- 1Cardiac Rehabilitation Center, Sapporo Ryokuai Hospital, Sapporo, Japan
- 2Department of Cardiovascular Medicine, Sapporo Ryokuai Hospital, Sapporo, Japan
Introduction: The period from ventilatory anaerobic threshold (VAT) to respiratory compensation point (RCP) during incremental exercise (isocapnic buffering phase) has been associated with exercise tolerance and skeletal muscle composition. However, several reports compare younger and older healthy adults, and specific age-related changes are unclear. This study aimed to examine the oxygen uptake (VO2) from VAT to RCP and its change over time in younger and older healthy adults.
Methods: A total of 126 consecutive participants were divided into two groups (95 younger and 31 older than 50 years of age) who underwent cardiopulmonary exercise testing, and VAT and RCP were determined. The ratio (RCP/VAT) and difference (ΔVO2 RCP-VAT) were calculated from the VO2 of VAT and RCP and compared between groups and ages. Statistical analyses included t-tests and Spearman’s correlation tests, and the significance level was set at <5%.
Results: RCP/VAT was not significantly different (1.40 ± 0.19 vs. 1.59 ± 0.24, p = 0.057) but weakly correlated with age (r = −0.229, p = 0.013, y = −0.0031x + 1.7588, lowering rate: 0.185%/year). Conversely, ΔVO2 RCP-VAT was significantly lower in the older group (7.7 ± 3.1 vs. 13.8 ± 4.9 ml/kg/min, p < 0.001) and correlated significantly with age (r = −0.499; p < 0.001; y = −0.1303x + 16.855; lowering rate, 0.914%/year).
Conclusion: ΔVO2 RCP-VAT was considered to be a poor indicator of lactate buffering capacity in the IB phase because both VAT and RCP were greatly affected by age-related decline. Conversely, RCP/VAT was suggested to be an index not easily affected by aging.
Introduction
In cardiac rehabilitation settings, exercise tolerance is generally assessed by cardiopulmonary exercise testing (CPET) using a combination of incremental exercise testing and expiratory gas analysis (Mann et al., 2013; Mezzani et al., 2013; JCS Joint Working Group, 2014; Price et al., 2016). The ventilatory anaerobic threshold (VAT) is the point at which carbon dioxide excretion (VCO2) increases in response to an increase in oxygen uptake (VO2) (Sullivan and Cobb, 1990; Nishijima et al., 2017, 2019). The respiratory compensatory point (RCP) is the point at which the exercise intensity increases and excessive carbon dioxide excretion by respiratory compensation begins due to accumulation of H+ ions (Balady et al., 2010). The RCP is partially dependent on the chemosensitivity of the carotid bodies and the accumulation of lactate during incremental exercise (Takano, 2000). Between the VAT and RCP is the isocapnic buffering phase (IB phase) in which the increased lactate is buffered by bicarbonate (Beaver et al., 1986a; Wasserman et al., 2012; Yen et al., 2015).
During the IB phase, oxygen uptake increases relative to ventilation (VE/VO2); however, carbon dioxide excretion does not (VE/VCO2). The IB phase is a period of lactate buffering activity because the lactate produced by exercise is buffered and utilized in vivo; it is therefore thought to be related to the lactate steady state without rapid acidosis (Dekerle et al., 2003; Keir et al., 2018; Iannetta et al., 2019). In athletes, the IB phase is further associated with maximal oxygen uptake, a criterion for exercise tolerance (Korkmaz Eryılmaz et al., 2018), and patients with coronary artery disease show a similar relationship (Yen et al., 2018). However, it has been reported that the IB phase is shorter in older individuals (Lenti et al., 2011).
In previous reports, the time in the IB phase (Tanehata et al., 1999; Nakade et al., 2019) and ΔVO2 RCP-VAT (Lenti et al., 2011; Carriere et al., 2019) have been used to define the IB phase; however, exercise tolerance indices such as peak VO2 decline with age. The time can only be compared with the same protocol [it may be possible to adjust it; however, an adjustment is not desirable because of the errors in the load acceleration and oxygen uptake (Hansen et al., 1987)]. Thus, ΔVO2 can be used to compare similar groups or those within the same age group; however, changes cannot be considered due to aging. It is not clear whether the shortening and decline in the indices of the IB phase used in the past are characteristically caused by aging or to what extent the decline is due to age-related changes. In the present study, we hypothesized that the ratio of oxygen uptake between RCP and VAT could be used as an indicator of IB phase because it can show how much oxygen uptake increases from VAT to RCP. By assessing the ratio of RCP to VAT, it would also be possible to easily show the lactate buffering capacity, and if it is the ratio of RCP to VAT oxygen uptake, it would be possible to make comparisons between ages.
Therefore, this study aimed to use the results of CPET to identify differences and changes over time in the IB phase using RCP/VAT and ΔVO2 RCP-VAT in healthy younger and older participants.
Materials and Methods
There were 126 healthy adult participants who completed a symptom-limited CPET in our hospital, for whom the VAT and RCP could be identified. Participants of previous studies (Kominami et al., 2015, 2021) and those who underwent screening tests at our hospital were enrolled; none of them had any diseases or had been treated with medications for indications such as cardiovascular or respiratory diseases. They were divided into two groups: <50 years (n = 95) and >50 years of age (n = 31). The participants’ characteristics are presented in Table 1.
Exercise Testing
Cardiopulmonary exercise testing was performed using a stationary bicycle (StrengthErgo 8; Mitsubishi Electric Engineering, Tokyo, Japan) and a breath-by-breath gas analyzer (AE-300S; Minato Ikagaku Co., Tokyo, Japan). Symptomatic maximal exercise was performed using a ramp protocol of 5–30 watts (W)/min according to age and condition after 2–3 min rest and warm-up of 0–10 W lasting 2–3 min. Rating of perceived exertion (RPE) at the end of the exercise was assessed using the Borg scale (Beaver et al., 1986a; Takano, 2000; Dekerle et al., 2003; Balady et al., 2010; Lenti et al., 2011; Wasserman et al., 2012; Yen et al., 2015, 2018; Nishijima et al., 2017, 2019; Keir et al., 2018; Korkmaz Eryılmaz et al., 2018; Carriere et al., 2019; Iannetta et al., 2019; Nakade et al., 2019).
Expiratory Gas Analysis Index
Ventilatory Anaerobic Threshold
The VAT was visually determined using the modified V-slope method as described by Sue et al. (1988), which is a modification of the method described by Beaver et al. (1986b).
The ventilatory equivalent method (the point at which VE/VO2 begins to rise without an increase in VE/VCO2) and end-tidal methods (PetO2 begins to rise without a decrease in PetCO2) was used as a complement (Balady et al., 2010; Wasserman et al., 2012).
Respiratory Compensation Point
Respiratory compensation point was comprehensively determined from the point where PetCO2 decreased, VE/VCO2 began to increase, and the inflection point of the VE/VCO2 slope.
The values of VAT VO2 and RCP VO2 were used to calculate RCP/VAT and ΔVO2 RCP-VAT (Figures 1A,B).
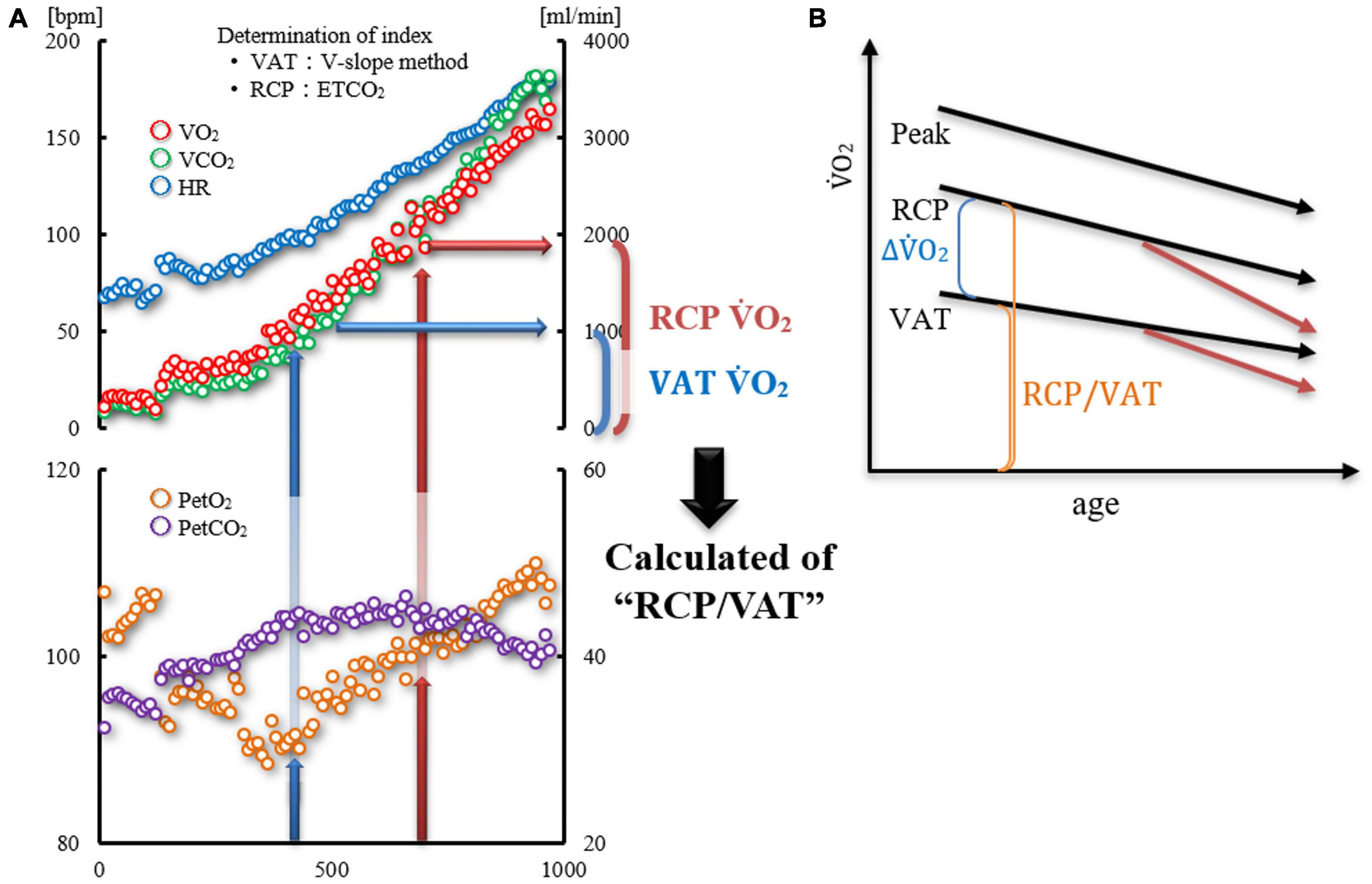
Figure 1. (A,B) Diagram showing the calculation of “RCP/VAT”. (A) Time trends of HR, VO2, VCO2, PETO2, and PETCO2 in one case. VAT was determined using the V-slope method and RCP from PETCO2 and VE/VCO2 slope. The RCP/VAT was calculated by dividing the RCP VO2 by the VAT VO2. (B) Age-related changes of VAT, RCP, and Peak VO2 (three black allows). It is believed that the decline in VAT is slower than that in RCP due to aging. Therefore, ΔVO2 RCP-VAT decreases with age; however, RCP/VAT may not change with age. If a disease associated with chronic inflammation, such as heart disease, develops, both VAT and RCP may further decrease, as shown by the red arrows, as well as ΔVO2 RCP-VAT and RCP/VAT.
VE/VCO2 Slope
VE/VCO2 slope as ventilation efficiency during incremental exercise load was calculated as the slope of linear regression from the start of exercise to RCP.
Oxygen Uptake Kinetics (ΔVO2/ΔWork Rate)
The VO2–work rate relationship during ramp exercise testing was evaluated by plotting the work rate on the x-axis and VO2 on the y-axis (Hansen et al., 1987; Wasserman et al., 2012). Both maximal and submaximal exercise data were plotted on the same graph. The initial time delay was removed from the analysis (Hansen et al., 1987). Each slope was calculated using linear regression for the maximum tests.
Statistical Analysis
Data are presented as the mean ± standard deviation. Statistical analyses were performed using Statistics for Excel 2012 (Social Survey Research Information Co., Tokyo, Japan). Student’s t-test was used for comparisons between the two groups. Pearson’s correlation coefficient was used to determine the correlation between age and each parameter, and the lowering rate with age was calculated from the regression coefficient of the regression line. The 95% confidence intervals and prediction intervals were also calculated for the relationship between age and RCP/VAT, ΔVO2/weight RCP-VAT, VAT VO2/weight, and RCP VO2/weight. The significance level was set at 5%.
Ethical Considerations
This research was conducted in accordance with the code of ethics of Sapporo Ryokuai Hospital and with due consideration for the protection of the participants’ personal information. Informed consent was obtained from all participants for their participation in the study and for publication of this report. The data obtained were de-linked and anonymized, and this study was conducted using the data for analysis. The authors confirmed that all participants could not be identified and that they were fully anonymized. Furthermore, the authors affirm that all mandatory health and safety procedures were complied within the course of conducting any experimental work reported in this paper.
Results
No adverse events such as arrhythmia, angina pectoris, or worsening of heart failure requiring treatment occurred during CPET. Regarding the characteristics of the participants, there were no significant differences in sex, weight, or BMI (Table 1).
Cardiopulmonary Exercise Testing Parameters
Each parameter in the CPET is listed in Table 2. Compared with the younger group, the older group showed significantly lower values of VAT and RCP VO2 (p < 0.001, respectively). Moreover, PetCO2 was significantly lower in the elderly group, and the VE/VCO2 slope was significantly higher (p < 0.001, respectively).
Furthermore, both ΔVO2/weight RCP-VAT and RCP/VAT showed a significant correlation with Peak VO2 (r = 0.629, p < 0.001 and r = 0.217, p = 0.017, respectively).
Respiratory Compensation Point/Ventilatory Anaerobic Threshold and ΔVO2/Weight Respiratory Compensation Point-Ventilatory Anaerobic Threshold
There was no significant difference in RCP/VAT between the younger and older groups (Table 2 and Figure 2). The coefficient of the linear regression equation was −0.0031, and the annual rate of decline was 0.185%, indicating that the effect of aging was not significant. ΔVO2/weight RCP-VAT was significantly lower in the older group, and age-related changes showed moderate negative correlation. The coefficient of the linear regression equation was −0.1303, and the annual rate of decline was 0.914%, indicating a significant effect of age-related changes.
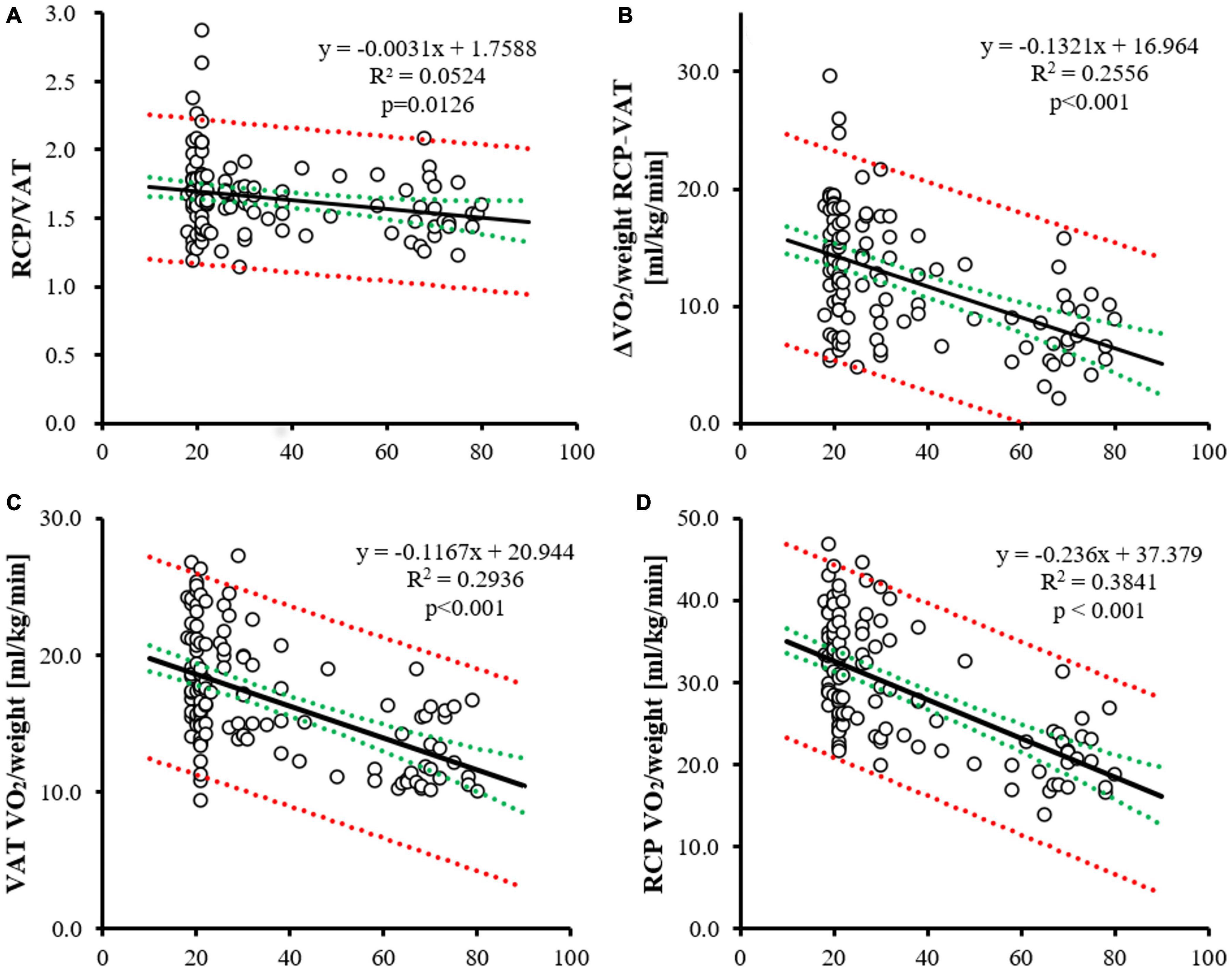
Figure 2. Age–related change of RCP/VAT, VO2/weight RCP-VAT, VAT and RCP VO2/weight. The age-related change of RCP/VAT (A), VO2/weight RCP-VAT (B), VAT (C) and RCP VO2/weight (D). The horizontal axis indicates age. For each panel, the correlation coefficient (linear regression equation and lowering rate per year; black line), 95% confidence intervals (red dotted line), and prediction intervals (green dotted line) are shown.
Discussion
In this study, IB during incremental exercise load was expressed as a ratio of oxygen uptake, which is different from that in previous studies. Moreover, the differences between healthy younger and elderly subjects and the changes over time were investigated. During the IB period from VAT to RCP, as the exercise intensity increases, lactate is buffered by HCO3–. Thus, a longer IB phase is less likely to lead to metabolic acidosis, allowing for the high-intensity exercise to continue for longer. Conversely, a short IB phase or an early onset of RCP may be associated with shortness of breath and fatigue. Our results show that the ratio of oxygen uptake during IB (RCP/VAT) is not significantly different in healthy older adults compared to healthy younger adults and is a modest indicator compared to the decline in VAT, RCP, and ΔVO2 RCP-VAT.
Age-Related Changes in Respiratory Compensation Point/Ventilatory Anaerobic Threshold
In this study, the decrease in VAT and RCP VO2/weight was −8.8 and −16.2 ml/min/year (−0.118 and −0.242 ml/kg/min/year, 0.627 and 0.722%/year), respectively, which was comparable to that reported in previous studies (Itoh et al., 2013). Similarly, the ΔVO2/weight RCP-VAT decreased with age. VAT is detected in CPET by the excretion of carbon dioxide in the exhaled air due to the buffering of lactate, which is produced by increased glycolysis associated with increased exercise intensity. The presence of increased lactate in the blood and carbon dioxide excretion in the exhaled air is well correlated, though there is a time delay. This association is derived from the proportion of slow-twitch fibers in skeletal muscle and CO2 storage in vivo (Ivy et al., 1980). The RCP is the period from the VAT through the IB phase to the onset of respiratory compensation. The buffering capacity of CO2/H+ produced by lactate is influenced by the fiber type in skeletal muscle; previous studies have shown an association with skeletal muscle composition, particularly type 2 fibers (Nakagawa and Hattori, 2002).
Several mechanisms are believed to allow the organism to rapidly maintain homeostasis in response to dynamic exercise (Bruce et al., 2019). The excretion of carbon dioxide during expiration is related to pulmonary blood flow, that is, cardiac output and ventilatory capacity. In our study, PETCO2, a measure of cardiac output, was significantly lower in the older group (though cardiac output was not measured directly and may therefore be dissociated from actual measurements). Additionally, the VE/VCO2 slope was higher in the older group, which may have contributed to the reduced capacity for lactate buffering and carbon dioxide excretion up to RCP, and the higher rate of decline in RCP VO2 than VAT with age.
In addition, ΔVO2/ΔWR, which indicates the oxygen utilization capacity of the peripheral motor muscle group, was not affected by age. In patients with specific risk factors, the reduced oxygen availability of the peripheral motor musculature facilitates anaerobic energy production during exercise, leading to the production of lactic acid and the buffering and excretion of CO2. In patients with heart failure, a decrease in cardiac output and vasodilatory capacity limits blood flow to peripheral exercise muscle groups, leading to a decrease in ΔVO2/ΔWR. In the present study, ΔVO2/ΔWR was not affected by aging, suggesting that the effect on energy production was small. However, we were not able to investigate related factors such as circulating blood volume, total body skeletal muscle mass, muscle composition, and plasma bicarbonate ion concentration concerning the accumulation and buffering capacity of CO2/H+ produced in vivo.
The results of this study showed that various indices of exercise (e.g., peak VO2 and VAT) decreased with age, and the rate of decrease was higher for RCP than for VAT. RCP/VAT, the ratio of RCP VO2 to VAT VO2, showed a modest negative correlation over time; however, there was no significant difference in RCP/VAT between the younger and older groups. RCP/VAT is considered a more moderate indicator of the effect of aging than ΔVO2/weight RCP-VAT. The results suggest that the ability to excrete CO2 produced in vivo or to accumulate and buffer CO2 is less affected by aging.
Respiratory Compensation Point/Ventilatory Anaerobic Threshold and ΔVO2 Respiratory Compensation Point-Ventilatory Anaerobic Threshold and Isocapnic Buffering Phase Time
For some IB phases that have been used in the past, the IB phase time can only be used for comparison in the same protocol. In addition, when the IB phase is expressed by ΔVO2/weight RCP-VAT, it is not possible to determine whether the decrease is only in that part of the whole exercise or the whole exercise, given that it is a cut-off of the oxygen uptake during exercise. In fact, as in a previous study (Carriere et al., 2019), peak VO2 and ΔVO2/weight RCP-VAT were correlated; however, since ΔVO2/weight RCP-VAT decreases with age, it is not suitable for comparison between groups of different ages. Conversely, the ratio of oxygen uptake, such as RCP/VAT, shows that the balance of exercise tolerance indices is maintained, though overall exercise tolerance decreased.
At low exercise intensities, oxidative phosphorylation is the main source of ATP production. As the exercise intensity increases, glycolysis increases, leading to lactate production and buffering. When the RCP is exceeded, lactic acidosis occurs. Since mitochondrial function including oxidative phosphorylation is not affected by aging or is only mildly affected (Lanza et al., 2005), VAT is considered to be less susceptible to the decline in mitochondrial function, except in certain diseases.
For a practical recommendation, by combining data with VAT and RCP VO2 and ΔVO2/weight RCP-VAT, we believe that RCP/VAT can possibly be used as a concise indicator for lactate buffering capacity and skeletal muscle composition ratio.
Research Limitations
This study had several limitations. First, because the analysis was based on only exhaled gas analysis, hematological parameters such as blood levels of lactate and bicarbonate ions, as well as hemodynamics, could not be evaluated in parallel. RCP is associated with maximal lactate steady state, and exercise therapy improves skeletal muscle function (oxidative capacity); however, in this study, it was difficult to clarify what led to the increase and improvement in RCP/VAT with exercise therapy. Second, RCP/VAT is an index that can be influenced by either an increase or decrease in one of the indices; a decrease in VAT may result in an increase in RCP/VAT. Third, the relationship with prognosis, as shown by IB phase time and ΔVO2/weight RCP-VAT, is unclear. Fourth, the effect of the degree of training on the lactate buffering capacity is unknown because we did not investigate the activity level or the degree of training in all participants. Finally, due to the small number of cases in the 30–60 age range, there is insufficient analysis of trends by age and by age group.
Conclusion
The ratio of oxygen uptake from VAT to RCP was not significantly lower in healthy older participants than in healthy younger participants. Although exercise tolerance decreases with age, age does not have a robust effect on lactate buffering capacity on exercise tolerance.
Data Availability Statement
The raw data supporting the conclusions of this article will be made available by the authors, without undue reservation.
Ethics Statement
Ethical review and approval was not required for the study on human participants in accordance with the local legislation and institutional requirements. Written informed consent for participation was not required for this study in accordance with the national legislation and the institutional requirements.
Author Contributions
KK and MA: conceptualization and methodology. KK: data curation, formal analysis, project administration, software, validation, visualization, and writing – original draft. MA: funding acquisition, resources, and supervision. KK, KI, TK, and MM: investigation. KI, TK, MM, and MA: writing – review and editing. All authors contributed to the article and approved the submitted version.
Funding
This work was performed at the Cardiac Rehabilitation Center, Sapporo Ryokuai Hospital, Sapporo, Japan.
Conflict of Interest
The authors declare that the research was conducted in the absence of any commercial or financial relationships that could be construed as a potential conflict of interest.
Publisher’s Note
All claims expressed in this article are solely those of the authors and do not necessarily represent those of their affiliated organizations, or those of the publisher, the editors and the reviewers. Any product that may be evaluated in this article, or claim that may be made by its manufacturer, is not guaranteed or endorsed by the publisher.
Acknowledgments
We would like to thank editage for English language editing.
References
Balady, G. J., Arena, R., Sietsema, K., Myers, J., Coke, L., Fletcher, G. F., et al. (2010). Clinician’s Guide to cardiopulmonary exercise testing in adults: a scientific statement from the American Heart Association. Circulation 122, 191–225. doi: 10.1161/CIR.0b013e3181e52e69
Beaver, W. L., Wasserman, K., and Whipp, B. J. (1986a). Bicarbonate buffering of lactic acid generated during exercise. J. Appl. Physiol. 60, 472–478. doi: 10.1152/jappl.1986.60.2.472
Beaver, W. L., Wasserman, K., and Whipp, B. J. (1986b). A new method for detecting anaerobic threshold by gas exchange. J. Appl. Physiol. 60, 2020–2027. doi: 10.1152/jappl.1986.60.6.2020
Bruce, R. M., Jolley, C., and White, M. J. (2019). Control of exercise hyperpnoea: contributions from thin-fibre skeletal muscle afferents. Exp. Physiol. 104, 1605–1621. doi: 10.1113/EP087649
Carriere, C., Corrà, U., Piepoli, M., Bonomi, A., Salvioni, E., Binno, S., et al. (2019). Isocapnic buffering period: from physiology to clinics. Eur. J. Prev. Cardiol. 26, 1107–1114. doi: 10.1177/2047487319829950
Dekerle, J., Baron, B., Dupont, L., Vanvelcenaher, J., and Pelayo, P. (2003). Maximal lactate steady state, respiratory compensation threshold and critical power. Eur. J. Appl. Physiol. 89, 281–288. doi: 10.1007/s00421-002-0786-y
Hansen, J. E., Sue, D. Y., Oren, A., and Wasserman, K. (1987). Relation of oxygen uptake to work rate in normal men and men with circulatory disorders. Am. J. Cardiol. 59, 669–674. doi: 10.1016/0002-9149(87)91190-8
Iannetta, D., de Almeida Azevedo, R., Keir, D. A., and Murias, J. M. (2019). Establishing the V̇o2 versus constant-work-rate relationship from ramp-incremental exercise: simple strategies for an unsolved problem. J. Appl. Physiol. 127, 1519–1527. doi: 10.1152/japplphysiol.00508.2019
Itoh, H., Ajisaka, R., Koike, A., Makita, S., Omiya, K., Kato, Y., et al. (2013). Heart rate and blood pressure response to ramp exercise and exercise capacity in relation to age, gender, and mode of exercise in a healthy population. J. Cardiol. 61, 71–78. doi: 10.1016/j.jjcc.2012.09.010
Ivy, J. L., Withers, R. T., Van Handel, P. J., Elger, D. H., and Costill, D. L. (1980). Muscle respiratory capacity and fiber type as determinants of the lactate threshold. J. Appl. Physiol. Respir. Environ. Exerc. Physiol. 48, 523–527. doi: 10.1152/jappl.1980.48.3.523
JCS Joint Working Group (2014). Guidelines for rehabilitation in patients with cardiovascular disease (JCS 2012). Circ. J. 78, 2022–2093. doi: 10.1253/circj.cj-66-0094
Keir, D. A., Pogliaghi, S., and Murias, J. M. (2018). The respiratory compensation point and the deoxygenation break point are valid surrogates for critical power and maximum lactate steady state. Med. Sci. Sports Exerc. 50, 2375–2378. doi: 10.1249/MSS.0000000000001698
Kominami, K., Nishijima, H., Imahashi, K., Katsuragawa, T., Murakami, M., and Akino, M. (2021). Gas exchange threshold to guide exercise training intensity of older individuals during cardiac rehabilitation. Medicine 100:e27540. doi: 10.1097/MD.0000000000027540
Kominami, K., Nishijima, H., Imahashi, K., Katsuragawa, T., Murakami, M., Yonezawa, K., et al. (2015). Very early lactate threshold in healthy young men as related to oxygen uptake kinetics. Medicine 94:e1559. doi: 10.1097/MD.0000000000001559
Korkmaz Eryılmaz, S., Polat, M., Soyal, M., and Aydoǧan, S. (2018). The relationship between the isocapnic buffering phase and ventilatory threshold in endurance athletes and team sport athletes during an incremental exercise test. Ann. Appl. Sport Sci. 6, 1–9. doi: 10.29252/aassjournal.6.1.1
Lanza, I. R., Befroy, D. E., and Kent-Braun, J. A. (2005). Age-related changes in ATP-producing pathways in human skeletal muscle in vivo. J. Appl. Physiol. 99, 1736–1744. doi: 10.1152/japplphysiol.00566.2005
Lenti, M., De Vito, G., Scotto di Palumbo, A., Sbriccoli, P., Quattrini, F. M., and Sacchetti, M. (2011). Effects of aging and training status on ventilatory response during incremental cycling exercise. J. Strength Cond. Res. 25, 1326–1332. doi: 10.1519/JSC.0b013e3181d99061
Mann, T., Lamberts, R. P., and Lambert, M. I. (2013). Methods of prescribing relative exercise intensity: physiological and practical considerations. Sports Med. 43, 613–625. doi: 10.1007/s40279-013-0045-x
Mezzani, A., Hamm, L. F., Jones, A. M., McBride, P. E., Moholdt, T., Stone, J. A., et al. (2013). Aerobic exercise intensity assessment and prescription in cardiac rehabilitation: a joint position statement of the European Association for Cardiovascular Prevention and Rehabilitation, the American Association of Cardiovascular and Pulmonary Rehabilitation and the Canadian Association of Cardiac Rehabilitation. Eur. J. Prev. Cardiol. 20, 442–467. doi: 10.1177/2047487312460484
Nakade, T., Adachi, H., Murata, M., and Naito, S. (2019). Relationship between respiratory compensation point and anaerobic threshold in patients with heart failure with reduced ejection fraction. Circ. J. 84, 76–82. doi: 10.1253/circj.CJ-19-0561
Nakagawa, Y., and Hattori, M. (2002). Relationship between muscle buffering capacity and fiber type during anaerobic exercise in human. J. Physiol. Anthropol. Appl. Human Sci. 21, 129–131. doi: 10.2114/jpa.21.129
Nishijima, H., Kominami, K., Kondo, K., Akino, M., and Sakurai, M. (2019). New method for the mathematical derivation of the ventilatory anaerobic threshold: a retrospective study. BMC Sports Sci. Med. Rehabil. 11:10. doi: 10.1186/s13102-019-0122-z
Nishijima, H., Kondo, K., Yonezawa, K., Hashimoto, H., and Sakurai, M. (2017). Quantification and physiological significance of the rightward shift of the V-slope during incremental cardiopulmonary exercise testing. BMC Sports Sci. Med. Rehabil. 9:9. doi: 10.1186/s13102-017-0073-1
Price, K. J., Gordon, B. A., Bird, S. R., and Benson, A. C. (2016). A review of guidelines for cardiac rehabilitation exercise programmes: is there an international consensus? Eur. J. Prev. Cardiol. 23, 1715–1733. doi: 10.1177/2047487316657669
Yen, Y. S., Yang, S. H., Chou, C. L., Su, D. C. J., Chow, J. C., and Chou, W. (2015). The Clinical Significance of Isocapnic Buffering Phase During Exercise Testing: an Overview. Int. J. Phys. Med. Rehabil. 3:3.
Sue, D. Y., Wasserman, K., Moricca, R. B., and Casaburi, R. (1988). Metabolic acidosis during exercise in patients with chronic obstructive pulmonary disease. Use of the V-slope method for anaerobic threshold determination. Chest 94, 931–938. doi: 10.1378/chest.94.5.931
Sullivan, M. J., and Cobb, F. R. (1990). The anaerobic threshold in chronic heart failure. Relation to blood lactate, ventilatory basis, reproducibility, and response to exercise training. Circulation 81, II47–II58.
Takano, N. (2000). Respiratory compensation point during incremental exercise as related to hypoxic ventilatory chemosensitivity and lactate increase in man. JPN. J. Physiol. 50, 449–455. doi: 10.2170/jjphysiol.50.449
Tanehata, M., Adachi, H., Oshima, S., Taniguchi, K., Itoh, H., Hasegawa, A., et al. (1999). The time from anaerobic threshold (AT) to respiratory compensation point reflects the rate of aerobic and anaerobic metabolism after the AT in chronic heart failure patients. JPN. Circ. J. 63, 274–277. doi: 10.1253/jcj.63.274
Wasserman, K., Hansen, J. E., Sue, D. Y., and Stringer, W. W. (2012). Principles of Exercise Testing and Interpretation: Including Pathophysiology and Clinical Application, 5th Edn. Philadelphia: Lippincott Williams & Wilkins, 9–61.
Keywords: isocapnic buffering phase, ventilatory anaerobic threshold, respiratory compensation point, aging, oxygen uptake, cardiopulmonary exercise testing
Citation: Kominami K, Imahashi K, Katsuragawa T, Murakami M and Akino M (2022) The Ratio of Oxygen Uptake From Ventilatory Anaerobic Threshold to Respiratory Compensation Point Is Maintained During Incremental Exercise in Older Adults. Front. Physiol. 13:769387. doi: 10.3389/fphys.2022.769387
Received: 02 September 2021; Accepted: 07 February 2022;
Published: 03 March 2022.
Edited by:
Leonardo Alexandre Peyré-Tartaruga, Federal University of Rio Grande do Sul, BrazilReviewed by:
Hamdi Chtourou, University of Sfax, TunisiaElisabetta Salvioni, Monzino Cardiology Center (IRCCS), Italy
Copyright © 2022 Kominami, Imahashi, Katsuragawa, Murakami and Akino. This is an open-access article distributed under the terms of the Creative Commons Attribution License (CC BY). The use, distribution or reproduction in other forums is permitted, provided the original author(s) and the copyright owner(s) are credited and that the original publication in this journal is cited, in accordance with accepted academic practice. No use, distribution or reproduction is permitted which does not comply with these terms.
*Correspondence: Kazuyuki Kominami, qqae3s4u9@gmail.com
†These authors have contributed equally to this work and share first authorship