- 1Guizhou Provincial Key Laboratory for Agricultural Pest Management of the Mountainous Region, Institute of Entomology, Guizhou University, Guiyang, China
- 2Guizhou Provincial Key Laboratory for Rare Animal and Economic Insect of the Mountainous Region, College of Biology and Environmental Engineering, Guiyang University, Guiyang, China
- 3China Tobacco Guizhou Industrial Co. Ltd, Guiyang, China
The Scalloped (Sd) is a transcription factor that regulates organ size control in the Hippo-signaling pathway. Recent studies have showed that Hippo signaling also functions in the innate immune response. Although the Sd gene has been reported in many insects, their immune functions remain unexplored. In this study, the LsSd gene of Lasioderma serricorne, with a complete open reading frame that encodes a protein composed of 402 amino acids was identified. LsSd was predominantly expressed in early pupae. Tissue-specific analyses revealed that the highest concentrations of LsSd were detected in the midgut and brain. At 1–24 h after Escherichia coli infection, LsSd expression increased substantially. However, LsSd expression was downregulated 3–12 h after Staphylococcus aureus infection. RNA interference-mediated silencing of the LsSd transcript resulted in deformed, considerably smaller, and degenerated wings. Meanwhile, LsCycE, LsDiap1, and LsVg, which are involved in cell proliferation and wing development, were drastically reduced when LsSd was depleted. In a survival assay, the LsSd knockdown considerably decreased the susceptibility to S. aureus, a gram-positive bacterium. In addition, knockdown of LsSd remarkably downregulated the transcription of LsCact in response to S. aureus stimulation, while upregulating the expression of five immune-related genes. Our results provide conclusive proof for the important roles of LsSd in the immune response of L. serricorne.
Introduction
The Hippo signaling pathway is a conserved signal transduction network that regulates organ size in a wide range of animal species (Misra and Irvine, 2018). In Drosophila melanogaster, the core of this pathway is composed of four proteins: the kinases Hippo and Warts (Wts) and their cofactors Salvador and Mob as a tumor suppressor (Liu et al., 2016). Genetic screening revealed that they were initially identified as Drosophila tumor suppressors. Loss-of-function mutations in any of these four genes dysregulate tissue growth and development in D. melanogaster (Staley and Irvine, 2012). Yorkie (Yki) is the central effector of the Hippo signaling pathway and plays a crucial role in the regulation of cell proliferation and apoptosis. Activation of this signaling cascade will phosphorylate the transcription co-activator Yki in order to promote apoptosis and limit organ size overgrowth (Zhao et al., 2011). When the Hippo signaling pathway kinase cascade is inactivated, Yki accumulates in the nucleus and binds to transcription factors to promote the expression of target genes involved in cell growth, proliferation, and survival.
The wing plays a crucial role in the environmental adaptation of insects. It enables insects to move efficiently for dispersal, feeding, mating, and escape from unfavorable environments and predators (Ye et al., 2022). Studies have shown that the Hippo-signaling pathway is necessary for wing formation during D. melanogaster development. For instance, mutations of Wts that increase Yki activity result in wing overgrowth, whereas loss of Yki activity decreases wing growth (Pan et al., 2018). Scalloped (Sd), a transcription factor, is a critical partner of Yki. It forms a transcriptional complex with Yki to promote cell growth and proliferation (Zhang et al., 2008). Sd can mediate various functions by interacting with tissue-specific co-factors, such as Vestigial (Vg), D. melanogaster homologue of Myocyte enhancer factor-2 (Dmef2), and Nerfin-1 (Bandura and Edgar, 2008; Deng et al., 2009; Vissers et al., 2018). Loss of Sd function results in Yki-dependent transcription and organ size reduction, whereas Sd activation promotes tissue overgrowths. Sd mutations in D. melanogaster result in abnormal wings with ectopic bristles and wing margin gaps (Campbell et al., 1991; Simmonds et al., 1998). In Bombyx mori, BmSd knockdown or knockout resulted in small, curled wings (Yin et al., 2020). Similarly, in Locusta migratoria, gene silencing of LmSd by RNA interference (RNAi) resulted in deformed wings (Zhang et al., 2021a). In addition to its function in wing development, Sd affects the development of other D. melanogaster organs, including the eyes, legs, optic lobe, and lymph glands (Garg et al., 2007; Ferguson and Martinez-Agosto, 2017).
Surprisingly, the Hippo signaling pathway is also associated with immune responses. In D. melanogaster, the Hippo–Yki pathway is required for the regulation of innate immunity. Loss of suppressors of the Hippo signaling pathway tumor or activation of Yki in fat bodies causes elevated Cactus (Cact) mRNA levels, which inhibits Toll receptor-mediated antimicrobial response and increases susceptibility to gram-positive bacterial infection. Furthermore, gram-positive bacteria activate Hippo–Yki signaling via the Toll-Myd88-Pelle cascade through Pelle-mediated phosphorylation and degradation of the Cka subunit of the STRIPAK PP2A complex (Liu et al., 2016). In the Chinese crab (Eriocheir sinensis), both gram-positive and gram-negative bacteria induced the activation of Hippo signaling and inhibited the expression of Cact, causing the nuclear accumulation of Dorsal from the Toll pathway to upregulate the expression of antimicrobial peptide genes (Yang et al., 2019). In conclusion, the aforementioned studies demonstrated that the Hippo signaling pathway is essential for the regulation of antibacterial immunity.
The cigarette beetle, L. serricorne (Fabricius), is a widespread and destructive storage pest worldwide due to its high reproductive potential and adaptability (Kim et al., 2003; Mahroof and Phillips, 2008). It causes damage to tobacco products, cereal grains, materials used in Chinese medicine, and processed foods (Abdelghany et al., 2016). It can cause massive economic damage to stored materials through larval feeding and feces production (Riudavets et al., 2007). In general, the effective management of L. serricorne relies heavily on the use of phosphine-containing fumigants, which has led to several risks, including environmental pollution, pest resurgence, and the development of insecticide resistance (Saglam et al., 2015). Owing to these detrimental effects, innovative and long-term control strategies are required. RNAi has considerable potential to prevent pest damage to stored food (Zhu and Palli, 2020). Given the importance of Sd in the Hippo-signaling pathway, it is anticipated that Sd will become an alternative molecular target of RNAi, thereby reducing the use of pesticides in pest control.
In this study, we identified the orthologs of Sd from L. serricorne (named as LsSd) and determined its mRNA profiles and gene functions in wing development and innate immune. These results will clarify the roles of LsSd in the immune response of L. serricorne and lay a foundation for further research on the functions of LsSd.
Materials and methods
Insect rearing
L. serricorne adults were collected from a tobacco warehouse in Guiyang city, Guizhou province, China, in the fall of 2016. The larvae were fed a mixture of crushed maize, beer yeast, and dried tobacco. The beetles were kept in an insectary at 25 ± 1°C under a 16 h: 8 h light-dark photoperiod and 60 ± 5% relative humidity (RH).
Gene cloning and sequence analysis
LsSd gene was identified by local-tBlastn searches using homolog sequences from Tenebrio molitor, Diabrotica virgifera, or Tribolium castaneum as queries against the L. serricorne pupae transcriptome (SRR13065789). LsSd cDNA were amplified by reverse transcriptase polymerase chain reaction (RT-PCR) using the LsSd-specific primers (Supplementary Table S1). Thermal cycling was carried out in a thermocycler (T100 Thermal Cycler, Bio-Rad, Hercules, CA, United States) with the following reaction program: 94°C for 3 min; 34 cycles of 94°C for 30 s, 57°C for 30 s, and elongation at 72°C for 1.5 min; and then an extension at 72°C for 10 min. Amplified PCR product was purified using a DiaSpim Column DNA Gel Extraction Kit (B110092, Sangon Bioech, China) and sequenced in both directions by Sangon Bioech.
The NCBI ORF finder (https://www.ncbi.nlm.nih.gov/orffinder/) was used to predict the open reading frame and protein sequence of LsSd. DNAMAN 6.0 software (LynnonBiosoft, Vaudreuil, Quebec, Canada) was used to analyze the cDNA sequence and deduce the amino acid sequence. Domain structures were determined by using ProCite (https://prosite.expasy.org) and SMART (http://smart.embl-heidelberg.de/smart/) servers. The molecular weight and isoelectric point were computed by the ExPASy Proteomics Server (http://www.expasy.ch). Using Clustal W, the amino acid sequences deduced for the Sds were aligned. Phylogenetic analysis and phylogenetic tree construction were performed using MEGA Version 6 with the neighbor-joining (NJ) method and a p-distance model with 1,000 bootstrap replicates.
Expression pattern of LsSd
RT-qPCR was used to determine the expression of LsSd in different developmental stages (early larvae (mixture of 1-2 old days), late larvae (30-day-old), early pupae (1-day-old), late pupae (5-day-old), early adults (1-day-old), and late adults (7-day-old)), different tissues from the late larvae (brain, integument, fat body, midguts, and Malpighian tubules) and different tissues from the early adults (head, thorax, abdomen, and wings) were used.
Gram-positive bacterium S. aureus (ATCC25923) and Gram-negative bacterium E. coli (ATCC25922) were purchased from Solarbio (Beijing, China). The bacterial cells were cultured in Luria-Bertani (LB) broth at 37°C. S. aureus and E. coli were cultured until their respective optical densities reached approximately 0.6 and 0.5 at 600 nm. Each late-stage larva was injected with 100 nL of the pathogen suspensions or endotoxin-free sterile water according to the method described previously (Yan et al., 2021). The larvae were transferred to an artificial diet and maintained in 9 cm diameter Petri dishes) The samples were collected at 1, 3, 6, 12, and 24 h after injection. Each treatment consisted of three replicates, and each replicate comprised a pool of 10 individuals. The total RNA was extracted from L. serricorne larvae using HP Total RNA kit (Omega Bio-Tek, Norcross, GA, United States). One microgram of total RNA was reverse-transcribed in a 20 μL reaction mixture containing the HiFiScript gDNA Removal cDNA Synthesis Kit (CW2582M, CWBIO, China) according to the manufacturer’s protocols. The RT-qPCR analysis was conducted with FastStart Essential DNA Green Master Mix (Roche, Indianapolis, IN, United States) and the primers listed in Supplementary Table S1. The thermal cycling was carried out in a CFX96 Touch™ Real-Time PCR Detection System (Bio-Rad, Hercules, CA, United States) under the following reaction conditions: 95°C for 10 min, then 40 cycles (95°C for 30 s and 60°C for 30 s), followed by melting from 65°C to 95°C. The RPL13a gene served as reference gene (Zhang et al., 2021b). The relative expression levels of the LsSd gene were determined using the 2−ΔΔCt method (Livak and Schmittgen, 2001). There were three biological replicates and three technical replicates for each biological replicate.
Functional analysis based on RNA interference
The LsSd targets fragment for RNAi was amplified by PCR using the gene-specific primers ds-LsSd-F and ds-LsSd-R (Supplementary Table S1). The double-stranded RNA (dsRNA) targeting the LsSd gene were prepared in vitro using the TranscriptAid T7 High Yield Transcription Kit (Thermo Scientific, Waltham, MA, United States). The purified dsRNA was quantified using a NanoDrop 2000 spectrophotometer (Thermo Scientific, Waltham, MA, United States). The quality and molecular size of dsRNA products were determined in a 1.2% agarose gel. GFP dsRNA (a nonspecific negative control) was synthesized as described previously (Yang et al., 2020).
To investigate the roles of LsSd in L. serricorne development, 300 ng gene-specific dsRNA was injected into the body cavity of each late larvae or early pupae. Ten larvae were sampled between 12 and 72 h after dsRNA injection. The efficiency of RNAi was detected by RT-qPCR, as described above. Post-injection insects were reared under the same conditions, as mentioned above, to observe their growth, development, and phenotypes. After LsSd knock down by dsRNA injection for 24 h, the relative expression levels of one wing development-related gene (LsVg) and two genes related to cell proliferation and death (LsCycE and LsDiap1) were analyzed by RT-qPCR as described above.
To further investigate the role of LsSd in immune defense, we injected ds-LsSd into late larvae and stimulated them with 200 nL of bacterial suspension (S. aureus OD600 = 1.2 or E. coli OD600 = 1) 24 h later using the previously described method. Transcript levels of LsSd, LsCact and six immune-related genes (LsAtt2, LsDef1, LsDef2, LsCole, LsLysC, and LsLysI) were determined at 12 h using RT-qPCR. Each group of fifty insects was to be observed for 5 days at 1-day intervals. Each experiment involved three biological replications.
Statistical analysis
The statistical analyses and graphs were generated using GraphPad Prism 8 software (GraphPad Software, San Diego, CA, United States). One-way analysis of variance (ANOVA) was followed by a Tukey HSD test was used to compare the gene expression level of LsSd under each normalization treatments. The Student’s t-test was used to determine statistical significance between treatment group and the control group. The Kaplan-Meir log-rank test was used to examine survival rates. p < 0.01 was regarded as extremely significant, while value of p < 0.05 was considered statistically significant.
Results
Bioinformatics analysis of the LsSd sequence
The putative cDNA sequence of LsSd was identified and validated using reverse transcriptase polymerase chain reaction (RT-PCR) from the L. serricorne pupae transcriptome. The open reading frame (ORF) contained 1,209 base pairs (bp) and encoded a polypeptide with 402 amino acids, a calculated molecular mass of 45.301 kDa, and an isoelectric point (pI) of 6.81. The ORF sequence (GenBank accession number: ON533736) was submitted to GenBank. Similar to Apis mellifera, Bombyx mandarina, and D. melanogaster, the amino acid sequence of LsSd contains an N-terminal TEA/ATTS (TEA) domain and a C-terminal YAP binding domain (YBD) (Figure 2A). Multiple sequence alignments showed that insect Sd proteins are highly conserved. (Supplementary Figure S1). To illustrate the molecular evolution of Sd, 14 mature Sd protein sequences were aligned to generate a neighbor-joining (NJ) tree. The dendrogram showed that Sd proteins of insects in each order clustered on a single branch (Figure 1B).
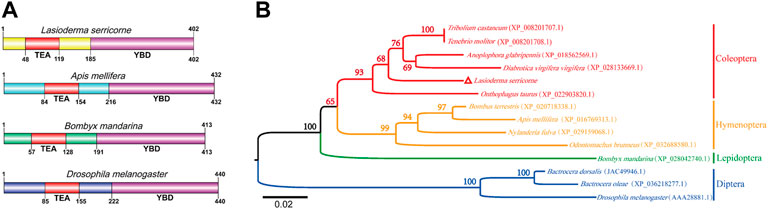
FIGURE 1. Phylogenetic tree and predicted structure of the Sd proteins in insects. (A) The deduced amino acid sequences were used to predict the domain of Sd proteins using SMART tool. (B) The phylogenetic tree of Sd protein was constructed by MEGA6 software using the neighbor-joining method with 1,000 bootstrap replications. Numbers at the branch nodes represent the level of bootstrap support for each branch.
Expression pattern analysis of LsSd
LsSd mRNA among developmental stages, different larvae tissues, and different adult tissues of L. serricorne was determined by RT-qPCR. mRNA levels of LsSd were widely expressed in diverse tissues and developmental stages (Figure 2). The LsSd transcription levels were considerably higher in pupae and adults than in larval instars (Figure 2A). The expression levels of LsSd were the highest in the midgut and brain compared with all other analyzed larval tissues. Only slight expression was detected in Malpighian tubules (Figure 2B). The expression levels of LsSd mRNA were found to be the highest in the abdomen of adult L. serricorne, followed by the thorax, wings, and head (Figure 2C).
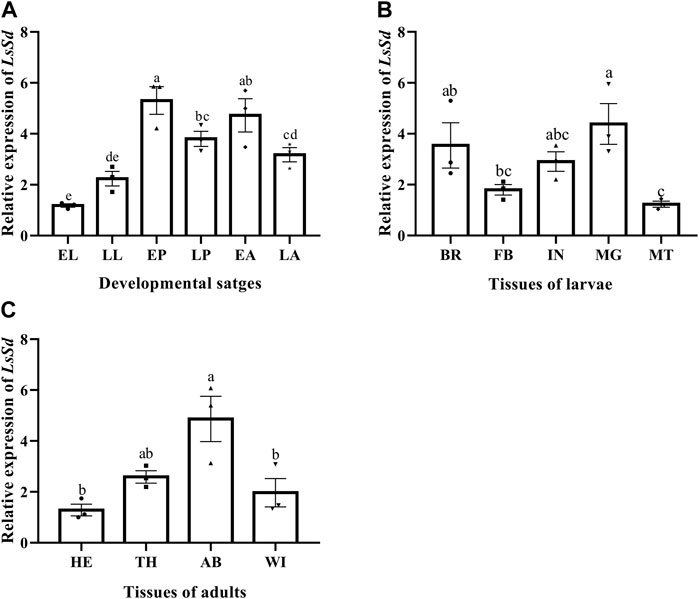
FIGURE 2. LsSd expression in the different developmental stages (A), different larval tissues (B) and different adult tissues (C) of L. serricorne. EL, early larvae; LL, late larvae; EP, early pupae; LP, late pupae; EA, early adults; LA, late adults; BA, brain; FB, fat body; IN, integument; MG, midgut; MT, Malpighian tubules; HE, head; TH, thorax; AB, abdomen; Wing, WI. Error bars indicate the SEM of three independent biological replications. Different letters above the bars show significant differences at the 0.05 level (one-way ANOVA followed by Tukey’s test).
Changes in LsSd mRNA expression levels in response to immune challenges with E. coli and S. aureus were measured (Figure 3). The LsSd mRNA expression levels are upregulated 1–24 h after an E. coli challenge, but decrease 3–12 h after an S. aureus infection, according to our findings. This finding suggests a putative role of LsSd in systemic immune signaling.
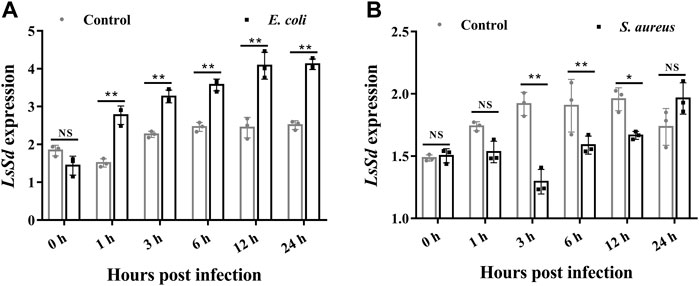
FIGURE 3. The mRNA transcript levels of LsSd in whole larvae (30-day-old) after microbial challenge. After injections of E. coli (A) and S. aureus (B), the mRNA transcript levels of LsSd were analyzed by RT-qPCR. Error bars indicate the SEM of three independent biological replications. Significant differences between the microbial challenge group and control group were determined using Student’s t-test (*p < 0.05, **p < 0.01).
Functional analysis of LsSd based on RNAi
To investigate the biological function of LsSd in L. serricorne wing development, dsRNA for LsSd and dsGFP were injected into early pupae. The RT-qPCR results showed that the transcription level was considerably reduced in comparison with dsGFP-injected pupae. After 72 h of treatment with dsLsSd, the RNAi efficiency was 56% compared with injections of GFP dsRNA (Figure 4A). RNAi in embryos can cause morphological defects in adults. Adult elytra and hindwings exhibited deformation in dsLsSd-injected individuals. Compared with the dsGFP-injected adults, the deformed elytra were smaller and more fragile. Meanwhile, the hindwings were curved and wrinkled (Figure 4B). To further investigate the causes of the changes in the wing structure and morphology of L. serricorne following LsSd RNAi, the expression levels of cell division and death genes were analyzed by RT-qPCR. The expression levels of LsCyclinE (LsCycE), LsDiap1, and LsVestigial (LsVg) were considerably lower than those of the control group (Figure 4C).
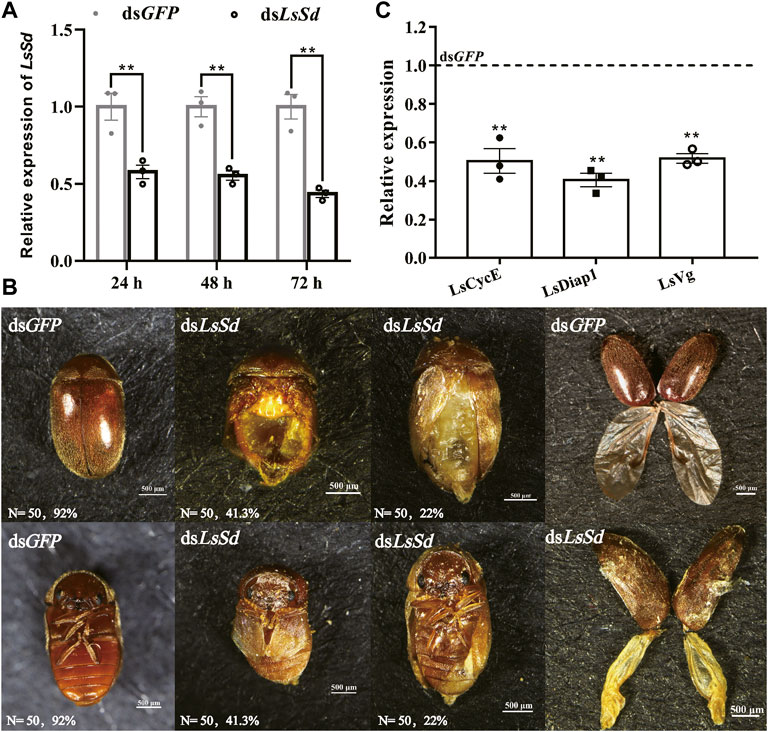
FIGURE 4. The effect of LsSd knockdown on adult phenotypic characterization in L. serricorne. (A) The expression levels of the LsSd gene after RNAi treatment in L. serricorne pupae. (B) Dorsal and ventral views of adults treated with dsLsSd and dsGFP, and structural comparison of wings after LsSd or GFP dsRNA injection. (C) The expression levels of genes related to cell proliferation and wing were examined by RT-qPCR after injection of dsLsSd in 24 h. Error bars indicate the SEM of three independent biological replications. Significant differences between control group and RNAi group were determined by Student’s t test. **p < 0.01.
Knockdown of LsSd promoted the survival rate of L. serricorne
To determine the role of LsSd in innate immunity, dsRNA was injected into L. serricorne larvae, which effectively inhibited LsSd expression (Figure 5A). There were no statistically significant differences between the pupation rates of dsLsSd-injected and control larvae (Figure 5B). Next, we challenged LsSd knockdown larvae with E. coli or S. aureus and then assessed the survival of these larvae for 5 days. There was no significant difference in survival between LsSd knockdown larvae infected with E. coli and controls (Figure 5C). However, LsSd RNAi-treated larvae exhibited decreased sensitivity to the gram-positive bacterium S. aureus (Figure 5D).
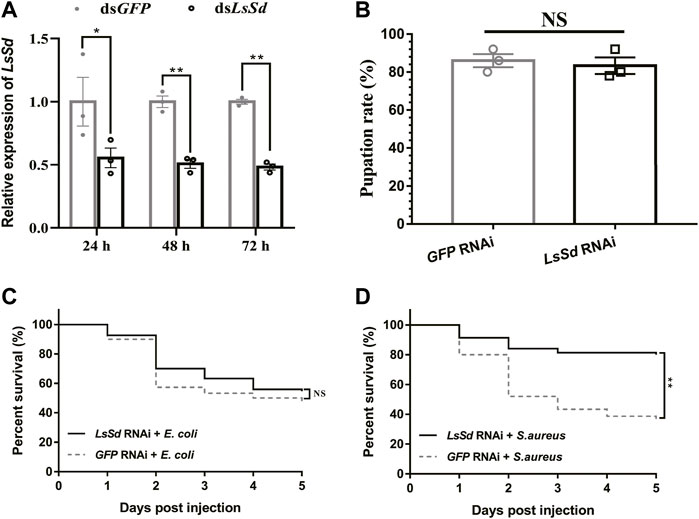
FIGURE 5. Susceptibility of L. serricorne larvae against E. coli and S. aureus infections after knockdown of LsSd. (A) RNAi efficiency of the LsSd gene was detected at 24, 48, and 72 h after dsRNA treatment in L. serricorne larvae. (B) Effect of LsSd RNAi on L. serricorne pupation rate. (C) E. coli and (D) S. aureus were inoculated at 24 h post LsSd RNAi, and larval survival was recorded daily for 5 days in each treatment group. Error bars indicate the SEM of three independent biological replications. Statistical analysis of survival analysis was carried out based on Kaplan-Meier method (Log-rank Chi-square test; **p < 0.01).
LsSd regulated AMPs’ expression via LsCact
It is demonstrated that Cact is a direct target of the Yki-Sd transcription factor complex (Liu et al., 2016). To determine whether LsSd also influenced AMP expression via LsCact in L. serricorne, we measured the transcript levels of LsCact in LsSd-silenced whole larvae following microbial challenge. After S. aureus infection of larvae injected with dsLsSd, the expression level of LsCact was considerably reduced (Figure 6A). In contrast, LsSd knockdown has no influence on LsCact expression under E. coli-challenged conditions (Figure 6A). Then, we determined the expression levels of six AMPs in L. serricorne larvae in response to a bacterial challenge (Figure 6B). The expression of LsAtt2, LsDef2, and LsCole was strong induced after E. coli infection (Figure 6B). The expression levels of LsDef2 and LsLysC were considerably upregulated following S. aureus infection (Figure 6C). These results demonstrated that such AMPs are necessary for host defense against infection. To determine whether LsSd regulated the expression of AMPs, we knocked down LsSd expression in larvae. This resulted in a significant upregulation of the expressions of LsDef1, LsDef2, LsCole, LsLysC, and LsLysI in response to S. aureus stimulation (Figure 6E). In contrast, LsSd knockdown decreased LsDef2, LsCole, and LsLysC expression in E. coli-challenged conditions (Figure 6D). These findings support the hypothesis that LsSd knockdown reduces susceptibility to S. aureus. Our findings imply that LsSd knockdown affected the transcription of LsCact and five immune-related genes (Figure 6F).
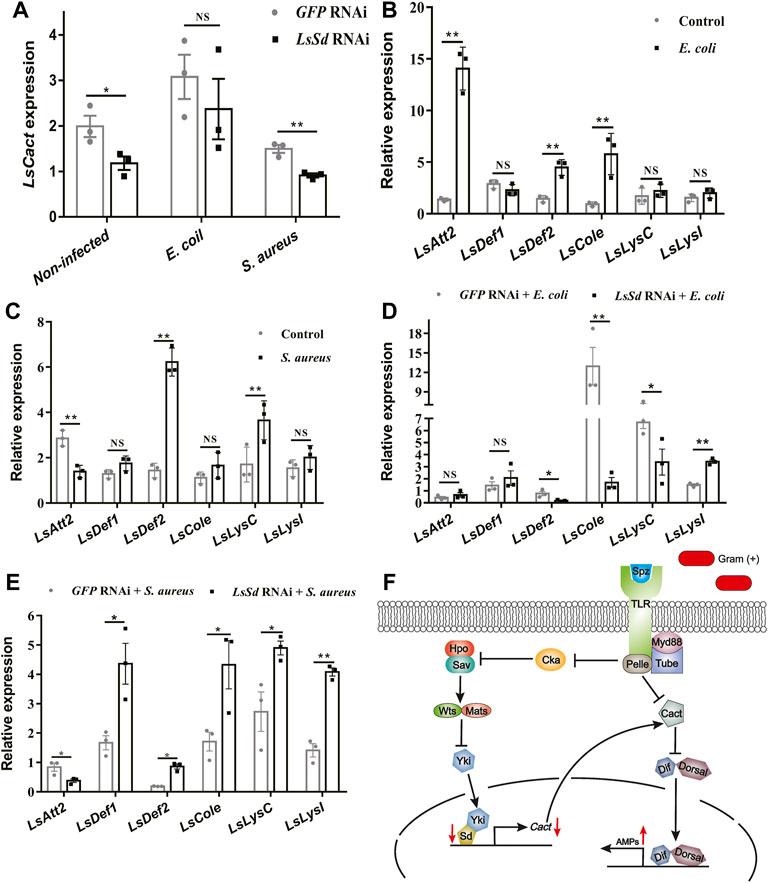
FIGURE 6. LsSd regulates production of anti-microbial peptides by acting LsCact. (A) LsSd-silenced larvae were treated with or without E. coli and S. aureus stimulations. The mRNA transcript levels of LsCact were analyzed at 12 h post-stimulation using RT-qPCR. After challenge injections of (B) E. coli (C) and S. aureus for 12 h, the mRNA transcript levels of six antimicrobial peptide genes were analyzed by RT-qPCR. (D) E. coli and (E) S. aureus were used to stimulate LsSd-silenced larvae. RT-qPCR was used to determine AMPs’ expression at 12 h post-stimulation. (F) Schematic representation based on previous studies (Liu et al., 2016; Huang et al., 2022) for LsSd-mediated regulation of AMPs in response to the Gram-positive bacteria S. aureus. Error bars indicate the SEM of three independent biological replications. Asterisks above bars indicate significant differences (*p < 0.05; **p < 0.01) analyzed by a Student’s t test.
Discussion
Insect wings are an important organ for successful radiation of this animal group. Insect wing development is a complex process that requires coordinated regulation of multiple signaling pathways, including Hippo, c-Jun N-terminal kinase (JNK), hedgehog (Hh), and Notch (Glise et al., 2002; Lin et al., 2016; Pan et al., 2018). Sd, a key component of the Hippo-signaling pathway, plays a crucial role in wing development (Ohde et al., 2009; Rohith and Shyamala, 2017). In this study, the complete ORF sequence encoding Scalloped from the cigarette beetle was identified and cloned. Bioinformatic analysis showed that LsSd protein has an N-terminal TEA domain and a C-terminal YBD, which is consistent with the structures of other LsSd proteins (Yin et al., 2020; Zhang et al., 2021a). Study have found only the TEA domain of Scalloped to be critical for wing development (Srivastava et al., 2002). TEAD (the mammalian homolog of Sd) YBD recruits YAP (the mammalian homolog of Yki) to target gene promoters, where it activates gene expression via the Hippo-signaling pathway (Tian et al., 2010). Multiple sequence alignment and phylogenetic analysis revealed that insect Sd proteins have been highly conserved throughout evolution, with LsSd and five other Coleopteran Sd proteins forming a distinct clade.
The differential expression of the LsSd gene in various tissues and developmental stages suggests that LsSd is involved in a vast array of biological processes. Tissue-specific expression analysis revealed that LsSd is highly expressed in the larval brain and midgut, which is consistent with the expression of HvSd from H. vigintioctopunctata (Ohde et al., 2009). The authors speculated that Sd directly or indirectly regulates the expression of genes encoding hormones in the brain, and also functions in larva-to-pupa transition (Ohde et al., 2009). We also found that LsSd transcript levels were higher in the early pupal stage. Due to the high level of LsSd expression in the early pupal stage, early pupae were chosen for RNAi studies. LsSd knockdown produced adults with curved and wrinkled wings, which is consistent with previous studies on Henosepilachna vigintioctopunctata (Ohde et al., 2009), B. mori (Yin et al., 2020), and L. migratoria (Zhang et al., 2021a). Coordination between cell proliferation and cell death is essential for wing growth and development. It has been reported that the Yki-Sd complex regulates the expression of genes involved in cell growth and proliferation, including the cell cycle regulator gene Cyclin E (CycE) and the cell death inhibitor gene Diap1 (Zhang et al., 2008; Shu and Deng, 2017). Here, we found that LsSd knockdown decreased the expression levels of LsCycE and LsDiap1. Vg is a target gene downstream of both (Decapentaplegic) Dpp and Wingless (Wg) signaling in D. melanogaster, and it plays a crucial role in the formation of the adult wing and halter structures (Fan et al., 2021). During wing development, Vg and Sd form a complex and activate the expression of genes involved in wing morphogenesis (Halder et al., 1998). In this study, knockdown of LsSd significantly decreased LsVg expression. These findings suggest that LsSd participates in the regulation of L. serricorne wing development and plays crucial roles.
Recent evidence suggests that Hippo-signaling pathway is essential for maintaining the homeostasis of the immune system (Liu et al., 2016; Yang et al., 2019). Activation of the Hippo signal inhibits production of the Yki-Sd transcription factor complex in Drosophila, resulting in decreased expression of Cactus, an inhibitor of NF-κB (IκB) in the Toll pathway (Valanne et al., 2011; Liu et al., 2016). Previous studies demonstrated that Yki silencing remarkably downregulated the expression of Cactus (Yang et al., 2019; Huang et al., 2022). Thus, we hypothesized that LsSd may be associated with innate immune in L. serricorne. This study demonstrated that E. coli infections increased LsSd mRNA expression, whereas S. aureus infections downregulated LsSd mRNA expression. To understand how this occurs in L. serricorne, we engineered LsSd-knockdown larvae and studied the mortality of larvae following bacterial infection. LsSd was confirmed as a negative regulator based on the survival analysis because its knockdown decreased susceptibility to S. aureus. The mortality rate of LsSd knockdown larvae was insignificant when infected with E. coli. This indicates that LsSd is linked to the innate immune response against S. aureus. Similarly, Gram-positive bacterial infection rather than Gram-negative bacteria infection acutely activates the Hippo-signaling pathway in D. melanogaster in vivo (Liu et al., 2016). Gram-positive and Gram-negative bacteria can activate the Hippo-signaling pathway in crabs and shrimp (Yang et al., 2019; Huang et al., 2022). In insects, the Toll signaling pathway is predominantly activated by Gram-positive bacteria (Valanne et al., 2011), whereas in crustaceans, the Toll signaling pathway is predominantly activated by both bacterial groups (Sun et al., 2017). In the present study, knockdown of LsSd significantly downregulated the transcription of LsCact in response to S. aureus stimulation, while upregulating the expression of five AMP genes: LsDef1, LsDef2, LsCole, LsLysC, and LsLysI. We further demonstrated that LsSd is a negative regulator in the immune defense of L. serricorne, by promoting the transcription of LsCact and negatively regulating the expression of antibacterial effectors.
In conclusion, we have confirmed that LsSd is involved in multiple biological processes. We confirmed that LsSd is essential for wing development in L. serricorne. Additionally, we discovered that LsSd is required for the innate immune-mediated host defense of L. serricorne larvae against S. aureus. Therefore, LsSd may play an important role in the immune response of L. serricorne. In the future, for L. serricorne control, we can develop novel pest control strategies based on the combination of RNAi of LsSd and microbial control.
Data availability statement
The datasets presented in this study can be found in online repositories. The names of the repository/repositories and accession number(s) can be found below: GenBank, ON533736.
Author contributions
Conceptualization, YZ; data curation, RD, WY, and XZ; investigation, YZ, JY; original draft preparation, YZ, RD, WY, and XZ; writing, review and editing, YZ, RD, and XZ.
Funding
This work was supported by the Program of Excellent Innovation Talents, Guizhou Province, China (Grant number 20206003), the Project of China Tobacco Guizhou Industrial Co., LTD (2017-25), and the Guizhou Province Graduate Research Fund YJSKYJJ [2021]053.
Acknowledgments
We thank all other members of JY and RD’s lab for their laboratory assistance.
Conflict of interest
Author XZ is employed by China Tobacco Guizhou Industrial Co., LTD. The remaining authors declare that the research was conducted in the absence of any commercial or financial relationships that could be construed as a potential conflict of interest.
The authors declare that this study received funding from China Tobacco Guizhou Industrial Co., LTD. The funder had the following involvement with the study: revised the manuscript.
Publisher’s note
All claims expressed in this article are solely those of the authors and do not necessarily represent those of their affiliated organizations, or those of the publisher, the editors and the reviewers. Any product that may be evaluated in this article, or claim that may be made by its manufacturer, is not guaranteed or endorsed by the publisher.
Supplementary material
The Supplementary Material for this article can be found online at: https://www.frontiersin.org/articles/10.3389/fphys.2022.1042897/full#supplementary-material
References
Abdelghany A. Y., Awadalla S. S., Abdel-Baky N. F., El-Syrafi H. A., Fields P. G. (2016). Efficacy of reduced risk insecticides on penetration into jute and polyethylene bags by Lasioderma serricorne (F.) (Coleoptera: Anobiidae). J. Stored Prod. Res. 69, 190–194. doi:10.1016/j.jspr.2016.08.009
Bandura J. L., Edgar B. A. (2008). Yorkie and scalloped: Partners in growth activation. Dev. Cell 14, 315–316. doi:10.1016/j.devcel.2008.02.010
Campbell S. D., Duttaroy A., Katzen A. L., Chovnick A. (1991). Cloning and characterization of the scalloped region of Drosophila melanogaster. Genetics 127, 367–380. doi:10.1093/genetics/127.2.367
Deng H., Hughes S. C., Bell J. B., Simmonds A. J. (2009). Alternative requirements for vestigial, scalloped, and Dmef2 during muscle differentiation in Drosophila melanogaster. Mol. Biol. Cell 20, 256–269. doi:10.1091/mbc.e08-03-0288
Fan W. M., Luo D., Zhang J. Z., Wang D., Shen J. (2021). Vestigial suppresses apoptosis and cell migration in a manner dependent on the level of JNK-Caspase signaling in the Drosophila wing disc. Insect Sci. 28, 63–76. doi:10.1111/1744-7917.12762
Ferguson G. B., Martinez-Agosto J. A. (2017). The TEAD family transcription factor Scalloped regulates blood progenitor maintenance and proliferation in Drosophila through PDGF/VEGFR receptor (Pvr) signaling. Dev. Biol. 425, 21–32. doi:10.1016/j.ydbio.2017.03.016
Garg A., Srivastava A., Davis M. M., O'keefe S. L., Chow L., Bell J. B. (2007). Antagonizing scalloped with a novel vestigial construct reveals an important role for scalloped in Drosophila melanogaster leg, eye and optic lobe development. Genetics 175, 659–669. doi:10.1534/genetics.106.063966
Glise B., Jones D. L., Ingham P. W. (2002). Notch and Wingless modulate the response of cells to Hedgehog signalling in the Drosophila wing. Dev. Biol. 248, 93–106. doi:10.1006/dbio.2002.0720
Halder G., Polaczyk P., Kraus M. E., Hudson A., Kim J., Laughon A., et al. (1998). The Vestigial and Scalloped proteins act together to directly regulate wing-specific gene expression in Drosophila. Genes Dev. 12, 3900–3909. doi:10.1101/gad.12.24.3900
Huang Y., Si Q., Du J., Ren Q. (2022). Yorkie negatively regulates the expression of antimicrobial proteins by inducing Cactus transcription in prawns Macrobrachium nipponense. Front. Immunol. 13, 828271. doi:10.3389/fimmu.2022.828271
Kim S.-I., Park C., Ohh M.-H., Cho H.-C., Ahn Y.-J. (2003). Contact and fumigant activities of aromatic plant extracts and essential oils against Lasioderma serricorne (Coleoptera: Anobiidae). J. Stored Prod. Res. 39, 11–19. doi:10.1016/s0022-474x(02)00013-9
Lin X. D., Xu Y. L., Yao Y., Wang B., Lavine M. D., Lavine L. C. (2016). JNK signaling mediates wing form polymorphism in Brown planthoppers (Nilaparvata lugens). Insect biochem. Mol. Biol. 73, 55–61. doi:10.1016/j.ibmb.2016.04.005
Liu B., Zheng Y. G., Yin F., Yu J. Z., Silverman N., Pan D. J. (2016). Toll receptor-mediated Hippo signaling controls innate immunity in Drosophila. Cell 164, 406–419. doi:10.1016/j.cell.2015.12.029
Livak K. J., Schmittgen T. D. (2001). Analysis of relative gene expression data using real-time quantitative PCR and the 2(-Delta Delta C(T)) method. Methods 25, 402–408. doi:10.1006/meth.2001.1262
Mahroof R. M., Phillips T. W. (2008). Life history parameters of Lasioderma serricorne (F.) as influenced by food sources. J. Stored Prod. Res. 44, 219–226. doi:10.1016/j.jspr.2007.12.001
Misra J. R., Irvine K. D. (2018). The Hippo signaling network and its biological functions. Annu. Rev. Genet. 52, 65–87. doi:10.1146/annurev-genet-120417-031621
Ohde T., Masumoto M., Morita-Miwa M., Matsuura H., Yoshioka H., Yaginuma T., et al. (2009). Vestigial and scalloped in the ladybird beetle: A conserved function in wing development and a novel function in pupal ecdysis. Insect Mol. Biol. 18, 571–581. doi:10.1111/j.1365-2583.2009.00898.x
Pan Y. W., Alegot H., Rauskolb C., Irvine K. D. (2018). The dynamics of Hippo signaling during Drosophila wing development. Development 145, dev165712. doi:10.1242/dev.165712
Riudavets J., Salas I., Pons M. J. (2007). Damage characteristics produced by insect pests in packaging film. J. Stored Prod. Res. 43, 564–570. doi:10.1016/j.jspr.2007.03.006
Rohith B. N., Shyamala B. V. (2017). Scalloped a member of the Hippo tumor suppressor pathway controls mushroom body size in Drosophila brain by non-canonical regulation of neuroblast proliferation. Dev. Biol. 432, 203–214. doi:10.1016/j.ydbio.2017.10.016
Saglam O., Edde P. A., Phillips T. W. (2015). Resistance of Lasioderma serricorne (Coleoptera: Anobiidae) to fumigation with phosphine. J. Econ. Entomol. 108, 2489–2495. doi:10.1093/jee/tov193
Shu Z., Deng W.-M. (2017). Differential regulation of Cyclin E by Yorkie-Scalloped signaling in organ development. G3 (Bethesda) 7, 1049–1060. doi:10.1534/g3.117.039065
Simmonds A. J., Liu X., Soanes K. H., Krause H. M., Irvine K. D., Bell J. B. (1998). Molecular interactions between Vestigial and Scalloped promote wing formation in Drosophila. Genes Dev. 12, 3815–3820. doi:10.1101/gad.12.24.3815
Srivastava A., Mackay J. O., Bell J. B. (2002). A vestigial: Scalloped TEA domain chimera rescues the wing phenotype of a scalloped mutation in Drosophila melanogaster. Genesis 33, 40–47. doi:10.1002/gene.10086
Staley B. K., Irvine K. D. (2012). Hippo signaling in Drosophila: Recent advances and insights. Dev. Dyn. 241, 3–15. doi:10.1002/dvdy.22723
Sun J. J., Xu S., He Z. H., Shi X. Z., Zhao X. F., Wang J. X. (2017). Activation of Toll pathway is different between kuruma shrimp and Drosophila. Front. Immunol. 8, 1151. doi:10.3389/fimmu.2017.01151
Tian W., Yu J. Z., Tomchick D. R., Pan D. J., Luo X. L. (2010). Structural and functional analysis of the YAP-binding domain of human TEAD2. Proc. Natl. Acad. Sci. U. S. A. 107, 7293–7298. doi:10.1073/pnas.1000293107
Valanne S., Wang J. H., Ramet M. (2011). The Drosophila Toll signaling pathway. J. Immunol. 186, 649–656. doi:10.4049/jimmunol.1002302
Vissers J. H. A., Froldi F., Schroder J., Papenfuss A. T., Cheng L. Y., Harvey K. F. (2018). The Scalloped and Nerfin-1 transcription factors cooperate to maintain neuronal cell fate. Cell Rep. 25, 1561–1576. e7. doi:10.1016/j.celrep.2018.10.038
Yan Y., Yang H., Xu K. K., Hu D. M., Yang W. J. (2021). Two lysozymes are involved in the larva-to-pupa transition and the antibacterial immunity of Lasioderma serricorne (Coleoptera: Anobiidae). J. Stored Prod. Res. 90, 101753. doi:10.1016/j.jspr.2020.101753
Yang L., Li X. J., Qin X., Wang Q. Y., Zhou K. M., Li H., et al. (2019). Deleted in azoospermia-associated protein 2 regulates innate immunity by stimulating Hippo signaling in crab. J. Biol. Chem. 294, 14704–14716. doi:10.1074/jbc.RA119.009559
Yang W. J., Xu K. K., Yan Y., Li C., Jin D. C. (2020). Role of chitin deacetylase 1 in the molting and metamorphosis of the cigarette beetle Lasioderma serricorne. Int. J. Mol. Sci. 21, 2449. doi:10.3390/ijms21072449
Ye Z.-F., Zhang P., Gai T.-T., Lou J.-H., Dai F.-Y., Tong X.-L. (2022). Sob gene is critical to wing development in Bombyx mori and Tribolium castaneum. Insect Sci. 29, 65–77. doi:10.1111/1744-7917.12911
Yin J., Zhang J., Li T., Sun X., Qin S., Hou C. X., et al. (2020). BmSd gene regulates the silkworm wing size by affecting the Hippo pathway. Insect Sci. 27, 655–664. doi:10.1111/1744-7917.12702
Zhang L., Ren F. F., Zhang Q., Chen Y. B., Wang B., Jiang J. (2008). The TEAD/TEF family of transcription factor scalloped mediates Hippo signaling in organ size control. Dev. Cell 14, 377–387. doi:10.1016/j.devcel.2008.01.006
Zhang X. H., Liu Z. X., Li M. D., Zhang Z. Y., Wen X. C., Zhang D. C., et al. (2021a). The transcription factor of the Hippo signaling pathway, LmSd, regulates wing development in Locusta migratoria. Int. J. Biol. Macromol. 179, 136–143. doi:10.1016/j.ijbiomac.2021.02.174
Zhang Y., Yang J. P., Dai R. H., Yan Y., Yang W. J., Hu D. M. (2021b). Stability evaluation of candidate reference genes for RT-qPCR normalization in Lasioderma serricorne (F.). J. Stored Prod. Res. 94, 101877. doi:10.1016/j.jspr.2021.101877
Zhao B., Tumaneng K., Guan K. L. (2011). The Hippo pathway in organ size control, tissue regeneration and stem cell self-renewal. Nat. Cell Biol. 13, 877–883. doi:10.1038/ncb2303
Keywords: Lasioderma serricorne, LsSd gene, Hippo-signaling pathway, wing development, immune response
Citation: Zhang Y, Yang J, Dai R, Yang W and Zhang X (2022) Immune function analysis of LsSd, a transcription factor of the Hippo signaling pathway, in the cigarette beetle Lasioderma serricorne. Front. Physiol. 13:1042897. doi: 10.3389/fphys.2022.1042897
Received: 13 September 2022; Accepted: 27 September 2022;
Published: 11 October 2022.
Edited by:
Muhammad Nadeem Abbas, Southwest University, ChinaReviewed by:
Zhiqiang Lu, Northwest A&F University, ChinaLei Wang, Anhui Agricultural University, China
Copyright © 2022 Zhang, Yang, Dai, Yang and Zhang. This is an open-access article distributed under the terms of the Creative Commons Attribution License (CC BY). The use, distribution or reproduction in other forums is permitted, provided the original author(s) and the copyright owner(s) are credited and that the original publication in this journal is cited, in accordance with accepted academic practice. No use, distribution or reproduction is permitted which does not comply with these terms.
*Correspondence: Renhuai Dai, cmhkYWk2OUAxNjMuY29t