- 1Department of Clinical and Experimental Medicine, School of Medicine, University of Florence, Florence, Italy
- 2Sports Medicine Unit, Careggi University Hospital, Florence, Italy
- 3Department of Pediatric Surgery, Meyer Children’s University Hospital, University of Florence, Florence, Italy
- 4Department of Biomedical, Experimental and Clinical Sciences “Mario Serio”, University of Florence, Florence, Italy
Background: Exercise training increases muscle VO2 by increasing O2 transport and O2 uptake while cardiac output increase might be limited by the conformation of the chest in subjects with pectus excavatum (PE).
Aims: The aim of the present study was to investigate the influence of physical activity (PA) on functional parameters of cardiopulmonary performance and stroke volume obtained at Cardiopulmonary Exercise Test (CPET) in PE.
Methods and Procedures: A cohort of adolescents (15 with PE and 15 age- and sex-matched healthy controls, HC) underwent Cardiopulmonary Exercise Test (CPET) and administration of the International Physical Activity Questionnaire – Short Form (IPAQ-SF) with estimation of weekly PA (METs h–1⋅week–1). Determinants of CPET parameters were investigated with multivariable linear regression analysis.
Results: As expected, when compared to HC, PE had lower VO2 max (37.2 ± 6.6 vs. 45.4 ± 6.4 mL⋅kg–1⋅min–1, p < 0.05), and VO2/HR max (O2 pulse, 12.1 ± 2.4 vs. 16.2 ± 3.6 mL⋅min–1⋅bpm–1, p < 0.05). Importantly, physical activity level was a predictor of VO2 max (adjusted for sex, body mass index, FEV1%, and presence of PE, β = 0.085; 95% Cl 0.010 to 0.160, p = 0.029) whereas O2 pulse was independent from PA level (β = 0.035; 95% Cl −0.004 to 0.074).
Conclusion: Physical activity is a determinant of VO2 max (cardiopulmonary performance), whereas it appears not to affect O2 pulse (a measure of stroke volume at peak exercise) related to constrained diastolic filling in PE.
Introduction
Pectus excavatum (PE) is the most common congenital chest wall deformity of childhood. It occurs with an incidence of approximately 1 every 300–400 children (Neviere et al., 2011) and it occurs more frequently in boys (1:5) (Jaroszewski et al., 2010). Pectus excavatum begins to appear in childhood and manifests itself more frequently during puberty.
Although initially considered only an esthetic problem, it gradually emerged that this deformity can also cause functional disorders (Shneerson, 1998). Depending on the severity of the malformation, pectus excavatum can cause psychological, anatomical and physiological disorders of varying degrees (Alaca and Yüksel, 2021).
Outward appearance can become a major problem in adolescence, especially for those who show visible disfigurement (Steinmann et al., 2011). This can be reflected in choosing clothing or in avoiding social and sporting activities (Ji et al., 2011; Alaca and Yüksel, 2021). Limitation of activities can lead to physical deconditioning. Rowland et al. (2005) showed that adolescents with moderate to severe PE have lower endurance performance, expressed as the highest workload achieved, than healthy controls. In the most serious cases the dislocated sternum may also compress heart and lungs with a resultant stroke volume reduction, primarily caused by constrained diastolic filling. Both the limitation of physical activities and anatomical alterations finally result in real physiologic impairment with a reduction in exercise capacity and VO2 max, predominately because of impaired cardiovascular performance, rather than ventilatory limitation (Malek et al., 2003; Cavestri et al., 2010).
Deciding whether a patient can benefit from exercise-based treatment or should undergo surgery is crucial. Surgery repair of pectus excavatum is associated with postoperative pain (Archer et al., 2020; Uhl et al., 2020) and requires an important commitment on the part of the patient and his family. The minimally invasive Nuss surgical procedure is currently preferred for PE repair in children and adolescents with moderate and severe chest wall depressions (Morshuis et al., 1994; Haller and Loughlin, 2000; Sigalet et al., 2007; Neviere et al., 2011, 2013; Kelly et al., 2013; Maagaard et al., 2013; O’Keefe et al., 2013). Conservative treatments, based on the promotion of physical activity (PA), are preferred for patients with milder deformities (Abid et al., 2017).
Anatomical severity of PE is commonly measured by Haller Index, the ratio of the transverse diameter of the rib cage to the minimum distance of the lower sternum from the vertebra (Haller and Loughlin, 2000). However, the lack of predictive value of the index on the effects of surgery indicates that simple anatomical measurement is not sufficient to define the complex structural changes of the PE (Morshuis et al., 1994; Tang et al., 2012; Satur et al., 2021). With the cardiopulmonary test, which allows an integrated evaluation of the respiratory and circulatory parameters, an impairment of both functional capacity (VO2 max) and cardiac output (O2 pulse) was highlighted in patients with PE (Morshuis et al., 1994; Sigalet et al., 2007; Tang et al., 2012; Maagaard et al., 2013; O’Keefe et al., 2013). However, no correlation between the PA levels and cardiopulmonary impairment has been described in PE. The question has particular clinical significance both for indications for surgical intervention and for the promotion of PA in this group of patients. The promotion of PA could indeed enhance the functional capacity (VO2 max) but would probably have limited effects on cardiac output.
Aim of this pilot study is to explore the possible differential influence of physical activity on cardiopulmonary performance and stroke volume parameters obtained at Cardiopulmonary Exercise Test (CPET) in PE.
Materials and Methods
Patients Investigated
In total, 15 consecutive patients (4 females, 11 males) with PE, referred to our Unit for functional evaluation between March 2020 and August 2021 were investigated. The diagnoses were established by a thoracic surgeon (AMe) with 30 years’ experience. All the patients were in good health and had no evidence of cardiac or respiratory disease; none were taking medication that would affect cardiorespiratory fitness. Criteria for exclusion were severe lung disease, pre-existing heart disease, or an underlying causative syndrome such as Marfan’s disease, previous repair of pectus excavatum by any technique; previous thoracic surgery; congenital heart disease or pregnancy. The healthy control (HC) group consisted of 15 age- and sex-matched adolescents who responded to a local advertisement. The study was conducted according to the guidelines of the Declaration of Helsinki, and approved by the Tuscany Region clinical research ethics board (Comitato Etico Regionale per la Sperimentazione Clinica della Regione Toscana; protocol code 15840_bio, date of approval February 24, 2020). All participants provided written informed consent. All participants went through the same examinations and tests throughout the study.
Evaluation of Physical Activity Levels
An evaluation was made using the International Physical Activity Questionnaire-Short Form (IPAQ-SF) (Hallal and Victora, 2004). The questionnaire calculates the metabolic equivalent (MET) value, and considers the frequency, duration (minutes), and intensity of physical activities performed for at least 10 min. over the last seven days. The questionnaire consists of four parts: (1) vigorous-intensity activity (requiring hard physical effort that makes breathing much more frequent than normal) such as lifting heavy objects, doing aerobics, or cycling at a certain speed, including how many minutes per day; (2) moderate-intensity activity (requiring moderate physical effort that makes breathing a little more frequent than normal), such as carrying light weights, riding a bike at a regular pace or playing tennis doubles, but not walking; (3) walking, divided in slow, moderate and fast pace; (4) time spent sitting, including reading, watching television, studying and video games. The total volume of PA accumulated over a week, calculated by multiplying the duration of the reported category by its assigned MET value, was expressed as METs h–1⋅week–1. The mean intensity of light PA, moderate PA, and vigorous PA was assigned as 3, 4, and 8 METs, respectively (Wahid et al., 2016; Cheng et al., 2018; Rosselli et al., 2020).
The IPAQ-SF is a valid (r = 0.67) and reliable tool (rho = 0.77–1.00) (Craig et al., 2003), that is recommended for assessing PA in young and middle-aged adults (15–69 years) [Mannocci et al. (2018); The International Physical Activity Questionnaire, Available from: Guidelines for Data Processing and Analysis of the International Physical Activity (IPAQ, 2021)].
Cardiopulmonary Exercise
Each participant was asked to refrain from strenuous exertion the day before the test day and to refrain from eating solid foods or carbohydrate-rich drinks in the 3 h prior to the test. The test was performed in the morning in controlled environmental conditions (temperature 18–24°C, relative humidity 30–60%). Prior to exercise testing, body mass and body height were measured using an electronic scale. Body Mass Index (BMI, kg ⋅m–2) was calculated as the body mass divided by body height squared. Forced vital capacity (FVC), forced expiratory volume in 1 s (FEV1), and Tiffeneau index (FEV1/FVC) were also measured before the exercise test and expressed in percentage of the predicted value.
A symptom-limited CPET progressive ramp protocol was performed on an electronically braked cycle ergometer (Ergoline). Briefly, participants were equipped with an oronasal face mask connected to a metabolic cart (COSMED Quark CPET). CO2 exhaled and O2 consumed were measured using the breath-by-breath method. The workload ramp was individualized to achieve maximum exertion within 8 to 12 min. according to the recent recommendations reporting that VO2peak is maximal in 10-min ramp protocols (Paridon et al., 2006; Takken et al., 2017).
Selection of the workload ramp was based on predicted values for height (Godfrey et al., 1971). Minimum watt increase (1, 2, or 5 watt) for each ramp was set up to achieve the most linear increase possible and therefore the more uniform physiological responses. After 3 min of rest, patients performed a 3 min pedaling warm-up without load at 50 rpm. When the incremental workload started, patients were asked to pedal at 60–80 rpm until muscle exhaustion. The test was terminated when participants were no longer able to maintain pedaling frequency despite verbal encouragement. The test was considered maximal when at least 2 of the following conditions were reached: (1) peak respiratory exchange ratio (RER) ≥ 1.10; (2) HR max ≥ 85% of age-predicted maximum; (3) a plateauing of oxygen uptake (increase < 150 mL⋅min–1) in VO2 over the last 30 s of the test. Tests were interrupted if signs of cardiovascular distress were present (ventricular arrhythmias, falling blood pressure, dizziness, etc.). A twelve-lead ECG, and oxygen saturation were continuously monitored.
Oxygen Consumption (VO2), Carbon dioxide Production (VCO2), Tidal Volume (VT), Breathing Frequency (BF), Ventilation (VE), Heart Rate (HR) and Work Rate (WR) were measured throughout the test. The ventilatory Anaerobic Threshold (AT) was determined using the V-slope and the ventilatory equivalent approaches. Other variables were the ratio of VO2 and heart rate (VO2/HR, termed O2 pulse, a measure of stroke volume), oxygen-uptake to work-rate relationship (VO2/W slope, a measure of work efficiency), the product of VO2 max (in milliliters per kilogram per minute) by systolic blood pressure (VO2 max x SBP max, a measure of peak circulatory power).
Z score values were calculated by adopting the prediction model developed by Blanchard et al. (2018) assessing the relationship of the dependent variable (VO2 max) with three independent variables, height, body mass, and age/sex.
Statistical Considerations
Statistical analyses were performed using SPSS 27 software (IBM, Armonk, NY). Sample size determination and power calculations were made for the volume of oxygen uptake/kg (VO2). With an estimated standard deviation of 20%, a difference between the 2 groups of 20%, an alpha of 0.05 and a power of 0.80, the number necessary for investigation was 15 subjects for each group.
All continuous variables are expressed as mean ± SD, unless specified. A paired, two-tailed Student’s t-test was used for comparison for continuous variables. Multivariable linear regression analysis was used to analyze the correlations between variables. Independent variables were sex, BMI, FEV1%, and presence of pectus excavatum; VO2 max, VO2/HR max or VO2/W slope as dependent variable. Assuming that the dependent variable is predicted by the set of 5 predictors and that the squared population multiple correlation coefficient is 0.30, that is that the 5 predictors account for 30% of the variance of the dependent variable, the sample size is n = 30 subjects (power 0.66).
A difference of p < 0.05 was considered statistically significant.
Results
There were no significant inter-group differences in age or gender. Body mass index was significantly lower in the PE group compared to the HC group. No difference was observed between patients and controls in the level of habitual activity; more precisely, in the PE group, time spent per week in vigorous or moderate PA, as well as the total METs h–1⋅week–1 were not significantly different from those in the HC group (Table 1).
Forced vital capacity (FVC) and FEV1 were lower in the PE group than in healthy controls. However, the FEV1/FVC ratios did not differ between the two groups (Table 2).
Maximal heart rate was 184 ± 13 bpm in patients with pectus excavatum and 186 ± 7 bpm in controls (ns), indicating an equivalent maximal exercise effort in the 2 groups. Conversely, SBP at peak exercise showed lower values in the PE than in the HC group.
VO2 max was lower in the PE group than in HC both in absolute (37.2 ± 6.6 vs. 45.4 ± 6.4 mL⋅Kg–1⋅min–1, p < 0.05) and in percentage of the predicted value (76.9 ± 12.5% vs. 100.3 ± 8.3%, p < 0.05) (Figure 1). Average z score of VO2 max was -1.3 ± 1.0 (95% CI −1.97 to −0.58) and 0.2 ± 0.6 (95% CI −0.20 to 0.93) respectively. Similarly, O2 pulse at exercise peak (VO2/HR max) was lower in the PE group than in HC both in absolute (12.1 ± 2.4 vs. 16.2 ± 3.6 mL⋅beat–1, respectively, p < 0.05) and in percentage of the predicted value (87.5 ± 12.7% vs. 112.3 ± 10.6%, p < 0.05) (Table 3). Likewise work efficiency, expressed by the VO2/W slope, was lower in the PE than HC group (Table 3).
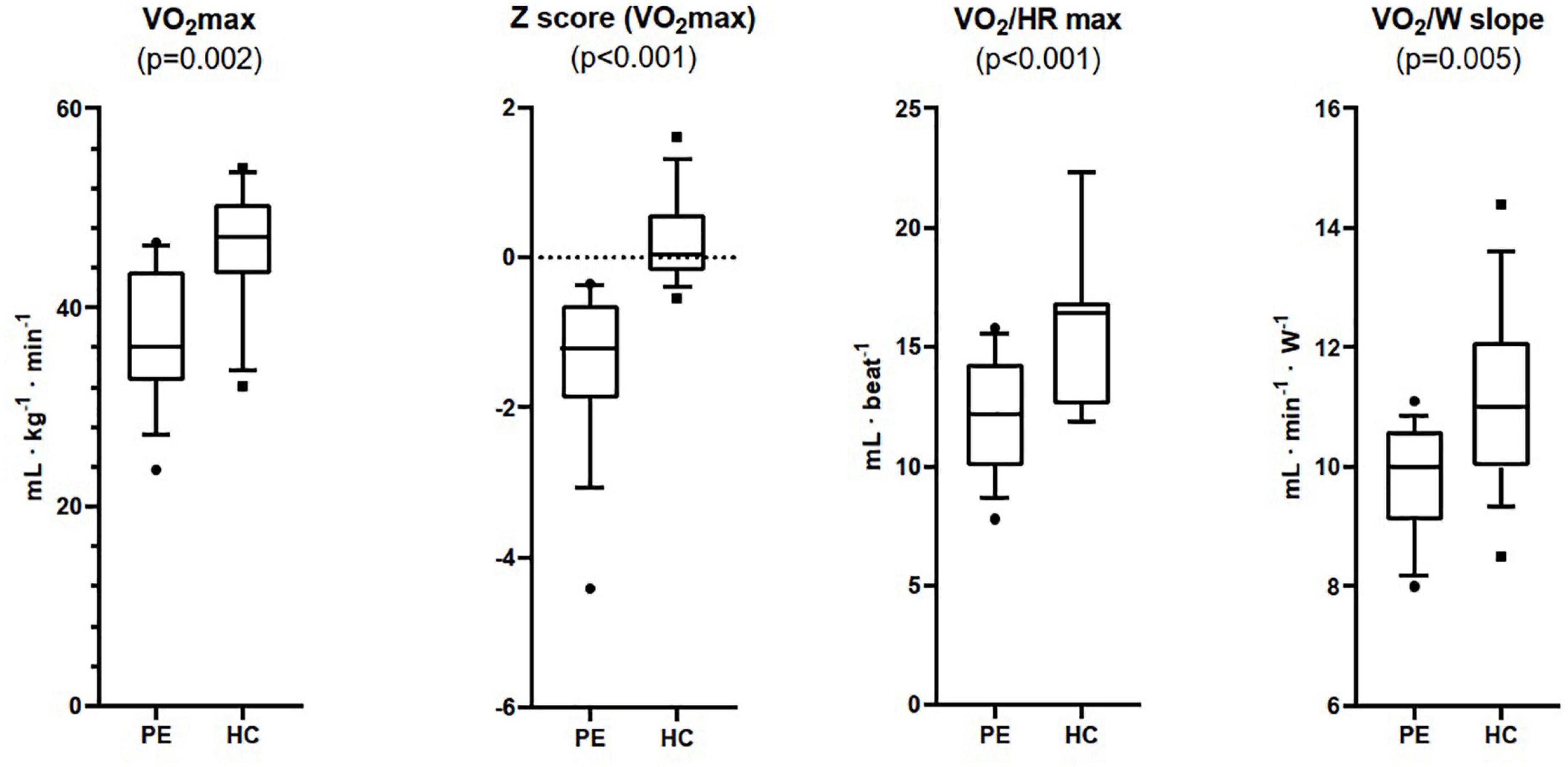
Figure 1. Maximum oxygen uptake (VO2 max), Z Score for VO2 max, maximum oxygen pulse (VO2/HR max), and work efficiency (VO2/W slope) measured at Cardiopulmonary Exercise Testing in patients with pectus excavatum (PE, n = 15), and healthy controls (HC, n = 15).
The relationships of cardiopulmonary performance (expressed by VO2 max), stroke volume at peak exercise (expressed as O2 pulse, VO2/HR max), and work efficiency (VO2/W slope) (all dependent variables) with average PA per week measured with IPAQ (independent variable) were then investigated at multivariable linear regression analysis adjusted for sex, BMI, FEV1%, and presence of pectus excavatum (Table 4).
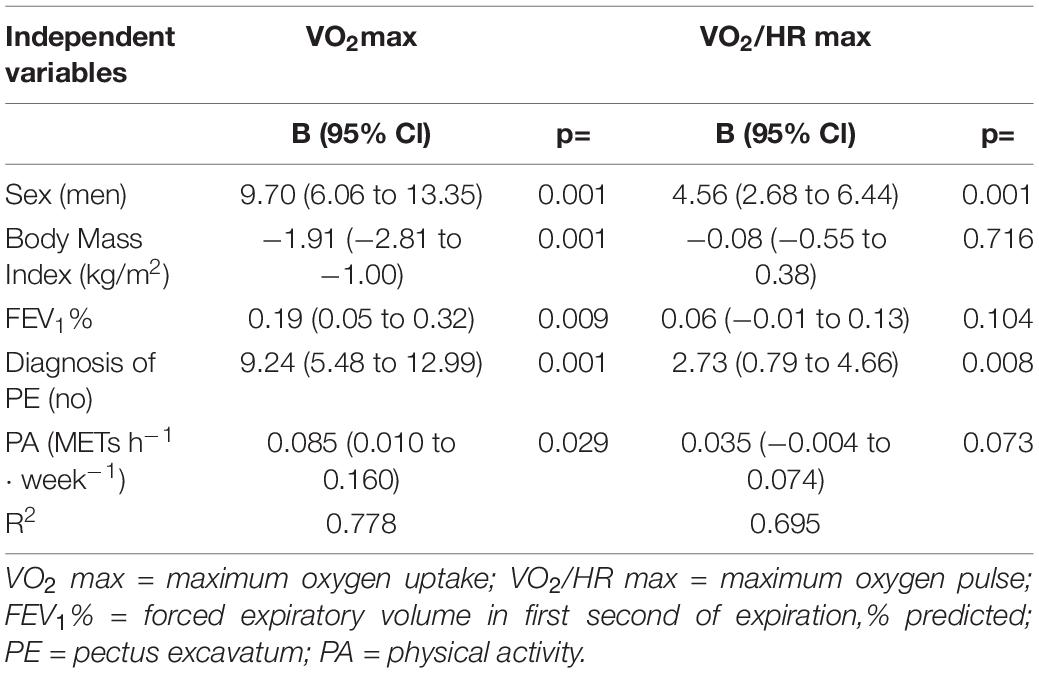
Table 4. Predictors of VO2 max, and VO2/HR max (dependent variables) at multivariable linear regression analysis.
VO2 max recognized among its determinants METs h–1⋅week–1. Conversely, both VO2/HR max and VO2/W slope were not affected by METs h–1⋅week–1 at linear regression analysis, adjusted with the same model (Figure 2).
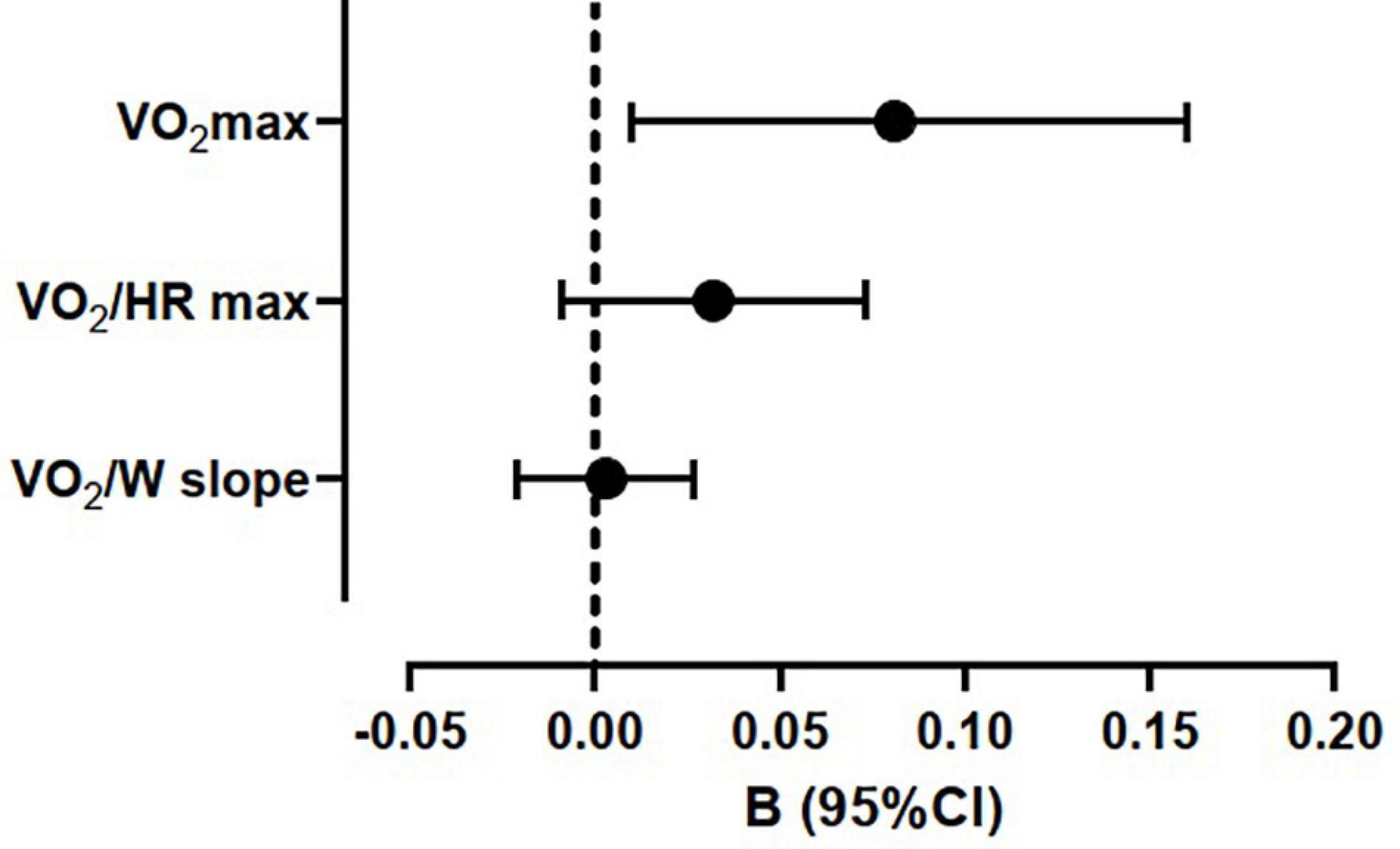
Figure 2. Regression coefficients of maximum oxygen uptake (VO2 max), maximum oxygen pulse (VO2/HR max), and work efficiency (VO2/W slope) with average weekly physical activity (METs h–1⋅week–1) at multiple linear regressions. The models were adjusted for sex, body mass index, forced expiratory volume in first second of expiration (% predicted), diagnosis of pectus excavatum, and physical activity (METs h–1⋅week –1).
Discussion
According to the present data, the average physical activity per week measured with the IPAQ questionnaire (Craig et al., 2003) was associated positively with a measurement of cardiopulmonary performance (VO2 max), whereas no association was observed with O2 pulse (VO2/HR max), a measure of stroke volume.
The relative role of psychological, anatomical and physiological alterations in determining functional impairment in PE is not easily discernible in the individual patient, although this point is crucial for the choice of the treatment strategy. A conservative strategy based on the promotion of PA may be useful to improve functional capacity, while the presence of an anatomical defect, with compression of the sternum resulting in a diastolic filling defect, could indicate a surgical choice. The results of this preliminary study seem to indicate that a cardiopulmonary test can help to assess the potential effectiveness of these two instruments. In patients with PE a reduced retrosternal space, leading to a diastolic dysfunction mainly in the right ventricle, could lead to a stroke volume reduction with a final impairment of cardiorespiratory fitness. In this study, O2 pulse, a measure of this mechanical parameter, was found to characterize the difference between PE and controls, independently of the estimation of PA or ventilatory function.
Cardiopulmonary performance expressed by VO2 max, also lower in PE compared to controls, instead also recognized among its determinants the average PA per week measured with the IPAQ. The promotion of PA in pectus excavatum, an important element in the treatment strategy of these patients, may improve cardiopulmonary performance, whereas stroke volume (O2 pulse) impairment that might be present in this condition seems to be independent from PA. A reduced O2 pulse at the peak of exercise could thus better address toward surgery.
Many studies have reported lower VO2 max in PE patients compared to controls (Jaroszewski et al., 2018; Obermeyer et al., 2018) and an increase in this parameter was observed after surgical repair (Maagaard and Heiberg, 2016; Obermeyer et al., 2018). Although physicians are very interested in cardiopulmonary test for this clinical condition, a consensus has not yet been established for the definition of a pathological threshold to address a patient to surgery. There have been few studies reporting reference values for submaximal and maximal cardiorespiratory fitness parameters, that may have interesting prognostic significance, such as O2 pulse, VO2/work slope, and the oxygen uptake efficiency slope. The main attention has been focused on VO2 max and O2 pulse, with limited attention to the great complexity of parameters offered by a cardiopulmonary test and their possible interaction with PA.
From our knowledge, this is the first attempt to correlate PA levels with cardiopulmonary test parameters in adolescents with PE.
Minimally invasive Nuss procedure for PE was consistently reported to improve stroke volume independently from the distance between surgery and functional evaluation due to the acute relief of constrained diastolic filling (Morshuis et al., 1994; Haller and Loughlin, 2000; Sigalet et al., 2007; Neviere et al., 2011, 2013; Kelly et al., 2013; Maagaard et al., 2013; O’Keefe et al., 2013). The increase in VO2 max was conversely not recorded when the assessment was carried out in the first months after surgery (Sigalet et al., 2007; O’Keefe et al., 2013). The present pilot study was dedicated to adding information in the decisional process between surgery and conservative strategies including the promotion of physical activity. Training program should be encouraged to increase cardiopulmonary performance expressed as VO2 max. However, a marked reduction in systolic stroke at peak exercise (expressed by the reduction in pulse O2) might instead suggest the surgical option.
For VO2 max the limit of normality was usually set at 80% of the predicted value (Blanchard et al., 2018). This lower limit seems to have been borrowed from the literature in forced spirometry (Miller et al., 2011), but its validity in categorizing normal cardiorespiratory fitness is lacking. This point, as well as the large inter-participant variability of VO2 max, has led to the possibility of using the Z-Score value, a statistic tool that indicates how far and in what direction (positive or negative) a measured value deviates from the population mean, expressed in units of the population SD. The limit of the ± 2 Z score directly identifies the 95% limit of a normal distribution and has often been chosen as the cut point to distinguish healthy from unhealthy. As a standardized measure, the Z-Score allows comparisons across different ages, genders and measurements. Most importantly, differently from adults, the use of the Z-Score allows more accurate assessments in children and adolescents, where small changes in age or pubertal stage produce big differences in sub-populations.
This is a pilot study with several limitations. First of all, an important limitation of the study is the limited number of participants studied. This limited number could be responsible for a type II error (beta) with the lack of correlation between PA and O2 pulse. The study must be considered a proof of principle study that may offer a perspective, although requiring confirmation. Second, there is a lack of accurate investigation of the diastolic function and of the morphological parameters used to characterize these patients, such as the echocardiographic indexes and the Haller index. Third, PA was measured with a questionnaire (IPAQ) and not an objective tool as accelerometer. Fourth, in the current study, differences in perceptions of body image and social appearance were not assessed. It may be important to assess the psychological impact by comparing the patient group with controls.
Conclusion
In conclusion, average PA per week measured with the IPAQ was found to be associated with cardiopulmonary performance expressed as VO2 max, whereas no relationship was observed with stroke volume at peak exercise, expressed by O2 pulse.
Data Availability Statement
The raw data supporting the conclusions of this article will be made available by the authors, without undue reservation.
Ethics Statement
The studies involving human participants were reviewed and approved by Comitato Etico Regionale per la Sperimentazione Clinica della Regione Toscana. Written informed consent to participate in this study was provided by the participants’ legal guardian/next of kin.
Author Contributions
PAM: conceptualization and writing—original draft preparation. LC and AP: methodology. AMo: formal analysis. LC, AP, AMe, MG, and FF: investigation. All authors have read and agreed to the published version of the manuscript.
Conflict of Interest
The authors declare that the research was conducted in the absence of any commercial or financial relationships that could be construed as a potential conflict of interest.
Publisher’s Note
All claims expressed in this article are solely those of the authors and do not necessarily represent those of their affiliated organizations, or those of the publisher, the editors and the reviewers. Any product that may be evaluated in this article, or claim that may be made by its manufacturer, is not guaranteed or endorsed by the publisher.
Acknowledgments
We would like to thank all participants who took part in this study. We also thanks Monica Betti, Sonia Degl’Innocenti, Elisabetta Grifoni, Patrizia La Rocca, Luana Mariotti, and Massimo Cirilli for their contribution to data collection. We are grateful to Annibale Biggeri from the Biostatistics Unit for support in statistical analyses.
References
Abid, I., Ewais, M. M., Marranca, J., and Jaroszewski, D. E. (2017). Pectus excavatum: a review of diagnosis and current treatment options. J. Am. Osteopath. Assoc. 117, 106–113. doi: 10.7556/jaoa.2017.021
Alaca, N., and Yüksel, M. (2021). Comparison of physical functions and psychosocial conditions between adolescents with pectus excavatum, pectus carinatum and healthy controls. Pediatr. Surg. Int. 37, 765–775. doi: 10.1007/s00383-021-04857-7
Archer, V., Robinson, T., Kattail, D., Fitzgerald, P., and Walton, J. M. (2020). Postoperative pain control following minimally invasive correction of pectus excavatum in pediatric patients: a systematic review. J. Pediatr. Surg. 55, 805–810. doi: 10.1016/j.jpedsurg.2020.01.023
Blanchard, J., Blais, S., Chetaille, P., Bisson, M., Counil, F. P., Huard-Girard, T., et al. (2018). New reference values for cardiopulmonary exercise testing in children. Med. Sci. Sports Exerc. 50, 1125–1133. doi: 10.1249/MSS.0000000000001559
Cavestri, B., Wurtz, A., Bart, F., Nevière, R., Aguilaniu, B., and Wallaert, B. (2010). [Cardiopulmonary exercise testing in patients with pectus excavatum]. Rev. Mal. Respir. 27, 717–723. doi: 10.1016/j.rmr.2010.06.015
Cheng, W., Zhang, Z., Yang, C., Diao, L., and Liu, W. (2018). Associations of leisure-time physical activity with cardiovascular mortality: a systematic review and meta-analysis of 44 prospective cohort studies. Eur. J. Prev. Cardiol. 25, 1864–1872. doi: 10.1177/2047487318795194
Craig, C. L., Marshall, A. L., Sjöström, M., Bauman, A. E., Booth, M. L., Ainsworth, B. E., et al. (2003). International physical activity questionnaire: 12-country reliability and validity. Med. Sci. Sports Exerc. 35, 1381–1395. doi: 10.1249/01.MSS.0000078924.61453.FB
Godfrey, S., Davies, C. T., Wozniak, E., and Barnes, C. A. (1971). Cardio-respiratory response to exercise in normal children. Clin. Sci. 40, 419–431. doi: 10.1042/cs0400419
Hallal, P. C., and Victora, C. G. (2004). Reliability and validity of the international physical activity questionnaire (IPAQ). Med. Sci. Sports Exerc. 36, 556. doi: 10.1249/01.mss.0000117161.66394.07
Haller, J. A., and Loughlin, G. M. (2000). Cardiorespiratory function is significantly improved following corrective surgery for severe pectus excavatum. Proposed treatment guidelines. J. Cardiovasc. Surg. 41, 125–130. doi: 10.1007/978-3-030-05108-2_9
IPAQ (2021). The International Physical Activity Questionnaire, Available from: Guidelines for Data Processing and Analysis of the International Physical Activity (IPAQ). Accessed at: http://www.ipaq.ki.se [Accessed on June, 30 2021]
Jaroszewski, D., Notrica, D., McMahon, L., Steidley, D. E., and Deschamps, C. (2010). Current management of pectus excavatum: a review and update of therapy and treatment recommendations. J. Am. Board Fam. Med. 23, 230–239. doi: 10.3122/jabfm.2010.02.090234
Jaroszewski, D. E., Velazco, C. S., Pulivarthi, V. S. K. K., Arsanjani, R., and Obermeyer, R. J. (2018). Cardiopulmonary function in thoracic wall deformities: what do we really know? Eur. J. Pediatr. Surg. 28, 327–346. doi: 10.1055/s-0038-1668130
Ji, Y., Liu, W., Chen, S., Xu, B., Tang, Y., Wang, X., et al. (2011). Assessment of psychosocial functioning and its risk factors in children with pectus excavatum. Health Qual Life Outcomes 9:28. doi: 10.1186/1477-7525-9-28
Kelly, R. E., Mellins, R. B., Shamberger, R. C., Mitchell, K. K., Lawson, M. L., Oldham, K. T., et al. (2013). Multicenter study of pectus excavatum, final report: complications, static/exercise pulmonary function, and anatomic outcomes. J. Am. Coll. Surg. 217, 1080–1089. doi: 10.1016/j.jamcollsurg.2013.06.019
Maagaard, M., and Heiberg, J. (2016). Improved cardiac function and exercise capacity following correction of pectus excavatum: a review of current literature. Ann. Cardiothorac. Surg. 5, 485–492. doi: 10.21037/acs.2016.09.03
Maagaard, M., Tang, M., Ringgaard, S., Nielsen, H. H., Frøkiær, J., Haubuf, M., et al. (2013). Normalized cardiopulmonary exercise function in patients with pectus excavatum three years after operation. Ann. Thorac. Surg. 96, 272–278. doi: 10.1016/j.athoracsur.2013.03.034
Malek, M. H., Fonkalsrud, E. W., and Cooper, C. B. (2003). Ventilatory and cardiovascular responses to exercise in patients with pectus excavatum. Chest 124, 870–882. doi: 10.1378/chest.124.3.870
Mannocci, A., Masala, D., Mei, D., Tribuzio, A. M., Villari, P., and La Torre, G. (2018). International physical activity questionnaire for adolescents (IPAQ A): reliability of an Italian version. Minerva Pediatr. 73, 383–390. doi: 10.23736/S0026-4946.16.04727-7
Miller, M. R., Quanjer, P. H., Swanney, M. P., Ruppel, G., and Enright, P. L. (2011). Interpreting lung function data using 80% predicted and fixed thresholds misclassifies more than 20% of patients. Chest 139, 52–59. doi: 10.1378/chest.10-0189
Morshuis, W. J., Folgering, H. T., Barentsz, J. O., Cox, A. L., van Lier, H. J., and Lacquet, L. K. (1994). Exercise cardiorespiratory function before and one year after operation for pectus excavatum. J. Thorac. Cardiovasc. Surg. 107, 1403–1409. doi: 10.1016/s0022-5223(94)70414-7
Neviere, R., Benhamed, L., Duva Pentiah, A., and Wurtz, A. (2013). Pectus excavatum repair improves respiratory pump efficacy and cardiovascular function at exercise. J. Thorac. Cardiovasc. Surg. 145, 605–606. doi: 10.1016/j.jtcvs.2012.07.078
Neviere, R., Montaigne, D., Benhamed, L., Catto, M., Edme, J. L., Matran, R., et al. (2011). Cardiopulmonary response following surgical repair of pectus excavatum in adult patients. Eur. J. Cardiothorac. Surg. 40, e77–e82. doi: 10.1016/j.ejcts.2011.03.045
Obermeyer, R. J., Cohen, N. S., and Jaroszewski, D. E. (2018). The physiologic impact of pectus excavatum repair. Semin. Pediatr. Surg. 27, 127–132. doi: 10.1053/j.sempedsurg.2018.05.005
O’Keefe, J., Byrne, R., Montgomery, M., Harder, J., Roberts, D., and Sigalet, D. L. (2013). Longer term effects of closed repair of pectus excavatum on cardiopulmonary status. J. Pediatr. Surg. 48, 1049–1054. doi: 10.1016/j.jpedsurg.2013.02.024
Paridon, S. M., Alpert, B. S., Boas, S. R., Cabrera, M. E., Caldarera, L. L., Daniels, S. R., et al. (2006). Clinical stress testing in the pediatric age group: a statement from the american heart association council on cardiovascular disease in the young, committee on atherosclerosis, hypertension, and obesity in youth. Circulation 113, 1905–1920. doi: 10.1161/CIRCULATIONAHA.106.174375
Rosselli, M., Ermini, E., Tosi, B., Boddi, M., Stefani, L., Toncelli, L., et al. (2020). Gender differences in barriers to physical activity among adolescents. Nutr. Metab. Cardiovasc. Dis. 30, 1582–1589. doi: 10.1016/j.numecd.2020.05.005
Rowland, T., Moriarty, K., and Banever, G. (2005). Effect of pectus excavatum deformity on cardiorespiratory fitness in adolescent boys. Arch. Pediatr. Adolesc. Med. 159, 1069–1073. doi: 10.1001/archpedi.159.11.1069
Satur, C. M. R., Cliff, I., and Watson, N. (2021). Can categorised values of maximal oxygen uptake discriminate patterns of exercise dysfunction in pectus excavatum: a prospective cohort study? BMJ Open Respir. Res. 8:e000940. doi: 10.1136/bmjresp-2021-000940
Shneerson, J. M. (1998). Rehabilitation in neuromuscular disorders and thoracic wall deformities. Monaldi Arch. Chest Dis. 53, 415–418.
Sigalet, D. L., Montgomery, M., Harder, J., Wong, V., Kravarusic, D., and Alassiri, A. (2007). Long term cardiopulmonary effects of closed repair of pectus excavatum. Pediatr. Surg. Int. 23, 493–497. doi: 10.1007/s00383-006-1861-y
Steinmann, C., Krille, S., Mueller, A., Weber, P., Reingruber, B., and Martin, A. (2011). Pectus excavatum and pectus carinatum patients suffer from lower quality of life and impaired body image: a control group comparison of psychological characteristics prior to surgical correction. Eur. J. Cardiothorac. Surg. 40, 1138–1145. doi: 10.1016/j.ejcts.2011.02.019
Takken, T., Bongers, B. C., van Brussel, M., Haapala, E. A., and Hulzebos, E. H. J. (2017). Cardiopulmonary exercise testing in pediatrics. Ann. Am. Thorac. Soc. 14, S123–S128. doi: 10.1513/AnnalsATS.201611-912FR
Tang, M., Nielsen, H. H., Lesbo, M., Frøkiær, J., Maagaard, M., Pilegaard, H. K., et al. (2012). Improved cardiopulmonary exercise function after modified Nuss operation for pectus excavatum. Eur. J. Cardiothorac. Surg. 41, 1063–1067. doi: 10.1093/ejcts/ezr170
Uhl, K. M., Wilder, R. T., Fernandez, A., Huang, H., Muhly, W. T., Zurakowski, D., et al. (2020). Postoperative pain and psychological outcomes following minimally invasive pectus excavatum repair: a report from the Society for Pediatric Anesthesia Improvement Network. Paediatr. Anaesth. 30, 1006–1012. doi: 10.1111/pan.13942
Keywords: exercise training, physical activity, cardiopulmonary exercise testing, O2 uptake, stroke volume, metabolic equivalent, pectus excavatum
Citation: Casatori L, Pellegrino A, Messineo A, Ghionzoli M, Facchini F, Modesti A and Modesti PA (2022) Differential Influence of Physical Activity on Cardiopulmonary Performance and Stroke Volume Assessed at Cardiopulmonary Exercise Test in Pectus Excavatum: A Pilot Study. Front. Physiol. 13:831504. doi: 10.3389/fphys.2022.831504
Received: 08 December 2021; Accepted: 10 January 2022;
Published: 03 February 2022.
Edited by:
Antonio Crisafulli, University of Cagliari, ItalyReviewed by:
Antonio Cittadini, University of Naples Federico II, ItalyFrancesco Giallauria, Federico II University Hospital, Italy
Copyright © 2022 Casatori, Pellegrino, Messineo, Ghionzoli, Facchini, Modesti and Modesti. This is an open-access article distributed under the terms of the Creative Commons Attribution License (CC BY). The use, distribution or reproduction in other forums is permitted, provided the original author(s) and the copyright owner(s) are credited and that the original publication in this journal is cited, in accordance with accepted academic practice. No use, distribution or reproduction is permitted which does not comply with these terms.
*Correspondence: Pietro Amedeo Modesti, cGEubW9kZXN0aUB1bmlmaS5pdA==
†These authors have contributed equally to this work and share first authorship