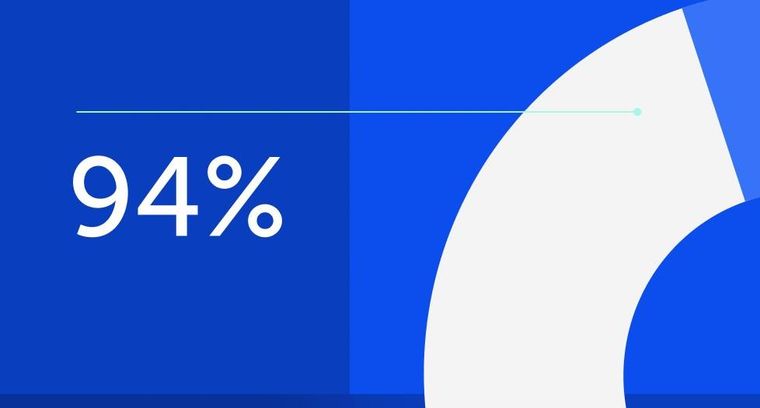
94% of researchers rate our articles as excellent or good
Learn more about the work of our research integrity team to safeguard the quality of each article we publish.
Find out more
REVIEW article
Front. Physiol., 25 January 2022
Sec. Renal Physiology and Pathophysiology
Volume 12 - 2021 | https://doi.org/10.3389/fphys.2021.802132
This article is part of the Research TopicInflammation in Hypertensive DisordersView all 5 articles
A correction has been applied to this article in:
Corrigendum: The Sweet and Salty Dietary Face of Hypertension and Cardiovascular Disease in Lebanon
According to the World Health Organization (WHO), an estimated 1.28 billion adults aged 30–79 years worldwide have hypertension; and every year, hypertension takes 7.6 million lives. High intakes of salt and sugar (mainly fructose from added sugars) have been linked to the etiology of hypertension, and this may be particularly true for countries undergoing the nutrition transition, such as Lebanon. Salt-induced hypertension and fructose-induced hypertension are manifested in different mechanisms, including Inflammation, aldosterone-mineralocorticoid receptor pathway, aldosterone independent mineralocorticoid receptor pathway, renin-angiotensin system (RAS), sympathetic nervous system (SNS) activity, and genetic mechanisms. This review describes the evolution of hypertension and cardiovascular diseases (CVDs) in Lebanon and aims to elucidate potential mechanisms where salt and fructose work together to induce hypertension. These mechanisms increase salt absorption, decrease salt excretion, induce endogenous fructose production, activate fructose-insulin-salt interaction, and trigger oxidative stress, thus leading to hypertension. The review also provides an up-to-date appraisal of current intake levels of salt and fructose in Lebanon and their main food contributors. It identifies ongoing salt and sugar intake reduction strategies in Lebanon while acknowledging the country’s limited scope of regulation and legislation. Finally, the review concludes with proposed public health strategies and suggestions for future research, which can reduce the intake levels of salt and fructose levels and contribute to curbing the CVD epidemic in the country.
Cardiovascular diseases (CVDs) are the leading cause of death globally, accounting for 17.9 million deaths annually (World Health Organization, 2021). The Eastern Mediterranean Region (EMR) is no exception, with CVDs accounting for approximately 50% of overall mortality in the region (Al Jawaldeh et al., 2018). In Lebanon, a Middle Eastern country of the Mediterranean basin, CVDs were estimated to contribute to approximately 47% of mortality in individuals above the age of 50 (World Health Organization, 2019). The major risk factor for CVD morbidity and mortality is hypertension (HTN), a cardiovascular condition that may result from suboptimal diets and food consumption practices. Like other countries of the Middle East, Lebanon is witnessing the nutrition transition, accompanied by its characteristic shifts in diet and lifestyle (Sibai et al., 2010). The hallmark of the nutrition transition is a shift away from the traditional Lebanese dietary pattern, a variant of the Mediterranean diet, toward a more westernized dietary pattern, characterized by increased intakes of energy-dense processed foods that are high in fat, salt, and added sugar. High intakes of salt and added or free sugar have been linked to the etiology of HTN in different cross-sectional and longitudinal studies (Stolarz-Skrzypek, 2011; Genovesi et al., 2021), hence potentially contributing to the increasing burden of CVDs.
The American Heart Association (AHA) has, in fact, been increasingly emphasizing the promotion and support of healthful behavior changes, including dietary practices, for the prevention and treatment of CVDs and their metabolic risk factors (Angell et al., 2020). Dietary strategies that reduce CVDs include adopting diets that are lower in salt and sugar (Sikand and Severson, 2020). The World Health Organization has specifically recommended limiting free sugar intake to less than 10 percent of calories per day (World Health Organization, 2015), with another conditional recommendation further limiting its intake to less than 5% of energy. Sources of free sugar mainly include table sugar and high-fructose corn syrup (HFCS) and naturally occurring sugar in fruit juices and syrups (Malik and Hu, 2015). Table sugar or sucrose is a disaccharide composed of one molecule of fructose and one glucose molecule, while HFCS contains up to 55% fructose (Malik and Hu, 2015). HFCS is widely used in commercial food like cereals, soft drinks, sports drinks, and others. Although fructose is a monosaccharide naturally present in fruits and honey and plays an essential role in glycolysis and gluconeogenesis (Wolf, 1995), its total consumption has been found to increase as a reflection of its use in processed foods. For instance, fructose intake has increased in the United States by 33% between the mid-1970s and the early 2000s, with the highest consumption observed in young adults between 19 and 22 years old mainly due to increased intake of sweetened beverages (Marriott et al., 2009). These facts raise a public health concern since studies showed that high fructose consumption is associated with insulin resistance, fatty liver disease, obesity, and hypertension (Stanhope et al., 2009; Jalal et al., 2010; Tappy and Lê, 2010). While several observational analyses have disputed the nature and magnitude of the association between sodium and vascular outcomes (Alderman, 2014; Campbell et al., 2014; Mente et al., 2014; O’Donnell et al., 2014), the available evidence supports lowering the population’s sodium consumption as an effective public health strategy (Trieu et al., 2015; Milajerdi et al., 2019). Dietary sodium intake is the sum of small amounts of sodium present in food. Sources of dietary sodium can be “discretionary” (from salt added during the preparation of food in the kitchen or at the table) or “non-discretionary” (from the sodium that is found naturally in food or added during industrial food transformation). The “non-discretionary” sources of sodium are mainly in the form sodium chloride, with ∼0.10 g being in the form of monosodium glutamate (MSG), sodium bicarbonate (baking soda), sodium nitrite, and sodium benzoate. Most developed countries’ diets are high in sodium, with approximately 70% of sodium in the diet is in processed food including breads, salted meats, canned goods, cereals, pastries, and food preparation (fast-food and sit-down restaurants) (Anderson et al., 2010; Centers for Disease Control and Prevention, 2012). For example, 1.5–2 g of salt per 100 g may be found in bread, while the sodium levels in meat and cheese is extremely high (up to 2.5 g/100 g). In a recent systematic review of prospective studies, there was evidence of a direct association between urinary excretion of sodium and the risk of CVD mortality. This association was more significant at sodium intakes exceeding 2.4 g/day (Milajerdi et al., 2019). The United Kingdom’s sodium reduction strategy provides further support with regards to the feasibility and impact of salt/sodium reduction interventions, showing a 15% decrease in the population’s sodium intake between 2003 and 2011 (Sadler et al., 2011), which was accompanied by a significant reduction in the population’s average blood pressure by 3/1.4 mm Hg (He et al., 2014). At the 66th World Health Assembly, which took place in 2013, the WHO Member States committed to the global target of a 30% relative reduction in mean population intake of sodium by 2025, against a 2010 baseline (Target 4) (World Health Organization, 2014). It is also expected that efforts to meet the global target on sodium reduction will contribute toward achieving the Sustainable Development Goals (SDGs), including Target 3.4 of reducing premature NCD-related mortality by at least a third (United Nations Statistics Division, 2016).
In a country where the intake of both sugar and salt increases with a concomitant increase in the burden of hypertension and CVDs, this review aims to describe the evolution of hypertension and CVDs in Lebanon and elucidate the potential mechanisms of fructose-induced hypertension, salt-induced hypertension, and the effect of salt-fructose combinations on blood pressure regulation. The review will also provide an up-to-date appraisal of current intake levels of salt and fructose in Lebanon and their main food contributors. It shall conclude with proposed public health strategies, which can reduce the intake levels of salt and fructose levels and contribute to curbing the CVD epidemic in the country.
Hypertension is a major cause of CVD and its consequences worldwide, yet its prevalence among countries varies. Three-quarters of the world’s hypertensive population lives in low- and middle-income countries, with limited health resources, little awareness of hypertension, and limited access to health treatment (Mills et al., 2020). Lebanon, a small upper-middle-income Arab country, was reported to have a prevalence of hypertension. Based on recent studies in Lebanon, hypertension affects one-third of the population, with another 30% being pre-hypertensive (Matar et al., 2015). More concerning is the reported secular trend, which has shown a threefold increase in the prevalence of hypertension in Lebanon over the last decade (Tohme et al., 2005). Most recently, epidemiological data assessing the prevalence, awareness rate, and control rate of hypertension in Lebanon reported a prevalence of 36.4%, with an awareness rate of 65.4% and a controlled rate of 61% (Noubani et al., 2018). The authors concluded that national public health interventions are urgently needed to combat the rising prevalence of hypertension, accomplish primary prevention, and better control the disease. Since 1995, CVDs have been the leading cause of death; CVDs accounted for 33% of deaths worldwide (Institute for Health Metrics and Evaluation and Global Burden of Disease Collaborative Network, 2017). CVD is the major cause of death and hospitalization reported by the Lebanese Ministry of Public Health, as it is in the rest of the world (Isma’eel et al., 2021). Although hypertension is a risk factor for CVD, in fact, systolic blood pressure (SBP) is more frequent than diastolic blood pressure (DBP) and is more closely linked to cardiovascular problems (Lloyd-Jones et al., 2000). A study reported that 24.9% of patients with uncontrolled DBP were associated with CVD, while 43.1% of patients with uncontrolled SBP were associated with CVD (Zeidan et al., 2016).
Compared to other countries globally, the incidence rate of hypertension and CVDs among the Lebanese population falls in the middle range. Being one of the Arab countries, when compared to other regions of the world, such as Sub-Saharan Africa and the United States, the Arab world had a higher crude prevalence of hypertension with 29.5% vs. 28% prevalence in the United States and 27.5% prevalence in Sub-Saharan Africa (Tailakh et al., 2014).
Salt-sensitive hypertension is an essential component of hypertension affecting approximately 50% of hypertensive patients, leading to a threefold increase in the risk of cardiovascular events compared to salt-resistant hypertensive patients. Excess dietary sodium (Na+) is a significant risk factor for hypertension and CVDs. Since table salt is the most prevalent source of dietary sodium, the World Health Organization (WHO) recommends that adults reduce sodium intake to less than 5 g of salt/day (2,000 g of sodium/day). A high salt diet induces hypertension through various mechanisms.
A correlation between salt-sensitive hypertension and renal inflammation has been the center of findings in research. Tubulointerstitial inflammation has been found to impair the excretion of salt from the kidneys. This leads to increased salt sensitivity, which thus can cause hypertension. It was also found that inflammation-suppressing drugs can prevent hypertension from developing (Herrera et al., 2006; Rodriguez-Iturbe and Johnson, 2010). In addition, it has been widely observed that inflammation and lymphocyte (CD3, CD4, CD8) and macrophage (CD-68) accumulation in kidneys and arteries are widespread among hypertensive patients (Franco et al., 2013; Rodríguez-Iturbe et al., 2014). Others found that kidney injury and thus inflammation causes enhanced sodium reabsorption, leading to hypertension in mice (Lombardi et al., 1999).
SGK-1, a downstream mediator of Mineral Corticoid receptors (MRs), activates sodium channels (ENaC) which can lead to salt adaptation and eventually hypertension. Sodium intake upregulates SGK-1, thus activating MRs and ultimately activating ENaCs. In normal individuals, Aldosterone elevates SGK-1 levels and activates ENaCs. However, in hypertensive individuals, aldosterone does not elevate SGK-1 levels and decreases ENaCs expression. Therefore, MRs activation by aldosterone, salt, and Rac1 is key in causing salt-sensitive hypertension (Farjah et al., 2003; Pilic et al., 2016).
MRs have also been observed to be activated by Rac-1 (a small GTPase, member of the Rho-guanine triphosphate hydroxylases family). Salt loading increases Rac1 activity in hypertensive individuals. This activates SGK-1 and MRs, which leads to sodium retention in kidneys, and eventually hypertension. Treatment with Rac-1 inhibitors can decrease blood pressure and salt sensitivity (Shibata et al., 2011). Thus, Rac1 is an upstream regulator of MRs and determines BP salt sensitivity (Hirohama and Fujita, 2019).
The renin-angiotensin-aldosterone system (RAAS) is the main coordinator of blood pressure and water-sodium homeostasis in the body. Normally, the RAAS is suppressed when high salt intake is provided, leading to decreased sodium reabsorption and blood pressure. Low-salt intake significantly increases RAAS components, leading to increased circulating renin and angiotensin II (And II) levels and aldosterone production to stimulate sodium reabsorption (Huan et al., 2012; Fujita, 2014). It has been observed that the RAS is either insufficiently suppressed or overly active in salt-sensitive hypertensive individuals (Fujita, 2014) and experimental rodent models (Kobori et al., 2003; Susic et al., 2011). Several studies have also examined the role of the intrarenal renin-angiotensin system (RAS) and found similar results, as evidenced by increased kidney renin and angiotensin II (Bayorh et al., 2005; Susic et al., 2011). For example, high salt feeding for 4 weeks in spontaneously hypertensive rats, failed to suppress RAS activity (Susic et al., 2011). An important feature of RAAS/RAS overactivation in salt sensitivity is the development of renal disease, both preceding and/or as a consequence of hypertension. RAS inhibition ameliorated salt-induced renal injury, indicating that continuous RAS activity contributes to organ damage in salt-sensitive hypertension (Varagic et al., 2008; Susic et al., 2009, 2011). In that regard, chronic Angiotensin II infusion combined with a high salt diet in rats has been shown to significantly increase renal infiltration of immune cells and ROS activity, demonstrating an important immunological role for RAAS in inducing renal injury (Ozawa et al., 2007; Lara et al., 2012; Itani et al., 2016; Norlander et al., 2017).
The Sympathetic Nervous System (SNS) is one of the key players that modulate renal function. Increased SNS activity has been observed in salt-sensitive hypertensive patients, especially in the kidneys, causing increased renin secretion, reduced renal blood flow, and increased renal tubular reabsorption (DiBona, 2005; Lohmeier et al., 2012). Under normal conditions, With-no-lysine kinase 4 (WNK4) inhibits the thiazide-sensitive sodium chloride cotransporter (NCC), resulting in increased renal sodium excretion. However, stimulation of adrenergic receptors, modeling the SNS, leads to WNK-4 downregulation and thus activation of NCC. This leads to sodium retention and increased blood pressure. This shows that the SNS can cause salt-sensitive hypertension through the WNK4-NCC pathway (Luzardo et al., 2015).
Several gene mutations and variants can be related to salt-sensitive hypertension. It is difficult to name all the genes related to salt-sensitive hypertension due to the various pathways and enzymes involved and the different mutations arising in different populations. However, some of the most studied and prominent genes related to salt-sensitive hypertension have been described. BP changes have been associated with DNA methylation in human genome-wide studies. Methylation levels, especially in promoter regions of genes. Pojoga et al. (2011) reported that mice with heterozygous knockout of lysine-specific demethylase-1 (LSD1, Kdm1a) induce histone H3 lysine 4 (H3K4) or H3K9 demethylation, had salt-sensitive hypertension. These mice were also associated with enhanced vascular contraction despite the suppressed RAAS and reduced relaxation via the NO-cGMP pathway. Therefore, a functional role of LSD1 in the development of salt-sensitive hypertension was deduced (Pojoga et al., 2011). In relation to the RAS, HSD11B2 is a gene encoding the kidney (11-HSD2) isozyme of 11β-hydroxysteroid dehydrogenase, which is associated with reduced activity of the protein resulting in salt sensitivity. The activity of this gene was lower in salt-sensitive individuals in comparison to salt-resistant individuals. The genotype of these people was further studied. Agarwal noticed that polymorphism G1065A exposed that the AA + GA genotypes were more frequent in salt-resistant individuals, suggesting a protective role for the A allele (Agarwal et al., 2000; Caprioli et al., 2008). Another gene, the cytochrome P-450 3A5 gene (CYP3A5), and its variants CYP3A5*1 (expressor) and *3 (reduced-expressor) were found related to salt sensitivity. Individuals with *1 carrier had higher blood pressure than *3/*3, but only those with low salt intake, suggesting that CYP3A5 variants could be implicated in salt sensitivity (Zhang et al., 2010).
The role of volume overload and impaired kidney natriuresis in salt-sensitive pathophysiology has been controversial. Some have argued that a certain degree of kidney dysfunction is necessary for the initiation of salt-sensitive hypertension. Moreover, most genetic alterations detected to date suggest that salt sensitivity is principally dependent on renal sodium handling. Defective natriuresis results in positive sodium and fluid balance, which rapidly causes hypervolemia or extracellular fluid volume expansion. Hypervolemia increases cardiac output in an attempt to improve kidney perfusion pressure and sodium and fluid excretion. This is accompanied by compromised kidney autoregulation, which further contributes to increased blood pressure. Data obtained from salt-sensitive individuals (Campese et al., 1991; Bidani et al., 2009; Burke et al., 2014) and experimental models (Karlsen et al., 1997; Burke et al., 2014; Ren et al., 2014) demonstrated dysfunctional renal vascular autoregulation.
In addition to the above, it has been shown that, in response to a declining kidney function, which is commonly seen with hypertension, RAAS activation increases blood pressure, total peripheral resistance, and kidney perfusion pressure to preserve glomerular filtration rate (GFR). In combination with a net loss of overall GFR, Augmented Ang II levels further amplify Na+ and fluid retention through stimulation of sodium reabsorption at the proximal tubule and the collecting duct (Ku et al., 2019). This effect is intensified in salt-sensitive individuals (Koomans et al., 1982). Importantly, elevated renal perfusion pressure also increases kidney immune cell infiltration, which leads to further kidney damage (Mori et al., 2008).
As mentioned before, the role of salt loading and impaired kidney natriuresis in the initiation of salt-sensitive hypertension is controversial. Many investigators attribute this pathology to dysfunctional regulation of systemic vascular resistance rather than abnormal increases in renal sodium retention or cardiac output (Sullivan et al., 1987; Greene et al., 1990; Khalil, 2006; Schmidlin et al., 2007, 2011). This theory of vaso-dysfunction holds that in response to salt loading, salt-sensitive individuals do not exhibit increases in cardiac output or sodium retention that are larger than those occurring in their salt-resistant counterparts (Sullivan et al., 1987; Greene et al., 1990; Schmidlin et al., 2007, 2011). Indeed, both salt-resistant and salt-sensitive subjects experience increases in cardiac output, but only salt-resistant individuals can offset the hypertensive effect of increased cardiac output by decreasing systemic vascular resistance. This contrasts with salt-sensitive subjects who demonstrate an inadequate decrease in systemic vascular resistance or a paradoxical increase in vascular resistance that increases mean arterial pressure. This is supported by observations in salt-sensitive individuals that the suboptimal decrease in systemic vascular resistance precedes blood pressure increase, indicating that the abnormal vascular resistance response is not a consequence of salt-induced increases in blood pressure (Morris et al., 2016). Importantly, the abnormal resistance response may vary across organs/tissues (Liard, 1981; van Paassen et al., 1996; Schmidlin et al., 1999). It is possible that this phenomenon is more pronounced at the kidney level and can significantly contribute to blood pressure elevation (van Paassen et al., 1996). Currently, it remains unclear which vasodilatory systems are responsible for this abnormality (Morris et al., 2016). Nitric Oxide (NO) is a potent vasodilator proposed to be a key regulator of vascular tone in salt sensitivity (Bech et al., 1998). In this regard, an important model of salt sensitivity that has been widely used includes a low dose of the nitric oxide synthase inhibitor, L-NAME, that does not elevate blood pressure per se, followed by a high salt diet (Itani et al., 2016; Van Beusecum et al., 2019; Wang et al., 2020). This model demonstrated increased blood pressure in response to salt intake due to subnormal vasodilatory responses combined with a normal salt-and salt-induced hypervolemia. Interestingly, controls’ blood volume reductions were associated with elevated urinary sodium excretion, indicating that high blood pressure, sodium retention, and hypervolemia are necessary (Wang et al., 2020).
Rats fed fructose over a long period gain weight and develop abdominal obesity (Tappy, 2018). In addition to that, feeding the rats with HFD increased the body weight of rats, BMI, AC/TC ratio, and adiposity index compared to the normal control group (Pai et al., 2020). Chronic fructose feeding renders animals leptin resistant. This disrupts normal energy homeostasis, favors positive energy storage, and thus predisposes the animal to obesity (Shapiro et al., 2008). High fructose intake has been connected to increased calorie intake and weight gain, and all of these factors are linked to high blood pressure. Epidemiological, animal and human studies correlate high fructose intake with increased blood pressure. In the International Study of Macro/Micronutrients and Blood Pressure in 2011, data collected from nearly 2,700 individuals presented a direct association of the sugar consumption (glucose, fructose, and sucrose) and sugar-sweetened beverages (SSB) with blood pressure increase independently from body weight and height (Brown et al., 2011). The International Study of Macro/Micronutrients and Blood Pressure also reported the direct correlation between sugar intake and blood pressure in a study on 4,680 men and women aged 40–59 years from Japan, China, the United Kingdom, and the United States (Chan et al., 2016). US adults who consumed added sugar more than 74 grams of fructose per day showed an increase in blood pressure independently from any hypertensive history (Jalal et al., 2010) and that US adults who consumed fewer SSBs per day for 18 months showed a significant reduction in their blood pressure (Chen et al., 2010). Animal studies have shown that dogs fed with a diet containing 60% of the calories as fructose for 4 weeks showed a significant increase in blood pressure, while dogs fed with 60% of the calories dextrose remained normotensive (Martinez et al., 1994). Mice fed with a diet in which 98% of the carbohydrates were from fructose for 7 weeks showed an increase in blood pressure (Farah et al., 2007) while rats fed with a high fructose diet showed increased blood pressure within 3–4 weeks (Juan et al., 1998; Hsieh et al., 2005). A high fructose diet induces hypertension through various mechanisms that interact and crosstalk with each other.
Studies showed that high fructose is associated with chronic inflammation. Glushakova et al. (2008) reported that treating human aortic endothelial cells with physiologic concentrations of fructose increases ICAM-1 mRNA and protein expression in a time- and dosage-dependent manner. Furthermore, high fructose increases the systemic secretion of inflammatory cytokines like IL-6 and IL-12 (Porto et al., 2015) and activates the inflammatory signaling in different tissues like the liver, heart, and kidney (Miller and Adeli, 2008). In addition, fructose-induced endothelial dysfunction is associated with significant macrophages and T cells infiltration in perivascular adipose tissue (Jia et al., 2014). It is also reported that TNF-α, IL-1β, and IL-6 are secreted under a high fructose diet, involved in insulin resistance, endothelial dysfunction, and chronic inflammation (Zhang et al., 2017). Further studies are needed to identify the role of immune cells and inflammatory cytokines in fructose-induced hypertension.
Endothelial cells play an important role in regulating vascular tone and thus blood pressure by synthesizing and releasing contracting and relaxing molecules. There is evidence that high-fructose diets impair endothelial activities. To illustrate, nitric oxide (NO) has long been recognized as a potent endothelium-released BP-lowering vasodilator generated by endothelial nitric oxide synthase (eNOS). According to Palanisamy and Venkataraman (2013), the expression of eNOS in fructose-fed rats is reduced to half in comparison to that of control rats, thus inducing hypertension. Alternatively, other studies reported that fructose increases the synthetic rate of vasoconstrictors, including Endothelin-1 (ET-1) and Angiotensin II (Ang II). A research observed increased blood pressure and ET-1 expression after feeding Wistar rats 60% fructose diet for 8 weeks (Abdelrahman et al., 2018). These results were expected since Endothelin-1 is a powerful vasoconstrictor that has been linked to an elevation in blood pressure in fructose-fed rats.
Additionally, the role of Ang II in fructose-induced hypertension has been supported by several studies. According to the researchers, endothelial cells expression of angiotensin type I receptors (AT1) was reported to be up-regulated in fructose-fed rats, and treatment with AT1 receptor antagonists reduces the effects of fructose on blood pressure (Shinozaki et al., 2004). Moreover, it was hypothesized that an interrelationship between ET-1 and Ang II may play a role in fructose-induced hypertension. Interestingly, the hypothesis was validated, and results indicate that Ang II depends on the actions of ET-1 to develop fructose-induced hypertension (Tran et al., 2009). Excess dietary fructose can cause endothelial dysfunction in various ways, according to the pathways discussed above.
Research on the role of renal nerves in long-term control of arterial pressure and hypertension implied that renal sympathetic nerve activity (RSNA) plays a critical role in blood pressure regulation (Osborn and Foss, 2017). The mechanisms of elevated RSNA in hypertension pathophysiology have been previously reported, including enhanced renal tubular sodium reabsorption, decreased urinary sodium excretion, decreased renal blood flow and GFR, and increased RAS activity (DiBona, 2005). In favor of this concept, it has been proposed that sympathetic activity rises in fructose enriched environment. An early study reported that sympathectomy (adrenal medullectomy combined with neurotoxic exposure) prevented hypertension in rats fed a high fructose diet (Verma et al., 1999). In line with other studies, Farah et al. (2006) concluded that fructose activates a sympathetic pathway causing an increase in BP in mice. At the molecular level, in fructose-fed mice, responses to alpha-adrenergic blockades were enhanced, indicating an increase in sympathetic nerve activation (Farah et al., 2006). On this basis, the causative effects of fructose on blood pressure increase appear to be the result of complicated and numerous pathways, and the activation of the RSNS is no exception.
Existing data indicate a correlation between dietary salt and blood pressure increase. However, the exact mechanism of high salt-induced hypertension is not entirely understood. It is known that there is a difference in salt sensitivity between individuals. Excessive salt intake has been associated with hypertension via mechanisms that are poorly understood. Traditionally, sodium was thought to distribute according to a two-compartment model, in which extracellular sodium was thought to equilibrate between the plasma and the interstitium and promote inflammation (Machnik et al., 2010). Genetics plays a crucial role in salt sensitivity, 74% in blacks (Svetkey et al., 1996) and 50% in the Chinese population (Machnik et al., 2009). Epigenetic factors play a role in salt sensitivity, including obesity, aging, and stress. A Cross-sectional study conducted on 1,688 participants (4–18 years) showed that sodium intake is associated with an increase in sugar-sweetened soft drinks consumption. Reducing salt-intake confines high sugar drinks consumption and decrease the risks of obesity and hypertension (He et al., 2008). Even though fructose intake increases blood pressure in rats (Nakagawa et al., 2006) and humans (Perez-Pozo et al., 2010), researchers reported a little increase in blood pressure with no induction of hypertension as a response to fructose intake (D’Angelo et al., 2005). In this context, salt was concluded to have a permissive effect for fructose-induced hypertension, in which the combination of high fructose (HF) and high salt (HS) was required for a hypertensive response which did not develop in rats fed either alone (Cabral et al., 2014; Klein and Kiat, 2015). This questions the relation between dietary fructose and dietary salt in elevating blood pressure.
Research has provided evidence for the role of Glut5 in hypertension pathology. Fructose is absorbed on the apical side of enterocytes in the intestine via the Glut5 transporter. While Glut5+/+ mice enhanced salt absorption in response to a fructose-rich diet for 14 weeks and augmented hypertension, Glut5–/– mice did not exhibit fructose-enhanced salt absorption and developed hypotension and nutrient malabsorption (Barone et al., 2009). Salt absorption in the intestine occurs via the sodium transporter Na+/H+: sodium-hydrogen exchanger 3 (NHE3) and the chloride exchangers Cl–/HCO3–: putative anion transporter 1 (PAT1) located at the apical side in the upper small intestine and the proximal kidney tubule and down-regulated in adenoma (DRA), located at the apical membranes in the mid to lower small intestine and the upper large intestine. Dietary fructose increases mRNA and protein levels of NHE3 and PAT1 in the jejunum. It has been shown in wild-type mice that increased dietary fructose intake increased the expression of PAT1 and Glut5 and increased salt absorption, while PAT1 knock-out mice showed no increase in salt absorption (Singh et al., 2008). Similarly, fructose-induced salt absorption was abolished in NHE3 (+ / +) mice (Soleimani and Alborzi, 2011). Taken together, these studies and others present a critical role of Glut5, NHE3, and PAT1 in fructose-induced salt absorption.
Excess dietary fructose activates the RAS in the kidneys and enhances the proximal tubular sodium reabsorption. For example, a week of fructose (20%) feeding did not change the rat’s blood pressure while adding high salt to diet-induced hypertension. Fructose stimulated NHE3 activity in proximal renal tubules and sensitized the rats to angiotensin II (Ang II) (Cabral et al., 2014). Molecularly, diacylglycerol (DAG), a fructose metabolite, activates protein kinase C (PKC), which in its turn sensitizes the proximal tubules to Ang II. Others have shown that fructose overload upregulates the Na+/K+-ATPase expression in the kidney (Rukavina Mikusic et al., 2018).
Two weeks of fructose (20%) feeding has been shown to upregulate the renal expression of the pro-renin-receptors (PRR) abrogated by allopurinol. Similarly, soluble PRR protein expression increased in response to High Fructose and uric acid (UA) in cultured HK-2 cells in a dose- and time-dependent manner. High Fructose also increased the renal NHE3 and Na+/K/2Cl cotransporter (NKCC2) expression and in vivo NKCC2 activity, which was abolished by a PRR inhibitor PRO20 or allopurinol. High Fructose also leads to activation of the intrarenal RAS system (Xu et al., 2017). This data together supports the role of fructose, via its metabolite uric acid, increases Na+ reuptake by upregulating PRR, which stimulates intrarenal RAS regulates NHE3 and NKCC2 expression. Moreover, fructose promotes sodium absorption by enhancing glucocorticoid activity with an anti-natriuretic effect. Rats drinking 10% fructose solution had increased expression level of 11beta-hydroxysteroid dehydrogenase Type 1 (11βHSD1) and hexose-6-phosphate dehydrogenase (H6PDH) and adipose tissue, together with enhanced GR nuclear accumulation (Bursać et al., 2013).
Several mechanisms have been proposed to explain the pro-hypertensive nature of Insulin, including activation of the sympathetic system (Rowe et al., 1981), growth-promoting activity on vascular smooth muscle cells (Pfeifle and Ditschuneit, 1981), and increased renal sodium reabsorption. Several studies have been performed in rats, dogs, and humans to delineate the anti-natriuretic effect of Insulin. Given that Insulin increases Na+ reabsorption by directly affecting the renal tubules through the epithelial Na+ channel (ENaC) (Zhang et al., 2005), to investigate the fructose-insulin-salt interaction, Sprague-Dawley rats 3 weeks of high fructose diet (66%) with either low (0.07%), normal (0.3%), or high (7.5%) NaCl concentrations. High fructose feeding with normal or high salt increased rat blood pressure, yet not with low salt. A decrease in the insulin receptor and mRNA expression in tissues was observed in response to salt alone as a protective mechanism to prevent excessive Na+ reabsorption in the presence of the high salt diet. Nonetheless, when fructose is introduced into the diet, high salt fails to reduce insulin receptor or mRNA levels in the kidneys, causing an increase in salt reabsorption and decreased urine Na+ concentration (Catena et al., 2003). The regulation of ENaC by Insulin involves the serum and glucocorticoid-inducible kinase (SGK1) through the activation of Phosphatidylinositide-3 (PI3)-kinase (Park et al., 1999) and phosphoinositide-dependent kinase 1 (PDK1) (Biondi et al., 2001). Rats that lack SGK1–/– failed to establish hypertension in response to high salt/high fructose (HS/HF) diet (Huang et al., 2006). On the other hand, α-Lipoic acid improves insulin sensitivity and prevents hypertension in rats fed a high fructose diet for 20 days (Thirunavukkarasu et al., 2004).
High salt diets have been linked to an increased risk of diabetes, leptin resistance, and obesity, regardless of calories (Lanaspa et al., 2018). The relationship between salt and fructose may be more complex; according to recent research, a high-salt diet may stimulate systems in the liver that result in fructose synthesis (endogenous fructose production) (Lanaspa et al., 2018). HS dietary intake activates the liver aldose reductase-fructokinase pathway leading to the production of fructose. In mice, it has been shown that HS intake stimulates Tonicity-responsive enhancer-binding protein (TonEBP or NFAT5), a regulator of cellular adaptation to hypertonicity and inflammation like macrophage activation and T-cell development (Lee et al., 2019). TonEBP activates the aldolase reductase, which induces the conversion to sorbitol, further metabolized to fructose by the sorbitol dehydrogenase. Fructose metabolism leads to ATP depletion, leptin resistance, fatty liver diseases, and metabolic syndrome with hypertension. Another example of the simultaneous role of fructose and salt in developing hypertension was when the fructose metabolism was blocked in fructokinase knockout mice, preventing the effects induced by HS (Lanaspa et al., 2018).
It has been shown that high fructose induces ROS levels, thus contributing to elevated blood pressure. Excessive oxidative stress levels arise with HS/HF diet. Studies have shown that feeding rats a 40% fructose and 3% NaCl-rich diet significantly decreased eNOS in the renal medulla compared to the rats fed a high salt diet alone (Nishimoto et al., 2002). Not only did blood pressure increase significantly in the HF/HS group (20% fructose (supplemented in drinking water); 4% NaCl) compared to the HF and the HS groups, but also the sodium excretion and the Nitric oxide (NO) excretion measured with the levels of NO metabolites (NO2/NO3) were significantly reduced in the HF/HS group (Gordish et al., 2017). Thus, Reduced NO availability contributes to fructose-induced salt-sensitive hypertension. According to Hall et al. (2012) nitric oxide deficiency causes salt-sensitive hypertension via several mechanisms, including improved sensitivity to vasoconstrictors, increased renal tubular sodium reabsorption, increased renal vascular resistance, and amplified renin release. Further investigation is warranted to identify the mechanisms by which salt and fructose promote ROS production.
Several studies have reported high salt intakes in Lebanon (Figure 1). Based on Bayesian hierarchical modeling, Powles et al. (2013), reported a sodium intake estimate of 3.130 g/person/day in Lebanon, and this intake level was estimated to contribute to 210 cardiometabolic deaths per million adults (Afshin et al., 2015). The estimate reported by Powles et al. (2013), is in line with values reported by dietary assessment studies conducted in Lebanon, showing that salt intake among adults is higher than the recommended maximum intake level. For instance, using data stemming from a nationally representative survey conducted in 2008/2009 amongst Lebanese adults (n = 2,543), average sodium intake was estimated at 2.900 g of sodium, with up to 60% of individuals consuming more than the upper limit of 2.000 g/day (Almedawar et al., 2015). Other dietary studies conducted amongst adults have reported even higher estimates ranging between 3.390 g/day (± 1.283) and 4.618 g/day (± 1.898) (Chouccair, 2016). The primary food groups contributing to daily salt intake were identified and included bread and bread-like products (26% of sodium intake), processed meat (12%) and dairy products (9%) (Almedawar et al., 2015). Studies based on 24-h urine collection, which is considered as the gold standard for the assessment of salt intake, reported an average intake of 3.550 g/day (± 1.654) while estimates based on spot urine analysis provided an estimate of 3.357 g/day (± 0.860) (Chouccair, 2016). Although different studies may use different methods of sodium intake assessment, intake levels observed amongst Lebanese adults can be compared to those reported by other countries. Accordingly, sodium intakes in Lebanon were found to be higher than those reported from Austria (2.2 g/day) (Hasenegger et al., 2018) and the UAE (2.71 g/day) (Jarrar et al., 2020), while being lower than estimates reported from Japan (4.16 g/day) (Udagawa et al., 2008), England (3.3 g/day) (Public Health England, 2020), Oman (4.12 g/day) (Ministry of Health-Oman, 2017; Al-Mawali et al., 2021), the US (3.47 g/day) (Clarke et al., 2021), Egypt (3.5 g/day) (Central Agency for Public Mobilization and Statistics et al., 2018), Iran (3.74 g/day) (Ministry of Health and Medical Education et al., 2016; Rezaei et al., 2018), Morocco (4.17 g/day) (The Kingdom of Morocco and World Health Organization, 2018) and China (5.01 g/day) (Fang et al., 2020).
Figure 1. Available data on sodium intake levels in Lebanon. References: For adults: Almedawar et al. (2015) and Chouccair (2016)-Thesis. For children and adolescents: Merhi (2017)-Thesis and Hamamji (2018)-Thesis.
Studies conducted amongst children and adolescents in Lebanon have also reported high sodium intake. In a national study of children aged 6–10 years, the average sodium intake was estimated at 2.894 ± 0.726 g/day based on spot urine analysis (Merhi, 2017); while dietary studies reported values ranging between 2.160 g/day (± 0.800) in 9–13-year-old girls and 2.450 g/day (± 800) in boys (Hamamji, 2018).
Data on sugar intake is scarce in Lebanon. Based on food availability data that are published by FAOSTAT (Food and Agriculture Organization of the United Nations, 2021), sugar supply was found to increase from 70 g (contributing 232 kcal) per person per day in 1961-63 to 127 g (408 kcal) in 2012–2013 (Figure 2). In a study conducted amongst Lebanese adults living in the capital Beirut, dietary fructose intake was estimated at 51.4 g/day, an estimate that is similar to that reported from the US (48.07 ± 35.73 g/day), while exceeding values reported from European countries, such as Germany and Finland, where fructose intake ranged between 18.4 and 40.6 g/day (Montonen et al., 2007; Schulze et al., 2008). This estimate of dietary fructose intake in Lebanon was also found to exceed the suggested upper limit of fructose intake (50 g/day) (Johnson et al., 2009).
Figure 2. Trend in dietary supply of sugar and sweeteners in Lebanon (Food and Agriculture Organization of the United Nations, 2021).
Based on the WHO definition of free sugar (FS), which includes added sugar in addition to sugars naturally present in honey, syrups, fruit juices, and fruit juice concentrate, a recent study estimated free sugar intake amongst Lebanese children and adolescents based on two national surveys. The study showed that the contribution of FS to daily energy intake (EI) increased from 8.5% in under-five children to 11.9% in school-aged children and adolescents (aged 5–18 years) (Jomaa et al., 2021). More than 40% of under-five children were found to exceed the WHO upper limit for FS intake (10% EI), while this proportion increased to 62% in older children and adolescents (Jomaa et al., 2021). The top contributors to FS intake in both age groups (under-five children and 5–18 years old) were SSBs and biscuits and chocolates (Jomaa et al., 2021).
Other available data suggest a high intake of sweetened foods and beverages in Lebanon. Based on a national survey, the consumption of sweets and SSB was reported to be in the range of 18% of energy intake in adults (Nasreddine et al., 2020). In a study that aimed at assessing the contribution of various dietary risk factors to cardiometabolic deaths in the EMR, Afshin et al. (2015) estimated the intake of SSBs at 185 g/day in Lebanon, an intake level that was associated with 59 cardiometabolic deaths per million adults in the country. More alarming is the observed trend in SSB consumption in Lebanon. A recent national study documented an increase in SSB among Lebanese adolescents, from 241.4 g/day in 1997 to 321.7 g/day in 2009 and 301.6 g/day in 2015 (Naja et al., 2021).
Like other countries of the EMR, Lebanon has witnessed a westernization of dietary practices during the past two decades, losing part of its cultural food heritage and dietary idiosyncrasies (Sibai et al., 2010; Nasreddine et al., 2019a). These shifts in diet and food consumption habits have been particularly seen as of the 1990s, after the end of the Lebanese civil war (Nasreddine et al., 2019a), when Lebanon experienced drastic socio-economic and socio-cultural transformations that have led to important changes in the food supply chain and food demand. Examples of such changes include rapid urbanization, technical development in the food industry sector, and the increasing importation of foods. These transformations may partially explain the observed changes in dietary patterns amongst the population, with lower adherence to the traditional Lebanese dietary pattern and a wider embracement of the western dietary patterns, characterized by higher salt and sugar contents (Sibai et al., 2010). These facts underline a public health concern, given that the western dietary pattern has been consistently associated with a higher risk of obesity, metabolic syndrome, and chronic disease.
Efforts to reduce salt and sugar intake are rather timid in Lebanon. National strategies and action plans for salt or sugar intake reduction are lacking (Al-Jawaldeh et al., 2021), and there has been no establishment of national targets for the population intake levels (Al-Jawaldeh et al., 2021). This is of concern given that governmental commitment has been described as an essential prerequisite for nutrition policy development and implementation to facilitate the availability and consumption of safe and nutritious foods that make up a healthy diet, including low salt and low sugar diets (Mozaffarian et al., 2018). Despite the lack of national agendas or strategies for reducing salt, the Lebanon Action for Salt and Health (LASH) initiative was launched in February 2012 as part of the Vascular Medicine Program at the (American University of Beirut Medical Center [AUBMC], 2014). The Lebanese Action for Sodium and Health (LASH) is one of 29 worldwide collaborating countries and members of the World Action of Salt and Health (WASH), a World Health Organization (WHO) initiative to raise salt awareness (American University of Beirut Medical Center [AUBMC], 2014). The main objectives of LASH are to optimize salt intake among the Lebanese population by raising awareness on salt-related health risks, providing professional training, conducting original salt research, connecting with global salt optimization leaders, and advocating for the development of salt reduction policies. LASH has been active in generating data on the population salt intake levels (Almedawar et al., 2015), salt levels in food products (particularly bread as the main staple food in Lebanon) (Food and Agriculture Organization of the United Nations, 2007), and in assessing the consumer’s knowledge, attitude, and behavior (KAB) toward salt (Nasreddine et al., 2014).
The research on KAB has highlighted important gaps in consumers’ knowledge, such as the relationship between salt and health (Nasreddine et al., 2014). In a study conducted amongst Lebanese consumers visiting supermarkets in Lebanon, less than one-quarter of the study population (21.5%) had correctly identified the main contributors of salt in the Lebanese diet, while more than a third overlooked salt content data on the food labels (Nasreddine et al., 2014). These findings will be used to develop culture-specific interventions to raise awareness amongst Lebanese consumers.
On another front, LASH contributes to developing product reformulation policies and establishing a national salt monitoring and evaluation system. LASH has collaborated with the Ministry of Industry and the Ministry of Health in Lebanon to voluntarily develop national salt standards in bread (American University of Beirut Medical Center [AUBMC], 2014; World Health Organization Regional Office for the Eastern Mediterranean, 2015). Legislation on salt reduction should have been proposed to concerned members of Parliament (World Health Organization Global Database on the Implementation of Nutrition Action [GINA], 2014). The scope of work of LASH will also include the establishment of a Lebanese salt-sensitive cohort in normotensive, pre-hypertensive, and hypertensive patients to identify inflammatory markers, genetic, and microbiome changes, improving health literacy within the Lebanese community with regards to high blood pressure and CVD; designing educational courses and flyers focusing on salt reduction, and developing and launching a “salt awareness campaign nationwide in association with the Lebanese Society of Cardiology (LSC) and Vascular Medicine Program.
Efforts on sugar reduction are lacking in Lebanon, and policies focusing on sugar reduction are completely absent. In addition, only a few studies have been conducted to assess sugar intake in the population (Afshin et al., 2015; Nasreddine et al., 2020; Jomaa et al., 2021; Naja et al., 2021), or to investigate the food environment in aspects pertinent to sugar consumption. For instance, acknowledging that exposure to food marketing may influence children’s food preferences and consumption patterns, including their consumption of sugar-sweetened foods and beverages, a recent study has performed a content analysis of food advertisements on local TV channels during children’s viewing time in Lebanon (Nasreddine et al., 2019b). The results showed that approximately 8 out of 10 food advertisements were for products that did not meet the standards specified by the WHO nutrient profile model and which sets specific criteria for foods/beverages that can be marketed to children based on their sugar, salt, and fat content. The study also showed that chocolates and sugar confectionaries and cakes and sweets were the most frequently advertised food categories to children, while savory snacks, cheeses, and sweetened beverages were also among the commonly advertised food categories. These findings suggest that television food advertising during children’s viewing times disproportionately promotes consuming foods high in fat, sugar, and salt, thus promoting unhealthy dietary patterns in children (Nasreddine et al., 2019b). Finally, food-based dietary guidelines (FBDGs) have been developed in Lebanon and include spelled recommendations pertinent to salt and sugar intake reduction (American University of Beirut, 2013). However, wider dissemination of these guidelines is needed to enhance its impact on dietary habits.
There is a dire need to develop and implement population sodium and sugar reduction policies in Lebanon. Securing governmental commitment toward these goals and establishing national salt and sugar reduction targets are needed to develop and implement such policies. More importantly, these policies and interventions ought to be multifaceted in their approach, including (1) product reformulation through engagement with the food industry in order to progressively reduce sodium/salt and sugar in processed food products; (2) implementation of consumer education and awareness campaigns to raise consumer awareness in relation to healthy dietary practices and the need to reduce salt and sugar intake; (3) the establishment and adoption of consumer-friendly food nutrition labeling regulations that include salt and sugar; (4) interventions within specific settings such as schools, workplaces, public institutions (World Health Organization, 2016); (5) implementing fiscal measures to encourage the production and consumption of foods with reduced salt and sugar content and (6) limiting the marketing of foods and beverages that are high in sugar and salt, as per the WHO recommendations (World Health Organization, 2016).
The implementation of multifaceted interventions is expected to be significantly more impactful as compared to fragmented and unidirectional interventions. For instance, the potential impact of 3 policies to decrease dietary salt intake was assessed in some countries of the EMR: a health awareness campaign, the labeling of packaged food, and the mandatory reformulation of processed foods to decrease salt content (Mason et al., 2014). The cumulative population health effects were determined as life-years gained (LYG) over a 10-year time frame. Findings showed that for Palestine, the combination of the three policies mentioned above, which would result in a 30% reduction in salt intake, would lead to an estimated cost savings of $6,000,000 and 2,682 life-years gains. In Syria, the combination of these three policies would result in an estimated cost savings of $39,000,000, and 31,674 life-years gained (Mason et al., 2014), while in Tunisia, this would be yield an estimated cost savings of $235,000,000 and 6,455 life-years gained (Mason et al., 2014). It is also important to highlight that incorporating a legislative component within these strategies and policies would be more impactful than implementing solely voluntary initiatives. Previous systematic reviews and modeling studies have emphasized that mandatory or legislative approaches tend to be more effective, producing larger reductions in the population’s salt or sugar intake levels (Nghiem et al., 2015; Hope et al., 2017; Hyseni et al., 2017). Implementing clear monitoring approaches is also essential to demonstrate program effectiveness and incite greater changes in salt and sugar intakes (Webster et al., 2014).
There is also the need for additional research to support policy development and implementation. Future studies should assess salt intake in various population and age groups, using validated methods such as the 24-h urinary excretion; evaluate sugar intake in various segments of the population using validated dietary approaches, and assess the impact of salt or sugar reduction on the organoleptic properties of culture-specific staple foods such as bread. Research should also examine the acceptability and effectiveness of different front-of-pack labeling schemes in Lebanon to support adopting such schemes in the country. Translational research projects in inflammation-mediated cardiovascular and renal disease are also needed, particularly salt-sensitive hypertension and oxidative stress changes leading to activation of the immune system. Similarly, it is crucial to design patient-targeted assessment tools, including cardiovascular health risk factors and health literacy.
This review highlights how high fructose and salt intake may contribute to the increasing burden of hypertension, a cardiovascular condition threatening population health and development (Figure 3). Salt has multiple effects on the immune system, causing nephritis, sodium reabsorption, and thus hypertension. Other mechanisms by which salt induces hypertension include the activation of MRs, RAS, and SNS. Besides, different genetic mutations and variants have been related to salt-sensitive hypertension. Similarly, fructose may induce intestinal, renal, and endothelial abnormalities and eventually lead to hypertension, insulin resistance, adipogenesis, and non-alcoholic fatty liver disease (NAFLD).
Figure 3. Proposed mechanisms by which salt and fructose consumptions lead to the hypertensive global burden. The high incidence of hypertension imposes a significant public health burden as 1.28 billion adults are hypertensive worldwide. Having hypertension increases the risk of having CVD, stroke, or chronic kidney disease. The mission of the LASH initiative is to optimize salt intake among the Lebanese population by imposing different reduction strategies and policies which aid in reducing the health burden from elevated blood pressure. The upper panel includes different pathways triggered by salt intake, which increases blood pressure. The lower panel includes different pathways triggered by sugar intake, which increases blood pressure. In the middle, the synergistic effect of salt and sugar on elevating blood pressure. WHO recommends 50 g of sugar intake and 5 g of salt intake per day. Rac-1, Rac family small GTPase 1; MR, mineralocorticoid receptor; RAS, renin-angiotensin system; SNS, sympathetic nervous system Glut5, glucose transporter 5; NHE3, sodium/hydrogen exchanger 3; PAT1, putative anion transporter 1; CVD, cardiovascular disease; LASH, Lebanese Action on Salt and Health.
Interestingly, different studies claim a synergistic relation between high salt and high fructose diets in inducing hypertension. For example, fructose has increased intestinal salt absorption by expressing specific transporters and decreasing sodium excretion by activating the RAS. In addition, some studies showed that fructose induces insulin expression, which has an anti-natriuretic and thus exerts a hypertensive effect. Available evidence suggests that the synergistic relation between salt and sugar may be bidirectional. Understanding the co-dependence of salt and fructose in regulating blood pressure may pave the way for new hypertension therapy approaches that focus on the various contributory pathways identified. Even though salt reduction was one of the top 3 priorities to reduce premature mortality from NCD by 25% by 2025 (Beaglehole et al., 2011), salt intake remains high in Lebanon. LASH has been working on raising salt awareness and optimizing salt consumption in Lebanon, yet the efforts on sugar reduction in the country are still completely absent. In an effort to curb the CVD epidemic in the country, there is a dire need for public health strategies aimed at reducing the intake of salt and sugar at the population level.
ML, MI, DM, ZR, LN, and HI contributed to the writing of the manuscript. All authors contributed to the article and approved the submitted version.
This study was supported by the American Society of Nephrology (ASN), Carl W. Gottschalk Research Scholar Award (M0048845), and the American University of Beirut Faculty of Medicine Medical Practice Plan (MPP) to HI.
The authors declare that the research was conducted in the absence of any commercial or financial relationships that could be construed as a potential conflict of interest.
All claims expressed in this article are solely those of the authors and do not necessarily represent those of their affiliated organizations, or those of the publisher, the editors and the reviewers. Any product that may be evaluated in this article, or claim that may be made by its manufacturer, is not guaranteed or endorsed by the publisher.
Abdelrahman, A. M., Al Suleimani, Y. M., Ashique, M., Manoj, P., and Ali, B. H. (2018). Effect of infliximab and tocilizumab on fructose-induced hyperinsulinemia and hypertension in rats. Biomed. Pharmacother. 105, 182–186. doi: 10.1016/j.biopha.2018.05.118
Afshin, A., Micha, R., Khatibzadeh, S., Fahimi, S., Shi, P., Powles, J., et al. (2015). The impact of dietary habits and metabolic risk factors on cardiovascular and diabetes mortality in countries of the Middle East and North Africa in 2010: a comparative risk assessment analysis. BMJ Open 5:e006385.
Agarwal, A. K., Giacchetti, G., Lavery, G., Nikkila, H., Palermo, M., Ricketts, M., et al. (2000). CA-Repeat polymorphism in intron 1 of HSD11B2: effects on gene expression and salt sensitivity. Hypertension 36, 187–194. doi: 10.1161/01.hyp.36.2.187
Al Jawaldeh, A., Rafii, B., and Nasreddine, L. (2018). Salt intake reduction strategies in the eastern Mediterranean Region. East Mediterr. Health J. 24, 1172–1180. doi: 10.26719/emhj.18.006
Al-Jawaldeh, A., Taktouk, M., Chatila, A., Naalbandian, S., Al-Thani, A.-A. M., Alkhalaf, M. M., et al. (2021). Salt reduction initiatives in the Eastern Mediterranean Region and evaluation of progress towards the 2025 global target: a systematic review. Nutrients 13:2676. doi: 10.3390/nu13082676
Al-Mawali, A., Jayapal, S. K., Morsi, M., Al-Shekaili, W., Pinto, A. D., Al-Kharusi, H., et al. (2021). Prevalence of risk factors of non-communicable diseases in the Sultanate of Oman: STEPS survey 2017. PLoS One 16:e0259239. doi: 10.1371/journal.pone.0259239
Almedawar, M. M., Nasreddine, L., Olabi, A., Hamade, H., Awad, E., Toufeili, I., et al. (2015). Sodium intake reduction efforts in Lebanon. Cardiovasc. Diagn. Ther. 5, 178–185. doi: 10.3978/j.issn.2223-3652.2015.04.09
American University of Beirut (2013). The Food-Based Dietary Guideline Manual for Promoting Healthy Eating in the Lebanese Adult Population. Beirut: Faculty of Agricultural and Food Sciences.
American University of Beirut Medical Center [AUBMC] (2014). Lebanese Action on Sodium and Health. Available online at: https://www.aub.edu.lb/fm/vmp/Pages/Lebanese-Action-on-Sodium-and-Health-.aspx (accessed November 27, 2021).
Anderson, C. A., Appel, L. J., Okuda, N., Brown, I. J., Chan, Q., Zhao, L., et al. (2010). Dietary sources of sodium in China, Japan, the United Kingdom, and the United States, women and men aged 40 to 59 years: the INTERMAP study. J. Am. Diet. Assoc. 110, 736–745. doi: 10.1016/j.jada.2010.02.007
Angell, S. Y., McConnell, M. V., Anderson, C. A., Bibbins-Domingo, K., Boyle, D. S., Capewell, S., et al. (2020). The American Heart Association 2030 impact goal: a presidential advisory from the American Heart Association. Circulation 141, e120–e138. doi: 10.1161/CIR.0000000000000758
Barone, S., Fussell, S. L., Singh, A. K., Lucas, F., Xu, J., Kim, C., et al. (2009). Slc2a5 (Glut5) is essential for the absorption of fructose in the intestine and generation of fructose-induced hypertension. J. Biol. Chem. 284, 5056–5066. doi: 10.1074/jbc.M808128200
Bayorh, M. A., Ganafa, A. A., Emmett, N., Socci, R. R., Eatman, D., and Fridie, I. L. (2005). Alterations in aldosterone and angiotensin II levels in salt-induced hypertension. Clin. Exp. Hypertens. 27, 355–367.
Beaglehole, R., Bonita, R., Horton, R., Adams, C., Alleyne, G., Asaria, P., et al. (2011). Priority actions for the non-communicable disease crisis. Lancet 377, 1438–1447.
Bech, J. N., Nielsen, C. B., Ivarsen, P., Jensen, K. T., and Pedersen, E. B. (1998). Dietary sodium affects systemic and renal hemodynamic response to NO inhibition in healthy humans. Am. J. Physiol. 274, F914–F923. doi: 10.1152/ajprenal.1998.274.5.F914
Bidani, A. K., Griffin, K. A., Williamson, G., Wang, X., and Loutzenhiser, R. (2009). Protective importance of the myogenic response in the renal circulation. Hypertension 54, 393–398. doi: 10.1161/HYPERTENSIONAHA.109.133777
Biondi, R. M., Kieloch, A., Currie, R. A., Deak, M., and Alessi, D. R. (2001). The PIF-binding pocket in PDK1 is essential for activation of S6K and SGK, but not PKB. EMBO J. 20, 4380–4390. doi: 10.1093/emboj/20.16.4380
Brown, I. J., Stamler, J., Van Horn, L., Robertson, C. E., Chan, Q., Dyer, A. R., et al. (2011). Sugar-sweetened beverage, sugar intake of individuals, and their blood pressure: international study of macro/micronutrients and blood pressure. Hypertension 57, 695–701. doi: 10.1161/HYPERTENSIONAHA.110.165456
Burke, M., Pabbidi, M. R., Farley, J., and Roman, R. J. (2014). Molecular mechanisms of renal blood flow autoregulation. Curr. Vasc. Pharmacol. 12, 845–858. doi: 10.2174/15701611113116660149
Bursać, B. N., Djordjevic, A. D., Vasiljević, A. D., Milutinović, D. D., Veličković, N. A., Nestorović, N. M., et al. (2013). Fructose consumption enhances glucocorticoid action in rat visceral adipose tissue. J. Nutr. Biochem. 24, 1166–1172. doi: 10.1016/j.jnutbio.2012.09.002
Cabral, P. D., Hong, N. J., Hye Khan, M. A., Ortiz, P. A., Beierwaltes, W. H., Imig, J. D., et al. (2014). Fructose stimulates Na/H exchange activity and sensitizes the proximal tubule to angiotensin II. Hypertension 63, e68–e73. doi: 10.1161/HYPERTENSIONAHA.113.02564
Campbell, N., Lackland, D. T., Niebylski, M. L., and Nilsson, P. M. (2014). Is reducing dietary sodium controversial? Is it the conduct of studies with flawed research methods that is controversial? A perspective from the World Hypertension League Executive Committee. J. Clin. Hypertens. 17, 85–86. doi: 10.1111/jch.12437
Campese, V. M., Parise, M., Karubian, F., and Bigazzi, R. (1991). Abnormal renal hemodynamics in black salt-sensitive patients with hypertension. Hypertension 18, 805–812. doi: 10.1161/01.hyp.18.6.805
Caprioli, J., Mele, C., Mossali, C., Gallizioli, L., Giacchetti, G., Noris, M., et al. (2008). Polymorphisms of EDNRB, ATG, and ACE genes in salt-sensitive hypertension. Can. J. Physiol. Pharmacol. 86, 505–510. doi: 10.1139/Y08-045
Catena, C., Cavarape, A., Novello, M., Giacchetti, G., and Sechi, L. A. (2003). Insulin receptors and renal sodium handling in hypertensive fructose-fed rats. Kidney Int. 64, 2163–2171. doi: 10.1046/j.1523-1755.2003.00313.x
Centers for Disease Control and Prevention (2012). Vital signs: food categories contributing the most to sodium consumption – United States, 2007-2008. MMWR Morb. Mortal Wkly. Rep. 61, 92–98.
Central Agency for Public Mobilization and Statistics, Ministry of Health and Prevention, and World Health Organization (2018). Egypt National STEPwise Survey for Noncommunicable Diseases Risk Factors Report 2017. Available online at: https://www.who.int/ncds/surveillance/steps/Egypt_STEPS_Survey_2017_Fact_Sheet.pdf?ua=1 (accessed November 27, 2021).
Chan, Q., Stamler, J., Griep, L. M., Daviglus, M. L., Horn, L. V., and Elliott, P. (2016). An update on nutrients and blood pressure. J. Atheroscler. Thromb. 23, 276–289. doi: 10.5551/jat.30000
Chen, L., Caballero, B., Mitchell, D. C., Loria, C., Lin, P. H., Champagne, C. M., et al. (2010). Reducing consumption of sugar-sweetened beverages is associated with reduced blood pressure: a prospective study among United States adults. Circulation 121, 2398–2406. doi: 10.1161/CIRCULATIONAHA.109.911164
Chouccair, S. R. (2016). Validation of a Food Frequency Questionnaire and a Spot Urine Sample for the Assessment of Dietary Sodium Intake in Lebanese Adults. Beirut: American University of Beirut.
Clarke, L. S., Overwyk, K., Bates, M., Park, S., Gillespie, C., and Cogswell, M. E. (2021). Temporal trends in dietary sodium intake among adults aged≥ 19 years—United States, 2003–2016. MMWR Morb. Mortal. Wkly. Rep. 70, 1478–1482. doi: 10.15585/mmwr.mm7042a4
D’Angelo, G., Elmarakby, A. A., Pollock, D. M., and Stepp, D. W. (2005). Fructose feeding increases insulin resistance but not blood pressure in Sprague-Dawley rats. Hypertension 46, 806–811. doi: 10.1161/01.HYP.0000182697.39687.34
DiBona, G. F. (2005). Physiology in perspective: the wisdom of the body. Neural control of the kidney. Am. J. Physiol. Regul. Integr. Comp. Physiol. 289, R633–R641. doi: 10.1152/ajpregu.00258.2005
Fang, K., He, Y., Fang, Y., and Lian, Y. (2020). Dietary sodium intake and food sources among Chinese adults: data from the CNNHS 2010-2012. Nutrients 12:453. doi: 10.3390/nu12020453
Farah, V., Elased, K. M., and Morris, M. (2007). Genetic and dietary interactions: role of angiotensin AT1a receptors in response to a high-fructose diet. Am. J. Physiol. Heart Circul. Physiol. 293, H1083–H1089. doi: 10.1152/ajpheart.00106.2006
Farah, V., Elased, K. M., Chen, Y., Key, M. P., Cunha, T. S., Irigoyen, M. C., et al. (2006). Nocturnal hypertension in mice consuming a high fructose diet. Auton. Neurosci. 130, 41–50. doi: 10.1016/j.autneu.2006.05.006
Farjah, M., Roxas, B. P., Geenen, D. L., and Danziger, R. S. (2003). Dietary salt regulates renal SGK1 abundance: relevance to salt sensitivity in the Dahl rat. Hypertension 41, 874–878. doi: 10.1161/01.HYP.0000063885.48344.EA
Food and Agriculture Organization of the United Nations (2021). FAOSTAT Food Balances. Available online at: http://www.fao.org/faostat/en/#data/FBS (accessed November 27, 2021).
Food and Agriculture Organization of the United Nations (2007). Nutrition Country Profile: Lebanese Republic. Nutrition and Consumer Protection Division. Rome: Food and Agriculture Organization.
Franco, M., Tapia, E., Bautista, R., Pacheco, U., Santamaria, J., Quiroz, Y., et al. (2013). Impaired pressure natriuresis resulting in salt-sensitive hypertension is caused by tubulointerstitial immune cell infiltration in the kidney. Am. J. Physiol. Renal Physiol. 304, F982–F990. doi: 10.1152/ajprenal.00463.2012
Fujita, T. (2014). Mechanism of salt-sensitive hypertension: focus on adrenal and sympathetic nervous systems. J. Am. Soc. Nephrol. 25, 1148–1155. doi: 10.1681/ASN.2013121258
Genovesi, S., Giussani, M., Orlando, A., Orgiu, F., and Parati, G. (2021). Salt and sugar: two enemies of healthy blood pressure in Children. Nutrients 13:697. doi: 10.3390/nu13020697
Glushakova, O., Kosugi, T., Roncal, C., Mu, W., Heinig, M., Cirillo, P., et al. (2008). Fructose induces the inflammatory molecule ICAM-1 in endothelial cells. J. Am. Soc. Nephrol. 19, 1712–1720. doi: 10.1681/ASN.2007121304
Gordish, K. L., Kassem, K. M., Ortiz, P. A., and Beierwaltes, W. H. (2017). Moderate (20%) fructose-enriched diet stimulates salt-sensitive hypertension with increased salt retention and decreased renal nitric oxide. Physiol. Rep. 5:e13162. doi: 10.14814/phy2.13162
Greene, A. S., Yu, Z. Y., Roman, R. J., and Cowley, A. W. Jr. (1990). Role of blood volume expansion in Dahl rat model of hypertension. Am. J. Physiol. 258(2 Pt 2), H508–H514. doi: 10.1152/ajpheart.1990.258.2.H508
Hall, J. E., Granger, J. P., do Carmo, J. M., da Silva, A. A., Dubinion, J., George, E., et al. (2012). Hypertension: physiology and pathophysiology. Compr. Physiol. 2, 2393–2442. doi: 10.1002/cphy.c110058
Hamamji, S. E. (2018). Intakes and Sources of Fat, Free Sugars and Salt Among Lebanese Children and Adolescents. Beirut: American University of Beirut.
Hasenegger, V., Rust, P., König, J., Purtscher, A. E., Erler, J., and Ekmekcioglu, C. (2018). Main sources, socio-demographic and anthropometric correlates of salt intake in Austria. Nutrients 10:311. doi: 10.3390/nu10030311
He, F. J., Marrero, N. M., and MacGregor, G. A. (2008). Salt intake is related to soft drink consumption in children and adolescents: a link to obesity? Hypertension 51, 629–634.
He, F. J., Pombo-Rodrigues, S., and MacGregor, G. A. (2014). Salt reduction in England from 2003 to 2011: its relationship to blood pressure, stroke and ischaemic heart disease mortality. BMJ Open 4:e004549. doi: 10.1136/bmjopen-2013-004549
Herrera, J., Ferrebuz, A., MacGregor, E. G., and Rodriguez-Iturbe, B. (2006). Mycophenolate mofetil treatment improves hypertension in patients with psoriasis and rheumatoid arthritis. J. Am. Soc. Nephrol. 17(12 Suppl. 3), S218–S225. doi: 10.1681/ASN.2006080918
Hirohama, D., and Fujita, T. (2019). Evaluation of the pathophysiological mechanisms of salt-sensitive hypertension. Hypertens. Res. 42, 1848–1857. doi: 10.1038/s41440-019-0332-5
Hope, S. F., Webster, J., Trieu, K., Pillay, A., Ieremia, M., Bell, C., et al. (2017). A systematic review of economic evaluations of population-based sodium reduction interventions. PLoS One 12:e0173600. doi: 10.1371/journal.pone.0173600
Hsieh, P. S., Tai, Y. H., Loh, C. H., Shih, K. C., Cheng, W. T., and Chu, C. H. (2005). Functional interaction of AT1 and AT2 receptors in fructose-induced insulin resistance and hypertension in rats. Metab. Clin. Exp. 54, 157–164. doi: 10.1016/j.metabol.2004.07.016
Huan, Y., Deloach, S., Keith, S. W., Goodfriend, T. L., and Falkner, B. (2012). Aldosterone and aldosterone: renin ratio associations with insulin resistance and blood pressure in African Americans. J. Am. Soc. Hypertens. 6, 56–65. doi: 10.1016/j.jash.2011.09.005
Huang, D. Y., Boini, K. M., Friedrich, B., Metzger, M., Just, L., Osswald, H., et al. (2006). Blunted hypertensive effect of combined fructose and high-salt diet in gene-targeted mice lacking functional serum- and glucocorticoid-inducible kinase SGK1. Am. J. Physiol. Regul. Integr. Comp. Physiol. 290, R935–R944. doi: 10.1152/ajpregu.00382.2005
Hyseni, L., Elliot-Green, A., Lloyd-Williams, F., Kypridemos, C., O’Flaherty, M., McGill, R., et al. (2017). Systematic review of dietary salt reduction policies: evidence for an effectiveness hierarchy? PLoS One 12:e0177535. doi: 10.1371/journal.pone.0177535
Institute for Health Metrics and Evaluation, and Global Burden of Disease Collaborative Network (2017). Global Burden of Disease Study 2016 (GBD 2016) Results. Seattle, WA: Institute for Health Metrics and Evaluation.2
Isma’eel, H., El Jamal, N., Al-Chaer, E., Haj-Ali, W., and Hamadeh, G. (2021). Reforming healthcare practice in view of the economic crisis in Lebanon: the case of cardiovascular care. Mediterr. J. Emerg. Med. Acute Care.
Itani, H. A., Xiao, L., Saleh, M. A., Wu, J., Pilkinton, M. A., Dale, B. L., et al. (2016). CD70 exacerbates blood pressure elevation and renal damage in response to repeated hypertensive stimuli. Circ. Res. 118, 1233–1243. doi: 10.1161/CIRCRESAHA.115.308111
Jalal, D. I., Smits, G., Johnson, R. J., and Chonchol, M. (2010). Increased fructose associates with elevated blood pressure. J. Am. Soc. Nephrol. 21, 1543–1549. doi: 10.1681/ASN.2009111111
Jarrar, A. H., Stojanovska, L., Apostolopoulos, V., Cheikh Ismail, L., Feehan, J., Ohuma, E. O., et al. (2020). Assessment of sodium knowledge and urinary sodium excretion among regions of the United Arab Emirates: a cross-sectional study. Nutrients 12:2747. doi: 10.3390/nu12092747
Jia, G., Aroor, A. R., Whaley-Connell, A. T., and Sowers, J. R. (2014). Fructose and uric acid: is there a role in endothelial function? Curr. Hypertens. Rep. 16:434. doi: 10.1007/s11906-014-0434-z
Johnson, R. J., Perez-Pozo, S. E., Sautin, Y. Y., Manitius, J., Sanchez-Lozada, L. G., Feig, D. I., et al. (2009). Hypothesis: could excessive fructose intake and uric acid cause type 2 diabetes? Endocr. Rev. 30, 96–116. doi: 10.1210/er.2008-0033
Jomaa, L., Hamamji, S., Kharroubi, S., Diab-El-Harakeh, M., Chokor, F. A. Z., and Nasreddine, L. (2021). Dietary intakes, sources, and determinants of free sugars amongst Lebanese children and adolescents: findings from two national surveys. Eur. J. Nutr. 60, 2655–2669. doi: 10.1007/s00394-020-02444-5
Juan, C. C., Fang, V. S., Hsu, Y. P., Huang, Y. J., Hsia, D. B., Yu, P. C., et al. (1998). Overexpression of vascular endothelin-1 and endothelin-A receptors in a fructose-induced hypertensive rat model. J. Hypertens. 16(12 Pt 1), 1775–1782. doi: 10.1097/00004872-199816120-00010
Karlsen, F. M., Andersen, C. B., Leyssac, P. P., and Holstein-Rathlou, N. H. (1997). Dynamic autoregulation and renal injury in Dahl rats. Hypertension 30, 975–983. doi: 10.1161/01.hyp.30.4.975
Khalil, R. A. (2006). Dietary salt and hypertension: new molecular targets add more spice. Am. J. Physiol. Regul. Integr. Comp. Physiol. 290, R509–R513. doi: 10.1152/ajpregu.00600.2005
Klein, A. V., and Kiat, H. (2015). The mechanisms underlying fructose-induced hypertension: a review. J. Hypertens. 33, 912–920. doi: 10.1097/HJH.0000000000000551
Kobori, H., Nishiyama, A., Abe, Y., and Navar, L. G. (2003). Enhancement of intrarenal angiotensinogen in Dahl salt-sensitive rats on high salt diet. Hypertension 41, 592–597. doi: 10.1161/01.HYP.0000056768.03657.B4
Koomans, H. A., Roos, J. C., Boer, P., Geyskes, G. G., and Mees, E. J. (1982). Salt sensitivity of blood pressure in chronic renal failure. Evidence for renal control of body fluid distribution in man. Hypertension 4, 190–197. doi: 10.1161/01.hyp.4.2.190
Ku, E., Lee, B. J., Wei, J., and Weir, M. R. (2019). Hypertension in CKD: core curriculum 2019. Am. J. Kidney Dis. 74, 120–131. doi: 10.1053/j.ajkd.2018.12.044
Lanaspa, M. A., Kuwabara, M., Andres-Hernando, A., Li, N., Cicerchi, C., Jensen, T., et al. (2018). High salt intake causes leptin resistance and obesity in mice by stimulating endogenous fructose production and metabolism. Proc. Natl. Acad. Sci. U.S.A. 115, 3138–3143.
Lara, L. S., McCormack, M., Semprum-Prieto, L. C., Shenouda, S., Majid, D. S., Kobori, H., et al. (2012). AT1 receptor-mediated augmentation of angiotensinogen, oxidative stress, and inflammation in ANG II-salt hypertension. Am. J. Physiol. Renal Physiol. 302, F85–F94. doi: 10.1152/ajprenal.00351.2011
Lee, H. H., An, S. M., Ye, B. J., Lee, J. H., Yoo, E. J., Jeong, G. W., et al. (2019). TonEBP/NFAT5 promotes obesity and insulin resistance by epigenetic suppression of white adipose tissue beiging. Nat. Commun. 10:3536. doi: 10.1038/s41467-019-11302-w
Liard, J. F. (1981). Regional blood flows in salt loading hypertension in the dog. Am. J. Physiol. 240, H361–H367. doi: 10.1152/ajpheart.1981.240.3.H361
Lloyd-Jones, D. M., Evans, J. C., Larson, M. G., O’Donnell, C. J., Roccella, E. J., and Levy, D. (2000). Differential control of systolic and diastolic blood pressure: factors associated with lack of blood pressure control in the community. Hypertension 36, 594–599. doi: 10.1161/01.hyp.36.4.594
Lohmeier, T. E., Iliescu, R., Liu, B., Henegar, J. R., Maric-Bilkan, C., and Irwin, E. D. (2012). Systemic and renal-specific sympathoinhibition in obesity hypertension. Hypertension 59, 331–338. doi: 10.1161/HYPERTENSIONAHA.111.185074
Lombardi, D., Gordon, K. L., Polinsky, P., Suga, S., Schwartz, S. M., and Johnson, R. J. (1999). Salt-sensitive hypertension develops after short-term exposure to angiotensin II. Hypertension 33, 1013–1019. doi: 10.1161/01.hyp.33.4.1013
Luzardo, L., Noboa, O., and Boggia, J. (2015). Mechanisms of salt-sensitive hypertension. Curr. Hypertens. Rev. 11, 14–21.
Machnik, A., Dahlmann, A., Kopp, C., Goss, J., Wagner, H., van Rooijen, N., et al. (2010). Mononuclear phagocyte system depletion blocks interstitial tonicity-responsive enhancer binding protein/vascular endothelial growth factor C expression and induces salt-sensitive hypertension in rats. Hypertension 55, 755–761. doi: 10.1161/HYPERTENSIONAHA.109.143339
Machnik, A., Neuhofer, W., Jantsch, J., Dahlmann, A., Tammela, T., Machura, K., et al. (2009). Macrophages regulate salt-dependent volume and blood pressure by a vascular endothelial growth factor-C-dependent buffering mechanism. Nat. Med. 15, 545–552. doi: 10.1038/nm.1960
Malik, V. S., and Hu, F. B. (2015). Fructose and cardiometabolic health: what the evidence from sugar-sweetened beverages tells us. J. Am. Coll. Cardiol. 66, 1615–1624. doi: 10.1016/j.jacc.2015.08.025
Marriott, B. P., Cole, N., and Lee, E. (2009). National estimates of dietary fructose intake increased from 1977 to 2004 in the United States. J. Nutr. 139, 1228S–1235S. doi: 10.3945/jn.108.098277
Martinez, F. J., Rizza, R. A., and Romero, J. C. (1994). High-fructose feeding elicits insulin resistance, hyperinsulinism, and hypertension in normal mongrel dogs. Hypertension 23, 456–463. doi: 10.1161/01.hyp.23.4.456
Mason, H., Shoaibi, A., Ghandour, R., O’Flaherty, M., Capewell, S., Khatib, R., et al. (2014). A cost effectiveness analysis of salt reduction policies to reduce coronary heart disease in four Eastern Mediterranean countries. PLoS One 9:e84445. doi: 10.1371/journal.pone.0084445
Matar, D., Frangieh, A. H., Abouassi, S., Bteich, F., Saleh, A., Salame, E., et al. (2015). Prevalence, awareness, treatment, and control of hypertension in Lebanon. J. Clin. Hypertens. 17, 381–388. doi: 10.1111/jch.12485
Mente, A., O’Donnell, M. J., Rangarajan, S., McQueen, M. J., Poirier, P., Wielgosz, A., et al. (2014). Association of urinary sodium and potassium excretion with blood pressure. N. Engl. J. Med. 371, 601–611.
Merhi, K. A. (2017). Urinary Sodium and Potassium Status of Lebanese School Aged Children. Beirut: American University of Beirut.
Milajerdi, A., Djafarian, K., and Shab-Bidar, S. (2019). Dose–response association of dietary sodium intake with all-cause and cardiovascular mortality: a systematic review and meta-analysis of prospective studies. Public Health Nutr. 22, 295–306. doi: 10.1017/S1368980018002112
Miller, A., and Adeli, K. (2008). Dietary fructose and the metabolic syndrome. Curr. Opin. Gastroenterol. 24, 204–209.
Mills, K. T., Stefanescu, A., and He, J. (2020). The global epidemiology of hypertension. Nat. Rev. Nephrol. 16, 223–237.
Ministry of Health and Medical Education, Tehran University of Medical Sciences, and National Institute of Health Research-Iran (2016). Atlas of Non-Communicable Diseases Risk- Factors Surveillance in the Islamic Republic of Iran–STEPS 2016. Available online at: https://www.who.int/ncds/surveillance/steps/STEPS_2016_Atlas_EN.pdf?ua=1 (accessed November 27, 2021).
Ministry of Health-Oman (2017). National Health Survey of Non-Communicable Diseases Risk Factors; Sultanate of Oman STEPS Survey Report 2017. Seeb: Center of Studies and Research.
Montonen, J., Järvinen, R., Knekt, P., Heliövaara, M., and Reunanen, A. (2007). Consumption of sweetened beverages and intakes of fructose and glucose predict type 2 diabetes occurrence. J. Nutr. 137, 1447–1454. doi: 10.1093/jn/137.6.1447
Mori, T., Polichnowski, A., Glocka, P., Kaldunski, M., Ohsaki, Y., Liang, M., et al. (2008). High perfusion pressure accelerates renal injury in salt-sensitive hypertension. J. Am. Soc. Nephrol. 19, 1472–1482. doi: 10.1681/ASN.2007121271
Morris, R. C. Jr., Schmidlin, O., Sebastian, A., Tanaka, M., and Kurtz, T. W. (2016). Vasodysfunction that involves renal vasodysfunction, not abnormally increased renal retention of sodium, accounts for the initiation of salt-induced hypertension. Circulation 133, 881–893. doi: 10.1161/CIRCULATIONAHA.115.017923
Mozaffarian, D., Angell, S. Y., Lang, T., and Rivera, J. A. (2018). Role of government policy in nutrition—barriers to and opportunities for healthier eating. BMJ 361:k2426. doi: 10.1136/bmj.k2426
Naja, F., Hwalla, N., Hachem, F., Abbas, N., Chokor, F. A. Z., Kharroubi, S., et al. (2021). Erosion of the Mediterranean diet among adolescents: evidence from an Eastern Mediterranean Country. Br. J. Nutr. 125, 346–356. doi: 10.1017/S0007114520002731
Nakagawa, T., Hu, H., Zharikov, S., Tuttle, K. R., Short, R. A., Glushakova, O., et al. (2006). A causal role for uric acid in fructose-induced metabolic syndrome. Am. J. Physiol. Renal Physiol. 290, F625–F631. doi: 10.1152/ajprenal.00140.2005
Nasreddine, L., Akl, C., Al-Shaar, L., Almedawar, M. M., and Isma’eel, H. (2014). Consumer knowledge, attitudes and salt-related behavior in the Middle-East: the case of Lebanon. Nutrients 6, 5079–5102. doi: 10.3390/nu6115079
Nasreddine, L., Ayoub, J. J., Hachem, F., Tabbara, J., Sibai, A. M., Hwalla, N., et al. (2019a). Differences in dietary intakes among Lebanese adults over a decade: results from two National Surveys 1997–2008/2009. Nutrients 11:1738. doi: 10.3390/nu11081738
Nasreddine, L., Taktouk, M., Dabbous, M., and Melki, J. (2019b). The extent, nature, and nutritional quality of foods advertised to children in Lebanon: the first study to use the WHO nutrient profile model for the Eastern Mediterranean Region. Food Nutr. Res. 63. doi: 10.29219/fnr.v63.1604
Nasreddine, L., Chamieh, M. C., Ayoub, J., Hwalla, N., Sibai, A.-M., and Naja, F. (2020). Sex disparities in dietary intake across the lifespan: the case of Lebanon. Nutr. J. 19:24. doi: 10.1186/s12937-020-00543-x
Nghiem, N., Blakely, T., Cobiac, L. J., Pearson, A. L., and Wilson, N. (2015). Health and economic impacts of eight different dietary salt reduction interventions. PLoS One 10:e0123915. doi: 10.1371/journal.pone.0123915
Nishimoto, Y., Tomida, T., Matsui, H., Ito, T., and Okumura, K. (2002). Decrease in renal medullary endothelial nitric oxide synthase of fructose-fed, salt-sensitive hypertensive rats. Hypertension 40, 190–194. doi: 10.1161/01.hyp.0000024267.71656.0d
Norlander, A. E., Saleh, M. A., Pandey, A. K., Itani, H. A., Wu, J., Xiao, L., et al. (2017). A salt-sensing kinase in T lymphocytes, SGK1, drives hypertension and hypertensive end-organ damage. JCI Insight 2:e92801. doi: 10.1172/jci.insight.92801
Noubani, A., Nasreddine, L., Sibai, A. M., Tamim, H., and Isma’eel, H. (2018). Prevalence, awareness, and control of hypertension in Greater Beirut Area, Lebanon. Int. J. Hypertens. 2018:5419861. doi: 10.1155/2018/5419861
O’Donnell, M., Mente, A., Rangarajan, S., McQueen, M. J., Wang, X., Liu, L., et al. (2014). Urinary sodium and potassium excretion, mortality, and cardiovascular events. N. Engl. J. Med. 371, 612–623.
Osborn, J. W., and Foss, J. D. (2017). Renal nerves and long-term control of arterial pressure. Compr. Physiol. 7, 263–320. doi: 10.1002/cphy.c150047
Ozawa, Y., Kobori, H., Suzaki, Y., and Navar, L. G. (2007). Sustained renal interstitial macrophage infiltration following chronic angiotensin II infusions. Am. J. Physiol. Renal Physiol. 292, F330–F339. doi: 10.1152/ajprenal.00059.2006
Pai, S. A., Martis, E. A., Munshi, R. P., Gursahani, M. S., Mestry, S. N., and Juvekar, A. R. (2020). Chrysin mitigated obesity by regulating energy intake and expenditure in rats. J. Tradit. Complement. Med. 10, 577–585. doi: 10.1016/j.jtcme.2019.09.002
Palanisamy, N., and Venkataraman, A. C. (2013). Beneficial effect of genistein on lowering blood pressure and kidney toxicity in fructose-fed hypertensive rats. Br. J. Nutr. 109, 1806–1812. doi: 10.1017/S0007114512003819
Park, J., Leong, M. L., Buse, P., Maiyar, A. C., Firestone, G. L., and Hemmings, B. A. (1999). Serum and glucocorticoid-inducible kinase (SGK) is a target of the PI 3-kinase-stimulated signaling pathway. EMBO J. 18, 3024–3033. doi: 10.1093/emboj/18.11.3024
Perez-Pozo, S. E., Schold, J., Nakagawa, T., Sanchez-Lozada, L. G., Johnson, R. J., and Lillo, J. L. (2010). Excessive fructose intake induces the features of metabolic syndrome in healthy adult men: role of uric acid in the hypertensive response. Int. J. Obes. 34, 454–461. doi: 10.1038/ijo.2009.259
Pfeifle, B., and Ditschuneit, H. (1981). Effect of insulin on growth of cultured human arterial smooth muscle cells. Diabetologia 20, 155–158.
Pilic, L., Pedlar, C. R., and Mavrommatis, Y. (2016). Salt-sensitive hypertension: mechanisms and effects of dietary and other lifestyle factors. Nutr. Rev. 74, 645–658. doi: 10.1093/nutrit/nuw028
Pojoga, L. H., Williams, J. S., Yao, T. M., Kumar, A., Raffetto, J. D., do Nascimento, G. R., et al. (2011). Histone demethylase LSD1 deficiency during high-salt diet is associated with enhanced vascular contraction, altered NO-cGMP relaxation pathway, and hypertension. Am. J. Physiol. Heart Circ. Physiol. 301, H1862–H1871. doi: 10.1152/ajpheart.00513.2011
Porto, M. L., Lírio, L. M., Dias, A. T., Batista, A. T., Campagnaro, B. P., Mill, J. G., et al. (2015). Increased oxidative stress and apoptosis in peripheral blood mononuclear cells of fructose-fed rats. Toxicol. In Vitro 29, 1977–1981. doi: 10.1016/j.tiv.2015.08.006
Powles, J., Fahimi, S., Micha, R., Khatibzadeh, S., Shi, P., Ezzati, M., et al. (2013). Global, regional and national sodium intakes in 1990 and 2010: a systematic analysis of 24 h urinary sodium excretion and dietary surveys worldwide. BMJ Open 3:e003733. doi: 10.1136/bmjopen-2013-003733
Public Health England (2020). Nation Diet and Nutrition Survey. Assessment of Salt Intake From Urinary Sodium in Adults (Aged 19 to 64 Years) in England. 2018/2019. Available online at: https://assets.publishing.service.gov.uk/government/uploads/system/uploads/attachment_data/file/876252/Report_England_Sodium_Survey_2018-to-2019__3_.pdf (accessed 25 March 2020).
Ren, Y., D’Ambrosio, M. A., Garvin, J. L., Peterson, E. L., and Carretero, O. A. (2014). Mechanism of impaired afferent arteriole myogenic response in Dahl salt-sensitive rats: role of 20-HETE. Am. J. Physiol. Renal Physiol. 307, F533–F538. doi: 10.1152/ajprenal.00283.2014
Rezaei, S., Mahmoudi, Z., Sheidaei, A., Aryan, Z., Mahmoudi, N., Gohari, K., et al. (2018). Salt intake among Iranian population: the first national report on salt intake in Iran. J. Hypertens. 36, 2380–2389. doi: 10.1097/HJH.0000000000001836
Rodriguez-Iturbe, B., and Johnson, R. J. (2010). The role of renal microvascular disease and interstitial inflammation in salt-sensitive hypertension. Hypertens. Res. 33, 975–980. doi: 10.1038/hr.2010.148
Rodríguez-Iturbe, B., Pons, H., Quiroz, Y., Lanaspa, M. A., and Johnson, R. J. (2014). Autoimmunity in the pathogenesis of hypertension. Nat. Rev. Nephrol. 10, 56–62.
Rowe, J. W., Young, J. B., Minaker, K. L., Stevens, A. L., Pallotta, J., and Landsberg, L. (1981). Effect of insulin and glucose infusions on sympathetic nervous system activity in normal man. Diabetes 30, 219–225. doi: 10.2337/diab.30.3.219
Rukavina Mikusic, N. L., Kouyoumdzian, N. M., Uceda, A., Del Mauro, J. S., Pandolfo, M., Gironacci, M. M., et al. (2018). Losartan prevents the imbalance between renal dopaminergic and renin angiotensin systems induced by fructose overload. l-Dopa/dopamine index as new potential biomarker of renal dysfunction. Metabolism 85, 271–285. doi: 10.1016/j.metabol.2018.04.010
Sadler, K., Nicholson, S., Steer, T., Gill, V., Bates, B., and Tipping, S. (2011). National Diet & Nutrition Survey—Assessment of Dietary Sodium in Adults (aged 19 to 64 Years) in England, 2011. London: Department of Health.
Schmidlin, O., Forman, A., Leone, A., Sebastian, A., and Morris, R. C. Jr. (2011). Salt sensitivity in blacks: evidence that the initial pressor effect of NaCl involves inhibition of vasodilatation by asymmetrical dimethylarginine. Hypertension 58, 380–385. doi: 10.1161/HYPERTENSIONAHA.111.170175
Schmidlin, O., Forman, A., Tanaka, M., Sebastian, A., and Morris, R. C. Jr. (1999). NaCl-induced renal vasoconstriction in salt-sensitive African Americans: antipressor and hemodynamic effects of potassium bicarbonate. Hypertension 33, 633–639. doi: 10.1161/01.hyp.33.2.633
Schmidlin, O., Sebastian, A. F. A., and Morris, R. C. Jr. (2007). What initiates the pressor effect of salt in salt-sensitive humans? Observations in normotensive blacks. Hypertension 49, 1032–1039. doi: 10.1161/HYPERTENSIONAHA.106.084640
Schulze, M. B., Schulz, M., Heidemann, C., Schienkiewitz, A., Hoffmann, K., and Boeing, H. (2008). Carbohydrate intake and incidence of type 2 diabetes in the European Prospective Investigation into Cancer and Nutrition (EPIC)-Potsdam Study. Br. J. Nutr. 99, 1107–1116. doi: 10.1017/S0007114507853360
Shapiro, A., Mu, W., Roncal, C., Cheng, K. Y., Johnson, R. J., and Scarpace, P. J. (2008). Fructose-induced leptin resistance exacerbates weight gain in response to subsequent high-fat feeding. Am. J. Physiol. Regul. Integr. Comp. Physiol. 295, R1370–R1375.
Shibata, S., Mu, S., Kawarazaki, H., Muraoka, K., Ishizawa, K.-I., Yoshida, S., et al. (2011). Rac1 GTPase in rodent kidneys is essential for salt-sensitive hypertension via a mineralocorticoid receptor–dependent pathway. J. Clin. Invest. 121, 3233–3243. doi: 10.1172/JCI43124
Shinozaki, K., Ayajiki, K., Nishio, Y., Sugaya, T., Kashiwagi, A., and Okamura, T. J. H. (2004). Evidence for a causal role of the renin-angiotensin system in vascular dysfunction associated with insulin resistance. Hypertension 43, 255–262. doi: 10.1161/01.HYP.0000111136.86976.26
Sibai, A. M., Nasreddine, L., Mokdad, A. H., Adra, N., Tabet, M., and Hwalla, N. (2010). Nutrition transition and cardiovascular disease risk factors in Middle East and North Africa countries: reviewing the evidence. Ann. Nutr. Metab. 57, 193–203. doi: 10.1159/000321527
Sikand, G., and Severson, T. (2020). Top 10 dietary strategies for atherosclerotic cardiovascular risk reduction. Am. J. Prev. Cardiol. 4:100106.
Singh, A. K., Amlal, H., Haas, P. J., Dringenberg, U., Fussell, S., Barone, S. L., et al. (2008). Fructose-induced hypertension: essential role of chloride and fructose absorbing transporters PAT1 and Glut5. Kidney Int. 74, 438–447. doi: 10.1038/ki.2008.184
Soleimani, M., and Alborzi, P. (2011). The role of salt in the pathogenesis of fructose-induced hypertension. Int. J. Nephrol. 2011:392708. doi: 10.4061/2011/392708
Stanhope, K. L., Schwarz, J. M., Keim, N. L., Griffen, S. C., Bremer, A. A., Graham, J. L., et al. (2009). Consuming fructose-sweetened, not glucose-sweetened, beverages increases visceral adiposity and lipids and decreases insulin sensitivity in overweight/obese humans. J. Clin. Invest. 119, 1322–1334. doi: 10.1172/JCI37385
Stolarz-Skrzypek, K. (2011). Sugar and salt in the pathogenesis of elevated blood pressure. Hypertension 57, 676–678. doi: 10.1161/HYPERTENSIONAHA.110.167429
Sullivan, J. M., Prewitt, R. L., Ratts, T. E., Josephs, J. A., and Connor, M. J. (1987). Hemodynamic characteristics of sodium-sensitive human subjects. Hypertension 9, 398–406. doi: 10.1161/01.hyp.9.4.398
Susic, D., Frohlich, E. D., Kobori, H., Shao, W., Seth, D., and Navar, L. G. (2011). Salt-induced renal injury in SHRs is mediated by AT1 receptor activation. J. Hypertens. 29, 716–723. doi: 10.1097/HJH.0b013e3283440683
Susic, D., Zhou, X., and Frohlich, E. D. (2009). Angiotensin blockade prevents salt-induced injury of the renal circulation in spontaneously hypertensive rats. Am. J. Nephrol. 29, 639–645. doi: 10.1159/000195633
Svetkey, L. P., McKeown, S. P., and Wilson, A. F. (1996). Heritability of salt sensitivity in black Americans. Hypertension 28, 854–858. doi: 10.1161/01.hyp.28.5.854
Tailakh, A., Evangelista, L. S., Mentes, J. C., Pike, N. A., Phillips, L. R., and Morisky, D. E. (2014). Hypertension prevalence, awareness, and control in Arab countries: a systematic review. Nurs. Health Sci. 16, 126–130. doi: 10.1111/nhs.12060
Tappy, L. (2018). Fructose-containing caloric sweeteners as a cause of obesity and metabolic disorders. J. Exp. Biol. 221(Pt Suppl. 1):jeb164202. doi: 10.1242/jeb.164202
Tappy, L., and Lê, K. A. (2010). Metabolic effects of fructose and the worldwide increase in obesity. Physiol. Rev. 90, 23–46. doi: 10.1152/physrev.00019.2009
The Kingdom of Morocco, and World Health Organization (2018). National Survey on Common Risk Factors for Non-Communicable Diseases 2017–2018, in STEP Wise Survey 2017–2018. Rabat: Kingdom of Morocco.
Thirunavukkarasu, V., Anitha Nandhini, A. T., and Anuradha, C. V. (2004). Lipoic acid attenuates hypertension and improves insulin sensitivity, kallikrein activity and nitrite levels in high fructose-fed rats. J. Comp. Physiol. B 174, 587–592. doi: 10.1007/s00360-004-0447-z
Tohme, R. A., Jurjus, A. R., and Estephan, A. (2005). The prevalence of hypertension and its association with other cardiovascular disease risk factors in a representative sample of the Lebanese population. J. Hum. Hypertens. 19, 861–868. doi: 10.1038/sj.jhh.1001909
Tran, L. T., MacLeod, K. M., and McNeill, J. H. (2009). Endothelin-1 modulates angiotensin II in the development of hypertension in fructose-fed rats. Mol. Cell. Biochem. 325, 89–97. doi: 10.1007/s11010-008-0023-z
Trieu, K., Neal, B., Hawkes, C., Dunford, E., Campbell, N., Rodriguez-Fernandez, R., et al. (2015). Salt reduction initiatives around the world–a systematic review of progress towards the global target. PLoS One 10:e0130247. doi: 10.1371/journal.pone.0130247
Udagawa, K., Miyoshi, M., and Yoshiike, N. (2008). Mid-term evaluation of “Health Japan 21”: focus area for the nutrition and diet. Asia Pac. J. Clin. Nutr. 17(Suppl. 2), 445–452.
United Nations Statistics Division (2016). SDG Indicators. Sustainable Development Goals. Available online at: https://unstats.un.org/sdgs/metadata?Text=&Goal=3&Target=3.4 (accessed November 27, 2021).
Van Beusecum, J. P., Barbaro, N. R., McDowell, Z., Aden, L. A., Xiao, L., Pandey, A. K., et al. (2019). High salt activates CD11c+ antigen-presenting cells via SGK (Serum Glucocorticoid Kinase) 1 to promote renal inflammation and salt-sensitive hypertension. Hypertension 74, 555–563. doi: 10.1161/HYPERTENSIONAHA.119.12761
van Paassen, P., de Zeeuw, D., Navis, G., and de Jong, P. E. (1996). Does the renin-angiotensin system determine the renal and systemic hemodynamic response to sodium in patients with essential hypertension? Hypertension 27, 202–208. doi: 10.1161/01.hyp.27.2.202
Varagic, J., Frohlich, E. D., Susic, D., Ahn, J., Matavelli, L., López, B., et al. (2008). AT1 receptor antagonism attenuates target organ effects of salt excess in SHRs without affecting pressure. Am. J. Physiol. Heart Circ. Physiol. 294, H853–H858. doi: 10.1152/ajpheart.00737.2007
Verma, S., Bhanot, S., and McNeill, J. H. (1999). Sympathectomy prevents fructose-induced hyperinsulinemia and hypertension. Eur. J. Pharmacol. 373, R1–R4. doi: 10.1016/s0014-2999(99)00301-5
Wang, C., Kawakami-Mori, F., Kang, L., Ayuzawa, N., Ogura, S., Koid, S. S., et al. (2020). Low-dose L-NAME induces salt sensitivity associated with sustained increased blood volume and sodium-chloride cotransporter activity in rodents. Kidney Int. 98, 1242–1252. doi: 10.1016/j.kint.2020.05.050
Webster, J., Trieu, K., Dunford, E., and Hawkes, C. (2014). Target salt 2025: a global overview of national programs to encourage the food industry to reduce salt in foods. Nutrients 6, 3274–3287. doi: 10.3390/nu6083274
Wolf, G. (1995). The effect of fasting and fructose and glucose infusion on gluconeogenesis and triose phosphate flux in rats in vivo. Nutr. Rev. 53, 299–301. doi: 10.1111/j.1753-4887.1995.tb01481.x
World Health Organization (2014). Salt Reduction and Iodine Fortification Strategies in Public Health. Geneva: World Health Organization.
World Health Organization (2015). Guideline: Sugars Intake for Adults and Children. Geneva: World Health Organization.
World Health Organization (2016). Global NCD Target: Reduce Salt Intake. Geneva: World Health Organization.
World Health Organization (2019). Global Action Plan on Physical Activity 2018-2030: More Active People for a Healthier World. Geneva: World Health Organization.
World Health Organization (2021). Cardiovascular Diseases. Available online at: https://www.who.int/health-topics/cardiovascular-diseases#tab=tab_1 (accessed November 11, 2021).
World Health Organization Global Database on the Implementation of Nutrition Action [GINA] (2014). Policy – Non Communicable Disease Prevention and Control Plan (NCD-PCP) for Lebanon 2015-2020 - Lebanon. Beirut-Lebanon: NCD National Focal Point in Lebanon and WHO Lebanon Office.
World Health Organization Regional Office for the Eastern Mediterranean (2015). WHO-EM/NUT/268/E - Report on the Technical Consultation on Salt and Fat Reduction Strategies in the Eastern Mediterranean Region. Tunis: World Health Organization.
Xu, C., Lu, A., Lu, X., Zhang, L., Fang, H., Zhou, L., et al. (2017). Activation of renal (Pro)renin receptor contributes to high fructose-induced salt sensitivity. Hypertension 69, 339–348. doi: 10.1161/HYPERTENSIONAHA.116.08240
Zeidan, R. K., Farah, R., Chahine, M. N., Asmar, R., Hosseini, H., Salameh, P., et al. (2016). Prevalence and correlates of coronary heart disease: first population-based study in Lebanon. Vasc. Health Risk Manag. 12, 75–84. doi: 10.2147/VHRM.S97252
Zhang, D. M., Jiao, R. Q., and Kong, L. D. (2017). High dietary fructose: direct or indirect dangerous factors disturbing tissue and organ functions. Nutrients 9:335. doi: 10.3390/nu9040335
Zhang, L., Miyaki, K., Wang, W., and Muramatsu, M. (2010). CYP3A5 polymorphism and sensitivity of blood pressure to dietary salt in Japanese men. J. Hum. Hypertens. 24, 345–350. doi: 10.1038/jhh.2009.74
Keywords: lifestyle, diet, salt, fructose, hypertension, immunity
Citation: Labban MM, Itani MM, Maaliki D, Radwan Z, Nasreddine L and Itani HA (2022) The Sweet and Salty Dietary Face of Hypertension and Cardiovascular Disease in Lebanon. Front. Physiol. 12:802132. doi: 10.3389/fphys.2021.802132
Received: 26 October 2021; Accepted: 20 December 2021;
Published: 25 January 2022.
Edited by:
Crystal A. West, Appalachian State University, United StatesReviewed by:
Aline M. A. De Souza, Georgetown University, United StatesCopyright © 2022 Labban, Itani, Maaliki, Radwan, Nasreddine and Itani. This is an open-access article distributed under the terms of the Creative Commons Attribution License (CC BY). The use, distribution or reproduction in other forums is permitted, provided the original author(s) and the copyright owner(s) are credited and that the original publication in this journal is cited, in accordance with accepted academic practice. No use, distribution or reproduction is permitted which does not comply with these terms.
*Correspondence: Lara Nasreddine, bG4xMEBhdWIuZWR1Lmxi; Hana A. Itani, aGk0MEBhdWIuZWR1Lmxi
†These authors have contributed equally to this work
‡These authors share senior and last authorship
Disclaimer: All claims expressed in this article are solely those of the authors and do not necessarily represent those of their affiliated organizations, or those of the publisher, the editors and the reviewers. Any product that may be evaluated in this article or claim that may be made by its manufacturer is not guaranteed or endorsed by the publisher.
Research integrity at Frontiers
Learn more about the work of our research integrity team to safeguard the quality of each article we publish.