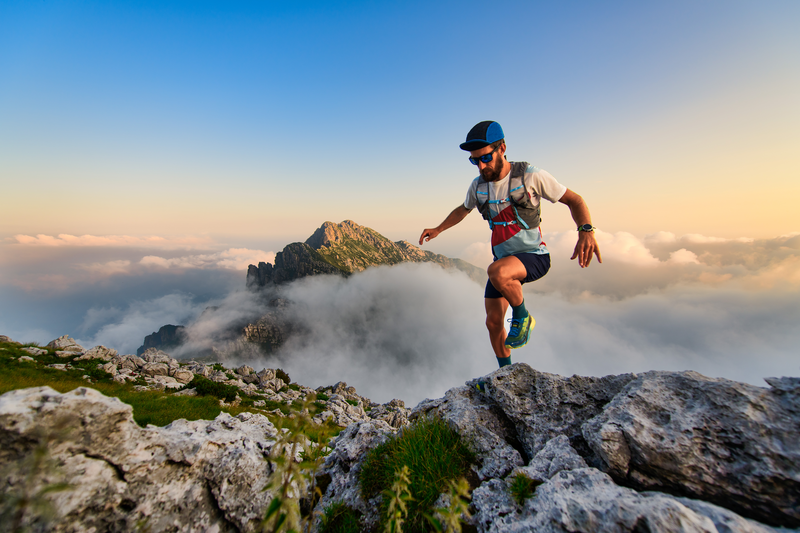
95% of researchers rate our articles as excellent or good
Learn more about the work of our research integrity team to safeguard the quality of each article we publish.
Find out more
ORIGINAL RESEARCH article
Front. Physiol. , 13 July 2022
Sec. Exercise Physiology
Volume 13 - 2022 | https://doi.org/10.3389/fphys.2022.797367
This article is part of the Research Topic Physiological and Biomechanical Determinants of Swimming Performance View all 12 articles
This study explored in the 50 m races of the four swimming strokes the performance parameters and/or technical variables that determined the differences between swimmers who reach the finals and those who do not. A total of 322 performances retrieved from the 2021 Budapest European championships were the focus of this study. The results of the performances achieved during the finals compared to the heats showed that the best swimmers did not excel during the heats, as a significant progression of performance was observed in most of the strokes as the competition progressed. Specifically, combining men and women, the swimmers had in freestyle a mean coefficient of variation (CV) of ∼0.6%, with a mean range of performance improvement (∆%) of ∆ = ∼0.7%; in breaststroke a mean CV of ∼0.5% and ∆ = −0.2%; in backstroke a mean CV of ∼0.5% and ∆ = −0.6%, and; in butterfly a mean CV of ∼0.7% and ∆ = −0.9%. For all strokes, it was a reduction of the underwater phase with the aim of increasing its speed. However, this result was not always transferred to the final performance. In any case, most of the swimmers tried to make improvements from the start of the race up to 15 m. Furthermore, the swimmers generated an overall increase in stroke rate as the rounds progressed. However, a decrease in stroke length resulted and, this balance appeared to be of little benefit to performance.
In the sport of swimming, race analysis, when combined with video sequences, provides crucial information in the development of swimmers’ performance (Gonjo and Olstad, 2021). Therefore, race performances are often analyzed during or after a championship and compared with those of other events to conduct changes in race strategy or technique for the enhancement of future events (Arellano et al., 1994; Marinho et al., 2009). In this sense, during major championships is required that swimmers qualify from the initial round (heats) to the following rounds (semi-finals and/or finals) (Tijani Jed et al., 2021; Cuenca-Fernández et al., 2021b), which means that individual performances may differ. In this regard, while the literature has provided sufficient information on the differences between strokes or distances (Morais et al., 2019; Gonjo and Olstad, 2021), or performance variability in middle- and long-distance swimming events (Hopkins et al., 1999; Skorski et al., 2013; Skorski et al., 2014), no attention has been paid to different strokes of the shorter sprint events (i.e., 50 m freestyle, breaststroke, backstroke and butterfly), probably due to only sprint freestyle is included in the Olympic swimming events list.
A widely held notion in international swimming is that progression between rounds is necessary to ensure that a swimmer qualifies from the heats to the semi-finals and then to the final, when medals are decided (Mujika et al., 2019). For instance, swimmers who participated at the 2004 Athens Olympics were 0.58% slower compared to their qualifying times (Issurin et al., 2008); however, medallists and finalists were able to progress between rounds by 0.35 and 0.12%, respectively. On this variability in performance, known as the intra-athlete coefficient of variation (CV), it has previously been reported that in closely matched competitions where swimmers strive to win a medal or reach a final, they must improve their performance by at least ∼0.5% for that change to have an impact on performance (Stewart and Hopkins, 2000; Trewin et al., 2004). In this regard, a CV of ∼0.5 and −0.6% was observed in United States and Australian Olympic swimmers in 50 and 100 m freestyle, respectively (Pyne et al., 2004). Thus, considering the evolution and all the rules’ modifications in the last 15 years, it is necessary to know whether these variations would occur nowadays in a sample of international swimmers. If so, this raises the question of where do swimmers manage such changes over the race?
In short-duration sports, such as the 50 m swimming, an all-out strategy is often employed (Abbiss and Laursen, 2008; McGibbon et al., 2018; Morais et al., 2021); despite the short duration, fatigue evoke a decrease in swim speed throughout the race (Morais et al., 2021). In this regard, planning and executing a proper race strategy is a key factor to excel in competitive swimming (Morais et al., 2019). It was recently shown that during the European Swimming Championships 2021, swimmers competing in the 100 and 200 m events progressed in their performance from round to round by increasing performance in the first key-moments of the race (Cuenca-Fernández et al., 2021b), indicating that the fastest swimmers did not perform at their best from the very beginning until they were trying to reach the final or win a medal. This strategy was suggested as a possible way to save energy that could allow swimmers to excel when needed (Stewart and Hopkins, 2000; (Cuenca-Fernández et al., 2021b). Indeed, achieving high performance in competitive swimming requires striking a fine balance between stability and variability of performance because, although swimmers need to achieve consistent results, they also need to be able to successfully adapting their stroke parameters to changes in the performance environment (such as the level of the other contenders) (Simbaña-Escobar et al., 2018). Therefore, although the strategy during the 50 m has previously been indicated as a rapid acceleration at the start followed by a progressive reduction in swim speed throughout the race (McGibbon et al., 2018; Morais et al., 2021), it is unknown whether this strategy happens in all rounds (e.g., even during the heats).
Swimming is a cyclic sport, yet its performance should not be conceived as a whole, but as a series of different segments that make up the race and that depend on different biomechanical and physiological adaptations (Hay et al., 1983; Marinho et al., 2009). The start, the clean swim, and the finish are the three main segments that make up the 50 m race (Gonjo and Olstad, 2021). However, such analysis can be even more detailed. E.g., the lap time can be divided into sub-sections including the split times, the time from 25 to 50 m (Morais et al., 2021), and the underwater phase. Furthermore, considering that the velocity of swimming is determined by the interplay between the stroke rate and the stroke length (Wakayoshi et al., 1995), the analysis of these stroke patterns may provide additional insights into the final results (Sánchez, Arellano, and Cuenca-Fernández, 2021). On the other hand, given that the best swimmers would be trying to perform at their best during the finals compared to the early rounds of competition, these variables could entail intentional modifications between rounds aimed to progress in performance. Therefore, analysis of each of these segments could provide further information on how swimmers are able to improve their performance throughout the rounds, i.e., progression within competition, in the four different swimming strokes. For that reason, this study aimed to: 1) study the coefficient of variation (CV) and performance progress (%∆) in total time (i.e., T50) in the four different swimming strokes, and; 2) specifically analyze which of the race segments and stroke variables are most modified to achieve improvement across the rounds. It was hypothesized that performance would improve over the rounds, and that these changes would be a consequence of the improvement in the performance variables corresponding to the different segments of the race.
European swimmers who competed in 50 m individual events at the 2021 Budapest European championships were the focus of this study. As some swimmers competed in more than one event, a total of 322 performances including 56 males (23.78 ± 3.25 years) and 60 females (24.66 ± 4.12 years) were analyzed. Data were gathered from the finalists (eight finalists x three rounds (i.e., heats, semi-final, and final) x four strokes (i.e., butterfly, backstroke, breaststroke, and freestyle) x two sexes (i.e., male and female)), and semi-finalists (16 semifinalists x two rounds (i.e., heats, semi-final) x four strokes (i.e., butterfly, backstroke, breaststroke, and freestyle) x two sexes (i.e., male and female). In one of the 50 butterfly semi-final there was a last-minute withdrawal, but there were two reserves who did not make the tiebreaker, thus, there were nine semifinalists.
Swimmers’ information and the official race times were retrieved from the official publicly available Budapest 2021 European Championships swimming website (http://len.eu). As this study was a retrospective analysis of publicly available data, without any experimental intervention, informed consent and ethical approval from the local committee was not required.
For each event, the results and changes in performance during the three rounds (i.e., heats, semi-finals, and final) were collected to analyse the process of sports performance. A Web Scraping routine in Python® was implemented to obtain the official data. The information was then checked by two independent researchers. To accomplish the first aim, the following variables were calculated using the final times:
- The intra-athlete CV: which represents the random variation in performance between rounds (Hopkins et al., 1999). Three different intra-athlete CVs were obtained: 1) between heats and semi-finals (H-SF); 2) between semi-finals and finals (SF-F), and; 3) between heats and finals (H-F), including all three rounds, total times and performance variables. The CV was calculated using the following equation:
- Relative change (%∆) in performance variables was calculated between rounds using the following equation:
where, Round 2 performance refers to the race time achieved on the second round and Round 1 performance refers to the race time achieved on the previous round. The criterion for performance progression, no change, or regression was %∆ being lower, equal, or higher than 0, respectively (Mujika et al., 2019).
The performance variables were obtained through indirect photogrammetric methodology, analysing the videos of the swimmer’s performance. This is an indispensable strategy and a major tool for coaches, analysts and researchers to collect qualitative and quantitative data (Smith et al., 2002; O’Donoghue, 2006). All the videos analysed were provided by the championship organisation. A set of 10 pan-tilt-zoom cameras, one for each lane, tracked the swimmer during the race. The video setup included fullHD cameras (1920 × 1,080 pixels resolution, f = 50 Hz Each lane (for each swimmer) had a pan-tilt-zoom camera (Panasonic HC-X1,000 Hybrid O.I.S 4K) tracking the swimmers. Hence, each camera (one per lane) followed along the swimming pool back and forth each swimmer. A calibration zone was defined using the red buoys of the pool lane as a reference (i.e., a distance of 5 m) to correct for the effect of camera position and perspective (Figure 1). A detailed description of the scaling procedures and the calculation of the measurement accuracy can be found in one of the Supplementary Material documents. The starting lights, which were visible from all the cameras, were used to synchronized the official timer with the time-stamp on the race analysis (Morais et al., 2019). The swimmer’s data was obtained after detailed observations by four evaluators through in-house customized software for performance analysis. The Intra-class Correlation Coefficient (ICC) was computed to verify the agreement among evaluators (n = 4). This ranged between 0.989 and 0.999, showing high agreement.
FIGURE 1. Basic graphical description of the procedure for measuring the swimmer’s entry distance into the water after the start. Similar procedures were used to measure emersion distance.
The following variables were measured: Start variables: 1) Reaction time: Defined as the time in seconds (s) from the starting signal until the swimmer moves into the block. Taken from the official results. 2) Flight time: Defined as the time in seconds (s) from when the swimmer leaves the block until the hand touches the water after the start. 3) Entry distance: Defined as the distance in meters (m) between the block wall and the point where the hand touches the water. 4) Underwater time (Und Time): The time in seconds (s) from when the swimmer hand’s touch the water until the swimmer’s head comes out of the water, or if this is not appreciable, when the hands meet at the midpoint of the first stroke. 5) Underwater distance (Und Distance): The distance in meters (m) covered during the underwater phase defined previously. 6) Underwater speed (Und Speed): Obtained by dividing the underwater distance by the time to cover it (m·s−1).
Race segments variables: Time 15–50 m (T15 to T50): Defined as the time in seconds (s), from the starting signal, until the swimmer’s head crosses the 1) 15, 2) 25, 2) 35, 4) 45 and 5) 50 m mark (the last one was obtained from the official competition results). 6) Finish time: Defined as the time in seconds (s), from 45 to 50 m. 7) Split25-50 m: Defined as the time in seconds (s), elapsed from 25 to 50 m.
Stroking variables (1,2) Stroke rate (SR): Collected at 15–25 and 35–45 m mark, were obtained using frequency measuring function for each 3 arm strokes and divided by the time elapsed during this action (to obtain the rate in Hertz), and multiplied by 60 (to obtain the rate in cycles/min), 3) final SR: Collected at 45–50 m mark, were obtained using frequency measuring function for each 2 arm strokes and divided by the time elapsed during this action (to obtain the rate in Hertz), and multiplied by 60 (to obtain the rate in cycles/min) (4, 5) average Stroke length (aSL): Collected at 15–25 and 35–45 m mark, were obtained by dividing the mean speed by the mean SR (in Hertz) (to obtain the length in meters/cycle), 6) final SL: Collected at 45–50 m mark, were obtained by dividing the mean speed by the mean SR (in Hertz) (to obtain the length in meters/cycle). The selected variables are noted by the literature on regular basis (Arellano et al., 1994; Mason and Cossor, 2000; Veiga et al., 2014; Morais et al., 2019; Gonjo and Olstad, 2021; Sánchez et al., 2021).
The Shapiro-wilk and Levene test were used to verify the normality and homoscedasticity of the data, respectively. All analyses were conducted differentially by sex (Shapiro et al., 2021). Linear mixed-effects models were applied between rounds (e.g., heats, semi-finals, and final), for all swimmers and performance variables to estimate means (fixed effects) and within-swimmer variations (random effects, modelled as variances) in accordance with Equation 1, as explained in previous studies (Stewart and Hopkins, 2000; Pyne et al., 2004). The fixed main effects were event (50 m freestyle, breaststroke, backstroke and butterfly), performance variables (i.e., the ones presented in Table 1) and rounds (e.g., heats, semi-finals, and final). The performance variables between rounds were compared with repeated-measures ANOVA and the differences between pairs of rounds (e.g., SF to F) were verified with Bonferroni post-hoc test. The effect sizes (η2) of the obtained variances were calculated and categorized (small = 0.01; medium = 0.06; large = 0.14). Pearson product-moment correlation coefficients (r) between all variables and times performances at 15, 25 and 50 m were obtained and interpreted as follows: 0.1 (low), 0.3 (moderate), 0.5 (large), 0.7 (very high) and 0.9 (nearly perfect) (Hopkins et al., 2009). Simple linear regression analyses were applied to evaluate the associations. All the statistical analyses were conducted in SPSS 24.0 (IBM, Chicago, IL, United States ) with significance level set at p < 0.05.
TABLE 1. Freestyle performance variables’ results, p values, and effect sizes (η2) between the different three rounds. Men (M); Women (W) (LEN European Senior Championships 2021).
Mean and Standard Deviations (SD) were obtained for all the variables and presented in conjunction with the result of the ANOVA test in Tables 1 to 4, the results for one stroke and both sexes are described in each table.
TABLE 2. Backstroke performance variables’ results, p values, and effect sizes (η2) between the different three rounds. Men (M); Women (W) (LEN European Senior Championships 2021).
TABLE 3. Breaststroke performance variables’ results, p values, and effect sizes (η2) between the different three rounds. Men (M); Women (W) (LEN European Senior Championships 2021).
TABLE 4. Butterfly performance variables’ results, p values, and effect sizes (η2) between the different three rounds. Men (M); Women (W) (LEN European Senior Championships 2021).
The values obtained of the linear mixed-effects model analyses, intra-subject CVs and ∆% progression are presented in Tables 5 to 8. A significant progression of performance was observed in most of the events over the rounds (i.e., from heats to semi-finals and then finals). The largest CV and ∆ was noted in butterfly events (CV∼0.7%; ∆ = −0.9%), followed-up by freestyle (CV∼0.6%; ∆∼0.7%), backstroke (CV∼0.5%; ∆ = −0.6%) and breaststroke (CV∼0.5%; ∆ = −0.2%). The CV changed in several key moments related to the start underwater variables. However, it is unclear which variable (distance or time) had a larger partial contribution to underwater speed.
TABLE 5. Freestyle intra-athletes’ coefficient of variation (CV) and relative change in performance (%∆). Men (M); Women (W); Heat (H); Semi-final (SF); Final (F) (LEN European Senior Championships 2021).
TABLE 6. Backstroke intra-athlete’s coefficient of variation (CV) and relative change in performance (%∆). Men (M); Women (W); Heat (H); Semi-final (SF); Final (F) (LEN European Senior Championships 2021).
TABLE 7. Breaststroke intra-athlete’s coefficient of variation (CV) and relative change in performance (%∆). Men (M); Women (W); Heat (H); Semi-final (SF); Final (F) (LEN European Senior Championships 2021).
TABLE 8. Butterfly intra-athlete’s coefficient of variation (CV) and relative change in performance (%∆). Men (M); Women (W); Heat (H); Semi-final (SF); Final (F) (LEN European Senior Championships 2021).
Correlation analyses between the different variables studied and T15, T25 and T50 on each sex group, stroke and differentiating the rounds are presented in Tables 9 to 12. In most events the correlation between T15 and T25, and between T25 and T50 was very large, however, the correlation between T15 and T50 was moderate or only large for the finalists. So, the improvements in the start and underwater segments of the race abovementioned did not have a strong impact on the final race time (i.e., T50). SR15-25 m and SR35-45 m increased over the competitions (freestyle and butterfly in both sexes, breaststroke and backstroke in men). Meanwhile, the SL was prone to decrease most of the times, trading off with the faster SR.
TABLE 9. Pearson correlation coefficients (r) of the 50 m Freestyle’s competition variables (LEN European Senior Championships 2021).
TABLE 10. Pearson correlation coefficients (r) of the 50 m Backstroke’s competition variables (LEN European Senior Championships 2021).
TABLE 11. Pearson correlation coefficients (r) of the 50 m Breaststroke’s competition variables (LEN European Senior Championships 2021).
TABLE 12. Pearson correlation coefficients (r) of the 50 m Butterfly’s competition variables (LEN European Senior Championships 2021).
The regression analysis for each variable and stroke are presented as Supplementary Material. Additionally, the final time achieved by the medallists in the different rounds (i.e., T50) was plotted against the performances achieved by the finalists, semi-finalists and rest of participants and presented as supplementary material (Supplementary Material).
The first aim of this research was to study the coefficient of variation (CV) and the progression of performance (%∆) in the 50 m event among swimmers who participated in different rounds of the same championship. It was hypothesised that if faster swimmers took the heats slower, a change in performance over the rounds would be detected. The results of the performances achieved during the finals compared to the heats showed that the best swimmers did not excel during the heats, as a significant progression of performance was observed in most of the strokes as the competition progressed. However, when comparing the performances in the final with those in the semi-finals, the progressions of performances in some strokes were poorer or not significant, due to the better performances achieved during the semi-final.
With reference to the 50 m freestyle, there were differences in CV between performances obtained in the finals and semi-finals compared to the heats (Table 5). These CV changes entailed a progressive reduction in the T50 as swimmers progressed between rounds. However, the performance achieved by the men during the final was worse compared to the semi-final (Table 1). Possibly, this failure could be the result of ineffective planning, or the swimmers’ inability to perform at their best under the pressure of international competition (Mujika et al., 2019), but also, it is likely that as the level of the contenders was quite even, many of them tried to perform really well in the semi-final to avoid being left out of the final. In breaststroke, only women obtained differences in T50 between performances obtained in the finals compared to the heats (Table 3). In men, although the CV represented changes in performance (Table 7), it appears that some contenders had performance deteriorations during the final, resulting in a mean ∆ = 0.2%. In any case, it is worth mentioning that, although their CV change was not positive for performance, some managed to reach medal positions, which means that this deterioration came from the difference result after having performed extraordinarily well during the heats. For further information on the performance of the medallists in comparison to the other contenders, it is recommended to consult supplementary material (Supplementary Material).
In the 50 m backstroke, the men showed differences in T50 CV between performances obtained in the finals compared to the heats (Table 6), without differences in women. For the men, these changes in CV meant a progressive reduction in T50 as swimmers progressed between rounds; however, the women’s time performances were better in the semi-final than in the rest of the rounds (Table 3). Therefore, the best male swimmers either did not excel during the heats and/or were able to obtain progressions in performance as the competition progressed. In this sense, it is important to mention that apart from the fact that the level of the finalists was quite similar, the world record in this event was broken in the final, so this influenced the results obtained. Finally, in the men’s 50 butterfly there were differences in the CV T50 for both men and women between the performances obtained in the finals and semi-finals compared to the heats (Table 8). These changes in CV meant a progressive reduction in T50 as the swimmers progressed between rounds, with the exception of the performance achieved by the men during the finals, which was the same as that achieved during the semi-finals (Table 4). Therefore, although the men and women did not excel during the heats, possibly the men were not able to achieve further performance progressions as the competition progressed because performance in the semi-finals was already really of high-level.
On the other hand, this study aimed to specifically analyse which of the key moments of the race or its subfactors are most modified to achieve improvement across the rounds. It was hypothesized that these changes would be a consequence of the improvement in the performance variables of the initial segment. This hypothesis was only partially confirmed as for some races the improvement came in the variables collected at the final stages of the race.
In sprint swimming, improving the start could make the difference between winning or not get a medal (García-Hermoso et al., 2017; Arellano et al., 2018; Sánchez et al., 2021). Therefore, several investigations have shown that swimmers should optimise the force-time distribution during the impulse phase (de Jesus et al., 2014; Vantorre et al., 2014; Cuenca-Fernández et al., 2015). Despite swimming start speed was not calculated, a good start is understood as an increase in speed since the swimmer leaves the block and reach the water could be achieved by either a combination of a reduction in execution time and an increase in distance of entry or a combination of both (Vantorre et al., 2014). Therefore, a good start cannot simply be explained by a single parameter (Gonjo and Olstad, 2020).
A change in flight time CV with a corresponding ∆% reduction (Table 5) was a common factor in both men and women progressing between heats and the final (Table 1). It appears that swimmers during the final intentionally tried to get to the water fast rather than trying to increase the hand’s entry distance. According to other authors (Kilani and Zeidan, 2004; Simbaña-Escobar et al., 2018; Morais et al., 2021), the best freestyle swimmers are especially faster in the start sections; however, a shorter flight time obtained a low magnitude on the correlations with any performance variable (i.e., T15, T25 and T50) (Table 9). In addition, during heats and semi-finals, men who achieved a longer entry distance obtained better performance results, while a slight increase was observed in women in semi-finals and finals compared to heats (Table 1). Therefore, it cannot be ruled out that both a reduction in flight time and an increase in entry distance can be modified by the swimmers to influence the speed of the start.
The reaction time did not differ between rounds, although the male finalists showed a significant reduction in flight time together with a non-significant increase in distance compared to previous rounds, which could be translated into an increase in speed (Table 2). On the contrary, in the women, this combination yielded worse results than those obtained in previous rounds. A previous study has shown that men react faster to an auditory stimulus when large muscle groups are involved (Spierer et al., 2010). In this study, the reaction time of men and women was similar, however, this yielded different results. In men, the best performers were those with a slower reaction time, but also those who combined a shorter flight time and a longer entry distance, attaining large to very high correlations (Table 10), which seems to be an indicative of a higher impulse achieved at the start (García-Hermoso et al., 2017). In contrast, the women with a slower reaction time seemed to achieve worse performances at T15 and T25, so for them this did not lead to a higher impulse at the start (Table 10). These differences could be explained by sex, as absolute leg power is higher in men than in women (García-Hermoso et al., 2017; Simbaña-Escobar et al., 2018).
Changes in start variables were not significant for either men or women (Table 7) and no decreasing or increasing trends were observed between rounds as the competition progressed to the final (Table 3). It is important to mention that the men did not obtain overall performance progressions in the final time (T50). However, apparently the women also did not vary the swim start variables as they progressed between rounds. Therefore, it is possible that the modifications in breaststroke come from other variations occurring in the underwater phase (Olstad et al., 2020; Sánchez et al., 2021).
Both men and women produced no variation in performance in any of the start variables to progress between rounds (Table 8). According to a previous study (Kilani and Zeidan, 2004), the swim start was a differentiating factor between finalists and semi-finalists in 50 m butterfly success. However, the large variations in performance were possibly caused by other variables rather than by the actions taken on the block. By the large magnitude of the correlations, those men who achieved a longer entry distance in the semi-finals were the ones who performed better in T15 and T25 (Table 12).
In previous studies, the underwater phase has been divided into two parts: the glide and the undulatory swim, differentiated by the moment at which the movement of the lower limbs begins (de Jesus et al., 2014; Vantorre et al., 2014). However, a limitation of current methods of competition analysis is that the camera setup is limited to the above-water view only, which means that underwater kinematic information cannot be assessed in detail (Gonjo and Olstad, 2021). In any case, the underwater swim during the start and turn segments must be adjusted to maximise average speed (Veiga et al., 2014), which means that good underwater performances cannot simply be explained by a single parameter (e.g., only underwater distance) (Sánchez et al., 2021).
The male finalists showed CV changes in underwater time and distance that represented a significant ∆% reduction (Table 5). However, the underwater speed of the final did not prove to be superior as a result (Table 1). In this regard, a previous study reported that a long underwater distance is not necessarily related to a fast finish time and suggested that some fast swimmers (as seen during this championship) might prioritise breaking the water quickly to maximise average forward speed (Veiga and Roig, 2016). However, those who achieved higher underwater speeds did not obtain correlations with race times (Table 9), questioning the current paradigm on the best approach to take to the underwater phase of the 50 m freestyle. Only the female finalists showed significant changes in their CV in the final compared to the previous rounds that involved increases in underwater speed (Table 1). As with the men, different profiles were observed with swimmers attempting to reduce distance underwater causing a loss of speed, and others gaining an increase in speed as a result of that reduction. Therefore, it seems that swimmers attempted different manners to increase such speed in order to improve final performance (Table 5).
There was a significant CV in the men between the final and semi-final which showed that the finalists reduced the time and distance of the underwater swim during the final to gain speed in the first few metres of the event, although these improvements were only significant when compared to the heats (Table 6). It has been reported that, in backstroke sprint events, swimmers move faster when performing dolphin kicks than swimming on the surface (Collard, 2007). In some cases (i.e., men in the semi-finals and women in the heats), swimmers with higher underwater distances obtained large correlations with T15 (Table 10); however, swimmers with superior underwater speeds were the best performers at T15 and T25 in most rounds. This is consistent with other research where maximising underwater speed was more important than displacing a long distance underwater (Gonjo and Olstad, 2021). In the women, no significant CVs were obtained for any of the underwater variables (Table 6).
Significant CV changes were obtained for underwater time and distance in the male finalists, indicating that during the final there was a ∆% reduction compared to the heats (Table 7). However, if this reduction was made with the aim of generating an increase in underwater speed, this was not the case (Table 3), with many swimmers demonstrating very different strategies from each other, as can be seen in the high SD obtained in the ∆% for this variable. A previous study carried out in short pool showed that, in men, a long underwater distance was related to a better final time (Sánchez et al., 2021); in this study, the same relationship was only found in T15, and only during the heats. In the case of the women, no significant changes were generated between rounds in any of the underwater time and distance variables; however, an increase on the underwater speed was detected in the semi-final. Actually, it appears that a short underwater time benefited performance at T25 during the semi-finals, but these relations were only moderate and did not translate to T50 (Table 11). Therefore, although a possible influence was plausible, the changes that occurred in T50 likely came from changes in other variables. A similar result was obtained previously (Olstad et al., 2020; Sánchez et al., 2021) since no correlations were obtained between the variables of emersion time with final time, and no differences were obtained between finalists and non-finalists.
Only in men, there was a reduction in underwater distance during the final (Table 8). Interestingly, those men and women who achieved a greater underwater distance achieved better results in T15 and T25, with very high correlations only during the heats, but only the men who reached a greater underwater speed achieved better results in T15 and T25 only during the final. According to Gonjo and Olstad (2020), average forward speed during the underwater phase is highly correlated with T15. In our study, the finalists obtained the same correlation also for T25 (Table 12), so possibly a reduced underwater phase was adopted during the final with the aim of gaining speed, although it was not effective for all swimmers.
For the start time at 15 m and the finish segment, there is a lack of knowledge in the sprint events in the long course (Gonjo and Olstad, 2021; Morais et al., 2021), even more so if what is studied is how these variables change over the different rounds.
In men, no significant CV was obtained in T15 as the time performances were similar between rounds (Table 1). According to other studies (Trinidad et al., 2020; Morais et al., 2021), in the comparison between faster and slower swimmers in 50 m freestyle, the largest differences are observed in T15. However, while T15 was the main predictor of T25 performance for both men and women, with very high to nearly perfect correlations, this variable did not affect T50 in the case of men (Table 9), possibly due to the different profiles found in the underwater phase, and the fact that some of the swimmers were able to progress even in the face of disadvantageous starts (or vice versa, fade after advantageous starts). The women showed changes in CV in T15, which led to improvements in performance in the semi-finals and final compared to the heats (Table 1).
With the exception of T25, the men did not obtain significant CV changes for the 35 and 45 m mark, as performances during the semi-finals were better than achieved in the finals. For the same variables, the women obtained changes in CV corresponding to ∆% reductions in swim time as the race progressed, especially between the semi-final and final compared to the heats (Table 5). In this case, it appears that improvements in T15 not only influenced final performance, but also that those with excellent performances early in the race were difficult to beat by other contenders in the middle of the 50 m-lap (Simbaña-Escobar et al., 2018). Therefore, in the case of the women, it was much more relevant a good development in the early stages of the race (15 and 25 m) to improve the final time obtained in the previous rounds.
For the Split25-50 m and finish time, there were CV changes and ∆% reductions in the men in the final and semi-finals compared to the heats (Table 5), so it is possible that regardless of the improvements obtained in the first metres of the event, some swimmers had the ability to avoid a sharp decrease in speed at the end (Simbaña-Escobar et al., 2018; Morais et al., 2021). In the case of the women, these variables did not improve as the competition progressed.
The men increased the speed of swimming between rounds since a significant CV change was obtained in all time variables in the comparison of the time of the final and heats and in most of the comparisons between the final and the semi-finals (Table 6). In the case of females, it appeared to be performance improvements during the semi-finals; however, the expected improvements were not obtained during the final (Table 3). The variable T15 obtained a very high correlation T25 performance in most cases but did not predict T50. In the case of T25 this variable appears not to be valid to predict T50 performance during the Final.
On the other hand, both men and women obtained CV changes in the finish time, with better performance during the semi-final than during the heats, so, in terms of swimming strategy, increasing the pace in the first split of the race (15 or 25 m) seemed to be a determining factor to reduce the final time, especially in men, as improvements here translated to final performance and neither the pace of the 25–50 split, nor the finish Time, had an influence on the worsening of these results. That said, lower Split25-50 and finish times obviously benefited better T50 performances (Table 10).
For both men and women, there were no changes in CV at T15 in the different rounds (Table 7), so the changes made in the previous underwater phase had no relevant effect on performance. Similarly, T15 was shown to predict at 25, but not T50. In other study (Sánchez et al., 2021), male and female 50 m breaststroke finalists had better T15 m values during finals compared to heats (p < 0.05), and these values were related to better final performance (r > 0.6); however, the participants in that study were national level swimmers and the relationships might be different among higher level contenders (i.e., international championship finalists and semi-finalists) (Hellard et al., 2008).
For T25, T35, and T45, in the men, CV changes were only significant in the semi-finals, as time performances appeared to be better than those achieved during the final (Table 7), confirming the fact that the winner and/or medallists may not always be the fastest of the tournament (Supplementary Material). On the contrary, women showed CV changes and ∆% reductions to progress between rounds, especially significant between the final and the heats (Table 3). Thus, performance changes in the women occurred mainly during the clean swim splits (T25, T35, and T45).
There were no variations or reductions in performance in the variables Split25-50 m and finish time, meaning that possibly the swimmers acquired high speed in the first stage of the race and found it very difficult to continue progressing in performance as the race proceeds.
Despite no improvement in the underwater phase, T15 m improved in men and almost in women (p = 0.054) to progress between rounds. In fact, the CV of T25, T35 and T45 changed in men and women in the finals, and especially in women in most of the semi-finalists (Table 8). These changes showed reductions in ∆% between rounds. It seems that starting the race at high speed to reduce the time to 15 m was more determinant for the women than the men to achieve better performance in T25 and T50. In this regard, Kilani and Zeidan (2004), reported that the first split of the race, including the swim start, was more determinant than the second to achieve a great result. In any case, both men and women who progressed between rounds to the final showed changes in Split25-50 CV, with significant reductions ∆% especially during the semi-finals (Table 4), indicating that they were able to improve performance both at the beginning and at the end of the race.
Changes in stroke patterns have been interpreted as a strategy used by swimmers to cope with performance changes within a race (Seifert et al., 2005; Hellard et al., 2008). Stroke rate is related to neuromuscular power and energetic capacities (Wakayoshi et al., 1995), whereas stroke length depends more on technical skill resulting from the increased propulsive force generated by the arms and legs (Seifert et al., 2005). The literature, in middle-distance swimming, has reported that high-level swimmers have a higher stroke rate and length than low-level swimmers (Hellard et al., 2008). However, evidence in sprint swimming showed that swimming speed, stroke rate and stroke length are not linearly related (Craig and Pendergast, 1979; Wakayoshi et al., 1995).
Although changes in CV were not always statistically significant, an overall increase in SR15-25 and SR35-45 appeared to be determinant for those men and women who progressed between rounds (Table 5). A high SR helps to maintain a high swim speed between stroke cycles and to overcome drag (Barbosa et al., 2010; Ribeiro et al., 2017; Simbaña-Escobar et al., 2018). Within the race, the values of this variable decreased progressively from 15–25 to 35–45 m, possibly as a consequence of fatigue, as reported previously (Cuenca-Fernández et al., 2021a; Morais et al., 2021). In the case of the male finalists, a significant CV change (higher SR) was observed in the last metres (Table 1), which would be consistent with the CV and ∆% results obtained for Split25-50 m and finish time. For the women, CV changes showed increases in SR in the second half of the event (i.e., from 35 to 45 m), to move into the semi-finals and final (Table 5). In terms of SL, CV changes accounted for ∆% reductions for both men and women between rounds. This was in agreement with Maglischo (2003), who stated that “when swimmers want to go faster, they increase their SR, although their SL decreases”. While the swimmers during the final showed higher SL values from 15 to 25 m compared to the previous rounds (Table 1), in most cases, the values at 35–45 m were higher than at 15–25 m, presumably as a consequence of the decrease of SR. According to some studies (Kilani and Zeidan, 2004; Arellano et al., 2018; Simbaña-Escobar et al., 2018; Morais et al., 2021), SL is one of the main factors responsible for the difference in swim speed in 50 m freestyle. In this sense, a higher SL could reflect a greater ability to transfer the propulsive thrust to the water (Cuenca-Fernández et al., 2020; Ruiz-Navarro et al., 2020). However, men did not obtain any significant correlation, and those female finalists who showed a high SL at the end of the race obtained very high positive correlations with T50, attaining worse performances (Table 9). Therefore, it cannot be ruled out that swimmers who were able to increase their SR while maintaining or decreasing in a non-meaningful way SL, gained advantages in progressing between rounds in the sprint freestyle.
The CV differences showed that increases in SR between rounds were common in men (Table 6), but these changes were not a consistent pattern in all women. As observed for the other strokes, higher SR was accompanied by reductions in SL (Table 2). In any case, higher or lower SR and SL were not a determining factor for those who performed better, and the SR final was only noticeable for the female finalists at the end of the race, possibly because most of them did not significantly increase SR15-25 and SR35-45 as they progressed between rounds. It has been reported that backstroke often leads to lower SR values due to the longer duration of the propulsion and recovery phases (Gonjo et al., 2020). Compared to other strokes, less propulsive drag force is applied by the hands during the push phase due to the wrist moving backwards with respect to the swimming direction. Thus, this would imply that the contribution of the other body parts to propulsion, such as the lower limbs, is much greater and could therefore be much less detectable if progressing between rounds.
The men maintained similar SR values throughout the race, however, the CV showed the increase in ∆% SR between rounds (Table 7). Similarly, the women obtained significant CV reflecting that they were able to increase SR especially during the final, but only in them, relationships were observed with improved performance. Previous studies have denoted that high SR (above 60 cycles/min) and lower glide is necessary for success in breaststroke sprint swimming (Kilani and Zeidan, 2004; Strzala et al., 2013); however, as swimmers increased SR as they progressed between heats, it resulted in a reduction of the glide phase and thus SL, especially in men (Table 3). Therefore, within the swimmers who were able to progress between rounds, the SR increase could be a relevant factor as showed in the women; however, when the increase in SR induces a severe reduction in SL, a worsening of performance may occur as demonstrated in the men.
Both men and women obtained CV differences with clear trends towards increased SR during the final and semi-finals compared to the heats (Table 4). Sprint butterfly swimmers have been reported to achieve high speed with very high SR, often exceeding 60 cycles per minute, as demonstrated in previous European swimming championships (Strzała et al., 2017). Furthermore, in the study of Seifert et al. (2008), more skilled butterfly swimmers had higher SR and SL than less skilled swimmers. In our study, however, only SR showed certain relationships in men with T50 during the heats, while SL did not seem to predict final performance in any case, with low to moderate correlations (Table 12). Similar to what was obtained for other strokes, the increase in SR was possibly the main cause of the decreases in SL in the second part of the race (SL35-45), as in both men and women CV changes with ∆% performance reductions were obtained in the finals and semi-finals compared to the heats.
During the different rounds of the 50 m competitions, intra-individual performances varied in a significant range of 0.5–0.7%. With the exception of the men’s breaststroke, there were significant improvements in T50 as the competition progressed, meaning that the best swimmers did not excel during the heats to perform at their best during the final. For all strokes, apart from slight improvements in the actions performed in the block, it was a common tendency to reduce the underwater phase and increase SR with the aim of increasing speed. However, this result was not always obtained or was not adequately transferred to the final performance.
It is important to bear in mind that elite sports are often composed of “outliers” performances coming from athletes with different backgrounds and, therefore, trends will always be somewhat influenced by this. In addition, high achievements are also influenced by post-training factors that increase with years of practice and the level of expertise to know how to move from heats, to semi-finals and finals. Clearly, top swimmers who are able to gather those qualities, will improve their performance in major international competitions and their chances of winning a medal.
The original contributions presented in the study are included in the article/Supplementary Material, further inquiries can be directed to the corresponding author.
RA contributed conception and design of the study and critically discussed the results. General coordination of the working group and funded project responsible. Corresponding author. FC-F and JR-N contributed conception and design of the study, collected video data, wrote the first draft of the manuscript, timecoded the video footage and manuscript review after the first draft. Statistics data analysis. TB externally reviewed the design of the study, critically discussed the results and reviewed the draft documents. GL-C, EM-O, OL-B, and AG video data collection of study variables. AG-P video and web results Python web scrapping and exporting to Excel database. All authors contributed to manuscript revision, read, and approved the submitted version.
This study was supported by a grant awarded by the Ministry of Science, Innovation and Universities (Spanish Agency of Research) and the European Regional Development Fund (ERDF); PGC2018-102116-B-I00 “SWIM II: Specific Water Innovative Measurements: Applied to the performance improvement” and the Spanish Ministry of Education, Culture and Sport: FPU 19/02477, FPU 16/02629, and FPU17/02761 grants.
The authors declare that the research was conducted in the absence of any commercial or financial relationships that could be construed as a potential conflict of interest.
All claims expressed in this article are solely those of the authors and do not necessarily represent those of their affiliated organizations, or those of the publisher, the editors and the reviewers. Any product that may be evaluated in this article, or claim that may be made by its manufacturer, is not guaranteed or endorsed by the publisher.
To Royal Spanish Swimming Federation for supporting the Project: “Performance Evaluation of National Team Swimmers” in cooperation with Granada University, Granada, Spain. To LEN (Ligue Européenne de Natation, Technical Committe) to provide de video footage to participating teams thanks to the cooperation with British Swimming (responsible: Oliver Logan and Adrian Campbell) and Innolab de Tongelreep (responsible: Carlo van der Heijden) and the group of cameramen.
The Supplementary Material for this article can be found online at: https://www.frontiersin.org/articles/10.3389/fphys.2022.797367/full#supplementary-material
Abbiss C. R., Laursen P. B. (2008). Describing and Understanding Pacing Strategies during Athletic Competition. Sports Med. 38 (3), 239–252. doi:10.2165/00007256-200838030-00004
Arellano R., Brown P., Cappaert J., Nelson R. C. (1994). Analysis of 50-, 100-, and 200-m Freestyle Swimmers at the 1992 Olympic Games. J. Appl. Biomechanics 10 (2), 189–199. doi:10.1123/jab.10.2.189
Arellano R., Ruíz-Teba A., Morales-Ortíz E., Gay A., Cuenca-Fernandez F., Llorente-Ferrón F., et al. (2018). Short Course 50m Male Freestyle Performance Comparison between National and Regional Spanish Swimmers. ISBS Proc. Arch. 36 (1), 139.
Barbosa T., Silva A. J., Reis A. M., Costa M., Garrido N., Policarpo F., et al. (2010). Kinematical Changes in Swimming Front Crawl and Breaststroke with the AquaTrainer® Snorkel. Eur. J. Appl. Physiol. 109 (6), 1155–1162. doi:10.1007/s00421-010-1459-x
Collard L. (2007). Average Swimming Speeds for 6 Sections of the 50-meter Backstroke Race: Comparison of Performance in Zones Swum Underwater or at the Surface in 12 International Short Course Events. Int. J. Perform. Analysis Sport 7 (3), 37–45. doi:10.1080/24748668.2007.11868408
Craig A. B., Pendergast D. R. (1979). Relationships of Stroke Rate, Distance Per Stroke, and Velocity in Competitive Swimming. Med. Sci. Sports Exerc. 11 (3), 278–283.
Cuenca-Fernández F., Boullosa D., Ruiz-Navarro J. J., Gay A., Morales-Ortíz E., López-Contreras G., et al. (2021a). Lower Fatigue and Faster Recovery of Ultra-short Race Pace Swimming Training Sessions. Res. Sports Med. 2021, 1–14. doi:10.1080/15438627.2021.1929227
Cuenca-Fernández F., Ruiz-Navarro J. J., Arellano Colomina R. (2020). Strength-velocity Relationship of Resisted Swimming: A Regression Analysis. ISBS Proc. Arch. 38 (1), 99.
Cuenca-Fernández F., Ruiz-Navarro J. J., González-Ponce A., López-Belmonte Ó., Gay A., Arellano R. (2021b). Progression and Variation of Competitive 100 and 200m Performance at the 2021 European Swimming Championships. Sports Biomech., 1–16. doi:10.1080/14763141.2021.1998591
Cuenca-Fernández F., Taladriz S., López-Contreras G., De la Fuente B., Argüelles J., Arellano R. (2015). “Relative Force and PAP in Swimming,” in Paper Presented in the Proceedings of: The 33rd International Society of Biomechanics in Sports, July 3–29, 2015. (France: University of Poitiers).
de Jesus K., Fernandes R. J., Vilas-Boas J. P., Sanders R. (2014). The Backstroke Swimming Start: State of the Art. J. Hum. Kinet. 42, 27–40. doi:10.2478/hukin-2014-0058
García-Hermoso A., Saavedra J. M., Arellano R., Navarro F. (2017). Relationship between Swim Start Wall Contact Time and Final Performance in Backstroke Events in International Swimming Championships. Int. J. Perform. Analysis Sport 17 (3), 232–243. doi:10.1080/24748668.2017.1331573
Gonjo T., Olstad B. H. (2020). Start and Turn Performances of Competitive Swimmers in Sprint Butterfly Swimming. J. Sports Sci. Med. 19 (4), 727–734. PMID:33239947.
Gonjo T., Fernandes R. J., Vilas-Boas J. P., Sanders R. (2020). Upper Body Kinematic Differences between Maximum Front Crawl and Backstroke Swimming. J. Biomechanics 98, 109452. doi:10.1016/j.jbiomech.2019.109452
Gonjo T., Olstad B. H. (2021). Race Analysis in Competitive Swimming: A Narrative Review. Int. J. Environ. Res. Public Health 18 (1), 69. doi:10.3390/ijerph18010069
Hay J., Guimaraes A., Grimston S. (1983). A Quantitative Look at Swimming Biomechanics. Swim. Tech. 20 (2), 11–17.
Hellard P., Dekerle J., Avalos M., Caudal N., Knopp M., Hausswirth C. (2008). Kinematic Measures and Stroke Rate Variability in Elite Female 200-m Swimmers in the Four Swimming Techniques: Athens 2004 Olympic Semi-finalists and French National 2004 Championship Semi-finalists. J. Sports Sci. 26 (1), 35–46. doi:10.1080/02640410701332515
Hopkins W. G., Hawley J. A., Burke L. M. (1999). Design and Analysis of Research on Sport Performance Enhancement. Med. Sci. Sports Exerc. 31 (3), 472–485. doi:10.1097/00005768-199903000-00018
Hopkins W. G., Marshall S. W., Batterham A. M., Hanin J. (2009). Progressive Statistics for Studies in Sports Medicine and Exercise Science. Med. Sci. Sports Exerc. 41, 3–12. doi:10.1249/mss.0b013e31818cb278
Issurin V., Kaufman L., Lustig G., Tenenbaum G. (2008). Factors Affecting Peak Performance in the Swimming Competition of the Athens Olympic Games. J. Sports Med. Phys. Fit. 48 (1), 1–8. PMID:18212703.
Kilani H., Zeidan W. (2004). “A Kinematc Comparison between the Semi-finals and the Finals for 50m Swimming Races of the Four Strokes,” in 22th International Conference on Biomechanics in Sports (Ottawa: Springer), 125–128.
Marinho D. A., Garrido N., Barbosa T. M., Canelas R., Silva A. J., Costa A. M., et al. (2009). Monitoring Swimming Sprint Performance during a Training Cycle. J. Phys. Educ. Sport/Citius Altius Fortius 25 (4).
Mason B., Cossor J. (2000). “What Can We Learn from Competition Analysis at the 1999 Pan Pacific Swimming Championships?,” in Proceedings of XVIII Symposium on Biomechanics in Sports: Applied Program: Application of Biomechanical Study in Swimming Editors R. Sanders, and Y. Hong. (Hong Kong: Department of Sports Science and Physical Education the Chinese University of Hong Kong), 75–82.
McGibbon K. E., Pyne D. B., Shephard M. E., Thompson K. G. (2018). Pacing in Swimming: A Systematic Review. Sports Med. 48 (7), 1621–1633. doi:10.1007/s40279-018-0901-9
Mohamed T. J., Zied A., Francisco C.-F., Abderraouf B. A. (2021). Physiological, Perceptual Responses, and Strategy Differences in Age-Group Swimmers between Heats and Semi-finals in the 400 Metres Freestyle Event. Int. J. Perform. Analysis Sport 21, 953–964. doi:10.1080/24748668.2021.1963107
Morais J. E., Barbosa T. M., Silva A. J., Veiga S., Marinho D. A. (2021). Profiling of Elite Male Junior 50 M Freestyle Sprinters: Understanding the Speed‐time Relationship. Scand. J. Med. Sci. Sports 32, 60–68. doi:10.1111/sms.14058
Morais J. E., Marinho D. A., Arellano R., Barbosa T. M. (2019). Start and Turn Performances of Elite Sprinters at the 2016 European Championships in Swimming. Sports Biomech. 18 (1), 100–114. doi:10.1080/14763141.2018.1435713
Mujika I., Villanueva L., Welvaert M., Pyne D. B. (2019). Swimming Fast when it Counts: A 7-year Analysis of Olympic and World Championships Performance. Int. J. Sports Physiology Perform. 14 (8), 1132–1139. doi:10.1123/ijspp.2018-0782
O’Donoghue P. (2006). The Use of Feedback Videos in Sport. Int. J. Perform. Analysis Sport 6 (2), 1–14. doi:10.1080/24748668.2006.11868368
Olstad B. H., Wathne H., Gonjo T. (2020). Key Factors Related to Short Course 100 M Breaststroke Performance. Ijerph 17 (17), 6257. doi:10.3390/ijerph17176257
Pyne D. B., Trewin C. B., Hopkins W. G. (2004). Progression and Variability of Competitive Performance of Olympic Swimmers. J. Sports Sci. 22 (7), 613–620. doi:10.1080/02640410310001655822
Ribeiro J., Figueiredo P., Morais S., Alves F., Toussaint H., Vilas-Boas J. P., et al. (2017). Biomechanics, Energetics and Coordination during Extreme Swimming Intensity: Effect of Performance Level. J. Sports Sci. 35 (16), 1–8. doi:10.1080/02640414.2016.1227079
Ruiz-Navarro J. J., Morouço P. G., Arellano R. (2020). Relationship between Tethered Swimming in a Flume and Swimming Performance. Int. J. Sports Physiology Perform. 15 (8), 1087–1094. doi:10.1123/ijspp.2019-0466
Sánchez L., Arellano R., Cuenca-Fernández F. (2021). Analysis and Influence of the Underwater Phase of Breaststroke on Short-Course 50 and 100m Performance. Int. J. Perform. Analysis Sport 21, 307–323. doi:10.1080/24748668.2021.1885838
Seifert L., Boulesteix L., Carter M., Chollet D. (2005). The Spatial-Temporal and Coordinative Structures in Elite Male 100-m Front Crawl Swimmers. Int. J. Sports Med. 26 (04), 286–293. doi:10.1055/s-2004-821010
Seifert L., Boulesteix L., Chollet D., Vilas-Boas J. P. (2008). Differences in Spatial-Temporal Parameters and Arm-Leg Coordination in Butterfly Stroke as a Function of Race Pace, Skill and Gender. Hum. Mov. Sci. 27 (1), 96–111. doi:10.1016/j.humov.2007.08.001
Shapiro J. R., Klein S. L., Morgan R. (2021). Stop 'controlling' for Sex and Gender in Global Health Research. BMJ Glob. Health 6 (4), e005714. doi:10.1136/bmjgh-2021-005714
Simbaña-Escobar D., Hellard P., Seifert L. (2018). Modelling Stroking Parameters in Competitive Sprint Swimming: Understanding Inter- and Intra-lap Variability to Assess Pacing Management. Hum. Mov. Sci. 61, 219–230. doi:10.1016/j.humov.2018.08.002
Skorski S., Faude O., Abbiss C. R., Caviezel S., Wengert N., Meyer T. (2014). Influence of Pacing Manipulation on Performance of Juniors in Simulated 400-m Swim Competition. Int. J. Sports Physiology Perform. 9 (5), 817–824. doi:10.1123/ijspp.2013-0469
Skorski S., Faude O., Rausch K., Meyer T. (2013). Reproducibility of Pacing Profiles in Competitive Swimmers. Int. J. Sports Med. 34 (2), 152–157. doi:10.1055/s-0032-1316357
Smith D. J., Norris S. R., Hogg J. M. (2002). Performance Evaluation of Swimmers. Sports Med. 32 (9), 539–554. doi:10.2165/00007256-200232090-00001
Spierer D. K., Petersen R. A., Duffy K., Corcoran B. M., Rawls-Martin T. (2010). Gender Influence on Response Time to Sensory Stimuli. J. Strength Cond. Res. 24 (4), 957–963. doi:10.1519/JSC.0b013e3181c7c536
Stewart A. M., Hopkins W. G. (2000). Consistency of Swimming Performance within and between Competitions. Med. Sci. Sports Exerc. 32 (5), 997–1001. doi:10.1097/00005768-200005000-00018
Strzala M., Krezalek P., Glab G., Kaca M., Ostrowski A., Stanula A., et al. (2013). Intra-cyclic Phases of Arm-Leg Movement and Index of Coordination in Relation to Sprint Breaststroke Swimming in Young Swimmers. J. Sports Sci. Med. 12 (4), 690–697. PMID:24421728.
Strzała M., Stanula A., Krężałek P., Ostrowski A., Kaca M., Głąb G. (2017). Butterfly Sprint Swimming Technique, Analysis of Somatic and Spatial-Temporal Coordination Variables. J. Hum. Kinet. 60, 51–62. doi:10.1515/hukin-2017-0089
Trewin C. B., Hopkins W. G., Pyne D. B. (2004). Relationship between World-Ranking and Olympic Performance of Swimmers. J. Sports Sci. 22 (4), 339–345. doi:10.1080/02640410310001641610
Trinidad A., Veiga S., Navarro E., Lorenzo A. (2020). The Transition from Underwater to Surface Swimming during the Push-Off Start in Competitive Swimmers. J. Hum. Kinet. 72, 61–67. doi:10.2478/hukin-2019-0125
Vantorre J., Chollet D., Seifert L. (2014). Biomechanical Analysis of the Swim-Start: a Review. J. Sports Sci. Med. 13 (2), 223–231. PMID:24790473.
Veiga S., Cala A., Frutos P., Navarro E. (2014). Comparison of Starts and Turns of National and Regional Level Swimmers by Individualized-Distance Measurements. Sports Biomech. 13 (3), 285–295. doi:10.1080/14763141.2014.910265
Veiga S., Roig A. (2016). Underwater and Surface Strategies of 200 M World Level Swimmers. J. Sports Sci. 34 (8), 766–771. doi:10.1080/02640414.2015.1069382
Keywords: race analysis, sprint swimming, start, performance, technique, kinematic
Citation: Arellano R, Ruiz-Navarro JJ, Barbosa TM, López-Contreras G, Morales-Ortíz E, Gay A, López-Belmonte Ó, González-Ponce Á and Cuenca-Fernández F (2022) Are the 50 m Race Segments Changed From Heats to Finals at the 2021 European Swimming Championships?. Front. Physiol. 13:797367. doi: 10.3389/fphys.2022.797367
Received: 18 October 2021; Accepted: 13 June 2022;
Published: 13 July 2022.
Edited by:
Peter A. Federolf, University of Innsbruck, AustriaReviewed by:
Karla De Jesus, Federal University of Amazonas, BrazilCopyright © 2022 Arellano, Ruiz-Navarro, Barbosa, López-Contreras, Morales-Ortíz, Gay, López-Belmonte, González-Ponce and Cuenca-Fernández. This is an open-access article distributed under the terms of the Creative Commons Attribution License (CC BY). The use, distribution or reproduction in other forums is permitted, provided the original author(s) and the copyright owner(s) are credited and that the original publication in this journal is cited, in accordance with accepted academic practice. No use, distribution or reproduction is permitted which does not comply with these terms.
*Correspondence: Raúl Arellano, ci5hcmVsbGFub0B1Z3IuZXM=
†These authors have contributed equally to this work and share first authorship
‡These authors share senior authorship
Disclaimer: All claims expressed in this article are solely those of the authors and do not necessarily represent those of their affiliated organizations, or those of the publisher, the editors and the reviewers. Any product that may be evaluated in this article or claim that may be made by its manufacturer is not guaranteed or endorsed by the publisher.
Research integrity at Frontiers
Learn more about the work of our research integrity team to safeguard the quality of each article we publish.