- 1Department of Internal Medicine, Amsterdam University Medical Center, University of Amsterdam, Amsterdam, Netherlands
- 2Department of Anesthesiology, Amsterdam University Medical Center, University of Amsterdam, Amsterdam, Netherlands
- 3Laboratory for Clinical Cardiovascular Physiology, Amsterdam University Medical Center, University of Amsterdam, Amsterdam, Netherlands
- 4Department of Internal Medicine, Medisch Centrum Leeuwarden, Leeuwarden, Netherlands
- 5Department of Pulmonary Medicine, Amsterdam University Medical Center, Vrije Universiteit Amsterdam, Amsterdam Cardiovascular Sciences, Amsterdam, Netherlands
- 6Medical Research Council Versus Arthritis Centre for Musculoskeletal Ageing Research, Division of Physiology, Pharmacology and Neuroscience, School of Life Sciences, The Medical School, University of Nottingham Medical School, Queen's Medical Centre, Nottingham, United Kingdom
The first step to exercise is preceded by the required assumption of the upright body position, which itself involves physical activity. The gravitational displacement of blood from the chest to the lower parts of the body elicits a fall in central blood volume (CBV), which corresponds to the fraction of thoracic blood volume directly available to the left ventricle. The reduction in CBV and stroke volume (SV) in response to postural stress, post-exercise, or to blood loss results in reduced left ventricular filling, which may manifest as orthostatic intolerance. When termination of exercise removes the leg muscle pump function, CBV is no longer maintained. The resulting imbalance between a reduced cardiac output (CO) and a still enhanced peripheral vascular conductance may provoke post-exercise hypotension (PEH). Instruments that quantify CBV are not readily available and to express which magnitude of the CBV in a healthy subject should remains difficult. In the physiological laboratory, the CBV can be modified by making use of postural stressors, such as lower body “negative” or sub-atmospheric pressure (LBNP) or passive head-up tilt (HUT), while quantifying relevant biomedical parameters of blood flow and oxygenation. Several approaches, such as wearable sensors and advanced machine-learning techniques, have been followed in an attempt to improve methodologies for better prediction of outcomes and to guide treatment in civil patients and on the battlefield. In the recent decade, efforts have been made to develop algorithms and apply artificial intelligence (AI) in the field of hemodynamic monitoring. Advances in quantifying and monitoring CBV during environmental stress from exercise to hemorrhage and understanding the analogy between postural stress and central hypovolemia during anesthesia offer great relevance for healthy subjects and clinical populations.
Introduction
In 2021, six National Guard soldiers in officer training were hospitalized after suffering dehydration during a six-mile “ruck march” with 35-pound backpacks at a military training school in Connecticut (Koenig, 2021). In 2002, the Washington Times reported the death of a young woman after participating in the Marine Corps Marathon (Nearman, 2002). Her death was attributed to severe hyponatremia following excessive hypotonic fluid intake. Thirteen percent out of 488 runners participating in the 2002 Boston Marathon were presented with hyponatremia (serum sodium concentration <135 mmol/l); 0.6% had severe hyponatremia (<120 mmol/l). More than 70% of 716 (38% female) runners reported water loading prior to the marathon (Almond et al., 2005). The runners attempted to maintain their central blood volume (CBV) at the cost of water intoxication just trying to prevent what in Band of Brothers First Lieutenant Harry F. Welsh of 101st Airborne Division warned Pvt. Albert Blithe for: “dehydration's a soldier's worst enemy” (Ambrose, 2001). The CBV is defined as the fraction of thoracic blood volume directly available to the left ventricle. It declines in response to the assumption of the upright position, either passive head-up tilt (HUT) or active standing up with a gravitational displacement of blood away from the thorax to the lower part of the body with a fall in venous return (Van Lieshout and Secher, 1999). Capillary transmural pressure increases in the dependent parts of the body with a non-linear accumulation of blood volume in the small vessels of the leg resulting in continued filtration into the tissue spaces and a further fall in circulating volume (Smith and Ebert, 1990; Truijen et al., 2012). The hydrostatic load of 5 min of quiet standing induces a transcapillary loss of plasma volume of ~400 ml (Lundvall and Bjerkhoel, 1994). The CBV may also become reduced in response to heat stress related to heavy physical exercise or to blood loss with the development of orthostatic intolerance (Wilson et al., 2006; Crandall et al., 2008, 2019; Gonzalez-Alonso et al., 2008; Lucas et al., 2013). Hemorrhage as a leading cause of death both in the military and civilian trauma casualty settings leads to a reduction in cerebral blood flow (CBF) and aggravates postural intolerance (Convertino et al., 2007, 2008, 2011; Crandall et al., 2019). Instruments to quantify CBV are not readily available, leaving decisions on fluid management based on the traditional parameters of blood pressure (BP) and heart rate (HR) (Jacobsen et al., 1986; Vincent and De Backer, 2013; van der Ster, 2019). Although these parameters are considered prime indicators of shock, they regularly do not reflect the loss of blood or fluid until syncope is imminent (Barcroft et al., 1944; McMichael, 1944; Secher et al., 1992; Bishop et al., 1993; Wo et al., 1993; Dabrowski et al., 2000; Harms et al., 2003). There is hardly a relationship between BP and oxygen delivery (Bartels et al., 2016) whereas tissues are in need of arterial blood flow rather than arterial pressure (Jarisch, 1928; Nichols and O'Rourke, 2005). In this mini-review, a perspective on central hypovolemia in humans is presented in relation to postural stress, exercise, and during anesthesia with a focus on early detection of central hypovolemia in the physiology lab and in the operating theater.
Postural Stress and Exercise
Obviously, in humans, the first step to exercise is preceded by the required assumption of the upright body position (Henry et al., 1951; Gauer and Thron, 1965; Wieling and van Lieshout, 1993). The act of standing itself involves physical exercise manifested by an increase in muscle sympathetic neural activity, ventilation, oxygen consumption, and carbon dioxide production (Bjurstedt et al., 1962; Wieling et al., 1996; Van Lieshout et al., 2001; Immink et al., 2006). The abrupt gravitational displacement of blood from the chest to the lower parts of the body upon standing elicits a fall in CBV and cardiac output (CO) (McMichael and Sharpey-Schafer, 1944; Harms et al., 1999, 2003). In parallel with this gravitational shift of blood, the sensitivity of the cardiac baroreflex decreases linearly, usually within 1 min (Westerhof et al., 2006; Truijen et al., 2012; Secher and Van Lieshout, 2013). Standing up creates a heart-brain hydrostatic gradient resulting in a reduction of CBF and a fall in CO by posture or cardiac disease contributes to it (Scheinberg and Stead, 1949; Hellstrøm et al., 1994; Ide et al., 1998; Pott et al., 2000; Van Lieshout et al., 2003; Bronzwaer et al., 2017b; Junejo et al., 2019, 2020; Vlastra et al., 2020; Claassen et al., 2021). Together with the acute vasodilatation in the active leg muscles, this sequence of events initiates autonomic cardiovascular reflex activity with an increase in HR and peripheral vascular resistance until an early steady state has been reached after ~2 min in the upright position with slightly reduced CO, elevated HR, and increased diastolic BP and with an impact on CBF and brain cortical oxygenation (Piorry, 1826; Hill, 1895; Sjöstrand, 1952; Gauer and Thron, 1965; Blomqvist and Stone, 1984; Bode, 1991; Levine et al., 1994; Wieling et al., 1996; Pott et al., 2000; Shoemaker et al., 2001; Van Lieshout et al., 2001; Harms et al., 2003, 2010, 2020; Immink et al., 2006). The effects of exercising in the upright vs. seated position on cardiac preload are exemplified by a lower HR during ergometer rowing than during treadmill running (Yoshiga and Higuchi, 2002). In a similar vein, the transition to the upright posture accompanying the majority of exercise modalities affects both the arterial supply to and the venous drainage from the brain (Van Lieshout et al., 2003; Dawson et al., 2004; Gisolf et al., 2004). The postural reduction in CBV and its magnitude during standing are relevant for exercise capacity since CBV determines CO and relates directly to work capacity and maximal oxygen uptake during exercise (Higginbotham et al., 1986; Van Lieshout and Secher, 1999; Dawson et al., 2007; Levine, 2008; Bada et al., 2012; Halliwill et al., 2013). The HR response to exercise not only relates to the active muscle mass but also to body position during exercise (Bevegård et al., 1960; Wang et al., 1960; Thadani and Parker, 1978; Kramer et al., 1982). In subjects presenting with what is designated as postural orthostatic tachycardia syndrome (POTS), standing upright has become an endeavor. The change of posture results in an excessive HR increment >30 with orthostatic intolerance but without orthostatic hypotension and a high HR response to a given level of exercise with reduced exercise capacity (Low et al., 1995, 1999; Joyner, 2012). POTS (Schondorf and Low, 1993) [also known as orthostatic tachycardia syndrome (Jacob et al., 1997) or orthostatic intolerance (Shannon et al., 2000)] is characterized by symptoms, such as fatigue, light-headedness, or dizziness, that come up as soon as the subject—free of orthostatic hypotension or evidence of cardiac or metabolic disease—assumes the standing position. POTS is heterogeneous in presentation and mechanisms involved are hypovolemia, deconditioning, and hyperadrenergic state with a reduced stroke volume (SV) (Masuki et al., 2007a,b; Low et al., 2009). Often a distant flu-like syndrome followed by a period of inactivity precedes POTS (Joyner, 2012). In 1871, Jacob Mendes Da Costa published the “Irritable Heart” which is considered as “a form of cardiac malady common among soldiers” (Da Costa, 1871). This concept of irritable heart was based on his observations during the Civil War with inspection, pulse rate, pulse quality, ventilatory rate, and auscultation as the measurement techniques available (Jarcho, 1959). Thomas Lewis recognized that what then was called the “effort syndrome” was, in fact, common among civilians and that of soldiers who suffered from it no less than 57% had been recruited from sedentary or light occupations before enlisting in the First World War (Lewis, 1919; Wood, 1941). The expression ‘postural tachycardia syndrome’ has replaced previous labels, such as Da Costa syndrome, soldier's heart, anxiety, exhaustion neuroses, and neurocirculatory asthenia (Oppenheimer and Rothschild, 1918; Wooley, 1976; Howell, 1985). Although psychological symptoms are common in POTS they usually are not causal (Masuki et al., 2007a). Nevertheless, heart and circulatory neurasthenia and soldier's heart continue to be considered as post-traumatic stress disorder by some (Dyde, 2011; Borges et al., 2020). Signs and symptoms of POTS resemble extreme deconditioning as observed following prolonged bed rest or spaceflight (Vernikos and Convertino, 1994; Levine et al., 1997; Gisolf et al., 2005; Joyner and Masuki, 2008) and most subjects suffering from this condition benefit from exercise training (Harms and van Lieshout, 2001; Shibata et al., 2012).
Post-exercise Hypotension and Recovery
In 1898, Leonard Hill made evident that after exertion BP falls to normal far more rapidly than the pulse frequency and recognized that BP becomes depressed below the normal resting BP after severe muscular work (Hill, 1898). As soon as the exercise halts the leg muscle pump no longer contributes to maintaining venous return and thus CBV (Romero et al., 2017). The elevated CO quickly returns to baseline but the vasodilatation in the previously exercised muscles is rather sustained. This results in a temporary imbalance between a reduced CO and a still enhanced peripheral vascular conductance with the development of PEH. The decline in SV during recovery in the seated but not in the supine position indicates that the contribution of CO to the maintenance of arterial pressure is smaller in the standing position (Halliwill et al., 2013). Systolic BP is lower during seated and supine recovery post-exercise at 50% and 75% of VO2peak vs. resting BP (Forjaz et al., 2004; Farinatti et al., 2009), and the lower peripheral resistance in the supine compared with the seated recovery position suggests resetting of the arterial baroreflex (Raine et al., 2001; White and Raven, 2014). An increased transfer function gain between diastolic BP and middle cerebral artery diastolic CBF velocity suggests a less effective dynamic cerebrovascular autoregulation in response to rapid decreases in BP during the initial 10 min of recovery from dynamic exercise (Ogoh et al., 2007). In addition, post-exercise pulmonary diffusion capacity for carbon monoxide (DLCO) is reduced with both membrane diffusion capacity and the capillary blood volume affected (Rasmussen et al., 1986; Hanel et al., 1994). Approximately 50% of the post-exercise reduction of DLCO has been attributed to a reduction in the pulmonary blood volume (Hanel et al., 1997). This, together with the reduction in venous return by loss of the muscle pump, more important in the upright vs. supine position, may be held responsible for symptoms of post-exercise orthostatic dizziness that regularly progresses to full vasovagal syncope (Holtzhausen et al., 1994; Van Lieshout et al., 2003; Takahashi et al., 2005; Roberts, 2007). A single bout of aerobic exercise is already sufficient to produce PEH, and attempts have been made to predict (Halliwill et al., 2013; Lacewell et al., 2014) and combat it (van Lieshout et al., 1992; Krediet et al., 2002, 2006). Across pre-hypertensive subjects, BP exhibits a strong positive correlation between the fall after a single session of aerobic exercise and the reduction observed after 8 weeks of aerobic training (Brito et al., 2018). A major precipitating factor for the development of PEH is prolonged exercise in the heat, resulting in hyperthermia and dehydration with bodyweight loss, and a significant reduction in CO, muscle, and skin blood flow (Gonzalez-Alonso, 1998; Hayes et al., 2000; Wilson et al., 2006; Luttrell and Halliwill, 2015; Dalmau, 2019). A recent meta-analysis suggests a larger PEH in men compared to women with an inverse association between PEH and age but a competitive half-marathon triggered PEH and a reduced cardiac baroreflex in men but not in women, leaving the physiology involved hitherto rather ill-understood (Carpio-Rivera et al., 2016; Brito et al., 2018; Mourot et al., 2020). In orthostatically intolerant athletes, recovery from postural dizziness related to PEH in the upright body position is facilitated by physical countermeasures, such as leg tensing and bending and contracting lower body muscles that engage the skeletal muscle pump to augment venous return after exercise (van Lieshout et al., 1992; Van Lieshout et al., 2001; Krediet et al., 2002, 2006). In healthy subjects, the leg-tensing maneuver lowers systemic vascular resistance with an elevation in central venous pressure (CVP), SV, and CBF. A similar effect was observed by Ray who demonstrated that in the first minute of 1-legged exercise in the upright position, CVP increased and sympathetic nerve activity became reduced (Ray, 1993). External counter measures, such as application of an impedance threshold device generating negative intrathoracic pressure to enhance venous return (Rickards et al., 2007; Convertino et al., 2012; Poh et al., 2014) or lower limb compression garments, though less practical, may reduce pre-syncopal signs and symptoms after exercise as well (Privett et al., 2010; Lacewell et al., 2014).
Estimation of CBV
To express which magnitude the CBV in a healthy subject should have and how to quantify it remains complex (Matzen et al., 1991; Brengelmann, 2019; Dalmau, 2019; Moller and Berger, 2019; Moller et al., 2019). In the supine position, healthy humans are normovolemic in that a maximal venous oxygen saturation (SvO2) is established (Harms et al., 2003; Truijen et al., 2010). From supine to upright, SvO2 decreases and with a blood loss of ~100 ml by gravitational relocation SvO2 is reduced by 1% (Harms et al., 2003; Secher and Van Lieshout, 2005). Thoracic electrical (bio-) impedance (TI) and its reciprocal admittance offer a non-invasive index of CBV changes in humans and animals (Patterson et al., 1978; Ebert et al., 1986; Matzen et al., 1990; Perko et al., 1991; Cai et al., 2000; Ogoh et al., 2003; van Lieshout et al., 2005). In the anesthetized pig, a change in the magnitude of TI appears to be an accurate non-invasive monitor of a blood volume deficit (Krantz et al., 2000). Comparisons have been made between TI and scintigraphy with technetium-99m labeled autologous red blood cells (Crandall et al., 2008). SvO2 requires a central venous line, scintigraphy is not practical during surgery and impossible to apply with changing body position, whereas TI is non-invasive but sensitive for postural movement of the liver to be avoided by careful electrode placement. A definition of normovolemia has been proposed as the CBV that ensures optimal CO and oxygen delivery to meet metabolic demand from rest to exercise (Secher and Van Lieshout, 2005; van Lieshout et al., 2005). Evidently, this does not offer a range of reference values or cut-off points, and the next best approach is by modifying the CBV by making use of environmental stressors, while quantifying biomedical parameters for thoracic blood volume (TI), systemic (CO), regional (cerebral) blood flow, and oxygen delivery. Accordingly, expanding the CBV (Ogoh et al., 2005, 2006, 2015) or reducing it is widely applied to study the cardio- and cerebrovascular effects of simulated bleeding in healthy humans. Graded central hypovolemia is created by postural stress, either active standing, HUT or simulated orthostasis by lower body “negative” (sub-atmospheric) pressure (LBNP) with or without adding HUT to aggravate the simulated gravitational load (Stevens and Lamb, 1965; Matzen et al., 1991; Rea et al., 1991; El-Bedawi and Hainsworth, 1994; Krediet et al., 2002; Hinojosa-Laborde et al., 2011, 2014; Truijen et al., 2012; Kay and Rickards, 2015; Rosenberg et al., 2021). Qualitatively comparable to the assumption of the upright position, LBNP induces venous pooling and enhances extravasation within the leg interstitial space deliberately reducing plasma volume and CBV (Matzen et al., 1991; Hinghofer-Szalkay et al., 1992, 1996; Jørgensen et al., 1993; Truijen et al., 2012). The hemodynamic responses to graded blood loss vs. LBNP are similar with the exception that the reduction in CBF represented by transcranial Doppler CBF velocity is larger during HUT (Figure 1, upper panel). This is likely attributable to the more pronounced reduction in end-tidal CO2 partial pressure and the gravitational effect on cerebral perfusion pressure with HUT (Johnson et al., 2014; Bronzwaer et al., 2017a).
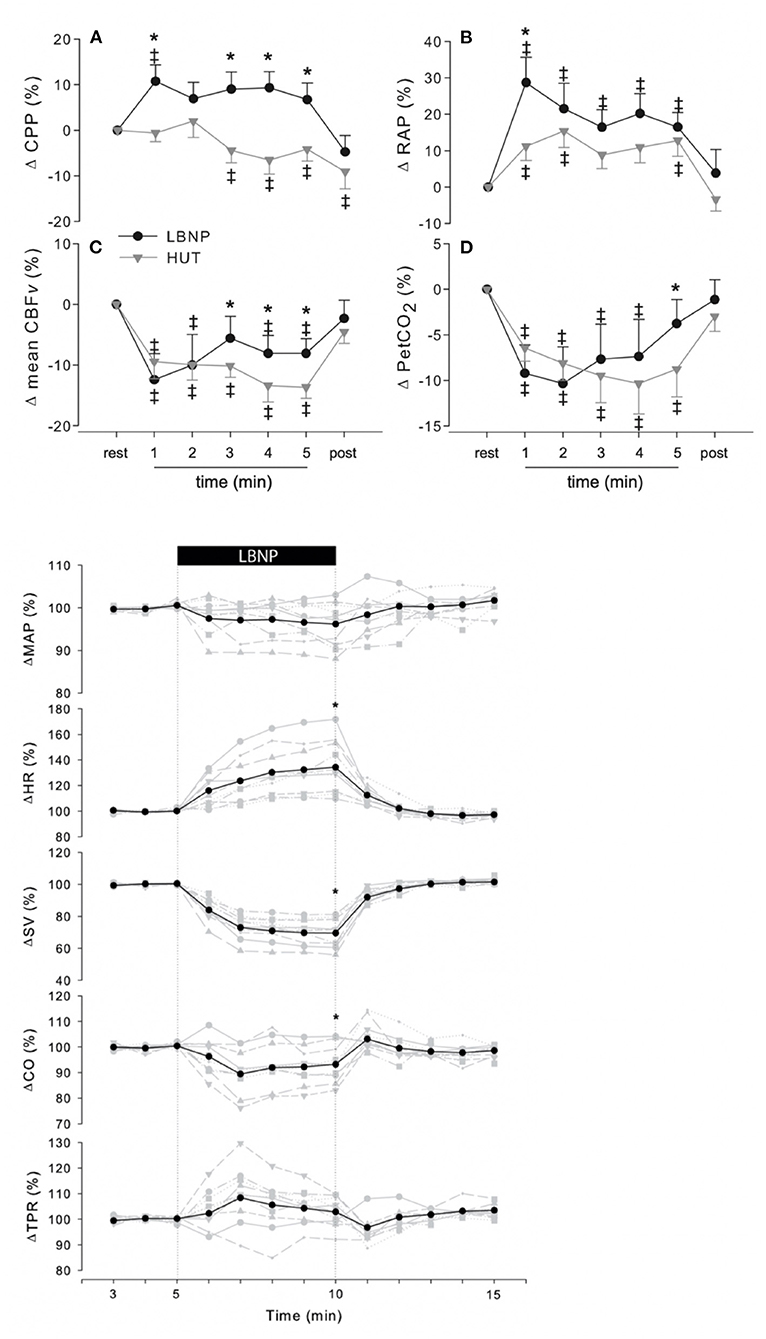
Figure 1. (A–D) Change in cerebrovascular response to 5 min LBNP−50 mmHg—(black circles) or 70◦ passive HUT (gray triangles). CPP, cerebral perfusion pressure; RAP, resistance area product; CBFv, cerebral blood flow velocity; PetCO2, end-tidal CO2; LBNP, lower body “negative” or sub-atmospheric pressure. p < 0.05 vs. rest; *p < 0.05 LBNP vs HUT; ‡p < 0.05 vs. rest. Lower panel: In 10 healthy volunteers, a total of 60 LBNP—50 mmHg—trials were performed (3 trials per subject per measurement day). Individual (gray) and averaged (black) hemodynamic responses to LBNP. Data were normalized to the last 2 min of rest. MAP, mean arterial pressure; HR, heart rate; SV, stroke volume; CO, cardiac output; TPR, total peripheral resistance; LBNP, LBNP, lower body “negative” or sub-atmospheric pressure. *p < 0.05 last 2 min of LBNP vs. last 2 min of rest. From Bronzwaer et al. (2016) and Bronzwaer et al. (2017a).
Monitoring CBV in Patients
Arterial hypotension in the emergency department and during surgery promotes progressive mismatching of oxygen delivery and demand with cardiac morbidity, renal impairment, and mortality (Jones et al., 2006; Brienza et al., 2009; Wesselink et al., 2018; Wijnberge et al., 2021). There are divergent opinions on which level of BP is indicative of arterial hypotension. In fact, given a lack of a universal definition of intraoperative hypotension, the reported incidence varies substantially with the chosen threshold (Davies et al., 2020). It has been argued that such definition is an individual cut-off value and has not accurately been derived from population-based data. It is obvious that a universally accepted standard definition of hypotension would facilitate further research into this topic (Brady and Hogue, 2013; Brady et al., 2020; Etemadi and Hogue, 2020; Wijnberge et al., 2021). Traditional patient monitoring in the emergency ward and the operating room includes HR, BP, electrocardiogram, and peripheral oxygen saturation but their use as predictors for incipient central hypovolemia is rather limited. Baroreflex control of BP makes it insensitive to blood loss up to about one liter, rendering assessment of volume status by BP monitoring not possible (Harms et al., 2003; van der Ster et al., 2018b). With the progression of central hypovolemia, cardiac preload declines until the tipping point where it has become too low to maintain a sufficient CO and when the limits of vasomotor reserve available for vasoconstriction have been reached BP drops (Schondorf and Wieling, 2000; Fu et al., 2004; Schiller et al., 2017). During World War II, the observation was repeatedly made that air raid victims in London City suffering from major blood loss presented with relative bradycardia rather than the expected tachycardia (Grant and Reeve, 1941). HR does change only minimally in the early stages, and when finally becoming beyond the “normal” range, the hypovolemic shock has already developed (Secher and Van Lieshout, 2010; Schiller et al., 2017; Suresh et al., 2018). Because of these limitations, several approaches, such as wearable sensors and advanced machine-learning techniques, have been suggested in an attempt to promote more sensitive metrics for the prediction of outcomes in civil patients and on the battlefield (Secher and Van Lieshout, 2005, 2010, 2013, 2016; Rickards et al., 2007, 2008; Convertino et al., 2011, 2020a,b; Ryan et al., 2011; Nadler et al., 2014; Schlotman et al., 2019; Rashedi et al., 2021).
Machine-Learning Based Central Hypovolemia Detection
In the last decade, efforts have been made to apply artificial intelligence (AI) and develop algorithms in the field of hemodynamic monitoring both in the operating room and on the battlefield (Convertino et al., 2008, 2011, 2020a; Rickards et al., 2014; Hatib et al., 2018; Schenk et al., 2021; Wijnberge et al., 2021). Published data on machine-learning algorithms based on arterial pressure waveform analysis are expected to play a supportive role in distinguishing normal from reduced CBV and thus left ventricular preload in healthy subjects and patients with the purpose to predict and prevent the occurrence of arterial hypotension in the anesthetized patient (Hatib et al., 2018; Connor, 2019; van der Ster et al., 2020). The earliest work in medical AI dates to the early 1970s, when the field of AI was ~15 years old. For the anesthesiologist, a monitoring system that assists in estimating the chance of developing intraoperative hypotension and predicting it would be of help (Watt et al., 1993; Mathis et al., 2018; Connor, 2019; van der Ven et al., 2020). Machine learning specifically for medicine is not new (Shortliffe, 1993; Patel et al., 2009; Convertino et al., 2011; Deo, 2015; Handelman et al., 2018), but the introduction of devices featuring models that have been trained using machine-learning algorithms has just started entering clinics. Randomized controlled trials in the field of AI-based applications of cardiovascular monitoring are as yet scarcely available (Angus, 2020; Kang et al., 2020) but progress is being made. As an example, the so-called hypotension prediction index (HPI), a machine-learning-based arterial hypotension predictive algorithm commercially available, has been proposed useful in the operating room environment (Maheshwari et al., 2020). It is based on arterial waveform features and has been claimed to predict intraoperative hypotension; the available evidence from recent clinical studies is summarized below (Davies et al., 2020; Maheshwari et al., 2020; Schneck et al., 2020; Wijnberge et al., 2020; Schenk et al., 2021). Maheshwari et al. reported a sensitivity of 88% (85–90%) and specificity of 87% (85–90%) to identify hypotensive episode 15 min in advance (area under the receiver operating characteristic curve 0.95). The fact that about 50% of the alerts were not followed by treatment was attributed to short warning time, complex treatment algorithm, or just clinicians ignoring the alert. HPI guidance did not reduce the incidence of hypotension <65 mmHg whereas that study did neither include episodes of hypotension caused by surgical manipulations (Etemadi and Hogue, 2020; Maheshwari et al., 2020). In an unblinded randomized clinical trial, patients were randomly assigned to receive either the HPI early warning system or standard care (n = 34 in each group), with a goal mean BP of at least 65 mmHg in both groups (Wijnberge et al., 2020). The median time-weighted average of hypotension was 0.10 mmHg [interquartile range (IQR), 0.01–0.43 mmHg)] in the intervention group vs. 0.44 mmHg (IQR, 0.23–0.72 mmHg) in the control group. The median time of hypotension per patient was 8 min (IQR, 1.3–26 min) in the intervention group vs. 33 min (IQR, 12–60 min) in the control group. In a 2-center retrospective analysis of 255 patients undergoing major surgery, the HPI predicted hypotension 5 min before a hypotensive event with a sensitivity and specificity of 85.8% (95% CI, 85.8–85.9%) and 85.8% (95% CI, 85.8–85.9%) (area under the curve, 0.926 [95% CI, 0.925–0.926]) (Davies et al., 2020). Intraoperative HPI-guided care did not reduce the time-weighted average of post-operative hypotension (Schenk et al., 2021). The warning for hypotension in these clinical studies generally appeared shorter than the 15 min previously reported in an offline validation study (Hatib et al., 2018). A reduction in CBV in the surgical patient is caused by insensible perspiration, hemorrhage, or by the accumulation of blood in the dependent parts of the body elicited or enhanced by regional anesthesia or surgery in sitting beach-chair position (Murphy et al., 2010; Larsen et al., 2014; Salazar et al., 2019). Accordingly, it represents a mimicry of the cardiovascular stress imposed by the assumption of the upright body position (Reithner et al., 1980; Secher and Van Lieshout, 2005; Hinojosa-Laborde et al., 2014; Larsen et al., 2014; Rickards et al., 2014; Schiller et al., 2017; Suresh et al., 2018). In a laboratory model of hemorrhage simulated by progressive central hypovolemia in healthy subjects by submitting them to either LBNP, HUT or both the global hypothesis tested was that AI-based methodologies may assist in monitoring and accordingly predict the progression from normo- to hypovolemia toward presyncope/cardiovascular collapse by extracting information of biomedical signals otherwise not routinely available. Figure 2, panel B, summarizes modeling of the non-invasive BP waveform (van der Ster et al., 2018b, 2020). During simulated hemorrhage in healthy subjects, volumetric parameters together with CBF velocity hemodynamics provided the most sensitive indication of the progression of central hypovolemia (Figure 2, panel C) (van der Ster et al., 2018a; van der Ster, 2019). One should realize that AI machine-learning algorithms do not entail a dynamic learning process evolving from use in clinical patient care (Schenk et al., 2021). In addition, the algorithm is unaware of the clinical situation and does neither provide any meaningful pathophysiological information. Inherently, there is no insight in the decisional process that leads to an early warning for intraoperative hypotension. Finally, it is up to clinicians to interpret and decide whether the predicted hypotensive episode can be ignored or requires intervention (Etemadi and Hogue, 2020). Another hurdle to overcome is to prove whether machine-learning-based prediction of hypotensive episodes actually does improve quality of care (Saugel et al., 2019; Etemadi and Hogue, 2020). In the laboratory model validation for larger reductions of CBV in humans is for obvious reasons not available. This constitutes a problem when realizing that in the second phase of impending shock when CBV has been reduced by about 30% a Bezold-Jarisch-like or vasovagal reflex may terminate sympathetic activity (Jarisch and Richter, 1939; Sander-Jensen et al., 1986; Campagna and Carter, 2003). Under those conditions, the HR response deviates from the traditionally expected tachycardia (Sander-Jensen et al., 1986). From experiments on cardiovascular reflex activity in humans subjected to LBNP, it has become evident that cardiovascular reflex patterns in response to a similar degree of exposure to LBNP are diverse and unpredictable among subjects, varying from a predominant effect on HR to a consistent increase in peripheral vascular resistance (Figure 1, lower panel) (Bronzwaer et al., 2016). Clinicians need hypotension predicting algorithms that operate with more precision and earlier warning. This requires novel methods better equipped to identify the variation in vasodepression and cardio-inhibition, especially in the run-up to cardiovascular collapse when compensatory mechanisms have become exhausted (Saugel et al., 2019; van Dijk et al., 2020). Future AI modeling should take the non-linear relationships between a volume loss and the cardiovascular response into account as well as the substantial inter-individual variability in how human body systems respond to environmental stress (Murray et al., 1968; Sander-Jensen et al., 1986; Bronzwaer et al., 2016, 2017a,b).
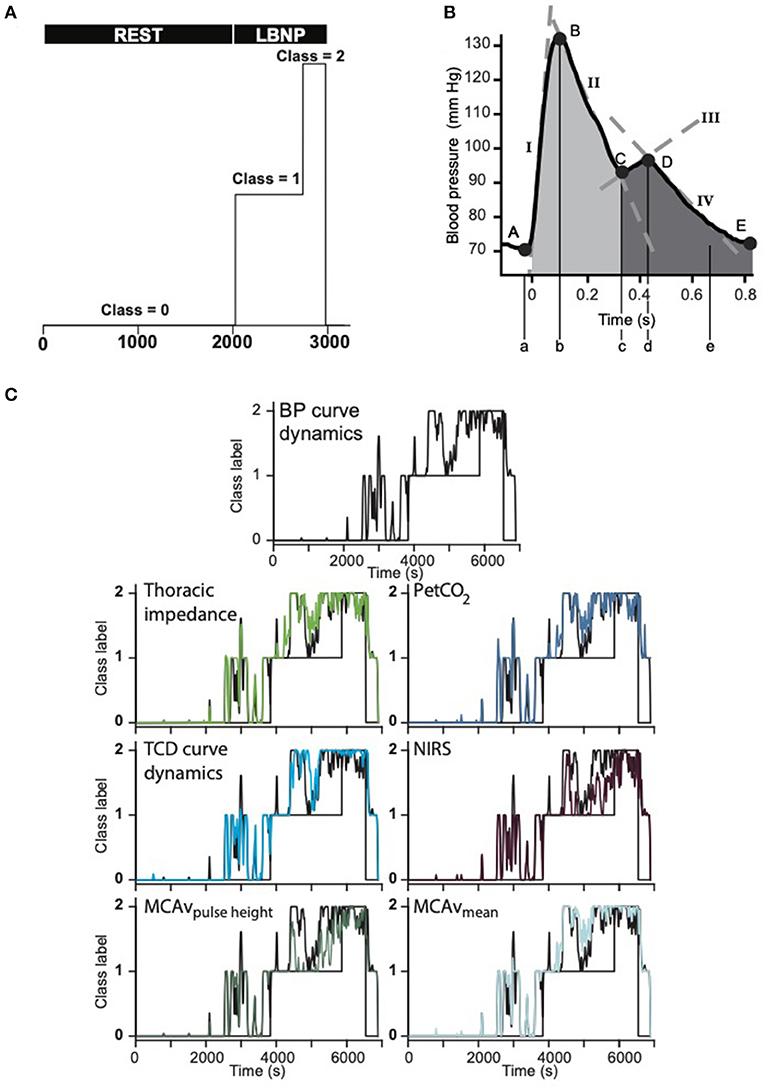
Figure 2. (A) Class definitions: baseline rest—class 0. LBNP (50 mmHg) defined as class 1, of which the last 25% as end-stage LBNP before pre-syncope (class 2). LBNP, lower body “negative” or sub-atmospheric pressure. (B) Single arterial pressure curve with five primary points (A–E). From these points, model parameters were estimated. Their accompanying time points are described with lower case letters. Tangent lines are described with roman numerals. Areas of interest are shaded. (C) In 42 (27 female) healthy subjects CBV was progressively reduced by LBNP until the onset of pre-syncope. The figure represents the output of six models compared to the blood pressure curve dynamics model (#1, top) in a single subject. Each subsequent graph shows the modulation of the addition of the annotated feature(s). In this subject the model for MCAv pulse height (bottom left) had the lowest error. Note that all model outputs increase with increasing duration of lower body negative pressure. BP, blood pressure; PetCO2, end-tidal carbon dioxide partial pressure; NIRS, near infrared spectroscopy; TCD, transcranial Doppler; MCAv, middle cerebral artery blood flow velocity; LBNP, lower body “negative” or sub-atmospheric pressure. Modified from van der Ster et al. (2018a,b).
Author Contributions
BvdS and JJvL drafted the manuscript and Y-SK and BW helped in the literature search and edited the manuscript. All authors approved the submitted version.
Funding
This research was supported by the Danish Cardiovascular Academy and an educational grant from Edwards Lifesciences (2010B0797).
Conflict of Interest
The authors declare that the research was conducted in the absence of any commercial or financial relationships that could be construed as a potential conflict of interest.
Publisher's Note
All claims expressed in this article are solely those of the authors and do not necessarily represent those of their affiliated organizations, or those of the publisher, the editors and the reviewers. Any product that may be evaluated in this article, or claim that may be made by its manufacturer, is not guaranteed or endorsed by the publisher.
Abbreviations
AI, artificial intelligence; BP, blood pressure; CBV, central blood volume; CO, cardiac output; CO2, carbon dioxide; CBF, cerebral blood flow; CVP, central venous pressure; DLCO, pulmonary diffusion capacity for carbon monoxide; EKG, electrocardiogram; HPI, hypotension prediction index; HR, heart rate; HUT, passive head-up tilt; LBNP, lower body ‘negative’; (sub-atmospheric) pressure; PEH, post-exercise hypotension; POTS, postural orthostatic tachycardia syndrome; SV, left ventricular stroke volume; TI, thoracic electrical (bio-) impedance; VO2, volume of oxygen consumption.
References
Almond, C. S., Shin, A. Y., Fortescue, E. B., Mannix, R. C., Wypij, D., Binstadt, B. A., et al. (2005). Hyponatremia among runners in the Boston Marathon. N. Engl. J. Med. 352, 1550–1556. doi: 10.1056/NEJMoa043901
Ambrose, S. E (2001). “Carentan,” in Band of Brothers, ed. S. Spielberg, and T. Hanks (Band of Brothers HBO Enterprises). Available online at: https://www.imdb.com/title/tt1247463/characters/nm0912012
Angus, D. C (2020). Randomized clinical trials of artificial intelligence. JAMA 323, 1043–1045. doi: 10.1001/jama.2020.1039
Bada, A. A., Svendsen, J. H., Secher, N. H., Saltin, B., and Mortensen, S. P. (2012). Peripheral vasodilation determines cardiac output in exercising humans: insight from atrial pacing. J. Physiol. 590, 2051–2060. doi: 10.1113/jphysiol.2011.225334
Barcroft, H., Edholm, O. G., McMichael, J., and Sharpey-Schafer, E. P. (1944). Posthaemorrhagic fainting. Study by cardiac output and forearm flow. Lancet 1, 489–491. doi: 10.1016/S0140-6736(00)74173-0
Bartels, K., Esper, S. A., and Thiele, R. H. (2016). Blood pressure monitoring for the anesthesiologist: a practical review. Anesth. Analg. 122, 1866–1879. doi: 10.1213/ANE.0000000000001340
Bevegård, B. S., Holmgren, A., and Jonsson, B. (1960). The effect of body position on the circulation at rest and during exercise, with special reference to the influence on the stroke volume. Acta Physiol. Scand. 49, 279–298. doi: 10.1111/j.1748-1716.1960.tb01953.x
Bishop, M. H., Shoemaker, W. C., Appel, P. L., Wo, C. J., Zwick, C., Kram, H. B., et al. (1993). Relationship between supranormal circulatory values, time delays, and outcome in severely traumatized patients. Crit. Care Med. 21, 56–63. doi: 10.1097/00003246-199301000-00013
Bjurstedt, H., Hesser, C. M., Liljestrand, G., and Matell, G. (1962). Effects of posture on alveolar-arterial CO2 and O2 differences and on alveolar dead space in man. Acta Physiol. Scand. 54, 65–82. doi: 10.1111/j.1748-1716.1962.tb02329.x
Blomqvist, C. G., and Stone, H. L. (1984). “Cardiovascular adjustments to gravitational stress,” in Handbook of Physiology - Section 2: The Cardiovascular System, Vol 3: Peripheral Circulation and Organ Blood Flow, eds. J. T. Shepherd, and F. M. Abboud (Washington, DC: American Physiological Society), 1025–1063.
Bode, H (1991). Cerebral blood flow velocities during orthostasis and physical exercise. Eur. J. Pediatr. 150, 738–743. doi: 10.1007/BF01958769
Borges, G. P., Tonon, J. H. A., Zunini, P., Martins da Silva, A. S., Garcia, M. F. V., de Azevedo-Marques Perico, C., et al. (2020). Soldier's heart: the forgotten circulatory neurasthenia - a systematic review. Int. Rev. Psychiatry 32, 510–519. doi: 10.1080/09540261.2020.1757925
Brady, K., and Hogue, C. W. (2013). Intraoperative hypotension and patient outcome: does “one size fit all?”. Anesthesiology 119, 495–497. doi: 10.1097/ALN.0b013e3182a10cce
Brady, K. M., Hudson, A., Hood, R., DeCaria, B., Lewis, C., and Hogue, C. W. (2020). Personalizing the definition of hypotension to protect the brain. Anesthesiology 132, 170–179. doi: 10.1097/ALN.0000000000003005
Brengelmann, G (2019). What if what you are looking for is not there? J. Appl. Physiol. 317, H393–H953. doi: 10.1152/japplphysiol.00155.2019
Brienza, N., Giglio, M. T., Marucci, M., and Fiore, T. (2009). Does perioperative hemodynamic optimization protect renal function in surgical patients? A meta-analytic study. Crit. Care Med. 37, 2079–2790. doi: 10.1097/CCM.0b013e3181a00a43
Brito, L. C., Fecchio, R. Y., Peçanha, T., Andrade-Lima, A., Halliwill, J. R., and Forjaz, C. L. M. (2018). Postexercise hypotension as a clinical tool: a “single brick” in the wall. J. Am. Soc. Hypertens. 12, e59–e64. doi: 10.1016/j.jash.2018.10.006
Bronzwaer, A. G., Verbree, J., Stok, W. J., Daemen, M. J., van Buchem, M. A., van Osch, M. J., et al. (2017a). The cerebrovascular response to lower-body negative pressure vs. head-up tilt. J. Appl. Physiol. 122, 877–883. doi: 10.1152/japplphysiol.00797.2016
Bronzwaer, A. G. T., Verbree, J., Stok, W. J., Daemen, M., van Buchem, M. A., van Osch, M. J. P., et al. (2017b). Aging modifies the effect of cardiac output on middle cerebral artery blood flow velocity. Physiol. Rep. 5:e13361. doi: 10.14814/phy2.13361
Bronzwaer, A. S., Verbree, J., Stok, W. J., van Buchem, M. A., Daemen, M. J., van Osch, M. J., et al. (2016). Cardiovascular response patterns to sympathetic stimulation by central hypovolemia. Front. Physiol. 7:235. doi: 10.3389/fphys.2016.00235
Cai, Y., Holm, S., Jenstrup, M., Strømstad, M., Eigtved, A., Warberg, J., et al. (2000). Electrical admittance for filling of the heart during lower body negative pressure in humans. J. Appl. Physiol. 89, 1569–1576. doi: 10.1152/jappl.2000.89.4.1569
Campagna, J. A., and Carter, C. (2003). Clinical relevance of the Bezold-Jarisch reflex. Anesthesiology 98, 1250–1260. doi: 10.1097/00000542-200305000-00030
Carpio-Rivera, E., Moncada-Jimenez, J., Salazar-Rojas, W., and Solera-Herrera, A. (2016). Acute effects of exercise on blood pressure: a meta-analytic investigation. Arq. Bras. Cardiol. 106, 422–433. doi: 10.5935/abc.20160064
Claassen, J. A. H. R., Thijssen, D. H. J., Panerai, R. B., and Faraci, F. M. (2021). Regulation of cerebral blood flow in humans: physiology and clinical implications of autoregulation. Physiol. Rev. 101, 1487–1559. doi: 10.1152/physrev.00022.2020
Connor, C. W (2019). Artificial intelligence and machine learning in anesthesiology. Anesthesiology 131, 1346–1359. doi: 10.1097/ALN.0000000000002694
Convertino, V. A., Moulton, S. L., Grudic, G. Z., Rickards, C. A., Hinojosa-Laborde, C., Gerhardt, R. T., et al. (2011). Use of advanced machine-learning techniques for noninvasive monitoring of hemorrhage. J. Trauma 71, S25–S32. doi: 10.1097/TA.0b013e3182211601
Convertino, V. A., Parquette, B., Zeihr, J., Traynor, K., Baia, D., Baumblatt, M., et al. (2012). Use of respiratory impedance in prehospital care of hypotensive patients associated with hemorrhage and trauma: a case series. J. Trauma Acute Care Surg. 73, S54–S59. doi: 10.1097/TA.0b013e3182606124
Convertino, V. A., Ryan, K. L., Rickards, C. A., Cooke, W. H., Idris, A. H., Metzger, A., et al. (2007). Inspiratory resistance maintains arterial pressure during central hypovolemia: implications for treatment of patients with severe hemorrhage. Crit. Care Med. 35, 1145–1152. doi: 10.1097/01.CCM.0000259464.83188.2C
Convertino, V. A., Ryan, K. L., Rickards, C. A., Salinas, J., McManus, J. G., Cooke, W. H., et al. (2008). Physiological and medical monitoring for en route care of combat casualties. J. Trauma 64, S342–S353. doi: 10.1097/TA.0b013e31816c82f4
Convertino, V. A., Schauer, S. G., Weitzel, E. K., Cardin, S., Stackle, M. E., Talley, M. J., et al. (2020a). Wearable sensors incorporating compensatory reserve measurement for advancing physiological monitoring in critically injured trauma patients. Sensors (Basel) 20:6413. doi: 10.3390/s20226413
Convertino, V. A., Wampler, M. R., Johnson, M., Alarhayem, A., Le, T. D., Nicholson, S., et al. (2020b). Validating clinical threshold values for a dashboard view of the compensatory reserve measurement for hemorrhage detection. J. Trauma Acute Care Surg. 89, S169–S174. doi: 10.1097/TA.0000000000002586
Crandall, C. G., Rickards, C. A., and Johnson, B. D. (2019). Impact of environmental stressors on tolerance to hemorrhage in humans. Am. J. Physiol. Regul. Integr. Comp. Physiol. 316, R88–R100. doi: 10.1152/ajpregu.00235.2018
Crandall, C. G., Wilson, T. E., Marving, J., Vogelsang, T. W., Kjaer, A., Hesse, B., et al. (2008). Effects of passive heating on central blood volume and ventricular dimensions in humans. J. Physiol. 586, 293–301. doi: 10.1113/jphysiol.2007.143057
Da Costa, J. M (1871). On irritable heart; a clinical study of a form of functional cardiac disorder and its consequences. Am. J. Med. Sci. 121, 2–52. doi: 10.1097/00000441-187101000-00001
Dabrowski, G. P., Steinberg, S. M., Ferrara, J. J., and Flint, L. M. (2000). A critical assessment of endpoints of shock resuscitation. Surg. Clin. N. Am. 80, 825–844. doi: 10.1016/S0039-6109(05)70098-0
Dalmau, R (2019). Towards a functional definition of euvolemia. J. Appl. Physiol. 127, 274–275. doi: 10.1152/japplphysiol.00165.2019
Davies, S. J., Vistisen, S. T., Jian, Z., Hatib, F., and Scheeren, T. W. L. (2020). Ability of an arterial waveform analysis-derived hypotension prediction index to predict future hypotensive events in surgical patients. Anesth. Analg. 130, 352–359. doi: 10.1213/ANE.0000000000004121
Dawson, E. A., Secher, N. H., Dalsgaard, M. K., Ogoh, S., Yoshiga, C. C., Gonzalez-Alonso, J., et al. (2004). Standing up to the challenge of standing: a siphon does not support cerebral blood flow in humans. Am. J. Physiol. Regul. Integr. Comp. Physiol. 287, R911–R914. doi: 10.1152/ajpregu.00196.2004
Dawson, E. A., Shave, R., Whyte, G., Ball, D., Selmer, C., Jans, O., et al. (2007). Preload maintenance and the left ventricular response to prolonged exercise in men. Exp. Physiol. 92, 383–390. doi: 10.1113/expphysiol.2006.035089
Deo, R. C (2015). Machine learning in medicine. Circulation 132, 1920–1930. doi: 10.1161/CIRCULATIONAHA.115.001593
Dyde, S (2011). The chief seat of mischief: soldier's heart in the First World War. J. Hist. Med. Allied Sci. 66, 216–248. doi: 10.1093/jhmas/jrq026
Ebert, T. J., Smith, J. J., Barney, J. A., Merrill, D. C., and Smith, G. K. (1986). The use of thoracic impedance for determining thoracic blood volume changes in man. Aviat. Space Environ. Med. 57, 49–53.
El-Bedawi, K. M., and Hainsworth, R. (1994). Combined head-up tilt and lower body suction: a test of orthostatic tolerance. Clin. Auton. Res. 4, 41–47. doi: 10.1007/BF01828837
Etemadi, M., and Hogue, C. W. (2020). Preventing intraoperative hypotension. Anesthesiology 133, 1170–1172. doi: 10.1097/ALN.0000000000003561
Farinatti, P. T. V., Nakamura, F. Y., and Polito, M. D. (2009). Influence of recovery posture on blood pressure and heart rate after resistance exercises in normotensive subjects. J. Strength Cond. Res. 23, 2487–2492. doi: 10.1519/JSC.0b013e3181b25e48
Forjaz, C., Cardoso, C., Rezk, C., Santaella, D., and Tinucci, T. (2004). Postexercise hypotension and hemodynamics: the role of exercise intensity. J. Sports Med. Phys. Fitness 44, 54–62.
Fu, Q., Witkowski, S., and Levine, B. D. (2004). Vasoconstrictor reserve and sympathetic neural control of orthostasis. Circulation 110, 2931–2937. doi: 10.1161/01.CIR.0000146384.91715.B5
Gauer, O. H., and Thron, H. L. (1965). “Postural changes in the circulation,” in Handbook of Physiology Section 2: The Cardiovascular System Vol. 3: The Peripheral Circulation, eds. J. T. Shepherd, and F. M. Abboud (Washington, DC: American Physiological Society), 2409–2439.
Gisolf, J., Immink, R. V., Van Lieshout, J. J., Stok, W. J., and Karemaker, J. M. (2005). Orthostatic blood pressure control before and after space flight, determined by time-domain baroreflex method. J. Appl. Physiol. 98, 1682–1690. doi: 10.1152/japplphysiol.01219.2004
Gisolf, J., van Lieshout, J. J., van Heusden, K., Pott, F., Stok, W. J., and Karemaker, J. M. (2004). Human cerebral venous outflow pathway depends on posture and central venous pressure. J. Physiol. 560, 317–327. doi: 10.1113/jphysiol.2004.070409
Gonzalez-Alonso, J (1998). Separate and combined influences of dehydration and hyperthermia on cardiovascular responses to exercise. Int. J. Sports Med. 19(Suppl. 2), S111–114. doi: 10.1055/s-2007-971972
Gonzalez-Alonso, J., Crandall, C. G., and Johnson, J. M. (2008). The cardiovascular challenge of exercising in the heat. J. Physiol. 586, 45–53. doi: 10.1113/jphysiol.2007.142158
Grant, R. T., and Reeve, E. B. (1941). Clinical observations on air-raid casualties. Br. Med. J. 2, 293–297. doi: 10.1136/bmj.2.4208.293
Halliwill, J. R., Buck, T. M., Lacewell, A. N., and Romero, S. A. (2013). Postexercise hypotension and sustained postexercise vasodilatation: what happens after we exercise? Exp. Physiol. 98, 7–18. doi: 10.1113/expphysiol.2011.058065
Handelman, G. S., Kok, H. K., Chandra, R. V., Razavi, A. H., Lee, M. J., and Asadi, H. (2018). eDoctor: machine learning and the future of medicine. J. Intern. Med. 284:603–619. doi: 10.1111/joim.12822
Hanel, B., Clifford, P. S., and Secher, N. H. (1994). Restricted postexercise pulmonary diffusion capacity does not impair maximal transport for O2. J. Appl. Physiol. 77, 2408–2412. doi: 10.1152/jappl.1994.77.5.2408
Hanel, B., Teunissen, I., Rabøl, A., Warberg, J., and Secher, N. H. (1997). Restricted postexercise pulmonary diffusion capacity and central blood volume depletion. J. Appl. Physiol. 83, 11–17. doi: 10.1152/jappl.1997.83.1.11
Harms, M. P., Finucane, C., Pérez-Denia, L., Jurachek, S., van Wijnen, V. K., Lipsitz, L. A., et al. (2020). Systemic and cerebral circulatory adjustment within the first 60 s after active standing: an integrative physiological view. Auton. Neurosci. 231:102756. doi: 10.1016/j.autneu.2020.102756
Harms, M. P., and van Lieshout, J. J. (2001). Cerebrovascular and cardiovascular responses associated with orthostatic intolerance and tachycardia. Clin. Auton. Res. 11, 35–38. doi: 10.1007/BF02317800
Harms, M. P., Wesseling, K. H., Pott, F., Jenstrup, M., Van Goudoever, J., Secher, N. H., et al. (1999). Continuous stroke volume monitoring by modelling flow from non-invasive measurement of arterial pressure in humans under orthostatic stress. Clin. Sci. (Lond). 97, 291–301. doi: 10.1042/CS19990061
Harms, M. P., Wieling, W., Colier, W. N., Lenders, J. W., Secher, N. H., and van Lieshout, J. J. (2010). Central and cerebrovascular effects of leg crossing in humans with sympathetic failure. Clin. Sci. (Lond). 118, 573–581. doi: 10.1042/CS20090038
Harms, M. P. M., van Lieshout, J. J., Jenstrup, M., Pott, F., and Secher, N. H. (2003). Postural effects on cardiac output and mixed venous oxygen saturation in humans. Exp. Physiol. 88, 611–616. doi: 10.1113/eph8802580
Hatib, F., Jian, Z., Buddi, S., Lee, C., Settels, J., Sibert, K., et al. (2018). Machine-learning algorithm to predict hypotension based on high-fidelity arterial pressure waveform analysis. Anesthesiology. 2018:663–674. doi: 10.1097/ALN.0000000000002300
Hayes, P., Lucas, J., and Shi, X. (2000). Importance of post-exercise hypotension in plasma volume restoration. Acta Physiol. Scand. 169, 115–124. doi: 10.1046/j.1365-201x.2000.00728.x
Hellstrøm, G., Magnusson, G., Saltin, B., and Wahlgren, N. G. (1994). Cerebral haemodynamic effects of physical exercise in patients with chronic heart failure. Cerebrovasc. Dis. 4, 256–261.
Henry, J. P., Gauer, O. H., Kety, S. S., and Kramer, K. (1951). Factors maintaining cerebral circulation during gravitational stress. J. Clin. Invest. 30, 292–300. doi: 10.1172/JCI102443
Higginbotham, M. B., Morris, K. G., Williams, R. S., McHale, P. A., Coleman, R. E., and Cobb, F. R. (1986). Regulation of stroke volume during submaximal and maximal upright exercise in normal man. Circ. Res. 58, 281–291. doi: 10.1161/01.RES.58.2.281
Hill, L (1895). The influence of the force of gravity on the circulation of the blood. J. Physiol. 18, 15–53. doi: 10.1113/jphysiol.1895.sp000556
Hill, L (1898). Arterial pressure in man while sleeping, resting, working, bathing. J. Physiol. 22, 26–30.
Hinghofer-Szalkay, H., Konig, E. M., Sauseng-Fellegger, G., and Zambo-Polz, C. (1992). Biphasic blood volume changes with lower body suction in humans. Am. J. Physiol. 263, H1270–H1275. doi: 10.1152/ajpheart.1992.263.4.H1270
Hinghofer-Szalkay, H. G., Vigas, M., Sauseng-Fellegger, G., Konig, E. M., Lichardus, B., and Jezova, D. (1996). Head-up tilt and lower body suction: comparison of hormone responses in healthy men. Physiol. Res. 45, 369–378.
Hinojosa-Laborde, C., Rickards, C. A., Ryan, K. L., and Convertino, V. A. (2011). Heart rate variability during simulated hemorrhage with lower body negative pressure in high and low tolerant subjects. Front. Physiol. 2:85. doi: 10.3389/fphys.2011.00085
Hinojosa-Laborde, C., Shade, R. E., Muniz, G. W., Bauer, C., Goei, K. A., Pidcoke, H. F., et al. (2014). Validation of lower body negative pressure as an experimental model of hemorrhage. J. Appl. Physiol. 116, 406–415. doi: 10.1152/japplphysiol.00640.2013
Holtzhausen, L. M., Noakes, T. D., Kroning, B., de Klerk, M., Roberts, M., and Emsley, R. (1994). Clinical and biochemical characteristics of collapsed ultra-marathon runners. Med. Sci. Sports Exerc. 26, 1095–1101. doi: 10.1249/00005768-199409000-00005
Howell, J. D (1985). “Soldier's heart”: the redefinition of heart disease and specialty formation in early twentieth-century Great Britain. Med. Hist. 29, 34–52. doi: 10.1017/S0025727300070502
Ide, K., Pott, F., Van Lieshout, J. J., and Secher, N. H. (1998). Middle cerebral artery blood velocity depends on cardiac output during exercise with a large muscle mass. Acta Physiol. Scand. 162, 13–20. doi: 10.1046/j.1365-201X.1998.0280f.x
Immink, R. V., Secher, N. H., Roos, C. M., Pott, F., Madsen, P. L., and van Lieshout, J. J. (2006). The postural reduction in middle cerebral artery blood velocity is not explained by PaCO2. Eur. J. Appl. Physiol. 96, 609–614. doi: 10.1007/s00421-006-0136-6
Jacob, G., Shannon, J. R., Black, B., Biaggioni, I., Mosqueda-Garcia, R., Robertson, R. M., et al. (1997). Effects of volume loading and pressor agents in idiopathic orthostatic tachycardia. Circulation 96, 575–580. doi: 10.1161/01.CIR.96.2.575
Jacobsen, J., Rorsgaard, S., and Secher, N. H. (1986). Bradycardia during hypotension following release of a tourniquet in orthopaedic surgery. Acta Anaesthesiol. Scand. 30, 511–514. doi: 10.1111/j.1399-6576.1986.tb02466.x
Jarcho, S (1959). Functional heart disease in the Civil War (Da Costa 1871). Am. J. Cardiol. 4, 809–817. doi: 10.1016/0002-9149(59)90130-4
Jarisch, A (1928). Kreislauffragen. Dtsch. Med. Wochenschr. 54, 1211–1213. doi: 10.1055/s-0028-1165452
Jarisch, A., and Richter, H. (1939). Der Bezold-Effekt - Eine Vergessene Kreislaufreaktion. Klin. Wochenschr. 18, 185–187. doi: 10.1007/BF01777614
Johnson, B. D., van Helmond, N., Curry, T. B., van Buskirk, C. M., Convertino, V. A., and Joyner, M. J. (2014). Reductions in central venous pressure by lower body negative pressure or blood loss elicit similar hemodynamic responses. J Appl Physiol 117, 131–141. doi: 10.1152/japplphysiol.00070.2014
Jones, A. E., Yiannibas, V., Johnson, C., and Kline, J. A. (2006). Emergency department hypotension predicts sudden unexpected in-hospital mortality: a prospective cohort study. Chest 130, 941–946. doi: 10.1016/S0012-3692(15)51124-0
Jørgensen, L. G., Perko, M., Perko, G., and Secher, N. H. (1993). Middle cerebral artery velocity during head-up tilt induced hypovolaemic shock in humans. Clin. Physiol. 13, 323–336. doi: 10.1111/j.1475-097X.1993.tb00333.x
Joyner, M. J (2012). Standing up for exercise: should deconditioning be medicalized? J. Physiol. 590, 3413–3414. doi: 10.1113/jphysiol.2012.238550
Joyner, M. J., and Masuki, S. (2008). POTS versus deconditioning: the same or different? Clin. Auton. Res. 18, 300–307. doi: 10.1007/s10286-008-0487-7
Junejo, R. T., Braz, I. D., Lucas, S. J., van Lieshout, J. J., Phillips, A. A., Lip, G. Y., et al. (2020). Neurovascular coupling and cerebral autoregulation in atrial fibrillation. J. Cereb. Blood Flow Metab. 40, 1647–1657. doi: 10.1177/0271678X19870770
Junejo, R. T., Braz, I. D., Lucas, S. J. E., van Lieshout, J. J., Lip, G. Y. H., and Fisher, J. P. (2019). Impaired cerebrovascular reactivity in patients with atrial fibrillation. J. Am. Coll. Cardiol. 73, 1230–1232. doi: 10.1016/j.jacc.2019.01.009
Kang, A. R., Lee, J., Jung, W., Lee, M., Park, S. Y., Woo, J., et al. (2020). Development of a prediction model for hypotension after induction of anesthesia using machine learning. PLoS One 15:e0231172. doi: 10.1371/journal.pone.0231172
Kay, V. L., and Rickards, C. A. (2015). Reproducibility of a continuous ramp lower body negative pressure protocol for simulating hemorrhage. Physiol. Rep. 3:e12640. doi: 10.14814/phy2.12640
Koenig, M (2021). Six National Guard soldiers are hospitalized after suffering dehydration during a six-mile 'ruck march' with 35-pound backpacks at military training school in Connecticut. pMailOnline Associated Newspapers Ltd.
Kramer, B., Massie, B., and Topic, N. (1982). Hemodynamic differences between supine and upright exercise in patients with congestive heart failure. Circulation 66, 820–825. doi: 10.1161/01.CIR.66.4.820
Krantz, T., Cai, Y., Lauritsen, T., Warberg, J., and Secher, N. H. (2000). Accurate monitoring of blood loss: thoracic electrical impedance during hemorrhage in the pig. Acta Anaesthesiol. Scand. 44, 598–604. doi: 10.1034/j.1399-6576.2000.00519.x
Krediet, C. T., van Dijk, N., Linzer, M., van Lieshout, J. J., and Wieling, W. (2002). Management of vasovagal syncope: controlling or aborting faints by leg crossing and muscle tensing. Circulation 106, 1684–1689. doi: 10.1161/01.CIR.0000030939.12646.8F
Krediet, C. T., van Lieshout, J. J., Bogert, L. W., Immink, R. V., Kim, Y. S., and Wieling, W. (2006). Leg crossing improves orthostatic tolerance in healthy subjects: a placebo-controlled crossover study. Am. J. Physiol. Heart Circ. Physiol. 291, H1768–1772. doi: 10.1152/ajpheart.00287.2006
Lacewell, A. N., Buck, T. M., Romero, S. A., and Halliwill, J. R. (2014). Postexercise syncope: Wingate syncope test and effective countermeasure. Exp. Physiol. 99, 172–186. doi: 10.1113/expphysiol.2013.075333
Larsen, S. L., Lyngeraa, T. S., Maschmann, C. P., Van Lieshout, J. J., and Pott, F. C. (2014). Cardiovascular consequence of reclining vs. sitting beach-chair body position for induction of anesthesia. Front. Physiol. 5:187. doi: 10.3389/fphys.2014.00187
Levine, B. D (2008). VO2max: what do we know, and what do we still need to know? J. Physiol. 586, 25–34. doi: 10.1113/jphysiol.2007.147629
Levine, B. D., Giller, C. A., Lane, L. D., Buckey, J. C., and Blomqvist, C. G. (1994). Cerebral versus systemic hemodynamics during graded orthostatic stress in humans. Circulation 90, 298–306. doi: 10.1161/01.CIR.90.1.298
Levine, B. D., Zuckerman, J. H., and Pawelczyk, J. A. (1997). Cardiac atrophy after bed-rest deconditioning: a nonneural mechanism for orthostatic intolerance. Circulation 96, 517–525. doi: 10.1161/01.CIR.96.2.517
Low, P. A., Novak, V., Spies, J. M., Novak, P., and Petty, G. W. (1999). Cerebrovascular regulation in the postural orthostatic tachycardia syndrome (POTS). Am. J. Med. Sci. 317, 124–133. doi: 10.1097/00000441-199902000-00007
Low, P. A., Opfer-Gehrking, T. L., Textor, S. C., Benarroch, E. E., Shen, W. K., Schondorf, R., et al. (1995). Postural tachycardia syndrome (POTS). Neurology 45(Suppl. 5):S19–S25.
Low, P. A., Sandroni, P., Joyner, M., and Shen, W. K. (2009). Postural tachycardia syndrome (POTS). J. Cardiovasc. Electrophysiol. 20, 352–358. doi: 10.1111/j.1540-8167.2008.01407.x
Lucas, R. A., Ganio, M. S., Pearson, J., and Crandall, C. G. (2013). Sweat loss during heat stress contributes to subsequent reductions in lower-body negative pressure tolerance. Exp. Physiol. 98, 473–480. doi: 10.1113/expphysiol.2012.068171
Lundvall, J., and Bjerkhoel, P. (1994). Failure of hemoconcentration during standing to reveal plasma volume decline induced in the erect posture. J. Appl. Physiol. 77, 2155–2162. doi: 10.1152/jappl.1994.77.5.2155
Luttrell, M. J., and Halliwill, J. R. (2015). Recovery from exercise: vulnerable state, window of opportunity, or crystal ball? Front. Physiol. 6:204. doi: 10.3389/fphys.2015.00204
Maheshwari, K., Shimada, T., Yang, D., Khanna, S., Cywinski, J. B., Irefin, S. A., et al. (2020). Hypotension prediction index for prevention of hypotension during moderate- to high-risk noncardiac surgery. Anesthesiology 133, 1214–1222. doi: 10.1097/ALN.0000000000003557
Masuki, S., Eisenach, J. H., Johnson, C. P., Dietz, N. M., Benrud-Larson, L. M., Schrage, W. G., et al. (2007a). Excessive heart rate response to orthostatic stress in postural tachycardia syndrome is not caused by anxiety. J. Appl. Physiol. 102, 896–903. doi: 10.1152/japplphysiol.00927.2006
Masuki, S., Eisenach, J. H., Schrage, W. G., Johnson, C. P., Dietz, N. M., Wilkins, B. W., et al. (2007b). Reduced stroke volume during exercise in postural tachycardia syndrome. J. Appl. Physiol. 103, 1128–1135. doi: 10.1152/japplphysiol.00175.2007
Mathis, M. R., Kheterpal, S., and Najarian, K. (2018). Artificial intelligence for anesthesia: what the practicing clinician needs to know: more than black magic for the art of the dark. Anesthesiology 129, 619–622. doi: 10.1097/ALN.0000000000002384
Matzen, S., Knigge, U., Schutten, H. J., Warberg, J., and Secher, N. H. (1990). Atrial natriuretic peptide during head-up tilt induced hypovolaemic shock in man. Acta Physiol. Scand. 140, 161–166. doi: 10.1111/j.1748-1716.1990.tb08987.x
Matzen, S., Perko, G., Groth, S., Friedman, D. B., and Secher, N. H. (1991). Blood volume distribution during head-up tilt induced central hypovolaemia in man. Clin. Physiol. 11, 411–422. doi: 10.1111/j.1475-097X.1991.tb00813.x
McMichael, J (1944). Clinical aspects of shock. J. Am. Med. Assoc. 124, 275–281. doi: 10.1001/jama.1944.02850050007003
McMichael, J., and Sharpey-Schafer, E. P. (1944). Cardiac output in man by a direct Fick methode: effect of posture, venous pressure change, atropine, and adrenaline. Br. Heart J. 6, 33–40. doi: 10.1136/hrt.6.1.33
Moller, P. W., and Berger, D. (2019). Reply to “What if what you are looking for is not there?” and “Towards a functional definition of euvolemia.” J. Appl. Physiol. 127, 276–276. doi: 10.1152/japplphysiol.00229.2019
Moller, P. W., Sondergaard, S., Jakob, S. M., Takala, J., and Berger, D. (2019). Effect of volume status on the estimation of mean systemic filling pressure. J. Appl. Physiol. 126, 1503–1513. doi: 10.1152/japplphysiol.00897.2018
Mourot, L., Fornasiero, A., Rakobowchuk, M., Isacco, L., Brighenti, A., Stella, F., et al. (2020). Post-exercise hypotension and reduced cardiac baroreflex after half-marathon run: in men, but not in women. Int. J. Environ. Res. Public Health 17:6337. doi: 10.3390/ijerph17176337
Murphy, G. S., Szokol, J. W., Marymont, J. H., Greenberg, S. B., Avram, M. J., Vender, J. S., et al. (2010). Cerebral oxygen desaturation events assessed by near-infrared spectroscopy during shoulder arthroscopy in the beach chair and lateral decubitus positions. Anesth. Analg. 111, 496–505. doi: 10.1213/ANE.0b013e3181e33bd9
Murray, R. H., Thompson, L. J., Bowers, J. A., and Albright, C. D. (1968). Hemodynamic effects of graded hypovolemia and vasodepressor syncope induced by lower body negative pressure. Am. Heart J. 76, 799–811. doi: 10.1016/0002-8703(68)90266-4
Nadler, R., Convertino, V. A., Gendler, S., Lending, G., Lipsky, A. M., Cardin, S., et al. (2014). The value of noninvasive measurement of the compensatory reserve index in monitoring and triage of patients experiencing minimal blood loss. Shock 42, 93–98. doi: 10.1097/SHK.0000000000000178
Nichols, W. W., and O'Rourke, M. F. (2005). McDonald's Blood Flow in Arteries: Theoretical, Experimental and Clinical Principles. London: Hodder Arnold.
Ogoh, S., Brothers, R. M., Barnes, Q., Eubank, W. L., Hawkins, M. N., Purkayastha, S., et al. (2005). The effect of changes in cardiac output on middle cerebral artery mean blood velocity at rest and during exercise. J. Physiol. 569, 697–704. doi: 10.1113/jphysiol.2005.095836
Ogoh, S., Brothers, R. M., Barnes, Q., Eubank, W. L., Hawkins, M. N., Purkayastha, S., et al. (2006). Effects of changes in central blood volume on carotid-vasomotor baroreflex sensitivity at rest and during exercise. J. Appl. Physiol. 101, 68–75. doi: 10.1152/japplphysiol.01452.2005
Ogoh, S., Fisher, J. P., Purkayastha, S., Dawson, E. A., Fadel, P. J., White, M. J., et al. (2007). Regulation of middle cerebral artery blood velocity during recovery from dynamic exercise in humans. J. Appl. Physiol. 102, 713–721. doi: 10.1152/japplphysiol.00801.2006
Ogoh, S., Hirasawa, A., Raven, P. B., Rebuffat, T., Denise, P., Lericollais, R., et al. (2015). Effect of an acute increase in central blood volume on cerebral hemodynamics. Am. J. Physiol. Regul. Integr. Comp. Physiol. 309, R902–R911. doi: 10.1152/ajpregu.00137.2015
Ogoh, S., Volianitis, S., Nissen, P., Wray, D. W., Secher, N. H., and Raven, P. B. (2003). Carotid baroreflex responsiveness to head-up tilt-induced central hypovolaemia: effect of aerobic fitness. J. Physiol. 551, 601–608. doi: 10.1113/jphysiol.2003.046029
Oppenheimer, B. S., and Rothschild, M. A. (1918). The psychoneurotic factor in the irritable heart of soldiers. J. Am. Med. Assoc. 70, 1919–1922. doi: 10.1001/jama.1918.26010250005006a
Patel, V. L., Shortliffe, E. H., Stefanelli, M., Szolovits, P., Berthold, M. R., Bellazzi, R., et al. (2009). The coming of age of artificial intelligence in medicine. Artif. Intell. Med. 46, 5–17. doi: 10.1016/j.artmed.2008.07.017
Patterson, R., Kubicek, W., Witsoe, D., and From, A. (1978). Studies on the effect of controlled volume change on the thoracic electrical impedance. Med. Biol. Eng. Comput. 16, 531–536. doi: 10.1007/BF02457804
Perko, G., Perko, M. J., Jansen, E., and Secher, N. H. (1991). Thoracic impedance as an index of body fluid balance during cardiac surgery. Acta Anaesthesiol. Scand. 35, 568–571. doi: 10.1111/j.1399-6576.1991.tb03349.x
Piorry, P. A (1826). Recherches sur l'influence de la pesanteur sur le cours du sang; diagnostic de la syncope et de l'apoplexie; cause et traitement de la syncope. Arch. Gén. Méd. 12, 527–544.
Poh, P. Y., Carter, R. 3rd, Hinojosa-Laborde, C., Mulligan, J., Grudic, G. Z., and Convertino, V. A. (2014). Respiratory pump contributes to increased physiological reserve for compensation during simulated haemorrhage. Exp. Physiol. 99, 1421–1426. doi: 10.1113/expphysiol.2014.081208
Pott, F., Van Lieshout, J. J., Ide, K., Madsen, P., and Secher, N. H. (2000). Middle cerebral artery blood velocity during a valsalva maneuver in the standing position. J. Appl. Physiol. 88, 1545–1550. doi: 10.1152/jappl.2000.88.5.1545
Privett, S. E., George, K. P., Whyte, G. P., and Cable, N. T. (2010). The effectiveness of compression garments and lower limb exercise on post-exercise blood pressure regulation in orthostatically intolerant athletes. Clin. J. Sport Med. 20, 362–367. doi: 10.1097/JSM.0b013e3181f20292
Raine, N. M., Cable, N. T., George, K. P., and Campbell, I. G. (2001). The influence of recovery posture on post-exercise hypotension in normotensive men. Med. Sci. Sports Exerc. 33, 404–412. doi: 10.1097/00005768-200103000-00012
Rashedi, N., Sun, Y., Vaze, V., Shah, P., Halter, R., Elliott, J. T., et al. (2021). Early detection of hypotension using a multivariate machine learning approach. Mil. Med. 186, 440–444. doi: 10.1093/milmed/usaa323
Rasmussen, B. S., Hanel, B., Jensen, K., Serup, B., and Secher, N. H. (1986). Decrease in pulmonary diffusion capacity after maximal exercise. J. Sports Sci. 4, 185–188. doi: 10.1080/02640418608732117
Ray, C. A (1993). Muscle sympathetic nerve responses to prolonged one-legged exercise. J. Appl. Physiol. 74, 1719–1722. doi: 10.1152/jappl.1993.74.4.1719
Rea, R. F., Hamhan, M., Clary, M. P., Randels, M. J., Dayton, P. J., and Strauss, R. G. (1991). Comparison of muscle sympathetic responses to hemorrhage and lower body negative pressure in humans. J. Appl. Physiol. 70, 1401–1405. doi: 10.1152/jappl.1991.70.3.1401
Reithner, L., Johansson, H., and Strouth, L. (1980). Insensible perspiration during anaesthesia and surgery. Acta Anaesthesiol. Scand. 24, 362–366. doi: 10.1111/j.1399-6576.1980.tb01564.x
Rickards, C. A., Ryan, K. L., Cooke, W. H., Lurie, K. G., and Convertino, V. A. (2007). Inspiratory resistance delays the reporting of symptoms with central hypovolemia: association with cerebral blood flow. Am. J. Physiol. Regul. Integr. Comp. Physiol. 293, R243–R250. doi: 10.1152/ajpregu.00087.2007
Rickards, C. A., Ryan, K. L., Cooke, W. H., Romero, S. A., and Convertino, V. A. (2008). Combat stress or hemorrhage? Evidence for a decision-assist algorithm for remote triage. Aviat. Space Environ. Med. 79, 670–676. doi: 10.3357/ASEM.2223.2008
Rickards, C. A., Vyas, N., Ryan, K. L., Ward, K. R., Andre, D., Hurst, G. M., et al. (2014). Are you bleeding? Validation of a machine-learning algorithm for determination of blood volume status: application to remote triage. J. Appl. Physiol. 116, 486–494. doi: 10.1152/japplphysiol.00012.2013
Roberts, W. O (2007). Exercise-associated collapse care matrix in the marathon. Sports Med. 37, 431–433. doi: 10.2165/00007256-200737040-00041
Romero, S. A., Minson, C. T., and Halliwill, J. R. (2017). The cardiovascular system after exercise. J. Appl. Physiol. 122, 925–932. doi: 10.1152/japplphysiol.00802.2016
Rosenberg, A. J., Kay, V. L., Anderson, G. K., Sprick, J. D., and Rickards, C. A. (2021). A comparison of protocols for simulating hemorrhage in humans: step versus ramp lower body negative pressure. J. Appl. Physiol. 130, 380–389. doi: 10.1152/japplphysiol.00230.2020
Ryan, K. L., Rickards, C. A., Hinojosa-Laborde, C., Gerhardt, R. T., Cain, J., and Convertino, V. A. (2011). Advanced technology development for remote triage applications in bleeding combat casualties. US Army Med. Dep. J. 61–72.
Salazar, D. H., Davis, W. J., Ziroglu, N., and Garbis, N. G. (2019). Cerebral desaturation events during shoulder arthroscopy in the beach chair position. JAAOS Glob. Res. Rev. 3:e007. doi: 10.5435/JAAOSGlobal-D-19-00007
Sander-Jensen, K., Secher, N. H., Bie, P., Warberg, J., and Schwartz, T. W. (1986). Vagal slowing of the heart during haemorrhage: observations from 20 consecutive hypotensive patients. Br. Med. J. 292, 364–366. doi: 10.1136/bmj.292.6517.364
Saugel, B., Kouz, K., Hoppe, P., Maheshwari, K., and Scheeren, T. W. L. (2019). Predicting hypotension in perioperative and intensive care medicine. Best Pract. Res. Clin. Anaesthesiol. 33, 189–197. doi: 10.1016/j.bpa.2019.04.001
Scheinberg, P., and Stead, E. A. (1949). The cerebral blood flow in male subjects as measured by the nitrous oxide technique. Normal values for blood flow, oxygen utilization, glucose utilization and peripheral resistance, with observations on the effect of tilting and anxiety. J. Clin. Invest. 28, 1163–1171. doi: 10.1172/JCI102150
Schenk, J., Wijnberge, M., Maaskant, J. M., Hollmann, M. W., Hol, L., Immink, R. V., et al. (2021). Effect of Hypotension Prediction Index-guided intraoperative haemodynamic care on depth and duration of postoperative hypotension: a sub-study of the Hypotension Prediction trial. Br. J. Anaesth. 125, 681–688. doi: 10.1016/j.bja.2021.05.033
Schiller, A. M., Howard, J. T., and Convertino, V. A. (2017). The physiology of blood loss and shock: new insights from a human laboratory model of hemorrhage. Exp. Biol. Med. 242, 874–883. doi: 10.1177/1535370217694099
Schlotman, T. E., Lehnhardt, K. R., Abercromby, A. F., Easter, B. D., Downs, M. E., Akers, L., et al. (2019). Bridging the gap between military prolonged field care monitoring and exploration spaceflight: the compensatory reserve. NPJ Microgravity 5:29. doi: 10.1038/s41526-019-0089-9
Schneck, E., Schulte, D., Habig, L., Ruhrmann, S., Edinger, F., Markmann, M., et al. (2020). Hypotension Prediction Index based protocolized haemodynamic management reduces the incidence and duration of intraoperative hypotension in primary total hip arthroplasty: a single centre feasibility randomised blinded prospective interventional trial. J. Clin. Monit. Comput. 34, 1149–1158. doi: 10.1007/s10877-019-00433-6
Schondorf, R., and Low, P. A. (1993). Idiopathic postural orthostatic tachycardia syndrome: an attenuated form of acute pandysautonomia? Neurology 43, 132–137. doi: 10.1212/WNL.43.1_Part_1.132
Schondorf, R., and Wieling, W. (2000). Vasoconstrictor reserve in neurally mediated syncope. Clin. Auton. Res. 10, 53–55. doi: 10.1007/BF02279891
Secher, N. H., Jacobsen, J., Friedman, D. B., and Matzen, S. (1992). Bradycardia during reversible hypovolaemic shock: associated neural reflex mechanisms and clinical implications. J. Clin. Exp. Pharmacol. Physiol. 19, 733–743. doi: 10.1111/j.1440-1681.1992.tb00411.x
Secher, N. H., and Van Lieshout, J. J. (2005). Normovolaemia defined by central blood volume and venous oxygen saturation. Clin. Exp. Pharmacol. Physiol. 32, 901–910. doi: 10.1111/j.1440-1681.2005.04283.x
Secher, N. H., and Van Lieshout, J. J. (2010). Heart rate during haemorrhage: time for reappraisal. J. Physiol. 588:19. doi: 10.1113/jphysiol.2009.184499
Secher, N. H., and Van Lieshout, J. J. (2013). “Circulatory shock,” in Autonomic Failure: A Textbook of Clinical Disorders of the Autonomic Nervous System, 5 Edn, eds. C. J. Mathias, and R. Bannister (Oxford: Oxford University Press), 754–760.
Secher, N. H., and Van Lieshout, J. J. (2016). “Hypovolemic shock,” in Clinical Fluid Therapy in the Perioperative Setting, 2 Edn, ed. R. G. Hahn (Cambridge: Cambridge University Press), 222–231.
Shannon, J. R., Flattem, N. L., Jordan, J., Jacob, G., Black, B. K., Biaggioni, I., et al. (2000). Orthostatic intolerance and tachycardia associated with norepinephrine-transporter deficiency. N. Engl. J. Med. 342, 541–549. doi: 10.1056/NEJM200002243420803
Shibata, S., Fu, Q., Bivens, T. B., Hastings, J. L., Wang, W., and Levine, B. D. (2012). Short-term exercise training improves the cardiovascular response to exercise in the postural orthostatic tachycardia syndrome. J. Physiol. 590, 3495–3505. doi: 10.1113/jphysiol.2012.233858
Shoemaker, J. K., Hogeman, C. S., Khan, M., Kimmerly, D. S., and Sinoway, L. I. (2001). Gender affects sympathetic and hemodynamic response to postural stress. Am. J. Physiol. Heart Circ. Physiol. 281, H2028–H2035. doi: 10.1152/ajpheart.2001.281.5.H2028
Shortliffe, E. H (1993). The adolescence of AI in medicine: will the field come of age in the ‘90s? Artif. Intell. Med. 5, 93–106. doi: 10.1016/0933-3657(93)90011-Q
Sjöstrand, T (1952). The regulation of the blood distribution in man. Acta Physiol. Scand. 26, 312–327. doi: 10.1111/j.1748-1716.1952.tb00912.x
Smith, J. J., and Ebert, J. (1990). General Response to Orthostatic Stress. Boca Raton, FL: CRC Press.
Stevens, P. M., and Lamb, L. E. (1965). Effects of lower body negative pressure on the cardiovascular system. Am. J. Cardiol. 16, 506–515. doi: 10.1016/0002-9149(65)90027-5
Suresh, M. R., Chung, K. K., Schiller, A. M., Holley, A. B., Howard, J. T., and Convertino, V. A. (2018). Unmasking the hypovolemic shock continuum: the compensatory reserve. J. Intensive Care Med. 34:696–706. doi: 10.1177/0885066618790537
Takahashi, T., Hayano, J., Okada, A., Saitoh, T., and Kamiya, A. (2005). Effects of the muscle pump and body posture on cardiovascular responses during recovery from cycle exercise. Eur. J. Appl. Physiol. 94, 576–583. doi: 10.1007/s00421-005-1369-5
Thadani, U., and Parker, J. O. (1978). Hemodynamics at rest and during supine and sitting bicycle exercise in normal subjects. Am. J. Cardiol. 41, 52–59. doi: 10.1016/0002-9149(78)90131-5
Truijen, J., Bundgaard-Nielsen, M., and van Lieshout, J. J. (2010). A definition of normovolaemia and consequences for cardiovascular control during orthostatic and environmental stress. Eur. J. Appl. Physiol. 109, 141–157. doi: 10.1007/s00421-009-1346-5
Truijen, J., Kim, Y. S., Krediet, C. T., Stok, W. J., Kolgen, R. S., Colier, W. N., et al. (2012). Orthostatic leg blood volume changes assessed by near-infrared spectroscopy. Exp. Physiol. 97, 353–361. doi: 10.1113/expphysiol.2011.061051
van der Ster, B. J. P., Bennis, F. C., Delhaas, T., Westerhof, B. E., Stok, W. J., and van Lieshout, J. J. (2018a). Support vector machine based monitoring of cardio-cerebrovascular reserve during simulated hemorrhage. Front. Physiol. 8:1057. doi: 10.3389/fphys.2017.01057
van der Ster, B. J. P., Westerhof, B. E., Stok, W. J., and van Lieshout, J. J. (2018b). Detecting central hypovolemia in simulated hypovolemic shock by automated feature extraction with principal component analysis. Physiol. Rep. 6:e13895. doi: 10.14814/phy2.13895
van der Ster, B. J. P., Wijnberge, M., Huntelaar, M, de Haan, J., van der Sluijs, M. C., Veelo, D. P., et al. (2020). P67 detecting preload reduction with machine learning on arterial waveform parameters. Artery Res. 25:S111. doi: 10.2991/artres.k.191224.098
van der Ster, J (2019). Machine Learning for Cardio- and Cerebrovascular Monitoring. Amsterdam: Universiteit van Amsterdam.
van der Ven, W. H., Veelo, D. P., Wijnberge, M., van der Ster, B. J., Vlaar, A. P., and Geerts, B. F. (2020). One of the first validations of an artificial intelligence algorithm for clinical use: the impact on intraoperative hypotension prediction and clinical decision-making. Surgery. 169, 1300–1303. doi: 10.1016/j.surg.2020.09.041
van Dijk, J. G., Ghariq, M., Kerkhof, F. I., Reijntjes, R., van Houwelingen, M. J., van Rossum, I. A., et al. (2020). Novel methods for quantification of vasodepression and cardioinhibition during tilt-induced vasovagal syncope. Circ. Res. 127, e126–e138. doi: 10.1161/CIRCRESAHA.120.316662
van Lieshout, J. J., Harms, M. P., Pott, F., Jenstrup, M., and Secher, N. H. (2005). Stroke volume of the heart and thoracic fluid content during head-up and head-down tilt in humans. Acta Anaesthesiol. Scand. 49, 1287–1292. doi: 10.1111/j.1399-6576.2005.00841.x
Van Lieshout, J. J., Pott, F., Madsen, P. L., van Goudoever, J., and Secher, N. H. (2001). Muscle tensing during standing: effects on cerebral tissue oxygenation and cerebral artery blood velocity. Stroke 32, 1546–1551. doi: 10.1161/01.STR.32.7.1546
Van Lieshout, J. J., and Secher, N. H. (1999). “Orthostatic stress and autonomic dysfunction,” in Exercise and Circulation in Health and Disease, 1 Edn., eds. B. Saltin, R. Boushel, N. H. Secher, and J. H. Mitchell (Champaign, IL: Human Kinetics), 305–324.
van Lieshout, J. J., ten Harkel, A. D., and Wieling, W. (1992). Physical manoeuvres for combating orthostatic dizziness in autonomic failure. Lancet 339, 897–898. doi: 10.1016/0140-6736(92)90932-S
Van Lieshout, J. J., Wieling, W., Karemaker, J. M., and Secher, N. H. (2003). Syncope, cerebral perfusion, and oxygenation. J. Appl. Physiol. 94, 833–848. doi: 10.1152/japplphysiol.00260.2002
Vernikos, J., and Convertino, V. A. (1994). Advantages and disadvantages of fludrocortisone or saline load in preventing post-spaceflight orthostatic hypotension. Acta Astronaut. 33, 259–266. doi: 10.1016/0094-5765(94)90133-3
Vincent, J.-L., and De Backer, D. (2013). Circulatory shock. N. Engl. J. Med. 369, 1726–1734. doi: 10.1056/NEJMra1208943
Vlastra, W., Nieuwkerk, A. C., Bronzwaer, A. S. G. T., Versteeg, A., Bron, E. E., Niessen, W. J., et al. (2020). Cerebral blood flow in patients with severe aortic valve stenosis undergoing transcatheter aortic valve implantation. J. Am. Geriatr. Soc. 69, 494–499. doi: 10.1111/jgs.16882
Wang, Y., Marshall, R. J., and Shepherd, J. T. (1960). The effect of changes in posture and of graded exercise on stroke volume in man. J. Clin. Invest. 39, 1051–1061. doi: 10.1172/JCI104120
Watt, R. C., Maslana, E. S., and Mylrea, K. C. (1993). Alarms and anesthesia: challenges in design of intelligent systems for patient monitoring. IEEE Eng. Med. Biol. Magaz. 12, 34–41. doi: 10.1109/51.248165
Wesselink, E. M., Kappen, T. H., Torn, H. M., Slooter, A. J. C., and van Klei, W. A. (2018). Intraoperative hypotension and the risk of postoperative adverse outcomes: a systematic review. Br. J. Anaesth. 121, 706–721. doi: 10.1016/j.bja.2018.04.036
Westerhof, B. E., Gisolf, J., Karemaker, J. M., Wesseling, K. H., Secher, N. H., and van Lieshout, J. J. (2006). Time course analysis of baroreflex sensitivity during postural stress. Am. J. Physiol. Heart Circ. Physiol. 291, H2864–2874. doi: 10.1152/ajpheart.01024.2005
White, D. W., and Raven, P. B. (2014). Autonomic neural control of heart rate during dynamic exercise: revisited. J. Physiol. 592, 2491–2500. doi: 10.1113/jphysiol.2014.271858
Wieling, W., Harms, M. P., ten Harkel, A. D., van Lieshout, J. J., and Sprangers, R. L. (1996). Circulatory response evoked by a 3 s bout of dynamic leg exercise in humans. J. Physiol. 494 (Pt 2), 601–611. doi: 10.1113/jphysiol.1996.sp021518
Wieling, W., and van Lieshout, J. J. (1993). Investigation and treatment of autonomic circulatory failure. Curr. Opin. Neurol. Neurosurg. 6, 537–543.
Wijnberge, M., Geerts, B. F., Hol, L., Lemmers, N., Mulder, M. P., Berge, P., et al. (2020). Effect of a machine learning-derived early warning system for intraoperative hypotension vs standard care on depth and duration of intraoperative hypotension during elective noncardiac surgery: the HYPE randomized clinical trial. JAMA 323, 1052–1060. doi: 10.1001/jama.2020.0592
Wijnberge, M., Schenk, J., Bulle, E., Vlaar, A. P., Maheshwari, K., Hollmann, M. W., et al. (2021). Association of intraoperative hypotension with postoperative morbidity and mortality: systematic review and meta-analysis. BJS Open 5:zraa018. doi: 10.1093/bjsopen/zraa018
Wilson, T. E., Cui, J., Zhang, R., and Crandall, C. G. (2006). Heat stress reduces cerebral blood velocity and markedly impairs orthostatic tolerance in humans. Am. J. Physiol. Regul. Integr. Comp. Physiol. 291, R1443–R1448. doi: 10.1152/ajpregu.00712.2005
Wo, C. C., Shoemaker, W. C., Appel, P. L., Bishop, M. H., Kram, H. B., and Hardin, E. (1993). Unreliability of blood pressure and heart rate to evaluate cardiac output in emergency resuscitation and critical illness. Crit. Care Med. 21, 218–223. doi: 10.1097/00003246-199302000-00012
Wood, P (1941). Da Costa's syndrome (or effort syndrome). Lecture 1. Br. Med. J. 1, 805–845. doi: 10.1136/bmj.1.4195.805
Wooley, C. F (1976). Where are the diseases of yesteryear? DaCosta's syndrome, soldiers heart, the effort syndrome, neurocirculatory asthenia - and the mitral valve prolapse syndrome. Circulation 53, 749–751. doi: 10.1161/01.CIR.53.5.749
Keywords: anesthesia, artificial intelligence, cardiovascular modeling, exercise, head-up tilt, hypovolemia, lower body negative pressure, POTS
Citation: van der Ster BJP, Kim Y-S, Westerhof BE and van Lieshout JJ (2021) Central Hypovolemia Detection During Environmental Stress—A Role for Artificial Intelligence? Front. Physiol. 12:784413. doi: 10.3389/fphys.2021.784413
Received: 27 September 2021; Accepted: 18 November 2021;
Published: 15 December 2021.
Edited by:
Bruno Moreira Silva, Federal University of São Paulo, BrazilReviewed by:
Leandro Campos De Brito, University of São Paulo, BrazilJuliano Casonatto, Universidade Norte do Paraná, Brazil
Aymen A. Alian, Yale University, United States
Copyright © 2021 van der Ster, Kim, Westerhof and van Lieshout. This is an open-access article distributed under the terms of the Creative Commons Attribution License (CC BY). The use, distribution or reproduction in other forums is permitted, provided the original author(s) and the copyright owner(s) are credited and that the original publication in this journal is cited, in accordance with accepted academic practice. No use, distribution or reproduction is permitted which does not comply with these terms.
*Correspondence: Johannes J. van Lieshout, j.j.vanlieshout@amsterdamumc.nl; orcid.org/0000-0002-3646-212