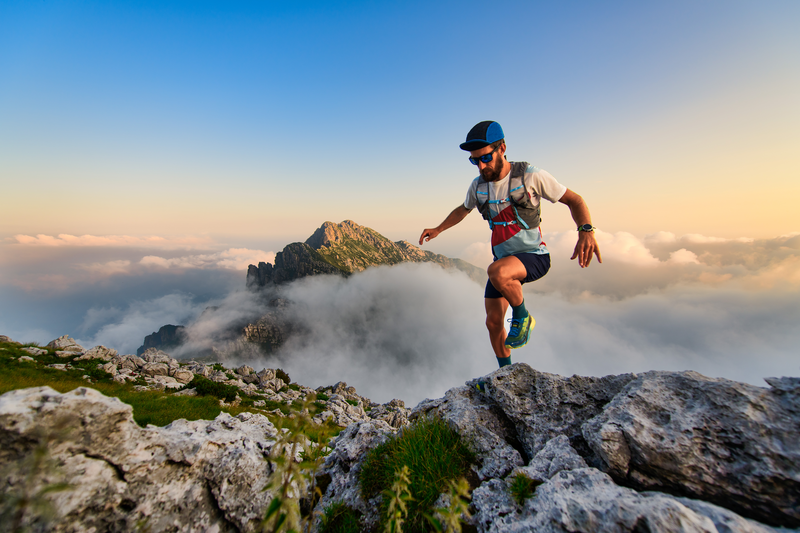
95% of researchers rate our articles as excellent or good
Learn more about the work of our research integrity team to safeguard the quality of each article we publish.
Find out more
ORIGINAL RESEARCH article
Front. Physiol. , 09 January 2023
Sec. Respiratory Physiology and Pathophysiology
Volume 13 - 2022 | https://doi.org/10.3389/fphys.2022.1090612
Background: The optimal ventilatory strategy for the face mask ventilation during anesthesia induction is still unknow.
Methods: We evaluated the effect of two positive end-expiratory pressure (PEEP) levels (0 cmH2O and 6 cmH2O) and two oxygen concentration levels (1.0 and .6) on non-hypoxemic apnea time during face mask ventilation of anesthesia induction. Sixty adult patients scheduled for elective surgery were enrolled in this study. The patients were randomized to receive anesthesia induction with four different ventilation strategy under volume-controlled ventilation. Patients assigned to the LOZP group received low fraction of inspiration O2 (FiO2 = .6) and 0 PEEP. Patients assigned to the LOHP group received low fraction of inspiration O2 (FiO2 = .6) and 6 cmH2O PEEP. Patients assigned to the HOZP group received high fraction of inspiration O2 (FiO2 = 1.0) and 0 PEEP. Patients assigned to the HOHP group received high fraction of inspiration O2 (FiO2 = 1.0) and 6cmH2O PEEP. After 3 min of ventilation, the patient was intubated but disconnected from the breathing circuit. Ventilation was not initiated until the pulse oximetry dropped to 90%. The primary outcome was non-hypoxemic apnea time defined as the time from cessation of ventilation to a pulse oximeter reading of 90%. The secondary outcome was the PaO2/FiO2 ratio immediately after ventilation.
Results: The non-hypoxemic apnea time was significantly longer in the group of HOHP when compared to the other three groups (192 s ± 70 s, 221 s ± 74 s, 284 s ± 101 s, and 353 s ± 85 s in the LOZP, LOHP, HOZP, and HOHP group, respectively). The PaO2/FiO2 ratio immediately after ventilation was significantly higher in the group of LOHP when compared to the other three groups (LOZP 393 ± 130, LOHP 496 ± 97, HOZP 335 ± 58, HOHP 391 ± 50). When compared the PaO2/FiO2 ratio immediately after ventilation to its value before administration of anesthesia, the PaO2/FiO2 ratio in the group of LOHP was improved, the group LOZP and HOHP remained the same, while the group HOZP significantly decreased.
Conclusion: Application of PEEP and 100% of oxygen during face mask ventilation of induction could maximize the non-hypoxemic apnea time. However, the use of PEEP and 60% of oxygen during preoxygenation resulted in improved PaO2/FiO2 ratio.
Induction of anesthesia is of crucial importance to both patient and anesthesiologists. One of the biggest challenges to be deal with during this period is endotracheal intubation, especially in patients with difficult airway or significant lung comorbidities. Hypoxemia may occur due to multiple attempts of endotracheal intubation, low oxygen reserve capacity or poor pulmonary gas exchange (Merry et al., 2009). Therefore, prolongation of non-hypoxemic apnea time and improvement of arterial partial pressure of oxygen is important to provide safe anesthetic induction.
At present, the standard face mask ventilation strategy after preoxygenation and induction of anesthesia is to apply bag-squeezing technique with 100% oxygen, which had been used for a long time since the establishment of modern general anesthesia. However, the combination of high oxygen fraction (FiO2), muscle relaxant and positive pressure ventilation accelerate the formation of atelectasis in dependent lung regions and reduction of end-expiratory lung volume during induction period, which further hindered the ventilation efficiency of the patient (Hedenstierna et al., 2019). Recent studies showed that applying PEEP during anesthesia induction alleviate formation of lung atelectasis, and effectively prolongs apnea time (Kim et al., 2021). It is also reported that application of low oxygen fraction could reduce atelectasis and improve PaO2/FiO2 perioperatively (Koo et al., 2019). However, to date there are no studies on effects of both PEEP and oxygen concentration on non-hypoxemic apnea time and PaO2/FiO2 ratio during face mask ventilation of anesthetic induction.
Therefore, in this study, we aimed to assess the effect of two positive PEEP levels (0 cmH2O and 6 cmH2O) and two FiO2 levels (1.0 and .6) on non-hypoxemic apnea time and PaO2/FiO2 ratio during face mask ventilation of anesthesia induction in adults.
This was a prospective, randomized controlled trial performed at Anesthesia and Operation Centre, First Medical Centre of Chinese PLA General Hospital. This study was approved by the Ethical Committee of Chinese PLA General Hospital in Beijing, China (protocol number: S2021-489-01, president of the ethics committee: Prof. Xiangyang Chu, Date of approval: 30 Sep 2021). Written informed consent was obtained from all subjects participating in the trial. The trial was registered prior to patient enrolment at the Chinese Clinical Trial Registry (ChiCTR2100053037, Principal investigator: Changsheng Zhang, Date of registration: 10 Nov 2021). This manuscript adheres to the applicable CONSORT guidelines.
From 11 Nov 2021 to 30 Dec 2021, 60 patients aged 18–80 years were enrolled in this study. We included patients who were scheduled for surgery under general anesthesia with endotracheal intubation. Further inclusion criteria were: American Society of Anesthesiology (ASA) Class I or II, age 18–80 years, and a body mass index less than 35 kg/m2. Patients were excluded if they had one or more of the following criteria: indwelling nasal gastric tube, anticipated difficult intubation, obstructive sleep apnea, history of documented chronic organ failure, hypertension, ischemic heart disease, atrioventricular block, incomplete or partial heart blocks.
All the anesthesiologists and investigators participating in this study had at least five years of experience as attending physicians at our institution. Eligible patients will be randomly assigned to four groups prior to their electively scheduled surgery with an allocation ratio of 1:1:1:1. Restricted randomization assignments was determined by a computerized random-number generator. The patient and investigator in the operating room were blinded to the patient allocation and intervention until all trial data were analyzed.
After arriving in the operation room, the patient was placed supine on the induction table. Venous access was obtained with an 18-gauge cannula placed in the left forearm. Electrocardiogram, pulse oximetry, and non-invasive blood pressure (cuff placed on the right upper arm) were continuously monitored. Under strict aseptic precautions, the radial artery was then cannulated with a 22-gauge cannula after local infiltration with 2% lidocaine and the arterial line flushed with heparinized saline. Baseline values of heart rate, blood pressure and SpO2 were recorded, and a sample of arterial blood gas (ABG1) was taken with the patient breathing room air.
Before anesthesia induction, patients were instructed to take five deep breaths under room air, while the whole breathing circuit (including the face mask) was filled with oxygen. Then, anesthesia was induced by anesthesiologist in charge of the case using midazolam .03 mg/kg, sufentanil .25 μg/kg, propofol 2 mg/kg, rocuronium .6 mg/kg. Ventilation was initiated after patients were unresponsive via an appropriately sized face mask, provided by an anesthesia machine (Fabius plus, Drager, AG, Lubeck/Germany). A “Guedel” style oropharyngeal airway was placed to ensure sufficient tidal volume. Ventilation was considered to be successful when the end-tidal oxygen concentration (EtO2) achieved 90% of the setting FiO2 and the patient’s expired tidal volume was similar to the volume set on the ventilator. To avoid gastric insufflation and aspiration, Sellick maneuver was applied during ventilation by an anesthesia nurse.
All the patients in this study received volume-controlled ventilation during ventilation for 90 s with a tidal volume of 6 mL/kg (ideal body weight), and a respiratory rate of 16 bpm–20 bpm, inspiration/expiration ratio of 1:2 and a gas flow rate of 10 L/min. Patients assigned to the LOZP group (Low FiO2 + ZEEP) received FiO2 = .6 and 0 PEEP. Patients assigned to the LOHP group (Low FiO2 + PEEP) received FiO2 = .6 and 6 cmH2O PEEP. Patients assigned to the HOZP group (High FiO2 + ZEEP) received FiO2 = 1.0 and 0 PEEP. Patients assigned to the HOHP group (High FiO2 + PEEP) received FiO2 = 1.0 and 6 cmH2O PEEP. A sample of arterial blood was obtained at the end of the 90 s mechanical ventilation period (ABG2).
At the end of the allocated mechanical ventilation for 90 s, the patient was intubated with an appropriately sized endotracheal tube using a video laryngoscope (VL310, UE Medical Corp., Zhejiang, China) but was not ventilated until pulse oximetry saturation reached 90%. The time from cessation of ventilation to a pulse oximeter reading of 96% (T100-96) and 96%–90% (T96-90) was recorded, respectively. When the pulse oximetry saturation reached 90%, mechanical ventilation was resumed with a tidal volume of 6 mL/kg, 12 breaths per minute, FiO2 = .6, 6 cmH2O PEEP and a gas flow rate of 2 L/min. The lowest pulse oximeter reading was recorded during this period. The time from resume of mechanical ventilation to a pulse oximeter reading of 96% (Tmin-96) and 96%–100% (T96-100) was also recorded. If the patient took more than 120s from a pulse oximeter reading of 96%–100%, a recruiting breath was applied.
The primary outcome was non-hypoxemic apnea time defined as the time from cessation of ventilation to a pulse oximeter reading of 90% (T100-90). The secondary outcome was the PaO2/FiO2 ratio at the time when ventilation was discontinued just prior to intubation. This study was a double-blind randomized controlled study where the patient and outcome assessor were blinded to study interventions until the study was completed.
According to our pilot study including 12 patients, the non-hypoxemic apnea time in the group LOZP, LOHP, HOZP, and HOHP was 190 s, 250 s, 248 s, and 336 s, respectively (standard deviation 99.8 s). Sample size calculations showed that 12 patients were needed in each group (a power of .8 and a two-sided p-value of less than .05). Therefore, we anticipated enrolling 15 subjects (12 + 20% possible dropouts) in each group.
The statistical analysis was conducted using Statistical Package for Social Sciences (SPSS Inc., Chicago, IL, Version 17.0 for Windows). Results are expressed as means and standard deviations, medians and ranges, or numbers and percentages. Repeated measures analyses of variance (ANOVAs) were used to analyze the difference of non-hypoxemic apnea time and PaO2/FiO2 ratio between each group. Other results were subjected to one-way ANOVA using the Tukey multiple comparison test. Normality of data was checked by measures of skewness and Kolmogorov Smirnov tests. A p-value of less than .05 was considered statistically significant.
In total, 60 adult patients scheduled for surgery under general anesthesia were enrolled in this study. No patients were excluded from further analysis (Figure 1). Intubation was performed at the first attempt by experienced anesthesiologists in all patients successfully. The demographic data did not differ between the four groups with regard to age and body mass index (BMI). The distribution as per sex, ASA status, hemoglobin, and surgery type was similar in all groups and statistically non-significant. Table 1 shows baseline demographics for each group.
FIGURE 1. Flow chart of 87 consecutive patients that scheduled for surgery under general anesthesia during the study period. After 27 patients were excluded for reasons stated above, 60 patients were randomized to four study groups.
The time from cessation of ventilation to a pulse oximeter reading of 90% (T100-90) was 192 s ± 70 s, 221 s ± 74 s, 284 s ± 101 s, and 353 s ± 85 s in the LOZP, LOHP, HOZP, and HOHP group, respectively (F = 10.99, p < .01). The T100-90 was significantly longer in the HOHP group when compared to other three groups (p < .05). And the T100-90 of HOZP group was significantly longer than the LOZP group (p < .05). The application of 6 cmH2O of PEEP did not significantly improve the non-hypoxemic apnea time when compared within the groups receiving the same FiO2 (Table 2).
The time from cessation of ventilation to a pulse oximeter reading of 96% (T100-96) was 144 s ± 47 s, 171 s ± 59 s, 214 s ± 82 s, and 287 s ± 69 s in the LOZP, LOHP, HOZP and HOHP group, respectively (F = 13.90, p < .01). T100-96 was significantly longer in the HOHP group when compared to the other three groups (p < .05). And the T100-96 of HOZP group was significantly longer than the LOZP group (p < .05). However, the T100-96 was not differed between the group of HOZP and LOHP (p = .280). The application of 6 cmH2O of PEEP significantly improved the T100-96 of the group of HOHP when compared to the group of HOZP. The time from a pulse oximeter reading of 96%–90% was slightly longer in groups applying 100% oxygen, but not statistically significant (p = .077) (Figure 2).
FIGURE 2. The non-hypoxemic apnea time after induction and the rising time of the SpO2 after ventilation resume. The non-hypoxemic apnea time (T100-90 and T100-96) was significantly longer in the group of HOHP when compared to the other three groups (p < .01). The rising time of the SpO2 did not differ among the groups (p > .05).
The lowest pulse oximeter reading after resuming mechanical ventilation did not differ among the four groups (Range from 80 to 89, 25th–75th percentiles 86). The time from resume of mechanical ventilation to a pulse oximeter reading of 96% and 96%–100% did not differ among each group (F = .906, p = .444, F = 2.718, p = .053, respectively). In group HOZP, the pulse oximeter reading of three subjects took more than 120s to reach 100% from 96%.
The PaO2 (as well as PaO2/FiO2 ratio) and PaCO2 did not differ among each group prior to ventilation (F = .646, p = .589, F = .739, p = .533, respectively). The PaO2 of the LOZP, LOHP, HOZP, and HOHP group at the time mask ventilation was stopped was 236 ± 71, 298 ± 58, 335 ± 58, 391 ± 50, respectively (F = 16.69, p < .01). In the post hoc multiple comparisons, the PaO2 of the LOZP group was lower than the other three groups (p < .05). The PaO2 of the HOHP group was significantly higher than groups applying 60% oxygen (p < .01). However, the PaO2 of the HOZP group did not differ from the LOHP group (p = .368), and the PaO2 of the HOHP group was slightly higher than the HOZP group, but not statistically significant (p = .071). Besides, the results of the PaCO2 after ventilation were similar across the groups (F = .185, p = .906).
The PaO2/FiO2 ratio of the LOZP, LOHP, HOZP and HOHP group immediately after ventilation was stopped was 393 ± 130, 496 ± 97, 335 ± 58, 391 ± 50, respectively (F = 8.472, p < .001). In the post hoc multiple comparisons, the PaO2/FiO2 ratio of the LOHP group was significantly higher than the HOZP group (p = .003), and slightly higher than LOZP group and HOHP group with p values of .052 and .079.
When compared the PaO2/FiO2 ratio after ventilation to its value with the patient breathing room air, the PaO2/FiO2 ratio in the group of LOHP was significantly improved (p < .01), the group LOZP and HOHP remained the same, while the group HOZP significantly decreased (p < .01) (Figure 3; Table 3).
FIGURE 3. Results of the PaO2/FiO2 ratio before and after induction of the four groups. The LOHP was significantly improved (p < .01), the group LOZP and HOHP remained the same, while the group HOZP significantly decreased (p < .01).
In this prospective, randomized controlled trial, we evaluated the effects of two levels of PEEP (0 cmH2O and 6 cmH2O) and two levels of FiO2 (1.0 and .6) on non-hypoxemic apnea time and PaO2/FiO2 ratio during face mask ventilation of anesthesia induction in adults. The major finding of the present study is that, application of 6 cmH2O of PEEP and 100% of oxygen during face mask ventilation during general anesthesia induction could maximize the non-hypoxemic apnea time in non-obese, non-critically ill elective surgery patients.
An expected finding in our study is that face mask ventilation after induction anesthesia with 100% of oxygen combined with PEEP provided the longest non-hypoxemic apnea time among all four groups. Previous studies have shown that, the use of continuous positive pressure during induction was well-established to decreases atelectasis formation and prolongs the non-hypoxemic apnea time among different populations from infants to adults, the non-obese to obese (Coussa et al., 2004; Gander et al., 2005; Tagaito et al., 2007; Isono, 2009; Kim et al., 2021). The application of PEEP was shown by CT scans to limit the atelectasis formation, therefore provides an increase in functional residual capacity and in the intrapulmonary oxygen store (Barbosa et al., 2014). As shown in our study, ventilation with the use of PEEP and 100% oxygen could more efficiently maintain the duration of non-hypoxic apnea than without PEEP or with a lower FiO2. Therefore, in clinical practice, application of PEEP during ventilation should be considered as a standard technique for all anesthesia induction, because it extends the non-hypoxemic apnea time, improves oxygenation, and increases the margin of safety before intubation.
With the rise of lung protective ventilation strategy in recent years, the needs for better PaO2/FiO2 ratio during anesthesia induction (or the peri-anesthesia period) are increasing, while anesthesiologists are still seeking better ventilation methods and longer non-hypoxemic apnea time (Young et al., 2019). Therefore, in this study, we tested the application of 6 cmH2O of PEEP and FiO2 of 60% during mask ventilation of ventilation. We found that the FiO2 of 60% and PEEP obtained similar non-hypoxemic apnea time when compared to the classical ventilation methods.
However, the PaO2/FiO2 ratio after mask ventilation of the two groups above mentioned are totally different. When compared to its value before induction, the LOHP group’s PaO2/FiO2 ratio increased significantly while the HOZP group decreased significantly. Although the application of 6 cmH2O of PEEP and FiO2 of 100% obtained the longest non-hypoxemic apnea time in this study, the PaO2/FiO2 ratio of these patients were not as high as patients receiving 6 cmH2O of PEEP and FiO2 of 60%. A possible explanation could be that 60% oxygen with PEEP decreases the most amount of atelectasis formation, hence increases the functional residual capacity, and at the same time decreases the level of intrapulmonary shunt. What’s more, there were three subjects in group HOZP took more than 120 s to reach a pulse oximeter reading 100% from 96%. This may also result from massive atelectasis formation especially during apnea period of the study procedure, which lead to a relatively slow recruitment of ventilated alveolus.
Anesthesia has become significantly safer over the past decades, especially during the establishment of artificial airway (Bainbridge et al., 2012). The application of visualization technology during airway management has made the intubation maneuver much safer (Lewis et al., 2016; Hoshijima et al., 2018). It was reported that the intubation duration was no more than 40 s when performed by experienced anesthesiologists in patients without difficult airway, and almost all the patients could be intubated at the first attempt (Li et al., 2017). Therefore, the classical ventilation methods (100% oxygen and ZEEP) already provide enough non-hypoxemic apnea duration for anesthesiologists to establish artificial airway in most circumstances. In recent years, there has been increasing evidence to suggest that the application of high FiO2 during mechanical ventilation can result in a variety of adverse effects (Meyhoff et al., 2012). Studies showed that the administration of pure oxygen after the administration of paralysis cause significantly more atelectasis formation than combined with air (Reber et al., 1996). As the air in the lungs is replaced by 100% oxygen during classical ventilation methods. Marco et al. reported that the atelectasis would develop as soon as the initiation of positive pressure ventilation, to a level of 4.1% ± 2.0% (Lundquist et al., 1995; Coussa et al., 2004). At the same time, the function residual capacity (FRC), which is closely related to the aerated lung volume, would also loss dramatically (Sreejit and Ramkumar, 2015). Moreover, Guniz et al. found that when compared to 40% FiO2, 80% FiO2 decreased expiratory tidal volumes and PaO2/FiO2 ratio, increase lactate levels and systematic oxidative stress, inhibiting antioxidant response (Koksal et al., 2016). It is also reported that high FiO2 ventilation may increase peripheral vascular and coronary artery vasoconstriction, decrease cardiac output (Harten et al., 2003). However, in patients with signs of difficult airways or any other reasons to require maximal safety during induction, 100% oxygen should always be preferable for anesthesiologists in order to secure the airway and prevent severe hypoxemia.
First, contradictory to classical techniques, preoxygenation was not carried out in our study to ensure equal oxygenation levels among each group prior to anesthesia induction. Of note, all patients were instructed to take five deep breaths before anesthesia induction to recruit the lungs and optimize the functional residual capacity. Besides, we prefilled the breathing circuit and face mask with oxygen to ensure effective face mask ventilation. Second, to minimize the incident of difficult intubation and ventilation, we eliminated patients with potential risks for difficult airway. The neck and mouth opening were evaluated before any procedures. Complete body examination also was completed before enrollment. During the induction period, in addition to the anesthesiologist in charge, there were also two additional physicians: the anesthesia resident, and the investigator, who is also a senior anesthesiologist. And other intubation tools or ventilation tools such as video stylet, flexible videoscope, and laryngeal mask were also available in the operating room. Third, during 90 s of face mask ventilation, all patients were subjected to two-handed mask ventilation with a “Guedel” style oropharyngeal airway placed. Fourth, a potential risk in our study is that face mask ventilation with PEEP is to expose a paralyzed patient to gastric insufflation thus increase the risk of regurgitation and aspiration.
First, we only tested two levels of PEEP (0 cmH2O and 6 cmH2O) in this study, the best PEEP during ventilation is still unknown. Second, the patients’ EtO2 in this study was monitored only visually by the attending anesthesiologist and the resident but it was not recorded during the face mask ventilation. Third, electric impedance tomography (EIT) and computed tomography (CT) were not used to monitor the amount of atelectasis before and after anesthesia induction.
In conclusion, the application of 6 cmH2O of PEEP during face mask ventilation of anesthesia induction could extend the non-hypoxemic apnea time, while significantly improving the PaO2/FiO2 ratio after ventilation when combined with a FiO2 of .6.
The raw data supporting the conclusion of this article will be made available by the authors, without undue reservation.
The studies involving human participants were reviewed and approved by Ethical Committee of Chinese PLA General Hospital. The patients/participants provided their written informed consent to participate in this study.
CZ, LB, WM and CL contributed to conception and design of the study. CH and XM performed the study. XP and YF organized the database. LM performed the statistical analysis. CH and XM wrote the first draft of the manuscript. CH, XM, CL, and XP wrote sections of the manuscript. All authors contributed to manuscript revision, read, and approved the submitted version.
This research was supported by the National Key Research and Development Program of China (grant number: 2018YFC2001900) and the National Natural Science Foundation of China (Grant No.81901096). This research did not receive any grant from funding agencies in the public or commercial sectors.
The authors declare that the research was conducted in the absence of any commercial or financial relationships that could be construed as a potential conflict of interest.
All claims expressed in this article are solely those of the authors and do not necessarily represent those of their affiliated organizations, or those of the publisher, the editors and the reviewers. Any product that may be evaluated in this article, or claim that may be made by its manufacturer, is not guaranteed or endorsed by the publisher.
Bainbridge D., Martin J., Arango M., Cheng D. (2012). Perioperative and anaesthetic-related mortality in developed and developing countries: A systematic review and meta-analysis. Lancet 380 (9847), 1075–1081. doi:10.1016/S0140-6736(12)60990-8
Barbosa F. T., Castro A. A., de Sousa-Rodrigues C. F. (2014). Positive end-expiratory pressure (PEEP) during anaesthesia for prevention of mortality and postoperative pulmonary complications. Cochrane Database Syst. Rev. 6, CD007922. doi:10.1002/14651858.CD007922.pub3
Coussa M., Proietti S., Schnyder P., Frascarolo P., Suter M., Spahn D. R., et al. (2004). Prevention of atelectasis formation during the induction of general anesthesia in morbidly obese patients. Anesth. Analg. 98 (5), 1491–1495. table of contents. doi:10.1213/01.ane.0000111743.61132.99
Gander S., Frascarolo P., Suter M., Spahn D. R., Magnusson L. (2005). Positive end-expiratory pressure during induction of general anesthesia increases duration of nonhypoxic apnea in morbidly obese patients. Anesth. Analg. 100 (2), 580–584. doi:10.1213/01.ANE.0000143339.40385.1B
Harten J. M., Anderson K. J., Angerson W. J., Booth M. G., Kinsella J. (2003). The effect of normobaric hyperoxia on cardiac index in healthy awake volunteers. Anaesthesia 58 (9), 885–888. doi:10.1046/j.1365-2044.2003.03333.x
Hedenstierna G., Tokics L., Scaramuzzo G., Rothen H. U., Edmark L., Ohrvik J. (2019). Oxygenation impairment during anesthesia: Influence of age and body weight. Anesthesiology 131 (1), 46–57. doi:10.1097/ALN.0000000000002693
Hoshijima H., Denawa Y., Tominaga A., Nakamura C., Shiga T., Nagasaka H. (2018). Videolaryngoscope versus macintosh laryngoscope for tracheal intubation in adults with obesity: A systematic review and meta-analysis. J. Clin. Anesth. 44, 69–75. doi:10.1016/j.jclinane.2017.11.008
Isono S. (2009). Obstructive sleep apnea of obese adults: Pathophysiology and perioperative airway management. Anesthesiology 110 (4), 908–921. doi:10.1097/ALN.0b013e31819c74be
Kim E. H., Lee J. H., Jang Y. E., Ji S. H., Cho S. A., Kim J. T., et al. (2021). Effect of positive end-expiratory pressure during anaesthesia induction on non-hypoxic apnoea time in infants: A randomised controlled trial. Eur. J. Anaesthesiol. 38 (10), 1012–1018. doi:10.1097/EJA.0000000000001400
Koksal G. M., Dikmen Y., Erbabacan E., Aydin S., Cakatay U., Sitar M. E., et al. (2016). Hyperoxic oxidative stress during abdominal surgery: A randomized trial. J. Anesth. 30 (4), 610–619. doi:10.1007/s00540-016-2164-7
Koo C. H., Park E. Y., Lee S. Y., Ryu J. H. (2019). The effects of intraoperative inspired oxygen fraction on postoperative pulmonary parameters in patients with general anesthesia: A systemic review and meta-analysis. J. Clin. Med. 8 (5), 583. doi:10.3390/jcm8050583
Lewis S. R., Butler A. R., Parker J., Cook T. M., Smith A. F. (2016). Videolaryngoscopy versus direct laryngoscopy for adult patients requiring tracheal intubation. Cochrane Database Syst. Rev. 11, CD011136. doi:10.1002/14651858.CD011136.pub2
Li Z. J., Lu K., Wang K., Zhao Y. Y., Huang X., Jin S. Q. (2017). Comparison of safe duration of apnea and intubation time in face mask ventilation with air versus 100% oxygen during induction of general anesthesia]. J. South. Med. Univ. 37 (12), 1643–1647. doi:10.3969/j.issn.1673-4254.2017.12.14
Lundquist H., Hedenstierna G., Strandberg A., Tokics L., Brismar B. (1995). CT-assessment of dependent lung densities in man during general anaesthesia. Acta Radiol. 36 (6), 626–632. doi:10.3109/02841859509176761
Merry A. F., Eichhorn J. H., Wilson I. H. (2009). Extending the WHO 'safe surgery saves lives' project through global oximetry. Anaesthesia 64 (10), 1045–1048. doi:10.1111/j.1365-2044.2009.06104.x
Meyhoff C. S., Jorgensen L. N., Wetterslev J., Christensen K. B., Rasmussen L. S., Group P. T. (2012). Increased long-term mortality after a high perioperative inspiratory oxygen fraction during abdominal surgery: Follow-up of a randomized clinical trial. Anesth. Analg. 115 (4), 849–854. doi:10.1213/ANE.0b013e3182652a51
Reber A., Engberg G., Wegenius G., Hedenstierna G. (1996). Lung aeration. The effect of pre-oxygenation and hyperoxygenation during total intravenous anaesthesia. Anaesthesia 51 (8), 733–737. doi:10.1111/j.1365-2044.1996.tb07885.x
Sreejit M. S., Ramkumar V. (2015). Effect of positive airway pressure during pre-oxygenation and induction of anaesthesia upon safe duration of apnoea. Indian J. Anaesth. 59 (4), 216–221. doi:10.4103/0019-5049.154998
Tagaito Y., Isono S., Remmers J. E., Tanaka A., Nishino T. (2007). Lung volume and collapsibility of the passive pharynx in patients with sleep-disordered breathing. J. Appl. Physiol. 103 (4), 1379–1385. doi:10.1152/japplphysiol.00026.2007
Keywords: positive end-expiratory pressure (PEEP), fraction of inspiration O2 (FiO2), preoxygenation, non-hypoxemic apnea time, mechanical ventilalion
Citation: Hao C, Ma X, Piao X, Fu Y, Ma L, Mi W, Berra L, Li C and Zhang C (2023) Effects of positive end-expiratory pressure and oxygen concentration on non-hypoxemic apnea time during face mask ventilation of anesthesia induction: A randomized controlled trial. Front. Physiol. 13:1090612. doi: 10.3389/fphys.2022.1090612
Received: 05 November 2022; Accepted: 12 December 2022;
Published: 09 January 2023.
Edited by:
Peter Markus Spieth, University Hospital Carl Gustav Carus, GermanyReviewed by:
Christopher Lotz, University Hospital Würzburg, GermanyCopyright © 2023 Hao, Ma, Piao, Fu, Ma, Mi, Berra, Li and Zhang. This is an open-access article distributed under the terms of the Creative Commons Attribution License (CC BY). The use, distribution or reproduction in other forums is permitted, provided the original author(s) and the copyright owner(s) are credited and that the original publication in this journal is cited, in accordance with accepted academic practice. No use, distribution or reproduction is permitted which does not comply with these terms.
*Correspondence: Changtian Li, bGljdGlvbjQ5OTVAMTYzLmNvbQ==; Changsheng Zhang, cG93ZXJ6Y3NAMTI2LmNvbQ==; Weidong Mi, d3dkZDE5NjJAMTYzLmNvbQ==
†These authors have contributed equally to this work
Disclaimer: All claims expressed in this article are solely those of the authors and do not necessarily represent those of their affiliated organizations, or those of the publisher, the editors and the reviewers. Any product that may be evaluated in this article or claim that may be made by its manufacturer is not guaranteed or endorsed by the publisher.
Research integrity at Frontiers
Learn more about the work of our research integrity team to safeguard the quality of each article we publish.