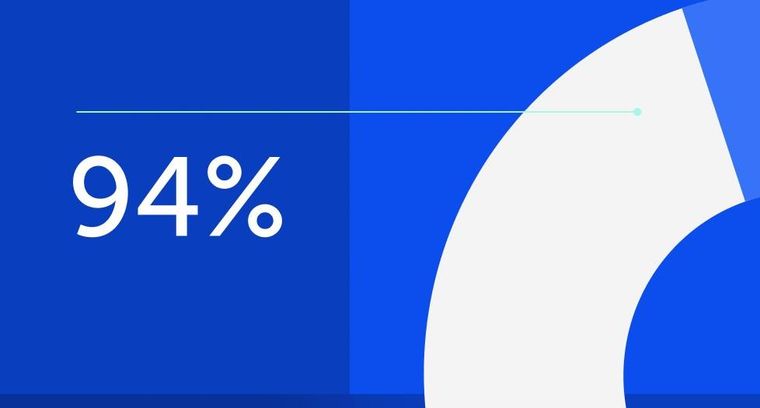
94% of researchers rate our articles as excellent or good
Learn more about the work of our research integrity team to safeguard the quality of each article we publish.
Find out more
REVIEW article
Front. Physiol., 03 January 2022
Sec. Renal Physiology and Pathophysiology
Volume 12 - 2021 | https://doi.org/10.3389/fphys.2021.792897
Chronic kidney disease (CKD) is a major public health problem that affects more than 10% of the population worldwide and has a high mortality rate. Therefore, it is necessary to identify novel treatment strategies for CKD. Incidentally, renal fibrosis plays a central role in the progression of CKD to end-stage renal disease (ESRD). The activation of inflammatory pathways leads to the development of renal fibrosis. In fact, interleukin-33 (IL-33), a newly discovered member of the interleukin 1 (IL-1) cytokine family, is a crucial regulator of the inflammatory process. It exerts pro-inflammatory and pro-fibrotic effects via the suppression of tumorigenicity 2 (ST2) receptor, which, in turn, activates other inflammatory pathways. Although the role of this pathway in cardiac, pulmonary, and hepatic fibrotic diseases has been extensively studied, its precise role in renal fibrosis has not yet been completely elucidated. Recent studies have shown that a sustained activation of IL-33/ST2 pathway promotes the development of renal fibrosis. However, with prolonged research in this field, it is expected that the IL-33/ST2 pathway will be used as a diagnostic and prognostic tool for renal diseases. In addition, the IL-33/ST2 pathway seems to be a new target for the future treatment of CKD. Here, we review the mechanisms and potential applications of the IL-33/ST2 pathway in renal fibrosis; such that it can help clinicians and researchers to explore effective treatment options and develop novel medicines for CKD patients.
Chronic kidney disease (CKD) is a clinical syndrome with definite changes in kidney structure and function that last for more than 3 months (Ammirati, 2020). Incidentally, CKD is the third leading cause of premature death in patients, following acquired immunodeficiency syndrome and diabetes. In fact, more than 10% of the world’s population is affected by CKD (Lv et al., 2018; Djudjaj and Boor, 2019), thereby making it a major public health problem issue. Interestingly, renal fibrosis occurs in severe cases of CKD, and it is considered as an underlying pathological process of this disease; moreover, renal fibrosis ultimately leads to the progression of CKD to end-stage renal disease (ESRD; Berchtold et al., 2017; Chen et al., 2018a; Martínez-Klimova et al., 2019). Therefore, the underlying mechanisms of renal fibrosis should be extensively studied to ensure a better understanding of CKD development because the inhibition of renal fibrosis may help to decrease the rate of CKD progression.
Fibrosis is a highly coordinated process that occurs as a result of the tissue repair response. Incidentally, the tissue repair response may become dysregulated if the healing response of tissues continues beyond normal wound healing, for instance during chronic inflammation, trauma, infection, metabolic disorders, inflammation, and autoimmunity. This results in the production of cytokines and chemokines. The release of these mediators leads to the local activation of collagen-producing mesenchymal cells, to a transition of various cell types into myofibroblasts as well as to the recruitment of fibroblast precursors (Weiskirchen et al., 2019; Henderson et al., 2020; Zhang and Zhang, 2020). The main pathological characteristics of renal fibrosis are renal interstitial fibroblast hyperplasia and the aberrant and excessive deposition of extracellular matrix (ECM). These changes destroy the normal tubular and interstitial structures of the kidneys (Humphreys, 2018; Zhang et al., 2020). Interestingly, inflammatory responses are central to the progression of renal fibrosis (Zhao et al., 2015; Chen et al., 2019). They involve various cytokine-mediated multi-signaling pathways, including transforming growth factor-beta (TGF-β), tumor necrosis factor-alpha (TNF-α), and leukocyte mediators (Lv et al., 2018; Chen et al., 2018a; Prakoura et al., 2019). Interleukin 33 (IL-33), the gene for which is located on chromosome 9, is a newly discovered member of the IL-1 cytokine family and a pivotal regulator of inflammatory and immune responses (Molofsky et al., 2015; Di Salvo et al., 2018; Chen et al., 2020). It functions in association with the suppression of tumorigenicity 2 (ST2) receptor. In fact, the IL-33/ST2 pathway is involved in causing an imbalance between widespread inflammation and tissue regeneration in several organs, such as the lungs, liver, skin, and gastrointestinal system, ultimately leading to fibrosis (Rankin et al., 2010; Tan et al., 2018; Wu et al., 2018; Imai et al., 2019). Due to the potential role of the IL-33/ST2 pathway in fibrotic diseases, it has received increasing attention. In fact, this review discusses the current progress in research related to the IL-33/ST2 pathway in renal fibrosis to provide new insights into developing treatment options for CKD patients.
In 1999, IL-33 was first identified in a canine model of arachnoid hemorrhage, which expressed the protein DV27 (Onda et al., 1999). Subsequently, in 2003, endogenous IL-33 and its mRNA were discovered in human tissues, and initially, defined as a nuclear factor from high endothelial venules (NF-HEV; Baekkevold et al., 2003). In 2005, Schmitz et al. (2005) discovered that the hydroxyl-terminal portion of NF-HEV resembles the three-dimensional folded structure of the IL-1 family of cytokines. Moreover, as a cytokine, this factor induces a type 2 immune response by binding to the ST2 receptor. Based on these observations, NF-HEV was classified as a new member of the IL-1 cytokine family, and it was named IL-33 (Schmitz et al., 2005). The IL-33 is a regulator of inflammation, and can induce T-helper 2 (Th2)-mediated innate and adaptive immune responses (Kakkar and Lee, 2008; Kotsiou et al., 2018). Additionally, IL-33 participates in the pathogenesis of renal, neurological, hepatic, pulmonary, and eye diseases. It is a cell cytokine that promotes inflammatory responses and has a characteristic of alarm (Moussion et al., 2008; Li et al., 2019b; Wang et al., 2020). Since the expression of IL-33 has been reported to increase in tissues during inflammation (Kotsiou et al., 2018), IL-33 is a potential mediator of various inflammatory diseases (Dinarello, 2005). Furthermore, IL-33 plays an invaluable role in various biological responses, such as regulation of immune responses, maintenance of tissue homeostasis, and tissue repair and remodeling (Drake and Kita, 2017).
The IL-33 receptor complex is a heterodimeric complex consisting of IL-1 receptor accessory protein (IL-1RACP) and ST2 encoded by IL-1receptor type 1(IL1R1; Cayrol and Girard, 2014). In fact, the IL-1RACP functions as a co-receptor for IL-33 signaling (Hong et al., 2019). The ST2, a member of the Toll-like receptor/IL-1 receptor superfamily, has four subtypes, including two variant forms (ST2LV and ST2V), a soluble form (sST2), and a membrane-bound form (ST2L; Bergers et al., 1994; Iwahana et al., 2004; Schmitz et al., 2005; Griesenauer and Paczesny, 2017). The ST2LV is a form of ST2L that is produced by selective splicing (Iwahana et al., 2004). The ST2V is mainly expressed in organs of the digestive system. Its overexpression in cell lines leads to restricted membrane localization (De la Fuente et al., 2015). The most significant subtypes are ST2L and sST2, and they are highly expressed in the kidneys, lungs, placenta, and stomach (Li et al., 2000; Kaur et al., 2020). The ST2L is a functional component of IL-33 signaling, and it promotes inflammation and conducts a Th2-type immune response by activating downstream molecules, such as myeloid differentiation primary response 88 (MyD88) and tumor necrosis factor receptor (TNFR)-associated factor 6 (TRAF6) proteins (Milovanovic et al., 2012; Takeda et al., 2018). The sST2 lacks transmembrane and intracellular domains and can act as a decoy receptor for IL-33 that can help to block IL-33 signaling (Xu et al., 2017; Antunes et al., 2018). It is expressed in mast cells and fibroblasts and its activity is induced by cytokines such as TNF-α.
When cells detect inflammatory signals, or in cases of tissue damage or necrosis occurs, IL-33 is rapidly released from the cell, and it binds to the heterodimeric receptor complex ST2L/IL1-RACP, eventually forming IL-33/ST2L/IL1-RACP complex (Schmitz et al., 2005; Chackerian et al., 2007). Subsequently, MyD88, IL-1 receptor-associated kinase 1 (IRAK1), IRAK4, and TRAF6 are recruited. This leads to the activation of signaling pathways, including nuclear factor kappa light chain enhancer of activated B cells (NF-κB), extracellular signal-regulated kinase (ERK1/2), c-Jun N-terminal kinase (JNK), and p38 mitogen-activated protein kinase (MAPK), ultimately leading to the production of several pro-inflammatory factors (Funakoshi-Tago et al., 2011; Chen et al., 2017; Li et al., 2018; Allegra et al., 2019). However, this pathway can be attenuated through diverse mechanisms. For instance, sST2 can act as a decoy receptor that competes with ST2L to bind with IL-33. Moreover, single immunoglobulin IL1-related receptor (SIGIRR) can also repress this pathway (Gao et al., 2015; Larsen et al., 2018; Shen et al., 2018; Liu et al., 2019d; Figure 1).
Figure 1. The interleukin-33 (IL-33)/suppression of tumorigenicity 2 (ST2) pathway. Activation of the IL-33/ST2 pathway: IL-33 binds to a receptor complex composed of ST2L and interleukin 1 receptor accessory protein (IL-1RACP) on the cell membrane. The interaction between the C-terminal domain of this receptor complex promotes the recruitment of myeloid differentiation primary response 88 (MyD88), IL-1 receptor-associated kinase 1 (IRAK1), IRAK4, and tumor necrosis factor receptor (TNFR)-associated factor 6 (TRAF6), thus leading to the activation of nuclear factor kappa light chain enhancer of activated B cells (NF-κB), c-Jun N-terminal kinase (JNK), extracellular signal-regulated kinase (ERK), and P38 pathways. The activation of these pathways facilitates the gene expression of chemokines and growth factors. The inhibition of the IL-33/ST2 pathway: soluble ST2 (sST2) acts as a decoy receptor for IL-33 to block the binding of IL-33 to the receptor. single immunoglobulin IL1-related receptor (SIGIRR) can destroy ST2L/IL1RAcP heterodimers.
Incidentally, IL-33 is widely expressed in various organs, including the heart, brain, kidneys, liver, spleen, and lungs. At the cellular level, IL-33 is mainly expressed by non-immune cells, including epithelial cells, endothelial cells, and fibroblasts (Schmitz et al., 2005; Martin and Martin, 2016; Chen et al., 2017; Drake and Kita, 2017). In addition, immune cells such as activated macrophages and dendritic cells are also sources of IL-33, but the expression level of IL-33 is low (Li et al., 2014a; Yang et al., 2016). In kidney, immunohistochemical analysis has revealed the presence of IL-33+ cells in the renal tubules of the patients with chronic allograft dysfunction (Xu et al., 2018). Similarly, in patients with renal allograft rejection, IL-33 has been detected in the renal tubules and interstitium (Yu et al., 2021). Furthermore, IL-33 is constitutively expressed in endothelial cells of large and small renal vessels (Akcay et al., 2011). Chen et al. (2016) have also reported the presence of a major population of IL-33+ cells among α-smooth muscle actin (α-SMA)+ interstitial myofibroblasts and a minor population among CD31+ peritubular vascular endothelial cells in the kidneys of unilateral ureteral obstruction (UUO) mice. In addition, IL-33 was constitutively expressed throughout the kidney in peritubular and periglomerular spaces of mice kidneys (Ferhat et al., 2018).
With respect to the IL-33 receptors, ST2L is selectively expressed by several immune cells involved in type 2 immune responses, such as macrophages, invariant natural killer T (iNKT) cells, mast cells, and lymphocytes (Kaur et al., 2015; Lu et al., 2015; Larsen et al., 2018; Kaur et al., 2020). The sST2 is mainly expressed in fibroblasts and epithelial cells (Akimoto and Takenaga, 2019). In the kidney, ST2 is strongly expressed in the glomeruli, renal tubules, and peritubular capillaries in patients with diffuse cutaneous systemic sclerosis (Manetti et al., 2010). Additionally, Yu et al. (2021) also reported ST2 may be localized to renal tubules and interstitial spaces. Another study reported that ST2 is expressed in macrophages, bipotent T and innate lymphoid cells (T/ILC), neutrophils, and monocytes in kidneys of mice after obstructive injury (Chen et al., 2018b). However, the localization of ST2 to other non-immune cells in murine kidneys has not been completely elucidated, and it requires further investigation.
The IL-33/ST2 pathway is widely involved in the fibrosis of various organs, including the airway, lungs, heart, intestine, liver, and skin (Table 1). As shown in Table 1, the role of this pathway in cardiac fibrosis is controversial. Sanada et al. (2007) observed that the IL-33/ST2 pathway limits overall ventricular fibrosis, whereas Ghali et al. (2020) reported IL-33 aggravated the deterioration of cardiac function and cardiac remodeling. In fact, it is associated with activation of myofibroblasts and an increase in the pro-fibrotic markers, such as connective tissue growth factor (CTGF) and TGF-β (Ghali et al., 2020). Overall, in majority of fibrotic diseases, this pathway mainly promotes inflammatory responses and facilitates organ fibrosis, ultimately leading to organ dysfunction.
Diabetes (Abd Rachman Isnadi et al., 2018), obstructive nephropathy (Martínez-Klimova et al., 2020), ischemia-reperfusion injury (IRI; Rossi et al., 2021), and lupus nephritis (Davidson, 2016) promote the development of CKD. In fact, there are many publications showing that the IL-33/ST2 pathway exacerbates the structural and functional damage of the kidneys in CKD (Table 2). Specifically, in studies of UUO model that is classical renal fibrosis model (Chevalier et al., 2009; Martínez-Klimova et al., 2019), IL-33 inhibited fibrosis in the early stages (Gatti et al., 2021). However, continuous stimulation resulted in significant upregulation of the IL-33/ST2 pathway (Chen et al., 2016; Li et al., 2019b; Gatti et al., 2021). In a word, the progression of renal disease is associated with the sustained activation of IL-33/ST2. Therefore, the IL-33/ST2 pathway appears to be a significant mechanism underlying renal fibrotic disease.
During chronic kidney injury and inflammation, endothelial cells, epithelial cells, and fibroblasts release IL-33 (Tonacci et al., 2019). This IL-33 activates ST2-expressing cells, such as polarized M2 macrophages, innate lymphoid type 2cells (ILC2s), CD4+ T cells, and iNKTs, thereby triggering inflammation and tissue repair responses. It has been reported that macrophages play an important role in obstructive kidney injury (Meng et al., 2014). Interestingly, IL-33, along with other cytokines, promotes the transformation of M0 macrophages into M2 macrophages (Liew et al., 2016), and persistence of these M2 macrophages contributes to fibrosis (Mosser and Edwards, 2008; Kim et al., 2015). Moreover, Li et al. (2019b) discovered that during UUO-induced renal fibrosis, IL-33 exacerbates renal injury and promotes renal fibrosis via the activity of M2 macrophages and an increased secretion of IL-13 and TGF-β1. Incidentally, ILC2s are activated in damaged kidneys; for instance increased amounts of ILC2s have been reported in the kidneys of UUO mice models (Chen et al., 2018b). Additionally, ILC2s promote lung and liver fibrosis, and they can secrete Th2-specific cytokines, such as IL-4 and IL-13 (Liu et al., 2019a), which, in turn, exhibit significant pro-fibrotic activity (Fertin et al., 1991; Wynn, 2003). However, one study reported that IL-33 for a short period of time may attenuate the IRI-induced renal damage via ILC2 activity (Riedel et al., 2017; Cao et al., 2018). This may have occurred due to the short duration and low dose of treatment with recombinant IL-33 (rIL-33). Akcay et al. (2011) demonstrated that a sustained high level of rIL-33 can exacerbate renal damage, but they did not specify the role of ILC2s in this process. Therefore, the role of ILC2s, stimulated by sustained high levels of rIL-33, in cases of renal fibrosis requires further investigation. Furthermore, IL-33 can also drive the activation of iNKTs and the infiltration of neutrophils into inflamed tissues (Bourgeois et al., 2011; Thierry et al., 2014; Ferhat et al., 2018). In fact, Ferhat et al. observed that IL-33 promotes IFN-γ/IL-17A production by iNKT cells in IRI, further amplifying the renal tissue injury (Ferhat et al., 2018). The CD4+ T cells are also vital for the progression of renal fibrosis (Liu et al., 2012). A previous study has reported that the infiltration of renal CD4+ T cells was reduced in acute kidney injury (AKI) mice model injected with sST2. Moreover, in wild-type mice, rIL-33 exacerbated AKI and raised levels of C-X-C motif ligand 1 (CXCL1), but similar results were not observed in CD4 deficient mice (Akcay et al., 2011). These results suggest that the deleterious effects of IL-33 are probably mediated via CD4+T cells.
In addition to effects of the IL-33/ST2 pathway on the abovementioned immune cells, a few studies also reported its effects on non-immune cells. IL-33 is involved in post-transplant interstitial fibrosis by activating the p38 MAPK signaling pathway and promoting epithelial-mesenchymal transition (EMT) in HK-2 cells (Xu et al., 2018). In fact, Chen et al. found elevated expression of IL-33 in mesenchymal myofibroblasts and perivascular endothelial cells of the renal tubules. They also confirmed that IL-33 deletion via gene knockdown reduced UUO-induced renal fibrosis (Chen et al., 2016). These observations suggest that the upregulation of the IL-33/ST2 pathway in case of obstructed kidney disease may promote tubular cell injury and interstitial fibrosis. Another study reported that upregulation of the IL-33/ST2 signaling pathway in case of systemic lupus erythematosus (SLE) promoted renal tubular cell injury and fibrosis primarily through renal EMT (Chen et al., 2017). Moreover, Liang et al. (2017) found that IL-33 worsened renal fibrosis after IRI. On the contrary, sST2 significantly inhibited collagen deposition in the kidneys of IRI-stressed mice by inhibiting IL-33. It is possible that IL-33 recruits bone marrow-derived fibroblasts and inflammatory cells into the kidney, thereby producing proinflammatory factors and profibrotic molecules (Liang et al., 2017). Similarly, Zhu et al. found that IL-33 induced the phenotypic transformation of bone marrow-derived monocytes into fibroblasts in a dose-dependent manner, thereby leading to a marked increase in the expression of α-SMA and fibronectin (Zhu et al., 2019).
Generally, the mechanisms of the IL-33/ST2 pathway in the progression of renal fibrosis can be summarized in two aspects (Figure 2). On the one hand, this pathway promotes the secretion of pro-inflammatory and pro-fibrotic factors, such as IL-4, IL-13, and TGF-β by some immune cells. These cytokines promote inflammation. Notably, TGF-β is an important central mediator of renal fibrosis (Meng et al., 2015). Some previous studies found that IL-33 expression was positively correlated with TGF-β (Li et al., 2014a; Kotsiou et al., 2018; Elsherbiny et al., 2020). This may be related to macrophages (Li et al., 2019b) or ILC2 (Nagashima and Iyoda, 2021). The specific link and mechanism between IL-33/ST2 and TFG-β need to be investigated further. On the other hand, the IL-33/ST2 pathway can promote fibrogenesis by triggering EMT of renal tubular epithelial cells and activating myeloid fibroblasts. Ultimately, these changes promote the secretion of collagen and fibronectin to facilitate fibrogenesis. However, the effect of this pathway on other non-immune cells of the kidney, such as collecting duct cells and podocytes, is unknown and requires further research.
Figure 2. Mechanisms of IL-33/ST2 involvement in renal fibrosis. When the kidney is injured, endothelial cells, epithelial cells, and fibroblasts release IL-33. IL-33 binds to ST2L on the cell membrane and activates downstream pathways. For immune cells, such as M2 macrophages, ILC2s, iNKTs, CD4+T cells, and neutrophils, IL-33 activates these cells and promotes the secretion of some cytokines that further aggravate inflammation. For other non-immune cells in the kidney, IL-33 can lead to EMT of renal tubular epithelial cells and activation of myofibroblasts. Eventually, COL1, α-SMA etc. are produced. Continued inflammation, EMT, and activation of myofibroblasts finally lead to renal fibrosis.
Patients with kidney disease lack clinical symptoms and have low sensitivity to serum creatinine (Cr) tests in the early stages. Moreover, a decreased glomerular filtration rate is usually detected in the moderate or severe stages of CKD; this means that patients miss the optimal period for treatment. Early detection and correction of modifiable risk factors can decrease the rate of decline in kidney function (Ruggenenti et al., 1999; McClellan and Flanders, 2003; de Zeeuw et al., 2006; Ho et al., 2013). Therefore, researchers aim to identify novel biological markers in patients with kidney disease that will allow early detection and treatment of the condition.
Currently, IL-33 and ST2 are used as biological markers mainly in patients with heart disease. In fact, sST2 has been shown to independently predict heart failure (HF), major cardiovascular events, and mortality of the patients (Seo et al., 2018; Alam et al., 2019; Homsak and Gruson, 2020). Incidentally, levels of cardiac ST2 mRNA and serum ST2 protein are known to increase in patients with myocardial infarction (Weinberg et al., 2002). Additionally, IL-33 has been associated with vascular disease dysfunction (Gungor et al., 2017). It has been reported that IL-33 effectively activates the type 2 cytokine milieu in the damaged heart and is associated with the deterioration of cardiac function as well as cardiac remodeling (Ghali et al., 2020). Furthermore, serum IL-33 and sST2 levels were elevated in patients with chronic HF (Zhang et al., 2012), and they continue to increase with the degradation of cardiac function (Xiang et al., 2021). What is more, the expression of IL-33 and ST2 in myocardial tissue from patients with end-stage HF was reported to be elevated. In addition, their levels were significantly correlated with the degree of cardiac fibrosis and the expression level of TGFβ1 (Tseng et al., 2018). It is evident that the IL-33/ST2 pathway plays a regulatory role in the progression of cardiac fibrosis, thereby making it a useful tool for the prognosis and diagnosis of heart failure.
It is well-known that the heart and kidneys are closely linked organs under physiological and pathophysiological conditions (Palazzuoli et al., 2015). Acute or chronic heart disease can directly lead to the deterioration of acute or chronic renal function and vice versa. This phenomenon referred to as the cardiorenal syndrome. Incidentally, the coexistence of cardiac and renal dysfunction can significantly increase the mortality and morbidity of the patients, as well as cost of patient care (Ronco et al., 2010; Cruz et al., 2011; Palazzuoli et al., 2015). HF is the main cardiovascular complication and the leading cause of mortality in patients with renal disease, especially the ones with ESRD (Bansal et al., 2017). It is clear that kidney disease and heart disease are closely related. Therefore, given that serum IL-33 and sST2 levels are known to reflect heart function, some researchers have investigated whether they might also reflect kidney function. One study reported that circulating levels of sST2 are independently and negatively associated with a poor diuretic response in patients with acute HF and renal dysfunction (Liu et al., 2019b). Another study revealed that serum IL-33 is a sensitive marker of renal function in patients with gout (Duan et al., 2016). Gungor et al. (2017) reported that IL-33 and ST2 levels increase with the gradual progression of the CKD stages. In fact, in a cohort study of critically ill patients, the ones with elevated sST2 expression had the worst prognosis (Dieplinger et al., 2016). However, some studies indicate that sST2 is a better indicator of risk for end-stage dialysis than IL-33 in patients with kidney disease (Bao et al., 2012; de Boer et al., 2015; Tuegel et al., 2018; Lukic et al., 2020; Yan et al., 2020). For instance, Bao et al. (2012) did not observe any difference in the serum IL-33 concentrations of patients with CKD and that of healthy individuals. However, sST2 serum level was elevated in patients. Furthermore, a significant correlation between the sST2 levels and the disease severity was found by correlation analysis (Bao et al., 2012). Similarly, Lukic et al. (2020) reported that sST2, but not IL-33, is associated with ESRD parameters, and the serum sST2 concentration is positively correlated with that of serum urea and creatinine. Hence, it is unclear whether IL-33 can be used as a reference indicator in patients with kidney disease. However, sST2 appears to be a promising biological marker for kidney diseases, and deserves further study and wide application in clinical practice.
Patients with ESRD require dialysis or kidney transplantation (Hewitson, 2009). However, this treatment modality is limited in the developing and underdeveloped countries due to the high costs (Nogueira et al., 2017). At present, glucocorticoids are the mainstay of treatment for kidney disease. However, their widespread clinical use is largely limited because of their association with a variety of serious adverse effects, such as infections, hypertension, and osteoporosis (Ponticelli and Locatelli, 2018; Vandewalle et al., 2018). Therefore, it is necessary to identify new therapeutic targets and medicines to prevent disease progression and ultimately death.
From the above discussion, it is evident that the IL-33/ST2 pathway plays a key role in renal fibrosis. In particular, in vivo studies in IL-33-deficient transgenic mice further supported the importance of the IL-33/ST2 pathway in the progression of renal fibrosis. For instance, the damage to the proximal tubules was reduced in the kidneys of the IL33−/− UUO model mice (Chen et al., 2016). Similarly, compared with wild-type mice, IRI mice lacking IL-33 showed reduced levels of tubular cell injury (Ferhat et al., 2018). Moreover, the SLE mice treated with anti-IL-33Ab, which has a protective effect on SLE, showed lower serum and renal levels of IL-1β, IL-6, and IL-17 than that in the untreated mice (Li et al., 2014b). Therefore, the IL-33/ST2 pathway may form a new therapeutic target for CKD.
In this regard, IL-33 blockers may be a novel treatment modality for CKD patients; for instance, the monoclonal antibody blockade of IL-33 is a novel immunotherapy agent. Incidentally, AstraZeneca is conducting the trial of a monoclonal antibody called MEDI3506 (anti-IL-33) in patients with diabetic nephropathy. However, this trial has not yet been completed (Jiang et al., 2021). It is known that the main side effect of monoclonal antibody administration is the risk of an adverse immune response (Hansel et al., 2010; Liu and Li, 2014). Therefore, this method requires extensive research. In addition, IL-2 and IL-33, especially as a hybrid cytokine (IL233-bearing IL-2 and IL-33 activities in one molecule), potentiate Tregs and ILC2s to prevent renal injury (Sabapathy et al., 2019). However, this method may be suitable only on a short-term basis as IL-33 becomes a deleterious factor with prolonged stimulation (Akcay et al., 2011).
Traditional Chinese Medicine (TCM) and other natural plant compounds offer certain therapeutic advantages over standard medicine, such as potentially lower prices and ease of access. Therefore, these form a good choice for the development of new medicines. Incidentally, the use of TCM has been extensively studied in cases of renal fibrosis (Table 3). However, there is limited information about TCM targeting the IL-33/ST2 pathway in the treatment of kidney diseases. Interestingly, Calycosin, an isoflavone, is the predominant component of Radix Astragali, which has been widely administered to ameliorate the symptoms of diabetes and diabetic nephropathy (Zhang et al., 2019). Calycosin treatment significantly reduced mRNA expression and protein levels of IL-33 and ST2 when compared to diabetic rats. Thereby, it affected the downstream pathway, p65 NF-κB. Finally, it delayed progression of renal fibrotic events (Elsherbiny et al., 2020). In addition, astragaloside IV (AS-IV; Bao et al., 2016), Cnidium Cnidii (Yang et al., 2020), and salidroside (Cai et al., 2020) have also been shown to reduce IL-33 to improve the disease condition. However, these studies were conducted in non-renal diseases, such as allergic inflammation. AS-IV, Cnidium cnidii, and salidroside have therapeutic effects on renal fibrosis (see Table 3). Thus, the role of these TCMs on IL-33/ST2 signaling in the kidney remains to be investigated.
Table 3. Mechanistic studies of Traditional Chinese Medicine (TCM) in the treatment of renal fibrosis.
Other natural plant compounds can also attenuate IL-33 expression. Chrysin (CR) is one of the flavonoids that are commonly used as a traditional medicine and found in many plant extracts. CR has an ameliorative effect on CKD (Ali et al., 2015). Moreover, CR reduced PbAc-induced renal inflammation and significantly reduced renal IL-33 levels (Kucukler et al., 2021). Zingerone (ZO), a component of dry ginger root, has several pharmacological activities owing to its antioxidant, anti-inflammatory and anti-apoptotic properties. Kandemir et al. (2018) demonstrated that ZO remarkably reduced the levels of IL-33 in VCM-induced nephrotoxicity.
In conclusion, blocking the IL-33/ST2 pathway may be a novel therapeutic strategy for treating renal diseases in the future. Calycosin, CR, and ZO can attenuate IL-33 to protect renal function, thereby making them potential candidates for developing new medicines. Other effective natural compounds targeting IL-33/ST2 still need to be discovered for the treatment of kidney diseases.
Kidney disease is a major public health problem worldwide. Although kidney transplantation and dialysis treatments are available, there is a significant lack of effective treatments. Therefore, in recent years, researchers have focused on the identification of new treatment targets for patients suffering from kidney diseases. Gradually, the role of the IL-33/ST2 pathway in fibrotic diseases has been established. Although its effects have been extensively studied in cardiac, pulmonary, and liver fibrosis (Willems et al., 2012; Sun et al., 2017; Drake and Prakash, 2020), research related to renal fibrosis still in the nascent stages. In this review, we have focused on discussing the role of the IL-33/ST2 pathway in the development and progression of renal fibrosis as well as the underlying mechanisms of this process. Incidentally, IL-33 activates the immune cells and promotes the secretion of certain cytokines, further aggravating inflammation. However, in other non-immune cells of the kidney, IL-33 can lead to EMT of renal tubular epithelial cells and activation of myofibroblasts. Additionally, many clinical studies have revealed that the IL-33/ST2 pathway may be an effective biomarker of kidney disease, and this is important for the early diagnosis of CKD and assessment of patient prognosis. However, further detailed and comprehensive studies are required for addressing the existing gaps in knowledge. Meanwhile, the IL-33/ST2 pathway appears to be a promising therapeutic target for renal fibrosis. TGF-β, a strong pro-fibrotic factor, and its relevant pathway have been extensively studied in cases of renal fibrosis (Loboda et al., 2016). However, IL-33 is a newly discovered pro-fibrotic factor. Hence, information related to the IL-33/ST2 pathway is still limited, and medicine targeting the IL-33/ST2 signaling is yet to be developed. We hope this review will contribute to the comprehensive studies of the IL-33/ST2 pathway in renal fibrosis and help in the development of novel therapeutic agents.
X-YT designed the ideas and wrote the first version of the manuscript. H-YJ contributed to resources. X-YT and H-YJ have contributed equally to this work. Y-RM reviewed the draft of the manuscript. All authors contributed to the article and approved the submitted version.
The study was sustained by National Natural Science Foundation of China (81973732).
The authors declare that the research was conducted in the absence of any commercial or financial relationships that could be construed as a potential conflict of interest.
All claims expressed in this article are solely those of the authors and do not necessarily represent those of their affiliated organizations, or those of the publisher, the editors and the reviewers. Any product that may be evaluated in this article, or claim that may be made by its manufacturer, is not guaranteed or endorsed by the publisher.
Abd Rachman Isnadi, M. F., Chin, V. K., Abd Majid, R., Lee, T. Y., Abdullah, M. A., Omenesa, R. B., et al. (2018). Critical roles of IL-33/ST2 pathway in neurological disorders. Mediat. Inflamm. 2018:5346413. doi: 10.1155/2018/5346413
Akcay, A., Nguyen, Q., He, Z., Turkmen, K., Won Lee, D., Hernando, A. A., et al. (2011). IL-33 exacerbates acute kidney injury. J. Am. Soc. Nephrol. 22, 2057–2067. doi: 10.1681/ASN.2010091011
Akimoto, M., and Takenaga, K. (2019). Role of the IL-33/ST2L axis in colorectal cancer progression. Cell. Immunol. 343:103740. doi: 10.1016/j.cellimm.2017.12.014
Alam, M. L., Katz, R., Bellovich, K. A., Bhat, Z. Y., Brosius, F. C., de Boer, I. H., et al. (2019). Soluble ST2 and Galectin-3 and progression of CKD. Kidney Int. Rep. 4, 103–111. doi: 10.1016/j.ekir.2018.09.013
Ali, B. H., Adham, S. A., Al Za'abi, M., Waly, M. I., Yasin, J., Nemmar, A., et al. (2015). Ameliorative effect of chrysin on adenine-induced chronic kidney disease in rats. PLoS One 10:e0125285. doi: 10.1371/journal.pone.0125285
Allegra, A., Innao, V., Tartarisco, G., Pioggia, G., Casciaro, M., Musolino, C., et al. (2019). The ST2/Interleukin-33 Axis in hematologic malignancies: The IL-33 paradox. Int. J. Mol. Sci. 20:5226. doi: 10.3390/ijms20205226
Ammirati, A. L. (2020). Chronic kidney disease. Rev. Assoc. Med. Bras. 66, s03–s09. doi: 10.1590/1806-9282.66.S1.3
An, G., Zhang, X., Wang, W., Huang, Q., Li, Y., Shan, S., et al. (2018). The effects of interleukin-33 on airways collagen deposition and matrix metalloproteinase expression in a murine surrogate of asthma. Immunology 154, 637–650. doi: 10.1111/imm.12911
Antunes, M. M., Araújo, A. M., Diniz, A. B., Pereira, R. V. S., Alvarenga, D. M., David, B. A., et al. (2018). IL-33 signalling in liver immune cells enhances drug-induced liver injury and inflammation. Inflamm. Res. 67, 77–88. doi: 10.1007/s00011-017-1098-3
Baekkevold, E. S., Roussigné, M., Yamanaka, T., Johansen, F. E., Jahnsen, F. L., Amalric, F., et al. (2003). Molecular characterization of NF-HEV, a nuclear factor preferentially expressed in human high endothelial venules. Am. J. Pathol. 163, 69–79. doi: 10.1016/S0002-9440(10)63631-0
Bansal, N., Katz, R., Robinson-Cohen, C., Odden, M. C., Dalrymple, L., Shlipak, M. G., et al. (2017). Absolute rates of heart failure, coronary heart disease, and stroke in chronic kidney disease: An analysis of 3 community-based cohort studies. JAMA Cardiol. 2, 314–318. doi: 10.1001/jamacardio.2016.4652
Bao, Y. S., Na, S. P., Zhang, P., Jia, X. B., Liu, R. C., Yu, C. Y., et al. (2012). Characterization of interleukin-33 and soluble ST2 in serum and their association with disease severity in patients with chronic kidney disease. J. Clin. Immunol. 32, 587–594. doi: 10.1007/s10875-011-9622-7
Bao, K. F., Yu, X., Wei, X., Gui, L. L., Liu, H. L., Wang, X. Y., et al. (2016). Astragaloside IV ameliorates allergic inflammation by inhibiting key initiating factors in the initial stage of sensitization. Sci. Rep. 6:38241. doi: 10.1038/srep38241
Berchtold, L., Friedli, I., Vallée, J. P., Moll, S., Martin, P. Y., and de Seigneux, S. (2017). Diagnosis and assessment of renal fibrosis: the state of the art. Swiss Med. Wkly. 147:w14442. doi: 10.4414/smw.2017.14442
Bergers, G., Reikerstorfer, A., Braselmann, S., Graninger, P., and Busslinger, M. (1994). Alternative promoter usage of the Fos-responsive gene Fit-1 generates mRNA isoforms coding for either secreted or membrane-bound proteins related to the IL-1 receptor. EMBO J. 13, 1176–1188. doi: 10.1002/j.1460-2075.1994.tb06367.x
Bourgeois, E. A., Levescot, A., Diem, S., Chauvineau, A., Bergès, H., Milpied, P., et al. (2011). A natural protective function of invariant NKT cells in a mouse model of innate-cell-driven lung inflammation. Eur. J. Immunol. 41, 299–305. doi: 10.1002/eji.201040647
Cai, H., Wang, J., Mo, Y., Ye, L., Zhu, G., Song, X., et al. (2020). Salidroside suppresses group 2 innate lymphoid cell-mediated allergic airway inflammation by targeting IL-33/ST2 axis. Int. Immunopharmacol. 81:106243. doi: 10.1016/j.intimp.2020.106243
Cao, Q., Wang, Y., Niu, Z., Wang, C., Wang, R., Zhang, Z., et al. (2018). Potentiating tissue-resident type 2 innate lymphoid cells by IL-33 to prevent renal ischemia-reperfusion injury. J. Am. Soc. Nephrol. 29, 961–976. doi: 10.1681/ASN.2017070774
Cayrol, C., and Girard, J. P. (2014). IL-33: an alarmin cytokine with crucial roles in innate immunity, inflammation and allergy. Curr. Opin. Immunol. 31, 31–37. doi: 10.1016/j.coi.2014.09.004
Chackerian, A. A., Oldham, E. R., Murphy, E. E., Schmitz, J., Pflanz, S., and Kastelein, R. A. (2007). IL-1 receptor accessory protein and ST2 comprise the IL-33 receptor complex. J. Immunol. 179, 2551–2555. doi: 10.4049/jimmunol.179.4.2551
Chen, W. Y., Chang, Y. J., Su, C. H., Tsai, T. H., Chen, S. D., Hsing, C. H., et al. (2016). Upregulation of Interleukin-33 in obstructive renal injury. Biochem. Biophys. Res. Commun. 473, 1026–1032. doi: 10.1016/j.bbrc.2016.04.010
Chen, D. Q., Feng, Y. L., Chen, L., Liu, J. R., Wang, M., Vaziri, N. D., et al. (2019). Poricoic acid A enhances melatonin inhibition of AKI-to-CKD transition by regulating Gas6/AxlNFκB/Nrf2 axis. Free Radic. Biol. Med. 134, 484–497. doi: 10.1016/j.freeradbiomed.2019.01.046
Chen, J., He, Y., Tu, L., and Duan, L. (2020). Dual immune functions of IL-33 in inflammatory bowel disease. Histol. Histopathol. 35, 137–146. doi: 10.14670/HH-18-149
Chen, W. Y., Li, L. C., and Yang, J. L. (2017). Emerging roles of IL-33/ST2 Axis in renal diseases. Int. J. Mol. Sci. 18:783. doi: 10.3390/ijms18040783
Chen, L., Yang, T., Lu, D. W., Zhao, H., Feng, Y. L., Chen, H., et al. (2018a). Central role of dysregulation of TGF-β/Smad in CKD progression and potential targets of its treatment. Biomed. Pharmacother. 101, 670–681. doi: 10.1016/j.biopha.2018.02.090
Chen, W. Y., Yang, J. L., Wu, Y. H., Li, L. C., Li, R. F., Chang, Y. T., et al. (2018b). IL-33/ST2 axis mediates hyperplasia of intrarenal urothelium in obstructive renal injury. Exp. Mol. Med. 50, 1–11. doi: 10.1038/s12276-018-0047-8
Chevalier, R. L., Forbes, M. S., and Thornhill, B. A. (2009). Ureteral obstruction as a model of renal interstitial fibrosis and obstructive nephropathy. Kidney Int. 75, 1145–1152. doi: 10.1038/ki.2009.86
Cruz, D. N., Gheorghiade, M., Palazzuoli, A., Ronco, C., and Bagshaw, S. M. (2011). Epidemiology and outcome of the cardio-renal syndrome. Heart Fail. Rev. 16, 531–542. doi: 10.1007/s10741-010-9223-1
Davidson, A. (2016). What is damaging the kidney in lupus nephritis? Nat. Rev. Rheumatol. 12, 143–153. doi: 10.1038/nrrheum.2015.159
de Boer, R. A., Daniels, L. B., Maisel, A. S., and Januzzi, J. L. Jr. (2015). State of the art: newer biomarkers in heart failure. Eur. J. Heart Fail. 17, 559–569. doi: 10.1002/ejhf.273
De la Fuente, M., MacDonald, T. T., and Hermoso, M. A. (2015). The IL-33/ST2 axis: role in health and disease. Cytokine Growth Factor Rev. 26, 615–623. doi: 10.1016/j.cytogfr.2015.07.017
de Zeeuw, D., Ramjit, D., Zhang, Z., Ribeiro, A. B., Kurokawa, K., Lash, J. P., et al. (2006). Renal risk and renoprotection among ethnic groups with type 2 diabetic nephropathy: a post hoc analysis of RENAAL. Kidney Int. 69, 1675–1682. doi: 10.1038/sj.ki.5000326
Di Salvo, E., Ventura-Spagnolo, E., Casciaro, M., Navarra, M., and Gangemi, S. (2018). IL-33/IL-31 Axis: A potential inflammatory pathway. Mediat. Inflamm. 2018:3858032. doi: 10.1155/2018/3858032
Dieplinger, B., Egger, M., Leitner, I., Firlinger, F., Poelz, W., Lenz, K., et al. (2016). Interleukin 6, galectin 3, growth differentiation factor 15, and soluble ST2 for mortality prediction in critically ill patients. J. Crit. Care 34, 38–45. doi: 10.1016/j.jcrc.2016.03.020
Dinarello, C. A. (2005). An IL-1 family member requires caspase-1 processing and signals through the ST2 receptor. Immunity 23, 461–462. doi: 10.1016/j.immuni.2005.10.004
Djudjaj, S., and Boor, P. (2019). Cellular and molecular mechanisms of kidney fibrosis. Mol. Asp. Med. 65, 16–36. doi: 10.1016/j.mam.2018.06.002
Drake, L. Y., and Kita, H. (2017). IL-33: biological properties, functions, and roles in airway disease. Immunol. Rev. 278, 173–184. doi: 10.1111/imr.12552
Drake, L. Y., and Prakash, Y. S. (2020). Contributions of IL-33 in non-hematopoietic lung cells to obstructive lung disease. Front. Immunol. 11:1798. doi: 10.3389/fimmu.2020.01798
Duan, L., Huang, Y., Su, Q., Lin, Q., Liu, W., Luo, J., et al. (2016). Potential of IL-33 for preventing the kidney injury via regulating the lipid metabolism in gout patients. J. Diabetes Res. 2016:1028401. doi: 10.1155/2016/1028401
Elsherbiny, N. M., Said, E., Atef, H., and Zaitone, S. A. (2020). Renoprotective effect of calycosin in high fat diet-fed/STZ injected rats: effect on IL-33/ST2 signaling, oxidative stress and fibrosis suppression. Chem. Biol. Interact. 315:108897. doi: 10.1016/j.cbi.2019.108897
Fanny, M., Nascimento, M., Baron, L., Schricke, C., Maillet, I., Akbal, M., et al. (2018). The IL-33 receptor ST2 regulates pulmonary inflammation and fibrosis to bleomycin. Front. Immunol. 9:1476. doi: 10.3389/fimmu.2018.01476
Ferhat, M., Robin, A., Giraud, S., Sena, S., Goujon, J. M., Touchard, G., et al. (2018). Endogenous IL-33 contributes to kidney ischemia-reperfusion injury as an Alarmin. J. Am. Soc. Nephrol. 29, 1272–1288. doi: 10.1681/ASN.2017060650
Fertin, C., Nicolas, J. F., Gillery, P., Kalis, B., Banchereau, J., and Maquart, F. X. (1991). Interleukin-4 stimulates collagen synthesis by normal and scleroderma fibroblasts in dermal equivalents. Cell. Mol. Biol. 37, 823–829.
Funakoshi-Tago, M., Tago, K., Sato, Y., Tominaga, S., and Kasahara, T. (2011). JAK2 is an important signal transducer in IL-33-induced NF-κB activation. Cell. Signal. 23, 363–370. doi: 10.1016/j.cellsig.2010.10.006
Gao, Q., Li, Y., and Li, M. (2015). The potential role of IL-33/ST2 signaling in fibrotic diseases. J. Leukoc. Biol. 98, 15–22. doi: 10.1189/jlb.3RU0115-012R
Gatti, F., Mia, S., Hammarström, C., Frerker, N., Fosby, B., Wang, J., et al. (2021). Nuclear IL-33 restrains the early conversion of fibroblasts to an extracellular matrix-secreting phenotype. Sci. Rep. 11:108. doi: 10.1038/s41598-020-80509-5
Ghali, R., Habeichi, N. J., Kaplan, A., Tannous, C., Abidi, E., Bekdash, A., et al. (2020). IL-33 induces type-2-cytokine phenotype but exacerbates cardiac remodeling post-myocardial infarction with eosinophil recruitment, worsened systolic dysfunction, and ventricular wall rupture. Clin. Sci. 134, 1191–1218. doi: 10.1042/CS20200402
Griesenauer, B., and Paczesny, S. (2017). The ST2/IL-33 Axis in immune cells during inflammatory diseases. Front. Immunol. 8:475. doi: 10.3389/fimmu.2017.00475
Gu, L. F., Ge, H. T., Zhao, L., Wang, Y. J., Zhang, F., Tang, H. T., et al. (2020b). Huangkui capsule ameliorates renal fibrosis in a unilateral ureteral obstruction mouse model Through TRPC6 dependent Signaling pathways. Front. Pharmacol. 11:996. doi: 10.3389/fphar.2020.00996
Gu, L., Hong, F., Fan, K., Zhao, L., Zhang, C., Yu, B., et al. (2020a). Integrated network pharmacology analysis and pharmacological evaluation to explore the active components and mechanism of Abelmoschus manihot (L.) Medik. On renal fibrosis. Drug Des. Devel. Ther. 14, 4053–4067. doi: 10.2147/dddt.S264898
Gungor, O., Unal, H. U., Guclu, A., Gezer, M., Eyileten, T., Guzel, F. B., et al. (2017). IL-33 and ST2 levels in chronic kidney disease: associations with inflammation, vascular abnormalities, cardiovascular events, and survival. PLoS One 12:e0178939. doi: 10.1371/journal.pone.0178939
Hansel, T. T., Kropshofer, H., Singer, T., Mitchell, J. A., and George, A. J. (2010). The safety and side effects of monoclonal antibodies. Nat. Rev. Drug Discov. 9, 325–338. doi: 10.1038/nrd3003
Henderson, N. C., Rieder, F., and Wynn, T. A. (2020). Fibrosis: from mechanisms to medicines. Nature 587, 555–566. doi: 10.1038/s41586-020-2938-9
Hewitson, T. D. (2009). Renal tubulointerstitial fibrosis: common but never simple. Am. J. Physiol. Ren. Physiol. 296, F1239–F1244. doi: 10.1152/ajprenal.90521.2008
Ho, J. E., Hwang, S. J., Wollert, K. C., Larson, M. G., Cheng, S., Kempf, T., et al. (2013). Biomarkers of cardiovascular stress and incident chronic kidney disease. Clin. Chem. 59, 1613–1620. doi: 10.1373/clinchem.2013.205716
Homsak, E., and Gruson, D. (2020). Soluble ST2: A complex and diverse role in several diseases. Clin. Chim. Acta 507, 75–87. doi: 10.1016/j.cca.2020.04.011
Hong, J., Kim, S., and Lin, P. C. (2019). Interleukin-33 and ST2 Signaling in tumor microenvironment. J. Interf. Cytokine Res. 39, 61–71. doi: 10.1089/jir.2018.0044
Humphreys, B. D. (2018). Mechanisms of renal fibrosis. Annu. Rev. Physiol. 80, 309–326. doi: 10.1146/annurev-physiol-022516-034227
Imai, J., Kitamoto, S., Sugihara, K., Nagao-Kitamoto, H., Hayashi, A., Morhardt, T. L., et al. (2019). Flagellin-mediated activation of IL-33-ST2 signaling by a pathobiont promotes intestinal fibrosis. Mucosal Immunol. 12, 632–643. doi: 10.1038/s41385-019-0138-4
Iwahana, H., Hayakawa, M., Kuroiwa, K., Tago, K., Yanagisawa, K., Noji, S., et al. (2004). Molecular cloning of the chicken ST2 gene and a novel variant form of the ST2 gene product, ST2LV. Biochim. Biophys. Acta 1681, 1–14. doi: 10.1016/j.bbaexp.2004.08.013
Jiang, W., Lian, J., Yue, Y., and Zhang, Y. (2021). IL-33/ST2 as a potential target for tumor immunotherapy. Eur. J. Immunol. 51, 1943–1955. doi: 10.1002/eji.202149175
Kakkar, R., and Lee, R. T. (2008). The IL-33/ST2 pathway: therapeutic target and novel biomarker. Nat. Rev. Drug Discov. 7, 827–840. doi: 10.1038/nrd2660
Kandemir, F. M., Yildirim, S., Kucukler, S., Caglayan, C., Mahamadu, A., and Dortbudak, M. B. (2018). Therapeutic efficacy of zingerone against vancomycin-induced oxidative stress, inflammation, apoptosis and aquaporin 1 permeability in rat kidney. Biomed. Pharmacother. 105, 981–991. doi: 10.1016/j.biopha.2018.06.048
Kaur, D., Chachi, L., Gomez, E., Sylvius, N., Singh, S. R., Ramsheh, M. Y., et al. (2020). ST2 expression and release by the bronchial epithelium is downregulated in asthma. Allergy 75, 3184–3194. doi: 10.1111/all.14436
Kaur, D., Gomez, E., Doe, C., Berair, R., Woodman, L., Saunders, R., et al. (2015). IL-33 drives airway hyper-responsiveness through IL-13-mediated mast cell: airway smooth muscle crosstalk. Allergy 70, 556–567. doi: 10.1111/all.12593
Kim, M. G., Kim, S. C., Ko, Y. S., Lee, H. Y., Jo, S. K., and Cho, W. (2015). The role of M2 macrophages in the progression of chronic kidney disease following acute kidney injury. PLoS One 10:e0143961. doi: 10.1371/journal.pone.0143961
Kotsiou, O. S., Gourgoulianis, K. I., and Zarogiannis, S. G. (2018). IL-33/ST2 Axis in organ fibrosis. Front. Immunol. 9:2432. doi: 10.3389/fimmu.2018.02432
Kucukler, S., Benzer, F., Yildirim, S., Gur, C., Kandemir, F. M., Bengu, A. S., et al. (2021). Protective effects of Chrysin Against oxidative stress and inflammation induced by Lead acetate in rat kidneys: a biochemical and histopathological approach. Biol. Trace Elem. Res. 199, 1501–1514. doi: 10.1007/s12011-020-02268-8
Larsen, K. M., Minaya, M. K., Vaish, V., and Peña, M. M. O. (2018). The role of IL-33/ST2 pathway in tumorigenesis. Int. J. Mol. Sci. 19:2676. doi: 10.3390/ijms19092676
Li, D., Guabiraba, R., Besnard, A. G., Komai-Koma, M., Jabir, M. S., Zhang, L., et al. (2014a). IL-33 promotes ST2-dependent lung fibrosis by the induction of alternatively activated macrophages and innate lymphoid cells in mice. J. Allergy Clin. Immunol. 134, 1422–1432.e1411. doi: 10.1016/j.jaci.2014.05.011
Li, R., Guo, Y., Zhang, Y., Zhang, X., Zhu, L., and Yan, T. (2019a). Salidroside ameliorates renal interstitial fibrosis by inhibiting the TLR4/NF-κB and MAPK Signaling pathways. Int. J. Mol. Sci. 20:1103. doi: 10.3390/ijms20051103
Li, P., Lin, W., and Zheng, X. (2014b). IL-33 neutralization suppresses lupus disease in lupus-prone mice. Inflammation 37, 824–832. doi: 10.1007/s10753-013-9802-0
Li, Y., Liu, J., Yu, T., Yan, B., and Li, H. (2019b). Interleukin-33 promotes obstructive renal injury via macrophages. Mol. Med. Rep. 20, 1353–1362. doi: 10.3892/mmr.2019.10324
Li, H., Tago, K., Io, K., Kuroiwa, K., Arai, T., Iwahana, H., et al. (2000). The cloning and nucleotide sequence of human ST2L cDNA. Genomics 67, 284–290. doi: 10.1006/geno.2000.6269
Li, L., Zhu, H., and Zuo, X. (2018). Interleukin-33 in systemic sclerosis: expression and pathogenesis. Front. Immunol. 9:2663. doi: 10.3389/fimmu.2018.02663
Liang, H., Xu, F., Wen, X. J., Liu, H. Z., Wang, H. B., Zhong, J. Y., et al. (2017). Interleukin-33 signaling contributes to renal fibrosis following ischemia reperfusion. Eur. J. Pharmacol. 812, 18–27. doi: 10.1016/j.ejphar.2017.06.031
Liao, Y., Tan, R. Z., Li, J. C., Liu, T. T., Zhong, X., Yan, Y., et al. (2020). Isoliquiritigenin attenuates UUO-induced renal inflammation and fibrosis by inhibiting Mincle/Syk/NF-kappa B Signaling pathway. Drug Des. Devel. Ther. 14, 1455–1468. doi: 10.2147/dddt.S243420
Liew, F. Y., Girard, J. P., and Turnquist, H. R. (2016). Interleukin-33 in health and disease. Nat. Rev. Immunol. 16, 676–689. doi: 10.1038/nri.2016.95
Liu, X., Huang, S., Wang, F., Zheng, L., Lu, J., Chen, J., et al. (2019c). Huangqi-Danshen decoction ameliorates adenine-induced chronic kidney disease by modulating mitochondrial dynamics. Evid. Based Complement. Alternat. Med. 2019:9574045. doi: 10.1155/2019/9574045
Liu, L., Kou, P., Zeng, Q., Pei, G., Li, Y., Liang, H., et al. (2012). CD4+ T lymphocytes, especially Th2 cells, contribute to the progress of renal fibrosis. Am. J. Nephrol. 36, 386–396. doi: 10.1159/000343283
Liu, L., and Li, Y. (2014). The unexpected side effects and safety of therapeutic monoclonal antibodies. Drugs Today 50, 33–50. doi: 10.1358/dot.2014.50.1.2076506
Liu, C., Qin, L., Ding, J., Zhou, L., Gao, C., Zhang, T., et al. (2019a). Group 2 innate lymphoid cells participate in renal fibrosis in diabetic kidney disease partly via TGF-β1 signal pathway. J. Diabetes Res. 2019:8512028. doi: 10.1155/2019/8512028
Liu, Y., Shi, G., Yee, H., Wang, W., Han, W., Liu, B., et al. (2019e). Shenkang injection, a modern preparation of Chinese patent medicine, diminishes tubulointerstitial fibrosis in obstructive nephropathy via targeting pericyte-myofibroblast transition. Am. J. Transl. Res. 11, 1980–1996.
Liu, X., Xiao, Y., Pan, Y., Li, H., Zheng, S. G., and Su, W. (2019d). The role of the IL-33/ST2 axis in autoimmune disorders: friend or foe? Cytokine Growth Factor Rev. 50, 60–74. doi: 10.1016/j.cytogfr.2019.04.004
Liu, J., Yang, Y., Zheng, C., Chen, G., Shen, Z., Zheng, S., et al. (2019b). Correlation of Interleukin-33/ST2 receptor and liver fibrosis progression in biliary atresia patients. Front. Pediatr. 7:403. doi: 10.3389/fped.2019.00403
Loboda, A., Sobczak, M., Jozkowicz, A., and Dulak, J. (2016). TGF-β1/Smads and miR-21 in renal fibrosis and inflammation. Mediat. Inflamm. 2016:8319283. doi: 10.1155/2016/8319283
Lu, J., Kang, J., Zhang, C., and Zhang, X. (2015). The role of IL-33/ST2L signals in the immune cells. Immunol. Lett. 164, 11–17. doi: 10.1016/j.imlet.2015.01.008
Lukic, R., Cupic, M., Gajovic, N., Jurisevic, M., Mijailovic, Z., Davidovic, B., et al. (2020). Increased systemic sST2 in patients with end stage renal disease contributes to milder liver damage during HCV infection. J. Infect. Dev. Ctries. 14, 519–526. doi: 10.3855/jidc.11741
Lv, W., Booz, G. W., Wang, Y., Fan, F., and Roman, R. J. (2018). Inflammation and renal fibrosis: recent developments on key signaling molecules as potential therapeutic targets. Eur. J. Pharmacol. 820, 65–76. doi: 10.1016/j.ejphar.2017.12.016
Manetti, M., Ibba-Manneschi, L., Liakouli, V., Guiducci, S., Milia, A. F., Benelli, G., et al. (2010). The IL1-like cytokine IL33 and its receptor ST2 are abnormally expressed in the affected skin and visceral organs of patients with systemic sclerosis. Ann. Rheum. Dis. 69, 598–605. doi: 10.1136/ard.2009.119321
Martin, N. T., and Martin, M. U. (2016). Interleukin 33 is a guardian of barriers and a local alarmin. Nat. Immunol. 17, 122–131. doi: 10.1038/ni.3370
Martínez-Klimova, E., Aparicio-Trejo, O. E., Gómez-Sierra, T., Jiménez-Uribe, A. P., Bellido, B., and Pedraza-Chaverri, J. (2020). Mitochondrial dysfunction and endoplasmic reticulum stress in the promotion of fibrosis in obstructive nephropathy induced by unilateral ureteral obstruction. Biofactors 46, 716–733. doi: 10.1002/biof.1673
Martínez-Klimova, E., Aparicio-Trejo, O. E., Tapia, E., and Pedraza-Chaverri, J. (2019). Unilateral ureteral obstruction as a model to investigate fibrosis-attenuating treatments. Biomol. Ther. 9:141. doi: 10.3390/biom9040141
Marvie, P., Lisbonne, M., L'Helgoualc'h, A., Rauch, M., Turlin, B., Preisser, L., et al. (2010). Interleukin-33 overexpression is associated with liver fibrosis in mice and humans. J. Cell. Mol. Med. 14, 1726–1739. doi: 10.1111/j.1582-4934.2009.00801.x
McClellan, W. M., and Flanders, W. D. (2003). Risk factors for progressive chronic kidney disease. J. Am. Soc. Nephrol. 14, S65–S70. doi: 10.1097/01.ASN.0000070147.10399.9E
Meng, X. M., Nikolic-Paterson, D. J., and Lan, H. Y. (2014). Inflammatory processes in renal fibrosis. Nat. Rev. Nephrol. 10, 493–503. doi: 10.1038/nrneph.2014.114
Meng, X. M., Tang, P. M., Li, J., and Lan, H. Y. (2015). TGF-β/Smad signaling in renal fibrosis. Front. Physiol. 6:82. doi: 10.3389/fphys.2015.00082
Milovanovic, M., Volarevic, V., Radosavljevic, G., Jovanovic, I., Pejnovic, N., Arsenijevic, N., et al. (2012). IL-33/ST2 axis in inflammation and immunopathology. Immunol. Res. 52, 89–99. doi: 10.1007/s12026-012-8283-9
Molofsky, A. B., Savage, A. K., and Locksley, R. M. (2015). Interleukin-33 in tissue homeostasis, injury, and inflammation. Immunity 42, 1005–1019. doi: 10.1016/j.immuni.2015.06.006
Mosser, D. M., and Edwards, J. P. (2008). Exploring the full spectrum of macrophage activation. Nat. Rev. Immunol. 8, 958–969. doi: 10.1038/nri2448
Moussion, C., Ortega, N., and Girard, J. P. (2008). The IL-1-like cytokine IL-33 is constitutively expressed in the nucleus of endothelial cells and epithelial cells in vivo: a novel 'alarmin'? PLoS One 3:e3331. doi: 10.1371/journal.pone.0003331
Nagashima, R., and Iyoda, M. (2021). The roles of kidney-resident ILC2 in renal inflammation and fibrosis. Front. Immunol. 12:688647. doi: 10.3389/fimmu.2021.688647
Nogueira, A., Pires, M. J., and Oliveira, P. A. (2017). Pathophysiological mechanisms of renal fibrosis: A review of animal models and therapeutic strategies. In Vivo 31, 1–22. doi: 10.21873/invivo.11019
Onda, H., Kasuya, H., Takakura, K., Hori, T., Imaizumi, T., Takeuchi, T., et al. (1999). Identification of genes differentially expressed in canine vasospastic cerebral arteries after subarachnoid hemorrhage. J. Cereb. Blood Flow Metab. 19, 1279–1288. doi: 10.1097/00004647-199911000-00013
Onk, D., Onk, O. A., Turkmen, K., Erol, H. S., Ayazoglu, T. A., Keles, O. N., et al. (2016). Melatonin attenuates contrast-induced nephropathy in diabetic rats: The role of Interleukin-33 and oxidative stress. Mediat. Inflamm. 2016:9050828. doi: 10.1155/2016/9050828
Palazzuoli, A., McCullough, P. A., Ronco, C., and Nuti, R. (2015). Kidney disease in heart failure: the importance of novel biomarkers for type 1 cardio-renal syndrome detection. Intern. Emerg. Med. 10, 543–554. doi: 10.1007/s11739-015-1246-0
Ponticelli, C., and Locatelli, F. (2018). Glucocorticoids in the treatment of glomerular diseases: pitfalls and pearls. Clin. J. Am. Soc. Nephrol. 13, 815–822. doi: 10.2215/cjn.12991117
Prakoura, N., Hadchouel, J., and Chatziantoniou, C. (2019). Novel targets for therapy of renal fibrosis. J. Histochem. Cytochem. 67, 701–715. doi: 10.1369/0022155419849386
Rankin, A. L., Mumm, J. B., Murphy, E., Turner, S., Yu, N., McClanahan, T. K., et al. (2010). IL-33 induces IL-13-dependent cutaneous fibrosis. J. Immunol. 184, 1526–1535. doi: 10.4049/jimmunol.0903306
Riedel, J. H., Becker, M., Kopp, K., Düster, M., Brix, S. R., Meyer-Schwesinger, C., et al. (2017). IL-33-mediated expansion of type 2 innate lymphoid cells protects from progressive glomerulosclerosis. J. Am. Soc. Nephrol. 28, 2068–2080. doi: 10.1681/ASN.2016080877
Ronco, C., McCullough, P., Anker, S. D., Anand, I., Aspromonte, N., Bagshaw, S. M., et al. (2010). Cardio-renal syndromes: report from the consensus conference of the acute dialysis quality initiative. Eur. Heart J. 31, 703–711. doi: 10.1093/eurheartj/ehp507
Rossi, M., Korpak, K., Doerfler, A., and Zouaoui Boudjeltia, K. (2021). Deciphering the role of Heme Oxygenase-1 (HO-1) expressing macrophages in renal ischemia-reperfusion injury. Biomedicine 9:306. doi: 10.3390/biomedicines9030306
Ruggenenti, P., Perna, A., Gherardi, G., Garini, G., Zoccali, C., Salvadori, M., et al. (1999). Renoprotective properties of ACE-inhibition in non-diabetic nephropathies with non-nephrotic proteinuria. Lancet 354, 359–364. doi: 10.1016/S0140-6736(98)10363-X
Sabapathy, V., Cheru, N. T., Corey, R., Mohammad, S., and Sharma, R. (2019). A novel hybrid cytokine IL233 mediates regeneration following doxorubicin-induced nephrotoxic injury. Sci. Rep. 9:3215. doi: 10.1038/s41598-019-39886-9
Sanada, S., Hakuno, D., Higgins, L. J., Schreiter, E. R., McKenzie, A. N., and Lee, R. T. (2007). IL-33 and ST2 comprise a critical biomechanically induced and cardioprotective signaling system. J. Clin. Invest. 117, 1538–1549. doi: 10.1172/JCI30634
Schmitz, J., Owyang, A., Oldham, E., Song, Y., Murphy, E., McClanahan, T. K., et al. (2005). IL-33, an interleukin-1-like cytokine that signals via the IL-1 receptor-related protein ST2 and induces T helper type 2-associated cytokines. Immunity 23, 479–490. doi: 10.1016/j.immuni.2005.09.015
Seo, S. M., Kim, S. H., Kim, Y., Yoon, H. E., and Shin, S. J. (2018). Prognostic utility of soluble suppression of Tumorigenicity 2 level as a predictor of clinical outcomes in incident Hemodialysis patients. Int. J. Med. Sci. 15, 730–737. doi: 10.7150/ijms.23638
Shen, Y. L., Jiang, Y. P., Li, X. Q., Wang, S. J., Ma, M. H., Zhang, C. Y., et al. (2019). ErHuang formula improves renal fibrosis in diabetic nephropathy rats by inhibiting CXCL6/JAK/STAT3 Signaling pathway. Front. Pharmacol. 10:1596. doi: 10.3389/fphar.2019.01596
Shen, J. X., Liu, J., and Zhang, G. J. (2018). Interleukin-33 in Malignancies: Friends or Foes? Front. Immunol. 9:3051. doi: 10.3389/fimmu.2018.03051
Sun, Z., Chang, B., Gao, M., Zhang, J., and Zou, Z. (2017). IL-33-ST2 Axis in liver disease: progression and challenge. Mediat. Inflamm. 2017:5314213. doi: 10.1155/2017/5314213
Takeda, T., Morita, H., Saito, H., Matsumoto, K., and Matsuda, A. (2018). Recent advances in understanding the roles of blood platelets in the pathogenesis of allergic inflammation and bronchial asthma. Allergol. Int. 67, 326–333. doi: 10.1016/j.alit.2017.11.008
Tan, Z., Liu, Q., Jiang, R., Lv, L., Shoto, S. S., Maillet, I., et al. (2018). Interleukin-33 drives hepatic fibrosis through activation of hepatic stellate cells. Cell. Mol. Immunol. 15, 388–398. doi: 10.1038/cmi.2016.63
Thierry, A., Giraud, S., Robin, A., Barra, A., Bridoux, F., Ameteau, V., et al. (2014). The alarmin concept applied to human renal transplantation: evidence for a differential implication of HMGB1 and IL-33. PLoS One 9:e88742. doi: 10.1371/journal.pone.0088742
Tonacci, A., Quattrocchi, P., and Gangemi, S. (2019). IL33/ST2 Axis in diabetic kidney disease: A literature review. Medicina 55:50. doi: 10.3390/medicina55020050
Tseng, C. C. S., Huibers, M. M. H., van Kuik, J., de Weger, R. A., Vink, A., and de Jonge, N. (2018). The Interleukin-33/ST2 pathway is expressed in the failing human heart and associated with pro-fibrotic Remodeling of the myocardium. J. Cardiovasc. Transl. Res. 11, 15–21. doi: 10.1007/s12265-017-9775-8
Tuegel, C., Katz, R., Alam, M., Bhat, Z., Bellovich, K., de Boer, I., et al. (2018). GDF-15, galectin 3, soluble ST2, and risk of mortality and cardiovascular events in CKD. Am. J. Kidney Dis. 72, 519–528. doi: 10.1053/j.ajkd.2018.03.025
Vandewalle, J., Luypaert, A., De Bosscher, K., and Libert, C. (2018). Therapeutic mechanisms of glucocorticoids. Trends Endocrinol. Metab. 29, 42–54. doi: 10.1016/j.tem.2017.10.010
Wang, X., Gao, Y., Tian, N., Wang, T., Shi, Y., Xu, J., et al. (2019b). Astragaloside IV inhibits glucose-induced epithelial-mesenchymal transition of podocytes through autophagy enhancement via the SIRT-NF-κB p65 axis. Sci. Rep. 9:323. doi: 10.1038/s41598-018-36911-1
Wang, J., Sun, L., Nie, Y., Duan, S., Zhang, T., Wang, W., et al. (2020). Protein kinase C δ (PKCδ) attenuates bleomycin induced pulmonary fibrosis via inhibiting NF-κB Signaling pathway. Front. Physiol. 11:367. doi: 10.3389/fphys.2020.00367
Wang, M., Yang, L., Yang, J., and Wang, C. (2019a). Shen Shuai IIRecipe attenuates renal injury and fibrosis in chronic kidney disease by regulating NLRP3 inflammasome and Sirt1/Smad3 deacetylation pathway. BMC Complement. Altern. Med. 19:107. doi: 10.1186/s12906-019-2524-6
Weinberg, E. O., Shimpo, M., De Keulenaer, G. W., MacGillivray, C., Tominaga, S., Solomon, S. D., et al. (2002). Expression and regulation of ST2, an interleukin-1 receptor family member, in cardiomyocytes and myocardial infarction. Circulation 106, 2961–2966. doi: 10.1161/01.cir.0000038705.69871.d9
Weiskirchen, R., Weiskirchen, S., and Tacke, F. (2019). Organ and tissue fibrosis: molecular signals, cellular mechanisms and translational implications. Mol. Asp. Med. 65, 2–15. doi: 10.1016/j.mam.2018.06.003
Willems, S., Hoefer, I., and Pasterkamp, G. (2012). The role of the interleukin 1 receptor-like 1 (ST2) and Interleukin-33 pathway in cardiovascular disease and cardiovascular risk assessment. Minerva Med. 103, 513–524.
Wu, X. M., Gao, Y. B., Xu, L. P., Zou, D. W., Zhu, Z. Y., Wang, X. L., et al. (2017). Tongxinluo inhibits renal fibrosis in diabetic nephropathy: involvement of the suppression of intercellular transfer of TGF-[formula: see text]1-containing exosomes from GECs to GMCs. Am. J. Chin. Med. 45, 1075–1092. doi: 10.1142/S0192415X17500586
Wu, L., Luo, Z., Zheng, J., Yao, P., Yuan, Z., Lv, X., et al. (2018). IL-33 can promote the process of pulmonary fibrosis by inducing the imbalance Between MMP-9 and TIMP-1. Inflammation 41, 878–885. doi: 10.1007/s10753-018-0742-6
Wynn, T. A. (2003). IL-13 effector functions. Annu. Rev. Immunol. 21, 425–456. doi: 10.1146/annurev.immunol.21.120601.141142
Xia, C. H., Han, X. T., Zhang, X., Zhu, Z. B., Guo, J., Cui, H. L., et al. (2019). Yiqihuoxue formula activates autophagy and offers renoprotection in a rat model of adenine-induced kidney disease. Evid. Based Complement. Alternat. Med. 2019:3423981. doi: 10.1155/2019/3423981
Xiang, N., Liao, H., Zhai, Z., and Gong, J. (2021). Expression and significance of inflammatory reactions mediated by the IL-33/ST2 signaling pathway in the serum of heart failure patients. Am. J. Transl. Res. 13, 8247–8252.
Xu, H., Turnquist, H. R., Hoffman, R., and Billiar, T. R. (2017). Role of the IL-33-ST2 axis in sepsis. Mil. Med. Res. 4:3. doi: 10.1186/s40779-017-0115-8
Xu, Z., Zhao, C., Wang, Z., Tao, J., Han, Z., Zhang, W., et al. (2018). Interleukin-33 levels are elevated in chronic allograft dysfunction of kidney transplant recipients and promotes epithelial to mesenchymal transition of human kidney (HK-2) cells. Gene 644, 113–121. doi: 10.1016/j.gene.2017.11.010
Xu, J., Zheng, J., Song, P., Zhou, Y., and Guan, S. (2016). IL-33/ST2 pathway in a bleomycin-induced pulmonary fibrosis model. Mol. Med. Rep. 14, 1704–1708. doi: 10.3892/mmr.2016.5446
Yan, C., Yu, L., Zhang, X. L., Shang, J. J., Ren, J., Fan, J., et al. (2020). Cytokine profiling in Chinese SLE patients: correlations with renal dysfunction. J Immunol. Res. 2020:8146502. doi: 10.1155/2020/8146502
Yang, Q., Kong, L., Huang, W., Mohammadtursun, N., Li, X., Wang, G., et al. (2020). Osthole attenuates ovalbumin-induced lung inflammation via the inhibition of IL-33/ST2 signaling in asthmatic mice. Int. J. Mol. Med. 46, 1389–1398. doi: 10.3892/ijmm.2020.4682
Yang, F., Zhu, P., Duan, L., Yang, L., and Wang, J. (2016). IL-33 and kidney disease (review). Mol. Med. Rep. 13, 3–8. doi: 10.3892/mmr.2015.4516
Yu, M. Y., Kwon, S., Moon, J. J., Kim, Y. C., Song, E. Y., Lee, H., et al. (2021). Role of the IL-33/ST2 pathway in renal allograft rejection. Exp. Cell Res. 405:112705. doi: 10.1016/j.yexcr.2021.112705
Zhang, S., Huang, Q., Cai, X., Jiang, S., Xu, N., Zhou, Q., et al. (2018). Osthole ameliorates renal fibrosis in mice by suppressing fibroblast activation and epithelial-mesenchymal transition. Front. Physiol. 9:1650. doi: 10.3389/fphys.2018.01650
Zhang, Y. Y., Tan, R. Z., Zhang, X. Q., Yu, Y., and Yu, C. (2019). Calycosin ameliorates diabetes-induced renal inflammation via the NF-κB pathway in vitro and in vivo. Med. Sci. Monit. 25, 1671–1678. doi: 10.12659/MSM.915242
Zhang, H. F., Xie, S. L., Chen, Y. X., Mai, J. T., Wang, J. F., Zhu, W. L., et al. (2012). Altered serum levels of IL-33 in patients with advanced systolic chronic heart failure: correlation with oxidative stress. J. Transl. Med. 10:120. doi: 10.1186/1479-5876-10-120
Zhang, M., and Zhang, S. (2020). T cells in fibrosis and fibrotic diseases. Front. Immunol. 11:1142. doi: 10.3389/fimmu.2020.01142
Zhang, Y., Zhu, X., Huang, X., Wei, X., Zhao, D., Jiang, L., et al. (2020). Advances in understanding the effects of erythropoietin on renal fibrosis. Front. Med. 7:47. doi: 10.3389/fmed.2020.00047
Zhao, Y. Y., Wang, H. L., Cheng, X. L., Wei, F., Bai, X., Lin, R. C., et al. (2015). Metabolomics analysis reveals the association between lipid abnormalities and oxidative stress, inflammation, fibrosis, and Nrf2 dysfunction in aristolochic acid-induced nephropathy. Sci. Rep. 5:12936. doi: 10.1038/srep12936
Zhu, F., Bai, X., Hong, Q., Cui, S., Wang, X., Xiao, F., et al. (2019). STAT3 inhibition partly abolishes IL-33-induced bone marrow-derived monocyte phenotypic transition into fibroblast precursor and alleviates experimental renal interstitial fibrosis. J. Immunol. 203, 2644–2654. doi: 10.4049/jimmunol.1801273
Keywords: renal fibrosis, chronic kidney disease, IL-33, ST2, mechanisms
Citation: Tan X-Y, Jing H-Y and Ma Y-R (2022) Interleukin-33/ Suppression of Tumorigenicity 2 in Renal Fibrosis: Emerging Roles in Prognosis and Treatment. Front. Physiol. 12:792897. doi: 10.3389/fphys.2021.792897
Received: 13 October 2021; Accepted: 02 December 2021;
Published: 03 January 2022.
Edited by:
James A. McCormick, Oregon Health and Science University, United StatesReviewed by:
Stelios Psarras, Biomedical Research Foundation of the Academy of Athens (BRFAA), GreeceCopyright © 2022 Tan, Jing and Ma. This is an open-access article distributed under the terms of the Creative Commons Attribution License (CC BY). The use, distribution or reproduction in other forums is permitted, provided the original author(s) and the copyright owner(s) are credited and that the original publication in this journal is cited, in accordance with accepted academic practice. No use, distribution or reproduction is permitted which does not comply with these terms.
*Correspondence: Yue-Rong Ma, bWF5cjY2NkAxNjMuY29t
†These authors have contributed equally to this work
Disclaimer: All claims expressed in this article are solely those of the authors and do not necessarily represent those of their affiliated organizations, or those of the publisher, the editors and the reviewers. Any product that may be evaluated in this article or claim that may be made by its manufacturer is not guaranteed or endorsed by the publisher.
Research integrity at Frontiers
Learn more about the work of our research integrity team to safeguard the quality of each article we publish.