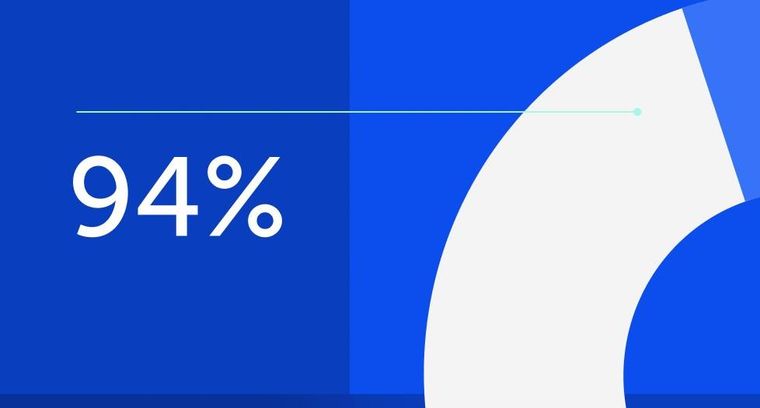
94% of researchers rate our articles as excellent or good
Learn more about the work of our research integrity team to safeguard the quality of each article we publish.
Find out more
ORIGINAL RESEARCH article
Front. Physiol., 16 December 2022
Sec. Avian Physiology
Volume 13 - 2022 | https://doi.org/10.3389/fphys.2022.1082009
This article is part of the Research TopicThe Association between Avian Physiology and Meat QualityView all 12 articles
A study was conducted to investigate the effects of tannic acid (TA) supplementation on growth performance, gut health, antioxidant capacity, gut microbiota, and meat yield and quality in broilers raised for 42 days. A total of 700 one-day-old male broiler chickens (Cobb500) were allocated into 5 treatments with 7 replicates of 20 birds per pen. There were five treatments: 1) tannic acid 0 (TA0: basal diet without TA); 2) tannic acid 0.25 (TA0.25: basal diet+0.25 g/kg TA); 3) tannic acid 0.5 (TA0.5: basal diet+0.5 g/kg TA); 4) tannic acid 1 (TA1: basal diet+1 g/kg TA); and 5) tannic acid 2 (TA2: basal diet+2 g/kg TA). The dietary phases included starter (D 0 to 18; crumble feed), grower (D 18 to 28; pellet feed), and finisher (D 28 to 42; pellet feed). On D 18, the supplementation of TA linearly reduced body weight (BW) and average daily feed intake (ADFI) (p < 0.05), and on D 28, the supplementation of TA linearly reduced BW, average daily gain (ADG), and feed conversion ratio (FCR) (p < 0.05). Relative mRNA expression of genes related to mucin production (MUC2), tight junction proteins (CLDN2 and JAM2), and nutrient transporters (B0AT1 and SGLT1) was linearly increased by the supplementation of TA (p < 0.05). The supplementation of TA tended to linearly increase the relative abundance of the family Enterobacteriaceae (p = 0.08) and quadratically increased the relative abundance of the families Lachnospiraceae and Ruminococcaceae in the cecal microbial communities (p < 0.05). On D 36, the ratio of the phyla Firmicutes and Bacteroidetes was quadratically reduced by the supplementation of TA (p < 0.05). On D 42, bone mineral density and the lean to fat ratio were linearly decreased by the supplementation of TA (p < 0.05). On D 43, total chilled carcass weight was linearly reduced (p < 0.05), and proportion of leg weight was increased by supplementation of TA (p < 0.05). The supplementation of TA linearly reduced pH of the breast meat (p < 0.05) and linearly increased redness (a*) (p < 0.05). Although the supplementation of TA positively influenced gut health and gut microbiota in the starter/grower phases, it negatively affected overall growth performance, bone health, and meat production in broilers on D 42.
In the past, antibiotic growth promoters (AGP) have been supplemented to broiler diets to enhance growth performance and gut health and to prevent diseases in broilers (Caly et al., 2015). Due to the public concerns about the spread of antibiotic resistant bacteria and their genes, there is a global movement to implement antibiotic-free production in the poultry industry (Haque et al., 2020). However, withdrawal of AGP without appropriate alternative strategies against bacterial infections could result in reduced production efficiency and broiler health and welfare issues by inducing severe microbial infection in broilers (Cervantes, 2015). It has been essential for the poultry industry to find appropriate bioactive compounds that can improve growth performance and gut health in antibiotic-free production. Diverse bioactive compounds, such as essential oils (Yang et al., 2021), amino acids (Teng et al., 2021), organic acids (Adil et al., 2010), plant extracts (Mogire et al., 2021; Yadav et al., 2022), and exogenous enzymes (Lu et al., 2020), have been studied and used as AGP alternatives in poultry production. Alternatives for AGP should be able to enhance growth performance, gut health, and meat production and quality of broilers and should be safe to the public and eco-friendly and cost-effective in broiler production (Yang et al., 2015).
Tannins, polyphenol compounds that can precipitate proteins, are considered as AGP alternatives in broiler production due to their effective antimicrobial, antioxidative, and anti-inflammatory effects in chickens (Choi and Kim, 2020). Tannic acid (TA), which is composed of 7–8 gallic acids molecules and one glucose molecule as a central core, is a standard of hydrolysable tannins and present in woods such as oak, chestnut, and acacia (Romani et al., 2006). Traditionally, TA was considered as an anti-nutritional factor due to its protein precipitation capacity, which can result in reduced nutrient digestibility and proteolytic activity in the liver of chickens (Marzo et al., 2002; Redondo et al., 2014). Many recent studies showed that the supplementation of TA at appropriate dosages (up to 2 g/kg) improved growth performance, gut health, immune system, and gut microbiota in broilers under non-challenge conditions and diverse challenging conditions (Eimeria spp., Salmonella spp., etc.) (Diaz Carrasco et al., 2018; Tonda et al., 2018; Ramah et al., 2020). Our previous study (Choi et al., 2022d) demonstrated that 0.5 g/kg TA increased activities of endogenous antioxidant enzymes, whereas higher than 1 g/kg TA exhibited antinutritional effects in broilers on D 21. However, it is still uncertain whether the supplementation of TA (up to 2 g/kg) would beneficially or negatively influence growth performance, gut health, antioxidant capacity, gut microbiota, and meat production and quality in broilers on D 42 (slaughter age). Therefore, this study was aimed to investigate the effects of TA supplementation (up to 2 g/kg TA) on growth performance, gut health, antioxidant capacity, gut microbiota, and meat yield and quality in broilers on D 42.
The current study was reviewed and approved by the Institutional Animal Care and Use Committee at the University of Georgia, Athens, GA. A total of 700 one-day-old Cobb 500 male broiler chickens were randomly allotted to 5 treatments with 7 replicates of 20 birds per pen in a completely randomized design. The five treatments included 1) tannic acid 0 (TA0; basal diet without TA); 2) tannic acid 0.25 (TA0.25; basal diet + 0.25 g/kg TA); 3) tannic acid 0.5 (TA0.5; basal diet + 0.5 g/kg TA); 4) tannic acid 1 (TA1; basal diet + 1 g/kg TA); and 5) tannic acid 2 (TA2; basal diet + 2 g/kg TA). The TA (> 99% purity; Chinese natural gall nuts) was purchased from Sigma-Aldrich Co. (St Louis, MO) and was included in the entire experimental period. Before TA was added to the basal diets, TA was premixed with 10 kg basal diets. The experiment period was divided into starter (D 0 to 18; crumble feed), grower (D 18 to D 28; pellet feed), and finisher (D 28 to 42; pellet feed) phases, and the diets were formulated to meet or exceed recommendation levels according to the Cobb 500 nutrient requirement guide (2018) (Table 1). Conditioning temperature was 80°C for the feed pelleting process. On D 28 to 35, all diets included 0.3% titanium dioxide (Acros Organics, Morris Plains, NJ) as an inert marker to determine nutrient digestibility. Birds were raised in floor pens (width: 1.52 m, length: 1.22 m, height: 0.61 m) equipped with one feeder and three drinker nipples per pen, and birds had free access to water and feed. Temperature and light were controlled in accordance with the recommendation of the Cobb 500 broiler management guide (2018). Body weight (BW) and feed disappearance were measured on D 18, 28, and 42 to calculate average daily gain (ADG), average daily feed intake (ADFI), and feed conversion ratio (FCR).
On D 18 and 36, one bird per pen was randomly selected and euthanized via cervical dislocation to collect samples of liver, intestinal tissue (mid-duodenum, mid-jejunum, and mid-ileum), and cecal content. All tissue samples were washed with PBS to remove remaining digesta and blood. Samples of liver, mid-jejunum tissue, and cecal content were snap-frozen and stored at –80°C for further analyses. For intestinal morphology, mid-duodenum, mid-jejunum, and mid-ileum samples were fixed in a 10% formaldehyde solution. On D 36, randomly selected four birds were euthanized, and digesta samples from 10 cm below Meckel’s diverticulum to the upper 10 cm of the ileo-cecal-colic junction were collected and oven-dried at 75°C until constant weight was achieved. On D 42, randomly selected one bird per pen was euthanized via cervical dislocation and scanned using dual-energy X-ray absorptiometry (DEXA, GE Healthcare, Madison, WI) to determine total tissue weight (g), bone mineral content (BMC; g), bone mineral density (BMD; g/cm2), lean weight (g), fat weight (g), body fat percentage (%), and lean:fat (g/g). On D 42, severity of foot pad dermatitis (FPD) was measured from all birds in each pen according to Eichner et al. (2007): score 0: no lesion; score 1: FPD covers less than 25% of the food pad; score 2: FPD covers 25%–50% of the food pad; and score 3: FPD covers more than 50% of the food pad. Both foot pads were checked in birds, and scores from both foot pads were averaged, and FPD incidence (%) was also calculated. Ammonia level (mg/kg) on the litter was measured using a Chillgard® RT Refrigerant Monitor (MSA, Cranberry Township, PA) connected to a HOBO® monitoring station (Onset, Bourne, MA) according to Aston et al. (2019).
Oven-dried feed (75°C till constant weight; 0.5 g) and ileal digesta (0.3 g) samples were ashed at 600°C overnight, and concentrations of titanium dioxide were determined according to Short et al. (1996). The concentration of crude protein (CP) was analyzed using nitrogen combustion analyses according to AOAC international (2000) analytical method 990.03. The crude fat (CF) was determined according to AOAC international (2000) analytical method 942.05. Apparent ileal digestibility (AID) of dry matter (DM), organic matter (OM), ash, CP, and CF was calculated according to Lin and Olukosi (2021).
After 72 h of fixation in 10% formalin solution, the fixed intestine samples were embedded in paraffin and cut into 4 μm, and the samples were stained with hematoxylin and eosin (H&E). The images of H&E-stained slides were taken using a microscope (BZ-X810; Keyence, Osaka, Japan). Five well-shaped villus and their corresponding crypts were selected per slide, and villus height (VH) and crypt depth (CD) were measured by using ImageJ (National Institutes of Health, Bethesda, MD). The VH to CD ratio (VH:CD) was calculated for each villi and crypt.
Around 100 mg of mid-jejunum (whole tissue) samples were homogenized in 1.8 ml PBS using a bead beater (Biospec Products, Bartlesville, OK). Afterwards, the samples were centrifuged at 12,000 × g for 15 min at 4°C, and the protein concentrations of the supernatants was determined using Pierce BCA protein assay kits according to the manufacturer’s instructions (Thermo Fisher Scientific, Waltham, MA). Activities of maltase and sucrase in the supernatants were analyzed according to the method of Lackeyram (2012). Briefly, 100 μl supernatants were mixed with 400 μl maltose (75 mM) and sucrose solution (75 mM), separately and incubated at 41°C for 30 min. Afterwards, the concentrations of glucose were determined using a Glucose Oxidase Reagent Set (Pointe Scientific, Canton, MI) according to the manufacturer’s protocol. To determine activities of lipase in the supernatants, the 10 times diluted supernatants (60 μl) were incubated with 1 mg/ml p-nitrophenyl palmitate solution (Sigma-Aldrich Co., St Louis, MO; 140 μl) at 41°C for 30 min according to the method of Elgharbawy et al. (2018). The activities of leucine aminopeptidase (LAP) were assayed by incubating 100 μl supernatant with 100 μl 1 mg/ml l-leucine-p-nitroanilide solution (Sigma-Aldrich Co., St Louis, MO) at 41°C for 30 min according to Maroux et al. (1973). To determine activities of alkaline phosphatase, the 20 μl supernatant (2 times dilution) and serum (10 times diluted) were incubated with 180 μl 10 mM p-nitrophenyl phosphate solution at 41°C for 60 min according to Lackeyram et al. (2010). The absorbance of the end products (p-nitrophenyl and p-nitroanilide) was determined at 400 nm by using a spectrophotometer (VICTOR Nivo, Perkin Elmer, Pontyclun, United Kingdom) and quantify using a prepared standard curve. The activities of the enzymes except alkaline phosphatase in the serum were expressed as their values per mg protein per min. The activities of serum alkaline phosphatase were expressed as their values per mL serum per min.
Approximately 100 mg of mid-jejunum (whole tissue) samples were homogenized using a bead beater (Biospec Products, Bartlesville, OK) in QIAzol lysis reagents (Qiagen, Valencia, CA). Afterwards, RNA was extracted according to the manufacturer’s protocol. RNA quantity and quality were measured by a NanoDrop 2000 spectrophotometer (Thermo Fisher Scientific). One microgram of RNA was used to synthesize the first-strand cDNA by using high-capacity cDNA synthesis kits (Applied Biosystems, Foster City, CA) according to the manufacturer’s instructions. The 20 μl cDNA was diluted with 80 μl water. Primers used in the study are listed in Table 2. Real-time reverse transcript (RT)-PCR was conducted using SYBR Green Master Mix with a Step Onethermocycler (Applied Biosystem). The final volume for PCR mixture was 10 μl which included 5 μl of SYBR Green Master Mix (Applied Biosystems), 1.5 μl of cDNA, 0.5 μl of forward and reverse primers (10 μM each), and 2.5 μl of water. The thermal cycle condition for all genes was 95°C denature for 10 min, 40 cycles at 95°C for 15 s and 60°C for 1 min, 95°C for 15 s, 60°C for 1 min, and 95°C for 15 s. After the PCR amplification, melting curve analysis and product size verification by gel electrophoresis were conducted to check the specificity of the PCR reactions. The geometric mean of Ct values of glyceraldehyde 3-phosphate dehydrogenase (GAPDH) and beta-actin were used as reference values to normalize all target genes’ mRNA abundance (Vandesompele et al., 2002). Relative mRNA abundance of target genes was calculated using the 2−ΔΔCT method, and the TA0 group was set as the control group (Livak and Schmittgen, 2001). Each sample was analyzed in duplicate, and the negative control, containing water instead of cDNA, was included in each run.
Approximately 100 mg of liver samples were homogenized using a bead beater (Biospec Products, Bartlesville, OK) in selected solution for each assay. Afterwards, the samples were centrifuged at 12,000 × g for 15 min at 4°C, and protein concentration of the supernatants were analyzed using Pierce BCA protein assay kits (Thermo Fisher Scientific) after 20-time sample dilution. The total antioxidant capacity (TAC) of the collected supernatant was analyzed using a commercial kit (QuantiCromAntioxidant Assay Kit, BioAssay Systems, Hayward, CA) after 2-time sample dilution. Concentrations of glutathione (GSH) and oxidized glutathione (GSSG) in the supernatant were analyzed using Caymans GSH assay kits (Cayman Chemical, Ann Arbor, MI) with 20- and 2-time sample dilutions, respectively. The activities of superoxide dismutase (SOD) in the supernatants were determined Caymans SOD assay kits (Cayman Chemical) after 400-time sample dilution. The TAC, concentrations of GSH and GSSG, and SOD activities were expressed as values per mg protein.
DNA was extracted from the cecal contents using QIAamp® DNA stool mini kits (Qiagen GmbH, Hilden, Germany) according to manufacturer’s protocol. After quality and quantity of extracted DNA were checked using a NanoDrop 2000 spectrophotometer (Thermo Fisher Scientific), the samples were sent to LC sciences (Houston, TX) for 16 s rRNA gene sequencing (Choi et al., 2022d). Qimme2 (version 2022.02) was used to process and analyze 16s rRNA gene sequences (Bolyen et al., 2019). According to Choi and Kim (2022), 16s rRNA sequences were processed. The sampling depth for both D 18 and 36 time points was set as 45,000. By using Qiime2’s built-in functions, alpha diversity, beta diversity, and phylum and family level composition were analyzed and presented.
On D 42, three birds per pen were randomly selected from each pen for processing, and feed was removed from the pen for 12 h (Wang J. et al., 2020). On D 43, the selected birds were individually weighed and transferred to the processing plant at the University of Georgia. Birds were shackled, electrically stunned, bled, scalded, and defeathered. Following head and feet removal, the carcasses were eviscerated. Weights of the hot carcass and abdominal fat collected from fat around cloaca, bursa of Fabricius, gizzard, and proventriculus (Castro et al., 2019) were recorded. The carcasses were rinsed and chilled in ice-cold water at 1°C for 4 h. Legs, breast muscle, tender, wings, and skeleton were separated by trained personnel, and their weights were recorded. Breast myopathies and quality defects including white striping [score 0 (normal), 1, 2, and 3 (severe)], woody breast [score 1 (normal), 2, and 3 (severe)], spaghetti meat [score 0 (normal), 1, and 2 (severe)], and petechial hemorrhagic lesions [score 0 (normal), 1, 2, and 3 (severe)] were assessed by a trained expert according to published criteria (Kuttappan et al., 2017; Pang et al., 2020; Baldi et al., 2021; Prisco et al., 2021).
Breast muscles from two birds per pen were stored at 1°C overnight for further meat quality analyses. Color and pH of the breast muscles were analyzed according to Brambila et al. (2018) with the modification. Color indicators including lightness (L*), redness (a*), and yellowness (b*) were determined in duplicate on the dorsal surface of breast meat by a Minolta Spectrophotometer CM-700 days (Konica Minolta Inc., Ramsey, NJ). Meat pH was analyzed (one measurement per fillet) by using a Thermo Scientific Orion Star™ A221 portable pH meter with a spear tipped probe (Thermal Scientific Orion 8163BNWP) (Thermo Fisher Scientific, Waltham, MA 02451, United States) that penetrated the cranial end of the intact breast muscle. Drip loss was analyzed by using a EZ-driploss method (Kaić et al., 2021). One cylindrical muscle core (2.5 cm diameter) was removed from the cranial side of the breast meat and trimmed to a similar height. The cores were weighed and placed in individual EZ containers (Danish Meat Research Institute, Taastrup, Denmark). The sealed containers were then stored in a refrigerator at 4°C. The samples were reweighted (approximately 7 g–8 g) after 48 h to determine drip loss (%). For thawing loss (%), intact breast samples were weighed and individually sealed in cooking bags before frozen at −20°C. The frozen samples were stored for 2 weeks at −20°C and were thawed at 4°C overnight and weighed again after liquid was removed. Cooking was performed by using a Henny Penny MCS-6 combi oven (Henny Penny Corp. Eaton, OH) on the Tender Steam setting at 84°C. Fillets were cooked ventral side up in stainless steel oven pans to an endpoint temperature of 74°C in the thickest part of the fillet (Brambila et al., 2018). A thermocouple system with hypodermic needle microprobes (Physitemp Instruments, Inc., Clifton, NJ) was used to monitor temperature. The samples were reweighed after the liquid was removed.
SAS (version 9.4; SAS Inst. Inc., Cary, NC) and GraphPad Prism (Version 9.1.0; GraphPad Software, San Diego, CA) were used for statistical analyses and graph construction. Treatment groups were compared using PROC MIXED followed by the Tukey’s individual comparison test. Orthogonal polynomial contrasts were conducted to assess the significance of linear or quadratic effects of the supplementation of TA in broilers. Pen was considered as the experimental unit, and values of individual birds in the same pen were averaged for meat analyses. Breast meat myopathy score and FPD score and incidence were analyzed using the Kruskal–Wallis test followed by the Dwass–Steel–Critchlow–Fligner post hoc test. Significance level was set at p < 0.05, and tendencies were also presented at 0.05 < p ≤ 0.10 (Choi et al., 2021).
Results of the growth performance are presented in Table 3. In the starter phase, the TA2 group had significantly lower BW and ADG compared to the TA0 group, and the supplementation of TA linearly decreased BW and ADG in broiler chickens (p < 0.01). The ADFI was also linearly reduced by the supplementation of TA in broilers in the starter phase (p < 0.05). In the grower phase, the supplementation of TA linearly reduced BW and ADG and linearly and quadratically increased FCR of broilers (p < 0.01). The TA2 group had significantly lower BW compared to the TA0 group. The TA2 group had significantly lower ADG compared to the TA0 and TA1 groups (p < 0.05). The TA0.5 group had a significantly lower ADG compared to the TA0 (p < 0.05). The TA2 group had the highest FCR (p < 0.05) among the treatment groups, and the TA1 group had significantly lower FCR compared to the TA0.5 group. No statistical differences were observed in the growth performance parameters of the finisher phase (p > 0.1). In the whole phase, the supplementation of TA linearly increased FCR (p < 0.01), and the TA2 group had significantly higher FCR compared to the TA0 group.
TABLE 3. Growth performance parameters including body weight (BW, g), average daily gain (ADG, g/d), average daily feed intake (ADFI, g/d), and feed conversion ratio (FCR, g/g) in broilers fed diets supplemented with tannic acid on D 42a.
As shown in Table 4, the TA0 group had significantly higher jejunal CD compared to the TA supplemented groups, and the supplementation of TA linearly (p < 0.01) and quadratically (p < 0.05) reduced jejunal CD on D 18. The TA0.5 and TA2 groups had greater jejunal VH:CD compared to the TA0 group (p < 0.05), and the supplementation of TA linearly increased jejunal VH:CD. The supplementation of TA tended to linearly decrease ileal VH (p = 0.075). The TA0.25 group tended to have lower ileal CD compared to the TA0 group (p = 0.057). The supplementation of TA quadratically decreased ileal CD and increased ileal VH:CD (p < 0.05). There were no statistical differences in the intestinal morphology on D 36 (p > 0.1).
TABLE 4. Duodenal, jejunal, and ileal morphology parameters including villus height (VH, µm), crypt depth (CD, µm), and VH:CD in broilers fed diets supplemented with tannic acid on D 18 and 36a.
As shown in Table 5, the supplementation of TA quadratically increased sucrase activities (p < 0.05) and quadratically decreased lipase activities in the jejunum tissue (p < 0.05). On D 36, jejunal lipase activities were quadratically decreased by the supplementation of TA (p < 0.05). However, no differences were observed in the activities of jejunal sucrase, LAP, intestinal alkaline phosphatase (IAP), and serum alkaline phosphatase (SAP) (p > 0.1).
TABLE 5. Activities of jejunal brush border digestive enzymes including maltase (nmol glucose released/mg protein/min), sucrase (nmol glucose released/mg protein/min), leucine aminopeptidase (LAP; nmol p-nitroaniline liberated/mg protein/min), intestinal alkaline phosphatase (IAP; μmol p-nitrophenol liberated/mg protein/min), lipase (mmol p-nitrophenyl phosphate liberated/mg protein/min), and serum alkaline phosphatase (SAP; μmol p-nitrophenol liberated/ml serum/min) in broilers fed diets supplemented with tannic acid on D 18 and 36a.
As shown in Table 6, the supplementation of TA linearly (p < 0.01) and quadratically (p < 0.05) increased AID of DM, OM, and CP on D 36. The TA0.25, TA0.5, TA1, and TA2 groups had significantly higher AID of DM compared to the TA0 group (p < 0.01). The AID of OM was significantly lower in the TA0 group compared to the TA0.25, TA0.5, TA1, and TA2 groups (p < 0.05). The TA2 group had significantly AID of ash compared to the TA0 group, and the supplementation of TA linearly increased AID of ash (p < 0.01). The TA0 group had the lowest AID of CP among the treatments (p < 0.05). No differences were observed in the AID of CF among the treatments (p > 0.1).
TABLE 6. Apparent ileal digestibility (%) of dry matter (DM), organic matter (DM), organic matter (OM), ash, crude protein (CP), and crude fat (CF) in broilers fed diets supplemented with tannic acid on D 18 and 36a.
Relative mRNA expression of genes related to tight junction proteins and nutrient transporters in the jejunum is presented in Table 7. On D 18, the TA1 group had significantly higher relative mRNA expression of zonula occludens 2 (Z O 2) compared to the TA0, TA0.25 and TA0.5 groups, and the supplementation of TA quadratically increased relative mRNA expression of Z O 2 (p < 0.05). The supplementation of TA linearly increased relative mRNA expression of claudin 2 (CLDN2; p < 0.01) and junctional adhesion molecule 2 (JAM2; p < 0.05). The TA2 group had significantly higher relative mRNA expression of mucin 2 (MUC2) compared to the TA0.25 group (p < 0.05), and the supplementation of TA linearly increase relative mRNA expression of MUC2 (p < 0.05). The TA1 group had significantly higher relative mRNA expression of sodium-dependent neutral amino acid transporter (B0AT1) compared to the TA0.25 group (p < 0.05), and the supplementation of TA linearly increase relative mRNA expression of B0AT1 (p < 0.05). The supplementation of TA tended to modulate (p = 0.061) and quadratically increased relative mRNA expression of peptide transporter 1 (PepT1) (p < 0.05). The TA1 and TA2 groups had significantly higher relative mRNA expression of sodium glucose cotransporter 1 (SGLT1) compared to the TA0.25 group, and the supplementation of TA linearly increased relative mRNA expression of SGLT1 (p < 0.01). The TA1 group had significantly higher relative mRNA expression of excitatory amino acid transporter 3 (EAAT3) compared to the TA0, TA0.25, and TA0.5 groups, and the supplementation of TA quadratically increased relative mRNA expression of EAAT3 (p < 0.05). The supplementation of TA tended to modulate (p = 0.064) and quadratically increased relative mRNA expression of mucin 2 (MUC2) on D 36 (p < 0.05). However, no differences in relative mRNA expression of tight junction proteins and nutrient transporters were observed among the treatments on D 36 (p > 0.1).
TABLE 7. Relative mRNA expression of gene associated with tight junction proteins and nutrients transporters in broilers fed diets supplemented with tannic acid on D 18 and 36a.
The supplementation of TA tended to modulate TAC in the liver on D 36 (p = 0.073). No differences were observed in concentrations of GSH and GSSG and activities of SOD in the liver on D 18 and 36 (Table 8; p > 0.1).
TABLE 8. Total antioxidant capacity (TAC; µM Trolox Equivalents/mg protein), concentrations of glutathione (GSH; µM/mg protein) and oxidized glutathione (GSSG; µM/mg protein), and activities of superoxide dismutase (SOD; U/mg protein) in broilers fed diets supplemented with tannic acid on D 18 and 36a.
Alpha diversity indices including faith’s phylogenetic diversity, observed features, pielou evenness, and shannon entropy in the cecal bacterial communities on D 18 and 36 are shown in Figure 1. On D 18, the TA1 and TA2 groups had significantly lower faith’s phylogenetic diversity (communities’ evolutionary distance) compared to the TA0 group (p < 0.05), and the supplementation of TA linearly reduced faith’s phylogenetic diversity and observed features (richnesss) in the cecal bacterial communities (p < 0.05). The supplementation of TA tended to linearly reduce shannon entropy (richness and evenness) in the cecal bacterial communities (p = 0.059). On D 36, the TA2 group had lower faith’s phylogenetic diversity and observed features compared to TA0, TA0.25, and TA0.5 groups. The supplementation of TA linearly reduced faith’s phylogenetic diversity (p < 0.01), observed features (p < 0.01), pielou evenness (evenness; p < 0.05), and shannon entropy (p < 0.01) in the cecal bacterial communities.
FIGURE 1. Alpha diversity parameters of the cecal microbial communities in the TA0 (tannic acid 0; basal diet without TA), TA0.25 (tannic acid 0.25; basal diet + 0.25 g/kg TA), TA0.5 (tannic acid 0.5; basal diet + 0.5 g/kg TA), TA1 (tannic acid 1; basal diet + 1 g/kg TA), and TA2 (tannic acid 2; basal diet + 2 g/kg TA) groups on D 18 and 36. All treatment groups (7 replicates per treatment) were compared using PROC MIXED followed by the Tukey’s individual comparison test. Different letters in the same row means significant differences (p < 0.05) among the treatments. Orthogonal polynomial contrasts were used to evaluate the significance of linear or quadratic effects of the supplementation of TA in broilers.
As shown in Figure 2, the TA1 group had significantly greater unweighted unifrac distance (the sum of the branch length without considering bacterial abundance) compared to the TA0 group on D 18. The TA0.5, TA1, and TA2 groups had significantly greater unweighted unifrac distance compared to the TA0.25 group. The TA0.25 group had significantly greater unweighted unifrac distance compared to the TA0.5 group (p < 0.05). The TA0.5 and TA2 groups had significantly greater weighted unifrac distance (the sum of the branch length with considering bacterial abundance) compared to the TA0 group. The TA0.5 and TA2 groups had significantly greater weighted unifrac distance compared to the TA0.25 group. On D 36, TA0.25 had significantly lower unweighted unifrac distance compared to the TA0 group. The TA2 group had significantly greater unweighted unifrac distance compared to the TA0.25 and TA0.5 groups. The TA0, TA0.25, and TA0.5 groups had significantly higher unweighted unifrac distance compared to the TA2 group. The TA1 and TA2 groups had significantly greater weighted unifrac distance compared to the TA0 group. The TA2 group had significantly higher weighted unifrac distance compared to the TA0.25 group. However, no visual differences were observed in the beta diversity indices including weighted and unweighted emperor on D 18 and 36 (Figure 3).
FIGURE 2. Beta diversity indices including unweighted and weighted unifrac of the cecal microbial communities in the TA0 (tannic acid 0; basal diet without TA), TA0.25 (tannic acid 0.25; basal diet + 0.25 g/kg TA), TA0.5 (tannic acid 0.5; basal diet + 0.5 g/kg TA), TA1 (tannic acid 1; basal diet + 1 g/kg TA), and TA2 (tannic acid 2; basal diet + 2 g/kg TA) groups on D 18 and 36. All treatment groups (7 replicates per treatment) were compared using PROC MIXED followed by the Tukey’s individual comparison test. Orthogonal polynomial contrasts were used to evaluate the significance of linear or quadratic effects of the supplementation of TA in broilers.
FIGURE 3. Visualized beta diversity parameters including unweighted and weighted unifrac of the cecal microbial communities in the TA0 (tannic acid 0; basal diet without TA), TA0.25 (tannic acid 0.25; basal diet + 0.25 g/kg TA), TA0.5 (tannic acid 0.5; basal diet + 0.5 g/kg TA), TA1 (tannic acid 1; basal diet + 1 g/kg TA), and TA2 (tannic acid 2; basal diet + 2 g/kg TA) groups on D 18 and 36. All treatment groups (7 replicates per treatment) were compared using PROC MIXED followed by the Tukey’s individual comparison test. Orthogonal polynomial contrasts were used to evaluate the significance of linear or quadratic effects of the supplementation of TA in broilers.
As shown in Figure 4, the relative abundance of the phylum Actinobacteria was linearly increased by the supplementation of TA on D 18. On D 36, the relative abundance of the phylum Firmicutes was quadratically reduced by the supplementation of TA, and the TA1 group had significantly lower relative abundance of the phylum Firmicutes compared to the TA0 group. The relative abundance of the phylum Bacteroidetes was linearly (p < 0.05) and quadratically (p < 0.05) increased by the supplementation of TA. The supplementation of TA quadratically decreased the ratio of the phyla Firmicutes and Bacteroidetes (p < 0.05).
FIGURE 4. Phylum-level composition of the cecal microbial communities in the TA0 (tannic acid 0; basal diet without TA), TA0.25 (tannic acid 0.25; basal diet + 0.25 g/kg TA), TA0.5 (tannic acid 0.5; basal diet + 0.5 g/kg TA), TA1 (tannic acid 1; basal diet + 1 g/kg TA), and TA2 (tannic acid 2; basal diet + 2 g/kg TA) groups on D 18 and 36. All treatment groups (7 replicates per treatment) were compared using PROC MIXED followed by the Tukey’s individual comparison test. Orthogonal polynomial contrasts were used to evaluate the significance of linear or quadratic effects of the supplementation of TA in broilers.
As shown in Figure 5, the supplementation of TA tended to linearly reduce the relative abundance of the family Enterobacteriaceae (p = 0.068) and tended to linearly increase the relative abundance of the family Planococcaceae (p = 0.071) on D 18. The relative abundance of the families Lachnospiraceae and Ruminococcaceae was quadratically increased by the supplementation of TA. On D 36, the supplementation of TA linearly decreased the relative abundance of the families Christensenellaceae and Erysipelotrichaceae (p < 0.05). The supplementation of TA linearly increased the relative abundance of the family Bacillaceae (p < 0.01). The supplementation of TA linearly increased the relative abundance of the family Lachnospiraceae (p < 0.05) and tended to quadratically increased the relative abundance of the family Lachnospiraceae (p = 0.051). The TA2 group had significantly higher relative abundance of the family Lachnospiraceae compared to the TA0.25 and TA0.5 groups.
FIGURE 5. Family-level composition of the cecal microbial communities in the TA0 (tannic acid 0; basal diet without TA), TA0.25 (tannic acid 0.25; basal diet + 0.25 g/kg TA), TA0.5 (tannic acid 0.5; basal diet + 0.5 g/kg TA), TA1 (tannic acid 1; basal diet + 1 g/kg TA), and TA2 (tannic acid 2; basal diet + 2 g/kg TA) groups on D 18 and 36. All treatment groups (7 replicates per treatment) were compared using PROC MIXED followed by the Tukey’s individual comparison test. Orthogonal polynomial contrasts were used to evaluate the significance of linear or quadratic effects of the supplementation of TA in broilers.
There were no differences in litter ammonia concentrations among the treatments on D 42 (Figure 6). The TA1 group had a significantly higher FPD score compared to the TA2 group on D 42. However, no differences were observed in the incidence of FPD among the treatments on D 42 (p > 0.1).
FIGURE 6. Litter ammonia concentration (mg/kg) and foot pad dermatitis score and incidence (%) in the TA0 (tannic acid 0; basal diet without TA), TA0.25 (tannic acid 0.25; basal diet + 0.25 g/kg TA), TA0.5 (tannic acid 0.5; basal diet + 0.5 g/kg TA), TA1 (tannic acid 1; basal diet + 1 g/kg TA), and TA2 (tannic acid 2; basal diet + 2 g/kg TA) groups on D 42. For litter ammonia concentration, all treatment groups (7 replicates per treatment) were compared using PROC MIXED followed by the Tukey’s individual comparison test, and different letters in the same row means significant differences (p < 0.05) among the treatments. Orthogonal polynomial contrasts were used to evaluate the significance of linear or quadratic effects of the supplementation of TA. Foot pad dermatitis score were analyzed using the Kruskal–Wallis test followed by the Dwass–Steel–Critchlow–Fligner post hoc test.
The supplementation of TA linearly reduced BMD (p < 0.01) and BMC (p < 0.05), and the TA2 group tended to have lower BMD (p = 0.051) and had significantly lower BMC (p < 0.05) compared to the TA0 group (p < 0.05) on D 42 (Table 9). The body fat percentage was linearly increased by the supplementation of TA (p < 0.05), and the supplementation of TA tended to reduce lean weight (p = 0.065). The lean:fat was linearly reduced by the supplementation of TA (p < 0.05).
TABLE 9. Bone health parameters including bone mineral content (BMC; g), bone mineral density (BMD; g/cm2) and body composition parameters including tissue weight (g), lean weight (g), fat weight (g), body fat percentage (%), and lean:fat (g/g) in broilers fed diets supplemented with tannic acid on D 42.
On D 43, hot weight was linearly decreased by the supplementation of TA (p < 0.05) (Table 10). The supplementation of TA tended to linearly increase abdominal fat weight (p = 0.077) and linearly increased abdominal fat percentage (p < 0.05). Proportion of leg weight was increased by the supplementation of TA (p < 0.05).
TABLE 10. Hot weight, abdominal fat (g and %) weight and meat yield in broilers fed diets supplemented with tannic acid on D 43a.
No differences were observed in average breast muscle myopathy scores for white striping, woody breast, spaghetti meat, or hemorrhagic lesions as shown in Figure 7 (p > 0.1). The supplementation of TA linearly reduced pH of the breast meat (p < 0.05; Table 11) and linearly increased redness (a*) (p < 0.01). The TA2 group had significantly greater redness value compared to the TA0 group. The yellowness (b*) tended to be increased due to the supplementation of TA (p = 0.086). The supplementation of TA quadratically modulated cooking loss (p < 0.05) in the breast meat, and the TA2 group tended to have lower cooking loss compared to the TA1 group (p = 0.054).
FIGURE 7. Breast muscle myopathies including white striping, woody breast, spaghetti meat, and hemorrhagic lesion in the TA0 (tannic acid 0; basal diet without TA), TA0.25 (tannic acid 0.25; basal diet + 0.25 g/kg TA), TA0.5 (tannic acid 0.5; basal diet + 0.5 g/kg TA), TA1 (tannic acid 1; basal diet + 1 g/kg TA), and TA2 (tannic acid 2; basal diet + 2 g/kg TA) groups on D 42. There were 21 replicates per treatment and core for each parameter was analyzed using the Kruskal–Wallis test followed by the Dwass–Steel–Critchlow–Fligner post hoc test.
TABLE 11. The pH, meat color (L*, a*, b*), drip loss (%), thawing loss (%), and cooking loss (%) in the breast meat of broilers fed diets supplemented with tannic acida.
Our previous study showed that higher than 1 g/kg TA exhibited antinutritional effects to reduce growth performance, whereas 0.5 g/kg TA increased antioxidant capacity in broilers on D 21 (Choi et al., 2022d). The supplementation of TA (0.5 g/kg–2.75 g/kg) improved gut barrier integrity and decreased oocyst shedding in broilers infected with Eimeria maxima (Choi et al., 2022c). The supplementation of TA (1 g/kg–2 g/kg) enhanced growth performance and gut health via antimicrobial and immunostimulatory effects in broilers infected with Salmonella Typhimurium (Choi et al., 2022a). Based on our previous studies, we aimed to evaluate the efficacy of the supplementation of TA in broilers raised for 42 days in floor pens in the current study to simulate actual conditions of broiler production. Sampling points were determined at D 18 and D 36 to represent starter/grower phase and finisher phase, respectively, in the current study. Therefore, the purpose of the study was to investigate the effects of the TA supplementation (up to 2 g/kg TA) on growth performance, intestinal morphology, activities of brush border digestive enzymes, AID of nutrients, relative mRNA expression of tight junction proteins and nutrient transporters, liver antioxidant capacity, bone health, body composition, and meat yield and quality in broilers on D 42.
In the current study, diets were crumbled and pelleted in the starter and grower/finisher phases, respectively. Almost all commercial broiler feeds are crumbled or pelleted in current broiler production (Brickett et al., 2007). The pelleting processing includes steaming (e.g., conditioning) with high temperature and pelleting (e.g., agglomeration) to produce large particles from small particles (Abdollahi et al., 2013). In these harsh conditions, stability and molecular or physical traits of feed additives can be altered (Choi et al., 2020a). Kim et al. (2010) reported that thermal process (e.g., autoclave heat) improved antioxidant capacity and antimicrobial effects of TA, and our unpublished data showed that pelleting temperature (80°C) improved antimicrobial effects of TA against S. Typhimurium in vitro conditions. However, steaming and agglomeration during feed processing may induce interactions of TA and nutrients (e.g., proteins, polysaccharides, etc.), which can decrease nutrient utilization in the gastrointestinal tract of chickens.
In the current study, supplementation of TA linearly reduced feed intake of broiler chickens in the starter phase while supplementation of TA did not influence feed intake in the grower and finisher phases. Tannins are known to induce astringent taste by forming complexes with salivary proline-rich proteins, which can decrease feed palatability and feed intake in animals (Treviño et al., 1992; Lee et al., 2010). In the starter and grower phases, BW and feed efficiency were linearly reduced, but no statistical differences were observed in the finisher phase in broilers fed dietary TA in the current study. These results is in consistent with our previous study suggested that young broilers are less tolerant to the intake of TA (Choi et al., 2022b). These results had different trends from our previous studies as follows. Choi et al. (2022d) reported that higher than 1 g/kg TA started to linearly reduce BW of broilers on D 21. However, growth retardation effects of TA were exhibited from 0.25 g/kg TA in the current study. Potentially, the pelleting process may have induced the interaction between TA and dietary nutrients (e.g., proteins). Although AID was not measured on D 18 in the current study, nutrient digestibility would have been severely decreased by the supplementation of TA. However, the supplementation of TA improved AID of DM, OM, ash, and CP on D 36 potentially as compensation effects and did not alter growth performance in the finisher phase of the current study, indicating that older birds have more tolerance to the TA supplementation. Potentially, mature gastrointestinal tracts (e.g., lower pH and higher pancreatic enzymes) may have hydrolyzed TA-nutrient complex in broilers on D 36 (Adamczyk et al., 2011). In contrast, a previous study by Tonda et al. (2018) reported that the supplementation of TA extract improved BWG and feed efficiency in cocci-vaccinated (live vaccine) broilers fed pelleted feed on D 0 to 21. This would be because live vaccines are known to spread coccidiosis in a flock and can decrease growth performance, which can provide challenging conditions to chickens, and this indicates the supplementation of TA extract could be effective in challenging conditions (Greif, 2000). However, the basal diets included a coccidiostat (monensin sodium; 500 mg/kg) to exclude the anti-coccidial effects of TA and was conducted in a hygienic laboratory scale facility, and therefore there would be limited gap to improve growth performance of broilers, which potentially explains reduced or maintained growth performance in broilers supplemented with TA in the current study.
On D 18, the TA supplementation linearly increased jejunal VH:CD and quadratically decreased ileal VH:CD along with reduced CD in the current study. Increased VH:CD indicates augmented nutrient digestion and absorption in chickens (Abd El-Hack et al., 2020). However, if increased VH:CD was accompanied with decreased CD, it cannot be considered as beneficial effects because deeper CD suggests more proliferation and differentiation of stems cells, which would move to the tip of the villus (Liu et al., 2020). Potentially, the TA supplementation caused an impairment in the development of intestinal morphology by decreasing nutrient utilization via forming complex with nutrients (e.g., proteins) (Shinde et al., 2015).
Activities of sucrase, a brush border digestive enzyme, in the jejunum tissue were quadratically increased by the TA supplementation on D 18 in the current study, which suggests that appropriate dosages of TA can still improve gut development in broilers (Yang et al., 2008). Moreover, relative mRNA expression of MUC2 and nutrients transporters including B0AT1, SGLT1, PepT1, and EAAT3 were linearly and quadratically increased by the TA supplementation. These data indicate that nutrient utilization capacity of the jejunum could be enhanced by the TA supplementation, but limited availability of nutrients due to interactions between TA and nutrients would be the main factor to decrease growth performance of broilers in the starter and grower phases. Otherwise, reduced availability of nutrients for intestinal absorption due to the formation of TA-nutrient complexes in the luminal side may have increased mRNA expression of nutrients as a resistant reaction to increase nutrient absorption in the gastrointestinal tract (Pinheiro et al., 2013).
Tight junction proteins and MUC2 are closely associated with gut barrier integrity of broilers (Choi et al., 2020b). In the present study, the TA supplementation linearly and quadratically increased relative mRNA expression of genes related to gut barrier integrity including ZO2, CLDN2, JAM2, and MUC2 in the jejunum. According to our previous study, the TA supplementation decreased gut permeability in broilers infected with E. maxima (Choi et al., 2022c). A previous study by Yu et al. (2020) reported that the supplementation of TA improved gut barrier integrity in weaned piglets. These results suggest that the supplementation of TA has potential to increase gut barrier integrity in broilers.
There were no differences in TAC, concentrations of GSH and GSSG, activities of SOD in the liver on D 18 and 36 among the treatments in the present study. Many in vitro studies showed that TA, a polyphonic compound, has strong antioxidant capacity (Andrade Jr et al., 2005; Gülçin et al., 2010). However, direct antioxidant effects of TA in the chickens were in question. This is because TA should stay inside of the chicken body for a sufficient time by maintaining appropriate forms to exhibit antioxidant capacity (Karakaya, 2004). Deposition of TA in the internal organs (e.g., liver) in broiler chickens should be further investigated. Choi et al. (2022d) showed that the supplementation of TA at 0.5 g/kg indirectly improved antioxidant system by enhancing activities of SOD in the liver. The differences would be originated from the pelleting process, which may reduce bioavailability of TA by forming TA-nutrient complexes. However, under the heat stress condition, the supplementation of TA (10 g/kg) showed potential to improve antioxidant capacity in broilers (Ebrahim et al., 2015).
In the present study, the supplementation of TA linearly decreased alpha diversity indices including faith’s phylogenetic diversity (communities’ evolutionary distance; D 18 and 36), observed features (richness; D 18 and 36), pielou evenness (evenness; D 36), and shannon entropy (richness and evenness; D 36). While it is still controversial, lower alpha diversity may indicate less stable and unmature microbial communities in the gastrointestinal tract of animals (Ebrahim et al., 2015). Moreover, beta diversity indices (unweighted and weighted unifrac) showed that different dosages of TA could modulate cecal microbial communities in broilers.
The relative abundance of the phylum Actinobacteria was linearly increased by the supplementation of TA on D 18 in the current study. The phylum Actinobacteria includes Bifidobacteria spp., which can improve gut barrier integrity and immune system of animals and is considered as a beneficial phylum in animals (Binda et al., 2018). On D 18, the supplementation of TA linearly reduced relative abundance of the family Enterobacteriaceae, which includes diverse pathogens such as Salmonella spp., Shigella, Escherichia coli, etc. Consistently, our previous study reported that the TA supplementation reduced cecal Salmonella Typhimurium load in the starter phase of broilers (Choi et al., 2022a). Moreover, the TA supplementation quadratically increased the relative abundance of the families Lachnospiraceae and Ruminococcaceae, which have an important role in maintain gut homeostasis by producing volatile fatty acid via fiber degradation (Biddle et al., 2013). Consistently, Koo and Nyachoti (2019) reported that the TA supplementation enhanced cecal volatile fatty acid production in pigs. However, on D 36, a ratio of the phyla Firmicutes and Bacteroidetes was quadratically reduced by the supplementation of TA in the current study. The lower ratio of the phyla Firmicutes and Bacteroidetes suggests a lower capacity of fiber degradation and production of short chain fatty acids, important energy sources for the host animals (Singh et al., 2012). In the current study, the TA supplementation linearly decreased the relative abundance of the families Christesenellaceae and Erysipelotrichaceae, which have an important role in fiber degradation to produce short chain fatty acids (Wasti et al., 2021). However, the TA2 group significantly increased the relative abundance of the family Lachnospiraceae compared to the TA0.25 and TA0.5 groups, and the TA supplementation linearly increased the relative abundance of the family Bacillaceae, which are positively correlated with growth performance and feed efficiency (Moula et al., 2018). While the supplementation of TA reduced the relative abundance of the families Christensenellaceae and Erysipelotrichaceae, the TA supplementation still increased the relative abundance of the families Lachnospiraceae and Bacillaceae in the current study.
Ammonia (NH3) in poultry houses can negatively affect the health of chickens and humans as well as harm the environment (Naseem and King, 2018). Chickens synthesize uric acid as the end product of purine and protein metabolism, and uric acid is converted into ammonia via microbial fermentation in the ceca or in the litter (Kim and Patterson, 2003a; b; Naseem and King, 2018). In the current study, we hypothesized that litter ammonia concentration could be reduced by the supplementation of TA because the TA supplementation increased AID of CP on D 36. Crude protein digestibility is closely associated with litter ammonia concentration (Brink et al., 2022). Moreover, Arzola-Alavarez et al. (2020) reported that the addition of pine bark tannin in the litter reduced ammonia accumulation in the poultry litter. Unabsorbed TA could be excreted to the litter and potentially modulate ammonia concentration in the litter. However, no differences were observed in the litter ammonia on D 42. The TA1 group had a significantly higher FPD score compared to the TA2 group, which implies that the supplementation of TA can modulate FPD score in broilers. Litter ammonia and FPD are closely associated (Youssef et al., 2011), and our current study also showed that litter ammonia and FPD score had similar trends. Otherwise, BW could simply affect litter ammonia because bigger birds would excrete more manure in the litter. The TA1 group had numerically close BW compared to the TA0 group, whereas the TA2 group had the numerically lowest BW on D 42 in the current study. These results may explain the trends of litter ammonia and FPD score of broilers fed diets supplemented with TA in the current study.
Our previous study showed that there was only a linear tendency (0.05 < p ≤ 0.10) to reduce bone health parameters including BMD and BMC in broilers on D 21, but no statistical differences were observed between the groups fed 0 g/kg TA and 2.5 g/kg TA (Choi et al., 2022d). However, in the present study, BMD and BMC were linearly reduced by the supplementation of TA in broilers, and 2 g/kg TA supplementation significantly reduced BMD and BMC compared to the control group on D 42. Possibly, the TA supplementation might have reduced utilization of calcium, phosphorous, and iron, which are important minerals for bone formation in broilers (Hassan et al., 2003; Afsana et al., 2004; Katsumata et al., 2009; Shang et al., 2015). Moreover, the pelleting process may have induced more formation of TA-mineral complexes, which dramatically reduced BMD and BMC in broilers. A previous study by Tomaszewska et al. (2018) also reported that inclusion of low-tannin faba bean (condensed tannins) negatively affected tibia traits (weight, reduction of the cross section area, and wall thickness) in broilers.
In the present study, the fat percentage measured by DEXA was linearly increased and the ratio of lean to fat decreased in broilers on D 42. This result is in stark contrast to our previous results reporting that the supplementation of TA increased the ratio of lean to fat in broilers on D 21 (Choi et al., 2022d). Discrepancies between the findings of these studies could have originated from differences in the supplementation period and age of birds (D 42 vs. D 21) and feed form (pelleted vs. mash). In our previous study (Choi et al., 2022d), fat accumulation was reduced in broilers D 21 due to less production of cecal volatile fatty acids, which potentially resulted in an imbalance of energy homeostasis. However, in the current study, the pelleting process may have decreased nutrient utilization by inducing the formation of TA-nutrient complexes, and young broilers, which did not have mature enough gastrointestinal tract to hydrolyze TA-nutrient complexes, may have decreased digestibility of energy and nutrients. To compensate limited growth rate at young stage due to reduced nutrient and energy utilization by the supplementation of TA, the broilers may have altered their body metabolism to increase the accumulation of fat (Kobylińska et al., 2022). Consistently, a previous study by Starčević et al. (2015) also showed that the supplementation of TA (5 g/kg) increased fat accumulation in the breast and thigh meat in broilers on D 35.
In the current study, absolute and relative weight of abdominal fat were increased by the supplementation of TA, which is consistent with DEXA results. The abdominal fat weight is a dependable parameter to represent body fat content because abdominal fat is the main and largest area (up to 4% of BW) of fat accumulation in broilers (Thomas et al., 1983; Fouad and El-Senousey, 2014). Furthermore, the supplementation of TA resulted in linearly increased leg meat and linearly decreased breast meat yield (p = 0.168), while statistical differences were not observed. Leg meat had higher fat content compared to breast meat (Pikul et al., 1985). Potentially, increased fat metabolism in broiler body by the supplementation of TA resulted in increased leg meat yield and decreased breast meat yield to increase fat accumulation in chickens. Fatty broiler meat and low yield of breast meat are not preferred in modern broiler production (Fouad and El-Senousey, 2014). Low pH of the breast meat results in higher lightness, lower redness, and higher yellowness by decreasing water binding capacity (Allen et al., 1997; Qiao et al., 2001). Our current study showed that the supplementation of TA decreased pH, increased redness and yellowness, and reduced cooking loss in the breast meat. This would be due to immatureness of breast meat and low growth performance caused by the TA supplementation. According to Bianchi et al. (2007), smaller broilers had lower pH and higher redness when compared to the bigger broilers. However, the lightness, an important factor to indicate pale, soft, and exudative (PSE)-like condition in poultry meat (Petracci et al., 2004), was not modulated due to the supplementation of TA in the current study. Moreover, the supplementation of TA did not dramatically altered those meat quality parameters, and the values were in still normal range (Hertanto et al., 2018). No differences were observed in the breast muscle myopathies such as white striping, woody breast, spaghetti meat, and hemorrhagic lesion in the current study. However, numerical reductions in the scores of spaghetti meat and hemorrhagic lesion in the breast meat would be associated with retarded growth rate and immatureness of breast meat in broilers fed dietary TA because breast meat myopathies are frequently observed in the fast-growing broilers (Caldas-Cueva and Owens, 2020). Therefore, the supplementation of TA did not significantly alter meat quality of broiler chickens.
In the current study, discrepant results from our previous studies and negative effects of the TA supplementation would be mainly attributed to the pelleting process on diets, which induced the formation of TA-nutrient complexes. In order to minimize or inhibit the interaction of TA and dietary nutrients during pelleting process, the encapsulation of TA can be a potential strategy (Choi et al., 2020a). Encapsulation process can provide protection for TA and release TA in the target site of the gastrointestinal tract, where many pathogens propagate (e.g., lower gut) (Choi et al., 2020a). A previous study by Wang M. et al. (2020) reported that encapsulated TA showed beneficial effects on gut health and microbiota of weaned piglets. Future studies should include: 1) investigation of appropriate methods to encapsulate TA and its stability during pelleting process and in the gastrointestinal tract; and 2) investigation into the effects of encapsulated TA on growth performance and gut health in broilers on D 42.
The TA supplementation up to 2 g/kg in pelleted diets positively affected gut microbiota, enhanced brush border digestive enzyme activities, upregulated genes related to gut barrier integrity and nutrient transportation in the starter/grower phases, and improved nutrient digestibility in the finisher phase. However, the supplementation of TA decreased overall growth performance and feed efficiency, increased fat accumulation, and negatively affected gut microbiota, bone health, and meat production in broilers on D 42. Therefore, further processing should be applied on TA to enhance their potential beneficial effects on broilers.
The authors acknowledge that the data presented in this study must be deposited and made publicly available in an acceptable repository, prior to publication. Frontiers cannot accept a manuscript that does not adhere to our open data policies.
The animal study was reviewed and approved by the Institutional Animal Care and Use Committee at the University of Georgia, Athens, GA.
JC and WK conceived and designed the study. JC, GL, DG, JW, BB, and ZH conducted the experiment. JC analyzed the data. BB and ZH advised and helped meat analyses. JC wrote the manuscript, and all authors critically review the paper. WK supervised all processes for this manuscript.
We appreciate lab members in ZH’s lab in the National Poultry Research Center, USDA-ARS, for their help in the meat analyses.
The authors declare that the research was conducted in the absence of any commercial or financial relationships that could be construed as a potential conflict of interest.
All claims expressed in this article are solely those of the authors and do not necessarily represent those of their affiliated organizations, or those of the publisher, the editors and the reviewers. Any product that may be evaluated in this article, or claim that may be made by its manufacturer, is not guaranteed or endorsed by the publisher.
Abd El-Hack M. E., El-Saadony M. T., Shafi M. E., Qattan S. Y., Batiha G. E., Khafaga A. F., et al. (2020). Probiotics in poultry feed: A comprehensive review. J. Anim. Physiol. Anim. Nutr. 104, 1835–1850. doi:10.1111/jpn.13454
Abdollahi M., Ravindran V., Svihus B. (2013). Pelleting of broiler diets: An overview with emphasis on pellet quality and nutritional value. Anim. Feed Sci. Technol. 179, 1–23. doi:10.1016/j.anifeedsci.2012.10.011
Adamczyk B., Adamczyk S., Smolander A., Kitunen V. (2011). Tannic acid and Norway spruce condensed tannins can precipitate various organic nitrogen compounds. Soil Biol. Biochem. 43, 628–637. doi:10.1016/j.soilbio.2010.11.034
Adil S., Banday T., Bhat G. A., Mir M. S., Rehman M. (2010). Effect of dietary supplementation of organic acids on performance, intestinal histomorphology, and serum biochemistry of broiler chicken.Veterinary Med. Int.
Afsana K., Shiga K., Ishizuka S., Hara H. (2004). Reducing effect of ingesting tannic acid on the absorption of iron, but not of zinc, copper and manganese by rats. Biosci. Biotechnol. Biochem. 68, 584–592. doi:10.1271/bbb.68.584
Allen C., Russell S., Fletcher D. (1997). The relationship of broiler breast meat color and pH to shelf-life and odor development. Poult. Sci. 76, 1042–1046. doi:10.1093/ps/76.7.1042
Andrade R. G., Dalvi L. T., Silva J. M. C., Lopes G. K., Alonso A., Hermes-Lima M. (2005). The antioxidant effect of tannic acid on the in vitro copper-mediated formation of free radicals. Arch. Biochem. Biophys. 437, 1–9. doi:10.1016/j.abb.2005.02.016
Arzola-Alavarez C., Castillo-Castillo Y., Anderson R., Hume M., Ruiz-Barrera O., Min B., et al. (2020). Influence of pine bark tannin on bacterial pathogens growth and nitrogen compounds on changes in composted poultry litter. Braz. J. Poult. Sci. 22. doi:10.1590/1806-9061-2018-0911
Aston E., Jackwood M., Gogal R., Hurley D., Fairchild B., Hilt D., et al. (2019). Ambient ammonia does not appear to inhibit the immune response to infectious bronchitis virus vaccination and protection from homologous challenge in broiler chickens. Vet. Immunol. Immunopathol. 217, 109932. doi:10.1016/j.vetimm.2019.109932
Baldi G., Soglia F., Petracci M. (2021). Spaghetti meat abnormality in broilers: Current understanding and future research directions. Front. Physiol. 12, 684497. doi:10.3389/fphys.2021.684497
Bianchi M., Petracci M., Sirri F., Folegatti E., Franchini A., Meluzzi A. (2007). The influence of the season and market class of broiler chickens on breast meat quality traits. Poult. Sci. 86, 959–963. doi:10.1093/ps/86.5.959
Biddle A., Stewart L., Blanchard J., Leschine S. (2013). Untangling the genetic basis of fibrolytic specialization by Lachnospiraceae and Ruminococcaceae in diverse gut communities. Diversity 5, 627–640. doi:10.3390/d5030627
Binda C., Lopetuso L. R., Rizzatti G., Gibiino G., Cennamo V., Gasbarrini A. (2018). Actinobacteria: A relevant minority for the maintenance of gut homeostasis. Dig. Liver Dis. 50, 421–428. doi:10.1016/j.dld.2018.02.012
Bolyen E., Rideout J. R., Dillon M. R., Bokulich N. A., Abnet C. C., Al-Ghalith G. A., et al. (2019). Reproducible, interactive, scalable and extensible microbiome data science using QIIME 2. Nat. Biotechnol. 37, 852–857. doi:10.1038/s41587-019-0209-9
Brambila G. S., Bowker B., Chatterjee D., Zhuang H. (2018). Descriptive texture analyses of broiler breast fillets with the wooden breast condition stored at 4° C and–20° C. Poult. Sci. 97, 1762–1767. doi:10.3382/ps/pew327
Brickett K., Dahiya J., Classen H., Gomis S. (2007). Influence of dietary nutrient density, feed form, and lighting on growth and meat yield of broiler chickens. Poult. Sci. 86, 2172–2181. doi:10.1093/ps/86.10.2172
Brink M., Janssens G. P., Demeyer P., Bağci Ø., Delezie E. (2022). Reduction of dietary crude protein and feed form: Impact on broiler litter quality, ammonia concentrations, excreta composition, performance, welfare, and meat quality. Anim. Nutr. 9, 291–303. doi:10.1016/j.aninu.2021.12.009
Caldas-Cueva J. P., Owens C. M. (2020). A review on the woody breast condition, detection methods, and product utilization in the contemporary poultry industry. J. Anim. Sci. 98, skaa207. doi:10.1093/jas/skaa207
Caly D. L., D'inca R., Auclair E., Drider D. (2015). Alternatives to antibiotics to prevent necrotic enteritis in broiler chickens: A microbiologist's perspective. Front. Microbiol. 6, 1336. doi:10.3389/fmicb.2015.01336
Castro F., Su S., Choi H., Koo E., Kim W. (2019). L-Arginine supplementation enhances growth performance, lean muscle, and bone density but not fat in broiler chickens. Poult. Sci. 98, 1716–1722. doi:10.3382/ps/pey504
Cervantes H. M. (2015). Antibiotic-free poultry production: Is it sustainable? J. Appl. Poult. Res. 24, 91–97. doi:10.3382/japr/pfv006
Choi J., Kim W. (2022). Interactions of microbiota and mucosal immunity in the ceca of broiler chickens infected with Eimeria tenella. Vaccines 10, 1941. doi:10.3390/vaccines10111941
Choi J., Kim W. K. (2020). Dietary application of tannins as a potential mitigation strategy for current challenges in poultry production: A review. Animals. 10, 2389. doi:10.3390/ani10122389
Choi J., Ko H., Tompkins Y. H., Teng P.-Y., Lourenco J. M., Callaway T. R., et al. (2021). Effects of Eimeria tenella infection on key parameters for feed efficiency in broiler chickens. Animals. 11, 3428. doi:10.3390/ani11123428
Choi J., Marshall B., Ko H., Shi H., Singh A. K., Thippareddi H., et al. (2022a). Antimicrobial and immunomodulatory effects of tannic acid supplementation in broilers infected with Salmonella Typhimurium. Poult. Sci. 101, 102111. doi:10.1016/j.psj.2022.102111
Choi J., Marshall B., Ko H., Shi H., Singh A. K., Thippareddi H., et al. (2022b). Antimicrobial and immunomodulatory effects of tannic acid supplementation in broilers infected with Salmonella Typhimurium. Poult. Sci. 101, 102111. doi:10.1016/j.psj.2022.102111
Choi J., Tompkins Y. H., Teng P.-Y., Gogal R. M., Kim W. K. (2022c). Effects of tannic acid supplementation on growth performance, oocyst shedding, and gut health of in broilers infected with Eimeria maxima. Animals. 12, 1378. doi:10.3390/ani12111378
Choi J., Wang L., Ammeter E., Lahaye L., Liu S., Nyachoti M., et al. (2020a). Evaluation of lipid matrix microencapsulation for intestinal delivery of thymol in weaned pigs. Transl. Anim. Sci. 4, 411–422. doi:10.1093/tas/txz176
Choi J., Wang L., Liu S., Lu P., Zhao X., Liu H., et al. (2020b). Effects of a microencapsulated formula of organic acids and essential oils on nutrient absorption, immunity, gut barrier function, and abundance of enterotoxigenic Escherichia coli F4 in weaned piglets challenged with E. coli F4. J. Anim. Sci. 98, skaa259. doi:10.1093/jas/skaa259
Choi J., Yadav S., Wang J., Lorentz B. J., Lourenco J. M., Callaway T. R., et al. (2022d). CT-based lung motion differences in patients with usual interstitial pneumonia and nonspecific interstitial pneumonia. Front. Physiol. 13, 867473. doi:10.3389/fphys.2022.867473
Diaz Carrasco J. M., Redondo E. A., Pin Viso N. D., Redondo L. M., Farber M. D., Fernandez Miyakawa M. E. (2018). Tannins and bacitracin differentially modulate gut microbiota of broiler chickens. Biomed. Res. Int. 2018, 1879168. doi:10.1155/2018/1879168
Ebrahim R., Liang J. B., Jahromi M. F., Shokryazdan P., Ebrahimi M., Li Chen W., et al. (2015). Protective potential of Lactobacillus species in lead toxicity model in broiler chickens. Animal 14, 755–761. doi:10.1017/S175173111600224X
Eichner G., Vieira S., Torres C., Coneglian J., Freitas D., Oyarzabal O. (2007). Litter moisture and footpad dermatitis as affected by diets formulated on an all-vegetable basis or having the inclusion of poultry by-product. J. Appl. Poult. Res. 16, 344–350. doi:10.1093/japr/16.3.344
Elgharbawy A. A., Hayyan A., Hayyan M., Rashid S. N., Nor M. R. M., Zulkifli M. Y., et al. (2018). Shedding light on lipase stability in natural deep eutectic solvents. Chem. Biochem. Eng. Q. 32, 359–370. doi:10.15255/cabeq.2018.1335
Fouad A., El-Senousey H. (2014). Nutritional factors affecting abdominal fat deposition in poultry: A review. Asian-Australas. J. Anim. Sci. 27, 1057–1068. doi:10.5713/ajas.2013.13702
Greif G. (2000). Immunity to coccidiosis after treatment with toltrazuril. Parasitol. Res. 86, 787–790. doi:10.1007/s004360000218
Gülçin İ., Huyut Z., Elmastaş M., Aboul-Enein H. Y. (2010). Radical scavenging and antioxidant activity of tannic acid. Arabian J. Chem. 3, 43–53. doi:10.1016/j.arabjc.2009.12.008
Haque M. H., Sarker S., Islam M. S., Islam M. A., Karim M. R., Kayesh M. E. H., et al. (2020). Sustainable antibiotic-free broiler meat production: Current trends, challenges, and possibilities in a developing country perspective. Biology 9, 411. doi:10.3390/biology9110411
Hassan I., Elzubeir E., El Tinay A. (2003). Growth and apparent absorption of minerals in broiler chicks fed diets with low or high tannin contents. Trop. Anim. Health Prod. 35, 189–196. doi:10.1023/a:1022833820757
Hertanto B., Nurmalasari C., Nuhriawangsa A., Cahyadi M., Kartikasari L. (2018). “The physical and microbiological quality of chicken meat in the different type of enterprise poultry slaughterhouse: A case study in karanganyar district,” in IOP conference series: Earth and environmental science. (Semarang, Indonesia: IOP Publishing), 012051.
Kaić A., Janječić Z., Žanetić A., Kelava Ugarković N., Potočnik K. (2021). EZ-DripLoss assessment in chicken breast meat using different sample areas, fiber orientation, and measurement intervals. Animals. 11, 1095. doi:10.3390/ani11041095
Karakaya S. (2004). Bioavailability of phenolic compounds. Crit. Rev. Food Sci. Nutr. 44, 453–464. doi:10.1080/10408690490886683
Katsumata S.-I., Katsumata-Tsuboi R., Uehara M., Suzuki K. (2009). Severe iron deficiency decreases both bone formation and bone resorption in rats. J. Nutr. 139, 238–243. doi:10.3945/jn.108.093757
Kim T., Silva J., Kim M., Jung Y. (2010). Enhanced antioxidant capacity and antimicrobial activity of tannic acid by thermal processing. Food Chem. 118, 740–746. doi:10.1016/j.foodchem.2009.05.060
Kim W., Patterson P. (2003a). Effect of minerals on activity of microbial uricase to reduce ammonia volatilization in poultry manure. Poult. Sci. 82, 223–231. doi:10.1093/ps/82.2.223
Kim W., Patterson P. (2003b). Production of an egg yolk antibody specific to microbial uricase and its inhibitory effects on uricase activity. Poult. Sci. 82, 1554–1558. doi:10.1093/ps/82.10.1554
Kobylińska M., Antosik K., Decyk A., Kurowska K. (2022). Malnutrition in obesity: Is it possible? Obes. Facts 15, 19–25. doi:10.1159/000519503
Koo B., Nyachoti C. M. (2019). Effects of thermally oxidized canola oil and tannic acid supplementation on nutrient digestibility and microbial metabolites in finishing pigs. J. Anim. Sci. 97, 2468–2478. doi:10.1093/jas/skz104
Kuttappan V., Owens C., Coon C., Hargis B., Vazquez-Anon M. (2017). Incidence of broiler breast myopathies at 2 different ages and its impact on selected raw meat quality parameters. Poult. Sci. 96, 3005–3009. doi:10.3382/ps/pex072
Lackeyram D. (2012). Expression of the small intestinal apical membrane hydrolases in the early-weaned piglet. Guelph, Ontario, Canada: University of Guelph.
Lackeyram D., Yang C., Archbold T., Swanson K. C., Fan M. Z. (2010). Early weaning reduces small intestinal alkaline phosphatase expression in pigs. J. Nutr. 140, 461–468. doi:10.3945/jn.109.117267
Lee S., Shinde P., Choi J., Kwon I., Lee J., Pak S., et al. (2010). Microwave-assisted extraction of human hair proteins. Anal. Biochem. 131, 281–283. doi:10.1016/j.ab.2010.08.021
Lin Y., Olukosi O. A. (2021). Qualitative and quantitative profiles of jejunal oligosaccharides and cecal short-chain fatty acids in broiler chickens receiving different dietary levels of fiber, protein and exogenous enzymes. J. Sci. Food Agric. 101, 5190–5201. doi:10.1002/jsfa.11165
Liu K., Jia M., Wong E. A. (2020). Delayed access to feed affects broiler small intestinal morphology and goblet cell ontogeny. Poult. Sci. 99, 5275–5285. doi:10.1016/j.psj.2020.07.040
Livak K. J., Schmittgen T. D. (2001). Analysis of relative gene expression data using real-time quantitative PCR and the 2(-Delta Delta C(T)) Method. methods 25, 402–408. doi:10.1006/meth.2001.1262
Lu P., Choi J., Yang C., Mogire M., Liu S., Lahaye L., et al. (2020). Effects of antibiotic growth promoter and dietary protease on growth performance, apparent ileal digestibility, intestinal morphology, meat quality, and intestinal gene expression in broiler chickens: A comparison. J. Anim. Sci. 98, skaa254. doi:10.1093/jas/skaa254
Maroux S., Louvard D., Barath J. (1973). The aminopeptidase from hog intestinal brush border. Biochim. Biophys. Acta 321, 282–295. doi:10.1016/0005-2744(73)90083-1
Marzo F., Urdaneta E., Santidrian S. (2002). Liver proteolytic activity in tannic acid-fed birds. Poult. Sci. 81, 92–94. doi:10.1093/ps/81.1.92
Mogire M. K., Choi J., Lu P., Yang C., Liu S., Adewole D., et al. (2021). Effects of red-osier dogwood extracts on growth performance, intestinal digestive and absorptive functions, and meat quality of broiler chickens. Can. J. Anim. Sci. 101, 687–703. doi:10.1139/cjas-2020-0191
Moula N., Hornick J.-L., Cabaraux J.-F., Korsak N., Daube G., Dawans E., et al. (2018). Effects of dietary black soldier fly larvae on performance of broilers mediated or not through changes in microbiota. J. Insects as Food Feed 4, 31–42. doi:10.3920/jiff2017.0011
Naseem S., King A. J. (2018). Ammonia production in poultry houses can affect health of humans, birds, and the environment—Techniques for its reduction during poultry production. Environ. Sci. Pollut. Res. Int. 25, 15269–15293. doi:10.1007/s11356-018-2018-y
Pang B., Bowker B., Yang Y., Zhang J., Zhuang H. (2020). Relationships between instrumental texture measurements and subjective woody breast condition scores in raw broiler breast fillets. Poult. Sci. 99, 3292–3298. doi:10.1016/j.psj.2019.12.072
Petracci M., Betti M., Bianchi M., Cavani C. (2004). Color variation and characterization of broiler breast meat during processing in Italy. Poult. Sci. 83, 2086–2092. doi:10.1093/ps/83.12.2086
Pikul J., Leszczynski D., Kummerow F. (1985). Influence of fat content and composition on malonaldehyde concentration in chicken meat and skin. Poult. Sci. 64, 311–317. doi:10.3382/ps.0640311
Pinheiro D. F., Pinheiro P. F., Buratini J., Castilho A. C., Lima P. F., Trinca L. A., et al. (2013). Maternal protein restriction during pregnancy affects gene expression and immunolocalization of intestinal nutrient transporters in rats. Clin. Sci. 125, 281–289. doi:10.1042/CS20120400
Prisco F., De Biase D., Piegari G., D'aquino I., Lama A., Comella F., et al. (2021). Pathologic characterization of white striping myopathy in broiler chickens. Poult. Sci. 100, 101150. doi:10.1016/j.psj.2021.101150
Qiao M., Fletcher D., Smith D., Northcutt J. (2001). The effect of broiler breast meat color on pH, moisture, water-holding capacity, and emulsification capacity. Poult. Sci. 80, 676–680. doi:10.1093/ps/80.5.676
Ramah A., Yasuda M., Ohashi Y., Urakawa M., Kida T., Yanagita T., et al. (2020). Different doses of tannin reflect a double-edged impact on broiler chicken immunity. Vet. Immunol. Immunopathol. 220, 109991. doi:10.1016/j.vetimm.2019.109991
Redondo L. M., Chacana P. A., Dominguez J. E., Fernandez Miyakawa M. E. (2014). Perspectives in the use of tannins as alternative to antimicrobial growth promoter factors in poultry. Front. Microbiol. 5, 118. doi:10.3389/fmicb.2014.00118
Romani A., Ieri F., Turchetti B., Mulinacci N., Vincieri F., Buzzini P. (2006). Analysis of condensed and hydrolysable tannins from commercial plant extracts. J. Pharm. Biomed. Anal. 41, 415–420. doi:10.1016/j.jpba.2005.11.031
Shang Y., Rogiewicz A., Patterson R., Slominski B., Kim W. (2015). The effect of phytase and fructooligosaccharide supplementation on growth performance, bone quality, and phosphorus utilization in broiler chickens. Poult. Sci. 94, 955–964. doi:10.3382/ps/pev044
Shinde A. S., Goel A., Mehra M., Rokade J., Bhadauria P., Mandal A. B., et al. (2015). Delayed post hatch feeding affects performance, intestinal morphology and expression pattern of nutrient transporter genes in egg type chickens. J. Nutr. Food Sci. 5, 1.
Short F., Gorton P., Wiseman J., Boorman K. (1996). Determination of titanium dioxide added as an inert marker in chicken digestibility studies. Animal feed Sci. Technol. 59, 215–221. doi:10.1016/0377-8401(95)00916-7
Singh K., Shah T., Deshpande S., Jakhesara S., Koringa P., Rank D., et al. (2012). High through put 16S rRNA gene-based pyrosequencing analysis of the fecal microbiota of high FCR and low FCR broiler growers. Mol. Biol. Rep. 39, 10595–10602. doi:10.1007/s11033-012-1947-7
Starčević K., Krstulović L., Brozić D., Maurić M., Stojević Z., Mikulec Ž., et al. (2015). Production performance, meat composition and oxidative susceptibility in broiler chicken fed with different phenolic compounds. J. Sci. Food Agric. 95, 1172–1178. doi:10.1002/jsfa.6805
Teng P.-Y., Choi J., Yadav S., Tompkins Y., Kim W. K. (2021). Effects of low-crude protein diets supplemented with arginine, glutamine, threonine, and methionine on regulating nutrient absorption, intestinal health, and growth performance of Eimeria-infected chickens. Poult. Sci. 100, 101427. doi:10.1016/j.psj.2021.101427
Thomas V. G., Mainguy S. K., Prevett J. P. (1983). Predicting fat content of geese from abdominal fat weight. J. Wildl. Manag. 47, 1115–1119. doi:10.2307/3808172
Tomaszewska E., Dobrowolski P., Klebaniuk R., Kwiecień M., Tomczyk-Warunek A., Szymańczyk S., et al. (2018). Gut-bone axis response to dietary replacement of soybean meal with raw low-tannin faba bean seeds in broiler chickens. Plos one 13, e0194969. doi:10.1371/journal.pone.0194969
Tonda R., Rubach J., Lumpkins B., Mathis G., Poss M. (2018). Effects of tannic acid extract on performance and intestinal health of broiler chickens following coccidiosis vaccination and/or a mixed-species Eimeria challenge. Poult. Sci. 97, 3031–3042. doi:10.3382/ps/pey158
Treviño J., Ortiz L., Centeno C. (1992). Effect of tannins from faba beans (Vicia faba) on the digestion of starch by growing chicks. Animal Feed Sci. Technol. 37, 345–349. doi:10.1016/0377-8401(92)90017-z
Vandesompele J., De Preter K., Pattyn F., Poppe B., Van Roy N., De Paepe A., et al. (2002). Accurate normalization of real-time quantitative RT-PCR data by geometric averaging of multiple internal control genes. Genome Biol. 3, RESEARCH0034–12. doi:10.1186/gb-2002-3-7-research0034
Wang J., Choi H., Kim W. (2020a). Effects of dietary energy level and 1, 3-diacylglycerol on growth performance and carcass yield in broilers. J. Appl. Poult. Res. 29, 665–672. doi:10.1016/j.japr.2020.04.004
Wang M., Huang H., Hu Y., Huang J., Yang H., Wang L., et al. (2020b). Effects of dietary microencapsulated tannic acid supplementation on the growth performance, intestinal morphology, and intestinal microbiota in weaning piglets. J. Anim. Sci. 98, skaa112. doi:10.1093/jas/skaa112
Wasti S., Sah N., Singh A. K., Lee C. N., Jha R., Mishra B. (2021). Dietary supplementation of dried plum: A novel strategy to mitigate heat stress in broiler chickens. J. Anim. Sci. Biotechnol. 12, 58–17. doi:10.1186/s40104-021-00571-5
Yadav S., Teng P.-Y., Singh A., Choi J., Kim W. (2022). Influence of Brassica spp. rapeseed and canola meal, and supplementation of bioactive compound (AITC) on growth performance, intestinal-permeability, oocyst shedding, lesion score, histomorphology, and gene expression of broilers challenged with E. maxima. Poult. Sci. 101, 101583. doi:10.1016/j.psj.2021.101583
Yang C., Chowdhury M. K., Hou Y., Gong J. (2015). Phytogenic compounds as alternatives to in-feed antibiotics: Potentials and challenges in application. Pathogens 4, 137–156. doi:10.3390/pathogens4010137
Yang C., Diarra M. S., Choi J., Rodas-Gonzalez A., Lepp D., Liu S., et al. (2021). Effects of encapsulated cinnamaldehyde on growth performance, intestinal digestive and absorptive functions, meat quality and gut microbiota in broiler chickens. Transl. Anim. Sci. 5, txab099. doi:10.1093/tas/txab099
Yang Y., Iji P., Kocher A., Mikkelsen L., Choct M. (2008). Effects of xylanase on growth and gut development of broiler chickens given a wheat-based diet. Asian-Australas. J. Anim. Sci. 21, 1659–1664. doi:10.5713/ajas.2008.80074
Youssef I., Beineke A., Rohn K., Kamphues J. (2011). Effects of litter quality (moisture, ammonia, uric acid) on development and severity of foot pad dermatitis in growing turkeys. Avian Dis. 55, 51–58. doi:10.1637/9495-081010-Reg.1
Keywords: tannic acid, gut microbiota, gut health, floor pen, pelleting process, meat production, and fat accumulation
Citation: Choi J, Liu G, Goo D, Wang J, Bowker B, Zhuang H and Kim WK (2022) Effects of tannic acid supplementation on growth performance, gut health, and meat production and quality of broiler chickens raised in floor pens for 42 days. Front. Physiol. 13:1082009. doi: 10.3389/fphys.2022.1082009
Received: 27 October 2022; Accepted: 07 December 2022;
Published: 16 December 2022.
Edited by:
Sandra G. Velleman, The Ohio State University, United StatesReviewed by:
Yuwares Malila, National Center for Genetic Engineering and Biotechnology (BIOTEC), ThailandCopyright © 2022 Choi, Liu, Goo, Wang, Bowker, Zhuang and Kim. This is an open-access article distributed under the terms of the Creative Commons Attribution License (CC BY). The use, distribution or reproduction in other forums is permitted, provided the original author(s) and the copyright owner(s) are credited and that the original publication in this journal is cited, in accordance with accepted academic practice. No use, distribution or reproduction is permitted which does not comply with these terms.
*Correspondence: Woo Kyun Kim, d2traW1AdWdhLmVkdQ==
Disclaimer: All claims expressed in this article are solely those of the authors and do not necessarily represent those of their affiliated organizations, or those of the publisher, the editors and the reviewers. Any product that may be evaluated in this article or claim that may be made by its manufacturer is not guaranteed or endorsed by the publisher.
Research integrity at Frontiers
Learn more about the work of our research integrity team to safeguard the quality of each article we publish.