- 1School of Physical Education and Sport Training, Shanghai University of Sport, Shanghai, China
- 2College of Physical Education and Health Sciences, Longyan University, Longyan, China
- 3School of Physical Education and Sport Science, Fujian Normal University, Fuzhou, China
- 4Physical Education Department, College of Education, United Arab Emirates University, Al Ain, United Arab Emirates
- 5Department of Sports Science and Clinical Biomechanics, SDU Sport and Health Sciences Cluster (SHSC), Faculty of Health Sciences, University of Southern Denmark, Odense, Denmark
- 6China Institute of Sport Science, Beijing, China
Purpose: The aim of this study was to investigate the physiological and perceptional responses to forward, forward-backward, and lateral shuttle running.
Methods: Twenty-four eligible male subjects performed a maximal oxygen uptake (VO2max) test and three directional modes (i.e., forward, forward-backward, and lateral) of 5-m shuttle running at the speed of 6 km⋅h–1 for 5 min on separate days. Heart rate (HR) and oxygen uptake (VO2) were continuously measured during the whole tests. Rating of perceived exertion (RPE) was inquired and recorded immediately after the test. Capillary blood samples were collected from the earlobe during the recovery to determine the peak value of blood lactate concentration ([La–]peak).
Results: Running directional mode had significant effects on HR (F = 72.761, P < 0.001, η2p = 0.760), %HRmax (F = 75.896, P < 0.001, η2p = 0.767), VO2 (F = 110.320, P < 0.001, η2p = 0.827), %VO2max (F = 108.883, P < 0.001, η2p = 0.826), [La–]peak (F = 55.529, P < 0.001, η2p = 0.707), and RPE (F = 26.268, P < 0.001, η2p = 0.533). All variables were significantly different between conditions (P ≤ 0.026), with the variables highest in lateral shuttle running and lowest in forward shuttle running. The effect sizes indicated large magnitude in the differences of all variables between conditions (ES = 0.86–2.83, large) except the difference of RPE between forward and forward-backward shuttle running (ES = 0.62, moderate).
Conclusion: These findings suggest that the physiological and perceptional responses in shuttle running at the same speed depend on the directional mode, with the responses highest in lateral shuttle running, and lowest in forward shuttle running.
Introduction
Shuttle running, in which acceleration, deceleration, and 180°change of direction (COD) are performed successively at a given distance, is often used in either sport training (Zadro et al., 2011) or fitness evaluations (Léger et al., 1988; Stewart et al., 2014). It can be not only performed at submaximal speed (Hatamoto et al., 2014; Stevens et al., 2015) but also be applied in high-intensity interval training (Bravo et al., 2008; Haydar et al., 2011; Zadro et al., 2011). Due to the speed fluctuation and directional change (Dellal et al., 2010), shuttle running may produce additional physical load when compared with constant straight-forward running at the same average speed (Osgnach et al., 2010; Zago et al., 2018). It has been widely proved that the inclusion of COD during running could elicit greater heart rate (HR), oxygen uptake (VO2), rating of perceived exertion (RPE), and blood lactate concentration ([La–]) than linear running for both submaximal (Buchheit et al., 2011) and high intensity (Dellal et al., 2010).
Shuttle running can be manipulated by the distance between each turning, mean speed, and directional mode. Previous studies focused primarily on the distance and speed of shuttle running. The distance usually used in shuttle running was 5 m, 10 m, or 20 m. For the same mean speed of shuttle runs, shorter distance indicates higher COD frequency and larger speed fluctuation. The peak value of blood lactate concentration ([La–]peak) could reach 3.8 ± 2.6 mmol⋅L–1 (Buchheit et al., 2011) and 9.78 ± 3.05 mmol⋅L–1 (Zago et al., 2018) after 20-m and 5-m shuttle running with 75% maximal aerobic speed for 5 min. Hatamoto et al. (2014) explored the effect of COD frequency on the energy cost of shuttle running at different speeds. The results indicated that VO2, HR, and RPE rose almost linearly as the COD frequency increased from 13 to 18 times per minute at different velocities (3, 4, 5, 6, and 7 km⋅h–1). Ashton and Twist (2015) demonstrated that more directional changes during intermittent shuttle running increased the physiological load on female athletes. With regard to the same distance shuttle runs, there was a consensus that the HR, RPE, and VO2 per unit time was significantly higher at greater speed (Buchheit et al., 2011; Hatamoto et al., 2013) while the results of VO2 per unit distance in different researches didn’t come to an agreement. Buchheit et al. (2011) reported that there was no significant difference in VO2 per meter between different speeds (45, 60, and 75% of maximal aerobic speed), whereas Stevens et al. (2015) and Zago et al. (2018) claimed that it would be significantly higher when the speed increased. However, researchers mainly focused on forward running, which has been widely studied in either the physiological demands or the acute biomechanical determinants (Ishii et al., 2011; Zamparo et al., 2015; Zago et al., 2019, 2021). Although forward running has received much attention, other directional modes of shuttle running have been less concerned.
Running can be briefly divided into forward, backward, lateral based on the direction, and these running modes exist in daily activities and sports. Since backward running and lateral running are not habitual exercise for humans, they are expected to induce higher physiological and perceptional responses than forward locomotion. A few studies compared the differences of physiological and perceptional responses between backward and forward running on the treadmill (Flynn et al., 1994; Masumoto et al., 2009), yet lateral running was seldom incorporated in the experiment design. Indeed, moving backwards or laterally is often integrated with COD in many sports, such as basketball, soccer, handball, badminton, and tennis (Williford et al., 1998; Maurus et al., 2019). Nevertheless, until now, there has been no published research on the comparison of physiological and perceptional responses to shuttle running with different directional modes. Moreover, knowledge about the physiological demands of exercise is useful to assess training/exercise load and to formulate training plans, exercise prescription, fatigue prevention strategies, and nutrition management (Zago et al., 2018).
Therefore, the present study was designed to investigate the physiological and perceptional responses to shuttle running with three directional modes (i.e., forward, forward-backward, and lateral running). We hypothesized that: (1) directional mode had significant effects on the physiological and perceptional responses to shuttle running, and (2) the physiological and perceptional responses to shuttle running were highest in lateral shuttle running, and lowest in forward shuttling running.
Materials and Methods
Participants
Twenty-eight male college students volunteered to participate in this study. All participants had more than 2 years (up to 10 years) soccer training experience and spent more than 6 h on physical exercises every week in the last 4 weeks. A Physical Activity Readiness Questionnaire (PAR-Q) was used to exclude subjects with cardiovascular or musculoskeletal problems. Four of them were not included in the final analysis because of data loss or injury problems during the whole procedures. Twenty-four eligible subjects had the following characteristics (mean ± SD): age = 19.6 ± 0.8 years, height = 175.4 ± 6.2 cm, weight = 67.1 ± 5.9 kg, VO2max = 60.3 ± 4.1 ml⋅kg–1⋅min–1. All the subjects included were in good health, not taking medications known to influence metabolic or cognitive functions.
Experimental Design
The experiment consisted of a maximal oxygen uptake (VO2max) test and three shuttle running tests, including forward shuttle running, forward-backward shuttle running, and lateral shuttle running. HR, VO2, [La–]peak, and RPE were recorded to reflect the physiological and perceptional responses of the exercises. Subjects were also instructed to refrain from high intensity and strenuous exercises during the last 24 h ahead of each test, to standardize their food and drink intake (no caffeine), as well as to have a regular sleep on test days. The purpose, procedures and risks of this study were explained to the participants both verbally and in written format. Prior to the formal tests, all participants provided informed consent document after understanding the whole tests and got familiar with the test protocols and experimental instruments. This study was approved by the Research Ethical Committee of Shanghai University of Sport (approval number: 102772020RT085).
Procedures
Maximal Oxygen Uptake Test
The subject’s VO2max was determined with an incremental test on a treadmill (Saturn® 300/100 r, H/P/Cosmos, Nussdorf-Traunstein, Germany) in a laboratory with the ambient temperature ranged from 20.3 to 23.5°C and the atmosphere pressure ranged from 100.8 to 102.7 kPa. The speed was increased by 1 km⋅h–1 every minute from an initial intensity of 8 km⋅h–1 and 1% gradient (Bravo et al., 2008; Kramer et al., 2018). HR and respiratory gas exchange were under real-time supervision with a HR monitor (T31, Polar-Electro, Kempele, Finland) and a portable gas analyzer (MetaMax 3B, Cortex, Leipzig, Germany). The gas analyzer was calibrated with ambient air (assumed concentration of 20.94% O2 and 0.03% CO2) and a standard gas of known composition (O2: 15.00%, CO2: 5.00%) for gas calibration, as well as a 3-L syringe for volume calibration according to the manufacturer’s instructions before the test. The subject’s rating of perceived exertion was assessed using Borg 6–20 scale (Borg, 1998) when the test was stopped. A capillary blood sample was collected from subject’s earlobe at the beginning of the 1st, 3rd, 5th, 7th, and 10th minute during the recovery. [La–] was analyzed with EKF lactate analyzer (Biosen C_line, EKF Diagnostic, Magdeburg, Germany). The test was immediately terminated if the subject waved hand to the researcher that indicated exhaustion. Other standards for termination were: (1) touching the handrail, (2) unsteady gait, and (3) failed to keep himself in the first half of the treadmill. Heart rate was recorded simultaneously with the respiratory data, which was collected continuously (mobile mean value of three consecutive breaths) using the breath-by-breath method. HRmax was the maximal value obtained during the test. VO2max was defined as the maximum moving average value in 30-s window (Stevens et al., 2015) and expressed in milliliters of oxygen per kilogram per minute (ml⋅kg–1⋅min–1). To ensure the VO2max was reached, the participant should meet at least 3 of the 5 following criteria (Howley et al., 1995; Midgley et al., 2007; Hatamoto et al., 2014; Ázara et al., 2017): (1) a VO2 plateau despite increasing speed (ΔVO2 between two consecutive stages < 2.1 ml⋅kg−1⋅min−1), (2) HR ≥ 95% individual age-predicted maximal HR (HRmax = 220 –age), (3) RER (respiratory exchange ratio, the ratio between the expired CO2 and the amount of O2 being consumed) ≥ 1.10, (4) RPE ≥ 18, and (5) [La–] ≥ 8 mmol⋅L−1. If not, the subject should take another VO2max test with same procedures after at least 48 h.
Shuttle Running Tests
Forward, forward-backward, and lateral shuttle running tests were conducted by each subject in a random order on separate days. For each trial, participants performed a 180°COD after covering 5 m at a mean speed of 6 km⋅h–1 and repeated this continuously for 5 min in order to reach a metabolic steady state (Ciprandi et al., 2018; Zago et al., 2018). The selection of 6 km⋅h−1 was because participants could hardly complete the lateral shuttle running at higher speeds for 5 min, as we have investigated in pilot study. Conditions took place at the same time (± 0.5 h) of day. Each participant was required to wear the same running shoes and similar suits for the tests. All protocols were conducted on the same flat synthetic rubber indoor course where ambient temperature ranged from 19 to 23°C and atmosphere pressure ranged from 101.7 to 103.2 kPa during the shuttle running tests.
Prior to starting each test, participant performed a 10-min warm-up including 5-min jogging and 5-min dynamic stretches as dictated by the researcher. Then, instruments which had been calibrated according to the manufacturers’ instructions were assembled and checked. During all the tests, running pace was governed by a metronomic device. The audio signal beeped every 3 s and indicated the moment that the participants needed to change direction and the supporting foot should pass over the turning line. Right foot and left foot were alternatively used to change direction in order to avoid unilateral fatigue (Ciprandi et al., 2018; Zago et al., 2018). Each participant chose his own preferred stride length and stride frequency because it has been demonstrated that naturally selected stride length-frequency combination resulted in the lowest oxygen consumption (Hogberg, 1952; Cavanagh and Williams, 1982; Mercer et al., 2008). In forward shuttle running, subjects were instructed to change direction with a sidestep technique. Body was accelerated toward the direction opposite of the push-off leg (Schot et al., 1995; Cochrane et al., 2007; Potter et al., 2014) and the pivoting foot should land perpendicularly to the running direction (Zago et al., 2018). Since participants faced the same direction without any body rotation throughout the forward-backward or lateral shuttle running, there was no special technical requirement in these two conditions. A researcher supervised the whole tests and provided oral instruction if the participant failed to pass over the turning line or follow the pace.
Heart rate and respiratory gas exchange were continuously measured with a HR monitor (T31, Polar-Electro, Kempele, Finland) and a portable gas analyzer (MetaMax 3B, Cortex, Leipzig, Germany). HR was determined as the mean of HR in the last 2 min (Hatamoto et al., 2013). The average value of VO2 (ml⋅kg–1⋅min–1) in the last 2 min (representing a steady state) was calculated to represent the VO2 of this condition (Buchheit et al., 2011; Hatamoto et al., 2013; Zamparo et al., 2014, 2015, 2016). Relative HRmax and relative VO2max were represented as %HRmax and %VO2max, which meant the ratio (in percentage form) of HR to individual HRmax and VO2 to individual VO2max, respectively. When the test was completed, the participant’s RPE [according to 6–20 Borg’s scale, Borg (1998)] was immediately inquired and recorded. During the recovery in sitting position, a capillary blood sample (10 μL) was collected from the participant’s earlobe in the 1st, 3rd, 5th, and 7th minute after each test (Li et al., 2018) and [La–]peak was used to analyze in this study. Since the data were compared within subject and we assumed that the resting metabolic rate fluctuated within a very narrow range during the test days, the pre-test values were not considered (Buglione and di Prampero, 2013; Zamparo et al., 2014).
Statistics
Data analyses were conducted with an academic statistics software (SPSS 25.0, IBM, Armonk, New York, NY, United States). Descriptive statistics were presented as mean ± SD within 95% confidence interval (95% CI) for all variables. The normality of each dependent variable was examined by Shapiro-Wilk test. When the normality distribution was rejected, the data were transformed by taking the natural logarithm to allow statistical comparisons that assume a normal distribution (Buchheit et al., 2011). Back-transformed values were presented in the text. The effect of running modes on the variables investigated in this study was assessed using a one-way analysis of variance (ANOVA) for repeated measures. Greenhouse–Geisser correction was performed if the sphericity assumption was violated. When significant effects were found, Bonferroni post hoc tests were applied to determine the differences between running modes. Effect sizes (Cohen’s d) of post hoc tests were calculated for the magnitude of differences. Threshold values were ≤ 0.20 (trivial), > 0.20–0.50 (small), > 0.50–0.80 (moderate), and > 0.80 (large), respectively. The level of statistical significance was set at p < 0.05.
Results
Physiological and perceptional responses to three modes of shuttle running are shown in Table 1. Statistical results revealed that running mode had significant effects on HR (F = 72.761, P < 0.001, η2p = 0.760), %HRmax (F = 75.896, P < 0.001, η2p = 0.767), VO2 (F = 110.320, P < 0.001, η2p = 0.827), %VO2max (F = 108.883, P < 0.001, η2p = 0.826), [La–]peak (F = 55.529, P < 0.001, η2p = 0.707), and RPE (F = 26.268, P < 0.001, η2p = 0.533).
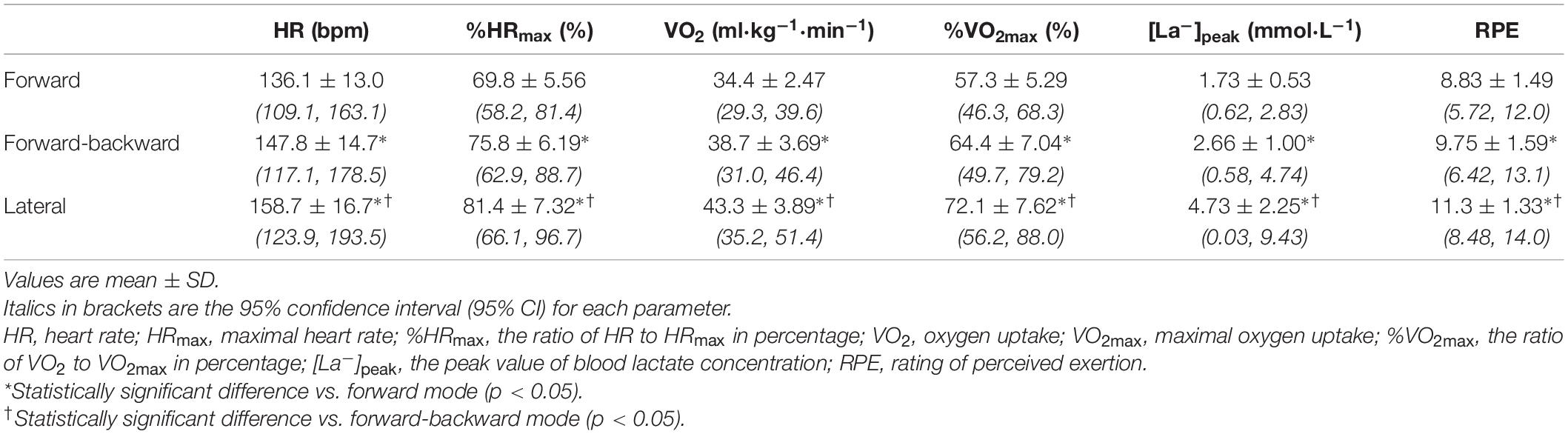
Table 1. Physiological and perceptional responses to three modes of 5-m shuttle running at 6 km⋅h–1.
Bonferroni post hoc tests showed that all variables investigated in this study were significantly different between conditions. HR, %HRmax, VO2, %VO2max, [La–]peak, and RPE in forward-backward shuttle running were higher than in forward exercise. Lateral shuttle running yielded the highest responses. The effect sizes indicated large magnitude in the differences of all variables between conditions except the difference of RPE between forward and forward-backward shuttle running. The individual physiological and perceptional responses to the same condition varied much between subjects (see Figure 1).
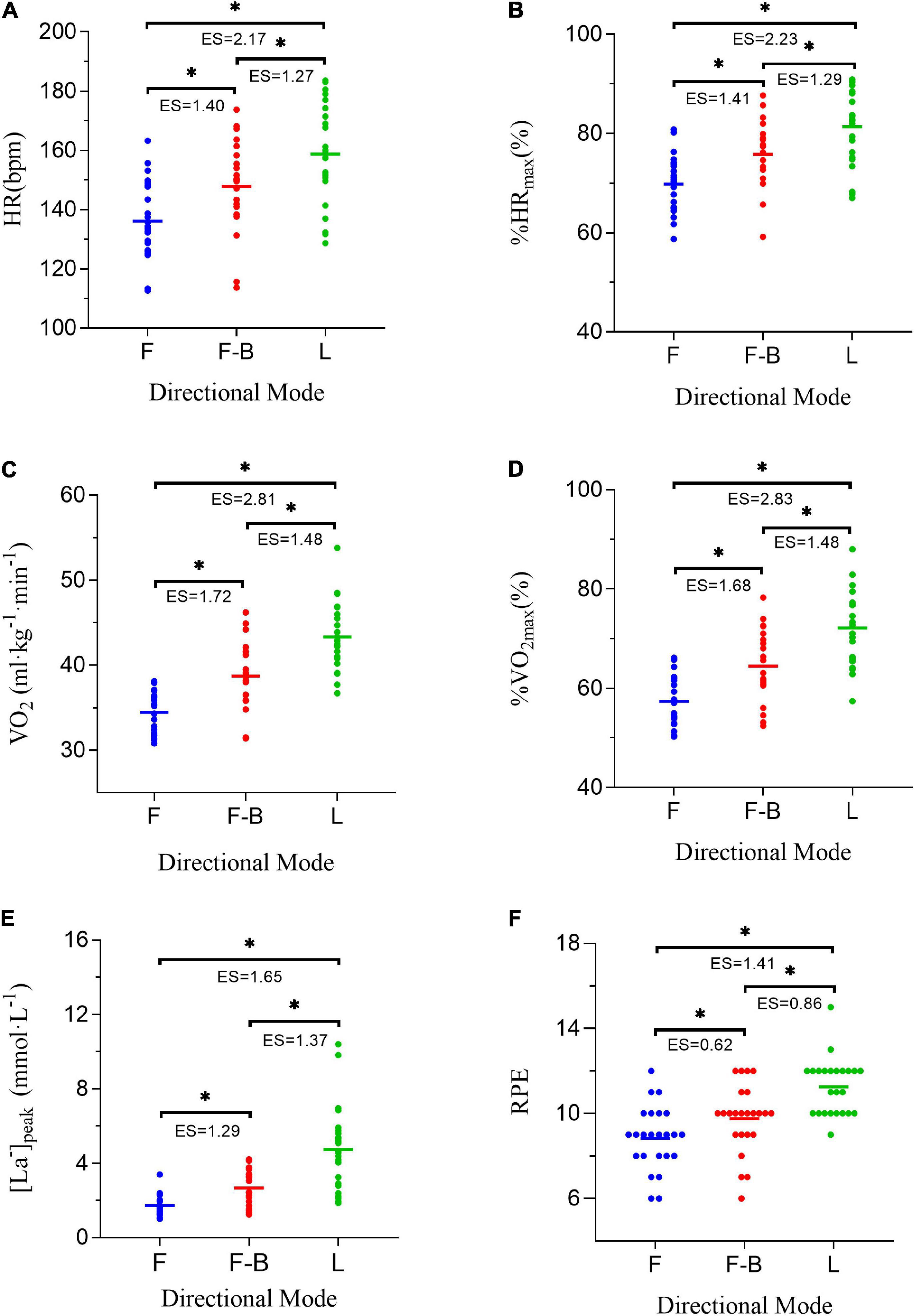
Figure 1. Physiological and perceptional responses to three modes of shuttle running: HR (A), %HRmax (B), VO2 (C), %VO2max (D), [La–]peak (E), RPE (F). HR, heart rate; HRmax, maximal heart rate; %HRmax, the ratio of HR to HRmax in percentage; VO2, oxygen uptake; VO2max, maximal oxygen uptake; %VO2max, the ratio of VO2 to VO2max in percentage; [La–]peak, the peak value of blood lactate concentration; RPE, rating of perceived exertion; F, forward shuttle running, F-B, forward-backward shuttle running; L, lateral shuttle running; ES, effect size. *Statistically significant difference (p < 0.05).
Discussion
The present study evaluated the HR, %HRmax, VO2, %VO2max, [La–]peak, and RPE of 5-m forward, forward-backward, and lateral shuttle running at the speed of 6 km⋅h–1. To our knowledge, it is probably the first study to investigate the physiological and perceptional responses to different directional modes of shuttle running. The results of this study showed that both lateral and forward-backward shuttle running elicited greater physiological and perceptional responses than forward shuttle running at the selected speed. All variables investigated in this study (HR, %HRmax, VO2, %VO2max, [La–]peak, and RPE) were significantly higher in forward-backward shuttle running than in forward shuttle running and the values in lateral shuttle running were significantly higher than the other two conditions. The findings verified our hypothesis that both lateral and forward-backward shuttle running could produce greater physiological demands compared with forward shuttle running. It indicated that to complete the same distance shuttle running at the same speed, running forwards was more efficient than running backwards or running sideways. The outcome was not surprising. Generally, humans are used to running or walking in the forward direction. Backward and lateral movements are applied in daily activities or sport exercises at times. With the alterations of visual field and neuromuscular activation, running backwards and running laterally are considered as unaccustomed tasks for humans compared with running forwards (Oyeyemi et al., 2017), especially performed continuously. As pointed by Schwane et al. (Schwane et al., 1983; Flynn et al., 1994), to complete a unfamiliar activity may require greater motor unit recruitments, resulting in increased energy cost.
Plenty of evidence has shown that forward shuttle running required higher physiological demands than constant straight-forward running at the same speed (Osgnach et al., 2010; Zago et al., 2018). So far, however, no published study has investigated the physiological responses of shuttle running involved backward and lateral locomotion. Despite all this, several studies concerning the physiological demands and mechanical characteristics about different directional modes of straight running could help us to understand the influence of running mode on the physical responses. In an early study, Reilly and Bowen (1984) determined the energy expenditure and perceptual fatigue of three directional modes (forward, backward, and lateral) of straight running on a treadmill at 5, 7, and 9 km⋅h–1. The results showed that both backward running and lateral running produced significantly greater energy demand and higher RPE than forward running at the investigated speeds. In another study (Williford et al., 1998), it was observed that HR and VO2 were significantly higher in lateral running and backward running than forward running. These findings were congruent with the results in the present study. However, the results of comparing backward running and lateral running were inconsistent. Reilly and Bowen (1984) reported that neither energy expenditure nor RPE was significantly different between backward and lateral running. Williford et al. (1998) found that the differences between backward running and lateral running were speed dependent. HR and VO2 were significantly higher in lateral running at the speed of 3 miles per hour (mph), while no significant difference was found at 5 mph. Besides, differences of the HR and VO2 between running modes appeared to dramatically greater at higher speed in these two studies. It indicated that speed and running mode may have an interaction effect on the physiological responses in shuttle running. In our study, lateral shuttle running elicit significantly higher physiological responses than forward-backward mode. Nevertheless, it was difficult to directly compare these findings because forward-backward shuttle running contained forward components. Further researches are needed to determine whether the physiological responses in lateral running is higher than running backwards.
In addition, Mayo et al. (2004) observed that the physiological responses to forward-motion and backward-motion exercise were similar while the lateral-motion exercise elicit significant greater VO2 and HR with the similar RER and RPE relative to forward and backward exercise at self-selected intensities. The findings indicated that lateral-motion exercise was performed with higher metabolism at the perceived similar intensities. It should be noted that forward and backward exercise were conducted on a treadmill while lateral-motion exercise was performed on a slideboard. We supposed that the physiological responses to running in different directions were similar at the same perceived intensities. However, the physiological responses to lateral running at the self-selected intensity may need further research.
Forward running and backward running are accomplished in sagittal plane but in opposite direction. In these two kinds of running, the total lower limb muscle work is similar at the same speed, but the muscles are activated concentrically and eccentrically with quite different sequences and extents of activation, accompanied by different ankle, knee, and hip joint moment and power output (Flynn et al., 1994). During forward running, ankle plantar flexors produced more power and played an important role in propulsion, whereas knee extensors were the primary source of propulsion in backward running because of the higher moment and power generation (DeVita and Stribling, 1991). Hip moment and power patterns of forward running and backward running were opposite in direction but similar in magnitude (DeVita and Stribling, 1991). Unlike forward and backward running, lateral running is performed in the frontal plane with prominent hip abduction-adduction and relatively smaller flexion-extension angles of hip, knee, and ankle. Thus, the groups of muscles activated in lateral movement and the magnitude of activation must be distinct from the forward or backward mode. Different neuromuscular firing patterns may be one of the explanations to the distinct physiological and perceptional responses in different modes of shuttle running. The differences of kinetics may be another reason for the different physical responses. Wright and Weyand (2001) investigated the application of ground force during forward running and backward running and noted that metabolic rates during running were associated with the rates of ground force application and the volume of muscle activated to produce support forces against the ground. Unfortunately, few researches have investigated the kinematic parameters and muscle activities of lateral running.
The changes in stride length and stride frequency may also contribute to the different physiological demands. According to the previous studies, subjects employed shorter stride length and higher stride frequency during backward running than during forward running at the same speed. These differences became larger at higher speeds (Flynn et al., 1994; Williford et al., 1998; Wright and Weyand, 2001). During lateral running, stride length was shorter and stride frequency was higher than forward running and backward running (Williford et al., 1998). Changes of stride length and stride frequency in different running modes may be one of reasons that caused distinct physiological and perceptional responses.
In the present study, there were some limitations should be discussed. First, the forward-backward shuttle running mode, other than backward mode, was applied in this study, resulting in the difficulty to compare the effects of backward and lateral modes. Nevertheless, backward shuttle running was difficult to performed and seldom happened in the match. Forward-backward shuttle running was much closer to the practice and often used in sport training. Second, the values at rest were not collected with the assumption of the baseline values of the same subject fluctuated within a narrow range during the test days and the random testing order could offset the variations. In some studies, authors assumed that all participants had the same baseline values, such as 3.5 ml⋅kg–1⋅min–1 (Buglione and di Prampero, 2013) or 5 ml⋅kg–1⋅min–1 for resting VO2 and 1 mmol⋅L–1 for resting [La–] (Buglione and di Prampero, 2013; Zamparo et al., 2014, 2015), which were subtracted from the total values to calculate the energy cost during locomotion. Moreover, the large differences of the physiological and perceptional responses between running modes demonstrated that the baseline values could be safely neglected in this study. Therefore, the absence of baseline values had little impact on our conclusions. Third, the present study only examined the physiological and perceptional responses to three modes of shuttle running at the speed of 6 km⋅h–1 in 5-m distance. However, shuttle running could be performed at various combinations of speed and distance. Other conditions were not involved in this preliminary study.
Conclusion
In conclusion, the physiological and perceptional responses in 5-m shuttle running at a mean speed of 6 km⋅h–1 depended on the running directional modes, with the responses highest in lateral shuttle running, and lowest in forward shuttle running. When utilized in home-based exercise, shuttle running could stimulate the cardiorespiratory and musculoskeletal system to different extents by performing with different directional modes. This preliminary study only investigated HR, %HRmax, VO2, %VO2max, [La–]peak, and RPE in forward, forward-backward and lateral 5-m shuttle running at 6 km⋅h–1. Further researches can be designed to explore more physiological and biomechanical variables at other intensities with various speeds and shuttle distances in order to determine the underlying reasons for these differences.
Data Availability Statement
The raw data supporting the conclusions of this article will be made available by the authors, without undue reservation.
Ethics Statement
The studies involving human participants were reviewed and approved by the Research Ethical Committee of Shanghai University of Sport. The patients/participants provided their written informed consent to participate in this study.
Author Contributions
YL and BL contributed to the study conception. XW, CG, and BL completed the study design. XW, GZ, LH, and MH performed the material preparation and the data collection. CG completed the data analysis and the first draft of the manuscript. YL and GN contributed the critical revision of the manuscript. YL acquired the funding support and facilitated the whole process. All authors have read and approved the final manuscript and agreed to be accountable for all aspects of the work.
Funding
This study was funded by the Science and Technology Commission of Shanghai Municipality (18080503400) and China Institute of Sport Science (Basic 20-37).
Conflict of Interest
The authors declare that the research was conducted in the absence of any commercial or financial relationships that could be construed as a potential conflict of interest.
Publisher’s Note
All claims expressed in this article are solely those of the authors and do not necessarily represent those of their affiliated organizations, or those of the publisher, the editors and the reviewers. Any product that may be evaluated in this article, or claim that may be made by its manufacturer, is not guaranteed or endorsed by the publisher.
Acknowledgments
We sincerely appreciate the subjects’ participation. We also thank Yongdi Zou, Xinxin Wang, Meixia Chen, Chao Bian, and Zhili Chen for their help in this study.
References
Ashton, R. E. M., and Twist, C. (2015). Number of directional changes alters the physiological, perceptual, and neuromuscular responses of netball players during intermittent shuttle running. J. Strength Cond. Res. 29, 2731–2737. doi: 10.1519/JSC.0000000000000933
Ázara, H., Farinatti, P., Midgley, A., Vasconcellos, F., Vigário, P., and Cunha, F. A. (2017). Standardized MET value underestimates the energy cost of treadmill running in men. Int. J. Sports Med. 38, 890–896. doi: 10.1055/s-0043-115739
Bravo, D., Impellizzeri, F., Rampinini, E., Castagna, C., Bishop, D., and Wisloff, U. (2008). Sprint vs. interval training in football. Int. J. Sports Med. 29, 668–674. doi: 10.1055/s-2007-989371
Buchheit, M., Haydar, B., Hader, K., Ufland, P., and Ahmaidi, S. (2011). Assessing running economy during field running with changes of direction: application to 20 m shuttle runs. Int. J. Sports Physiol. Perform. 6, 380–395. doi: 10.1123/ijspp.6.3.380
Buglione, A., and di Prampero, P. E. (2013). The energy cost of shuttle running. Eur. J. Appl. Physiol. 113, 1535–1543. doi: 10.1007/s00421-012-2580-9
Cavanagh, P., and Williams, K. (1982). The effect of stride length variation on oxygen uptake during distance running. Med. Sci. Sports Exerc. 14, 30–35. doi: 10.1249/00005768-198201000-00006
Ciprandi, D., Lovecchio, N., Piacenza, M., Limonta, E., Esposito, F., Sforza, C., et al. (2018). Energy cost of continuous shuttle running: comparison of 4 measurement methods. J. Strength Cond. Res. 32, 2265–2272. doi: 10.1519/JSC.0000000000002366
Cochrane, J. L., Lloyd, D. G., Buttfield, A., Seward, H., and McGivern, J. (2007). Characteristics of anterior cruciate ligament injuries in Australian football. J. Sci. Med. Sport 10, 96–104. doi: 10.1016/j.jsams.2006.05.015
Dellal, A., Keller, D., Carling, C., Chaouachi, A., Wong, D. P., and Chamari, K. (2010). Physiologic effects of directional changes in intermittent exercise in soccer players. J. Strength Cond. Res. 24, 3219–3226. doi: 10.1519/JSC.0b013e3181b94a63
DeVita, P., and Stribling, J. (1991). Lower extremity joint kinetics and energetics during backward running. Med. Sci. Sports Exerc. 23, 602–610.
Flynn, T. W., Connery, S. M., Smutok, M. A., Zeballos, R. J., and Weisman, I. M. (1994). Comparison of cardiopulmonary responses to forward and backward walking and running. Med. Sci. Sports Exerc. 26:89. doi: 10.1249/00005768-199401000-00015
Hatamoto, Y., Yamada, Y., Higaki Kiyonaga, A., Tanaka, H., and Fujii, T. (2013). A novel method for calculating the energy cost of turning during running. Open Access J. Sports Med. 4, 117–122. doi: 10.2147/OAJSM.S39206
Hatamoto, Y., Yamada, Y., Sagayama, H., Higaki, Y., Kiyonaga, A., and Tanaka, H. (2014). The relationship between running velocity and the energy cost of turning during running. PLoS One 9:e81850. doi: 10.1371/journal.pone.0081850
Haydar, B., Haddad, H. A., Ahmaidi, S., and Buchheit, M. (2011). Assessing inter-effort recovery and change of direction ability with the 30-15 intermittent fitness test. J. Sports Sci. Med. 10:346. doi: 10.2519/jospt.2011.0413
Hogberg, P. (1952). How do stride length and stride frequency influence the energy-output during running? Arbeitsphysiologie 14, 437–441. doi: 10.1007/BF00934423
Howley, E. T., Bassett, D. R., and Welch, H. G. (1995). Criteria for maximal oxygenuptake. Med. Sci. Sports Exerc. 27, 1292–1301. doi: 10.1249/00005768-199509000-00009
Ishii, H., Nagano, Y., Ida, H., Fukubayashi, T., and Maruyama, T. (2011). Knee kinematics and kinetics during shuttle run cutting: comparison of the assessments performed with and without the point cluster technique. J. Biomech. 44, 1999–2003. doi: 10.1016/j.jbiomech.2011.05.001
Kramer, M., Du Randt, R., Watson, M., and Pettitt, R. W. (2018). Oxygen uptake kinetics and speed-time correlates of modified 3-minute all-out shuttle running in soccer players. PLoS One 13:e0201389. doi: 10.1371/journal.pone.0201389
Léger, L. A., Mercier, D., Gadoury, C., and Lambert, J. (1988). The multistage 20 metre shuttle run test for aerobic fitness. J. Sports 6, 93–101.
Li, Y., Niessen, M., Chen, X., and Hartmann, U. (2018). Method-induced differences of energy contributions in adolescent female kayaking. Int. J. Sports Physiol. Perform. 13, 9–13. doi: 10.1123/ijspp.2016-0491
Masumoto, K., Hamada, A., Tomonaga, H., Kodama, K., Amamoto, Y., Nishizaki, Y., et al. (2009). Physiological and perceptual responses to backward and forward treadmill walking in water. Gait Posture 29, 199–203. doi: 10.1016/j.gaitpost.2008.08.008
Maurus, P., Asmussen, M. J., Cigoja, S., Nigg, S. R., and Nigg, B. M. (2019). The submaximal lateral shuffle test: a reliability and sensitivity analysis. J. Sports Sci. 37, 2066–2074. doi: 10.1080/02640414.2019.1620988
Mayo, J., Lyons, B., Honea, K., Alvarez, J., and Byrum, R. (2004). Comparison of forward-, backward-, and lateral-motion exercise at self-selected intensities. J. Sport Rehabil. 13, 67–74. doi: 10.1123/jsr.13.1.67
Mercer, J., Dolgan, J., Griffin, J., and Bestwick, A. (2008). The physiological importance of preferred stride frequency during running at different speeds. J. Exerc. Physiol. Online 11, 26–32.
Midgley, A. W., McNaughton, L. R., Polman, R., and Marchant, D. (2007). Criteria for determination of maximal oxygen uptake: a brief critique and recommendations for future research. Sports Med. 37, 1019–1028. doi: 10.2165/00007256-200737120-00002
Osgnach, C., Poser, S., Bernardini, R., Rinaldo, R., and Di Prampero, P. E. (2010). Energy cost and metabolic power in elite soccer: a new match analysis approach. Med. Sci. Sports Exerc. 42, 170–178. doi: 10.1249/MSS.0b013e3181ae5cfd
Oyeyemi, A. Y., Lawan, A., Akpeli, G. J., and Oyeyemi, A. L. (2017). Comparison of cardiovascular responses following self-selected maximal effort in forward, backward and sideways walking. Arch. Med. Biomed. Res. 3:67. doi: 10.4314/ambr.v3i2.3
Potter, D., Reidinger, K., Szymialowicz, R., Martin, T., and Garbalosa, J. C. (2014). Sidestep and crossover lower limb kinematics during a prolonged sport-like agility test. Int. J. Sports Phys. Ther. 9, 617–627.
Reilly, R., and Bowen, T. (1984). Exertional costs of changes in directional modes of running. Percept. Mot. Skills 58, 149–150. doi: 10.2466/PMS.58.1.149-150
Schot, P., Dart, J., and Schuh, M. (1995). Biomechanical analysis of two change-of-direction maneuvers while running. J. Orthop. Sports Phys. Ther. 22, 254–258. doi: 10.2519/jospt.1995.22.6.254
Schwane, J. A., Johnson, S. R., Armstrong, R. B., and Vandenakker, C. B. (1983). Delayed-onset muscular soreness and plasma CPK and LDH activities after downhill running. Med. Sci. Sports Exerc. 15, 51–56. doi: 10.1249/00005768-198315010-00010
Stevens, T. G. A., De Ruiter, C. J., Van Maurik, D., Van Lierop, C. J. W., Savelsbergh, G. J. P., and Beek, P. J. (2015). Measured and estimated energy cost of constant and shuttle running in soccer players. Med. Sci. Sports Exerc. 47, 1219–1224. doi: 10.1249/MSS.0000000000000515
Stewart, P. F., Turner, A. N., and Miller, S. C. (2014). Reliability, factorial validity, and interrelationships of five commonly used change of direction speed tests: reliability of field-based CODS tests. Scand. J. Med. Sci. Sports 24, 500–506. doi: 10.1111/sms.12019
Williford, H. N., Olson, M. S., Gauger, S., Duey, W. J., and Blessing, D. L. (1998). Cardiovascular and metabolic costs of forward, backward, and lateral motion. Med. Sci. Sports Exerc. 30, 1419–1423. doi: 10.1097/00005768-199809000-00011
Wright, S., and Weyand, P. G. (2001). The application of ground force explains the energetic cost of running backward and forward. J. Exp. Biol. 204, 1805–1815. doi: 10.1242/jeb.204.10.1805
Zadro, I., Sepulcri, L., Lazzer, S., Fregolent, R., and Zamparo, P. (2011). A protocol of intermittent exercise (shuttle runs) to train young basketball players. J. Strength Cond. Res. 25, 1767–1773. doi: 10.1519/JSC.0b013e3181da85d1
Zago, M., David, S., Bertozzi, F., Gatti, A., Salaorni, F., Tarabini, M., et al. (2021). Fatigue induced by repeated changes of direction in Élite Female Football (Soccer) players: impact on lower limb biomechanics and implications for ACL injury prevention. Front. Bioeng. Biotechnol. 9:586. doi: 10.3389/fbioe.2021.666841
Zago, M., Esposito, F., Bertozzi, F., Tritto, B., Rampichini, S., Galvani, C., et al. (2019). Kinematic effects of repeated turns while running. Eur. J. Sport Sci. 19, 1072–1081. doi: 10.1080/17461391.2019.1578416
Zago, M., Esposito, F., Rausa, G., Limonta, E., Corrado, F., Rampichini, S., et al. (2018). Kinematic algorithm to determine the energy cost of running with changes of direction. J. Biomech. 76, 189–196. doi: 10.1016/j.jbiomech.2018.05.028
Zamparo, P., Bolomini, F., Nardello, F., and Beato, M. (2015). Energetics (and kinematics) of short shuttle runs. Eur. J. Appl. Physiol. 115, 1985–1994. doi: 10.1007/s00421-015-3180-2
Zamparo, P., Pavei, G., Nardello, F., Bartolini, D., Monte, A., and Minetti, A. E. (2016). Mechanical work and efficiency of 5 + 5 m shuttle running. Eur. J. Appl. Physiol. 116, 1911–1919. doi: 10.1007/s00421-016-3443-6
Keywords: shuttle runs, change of direction, running modes, physiological demands, oxygen consumption
Citation: Gao C, Wang X, Zhang G, Huang L, Han M, Li B, Nassis GP and Li Y (2022) Comparison of Physiological and Perceptional Responses to 5-m Forward, Forward-Backward, and Lateral Shuttle Running. Front. Physiol. 12:780699. doi: 10.3389/fphys.2021.780699
Received: 21 September 2021; Accepted: 13 December 2021;
Published: 17 February 2022.
Edited by:
Emiliano Cè, University of Milan, ItalyReviewed by:
Marta Borrelli, Università degli Studi di Milano, ItalyFilippo Bertozzi, University of Milan, Italy
Copyright © 2022 Gao, Wang, Zhang, Huang, Han, Li, Nassis and Li. This is an open-access article distributed under the terms of the Creative Commons Attribution License (CC BY). The use, distribution or reproduction in other forums is permitted, provided the original author(s) and the copyright owner(s) are credited and that the original publication in this journal is cited, in accordance with accepted academic practice. No use, distribution or reproduction is permitted which does not comply with these terms.
*Correspondence: Yongming Li, liyongming@sus.edu.cn