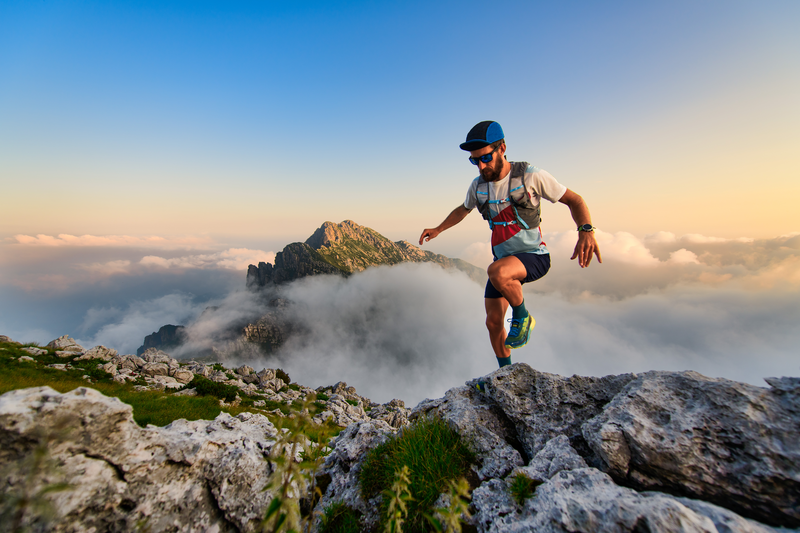
95% of researchers rate our articles as excellent or good
Learn more about the work of our research integrity team to safeguard the quality of each article we publish.
Find out more
EDITORIAL article
Front. Physiol. , 14 December 2022
Sec. Red Blood Cell Physiology
Volume 13 - 2022 | https://doi.org/10.3389/fphys.2022.1103647
This article is part of the Research Topic Comparative Biology of Red Blood Cells View all 5 articles
Editorial on the Research Topic
Comparative biology of red blood cells
Our interest in the broad diversity of red blood cell (RBC) sizes, composition, gas transport strategies, rheological properties, and metabolism across the animal kingdom is fueled by several reasons. Biologists follow the developmental and evolutionary aspects of this RBC diversity that enables the hosts to survive and populate habitats that vary in O2 concentration and nutritional abundance matching energy supply and demand (Nikinmaa, 1990). Translational researchers view animals as models to study human diseases, although numerous studies urge for care in translation of the findings obtained using rodents or other mammals as well as insects, nematodes and fishes to the human patients (Smith, 1981; van der Worp et al., 2010; Robinson et al., 2019; Doncheva et al., 2021; Mukherjee et al., 2022). Finally, veterinarians are focused on treatment of diseases of their animal patients associated with RBC abnormalities (Brooks et al., 2022). These three groups of scientists do not speak “the same language” and the journals publishing the findings in clinical hematology do recognize the publications dealing with comparative aspects of RBC physiology. This topic was a challenging attempt to fuse the interests of both sides, applying the methodological approaches developed for human RBC to the animal cells. The four papers forming this volume meet a common ground touching upon various parameters in control of RBC rheology and life-span. These parameters include the regulation of cell hydration and volume (Lu et al.), oxygenation of hemoglobin (Bertolone et al.; Lu et al.; Man et al.), metabolism and antioxidative defense systems (Klichkhanov et al.; Bertolone et al.). In their study Kichkhabnov et al. monitor the changes in production of NO and in the markers of oxidative stress and antioxidant enzymes in RBCs of the ground squirrels while active, during hibernation, and during the arousal phase when their body temperature and metabolic activity undergo massive and rapid transition. Robust antioxidative defense mechanisms in RBCs of these animals are described allowing to avoid hemolytic crisis despite the increase in oxidative load during arousal. The inability of guinea pigs to produce their own ascorbate makes them dependent on its dietary intake and prone to scurvy development. Another dark side of this metabolic feature, as described by Bertolone et al., is higher susceptibility of RBCs of guinea pigs to blood storage lesions.
Sickling of RBC is a phenomenon that most of us associate with human sickle cell disease. However, this mechanism has been evolved much earlier and is used to combat blood infection by fishes, reptiles, and other mammals (Steinberg, 2019). In humans, as well as in other species, hemoglobin aggregation is not occurring in all cells, but in a fraction of them. In their work Man et al. introduce an OcclusionChip that may detect the abundance of cells in the fraction that loses deformability and may initiate thrombosis. They evaluate the sensitivity of this approach using blood of healthy people and sickle cell disease patients comparing this with the outcome of oxygen gradient ektacytometry. The use of the OcclusionChip for animal blood samples with “sickling red blood cells” should provide important information on the impact of sickling of RBCs of different species on their rheological properties. Regulation of the K-Cl cotransporter (KCC) contributes to the maintenance of RBC volume, and its hyperactivation in cells of patients may induce aggregation of sickle hemoglobin (HbS) and promote the loss of deformability due to the irreversible sickling. The study of Lu et al. explores the role of bicarbonate, pH, and urea levels in plasma, as well as hemoglobin oxygenation, in regulation of the KCC function in low K+ sheep RBCs. The obtained data shed light on some of the underexplored aspects of dehydration mechanisms in human sickle cell disease patients.
In line with the publications of this Research Topic we hope to further stimulate translational RBC research. Neither is it explicidly limited to the utilization of transgenic (mouse) models to mimic human diseases with all the well-known associated challenges nor to use animals as disease models for practical reasons, e.g. (Thomas et al., 2001), but the use of natural genetic and functional heterogeneity of RBCs from various species such as the elliptic camel RBCs as models for elliptocytosis (Smith et al., 1979; Amin and Sirs, 1985; Windberger et al., 2018; Baier et al., 2021), naturally sickling RBCs of deer, sheep, goat, genets and mongoose, Gulf toadfish, iguana, snakes, or fishes for sickle cell disease (Steinberg, 2019), cow and sheep RBC as models for lipid alterations (Engen and Clark, 1990; Nouri-Sorkhabi et al., 1996; Ivanov, 2007), and cattle (Inaba et al., 1996; Jay, 1996) or even lamprey (Cameron et al., 2000) RBCs as a model of Band 3 protein deficiency. We are looking forward to it.
All authors listed have made a substantial, direct, and intellectual contribution to the work and approved it for publication.
The authors declare that the research was conducted in the absence of any commercial or financial relationships that could be construed as a potential conflict of interest.
All claims expressed in this article are solely those of the authors and do not necessarily represent those of their affiliated organizations, or those of the publisher, the editors and the reviewers. Any product that may be evaluated in this article, or claim that may be made by its manufacturer, is not guaranteed or endorsed by the publisher.
Amin T. M., Sirs J. A. (1985). The blood rheology of man and various animal species. Exp. Physiol. 70, 37–49. doi:10.1113/expphysiol.1985.sp002895
Baier D., Müller T., Mohr T., Windberger U. (2021). Red blood cell stiffness and adhesion are species-specific properties strongly affected by temperature and medium changes in single cell force spectroscopy. Molecules 26, 2771. doi:10.3390/molecules26092771
Brooks M. B., Harr K. E., Seelig D. M., Wardrop K. J., W., Weiss D. J. (Editors) (2022). Schalm's veterinary hematology (New York, NY: Willey Blackwell).
Cameron B. A., Gilmour K., Forster R., Ko K., Tufts B. L. (2000). Unique distribution of the anion exchange protein in the sea lamprey, Petromyzon marinus. J. Comp. Physiology B Biochem. Syst. Environ. Physiology 170, 497–504. doi:10.1007/s003600000127
Doncheva N. T., Palasca O., Yarani R., Litman T., Anthon C., Groenen M. a. M., et al. (2021). Human pathways in animal models: Possibilities and limitations. Nucleic Acids Res. 49, 1859–1871. doi:10.1093/nar/gkab012
Engen R. L., Clark C. L. (1990). High-performance liquid chromatography determination of erythrocyte membrane phospholipid composition in several animal species. Am. J. Vet. Res. 51, 577–580.
Inaba M., Yawata A., Koshino I., Sato K., Takeuchi M., Takakuwa Y., et al. (1996). Defective anion transport and marked spherocytosis with membrane instability caused by hereditary total deficiency of red cell band 3 in cattle due to a nonsense mutation. J. Clin. Invest. 97, 1804–1817. doi:10.1172/jci118610
Ivanov I. T. (2007). Allometric dependence of the life span of mammal erythrocytes on thermal stability and sphingomyelin content of plasma membranes. Comp. Biochem. Physiology Part A Mol. Integr. Physiology 147, 876–884. doi:10.1016/j.cbpa.2007.02.016
Jay D. G. (1996). Role of band 3 in homeostasis and cell shape. Cell 86, 853–854. doi:10.1016/s0092-8674(00)80160-9
Mukherjee P., Roy S., Ghosh D., Nandi S. K. (2022). Role of animal models in biomedical research: A review. Lab. Anim. Res. 38, 18. doi:10.1186/s42826-022-00128-1
Nikinmaa M. (1990). Vertebrate red blood cells. Adaptations of function to respiratory requirements. Berlin, Heidelberg: Springer-Verlag.
Nouri-Sorkhabi M. H., Agar N. S., Sullivan D. R., Gallagher C., Kuchel P. W. (1996). Phospholipid composition of erythrocyte membranes and plasma of mammalian blood including Australian marsupials; quantitative 31P NMR analysis using detergent. Comp. Biochem. Physiology Part B Biochem. Mol. Biol. 113, 221–227. doi:10.1016/0305-0491(95)02011-x
Robinson N. B., Krieger K., Khan F. M., Huffman W., Chang M., Naik A., et al. (2019). The current state of animal models in research: A review. Int. J. Surg. 72, 9–13. doi:10.1016/j.ijsu.2019.10.015
Smith J. E. (1981). Animal models of human erythrocyte metabolic abnormalities. Clin. Haematol. 10, 239–251. doi:10.1016/s0308-2261(21)00218-6
Smith J. E., Mohandas N., Shohet S. B. (1979). Variability in erythrocyte deformability among various mammals. Am. J. Physiology-Heart Circulatory Physiology 236, H725–H730. doi:10.1152/ajpheart.1979.236.5.h725
Steinberg M. H. (2019). "Sickling" in vertebrates: Animal studies vs. sickle cell disease. Blood Rev. 36, 88–94. doi:10.1016/j.blre.2019.05.001
Thomas S. L., Egée S., Lapaix F., Kaestner L., Staines H. M., Ellory J. C. (2001). Malaria parasitePlasmodium gallinaceumup-regulates host red blood cell channels. FEBS Lett. 500, 45–51. doi:10.1016/s0014-5793(01)02579-0
van der Worp H. B., Howells D. W., Sena E. S., Porritt M. J., Rewell S., O'Collins V., et al. (2010). Can animal models of disease reliably inform human studies? PLoS Med. 7, e1000245. doi:10.1371/journal.pmed.1000245
Keywords: red blood cells, animal models, adaptation, deformability, sickle cell disease, metabolism, K-Cl contransporter, hibernation
Citation: Bogdanova A and Kaestner L (2022) Editorial: Comparative biology of red blood cells. Front. Physiol. 13:1103647. doi: 10.3389/fphys.2022.1103647
Received: 20 November 2022; Accepted: 06 December 2022;
Published: 14 December 2022.
Edited by:
Nicola Conran, State University of Campinas, BrazilReviewed by:
Egee Stéphane, UMR8227 Laboratoire de Biologie Intégrative des Modèles Marins, FranceCopyright © 2022 Bogdanova and Kaestner. This is an open-access article distributed under the terms of the Creative Commons Attribution License (CC BY). The use, distribution or reproduction in other forums is permitted, provided the original author(s) and the copyright owner(s) are credited and that the original publication in this journal is cited, in accordance with accepted academic practice. No use, distribution or reproduction is permitted which does not comply with these terms.
*Correspondence: Anna Bogdanova, YW5uYWJAYWNjZXNzLnV6aC5jaA==; Lars Kaestner, bGFyc19rYWVzdG5lckBtZS5jb20=
†These authors have contributed equally to this work
Disclaimer: All claims expressed in this article are solely those of the authors and do not necessarily represent those of their affiliated organizations, or those of the publisher, the editors and the reviewers. Any product that may be evaluated in this article or claim that may be made by its manufacturer is not guaranteed or endorsed by the publisher.
Research integrity at Frontiers
Learn more about the work of our research integrity team to safeguard the quality of each article we publish.