- 1Department of Medicine, Division of Pulmonary and Critical Care Medicine, University of California, Irvine, CA, United States
- 2Department of Medicine, Division of Pulmonary and Critical Care Medicine, VA Long Beach Healthcare System, Long Beach, CA, United States
- 3The Lundquist Institute for Biomedical Innovation at Harbor-UCLA Medical Center, Torrance, CA, United States
Lung resection surgery carries significant risks of postoperative pulmonary complications (PPC). Cardiopulmonary exercise testing (CPET) is performed to predict risk of PPC in patients with severely reduced predicted postoperative forced expiratory volume in one second (FEV1) and diffusion of carbon monoxide (DLCO). Recently, resting end-tidal partial pressure of carbon dioxide (PETCO2) has been shown as a good predictor for increased risk of PPC. However, breath-breath breathing pattern significantly affects PETCO2. Resting physiologic dead space (VD), and physiologic dead space to tidal volume ratio (VD/VT), may be a better predictor of PPC than PETCO2. The objective of this study was to prospectively determine the utility of resting measurements of VD and VD/VT in predicting PPC in patients who underwent robotic-assisted lung resection for suspected or biopsy-proven lung malignancy. Thirty-five consecutive patients were included in the study. Patients underwent preoperative pulmonary function testing, symptom-limited CPET, and a 6-min walk test. In the first 2 min prior to the exercise portion of the CPET, we obtained resting VT, minute ventilation (
Introduction
Preoperative evaluation of lung function is the standard of care to estimate the risks of postoperative pulmonary complications (PPC) following lung resection for lung nodules, either biopsy-proven, or suspicious for cancer. Lung resection surgery carries significant risks, including postoperative respiratory failure, pneumonia, and atelectasis, resulting in prolonged hospital length of stay and mortality. Preoperatively, cardiopulmonary exercise testing (CPET), stair climb or shuttle-walk tests, as well as forced expiratory volume in 1 s (FEV1), diffusion capacity for carbon monoxide (DLCO) have been utilized to assess patients’ risks of PPC. If there is no increased cardiac risk for lung resection surgery, but severely reduced postoperative predicted FEV1 and/or DLCO, or poor performance of stair climb or shuttle-walk test, current guidelines recommend CPET (Brunelli et al., 2013). With CPET, subjects who underwent lobectomy or pneumonectomy, maximum oxygen utilization or peak oxygen uptake (Peak
In healthy individuals,
where k is constant, equals to 863. From Eq. 1, as Roman et al. (2013) demonstrated, high resting
In a recent retrospective study, Brat et al. (2016) demonstrated elevated
The primary objective of our study was to determine if resting VD or VD/VT could reliably predict the risk of PPC within 90 days of robotic-assisted lung resection surgery in patients with a suspicious or biopsy-proven lung malignancy. The secondary objective was to compare the utility of resting VD or VD/VT with resting PETCO2 as a predictor of PPC in our population. We hypothesized that elevated resting VD or VD/VT will be useful and a better predictor of PPC risk than PETCO2.
Materials and Methods
Participants
Seventy-four patients with suspected or biopsy-proven lung malignancy were referred for lobectomy or segmentectomy by the multi-disciplinary team for lung cancer management at the Veteran Affairs Healthcare System, Long Beach, from January 2018 to January 2019. Thirty-nine patients were excluded for the following reasons: 1) declined to consent for the study (n = 11), declined or unable to perform CPET (n = 22), declined lung resection (n = 4), or had lung resection at another institution (n = 2). We studied the remaining 35 patients consecutively. Prior to the study each patient signed a written informed consent. Patients had to meet the following criteria: age greater than 18 years, non-pregnant, and ability to perform pulmonary function tests, six-minute walk test (6MWT), and CPET with no contraindications (Datta et al., 2015).
Study Design
This was a prospective observational study approved by the institutional review board of the Veteran Affairs Healthcare System, Long Beach. Patients underwent preoperative pulmonary function testing, 6MWT, and CPET.
Spirometry and breath-by-breath gas analysis during CPET was performed using Vmax EncoreTM System (Vyaire Medical, Irvine, CA, United States). CPET was performed on average 12 days prior to surgery on incremental cycle ergometer (VIAsprint 150PTM, AIM, Sylmar, CA, United States). All equipment was calibrated prior to every test. After allowing the patient to adapt to the breathing apparatus and seated quietly on the cycle ergometer for at least 15 min, CPET commenced. CPET involved 2 min of rest, followed by 2 min of unloaded pedaling, then continued with application of ramp-incremental work-rate profile (5–15 W/min) to the point of symptom limitation (Datta et al., 2015). During CPET an average of 10 s data points were displayed. No patients discontinued CPET for cardiac events. Single arterial blood sample was obtained from each patient proximate to the CPET 2-min rest period via radial artery puncture using standard technique, and immediately analyzed via blood gas analyzer (RapidPoint 400 Series, Bayer Healthcare Systems, Oxnard, CA). Samples were obtained at room temperature and corrected for body temperature of 37°C. From Eq. 1, utilizing resting
One board-certified cardiothoracic surgeon (AA) performed all lung resections using robotic-assisted surgery. The surgical team managed patients postoperatively with patients mobilized as soon as tolerated. PPCs were recorded after a thorough chart review and included events immediately after surgery up to 90 days post-surgery. The PPCs included 1) pneumonia defined as increased sputum production, leukocytosis, fever, positive sputum culture, and consolidation on chest x-ray, 2) respiratory failure requiring invasive or non-invasive mechanical ventilation, or 3) atelectasis requiring bronchoscopy.
Statistical Analysis
For this pilot study, a sample size of 24 subjects was required as determined using a mean difference of 25% according to the morbidity of postoperative lung resection reported by Brunelli et al. (2012), standard deviation of 30%, power of 0.80, and alpha of 0.05. Patients were grouped into those with and without PPC. Continuous variables were expressed as mean and standard deviation or standard error of the mean as appropriate; and compared using the unpaired two-tailed Student’s t test. Categorical variables, or those that did not pass the normality test and/or equal variance test, were compared using the Mann-Whitney Rank Sum test, and median with interquartile range values are reported. Differences in proportion were evaluated using the Fisher exact test. Receiver operating characteristic (ROC) curves were evaluated for threshold values for variables of interest, i.e., VD, VD/VT and PETCO2. In ROC, sensitivity, the dependent variable is the ability of a test to correctly identify patients with PPC, while specificity is the ability of a test to correctly identify those without PPC. Ideally, the threshold value has a sensitivity proximate 1.0 and 1-specificity (the independent variable) proximate 0, or the area under the ROC curve is close to 1.0. When the area under the ROC is statistically significant, the threshold value was determined from the highest sum of sensitivity and specificity according to the Youden approach (Bewick et al., 2004). Data were analyzed using Sigmaplot v.14 software (Systat Software Inc., San Jose, CA, United States).
Results
Postoperative pulmonary complications occurred in 14 (40%) out of 35 patients with one or more complications occurring in the same patient. There was no mortality. All patients underwent lobectomy, except one patient in each group, with and without PPC, had segmentectomy.
Table 1 shows the baseline characteristics of patients in both groups with and without PPC. Average age in both groups was 70 years, and all patients were male Veterans. Tobacco use did not differ between groups, but was somewhat higher in those with (40 packyears) than without (25 packyears) PPC. A similar trend was shown in the prevalence of COPD with 79% of those having PPC compared to 52% in those without PPC. The prebronchodilator spirometry, DLCO, and 6-min Walk distance were similar in both groups (Table 2).
Table 3 demonstrates the measured variables at rest and with exercise during CPET. At rest, the PPC group had significantly elevated VD, average was 0.318 L versus 0.230 L in those without PPC (p < 0.006). VD/VT tended to be greater (p = 0.051) in the PPC group, while PETCO2, other ventilatory variables, and arterial blood gases were not significantly different from those without PPC (Table 3). Resting hyperventilation was not observed during CPET as corroborated by the pH and PaCO2.
With exercise, peak
Postoperative pneumonia and atelectasis requiring therapeutic bronchoscopy were the most common PPCs (Table 4). As expected, patients with PPCs had significantly extended ICU and hospital length of stay compared with the group without PPC.
Figure 1 shows the ROC analysis for VD, VD/VT and PETCO2. The area under the ROC curve (AUC) was statistically significant for VD only (0.81, p = 0.002). The AUC for VD/VT and PETCO2 was 0.68 (p = 0.077) and 0.53 (p = 0.839), respectively. A threshold value for VD at or below 0.229 L appears to be an acceptable predictor for the lack of PPC with sensitivity of 62% (95% Confidence Interval (CI): 38%–82%), and specificity of 93% (95% CI: 66%–100%). The positive and negative likelihood ratio was 8.7 and 0.4, respectively.
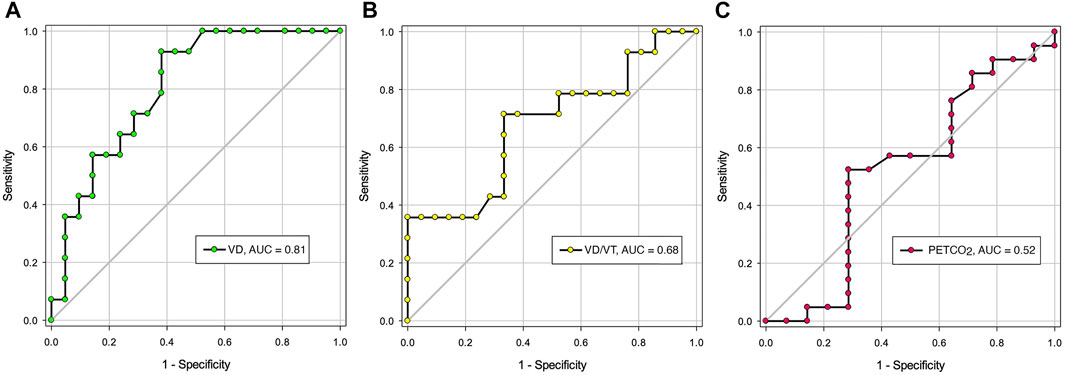
FIGURE 1. The area under the Receiver Operating Characteristic Curves for resting VD, VD/VT and PETCO2. (A). Area under the curve (AUC) for VD: 0.81 (p = 002); (B). AUC for VD/VT: 0.68 (p = 0.077); (C). AUC for PETCO2: 0.52 (p = 0.839). Definition of abbreviations: VD, dead space volume; VD/VT, dead space to tidal volume ratio; PETCO2, end-tidal CO2 pressure. NS, not significant.
Discussion
In this pilot study, our major findings were, 1) the preoperatively measured resting physiological dead space volume (VD) was the only variable separating groups of patients with and without PPC; 2) resting VD was also a significant predictor of PPC after robotic-assisted lung cancer resection surgery with a threshold value of 0.229 L; 3) resting end-tidal PCO2 was not useful in predicting the frequency of PPC; and 4) the PPC following robotic-assisted lung resection surgery was relatively high of 40%.
Resting Physiological Dead Space
Resting VD, the sum of anatomical and alveolar dead space, was obtained from ventilatory efficiency (
While VD differed significantly between groups with and without PPC, VD/VT did not. The lack of significant difference in VD/VT was possibly related to the mildly elevated VT in the PPC group despite statistically insignificant (Table 3). Patients with a high resting VD may require higher compensatory VT to achieve effective ventilation and removal of CO2. Thus, the VD/VT ratio was similar for both groups with and without PPC. There have been several studies in healthy subjects describing this phenomenon of elevated VT in response to elevated VD during exercise (Wasserman et al., 1967), and at rest (Krishnan et al., 1997). The possible mechanisms may include alterations in the PCO2 time profile or oscillations sensed by airway and/or pulmonary receptors, carotid chemoreceptors, or the central chemoreceptors (McParland et al., 1991). However, studies have shown that airway receptors (Krishnan et al., 1997) and carotid chemoreceptors (Syabbalo et al., 1993) do not play a major role in the mechanism of increased VT in response to elevated VD. Central chemoreceptors may possibly have a predominant role.
As shown in Table 2, a large percentage of our patients with PPC had mild COPD (prebronchodilator FEV1/FVC of 65.8%) with an average resting VD/VT of 0.35. Our findings were comparable to that of Elbehairy et al. (2015) with their mild COPD patients. The average prebronchodilator FEV1/FVC was 59.5% and resting VD/VT of 0.37. VT and frequency were not reported; however, minute ventilation (
In this trial or experimental cohort, resting VD appears a useful predictor of PPC following lung resection surgery at a threshold value of 0.229 L, with area under ROC curve of 0.81 (Figure 1). This confers the advantage for those patients who cannot or decline to perform CPET in the preoperative evaluation of postoperative complications risk after lung resection surgery. The positive likelihood ratio was 8.7, suggesting that a positive result is 8.7 times as likely for a patient who experienced PPC as one who did not. At the above threshold value, we obtained a sensitivity of 62% and specificity of 93%. The Youden index is 55% [(62% + 93%)−100%] suggesting that the resting VD threshold value yields an appreciable fraction that may be misclassified (Bewick et al., 2004). For this reason, this threshold value needs to be prospectively validated in a large number of patients.
Resting End-Tidal PCO2
A recent study of Brat et al. (2016) demonstrated that resting PETCO2 was a strong predictor of PPC following lung resection surgery. In contrast, our results differed in that resting PETCO2 was similar for both groups with and without PPC (Table 3). Differences might be related to patient characteristics such as 36% of their cohort were female; and/or variability in PETCO2 estimate. PETCO2 is critically influenced by breath-by-breath changes in the pattern of breathing. It is determined by the timing of end-exhalation during the alveolar phase-III of CO2 of each breath. In patients with airflow limitation a plateau of alveolar phase-III could not be attained resulting in large variability of its measurement and a large gradient between PaCO2 and PETCO2 (Sinha et al., 2011). Furthermore, particularly in COPD patients, wasted ventilation contaminates the measurements, as inhaled CO2 that doesn’t take part in gas-exchange is exhaled and dilutes the mixed expired CO2. Our study did not show significant difference in PaCO2-PETCO2 between both groups, probably related to variability of PETCO2.
Postoperative Pulmonary Complications Following Robotic-Assisted Lung Surgery
CPET has been considered as the gold standard in the evaluation of PPC following lung resection (Brunelli et al., 2013). Interestingly, neither Peak
Study Strength and Limitation
The strength of our study is the prospective design of the study and the use of PaCO2 rather than PETCO2 in the calculation of VD. However, our study has several shortcomings. First, our population consists of only male gender from a single institution, the Veterans Affairs Healthcare System. The results of this study, therefore, may not be applicable to the female population and a multi-center study would be desirable. Second, although the number of subjects studied exceeded the calculated sample size, the present study is relatively small involving a trial cohort and will require a validation cohort to test whether resting VD will hold as a reliable predictor of PPC risk. Furthermore, a multivariate analysis to assess the confounding effects of COPD cannot be performed. Nonetheless, resting VD stood out as a potentially useful predictor of PPC risk after robotic-assisted lung surgery. Third, all lung resections involved lobectomy except for two segmentectomies, and no pneumectomy was performed. Hence, resting VD as predictor of PPC is only applicable in those patients with lobectomies.
Conclusion
In summary, our prospective study demonstrated that following robotic-assisted lung resection for suspected or biopsy-proven lung cancer, resting VD obtained preoperatively separated those patients with and without postoperative pulmonary complications. Resting VD is also a potential predictor for postoperative pulmonary complications risk. However, this will have to be further validated with a large number of patients.
Data Availability Statement
The original contributions presented in the study are included in the article, further inquiries can be directed to the corresponding author.
Ethics Statement
The studies involving human participants were reviewed and approved by Institutional Review Board of the VA Long Beach Healthcare System. The patients/participants provided their written informed consent to participate in this study.
Author Contributions
RG designed the study, conducted the experiments, analyzed and critically interpreted the data and drafted the manuscript; SC conducted the experiments, and analyzed the data; AA designed the study and performed the robotic-assisted surgery; JP provided consultation for CPET, dead space calculation, and critically reviewed the manuscript; CS designed the study, critically analyzed, interpreted the data, drafted and revised the manuscript. All authors had full access to all of the study data, critically revised the manuscript for important intellectual content, approved the final version of the manuscript, and took responsibility for the integrity of the data and the accuracy of the data analysis.
Funding
Southern California Institute for Research and Education (SCIRE).
Conflict of Interest
The authors declare that the research was conducted in the absence of any commercial or financial relationships that could be construed as a potential conflict of interest.
Publisher’s Note
All claims expressed in this article are solely those of the authors and do not necessarily represent those of their affiliated organizations, or those of the publisher, the editors and the reviewers. Any product that may be evaluated in this article, or claim that may be made by its manufacturer, is not guaranteed or endorsed by the publisher.
Acknowledgments
The authors thank the contributions of Thomas Clevenger and Angela Tadeo for performing the pulmonary function and cardiopulmonary exercise testing.
References
Bewick V., Cheek L., Ball J. (2004). Statistics Review 13: Receiver Operating Characteristic Curves. Crit. Care. 8 (6), 508–512. doi:10.1186/cc3000
Brat K., Tothova Z., Merta Z., Taskova A., Homolka P., Vasakova M., et al. (2016). Resting End-Tidal Carbon Dioxide Predicts Respiratory Complications in Patients Undergoing Thoracic Surgical Procedures. Ann. Thorac. Surg. 102 (5), 1725–1730. doi:10.1016/j.athoracsur.2016.05.070
Brunelli A., Belardinelli R., Pompili C., Xiumé F., Refai M., Salati M., et al. (2012). Minute Ventilation-to-Carbon Dioxide Output (e/co2) Slope Is the Strongest Predictor of Respiratory Complications and Death After Pulmonary Resection. Ann. Thorac. Surg. 93, 1802–1806. doi:10.1016/j.athoracsur.2012.03.022
Brunelli A., Belardinelli R., Refai M., Salati M., Socci L., Pompili C., et al. (2009). Peak Oxygen Consumption during Cardiopulmonary Exercise Test Improves Risk Stratification in Candidates to Major Lung Resection. Chest 135, 1260–1267. doi:10.1378/chest.08-2059
Brunelli A., Kim A. W., Berger K. I., Addrizzo-Harris D. J. (2013). Physiologic Evaluation of the Patient with Lung Cancer Being Considered for Resectional Surgery. Chest 143 (5 Suppl. l), e166S–e190S. doi:10.1378/chest.12-2395
Datta D., Normandin E., ZuWallack R. (2015). Cardiopulmonary Exercise Testing in the Assessment of Exertional Dyspnea. Ann. Thorac. Med. 10 (2), 77–86. doi:10.4103/1817-1737.151438
Elbehairy A. F., Ciavaglia C. E., Webb K. A., Guenette J. A., Jensen D., Mourad S. M., et al. (2015). Pulmonary Gas Exchange Abnormalities in Mild Chronic Obstructive Pulmonary Disease. Implications for Dyspnea and Exercise Intolerance. Am. J. Respir. Crit. Care Med. 191 (12), 1384–1394. doi:10.1164/rccm.201501-0157OC
Keteyian S. J., Isaac D., Thadani U., Roy B. A., Bensimhon D. R., McKelvie R., et al. (2009). Safety of Symptom-Limited Cardiopulmonary Exercise Testing in Patients with Chronic Heart Failure Due to Severe Left Ventricular Systolic Dysfunction. Am. Heart J. 158 (4 Suppl. l), S72–S77. doi:10.1016/j.ahj.2009.07.014
Krishnan B. S., Stockwell M. J., Clemens R. E., Gallagher C. G. (1997). Airway Anesthesia and Respiratory Adaptations to Dead Space Loading and Exercise. Am. J. Respir. Crit. Care Med. 155 (2), 459–465. doi:10.1164/ajrccm.155.2.9032179
Lewis D. A., Sietsema K. E., Casaburi R., Sue D. Y. (1994). Inaccuracy of Noninvasive Estimates of VD/VT in Clinical Exercise Testing. Chest 106 (5), 1476–1480. doi:10.1378/chest.106.5.1476
McParland C., Mink J., Gallagher C. G. (1991). Respiratory Adaptations to Dead Space Loading during Maximal Incremental Exercise. J. Appl. Physiology 70 (1), 55–62. doi:10.1152/jappl.1991.70.1.55
Mezzani A. (2017). Cardiopulmonary Exercise Testing: Basics of Methodology and Measurements. Ann. ATS 14 (Suppl. 1), S3–S11. doi:10.1513/AnnalsATS.201612-997FR
Robertson H. T. (2015). Dead Space: the Physiology of Wasted Ventilation. Eur. Respir. J. 45 (6), 1704–1716. doi:10.1183/09031936.00137614
Roman M. A., Casaburi J. D., Porszasz J., Casaburi R. (2013). Noninvasive Assessment of Normality of V D/V T in Clinical Cardiopulmonary Exercise Testing Utilizing Incremental Cycle Ergometry. Eur. J. Appl. Physiol. 113 (1), 33–40. doi:10.1007/s00421-012-2407-8
Sinha P., Flower O., Soni N. (2011). Deadspace Ventilation: a Waste of Breath!. Intensive Care Med. 37 (5), 735–746. doi:10.1007/s00134-011-2194-4
Smith J. R., Olson T. P. (2019). Ventilatory Constraints Influence Physiological Dead Space in Heart Failure. Exp. Physiol. 104 (1), 70–80. doi:10.1113/EP087183
Syabbalo N. C., Zintel T., Watts R., Gallagher C. G. (1993). Carotid Chemoreceptors and Respiratory Adaptations to Dead Space Loading during Incremental Exercise. J. Appl. Physiology 75 (3), 1378–1384. doi:10.1152/jappl.1993.75.3.1378
Velez-Cubian F. O., Ng E. P., Fontaine J. P., Toloza E. M. (2015). Robotic-Assisted Videothoracoscopic Surgery of the Lung. Cancer control. 22 (3), 314–325. doi:10.1177/107327481502200309
Veluswamy R. R., Whittaker Brown S.-A., Mhango G., Sigel K., Nicastri D. G., Smith C. B., et al. (2020). Comparative Effectiveness of Robotic-Assisted Surgery for Resectable Lung Cancer in Older Patients. Chest 157 (5), 1313–1321. doi:10.1016/j.chest.2019.09.017
Wasserman K., Van Kessel A. L., Burton G. G. (1967). Interaction of Physiological Mechanisms during Exercise. J. Appl. Physiology 22 (1), 71–85. doi:10.1152/jappl.1967.22.1.71
Whipp B. J. (2006). Exercise Physiology. Wiley Encyclopedia of Biomedical Engineering, 1–14. Available at: https://onlinelibrary.wiley.com/doi/epdf/10.1002/9780471740360.ebs0464.
Keywords: physiologic dead space, postoperative predictor, pulmonary complications, robotic-assisted lung resection, lung cancer
Citation: Godbole R, Church SB, Abolhoda A, Porszasz J and Sassoon CSH (2022) Resting Physiologic Dead Space as Predictor of Postoperative Pulmonary Complications After Robotic-Assisted Lung Resection: A Pilot Study. Front. Physiol. 13:803641. doi: 10.3389/fphys.2022.803641
Received: 28 October 2021; Accepted: 17 June 2022;
Published: 18 July 2022.
Edited by:
Andrew John Halayko, University of Manitoba, CanadaReviewed by:
Devin B. Phillips, Queen’s University, CanadaMarta Guðjónsdóttir, University of Iceland, Iceland
Copyright © 2022 Godbole, Church, Abolhoda, Porszasz and Sassoon. This is an open-access article distributed under the terms of the Creative Commons Attribution License (CC BY). The use, distribution or reproduction in other forums is permitted, provided the original author(s) and the copyright owner(s) are credited and that the original publication in this journal is cited, in accordance with accepted academic practice. No use, distribution or reproduction is permitted which does not comply with these terms.
*Correspondence: Catherine S. H. Sassoon, Y3Nhc3Nvb25AdWNpLmVkdQ==