokinin-A and angiotensin II are highly dependent on extracellular Ca2+, warranting further investigation into their clinical use for the management of bladder dysfunction.
A limitation of this study was the use of single-dose applications of receptor agonists to examine changes in frequencies and amplitudes of phasic contractions over 30-min time periods. It should be noted that in detrusor studies of carbachol-induced bladder contractions, human tissue responded to inhibited extracellular Ca2+ to a greater extent than pig tissue (Wuest et al., 2007), however, this has not been demonstrated in urothelial and lamina propria studies. Future studies could investigate the potential role of ageing in influencing receptor responses to extracellular contractions, such as histamine (Stromberga et al., 2020a), as well as explore the influence of other GPCRs and the influence of Ca2+ on their responses.
This study identified a prominent role of extracellular Ca2+ for urinary bladder contractile activity. The responses obtained from muscarinic, histamine, 5-HT, neurokinin-A, prostaglandin E2 and angiotensin II receptor activation are highly sensitive to extracellular Ca2+, presenting a potential mechanism underlying bladder dysfunction.
The raw data supporting the conclusion of this article will be made available by the authors, without undue reservation.
Data was collected by CP. The study design, analysis of data, and preparation of manuscript received equal contribution from all authors CP, RC-W, and CM.
CP was supported by an Australian Government Research Training Program Scholarship.
The authors declare that the research was conducted in the absence of any commercial or financial relationships that could be construed as a potential conflict of interest.
All claims expressed in this article are solely those of the authors and do not necessarily represent those of their affiliated organizations, or those of the publisher, the editors and the reviewers. Any product that may be evaluated in this article, or claim that may be made by its manufacturer, is not guaranteed or endorsed by the publisher.
Andersson K. E., McCloskey K. D. (2014). Lamina Propria: the Functional center of the Bladder? Neurourol. Urodynam. 33 (1), 9–16. doi:10.1002/nau.22465
Birder L., Andersson K.-E. (2013). Urothelial Signaling. Physiol. Rev. 93 (2), 653–680. doi:10.1152/physrev.00030.2012
Business Queensland (2015). Using Animals for Scientific Purposes. Queensland Government. [Online] Available at: https://www.business.qld.gov.au/industries/farms-fishing-forestry/agriculture/livestock/animal-welfare/animals-science/using-animals (Accessed March 3, 2021).
Chapple C. R., Osman N. I., Birder L., Dmochowski R., Drake M. J., van Koeveringe G., et al. (2018). Terminology Report from the International Continence Society (ICS) Working Group on Underactive Bladder (UAB). Neurourol. Urodynam. 37 (8), 2928–2931. doi:10.1002/nau.23701
de Groat W. C. (2004). The Urothelium in Overactive Bladder: Passive Bystander or Active Participant? Urology 64 (6), 7–11. doi:10.1016/j.urology.2004.08.063
Deng J., He P., Zhong X., Wang Q., Li L., Song B. (2012). Identification of T-type Calcium Channels in the Interstitial Cells of Cajal in Rat Bladder. Urology 80 (6), e1–13891387. doi:10.1016/j.urology.2012.07.031
Fry C. H., McCloskey K. D. (2019). Spontaneous Activity and the Urinary Bladder. Adv. Exp. Med. Biol. 1124, 121–147. doi:10.1007/978-981-13-5895-1_5
Fry C. H., Sui G.-P., Kanai A. J., Wu C. (2007). The Function of Suburothelial Myofibroblasts in the Bladder. Neurourol. Urodyn. 26 (6), 914–919. doi:10.1002/nau.20483
Fry C. H., Vahabi B. (2016). The Role of the Mucosa in normal and Abnormal Bladder Function. Basic Clin. Pharmacol. Toxicol. 119, 57–62. doi:10.1111/bcpt.12626
Grundy L., Chess-Williams R., Brierley S. M., Mills K., Moore K. H., Mansfield K., et al. (2018). NKA Enhances Bladder-Afferent Mechanosensitivity via Urothelial and Detrusor Activation. Am. J. Physiology-Renal Physiol. 315 (4), F1174–f1185. doi:10.1152/ajprenal.00106.2018
Hashitani H., Mitsui R., Miwa-Nishimura K., Lam M. (2018). Role of Capillary Pericytes in the Integration of Spontaneous Ca2+ Transients in the Suburothelial Microvasculature In Situ of the Mouse Bladder. J. Physiol. 596 (16), 3531–3552. doi:10.1113/jp275845
Hashitani H., Yanai Y., Suzuki H. (2004). Role of Interstitial Cells and gap Junctions in the Transmission of Spontaneous Ca2+signals in Detrusor Smooth Muscles of the guinea-pig Urinary Bladder. J. Physiol. 559, 567–581. doi:10.1113/jphysiol.2004.065136
Heppner T. J., Hennig G. W., Nelson M. T., Vizzard M. A. (2017). Rhythmic Calcium Events in the Lamina Propria Network of the Urinary Bladder of Rat Pups. Front. Syst. Neurosci. 11, 87. doi:10.3389/fnsys.2017.00087
Heppner T. J., Layne J. J., Pearson J. M., Sarkissian H., Nelson M. T. (2011). Unique Properties of Muscularis Mucosae Smooth Muscle in guinea Pig Urinary Bladder. Am. J. Physiology-Regulatory, Integr. Comp. Physiol. 301 (2), R351–R362. doi:10.1152/ajpregu.00656.2010
Ikeda Y., Kanai A. (2008). Urotheliogenic Modulation of Intrinsic Activity in Spinal Cord-Transected Rat Bladders: Role of Mucosal Muscarinic Receptors. Am. J. Physiology-Renal Physiol. 295 (2), F454–F461. doi:10.1152/ajprenal.90315.2008
Johnston L., Carson C., Lyons A. D., Davidson R. A., McCloskey K. D. (2008). Cholinergic-induced Ca2+ Signaling in Interstitial Cells of Cajal from the guinea Pig Bladder. Am. J. Physiology-Renal Physiol. 294 (3), F645–F655. doi:10.1152/ajprenal.00526.2007
Kanai A., Roppolo J., Ikeda Y., Zabbarova I., Tai C., Birder L., et al. (2007). Origin of Spontaneous Activity in Neonatal and Adult Rat Bladders and its Enhancement by Stretch and Muscarinic Agonists. Am. J. Physiology-Renal Physiol. 292, F1065–F1072. doi:10.1152/ajprenal.00229.2006
Kim D. K. (2017). Current Pharmacological and Surgical Treatment of Underactive Bladder. Investig. Clin. Urol. 58, S90–S98. doi:10.4111/icu.2017.58.S2.S90
Kushida N., Fry C. H. (2016). On the Origin of Spontaneous Activity in the Bladder. BJU Int. 117 (6), 982–992. doi:10.1111/bju.13240
Lee K., Mitsui R., Kajioka S., Naito S., Hashitani H. (2016). Role of PTHrP and Sensory Nerve Peptides in Regulating Contractility of Muscularis Mucosae and Detrusor Smooth Muscle in the guinea Pig Bladder. J. Urol. 196 (4), 1287–1294. doi:10.1016/j.juro.2016.04.082
Lim I., Mitsui R., Kameda M., Sellers D. J., Chess‐Williams R., Hashitani H. (2021). Comparative Effects of Angiotensin II on the Contractility of Muscularis Mucosae and Detrusor in the Pig Urinary Bladder. Neurourol. Urodynam. 40 (1), 102–111. doi:10.1002/nau.24548
Maggi C. A., Santicioli P., Geppetti P., Parlani M., Astolfi M., Bianco E. D., et al. (1989). The Effect of Calcium Free Medium and Nifedipine on the Release of Substance P-like Immunoreactivity and Contractions Induced by Capsaicin in the Isolated guinea-pig and Rat Bladder. Gen. Pharmacol. Vasc. Syst. 20 (4), 445–456. doi:10.1016/0306-3623(89)90194-8
Mitsui R., Hashitani H. (2020). Synchrony of Spontaneous Ca2+ Activity in Microvascular Mural Cells. J. Smooth Muscle Res. 56 (0), 1–18. doi:10.1540/jsmr.56.1
Mitsui R., Lee K., Uchiyama A., Hayakawa S., Kinoshita F., Kajioka S., et al. (2020). Contractile Elements and Their Sympathetic Regulations in the Pig Urinary Bladder: a Species and Regional Comparative Study. Cell Tissue Res 379 (2), 373–387. doi:10.1007/s00441-019-03088-6
Mizuno N., Itoh H. (2009). Functions and Regulatory Mechanisms of Gq-Signaling Pathways. Neurosignals 17 (1), 42–54. doi:10.1159/000186689
Moro C., Edwards L., Chess-Williams R. (2016). 5-HT2A Receptor Enhancement of Contractile Activity of the Porcine Urothelium and Lamina Propria. Int. J. Urol. 23 (11), 946–951. doi:10.1111/iju.13172
Moro C., Leeds C., Chess-Williams R. (2012). Contractile Activity of the Bladder Urothelium/lamina Propria and its Regulation by Nitric Oxide. Eur. J. Pharmacol. 674 (2-3), 445–449. doi:10.1016/j.ejphar.2011.11.020
Moro C., Phelps C., Veer V., Clark J., Glasziou P., Tikkinen K. A. O., et al. (2021). The Effectiveness of Parasympathomimetics for Treating Underactive Bladder: A Systematic Review and Meta‐analysis. Neurourology and Urodynamics 41 (1), 127–139. doi:10.1002/nau.24839
Moro C., Tajouri L., Chess-Williams R. (2013). Adrenoceptor Function and Expression in Bladder Urothelium and Lamina Propria. Urology 81 (1), e1–211. doi:10.1016/j.urology.2012.09.011
Moro C., Uchiyama J., Chess-Williams R. (2011). Urothelial/lamina Propria Spontaneous Activity and the Role of M3 Muscarinic Receptors in Mediating Rate Responses to Stretch and Carbachol. Urology 78 (6), e9–1442. doi:10.1016/j.urology.2011.08.039
Poyser N. L. (1984). Effect of Using Calcium-free Krebs' Solution on Basal and A23187-Stimulated Prostaglandin Output from the Day 15 guinea-pig Uterus Superfused In Vitro. Prostaglandins, Leukot. Med. 13 (3), 259–269. doi:10.1016/0262-1746(84)90038-6
Stromberga Z., Chess-Williams R., Moro C. (2020a). Alterations in Histamine Responses between Juvenile and Adult Urinary Bladder Urothelium, Lamina Propria and Detrusor Tissues. Sci. Rep. 10 (1), 1–9. doi:10.1038/s41598-020-60967-7
Stromberga Z., Chess-Williams R., Moro C. (2019). Histamine Modulation of Urinary Bladder Urothelium, Lamina Propria and Detrusor Contractile Activity via H1 and H2 Receptors. Sci. Rep. 9 (1), 3899. doi:10.1038/s41598-019-40384-1
Stromberga Z., Chess-Williams R., Moro C. (2020b). Prostaglandin E2 and F2alpha Modulate Urinary Bladder Urothelium, Lamina Propria and Detrusor Contractility via the FP Receptor. Front. Physiol. 11 (705), 1–11. doi:10.3389/fphys.2020.00705
Sui G.-P., Wu C., Roosen A., Ikeda Y., Kanai A. J., Fry C. H. (2008). Modulation of Bladder Myofibroblast Activity: Implications for Bladder Function. Am. J. Physiology-Renal Physiol. 295 (3), F688–F697. doi:10.1152/ajprenal.00133.2008
Wuest M., Hiller N., Braeter M., Hakenberg O. W., Wirth M. P., Ravens U. (2007). Contribution of Ca2+ Influx to Carbachol-Induced Detrusor Contraction Is Different in Human Urinary Bladder Compared to Pig and Mouse. Eur. J. Pharmacol. 565 (1-3), 180–189. doi:10.1016/j.ejphar.2007.02.046
Yamanishi T., Yasuda K., Kamai T., Tsujii T., Sakakibara R., Uchiyama T., et al. (2004). Combination of a Cholinergic Drug and an Alpha-Blocker Is More Effective Than Monotherapy for the Treatment of Voiding Difficulty in Patients with Underactive Detrusor. Int. J. Urol. 11 (2), 88–96. doi:10.1111/j.1442-2042.2004.00753.x
Yeowell G., Smith P., Nazir J., Hakimi Z., Siddiqui E., Fatoye F. (2018). Real-world Persistence and Adherence to Oral Antimuscarinics and Mirabegron in Patients with Overactive Bladder (OAB): a Systematic Literature Review. BMJ Open 8 (11), e021889. doi:10.1136/bmjopen-2018-021889
Keywords: bladder mucosa, G protein-coupled receptor (GCPR), l-type calcium channel, lamina propria, spontaneous activity, underactive bladder, muscularis mucosae
Citation: Phelps C, Chess-Williams R and Moro C (2022) The Dependence of Urinary Bladder Responses on Extracellular Calcium Varies Between Muscarinic, Histamine, 5-HT (Serotonin), Neurokinin, Prostaglandin, and Angiotensin Receptor Activation. Front. Physiol. 13:841181. doi: 10.3389/fphys.2022.841181
Received: 22 December 2021; Accepted: 14 March 2022;
Published: 31 March 2022.
Edited by:
Nick Spencer, Flinders University, AustraliaReviewed by:
Betty Exintaris, Monash University, AustraliaCopyright © 2022 Phelps, Chess-Williams and Moro. This is an open-access article distributed under the terms of the Creative Commons Attribution License (CC BY). The use, distribution or reproduction in other forums is permitted, provided the original author(s) and the copyright owner(s) are credited and that the original publication in this journal is cited, in accordance with accepted academic practice. No use, distribution or reproduction is permitted which does not comply with these terms.
*Correspondence: Christian Moro, Y21vcm9AYm9uZC5lZHUuYXU=
Disclaimer: All claims expressed in this article are solely those of the authors and do not necessarily represent those of their affiliated organizations, or those of the publisher, the editors and the reviewers. Any product that may be evaluated in this article or claim that may be made by its manufacturer is not guaranteed or endorsed by the publisher.
Research integrity at Frontiers
Learn more about the work of our research integrity team to safeguard the quality of each article we publish.