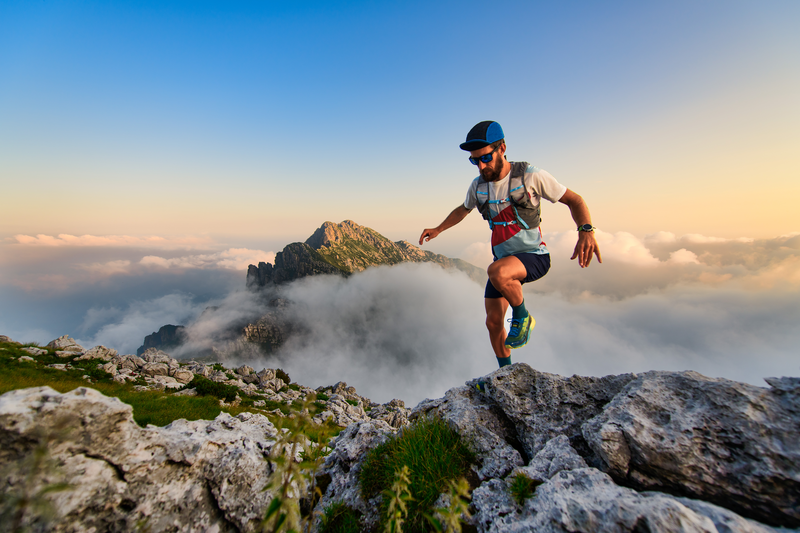
94% of researchers rate our articles as excellent or good
Learn more about the work of our research integrity team to safeguard the quality of each article we publish.
Find out more
SYSTEMATIC REVIEW article
Front. Physiol. , 15 December 2022
Sec. Exercise Physiology
Volume 13 - 2022 | https://doi.org/10.3389/fphys.2022.1046625
This article is part of the Research Topic Efficacy of Small Muscle Mass Exercise Training to Promote Health View all 6 articles
Objective: To perform a meta-analysis on the efficacy and dose-response relationship of blood flow restriction training on muscle strength reported worldwide.
Methods: Thirty-four eligible articles with a total sample size of 549 participants were included in the meta-analysis. This study was performed using the method recommended by the Cochrane Handbook (https://training.cochrane.org/handbook), and the effect size was estimated using the standardized mean difference (SMD) and using RevMan 5.3 software (The Nordic Cochrane Centre, The Cochrane Collaboration, Copenhagen, 2014).
Results: The meta-analysis showed that blood flow restriction training increased the lower limb extensor muscle strength [SMD = 0.72, 95%; confidence interval (CI): 0.43 to 1.00, p < 0.01], knee extensor isokinetic torque SMD = 0.48 [95% CI: 0.24 to 0.73, p < 0.01], knee flexor isokinetic torque SMD = 0.39 [95% CI: 0.11 to 0.67, p < 0.01], and squat one-repetition maximum [SMD = 0.28, 95% CI: 0.01 to 0.55, p < 0.01]. There was no publication bias. Evaluation of dose-response relationship showed that the training load, mode, frequency, duration, and maximum cuff pressure affected the muscle function.
Conclusion: blood flow restriction training. 16 significantly improved lower limb muscle strength, and the optimal training conditions consisted of a weight load smaller or equal to 30% of one-repetition maximum, training duration longer than 4 weeks, frequency of more than 3 times/week, and maximum cuff pressure lower than 200 mmHg.
Systematic Review Registration: website, identifier registration number.
Blood flow restriction training was introduced in 1983 as a novel training method in which the blood flow is restricted by applying pressure to the proximal muscle extremities with a specially designed constricting device, such as blood pressure cuff or tourniquet (Sheng et al., 2019). The blood flow restriction increases the mass of exercising muscles (Manini and Clark, 2009). It is also known as KAATSU training, with the advantages of low training risk and intensity but high frequency (Manini and Clark, 2009; Abe et al., 2012). In recent years, bloo1d flow restriction training has been widely used in competitive sports, public fitness, and rehabilitation. Studies have confirmed the beneficial effects of blood flow restriction training on athletes in track and field, soccer, rugby, tennis, handball, and basketball by improving the maximum strength and isokinetic torque strength of the upper and lower limb muscles (Kubota et al., 2008; Kubota et al., 2011; Xu and Wang, 2013; Pope et al., 2015; Tennent et al., 2017; Li et al., 2019; Wang et al., 2019). A meta-analysis by Wu et al. of 14 studies showed that blood flow restriction training increased the standard extensor strength of lower limbs and the cross-sectional area of quadriceps (Wu et al., 2019). Niu et al. conducted a meta-analysis of 47 studies and concluded that blood flow restriction training significantly increased muscles’ mass and maximum strength (Niu et al., 2020). Loenneke et al. found in a meta-analysis that 15%–30% one-repetition maximum (1RM)/maximal voluntary contraction (MVC) had a better muscle training effect (Abe et al., 2012). Similarly, Slysz et al. confirmed the effectiveness of blood flow restriction training on muscle hypertrophy and strength, with analysis of 47 studies (Slysz and Burr, 2016). Although the effects of blood flow restriction training on the body have been confirmed, there is still a lack of evidence-based on the “dose-response” relationship of blood flow restriction training (Manini and Clark, 2009). Duo to the above, this study is the first to systematically evaluate the intervention intensity, cycle, frequency, and pressure peak of blood flow restriction training, and conduct a four-item meta-analysis study on the effect of blood flow restriction training on lower limb muscle strength. In this study, a dose-effect relationship will be observed between blood flow restriction training and lower extremity muscle strength in order to evaluate the training effect more quantitatively (Aune et al., 2016) and to provide a more objective and reliable blood flow restriction training plan, in order to produce scientific and reliable results—quantitative guidance.
This meta-analysis was performed according to the Preferred Reporting Items for Meta-Analyses (PRISMA) guidelines (Cumpston et al., 2019). A computer-assisted literature search was performed in China National Knowledge Infrastructure, Wan-Fang Med Online, Baidu Scholar, Web of Science, PubMed, Elsevier, Embase, and EBSCOhost. The keywords were “pressurized training”, “blood flow restriction training”, “blood blockage; training”, and “KAATSU training”. The English keywords were “KAATSU training” [Title/Abstract] OR “blood flow restriction training” [Title/Abstract] OR “BFRT” [Title/Abstract] OR “blood flow restriction exercise” [Title/Abstract] OR “occlusion training” [Title/Abstract] “Restricted Leg Blood Flow” [Title/Abstract]. We searched articles published from inception to October 2022. All retrieved articles in Chinese and English were imported into EndNote (version X9, Clarivate Analytics) for deduplication. Two independent reviewers (HJX and ZZY) screened articles against the inclusion and exclusion criteria, and discrepancies were resolved by discussion and consensus with a third reviewer (SJ). The following data were extracted: 1) citation information of included studies, such as title, first author, journal, and time of publication; 2) baseline characteristics of participants, including a sample size of each group, and the age and sex of participants; 3) details of interventions such as duration, frequency, load, maximal cuff peak; 4) key elements of bias risk assessment; 5) outcome indicators and measures.
According to the PICOS (Liu et al., 2017) principle (patient/population, intervention, comparison/control, outcome and study design), trials were eligible for inclusion if they met the criteria as follows: 1) participants were defined as healthy individuals; 2) the types of studies include randomized controlled trials (RCTs) or self-controlled trials, regardless of blinding or allocation concealment, and the control groups were daily activities or regular physical activities without interventions. 3) the outcomes measures of the trial included functional indicators include knee flexor (extensor) isokinetic torque, maximum squat strength, and lower limb extensor strength. 4) the selected articles were peer-reviewed publications written in English.
Trials were excluded when they met any of the following exclusion criteria: 1) Trials without randomized, self-controlled, or randomized crossover design; 2) lack of appropriate outcome indicators; 3) conference abstracts and overviews; 4) articles with inappropriate subjects (animals).
The quality of articles as indicated by the risk of bias in RCT was assessed using the method recommended by the Cochrane Handbook (https://training.cochrane.org/handbook). The articles were ranked at high, low, or unclear risk of bias. The low, high, and unclear risk of bias is represented by three colors in the evaluation plot, green for low, yellow for uncertain, and red for high.
RevMan, Computer program, version 5.3 (The Nordic Cochrane Centre, The Cochrane Collaboration, Copenhagen, 2014) and using STATA software version 17 (STATA Corp, College Station, TX, USA) for data analysis. Q test was used to assess the heterogeneity of data in articles (α = 0.1). The effect size was calculated using different models based on the heterogeneity between studies. I2 ≤ 50% (p ≥ 0.1) was regarded as minimal heterogeneity between studies, and a fixed-effects model was adopted. A random-effects model was used otherwise. The effect size was expressed as standardized mean difference (SMD), 95% CI, and subgroup analysis for moderators, with effect sizes of 0.2, 0.5, and 0.8 defined as small, medium, and medium-large, respectively.
A total of 1,070 English articles and 295 Chinese articles were retrieved, and 29 English articles (Abe et al., 2005; Beekley et al., 2005; Yasuda et al., 2005; Fujita et al., 2008; Madarame et al., 2008; Abe et al., 2010a; Abe et al., 2010b; Park et al., 2010; Sumide et al., 2010; Madarame et al., 2011; Tomohiro et al., 2011; Yasuda et al., 2011; Godawa et al., 2012; Keramidas et al., 2012; Martín-Hernández et al., 2012; Tetsuo et al., 2012; Luebbers et al., 2014; Yasuda et al., 2014; Barcelos et al., 2015; Fahs et al., 2015; Lixandrão et al., 2015; Vechin et al., 2015; Yasuda et al., 2015; Kim et al., 2016; Luebbers et al., 2017; Slysz and Burr, 2017; Bjørnsen et al., 2019; Harper et al., 2019; Schwiete et al., 2021; Teixeira et al., 2021) and 5Chinese articles (Liu et al., 2018; Lu et al., 2020; Zhang et al., 2020; Li et al., 2022; Tang and Qu, 2022) were included in the meta-analysis. The flow diagram of the study selection is shown in Figure 1.
A total of 34 articles with 49 studies (with some articles containing multiple studies) were included for 145 meta-analysis, with 549 participants in intervention groups and 549 participants in control groups. Participants were of mixed gender, age between 18 and 78 years and were males (70%) and were females (30%). RCT were used in all studies with blood flow restriction training as the intervention. The training load weight (based on one-repetition maximum) is divided into three categories: a low load (20%–50% 1RM), a medium load (50%–80% 1RM), and a high load (80%–100% 1RM). The training could be short-term training (1–2 weeks) or chronic, and the duration, frequency, and cuff pressure are shown in Table 1.
The quality of 34 articles with RCT was evaluated with Cochrane risk assessment tool. Three articles mentioned allocation concealment, and the risk of bias was low. The remaining articles mentioned randomization through detailed methods, and allocation concealment was absent, therefore the risk of bias was unclear. In the 23 articles using blinding the risk of bias was low. All publications reported the loss of follow-up and were of high quality (Figure 2).
FIGURE 2. The bias of the included studies. Note: “+” represents low risk, “−” high risk, and “?” unclear.
The functional indicators described in the 34 articles added to the present analysis were categorized into knee extensor isokinetic torque, knee flexor isokinetic torque, the lower limb extensor muscle strength, and squat one-repetition maximum (1RM). A total of 11 studies with 220 participants were included for the lower limb extensor muscle strength, with 11 individual effect sizes, an overall effect size of SMD = 0.72 [95% CI: 0.43 to 1.00, p < 0.01], and heterogeneity (I2 = 51%, p = 0.02). Seventeen studies with 283 participants were included for knee extensor isokinetic torque, with 17 individual effect sizes, an overall effect size of SMD = 0.48 [95% CI: 0.24 to 0.73, p < 0.01], and no heterogeneity (I2 = 24%, p = 0.18). A total of 13 studies with 208 participants were included for knee flexor isokinetic torque, with 13 individual effect sizes, an overall effect size of SMD = 0.39 [95% CI: 0.11 to 0.67, p < 0.01], and no heterogeneity (I2 = 0%, p = 0.86). Fourteen studies with 249 participants were included for squat 1RM, with 14 individual effect sizes, an overall effect size of SMD = 0.36 [95% CI:0.10 to 0.62, p < 0.01], and no heterogeneity (I2 = 0%, p = 0.93). This above are shown in Figure 3.
FIGURE 3. The forest plot of four outcome indicators. (A) Forest plot for lower limb extensor muscle strength, (B) Forest plot for knee flexor isokinetic torque, (C) Forest plot for knee extensor isokinetic torque, (D) Forest plot for squat IRM.
Based on the included articles, the dose-response relationship was evaluated in the training load, training duration, training frequency, and maximum cuff pressure.
1) Training load. A total of 18 studies with 290 participants were included in this group. A training load of ≤30% 1RM showed a higher effect size of d = 0.583 [SMD = 0.583, 95% CI: 0.279 to 0.852, p < 0.01]. A training load of >30% 1RM showed an effect size of d = 0.564 [SMD = 0.564, 95% CI: 0.215 to 0.869, p < 0.01]. Both conditions achieved moderate training effects. The Cohen effect sizes <0.20 were considered small, 0.20 < d < 0.80 moderate, and ≥0.8 large. The above data indicate that ≤30% 1RM achieved better training effect.
2) Training duration. A total of 18 studies with 290 participants were included in this group. A training duration of >4 weeks showed a higher effect size of d = 0.636 [SMD = 0.636, 95% CI: 0.309 to 0.963, p < 0.01]. A training duration of ≤4 weeks showed an effect size of d = 0.589 [SMD = 0.589, 95% CI: 0.032 to 0.782, p < 0.01]. Both conditions achieved moderate training effects. The above data suggest that >4 weeks achieved better training effect.
3) Training frequency. A total of 18 studies with 290 participants were included in this group. A training frequency of ≥3 times/week showed a higher effect size of d = 0.622 [SMD = 0.622, 95% CI:0.325 to 0.919, p < 0.01]. A training frequency of 1–2 times/week showed an effect size of d = 0.423 [SMD = 0.423, 95% CI: −0.062 to 0.672, p > 0.05]. The above data suggest that ≥3 times/week achieved better training effect.
4) Maximum cuff pressure. A total of 18 studies with 290 participants were included in this group. A 201 maximum cuff pressure of ≤200 mmHg showed a higher effect size of d = 0.668 [SMD = 0.668, 95% CI: 0.353 to 0.983, p < 0.01], whereas a maximum cuff pressure of >200 mmHg showed an effect size of d = 0.306 [SMD = 0.306, 95% CI: −0.110 to 0.721, p < 0.05]. The above data indicate that a maximum cuff pressure of ≤200 mmHg achieved better training effect. This above are shown in Table 2.
TABLE 2. Subgroup analysis of blood flow restriction training effect on knee extensor isokinetic torque.
1) Training load. A total of 13 studies with 208 participants were included in this group, and training load of ≤30% 1RM showed a higher effect size of d = 0.442 [SMD = 0.442, 95% CI: 0.154 to 0.730, p < 0.01]. Training load of da>30% 1RM showed effect size of d = 0.001 [SMD = 0.001, 95% CI: 0.389 to 0.823, p > 0.05]. The above data indicate that ≤30% 1RM achieved a good training effect.
2) Training duration. A total of 13 studies with 208 participants were included in this group. A training duration of >4 weeks showed a higher effect size of d = 0.606 [SMD = 0.606, 95% CI: 0.389 to 0.823, p < 0.01]. A training duration of ≤4 weeks showed an effect size of d = 0.583 [SMD = 0.583, 95% CI: 0.053 to 1.018, p < 0.01]. Both conditions achieved moderate training effects. The above data suggest that a training duration >4 weeks achieved better training effect.
3) Training frequency. A total of 13 studies with 208 participants were included in this group. A training frequency of ≥3 times/week showed a higher effect size of d = 0.463 [SMD = 0.463, 95% CI: 0.140 to 0.787, p < 0.01]. A training frequency of 1–2 times/week showed an effect size of d = 0.289 [SMD = 0.289, 95% CI: −0.205 to 0.672, p > 0.05]. The above data suggest that a frequency ≥3 times/week achieved better training effect.
4) Maximum cuff pressure. A total of 13 studies with 208 participants were included in this group. A maximum cuff pressure of ≤200 mmHg showed a higher effect size of d = 0.683 [SMD = 0.683, 95% CI: 0.018 to 0.799, p < 0.01]. A maximum cuff pressure of >200 mmHg showed an effect size of d = 0.559 [SMD = 0.559, 95% CI: 0.165 to 0.953, p < 0.01]. Both conditions achieved moderate training effects. The above data indicate that a maximum cuff pressure ≤200 mmHg achieved better training effect (Table 3).
TABLE 3. Subgroup analysis of blood flow restriction training effect on knee flexor isokinetic torque.
1) Training load. A total of 11 studies with 220 participants were included in this group. A training load of ≤30% 1RM showed a higher effect size of d = 0.880 [SMD = 0.880, 95% CI: 0.494 to 1.2660, p < 0.01]. A training load of >30% 1RM showed an effect size of d = 0.548 [SMD = 0.548, 95% CI: 0.098 to 0.999, p < 0.05]. Both conditions achieved moderate training effects. The above data indicate that ≤30% 1RM achieved better training effect.
2) Training duration. A total of 11 studies with 220 participants were included in this group. A training duration of >4 weeks showed a higher effect size of d = 1.109 [SMD = 1.109, 95% CI: 0.818 to 1.400, p < 0.01]. A training duration of ≤4 showed an effect size of d = 0.943 [SMD = 0.943, 95% CI: 0.409 to 1.477, p < 0.01]. Both conditions achieved moderate training effects. The above data suggest that a training duration >4 weeks achieved better training effect.
3) Training frequency. A total of 11 studies with 220 participants were included in this group. A training frequency of ≥3 times/week showed a higher effect size of d = 0.653 [SMD = 0.653, 95% CI: 0.363 to 0.941, p < 0.01]. A training frequency of 1–2 times/week showed an effect size of d = 2.958 [SMD = 2.958, 95% CI: 1.578 to 4.338, p > 0.05], and the risk of publication bias was high because there was only one trial. The above data suggest that a training frequency ≥ three times/week achieved a better effect.
4) Maximum cuff pressure. A total of 11 studies with 220 participants were included in this group. A maximum cuff pressure of ≤200 mmHg showed a higher effect size of d = 0.653 [SMD = 0.653, 95% CI: 0.352 to 0.954, p < 0.01]. A maximum cuff pressure of >200 mmHg showed an effect size of d = 0.566 [SMD = 0.566, 95% CI: 0.337 to 0.796, p < 0.01]. Both conditions achieved moderate training effects. The above data indicate that a maximum cuff pressure ≤200 mmHg achieved better training effects (Table 4).
TABLE 4. Subgroup analysis of blood flow restriction training effect on lower limb extensor muscle strength.
1) Training load. A total of 18 studies with 448 participants were included in this group. A training load of >30% 1RM showed a higher effect size of d = 0.639 [SMD = 0.639, 95% CI: 0.389 to 0.889, p < 0.01]. A training load of ≤30% 1RM showed an effect size of d = 0.325 [SMD = 0.325, 95% CI: 0.110 to 0.638, p < 0.01]. Both conditions achieved moderate training effects. The above data indicate that ≤30% 1RM achieved better training effect.
2) Training duration. A total of 18 studies with 448 were included in this group. A training duration of >4 weeks showed a higher effect size of d = 0.606 [SMD = 0.606, 95% CI: 0.389 to 0.823, p < 0.01]. A training duration of ≤4 weeks showed an effect size of d = 0.413 [SMD = 0.413, 95% CI: 0.047 to 0.873 p < 0.05]. Both conditions achieved moderate training effects. The above data indicate that >4 weeks achieved better training effect.
3) Training frequency. A total of 17 studies with 455 participants were included in this group. A training frequency of ≥3 times/week showed a higher effect size of d = 0.550 [SMD = 0.550, 95% CI: 0.349 to 0.752, p < 0.01], whereas a training frequency of 1–2 times/week showed an effect size of d = 0.629 [SMD = 0.429, 95% CI: 0.262to 0.790, p < 0.01]. Both conditions achieved moderate training effects. The above data indicate that a training frequency of 1–2 times/week achieved better training effect.
4) Maximum cuff pressure. A total of 18 studies with 455 participants were included in this group. A maximum cuff pressure of ≤200 mmHg showed a higher effect size of d = 0.653 [SMD = 0.653, 95% CI: 0.195 to 0.867, p < 0.01]. A maximum cuff pressure of >200 mmHg showed an effect size of d = 0.566 [SMD = 0.566, 95% CI: 0.337 to 0.796, p = 0.05]. Both conditions achieved moderate training effects. The above data indicate that a maximum cuff pressure ≤200 mmHg achieved better training effect. This above are shown in Table 5.
Begg’s funnel plot was used to assess the publication bias of knee extensor’s, knee flexor isokinetic torque, lower limb extensor muscle strength, and squat 1RM. The symmetrical funnel plots suggested no publication bias for the four indicators. This above are shown in Figure 4.
FIGURE 4. Publication bias plot. (A) knee extensor isokinetic torque, (B) knee flexor isokinetic torque, (C) The lower limb extensor muscle strength, (D) Squat IRM.
The sensitivity analysis is based on knee extensor’s, knee extensor’s, torque, lower limb extensor muscle strength, and squat 1RM. Sensitivity analysis was performed using the leave-one-out method, in which dubious literature was excluded one by one to test for the presence of literature that significantly influenced the conclusion. The results are shown in Figure 5, which shows that after excluding any study, the degree of bias of the point estimates of the new combined effect sizes obtained by subjecting the remaining studies to a new Meta-analysis was not significant, suggesting good robustness of the conclusion and that no single study significantly influenced the conclusion, so all included literature was retained. This above are shown in Figure 5.
FIGURE 5. Sensitivity analysis. (A) knee extensor isokinetic torque, (B) knee flexor isokinetic torque, (C) The lower limb extensor muscle strength, (D) Squat IRM.
Blood flow restriction training had a positive effect on participant’s lower limb muscle function, and all studies showed moderate effect sizes for function improvement. Comparative analysis showed that Blood flow restriction training increased the knee extensor isokinetic torque, knee flexor isokinetic torque, lower limb extensor muscle strength, and squat 1RM. In particular, the improvement of lower limb extensor muscle strength was high and with low heterogeneity. Load measured by percentages of 1RM and MVC is an important factor influencing the training effect, and 20%–30% 1RM has been recommended for optimal training performance (Manini and Clark, 2009), which was confirmed in the present study. Abe et al. used 20% 1RM for a 2-week blood flow restriction training of healthy male participants (Abe et al., 2012). They found significant increases in the maximum squat strength (16.8%) and leg curl strength (22.6%) for 2 weeks (Zhang et al., 2020), similar to the training effect in another study using 30% 1RM over 10 weeks (Luebbers et al., 2017). Although the training effect increases with training load, an excessive load may not lead to beneficial results. Jessee et al. compared 50% 1RM with 30% 1RM and found little difference in muscle hypertrophy (Madarame et al., 2011; Jessee et al., 2018). Moreover, studies showed that blood flow restriction training did not increase muscle activation at high load (70% 1RM) (Takarada et al., 2002) and decreased muscle activation at > 70% 1RM (Dankel et al., 2017). In addition, blood flow restriction training at 80% 1RM showed no significant improvement in muscle hypertrophy and strength (Luebbers et al., 2014; Winchester et al., 2020). As muscle resistance increases, muscle contraction has internal restrictions on blood flow, and external cuff pressure may fail to produce a positive effect. In addition, excessive blood flow restriction causes severe fatigue (Teixeira et al., 2018). Based on previous findings (Niu et al., 2020), the effect may be optimal at training loads not exceeding 30% 1RM.
The studies of the training duration showed that the effect size increased with the number of weeks of training and reached a maximum at ≥ 4 weeks. Blood flow restriction training increases not only muscle hypertrophy and strength but also the adaptation of muscles to physiological load. Therefore, the training should be performed in a low-load, high-frequency, and incremental manner.
The frequency of blood flow restriction training ranged from 2 to 5 times/week (5 days per week) (Yasuda et al., 2005; Liu et al., 2018). Fujita et al. found that the maximum strength of the knee extensor was increased after 6 days (twice a day) of training at 20% 1RM (Fujita et al., 2008). In contrast, blood flow restriction training to subjective exhaustion was reported to reduce training frequency (Winchester et al., 2020). Research has shown that although acute blood flow restriction training enhanced muscle activation, low-intensity blood flow restriction training was less effective than high-intensity resistance training in improving muscle strength; however, the effect of blood flow restriction training gradually appeared with the increase of training frequency (Fitschen et al., 2015). The present study showed that the performance was optimal at > 3 times/week.
Cuff pressure is an important factor affecting blood flow restriction training and the blood flow restriction in extremities, and thus a fundamental condition of this training method. Cuff pressure causes difficulty in convective oxygen transport, leading to the accumulation of metabolites such as lactate and increased secretion of the growth hormone (Takano et al., 2005; Fatela et al., 2018), thus achieving similar effects as high-intensity exercises. The peak-value effect size varied in the range of cuff pressure (80–200 mmHg) applied in the analyzed studies, and the data suggest that a maximum cuff pressure ≤ of 200 mmHg had the best effect on increasing muscle function.
Based on the above analyses, the following aspects should be noted in future studies. 1) A meta-analysis should be based on a comprehensive collection of previous studies, and the unavailability of unpublished articles and unreported negative results should always be addressed. 2) In this study, we only considered the effects of blood flow restriction training on muscle strength indicators, and future studies could include muscle function, safety, and gender differences. 3) The dose-response relationship for the elderly and rehabilitation populations needs to be further explored. 4) The participants in the included studies could have very different training status. 5) The age range was quite varied (18–78). Aging effects should be further concerned.
Blood flow restriction training significantly improved lower limb muscle strength. The optimal blood f1ow restriction training conditions observed in this meta-analysis were a training load of ≤30% 1RM, training duration of >4 weeks, training frequency of >3 times/week, and a maximum cuff pressure of ≤200 mmHg.
The original contributions presented in the study are included in the article/supplementary material, further inquiries can be directed to the corresponding author.
QY: Methodology, Data curation, Statistical analysis, Visualization, Writing, and Editing. XH: Methodology, Investigation, Data curation and Visualization YL: Methodology and Investigation, Data curation and Visualization YZ: Investigation. CD: Investigation. GL: Methodology, Writing, Critical revision, Revising. JS: Methodology, Writing, Revising, Funding acquisition. All authors have approved the final version of the manuscript.
This study obtained in 2020 and is supported by the national social science fund late outstanding doctoraldissertation project funding (project number: 20 FTYB008).
The authors declare that the research was conducted in the absence of any commercial or financial relationships that could be construed as a potential conflict of interest.
All claims expressed in this article are solely those of the authors and do not necessarily represent those of their affiliated organizations, or those of the publisher, the editors and the reviewers. Any product that may be evaluated in this article, or claim that may be made by its manufacturer, is not guaranteed or endorsed by the publisher.
Abe T., Fujita S., Nakajima T., Sakamaki M., Ozaki H., Ogasawara R., et al. (2010b). Effects of low-intensity cycle training with restricted legblood flow on thigh muscle volume and vo2max in young men. J. Sports Sci. Med. 9 (3), 452–458. doi:10.1111/j.1475-097X.2012.01126.x
Abe T., Kawamoto K., Yasuda T., Kearns C. F., Midorikawa T., Sato Y. (2005). Eight days KAATSU-resistance training improved sprint but not jump performance in collegiate male track and field athletes. Int. J. KAATSU Ttaining. Res. 1 (1), 19–23. doi:10.3806/ijktr.1.19
Abe T., Loenneke J. P., Fahs C. A., Rossow L. M., Thiebaud R. S., Bemben M. G. (2012). Exercise intensity and muscle hypertrophy in blood flow–restricted limbs and non-restricted muscles: A brief review. Clin. Physiol. Funct. Imaging 32 (4), 247–252. doi:10.1111/j.1475-097X.2012.01126.x
Abe T., Sakamaki M., Fujita S., Ozaki H., Sugaya M., Sato Y., et al. (2010). Effects of low-intensity walk training with restricted leg blood flow on muscle strength and aerobic capacity in older adults. J. Geriatr. Phys. Ther. 33 (1), 34–40.
Aune D., Sen A., Henriksen T., Saugstad O. D., Tonstad S. (2016). Physical activity and the risk of gestational diabetes mellitus: A systematic review and dose-response meta-analysis of epidemiological studies. Eur. J. Epidemiol. 31 (10), 967–997. doi:10.1007/s10654-016-0176-0
Barcelos L. C., Nunes P. R., Desouza L. R., de Oliveira A. A., Furlanetto R., Marocolo M., et al. (2015). Low-load resistance training promotes muscular adaptation regardless of vascular occlusion, load, or volume. Eur. J. Appl. Physiol. 115 (7), 1559–1568. doi:10.1007/s00421-015-3141-9
Beekley M. D., Sato Y., Abe T. (2005). KAATSU-walk training increases serum bone-specific alkaline phosphatase in young men. Int. J. KAATSU Ttaining. Res. 1 (2), 77–81. doi:10.3806/ijktr.1.77
Bjørnsen T., Wernbom M., Kirketeig A., Paulsen G., Samnoy L., Bækken L., et al. (2019). Type 1 muscle fiber hypertrophy after blood flow–restricted training in powerlifters. Med. Sci. Sports Exerc. 51, 288–298. doi:10.1249/MSS.0000000000001775
Cumpston M., Li T., Page M. J., Chandler J., Welch V. A., Higgins J. P., et al. (2019). Updated guidance for trusted systematic reviews: A new edition of the Cochrane Handbook for systematic reviews of interventions. Cochrane Database Syst. Rev. 10, ED000142. doi:10.1002/14651858.ED000142
Dankel S. J., Buckner S. L., Jessee M. B., Mattocks K. T., Mouser J. G., Counts B. R., et al. (2017). Can blood flow restriction augment muscle activation during high-load training? Clin. Physiol. Funct. Imaging 38, 291–295. doi:10.1111/cpf.12414
Fahs C. A., Loenneke J. P., Thiebaud R. S., Rossow L. M., Kim D., Abe T., et al. (2015). Muscular adaptations to fatiguing exercise with and without blood flow restriction. Clin. Physiol. Funct. Imaging 35 (3), 167–176. doi:10.1111/cpf.12141
Farup J., Paoli F. D., Bjerg K., RiiS S., Ringgard S., Vissing K. (2015). Blood flow restricted and traditional resistance training performed to fatigue produce equal muscle hypertrophy. Scand. J. Med. Sci. Sports 25, 754–763. doi:10.1111/sms.12396
Fatela P., Reis J. F., Mendonca G. V., Freitas T., Valamatos M. J., Avela J., et al. (2018). Acute neuromuscular adaptations in response to low- intensity blood-flow restricted exercise and high-intensity resistance exercise: Are there any differences? J. Strength Cond. Res. 32 (4), 902–910. doi:10.1519/JSC.0000000000002022
Fitschen P. J., Kistler B. M., Jeong J. H., Chung H. R., Wu P. T., Walsh M. J., et al. (2015). Perceptual effects and efficacy of intermittent or continuous blood flow restriction resistance training. Clin. Physiol. Funct. Imaging 34 (5), 356–363. doi:10.1111/cpf.12100
Fujita T., Brechue W. F., Kurita K., Sato Y., Abe T. (2008). Increased muscle volume and strength following six days of low-intensity resistance training with restricted muscle blood flow. Int. J. KAATSU Ttaining. Res. 4 (1), 1–8. doi:10.3806/ijktr.4.1
Godawa T. M., Credeur D. P., Welsch M. A. (2012). Influence of compressive gear on power lifting performance: Role of blood flow restriction training. J. Strength Cond. Res. 26, 1274–1280. doi:10.1519/JSC.0b013e3182510643
Harper S., Roberts L., Layne A., Jaeger B. C., Gardner A. K., Sibille K. T., et al. (2019). Blood-flow restriction resistance exercise for older adults with knee osteoarthritis: A pilot randomized clinical trial. J. Clin. Med. 8 (2), E265. doi:10.3390/jcm8020265
Jessee M. B., Mouser J. G., Buckner S. L., Dankel S. J., Mattocks K. T., Abe T., et al. (2018). Effects of load on the acute response of muscles proximal and distal to blood florestriction. J. physiological Sci. 68 (3), 1–11.
Keramidas M. E., Kounalakis S. N., Geladas N. D. (2012). The effect of interval training combined with thigh cuffs pressure on maximal and submaximal exercise performance. Clin. Physiol. Funct. Imaging 32, 205–213. doi:10.1111/j.1475-097X.2011.01078.x
Kim D., Singh H., Loenneke J. P., Thiebaud R. S., Fahs C. A., Rossow L. M., et al. (2016). Comparative effects of vigorous-intensity and low- intensity blood flow restricted cycle training and detraining on muscle mass, strength, and aerobic capacity. J. Strength Cond. Res. 30 (5), 1453–1461. doi:10.1519/JSC.0000000000001218
Kubota A., Sakuraba K., Koh S., Ogura Y., Tamura Y. (2011). Blood flow restriction by low compressive force prevents disuse muscular weakness. J. Sci. Med. Sport 14 (2), 95–99. doi:10.1016/j.jsams.2010.08.007
Kubota A., Sakuraba K., Sawaki K., Sumide T., Tamura Y. (2008). Prevention of disuse muscular weakness by restriction of blood flow. Med. Sci. Sports Exerc. 40 (3), 529–534. doi:10.1249/MSS.0b013e31815ddac6
Li Z. Y., Yu S. K., Lou H. Y., Wang Y., et al. (2022). Effect of KAATSU resistance training on body limb circumference, maximum strength and agility of college student male tennis players. FUjian Sports Sci. Technol. 41 (03), 49–54.
Li Z. Y., Zhao Z. G., Wang M. B., Chen C., Wei W. Z., Liang Y. J., et al. (2019). Effect of 4 weeks KAATSU training on body composition and maximum strength of male handball players. China Sport Sci. Technol. 55 (05), 37–43. doi:10.16470/j.csst.2019045
Liu L., Li J., Zhao D. M., Li J., Ning N., et al. (2017). Application of blood flow restriction training in rehabilitation for patients after knee arthroscopy. J. Nurs. Sci. 32 (24), 82–84. doi:10.3870/j.issn.1001-4152.2017.24.082
Liu Y. L., Ye Q., Liu H. W. (2018). Effects of KAATSU and resistance training on bone mineral density, insulin sensitivity, muscle strength and hormone secretion in pre-diabetic state population. Chin. J. Osteoporos. 24 (11), 56–63). doi:10.3969/j.issn.1006.7108.2018.11.011
Lixandrão M. E., Ugrinowitsch C., Laurentino P., Libardi C. A., Aihara A. Y., Cardoso F. N., et al. (2015). Effects of exercise intensity and occlusion pressure after 12 weeks of resistance training with blood-flow restriction. Eur. J. Appl. Physiol. 115 (12), 2471–2480. doi:10.1007/s00421-015-3253-2
Lu J. M., Liu S. Y., Sun P., Lian Z. Q. (2020). Effects of low intensity resistance training of blood flow restriction with different occlusion pressure on lower limb muscle and cardiopulmonary function of college students. Chin. J. Appl. Physiology 36 (06), 595–599. doi:10.12047/j.cjap.6032.2020.125
Luebbers P. E., Fry A. C., Kriley L. M., Butler M. S. (2014). The effects of a 7-week practical blood flow restriction program on well-trained collegiate athletes. J. Strength Cond. Res. 28 (8), 2270–2280. doi:10.1519/JSC.0000000000000385
Luebbers P. E., Witte E. V., Oshel J. Q. (2017). The effects of practical blood flow restriction training on adolescent lower body strength. J. Strength & Cond. Res. 28 (8), 2270–2280. doi:10.1519/JSC.0000000000002302
Madarame H., Neya M., Ochi E., Nakazato K., Sato Y., Ishii N. (2008). Cross-transfer effects of resistance training with blood flow restriction. Med. Sci. Sports Exerc. 40 (2), 258–263. doi:10.1249/mss.0b013e31815c6d7e
Madarame H., Ochi E., Tomioka Y., NaKazato K., Ishii N. (2011). Blood flow-restricted training does not improve jump performance in untrained young men. Acta Physiol. hung. 98 (4), 465–471. doi:10.1556/APhysiol.98.2011.4.10
Manini T. M., Clark B. C. (2009). Blood flow restricted exercise and skeletal muscle health. Exerc. Sport Sci. Rev. 37 (2), 78–85. doi:10.1097/JES.0b013e31819c2e5c
Martín-Hernández P., Menendez H., Ferrero C., Loenneke J. P., Herrero A. J. (2012). Muscular adaptations after two different volumes of blood flow- restricted training. Scand. J. Med. Sci. Sports 23 (2), e114–e120. doi:10.1111/sms.12036
Niu Y. J., Qiao Y. C., Fan Y. Z. (2020). Effects of blood flow restriction training on muscle morphology and function of subjects: A meta-analysis. J. Cap. Univ. Phys. Educ. Sports (1), 25–34. doi:10.14036/j.cnki.cn11-4513.2020.01.005
Park S., Kim J. K., Choi H. M., Kim H. G., Beekley M. D., Nho H. (2010). Increase in maximal oxygen uptake following 2-week walk training with blood flow occlusion in athletes. Eur. J. Appl. Physiol. 469109 (4), 591–600. doi:10.1007/s00421-010-1377-y
Pope Z. K., Willardson J. M., Schoenfeld B. J., Emmett J. D., Owen J. D. (2015). Hypertrophic and strength responses to eccentric resistance training with blood flow restriction: A pilot study. Int. J. Sports Sci. Coach. 10 (5), 919–931. doi:10.1260/1747-9541.10.5.919
Vinícius R. V., Letieri H., Lamboglia C. G., Rees J. L., Gomes B. B. (2018). Effect of 16 weeks of resistance exercise and detraining comparing two methods of blood flow restriction in muscle strength of healthy older women: A randomized controlled trial. Exp. Gerontol. 114, 78–86. doi:10.1016/j.exger.2018.10.017
Schwenzer T., Schwenzer C., Schwenzer M., Fernandes A. R., Parcell A. C., RicardM. , et al. (2008). Effects of strength training and vascular occlusion. Int. J. Sports Med. 29 (08), 664–667. doi:10.1055/s-2007-989405
Schwiete C., Franz A., Roth C., Behringer M. (2021). Effects of resting vs. Continuous blood-flow restriction- training on strength, fatigue resistance, muscle thickness, and perceived discomfort. Front. Physiol. 12, 663665. doi:10.3389/fphys.2021.663665
Sheng J. J., Wei W. Z., Sun K., Zhao Z. G. (2019). Physiological load and effect on muscle hypertrophy of slow downhill walking with KAATSU. China Sport Sci. Technol. 055 (003), 13–19. doi:10.16470/j.csst.2019025
Slysz J., Burr J. F. (2017). The effects of blood flow restricted electrostimulation on strength & hypertrophy. J. Sport Rehabilitation 27 (3), 257–262. doi:10.1123/jsr.2017-0002
Slysz J., Burr J. F. (2016). The efficacy of blood flow restricted exercise: A systematic review & meta- analysis. J. Sci. Med. Sport 19 (8), 669–675. doi:10.1016/j.jsams.2015.09.005
Sumide T., Sakuraba K., Sawaki K., Ohmura H., Tamura Y. (2010). Effect of resistance exercise training combined with relatively low vascular occlusion. J. Sci. Med. Sport 12 (1), 107–112. doi:10.1016/j.jsams.2007.09.009
Takano H., Morita T., Iida H., Asada K. i., Kato M., Uno K., et al. (2005). Hemodynamic and hormonal responses to a short-term low- intensity resistance exercise with the reduction of muscle blood flow. Eur. J. Appl. Physiol. 95 (1), 65–73. doi:10.1007/s00421-005-1389-1
Takarada Y., Sato Y., Ishii N. (2002). Effects of resistance exercise combined with vascular occlusion on muscle function in athletes. Eur. J. Appl. Physiol. 86 (4), 308–314. doi:10.1007/s00421-001-0561-5
Tang Y. D., Qu C. G. (2022). Effect of 4 weeks KAATSU resistance training on lower limb muscle strength and sprint ability of male road cyclists. Liaoning Sports Sci. Technol. 44 (05), 63–69. doi:10.13940/j.cnki.lntykj.2022.05.022
Teixeira E. L., Barroso R., Silva-Batista C., Laurentino G. C., Loenneke J. P., Roschel H., et al. (2018). Blood flow restriction increases metabolic stress but decreases muscle activation during high-load resistance exercise. Muscle Nerve 57, 107–111. doi:10.1002/mus.25616
Teixeira E. L., Painelli V. D., Silva-batistal C., de Souza Barros T., Longo A. R., Lasevicius T., et al. (2021). Blood flow restriction does not attenuate short-term detraining-induced muscle size and strength losses after resistance training with blood flow restriction. J. Strength Cond. Res. 35 (8), 2082–2088. doi:10.1519/JSC.0000000000003148
Tennent D. J., Hylden C. M., Johnson A. E., Burns T. C., Wilken J. M., Owens J. G. (2017). Blood flow restriction training after knee arthroscopy: A randomized controlled pilot study. Clin. J. Sport Med. 27 (3), 245–252. doi:10.1097/JSM.0000000000000377
Tetsuo Y., Richard S. F., Jennifer L. (2012). Occlusion training increases muscular strength in division ia football players. J. Strength Cond. Res. 26 (9), 2523–2529. doi:10.1519/JSC.0b013e31823f2b0e
Tomohiro Y., Riki O., Mikako S., Ozaki H., Sato Y., Abe T. (2011). Combined effects of low-intensity blood flow restriction training and high-intensity resistance training on muscle strength and size. Eur. J. Appl. Physiol. 111 (10), 2525–2533. doi:10.1007/s00421-011-1873-8
Vechin F. C., Libardi C. A., Conceição M. S., Damas F. R., Lixandrao M. E., Berton R. P. B., et al. (2015). Comparisons between low-intensity resistance training with blood flow restriction and high-intensity resistance training onquadriceps muscle mass and strength in elderly. J. Strength Cond. Res. 29 (4), 1071–1076. doi:10.1519/JSC.0000000000000703
Wang M. B., Li Z. Y., Wei W. Z., Zhao Z. G., Chen C., Huang J. P., et al. (2019). Empirical study of KAATSU training effect on lower limb of male elite handball player. China Sport Sci. Technol. 055 (005), 30–36. doi:10.16470/j.csst.2019055
Winchester L. J., Morris C. E., Badinger J., Wiczynski T. L., VanWye W. R. (2020). Blood flow restriction at high resistance loads increases the rate of muscular fatigue, but does not increase plasma markers of myotrauma or inflammation. J. Strength Cond. Res. 34 (9), 2419–2426. doi:10.1519/JSC.0000000000003742
Wu Y., Li Q., Bao D. P. (2019). The effects of KAATSU training on the lower limb: A meta-analysis. China Sport Sci. Technol. 055 (003), 20–26. doi:10.16470/j.csst.2019030
Xu F., Wang J. (2013). KAATSU training: Interpretation and applications. China Sport Sci. 370 (12), 71–80. doi:10.16469/j.css.2013.12.015
Yasuda T., Fukumura K., Fukuda T., Uchida Y., Iida H., MeguroM. , et al. (2015). Muscle size and arterial stiffness after blood flow- restricted low-intensity resistance training in older adults. Scand. J. Med. Sci. Sports 24 (5), 799–806. doi:10.1111/sms.12087
Yasuda T., Fukumura K., Sato Y., Yamasoba T., Nakajima T. (2014). Effects of detraining after blood flow-restricted low- intensity training on muscle size and strength in older adults. Aging Clin. Exp. Res. 26 (5), 561–564. doi:10.1007/s40520-014-0208-0
Yasuda T., Ogasawara R., Sakamaki M., Bemben M. G., Abe T. (2011). Relationship between limb and trunk muscle hypertrophy following high-intensity resistance training and blood flow–restricted low-intensity resistance training. Clin. Physiol. Funct. Imaging 31 (5), 347–351. doi:10.1111/j.1475-097X.2011.01022.x
Yasuda T., Yasuda T., Midorikawa T., Sato Y., Kearns C. F., Inoue K., et al. (2005). Skeletal muscle size and circulating IGF-1 are increased after two weeks of twice daily KAATSU resistance training. Int. J. KAATSU Ttaining. Res. 1 (1), 6–12. doi:10.3806/ijktr.1.6
Keywords: blood flow restriction, dose-response relationship, muscle strength, meta-analysis, lower limb muscle strength
Citation: Yang Q, He XJ, Li YD, Zhang YZ, Ding CS, Li GX and Sun J (2022) Dose-response relationship of blood flow restriction training on isometric muscle strength, maximum strength and lower limb extensor strength: A meta-analysis. Front. Physiol. 13:1046625. doi: 10.3389/fphys.2022.1046625
Received: 16 September 2022; Accepted: 08 November 2022;
Published: 15 December 2022.
Edited by:
Emiliano Cè, University of Milan, ItalyReviewed by:
Žiga Kozinc, University of Primorska, SloveniaCopyright © 2022 Yang, He, Li, Zhang, Ding, Li and Sun. This is an open-access article distributed under the terms of the Creative Commons Attribution License (CC BY). The use, distribution or reproduction in other forums is permitted, provided the original author(s) and the copyright owner(s) are credited and that the original publication in this journal is cited, in accordance with accepted academic practice. No use, distribution or reproduction is permitted which does not comply with these terms.
*Correspondence: Guo Xing Li, MTEwNzdAZ3pzcG9ydC5lZHUuY24=; Jian Sun, c3VuamlhbkBnenNwb3J0LmVkdS5jbg==
†These authors share first authorship
Disclaimer: All claims expressed in this article are solely those of the authors and do not necessarily represent those of their affiliated organizations, or those of the publisher, the editors and the reviewers. Any product that may be evaluated in this article or claim that may be made by its manufacturer is not guaranteed or endorsed by the publisher.
Research integrity at Frontiers
Learn more about the work of our research integrity team to safeguard the quality of each article we publish.