- 1University Rennes, M2S (Laboratoire Mouvement, Sport, Santé), Rennes, France
- 2Institut International des Sciences du Sport (2I2S), Irodouer, France
- 3Division of Education, Faculty of Arts and Sciences, Department of Physical Education, University of Balamand, Tripoli, Lebanon
- 4Department of Physical Education and Sport Sciences, Faculty of Humanities and Social Sciences, University of Kurdistan, Sanandaj, Iran
- 5SRM College of Physiotherapy, SRM Institute of Science and Technology, Chennai, India
- 6Centre for Intelligent Healthcare, Coventry University, Coventry, United Kingdom
- 7Department of Exercise & Sport Science, University of North Carolina, Chapel Hill, NC, United States
- 8Department of Nutrition, University of North Carolina, Chapel Hill, NC, United States
- 9Department of Exercise & Sport Management, Kennesaw State University, Kennesaw, GA, United States
- 10Higher Institute of Sport and Physical Education of Ksar-Said, University of Manouba, Tunis, Tunisia
Background: Osteoporosis causes bone fragility, increasing the risk of fractures. Evidence suggests a strong correlation between obesity and fracture risk. Physical training is known to enhance bone resistance and protect from fracture; however, its osteogenic effect in the presence of obesity remains unknown.
Objective: We sought to evaluate the influence of exercise training on bone health indices in individuals with obesity.
Methods: This systematic literature search was conducted using common electronic databases from inception - December 2019. The following key terms (and synonyms searched for by the MeSH database) were included and combined using the operators “AND,” “OR,” “NOT”: [(“body mass index” OR obesity OR obese OR overweight OR fat mass) AND (“bone mineral density” OR “bone mineral content” OR “peak bone mass” OR “mechanical loading” OR “Osteoporosis” OR “bone geometry” OR “bone resistance”) AND (“exercise training” OR “physical training” OR “strength training,” OR “resistance training” OR “aerobic training” OR “combined training”)].
Results: After screening, 10 studies (889 initial records) were included in the final analysis (8 different countries, 263 participants). Two studies investigated males, six females, and two, both sexes. The training duration was at least eight weeks with 2–3 sessions/week. Physical training displayed a significant trivial impact on the whole body (WB) BMD (0.13 SMD; 95% CI [0.00, 0.26], p = 0.046). Subgroup analyses indicated a significant small increase in the WB BMD (0.27 SMD; 95% CI [0.00, 0.53], p = 0.048) in the endurance training group, a non-significant trivial increase in the WB BMD (0.11 SMD; 95% CI [−0.06, 0.29], p = 0.203) in the resistance group, and a non-significant trivial increase in the WB BMD (0.03 SMD; 95% CI [−0.26, 0.32], p = 0.86) in the combined training group. In addition, a significant small decrease was found in the weight of trained subjects (−0.24 SMD; 95% CI [−0.42, −0.05], p = 0.011).
Conclusion: Physical training has little to no effect on the WB BMD in subjects with overweight/obesity. Currently, insufficient evidence to advocate for any specific type of exercise for enhancing bone health exists for overweight/obese individuals. Investigations examining the impact of varying types of physical exercise on WB BMD of obese individuals are needed.
Introduction
The worldwide incidence of obesity continues to grow and is largely attributable to an imbalance between energy intake and energy expenditure, which leads to an increase in body fat accumulation, which adversely affects health (Gonnelli et al., 2014). For example, the accumulation of adipose tissue is associated with various non-communicable disorders, including cardiovascular disease, type 2 diabetes, and cancers (Cao, 2011). Moreover, recent data has demonstrated a pathophysiological link between adiposity and osteoporosis (Cortet and Roux, 2016), where it is suggested that there is a decreased bone mineral density (BMD) and an increase in the fracture risk among obese subjects (Shapses and Sukumar, 2012; Gonnelli et al., 2014; Cortet and Roux, 2016).
Osteoporosis is a systemic skeletal disease characterized by a low BMD and microarchitectural deterioration of bone tissue, which leads to an increased risk of developing spontaneous and traumatic bone fractures (Health, 2001), and it represents a major contributor to the global burden of disease (Torres-Costoso et al., 2020). Genetic and hormonal factors, poor diet, excess caloric intake, and/or lower physical activity are known to be involved in the development of obesity and osteoporosis (Shapses and Sukumar, 2012; Weaver et al., 2016).
The relationship between adipose and bone tissue is complex; indeed, a greater body-weight is generally considered to be beneficial to bone health due to the positive effect of mechanical loading on bone formation (Cao, 2011). Moreover, a decreased body mass index (BMI) is a risk factor for lower BMD and predicts greater bone loss in older individuals (Nguyen et al., 1998). In contrast, the potentially positive influence of fat accumulation (i.e., increased weight) on bone health remains controversial; indeed, evidence suggests that excessive adiposity may be detrimental to bone health and increases fracture risk (Shapses and Sukumar, 2012). Furthermore, studies have suggested that the associated increases of BMD and weight are not proportional. Researchers have found in individuals with obesity, that composite indices of femoral neck strength and BMD per unit BMI are significantly lower compared to individuals with normal-weight (Laet et al., 2005; El Khoury et al., 2017). Also, several authors have indicated that fat distribution affects the bone differently; while subcutaneous fat is positively associated with bone mass, visceral and marrow fat are negatively correlated with BMD (Gilsanz et al., 2009; Russell et al., 2010).
Obesity putatively affects bone metabolism through several mechanisms. Both osteoblasts and adipocytes are derived from a common mesenchymal stem cell and agents inhibiting osteoblastogenesis and increasing adipogenesis (Cao, 2011). Obesity is also associated with chronic inflammation, and adipocytes secrete proinflammatory cytokines which can promote osteoclast activity and bone resorption (Cao et al., 2005). In addition, it has been demonstrated that obesity and osteoporosis may be associated with increased production of proinflammatory cytokines and elevated oxidative stress (Wellen and Hotamisligil, 2003; Mundy, 2007). The excessive secretion of leptin and/or decreased production of adiponectin by adipocytes in obesity may also either directly affect the bone formation or indirectly affect bone resorption (Elefteriou et al., 2004; Oshima et al., 2005). Finally, a high-fat diet may alternate intestinal calcium absorption resulting in a decrease in calcium availability for bone formation (Nelson et al., 1998).
Due to the increased prevalence of osteoporosis, non-pharmacological treatment and/or preventive strategies are highly sought-after. Epidemiological and clinical trial research confirms the positive impact of regular physical activity on bone and body composition (Slemenda et al., 1991; Bailey et al., 1999; Specker and Binkley, 2003), and physical exercise is capable of maximizing peak bone mass in younger years and minimizing age-related bone loss in individuals with lean (Babatunde and Forsyth, 2013). These effects are dependent, primarily, on the type of exercise; high impact and resistance exercises are the most recommended strategies to increase BMD and thereby increase bone resistance and decrease fracture risk (Kelley et al., 2001; Weaver et al., 2016). The majority of published studies have investigated the influence of this type of training and showed positive, consistent results (Guadalupe-Grau et al., 2009). Conversely, relatively fewer studies have explored in individuals with obesity the impact of aerobic and combined physical activities (PA) on bone parameters, and incumbent findings remain controversial (El Hage et al., 2009; Guadalupe-Grau et al., 2009). PA modulate bone remodeling through mechanical stimuli, which results in improvements in mineralization and bone geometry. In addition, PA can optimize body composition by increasing lean mass and decreasing fat mass (Guadalupe-Grau et al., 2009).
How obesity influences the effect of PA on bone health is, so far, unclear (Menzel et al., 2015), and the generalizability of previous research conducted in subjects with normal-weight to individuals with overweight/obesity is ambiguous. To date, aerobic, resistance, and other types of exercises have been used to explore the osteogenic response in the presence of obesity, although diverse results have been recorded. Therefore, the first aim of this systematic review and meta-analysis was to assess the impact of PA on bone health indices in individuals with obesity and the second to investigate whether the type of PA can modulate this effect.
Methods
Eligibility Criteria
PICOS (Population, Intervention, Comparison, Outcome, and Study design) criteria were used as the inclusion criteria for the current review (see Table 1) (Moher et al., 2015). This systematic review included original studies (randomized or non-randomized) for which the full texts were available and that performed interventions with exercise training, included two or more weeks of follow-up, involved individuals with overweight or obesity (BMI ≥ 25 kg.m−2), included one or both sexes, and specifically evaluated whole-body BMD, before and after the intervention, in individuals with obesity.
Studies were excluded if they (1) did not meet the minimum requirements of an experimental study design (e.g., case reports), (2) did not meet the minimum requirements regarding training design (e.g., lack of information on volume, frequency, training methodology), (3) were not written in English or French, (4) involved individuals were not overweight/obese, (5) did not include the measurement of whole body bone mineral density (WB BMD) or (6) did not include sufficient information that allows the determination of effect size. Additionally, the following exclusion criteria were adopted to reduce confounding factors: duplicate publications or sub-studies of included studies, studies involving pathologies, and studies associating exercise with a nutritional intervention (e.g., nutrition counseling, balanced or hypocaloric diets, and supplements) or pharmacological drugs. Moreover, review articles were not included in the current systematic review.
Literature Search Strategy
This systematic review and meta-analysis is reported in accordance with the Preferred Reporting Items for Systematic Reviews and Meta-Analyses (PRISMA) statement and the Cochrane Handbook for Systematic Reviews of Interventions (Higgins and Deeks, 2011).
We searched the following electronic databases (up to December 2019) without a period limit: Cochrane Library, PubMed, Science Direct, Scopus, SPORTDiscus, and Web of Science. Additionally, a manual search for published studies in Google Scholar was conducted for the gray literature analysis. The following key terms (and synonyms searched for by the MeSH database) were included and combined using the operators “AND,” “OR,” “NOT”: [(“body mass index” OR obesity OR obese OR overweight OR fat mass) AND (“bone mineral density” OR “bone mineral content” OR “peak bone mass” OR “mechanical loading” OR “Osteoporosis” OR “bone geometry” OR “bone resistance”) AND (“exercise training” OR “physical training” OR OR “strength training,” OR “resistance training” OR “aerobic training” OR “combined training”)]. Only eligible full texts in English or French were considered for analysis.
Three investigators among the authors (AB, KS, and REH) independently performed searches in the electronic databases, and disagreements were solved by consensus.
Study Selection
Inclusion or exclusion of articles was decided with the application of the PICOS criteria (see Table 1) to the title, abstract, and/or full text of articles. The data-collection process is presented in Figure 1 (Liberati et al., 2009). The titles and abstracts of the selected articles were independently assessed by two researchers (AB and AJ). The reviewers were not blinded to the authors, institutions, or journals associated with the manuscripts. Abstracts that provided insufficient information about the inclusion and exclusion criteria were retrieved for full-text analysis. Furthermore, the researchers independently analyzed the full text and determined the eligibility of the studies, and disagreements were resolved by consensus. To avoid double-counting participants or to clarify questions about the methods, the corresponding authors were contacted if needed. Furthermore, when necessary, the corresponding author was also contacted via e-mail to provide data not included in the published research. Two researchers (AB and KS) independently performed the data extraction, and disagreements were resolved by consensus.
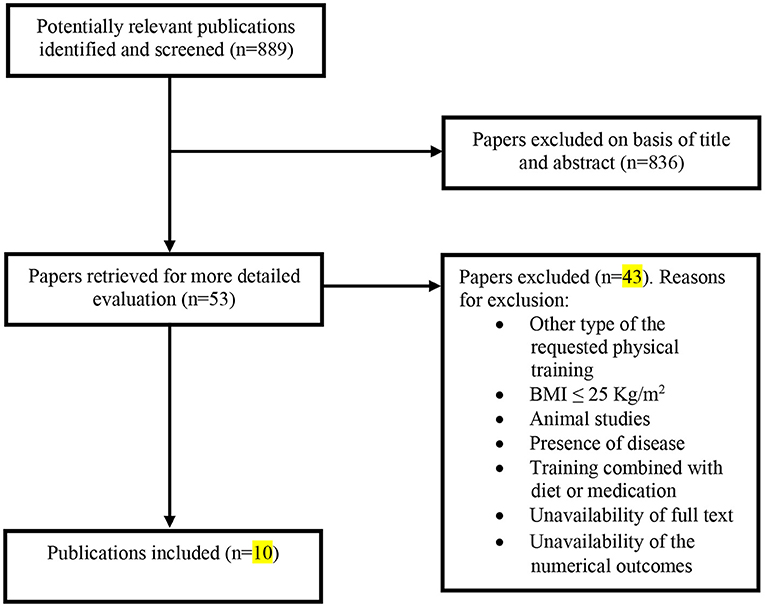
Figure 1. Flow diagram for the selection of studies. Selection process for research articles (n = 10) included in this systematic review. Adapted version of the recommendations in the PRISMA (Preferred Reporting Items for Systematic Reviews and Meta-Analyses) statement (Moher et al., 2010).
Quality Assessment
Study quality was assessed using the Physiotherapy Evidence Database (PEDro) scale (http://www.pedro.fhs.usyd.edu.au), which has been shown to have good reliability and validity (Maher et al., 2003). The PEDro scale has 11 possible points that examine external validity (criterion 1) and internal validity (criteria 2–9) of controlled trials and whether there is sufficient statistical information for interpreting results (criteria 10–11). The items of the scale are: (i) eligibility criteria were specified; (ii) subjects were randomly allocated to groups; (iii) allocation was concealed; (iv) groups were similar at baseline; (v) subjects were blinded; (vi) therapists who administered the treatment were blinded; (vii) assessors were blinded; (viii) measures of key outcomes were obtained from more than 85 % of subjects; (ix) data were analyzed by intention to treat; (x) statistical comparisons between groups were conducted, and (xi) point measures and measures of variability were provided. The first criterion is not included in the final score. Moreover, because of the nature of physical activity interventions, patient and therapy blinding and allocation are difficult; therefore, the total score a trial could receive was 8 points. A cut-off of 6 on the PEDro scale was used to indicate high-quality studies, as this has been reported to be sufficient to determine high quality vs. low quality in previous studies (Maher et al., 2003). The studies were evaluated by two experienced investigators (AB and AJ) and in the event of disagreement, a third reviewer (HZ) was invited to review.
Statistics
Effect sizes (ES) were computed to discern the standardized effect of acute and long-term training on the outcome variables (WB BMD (g/cm2) and weight (kg)). Mean group differences in WB BMD and weight between pre-and post-intervention periods were divided by a pooled SD, based on the assumption of a large correlation (r = 0.7) for each group. The standardized mean differences (SMD) were calculated to determine Cohen's d for each study and Hedge's g was used to account for potential bias in small sample sizes.
Given that BMD and weight values are continuous data, the weighted mean difference method was used for combining study effect size estimates. Weighting assigned to each study group comparison in the analysis was in the inverse proportion to the variance.
When data could be pooled, these effect sizes and 95% confidence intervals (95% CI) were calculated using random-effects models (DerSimonian and Laird approach), that account for true variation in effects between studies, as well as random error within a single study. Values for ES were defined as trivial (<0.2), small (0.2–0.6), moderate (0.6–1.2), large (1.2–2.0), and very large >2 (Hopkins et al., 2009). A negative effect size value indicated that the WB BMD or the weight decreased after the intervention, while a positive effect size indicated there was an increase. Cochrane's Q and the I2 index were used to assess heterogeneity. The alpha value for statistical significance for Q was set at p < 0.10, because it tends to suffer from low differential power (Higgins et al., 2003). For I2, values of 25, 50, and 75% were used to indicate low, moderate, and high heterogeneity, respectively (Higgins et al., 2003). Furthermore, Z test analysis was used to examine if the overall ES's were significantly different from zero.
We contacted authors to obtain any missing numerical data needed for analysis. For interventions with 2 or more arms including the same type of training (El Hage et al., 2009), in the meta-analysis, we combined the 2 arms using standard procedures, as outlined in Section 9.3.9 of the Cochrane Handbook (Higgins and Deeks, 2011). Subgroup analysis was used to investigate differences in the magnitude of the exercise-induced osteogenic effect across studies dependent to the type of training (endurance, resistance, and combined training). Potential publication bias was assessed through visual inspection of funnel plots. All statistical analyses were conducted using R (version 4.0.3; The R Foundation for Statistical Computing, Vienna, Austria) (The R Development Core Team; R Core Team, 2020) and the “metafor” package (Viechtbauer, 2010). Unless otherwise stated, p < 0.05 was used to demarcate statistical significance.
Results
Study Selection
Overall, our search yielded 889 records (Figure 1). After the screening of titles, abstracts, and full texts, 10 studies were included in our final analysis, and the characteristics of these studies are shown in Table 2. These studies were performed in 8 different countries (Lebanon, Canada, Brazil, USA, Taiwan, Korea, Spain, and Sweden), where 2 studies investigated only male subjects, 6 studies investigated only females, and 2 studies investigated both sexes. These papers included 15 training groups (9 for females, 5 for men, and 1 for men and females) and involved 263 trained participants in total. One study (Bocalini et al., 2009) included the measurement of body weight only. The training duration was at least 8 weeks and ranged, for the majority of studies, between 12 and 48 weeks. One study reported a training duration of 96 weeks (Warren and Chua, 2008). The training frequency was at least 2–3 sessions per week, and six studies reported compliance to the training, which ranged between 71 and 94%. The included long-term studies (24 > weeks) were classified as ‘high-quality’ studies (mean 8.2 in the PEDro scale score) (Table 3).
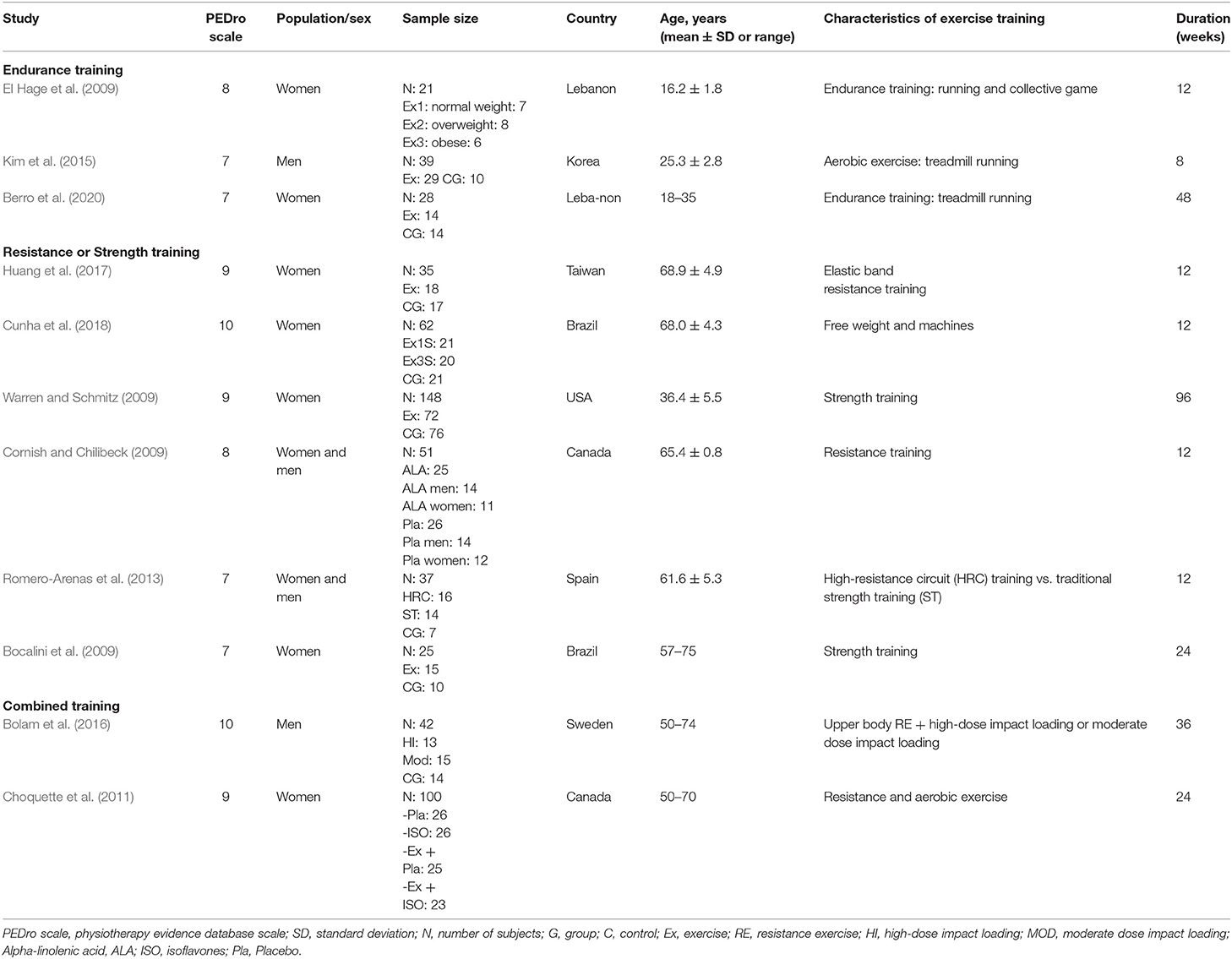
Table 2. Characteristics of the studies that examined the effect of exercise training on bone health indices in individual with obesity.
Table 4 summarizes the studies that examined the effects of exercise training (endurance training, strength and resistance training, and combined training) on bone health indices in individuals with obesity. Nine studies, consisting of 14 trained groups (8 for females, 5 for men, and 1 for men and females) and 240 participants, were included in the meta-analysis investigating the effect of exercise on the WB BMD, as shown in the forest plot (Figure 2). Our analysis indicated a significant trivial increase in the WB BMD (0.13 SMD; 95% CI [0.00, 0.26], p = 0.046). The I2 (0.0%) parameter indicated the homogeneity of the results, whilst the visual inspection of the funnel plot (Figure 3) did not reveal a substantial asymmetry.
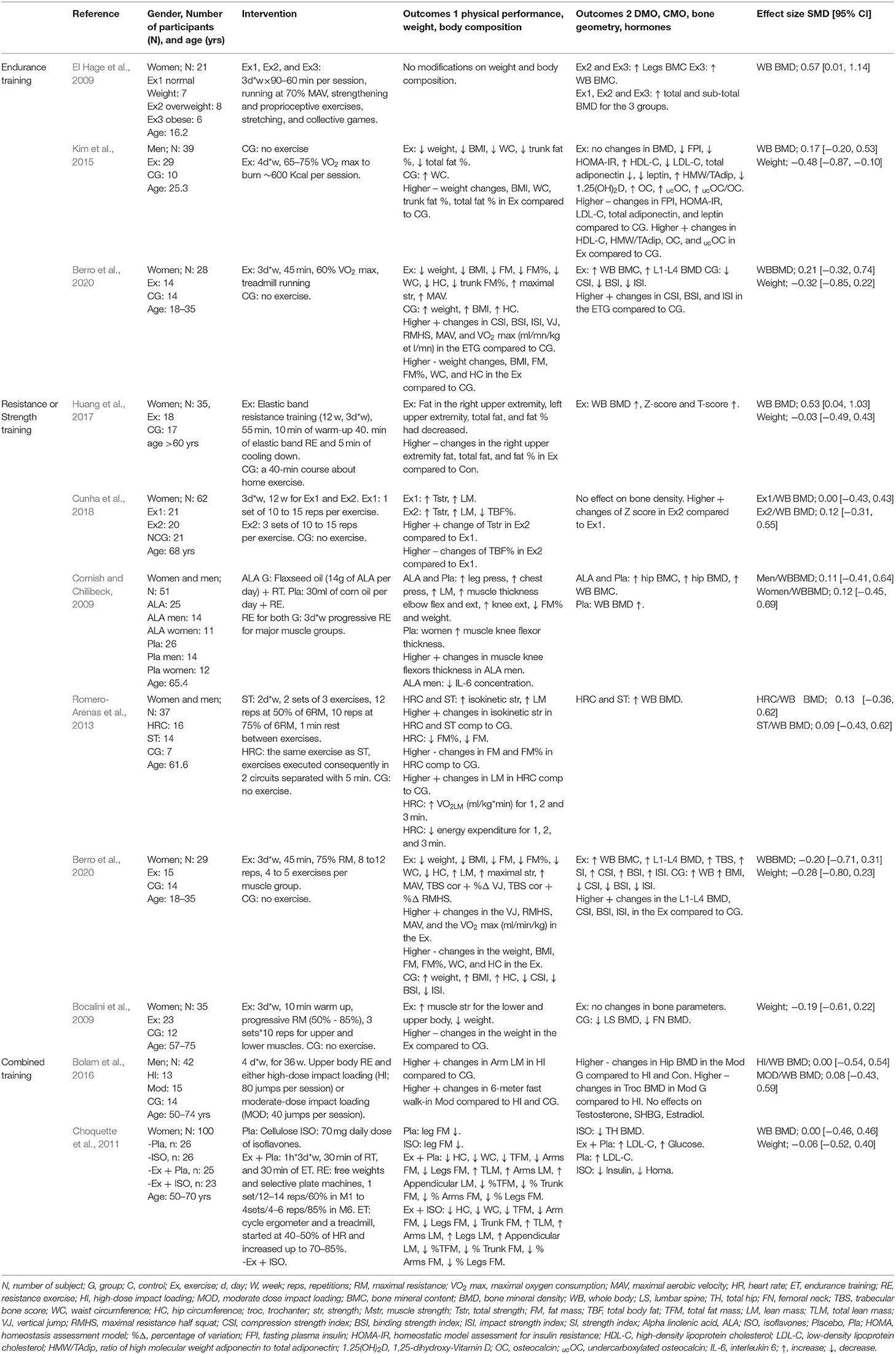
Table 4. Studies examined the effects of exercise training on bone health indices in individual with obesity.
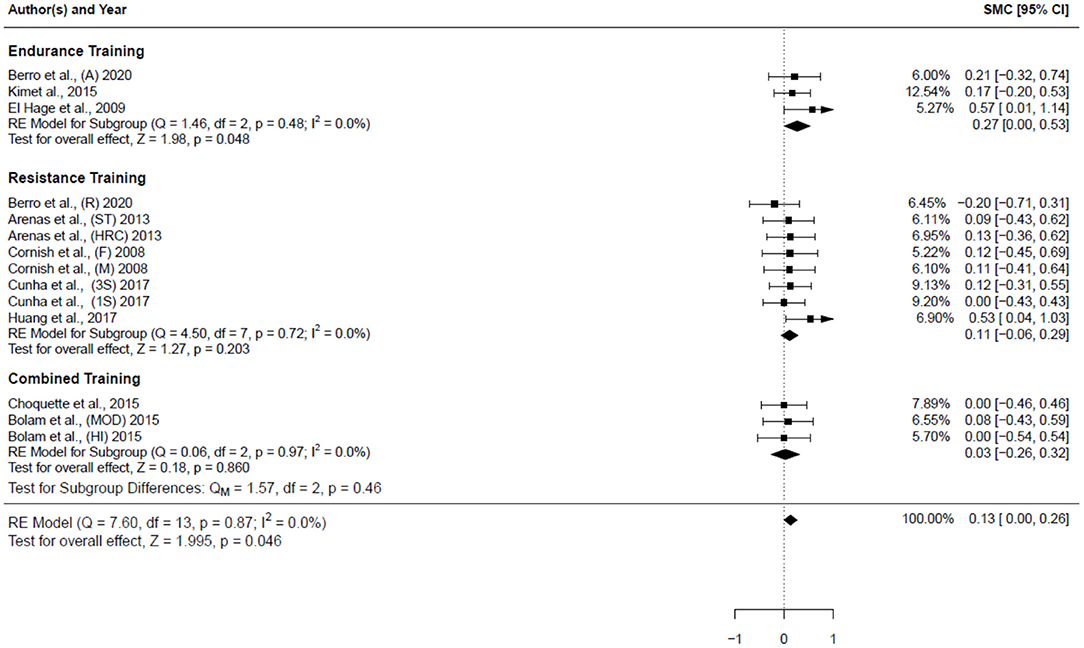
Figure 2. Forest plot for the estimated standardized effect size changes in WB BMD. Three subgroups were included endurance training, resistance training, and combined training. The black squares represent the standardized mean difference, while the left and right extremes of the squares represent the corresponding 95% confidence intervals. The middle of the black diamond represents the overall standardized mean difference, while the left and right extremes of the diamond represent the corresponding 95% confidence intervals. SMC, standardized mean change; A, aerobic; R, resistance; ST, strength training; HRC, Hight resistance circuit; F, females; M, males; 3S, 3 sets; 1S, 1 set; MOD, moderate dose impact loading; HI, high-dose impact loading.
The subgroup of endurance training, as shown in the forest plot (Figure 2), included 3 studies (3 groups: 2 females and 1 man) and 57 participants. A significant small increase in the WB BMD (0.27 SMD; 95% CI [0.00, 0.53], p = 0.048) was detected among these studies, and the I2 (0.0%) parameter indicated homogeneity of the results.
The subgroup of resistance training (Figure 2) included 5 studies (8 groups: 6 females, 1 males, and 1 man and females) and 153 participants. The result showed a non-significant trivial increase in the WB BMD (0.11 SMD; 95% CI [−0.06, 0.29], p = 0.203) was detected among these studies. The I2 (0.0%) parameter indicated homogeneity of the results.
The subgroup of combined training (Figure 2) included 2 studies (3 groups: 1 females, 2 males) and involved 53 participants; it assessed the impact of the combined training on the WB BMD. A non-significant trivial increase in the WB BMD (0.03 SMD; 95% CI [−0.26, 0.32], p = 0.86) was detected among these studies. The I2 (0.0%) parameter indicates the homogeneity of the results. Concerning the impact of the type of physical training on the WB BMD, the comparison between the subgroups (Figure 2) did not reveal any significant differences (p = 0.46).
Five studies (6 groups: 5 females, 1 male), involving 124 participants, investigated the impact of physical activity on weight (Figure 4). The results showed a small significant decrease in the weight of subjects (−0.24 SMD; 95% CI [−0.42, −0.05], p = 0.011). The I2 (0.0%) parameter indicated homogeneity of the results.
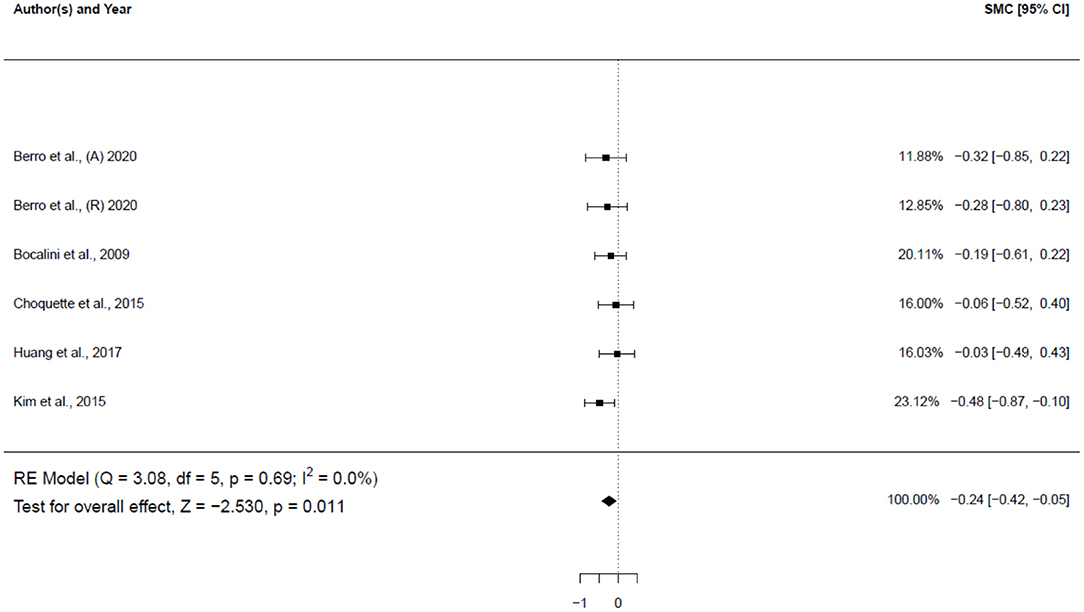
Figure 4. Forest plot for the estimated standardized effect size changes in weight. The black squares represent the standardized mean difference, while the left and right extremes of the squares represent the corresponding 95% confidence intervals. The middle of the black diamond represents the overall standardized mean difference, while the left and right extremes of the diamond represent the corresponding 95% confidence intervals. SMC, standardized mean change A, aerobic; R, resistance.
Discussion
To the best of our knowledge, this is the first meta-analysis to have investigated the influence of the type of physical activity intervention on bone mass in individuals with overweight/obesity. The results show that physical activity is likely to increase the WB BMD in individuals with obesity.
The findings of the present study are supportive of two recent meta-analyses (Ashe et al., 2021; Clemente et al., 2021). Ashe and colleagues (Ashe et al., 2021) suggested that physical activity has little effect on the total hip BMD, and little to no effect on femoral neck BMD, lumbar spine BMD, and WB BMD in males. The other meta-analysis assessed the effects of small-sided games-based training programs on BMD in untrained adults (Clemente et al., 2021) and reported a trivial effect and small effect for this type of training on the WB BMD and lower limbs BMD, respectively when compared to the control groups. The modest osteogenic expression found in the WB BMD, compared to other bone sites, could reflect a site-specific effect for physical exercise. In addition, while the benefits of weight-bearing exercises on bone are well established in the literature (Weaver et al., 2016; Beck et al., 2017), such benefits are generally related to baseline BMD. Accordingly, BMD is, therefore, not expected to largely increase in subjects with overweight, since they already have high BMD values (Qiao et al., 2020).
To further understand the influence of physical exercise on bone, it is important to better comprehend how varying weight statuses may be related to bone health. Accordingly, our results demonstrated a small, but significant, reduction in body weight. Indeed, this outcome extends previous meta-analytic results showing that physical activity promotes weight loss in adolescents with obesity (Stoner et al., 2019). Weight loss is generally associated with bone loss and increased fracture risk in both males and females (Cummings and Nevitt, 1994; Meyer et al., 1995, 1998; Langlois et al., 1996; Lee et al., 2010). Hence, this meta-analysis suggests that the osteogenic effect of the exercise could be tempered by the associated weight loss and that physical activity has the potential to overcome its detrimental effect on BMD among individuals with overweight/obesity. Indeed, these results support the beneficial use of a physical activity to promote weight loss and preserve bone mass in subjects with overweight/obesity.
Effects of Exercise Training on Bone Health Indices in Individuals With Obesity
Effects of Endurance Training
Our analysis showed that endurance training slightly increases the bone density in individuals with obesity. The three studies included (El Hage et al., 2009; Kim et al., 2015; Berro et al., 2020) were of high quality, included both sexes, and the population was restricted to adolescents (El Hage et al., 2009) and adults (Kim et al., 2015; Berro et al., 2020). Two studies used treadmill running as an intervention (Kim et al., 2015; Berro et al., 2020), and the third one used team sports (El Hage et al., 2009). Albeit in the short duration period, the highest increase in the WB BMD was noted among adolescents with obesity who used collective aerobic exercise (El Hage et al., 2009) compared to the treadmill running adult populations (Kim et al., 2015; Berro et al., 2020). It is relatively well established in the literature that the potential of the osteogenic response during adolescence is higher compared to adulthood (Bonjour et al., 2009; Golden and Abrams, 2014). Interestingly, the osteogenic effect of the collective aerobic exercise appeared to be higher than the classic treadmill running. Aerobic exercise is a low-cost and easy to perform physical activity; indeed, these two characteristics are important when compliance is needed to obtain the desired effect. The studies included in this review indicated that weight-bearing aerobic exercise can have a positive impact on bone health among adolescents and adults with obesity.
Effects of Resistance or Strength Training
Our analysis demonstrated that resistance training has little to no effect on the WB BMD. The training programs ranged between 12 to 96 weeks and involved free weights, elastic bands, machines, and circuit training as the interventions for both genders. A previous meta-analysis (Kelley et al., 2001) examined the impact of the resistance exercise on BMD in females (consisting of 29 studies) and found that resistance exercise had a positive effect on BMD at the lumbar spine of all females, and the femur and radius sites for postmenopausal females. However, we did not include these parameters in our analytical model, which precludes any direct comparison.
In our analysis, seven papers had an average participant age of more than 55 years (Bocalini et al., 2009; Cornish and Chilibeck, 2009; Romero-Arenas et al., 2013; Huang et al., 2017; Cunha et al., 2018), and postmenopausal females represented the majority of the subjects. Age is an important factor which influences the responsiveness of bone to exercise. It was previously reported (Berger et al., 2010) that the peak bone mass (PBM) for the trabecular and cortical bone occurs before 40 and 33 years for females and males, respectively. Bone loss begins after PBM and accelerates in females with the onset of menopause (Weaver et al., 2016). Accordingly, physical activity must overcome bone loss before inducing any gain. In addition, as mentioned earlier, obesity is associated with higher BMD (Qiao et al., 2020); thus, the osteogenic response would be expected to be low among subjects with overweight/obesity.
The greatest increase in WB BMD was reported in the study of (Huang et al., 2017) despite the short duration of their training program. One possible explanation for this result is the trivial weight loss observed in this investigation. In fact, this study reported the smallest effect concerning weight loss among all studies. Also, these authors did not control for dietary factors, which can influence the impact of exercise, weight variation, and, consequently, the bone mass (Huang et al., 2017). Resistance training is recognized as an efficacious, non-pharmacological intervention to enhance bone mass (Weaver et al., 2016; Beck et al., 2017). Despite the absence of a significant effect, considering the population age and weight status, this result might suggest that the resistance exercise can combat the bone loss associated with aging in individuals with overweight/obesity.
Effects of Combined Training
This meta-analysis showed that the combined exercise training had little to no effect on the WB BMD. Two studies were included in the analysis; Choquette et al. (2011) and Bolam et al. (2016) investigated the influence of the combined exercise training on WB BMD in males and females with overweight/obesity, with an age range between 50 and 74 years. A previous meta-analysis in postmenopausal females showed that the risk of fractures in the combined exercise groups was significantly lower than in controls. The percentage change of BMD of the spine, trochanter, femoral neck, but not the total hip, was in favor of the intervention group. Our observed effect size may be attributable to the age and the weight status of participants, which can potentially diminish the osteogenic response of the physical exercise. Nevertheless, the present results suggest that the combined training may have the potential to combat bone loss in older adults.
Notably, the subgroups comparison did not reveal any significant differences among the types of training. The highest effect was observed in the endurance training, in which the age of the samples was lower compared to the other subgroups.
Although we have presented a novel addition to the literature, this meta-analysis has some limitations that must be mentioned. The number of studies that investigated the impact of endurance and combined training on bone health in subjects with overweight/obesity was relatively small. Furthermore, the training program's duration was highly variable, ranging from eight (Kim et al., 2015) to ninety-six (Warren and Schmitz, 2009) weeks. To this point, the resorption activity in a basic multicellular unit in adult human bone takes ~3 weeks and the formation response 3 to 4 months (Sims and Martin, 2014), which means that a longer program could induce a higher osteogenic effect and as such be more impactful. Also, age and gender are two well-known moderators of the relation between fat and bone (Dolan et al., 2017). For example, younger individuals tend to exhibit a higher osteogenic effect with physical activity compared to older subjects (Bonjour et al., 2009; Golden and Abrams, 2014). The studies incorporated in this analysis included both sexes, adolescents, adults, and the elderly. Unfortunately, due to the few number of studies we identified in our analysis, sex and age could not be considered as moderators, in addition to the type of physical exercise.
Conclusion
Our systematic review and meta-analysis suggests that physical training have little to no effect on the WB BMD in subjects with overweight obesity. This conclusion, however, is based upon a limited number of available studies. Furthermore, there is also insufficient evidence at this time to advocate a specific type of exercise for enhancing bone health. Additional well-designed randomized controlled trials, investigating the impact of different types of physical exercise on bone health, in both sexes, and among individuals with overweight/obesity, especially during adolescence and adulthood, are needed before any firm recommendations can be made.
Data Availability Statement
The original contributions presented in the study are included in the article/supplementary files, further inquiries can be directed to the corresponding author/s.
Author Contributions
HZ, AJB, and RE contributed to the conception or designof the work. AJB, SK, AJ, and RE independently performed searches in the electronic databases, evaluated articles, and extracted data for the review. All authors confirm responsibility for the following: review conception and design, interpretation reviewed the results, and approved the final version of results, manuscript preparation, writing, editing, the manuscript.
Conflict of Interest
The authors declare that the research was conducted in the absence of any commercial or financial relationships that could be construed as a potential conflict of interest.
Publisher's Note
All claims expressed in this article are solely those of the authors and do not necessarily represent those of their affiliated organizations, or those of the publisher, the editors and the reviewers. Any product that may be evaluated in this article, or claim that may be made by its manufacturer, is not guaranteed or endorsed by the publisher.
Acknowledgments
The authors wish to thank Dr. Sajad Ahmadizad for his comments on this study.
References
Ashe, M. C., Santos, I. K. D., Edward, N. Y., Burnett, L. A., Barnes, R., Fleig, L., et al. (2021). Physical activity and bone health in men: a systematic review and meta-analysis. J. Bone Metab. 28, 27–39. doi: 10.11005/jbm.2021.28.1.27
Babatunde, O. O., and Forsyth, J. J. (2013). Quantitative Ultrasound and bone's response to exercise: a meta-analysis. Plos ONE. 53:11. doi: 10.1016/j.bone.2012.12.011
Bailey, D., McKay, H., Mirwald, R., Crocker, P., and Faulkner, R. (1999). A six-year longitudinal study of the relationship of physical activity to bone mineral accrual in growing children: the university of Saskatchewan bone mineral accrual study. J. Bone Mineral Res. 14, 1672–1679. doi: 10.1359/jbmr.1999.14.10.1672
Beck, B. R., Daly, R. M., Singh, M. A. F., and Taaffe, D. R. (2017). Exercise and Sports Science Australia (ESSA) position statement on exercise prescription for the prevention and management of osteoporosis. J. Sci. Med. sport. 20, 438–445. doi: 10.1016/j.jsams.2016.10.001
Berger, C., Goltzman, D., Langsetmo, L., Joseph, L., Jackson, S., Kreiger, N., et al. (2010). CaMos Research Group. Peak bone mass from longitudinal data: implications for the prevalence, pathophysiology, and diagnosis of osteoporosis. J. Bone Miner. Res. 25, 1948–1957. doi: 10.1002/jbmr.95
Berro, A. J., Kazwini, S. A., Ahmaidi, S. A., and El Hage, R. A. (2020). Effects of 12 months of resistance training vs. endurance training on bone mineral density, hip geometry indices and trabecular bone score in a group of young overweight women. Sci. Sports. 35, 318–319. doi: 10.1016/j.scispo.2020.03.002
Bocalini, D. S., Serra, A. J., dos Santos, L., Murad, N., and Levy, R. F. (2009). Strength training preserves the bone mineral density of postmenopausal women without hormone replacement therapy. J. Aging Health. 21, 519–527. doi: 10.1177/0898264309332839
Bolam, K. A., Skinner, T. L., Jenkins, D. G., Galvão, D. A., and Taaffe, D. R. (2016). The osteogenic effect of impact-loading and resistance exercise on bone mineral density in middle-aged and older men: a pilot study. Gerontology. 62, 22–32. doi: 10.1159/000435837
Bonjour, J. P., Chevalley, T., Ferrari, S., and Rizzoli, R. (2009). The importance and relevance of peak bone mass in the prevalence of osteoporosis. Salud publica de Mexico. 51, s5–s17. doi: 10.1590/S0036-36342009000700004
Cao, J. J. (2011). Effects of obesity on bone metabolism. J. Orthoped. Surg. Res. 6, 1–9. doi: 10.1186/1749-799X-6-30
Cao, J. J., Wronski, T. J., Iwaniec, U., Phleger, L., Kurimoto, P., Boudignon, B., et al. (2005). Aging increases stromal/osteoblastic cell-induced osteoclastogenesis and alters the osteoclast precursor pool in the mouse. J. Bone Miner. Res. 20, 1659–1668. doi: 10.1359/JBMR.050503
Choquette, S., Riesco, É., Cormier, É., Dion, T., Aubertin-Leheudre, M., and Dionne, I. J. (2011). Effects of soya isoflavones and exercise on body composition and clinical risk factors of cardiovascular diseases in overweight postmenopausal women: a 6-month double-blind controlled trial. Br. J. Nutr. 105, 1199–1209. doi: 10.1017/S0007114510004897
Clemente, F. M., Ramirez-Campillo, R., Sarmento, H., Castillo, D., Raya-González, J., Rosemann, T., et al. (2021). Effects of recreational small-sided soccer games on bone mineral density in untrained adults: a systematic review and meta-analysis. Healthcare. 9:457. doi: 10.3390/healthcare9040457
Cornish, S. M., and Chilibeck, P. D. (2009). Alpha-linolenic acid supplementation and resistance training in older adults. App. Physiol. Nutri. Metabol. 34, 49–59. doi: 10.1139/H08-136
Cortet, B., and Roux, C. (2016). Obésité et ostéoporose. Revue du rhumatisme monographies. 83, 25–28. doi: 10.1016/j.monrhu.2015.09.003
Cummings, S. R., and Nevitt, M. (1994). Non-skeletal determinants of fractures: the potential importance of the mechanics of falls. Osteoporosis Int. 4, S67–S70. doi: 10.1007/BF01623439
Cunha, P. M., Ribeiro, A. S., Tomeleri, C. M., Schoenfeld, B. J., Silva, A. M., Souza, M. F., et al. (2018). The effects of resistance training volume on osteosarcopenic obesity in older women. J. Sports Sci. 36, 1564–1571. doi: 10.1080/02640414.2017.1403413
Dolan, E., Swinton, P. A., Sale, C., Healy, A., and O'Reilly, J. (2017). Influence of adipose tissue mass on bone mass in an overweight or obese population: systematic review and meta-analysis. Nutrition reviews. 75, 858–870. doi: 10.1093/nutrit/nux046
El Hage, R., Jacob, C., Moussa, E., Youssef, H., and Jaffré, C. E. (2009). semaines d'entraînement en endurance sur le contenu minéral osseux et la densité minérale osseuse chez des adolescentes obèses, ensurpoids et normales. Sci. Sports. 24, 210–213. doi: 10.1016/j.scispo.2008.09.004
El Khoury, C., Toumi, H., Lespessailles, E., Pinti, A., El Khoury, G., Maalouf, G., et al. (2017). Decreased composite indices of femoral neck strength in young obese men. J. Clin. Densitom. 20, 268–270. doi: 10.1016/j.jocd.2016.03.013
Elefteriou, F., Takeda, S., Ebihara, K., Magre, J., Patano, N., Kim, C. A., et al. (2004). Serum leptin level is a regulator of bone mass. Proc. Natl. Acad. Sci. USA. 101, 3258–63. doi: 10.1073/pnas.0308744101
Gilsanz, V., Chalfant, J., Mo, A. O., Lee, D. C., Dorey, F. J., and Mittelman, S. D. (2009). Reciprocal relations of subcutaneous and visceral fat to bone structure and strength. J. Clinic. Endocrinol. Metabol. 94, 3387–3393. doi: 10.1210/jc.2008-2422
Golden, N. H., and Abrams, S. A. (2014). Optimizing bone health in children and adolescents. Pediatrics. 134, e1229–e43. doi: 10.1542/peds.2014-2173
Gonnelli, S., Caffarelli, C., and Nuti, R. (2014). Obesity and fracture risk. Clinical cases in mineral and bone metabolism: the official journal of the Italian Society of Osteoporosis, Mineral Metabolism, and Skeleta l. Diseases. 11:9. doi: 10.11138/ccmbm/2014.11.1.009
Guadalupe-Grau, A., Fuentes, T., Guerra, B., and Calbet, J. A. (2009). Exercise and bone mass in adults. Sports Med. 39, 439–468. doi: 10.2165/00007256-200939060-00002
Health N. (2001). NIH consensus development panel on osteoporosis prevention, diagnosis, and therapy. South Med. J. 94, 569–73. doi: 10.1097/00007611-200194060-00004
Higgins, J., and Deeks, J. (2011). |Selecting studies and collecting data,” in Cochrane Handbook for Systematic Reviews of Interventions Version 5.1, eds Higgins, J. P. T., Green S. (The Cochrane Collaboration). Available online at: handbook cochrane org. (accessed March 20, 2011).
Higgins, J. P., Thompson, S. G., Deeks, J. J., and Altman, D. G. (2003). Measuring inconsistency in meta-analyses. Bmj. 327, 557–560. doi: 10.1136/bmj.327.7414.557
Hopkins, W., Marshall, S., Batterham, A., and Hanin, J. (2009). Progressive statistics for studies in sports medicine and exercise science. Med. Sci. Sports Exer. 41:3. doi: 10.1249/MSS.0b013e31818cb278
Huang, S. W., Ku, J. W., Lin, L. F., Liao, C. D., Chou, L. C., and Liou, T. H. (2017). Body composition influenced by progressive elastic band resistance exercise of sarcopenic obesity elderly women: a pilot randomized controlled trial. Eur. J. Phys. Rehabil. Med. 53, 556–563. doi: 10.23736/S1973-9087.17.04443-4
Kelley, G. A., Kelley, K. S., and Tran, Z. V. (2001). Resistance Training and Bone Mineral Density in Women: A Meta-analysis of Controlled Trials. London: LWW.
Kim, Y. S., Nam, J. S., Yeo, D. W., Kim, K. R., Suh, S. H., and Ahn, C. W. (2015). The effects of aerobic exercise training on serum osteocalcin, adipocytokines and insulin resistance on obese young males. Clinic. Endocrinol.. 82, 686–694. doi: 10.1111/cen.12601
Laet, D., Kanis, C., Odén, J. A., Johanson, A., Johnell, H., et al. (2005). A. Body mass index as a predictor of fracture risk: a meta-analysis. Osteoporos Int. 16, 1330–1338. doi: 10.1007/s00198-005-1863-y
Langlois, J. A., Harris, T., Looker, A. C., and Madans, J. (1996). Weight change between age 50 years and old age is associated with risk of hip fracture in white women aged 67 years and older. Archiv. Intern. Med. 156, 989–994. doi: 10.1001/archinte.1996.00440090089009
Lee, J. S., Visser, M., Tylavsky, F. A., Kritchevsky, S. B., Schwartz, A. V., Sahyoun, N., et al. (2010). Study. Weight loss and regain and effects on body composition: the health, aging, and body composition study. J. Gerontol. A Biol. Sci. Med. Sci. 65, 78–83. doi: 10.1093/gerona/glp042
Liberati, A., Altman, D. G., Tetzlaff, J., Mulrow, C., Gøtzsche, P. C., and Ioannidis, J. P. (2009). Statement for reporting systematic reviews meta-analyses of studies that evaluate health care interventions: explanation elaboration. PLoS ONE 6:e1000100. doi: 10.1371/journal.pmed.1000100
Maher, C. G., Sherrington, C., Herbert, R. D., Moseley, A. M., and Elkins, M. (2003). Reliability of the PEDro scale for rating quality of randomized controlled trials. Physic. Therapy. 83, 713–721. doi: 10.1093/ptj/83.8.713
Menzel, J., Giuseppe, D. I., Wientzek, R., Kroke, A., Boeing, A., et al. (2015). activity, bone health, and obesity in peri-/pre-and postmenopausal women: results from the EPIC-Potsdam study. Calcified tissue Int. 97, 376–384. doi: 10.1007/s00223-015-0027-0
Meyer, H., Tverdal, A., and Selmer, R. (1998). Weight variability, weight change and the incidence of hip fracture: a prospective study of 39000 middle-aged norwegians. Osteoporosis Int. 8, 373–378. doi: 10.1007/s001980050077
Meyer, H. E., Tverdal, A., and Falch, J. A. (1995). Changes in body weight and incidence of hip fracture among middle-aged Norwegians. BMJ: Br. Med. J. 311:91. doi: 10.1136/bmj.311.6997.91
Moher, D., Liberati, A., Tetzlaff, J., and Altman, D. G. (2010). Preferred reporting items for systematic reviews and meta-analyses: the PRISMA statement. Int. J. Surg. 8, 336–341. doi: 10.1016/j.ijsu.2010.02.007
Moher, D., Shamseer, L., Clarke, M., Ghersi, D., Liberati, A., Petticrew, M., et al. (2015). Preferred reporting items for systematic review and meta-analysis protocols (PRISMA-P) 2015 statement. Syst. Rev. 4:1. doi: 10.1186/2046-4053-4-1
Mundy, G. R. (2007). Osteoporosis and inflammation. Nutr. Rev. 65, S147–S51. doi: 10.1301/nr.2007.dec.S147-S151
Nelson, S. E., Frantz, J. A., and Ziegler, E. E. (1998). Absorption of fat and calcium by infants fed a milk-based formula containing palm olein. J. Am. Coll. Nutr. 17, 327–332. doi: 10.1080/07315724.1998.10718770
Nguyen, T., Sambrook, P., and Eisman, J. (1998). Bone loss, physical activity, and weight change in elderly women: the Dubbo Osteoporosis Epidemiology Study. J. Bone Miner. Res. 13, 1458–1467. doi: 10.1359/jbmr.1998.13.9.1458
Oshima, K., Nampei, A., Matsuda, M., Iwaki, M., Fukuhara, A., Hashimoto, J., et al. (2005). Adiponectin increases bone mass by suppressing osteoclast and activating osteoblast. Biochem. Biophys. Res. Commun. 331, 520–6. doi: 10.1016/j.bbrc.2005.03.210
Qiao, D., Liu, L. i Y, Zhang, X., Qian, X., Zhang, H., et al. (2020). of obesity with bone mineral density and osteoporosis in adults: a systematic review and meta-analysis. Public Health. 180, 22–28. doi: 10.1016/j.puhe.2019.11.001
R Core Team. (2020). R: A Language and Environment for Statistical Computing. Vienna: R Foundation for Statistical Computing. Available online at: https://www.R-project.org/.
Romero-Arenas, S., Blazevich, A. J., Martínez-Pascual, M., Pérez-Gómez, J., Luque, A. J., López-Román, F. J., et al. (2013). Effects of high-resistance circuit training in an elderly population. Exp. Gerontol. 48, 334–340. doi: 10.1016/j.exger.2013.01.007
Russell, M., Mendes, N., Miller, K. K., Rosen, C. J., Lee, H., Klibanski, A., et al. (2010). Visceral fat is a negative predictor of bone density measures in obese adolescent girls. J. Clin. Endocrinol. Metab. 95, 1247–1255. doi: 10.1210/jc.2009-1475
Shapses, S. A., and Sukumar, D. (2012). Bone metabolism in obesity and weight loss. Ann. Rev. Nutr. 32, 287–309. doi: 10.1146/annurev.nutr.012809.104655
Sims, N. A., and Martin, T. J. (2014). Coupling the activities of bone formation and resorption: a multitude of signals within the basic multicellular unit. BoneKEy Rep. 3:12. doi: 10.1038/bonekey.2013.215
Slemenda, C. W., Miller, J. Z., Hui, S. L., Reister, T. K., and Johnston, C. C. (1991). Role of physical activity in the development of skeletal mass in children. J. Bone Miner. Res. 6, 1227–1233. doi: 10.1002/jbmr.5650061113
Specker, B., and Binkley, T. (2003). Randomized trial of physical activity and calcium supplementation on bone mineral content in 3-to 5-year-old children. J. Bone Miner. Res. 18, 885–892. doi: 10.1359/jbmr.2003.18.5.885
Stoner, L., Beets, M. W., Brazendale, K., Moore, J. B., and Weaver, R. G. (2019). Exercise dose and weight loss in adolescents with overweight–obesity: a meta-regression. Sports Med. 49, 83–94. doi: 10.1007/s40279-018-01040-2
Torres-Costoso, A., López-Muñoz, P., Martínez-Vizcaíno, V., Álvarez-Bueno, C., and Cavero-Redondo, I. (2020). Association between muscular strength and bone health from children to young adults: a systematic review and meta-analysis. Sports Med. 50, 1163–1190. doi: 10.1007/s40279-020-01267-y
Viechtbauer, W. (2010). Conducting meta-analyses in R with the metafor package. J. Statistic. Softw. 36, 1–48. doi: 10.18637/jss.v036.i03
Warren, M., and Schmitz, K. H. (2009). Safety of strength training in premenopausal women: musculoskeletal injuries from a two-year randomized trial. Am. J. Health Promot. 23, 309–314. doi: 10.4278/ajhp.07081584
Warren, M. P., and Chua, A. T. (2008). Exercise-induced amenorrhea and bone health in the adolescent athlete. Ann. New York Acad. Sci. 1135, 244–252. doi: 10.1196/annals.1429.025
Weaver, C. M., Gordon, C. M., Janz, K. F., Kalkwarf, H. J., Lappe, J. M., Lewis, R., et al. (2016). The National Osteoporosis Foundation's position statement on peak bone mass development and lifestyle factors: a systematic review and implementation recommendations. Osteoporos Int. 27, 1281–1386. doi: 10.1007/s00198-015-3440-3
Keywords: bone health, exercise, bone mineral density, bone mineral content, resistance exercise and aerobic exercise, combined training
Citation: Zouhal H, Berro AJ, Kazwini S, Saeidi A, Jayavel A, Clark CCT, Hackney AC, VanDusseldorp TA, Ben Abderrahman A and El Hage R (2022) Effects of Exercise Training on Bone Health Parameters in Individuals With Obesity: A Systematic Review and Meta-Analysis. Front. Physiol. 12:807110. doi: 10.3389/fphys.2021.807110
Received: 01 November 2021; Accepted: 20 December 2021;
Published: 14 February 2022.
Edited by:
Giuseppe De Vito, University of Padua, ItalyReviewed by:
Patrik Drid, University of Novi Sad, SerbiaNitita Piya-amornphan, Walailak University, Thailand
Copyright © 2022 Zouhal, Berro, Kazwini, Saeidi, Jayavel, Clark, Hackney, VanDusseldorp, Ben Abderrahman and El Hage. This is an open-access article distributed under the terms of the Creative Commons Attribution License (CC BY). The use, distribution or reproduction in other forums is permitted, provided the original author(s) and the copyright owner(s) are credited and that the original publication in this journal is cited, in accordance with accepted academic practice. No use, distribution or reproduction is permitted which does not comply with these terms.
*Correspondence: Hassane Zouhal, aGFzc2FuZS56b3VoYWxAdW5pdi1yZW5uZXMyLmZy; Trisha A. VanDusseldorp, dHZhbmR1c3NAa2VubmVzYXcuZWR1
†These authors share last authorship