- 1Department of Physiology, Ribeirão Preto School of Medicine, São Paulo University, Ribeirão Preto, Brazil
- 2Department of Physiological Sciences, Center of Biological Sciences, Federal University of Santa Catarina, Florianópolis, Brazil
The dorsal raphe (DR) nucleus is involved in a myriad of physiological functions, such as the control of sleep-wake cycle, motivation, pain, energy balance, and food intake. We have previously demonstrated that in ad libitum fed rats the intra-DR administration of phenylephrine, an α-1 receptor agonist, does not affect food intake, whereas clonidine, an α-2 receptor agonist, potently stimulates food intake. These results indicated that in fed rats an increased adrenergic tonus blocked food intake, since the activation of α-2 auto-receptors, which decreases pre-synaptic release of adrenaline/noradrenaline, affected food intake. Thus, in this study we assessed whether the response to adrenergic stimuli would differ after overnight fasting, a situation of low adrenergic activity in the DR. Intra-DR administration of adrenaline and noradrenaline blocked food intake evoked by overnight fasting. Similarly, phenylephrine administration decreased hunger-induced food intake. These changes in food intake were accompanied by changes in other behaviors, such as increased immobility time and feeding duration. On the other hand, intra-DR administration of clonidine did not affect food-intake or associated behaviors. These results further support the hypothesis that in fed animals, increased adrenergic tonus in DR neurons inhibiting feeding, while in fasted rats the adrenergic tonus decreases and favors food intake. These data indicate a possible mechanism through which adrenergic input to the DRN contributes to neurobiology of feeding.
Introduction
The raphe nuclei are distinct brain loci composed of groups of neurons located along the brainstem that have been implicated in many physiological functions such as the control of the sleep-wake cycle, motivation, pain, energy balance, and food intake (Berger et al., 2009; Pytliak et al., 2011; Schneeberger et al., 2019). One of these nuclei is the dorsal raphe nucleus (DR) which is located beneath the cerebral aqueduct and constitutes a collection of neurons with distinct morphology, projections, and neurochemical phenotypes (Adell et al., 2002). The DR sends neuronal projections to many forebrain structures, including a robust innervation to the hypothalamus, an important area that influences food intake (Muzerelle et al., 2016; Ren et al., 2019).
Several studies indicate that the DR has a pivotal role in feeding behavior (Stachniak et al., 2014; Anderberg et al., 2017; Bruschetta et al., 2020). Immunohistochemical studies revealed that food intake triggers neural activity in this nucleus (Wu et al., 2014). Moreover, optogenetic activation of specific GABAergic DR neurons has been shown to increase food intake, while activation of glutamatergic DR neurons suppresses feeding (Nectow et al., 2017). The DR is the main source of serotonin (5-HT) in the central nervous system, a neurotransmitter associated with satiety (Blundell and Latham, 1979; Blundell, 1991). Pharmacological approaches demonstrated that injection of 5-HT or 8-OH-DPAT, a 5-HT1A receptor agonist, into the DR induces feeding in satiated rats. These effects were attributed to the activation of inhibitory DR 5-HT1A somatodendritic autoreceptors, which may regulate 5-HT release (Hutson et al., 1986; Fletcher and Davies, 1990).
DR neural activity is also sensitive to endogenous catecholamines (Adell et al., 2002), receiving substantial noradrenergic input, especially from the commissural part of the nucleus of the solitary tract (A2) and the locus coeruleus (A6) (Peyron et al., 1996). High levels of mRNA for the α-1 adrenoceptors are present in the DR (Pieribone et al., 1994; Day et al., 1997) and in DR 5-HT neurons (Day et al., 2004). However, studies documented a only moderate presence of α-2 adrenoceptors in the DR (Unnerstall et al., 1985; Rosin et al., 1993; Talley et al., 1996) with no significant expression of α-2 adrenoceptor mRNA. These data suggest that these receptors are located presynaptically in noradrenergic terminals in this nucleus (McCune et al., 1993; Nicholas et al., 1993; Scheinin et al., 1994). Electrophysiological and microdialysis experiments disclosed that activation of α-1 adrenoceptors leads to an increase in local serotonin release and increase the firing rate of DR 5-HT neurons, while the activation of α-2 adrenoceptors leads to a decrease of serotonin release in this nucleus (Baraban and Aghajanian, 1980; Vandermaelen and Aghajanian, 1983; Bortolozzi and Artigas, 2003; Pudovkina et al., 2003). Moreover, lesions with DSP-4, a neurotoxin that impairs noradrenergic projections, abolishes the effects of local clonidine injection on 5-HT release, which suggests that, when administered into the DR, it acts predominantly on α-2 autoreceptors (Bortolozzi and Artigas, 2003).
In a recent study, we demonstrated that injection of α-2 agonist clonidine into the DR of satiated rats evoked hyperphagia (Flores et al., 2021). The feeding response induced by clonidine was similar to that found after noradrenaline or adrenaline injections into the DR, suggesting that this hyperphagia depends on α-2 adrenoceptors activation, while injection of a specific α-1 agonist did not affect food intake (Flores et al., 2021). Based on these previous data, we hypothesized that injection of α-adrenoceptor agonists into DR may also affect ingestive responses in fasted animals. To better understand the functional role of DR α-adrenoceptors in feeding behavior, this study aims to evaluate the effects of pharmacological manipulations of α-adrenergic agonists in the DR on food intake after fasting.
Materials and Methods
Animals
Male Wistar rats (weighing 270–300 g at the time of surgery) were group-housed in a temperature-controlled (21 ± 2°C) room, 12:12 light–dark cycle (lights on at 7:00 a.m.) with standard rodent chow and water available ad libitum. The animals were housed in groups of five per cage until the day of the experiments. The experimental procedures were conducted in compliance with the recommendations of the Ethics Committee for the use of Experimental Animals (CEUA) of the Federal University of Santa Catarina, SC, Brazil (CEUA protocol: PP0075). All efforts were made to minimize the number of animals used and their pain and discomfort.
Stereotaxic Surgery
Rats were anesthetized with a mixture of xylazine (13 mg kg–1) and ketamine (87 mg kg–1) injected intraperitoneally and underwent stereotaxic surgery for implantation of guide cannula for subsequent drug microinjection into the DR. The stainless steel guide cannula (30 G, 18 mm) was implanted about 2 mm dorsolateral to DR in order to not injure the DR, according to the coordinates (anteroposterior to bregma: + 7.9 mm, lateral: + 2.2 mm and dorsoventral:-4.8 mm) as described by Paxinos and Watson (2005). The cannula was anchored to the skull with dental cement and the implant stabilized with jeweler screws. A removable stylet was introduced to keep the cannula free from blockage until the day of the experiment. To prevent the rupture of the superior sagittal sinus and obstruction of the cerebral aqueduct during stereotaxic surgery, the stereotaxic bar was tilted 20°.
After surgery, the rats were housed in groups of five with free access to food and water for 1 week for post-surgical recovery.
Drugs and Injections
Drug or vehicle injections were performed using a needle (33G, 20 mm length) extending 2 mm beyond the ventral tip of the guide cannula and connected by polyethylene tubing (PE10) to a 1 μl SGE® syringe. The injected volumes (0.4 μl) were administered over 60 s, followed by a further 60 s with the needle still inside the guide cannula for better diffusion of the solution. The adrenergic agonists adrenaline (AD) and noradrenaline (NA) (Sigma Chemical Co., United States) were injected at doses of 6, 20, and 60 nmol. The α-1 adrenergic agonist phenylephrine (PHE) and the α-2 adrenergic agonist clonidine (CLO) (Tocris, United States) were injected at doses of 6 and 20 nmol. A sterile solution of 0.9% NaCl (VEH) was used as a vehicle for drug dilution or injected alone in the control groups. The drug doses used were based on previous studies from our research group (dos Santos et al., 2009; Mansur et al., 2010). Each animal received only one injection: a dose of one drug or the corresponding vehicle.
Experimental Procedures and Behavioral Assessment
After the post-surgical period, rats were habituated to the recording box for two consecutive days (60 min each day) before the experimental session. On the day before the experiment, 30 min before the light was turned off, food was removed from home cages. Rats remained approximately 14–16 h without access to food, but with free access to water. Immediately after microinjections, rats were placed in a recording box containing rodent pellet chow (Nuvilab CR-1, regular diet: 3.85 kcal/g, 10% kcal fat, 20% kcal protein, and 70% kcal carbohydrate; Nuvital, Brazil) in a feeder and water in a bottle placed outside the test box with a spout that projected through the wall of the box. The digital recording of the session (60 min) was initiated with a webcam perpendicularly located 60 cm above the recording chamber floor, and the amount of food and water intake was recorded by the difference between food or water weight at the beginning and at the end of the recording period. At the end of the recording period, any food that occasionally spilled on the cage floor was recovered and weighed with the food that remained in the feeder. The recording box has measures of length and width similar to those of the home cages (49 × 34 cm), but with higher sides (40 cm) to prevent escapes. A researcher blinded to the experimental groups was designated to analyze the video-recorded behavioral parameters using EthoLog 2.2.5 software (Ottoni, 2000).
The variables analyzed for food intake were the amount of chow consumed, the latency to start the behavior (in seconds), the frequency (number of times that the animal exhibited the feeding behavior), and the total duration of behavior (in seconds) during the 60 min of recording. For fluid intake, the amount of water drunk was analyzed. For non-ingestive behaviors (locomotion, grooming, rearing and immobility) the duration of these parameters was analyzed. The behavioral categories were defined in previous studies by Halford et al. (1998) and are described in Table 1. To avoid the influence of variation of the time during the day, all experimental procedures were started 1 h after the lights turned on, from 8:00 am to 10:00 am (light cycle).
Histological Confirmation of Drug Injection Site
At the end of each experiment, rats were deeply anesthetized with a mixture of xylazine (13 mg kg–1) and ketamine (87 mg kg–1) injected intraperitoneally and then transcardially perfused with saline (0.9% NaCl) followed by 10% formalin. Brains were removed, kept in formalin and sliced in coronal plane (50 μm) using a cryostat. Sections were stained with cresyl violet and the position of the injection was assessed using a light microscope. The Paxinos and Watson rat atlas (Paxinos and Watson, 2005) was used to verify the injection sites (DR). Only data from rats with cannula correctly placed in the DR were included in the study (approximately 85% of the total of implanted animals).
Statistical Analysis
Behavioral data were analyzed by one-way ANOVA followed by Tukey post hoc analysis. Correlations between the amount of food intake and the amount of water intake were performed using Pearson’s parametric correlation. Results are expressed as mean ± standard error of the mean (SEM). In all statistical analyses, only p < 0.05 were accepted as statistically significant. The statistical analysis was performed with the GraphPad Prism 6.01 software (GraphPad Software, Inc., 2012).
Results
All rats included in statistical analyses (n = 112) had injection sites confirmed to be in the DR by histological analysis (Figures 1A,B).
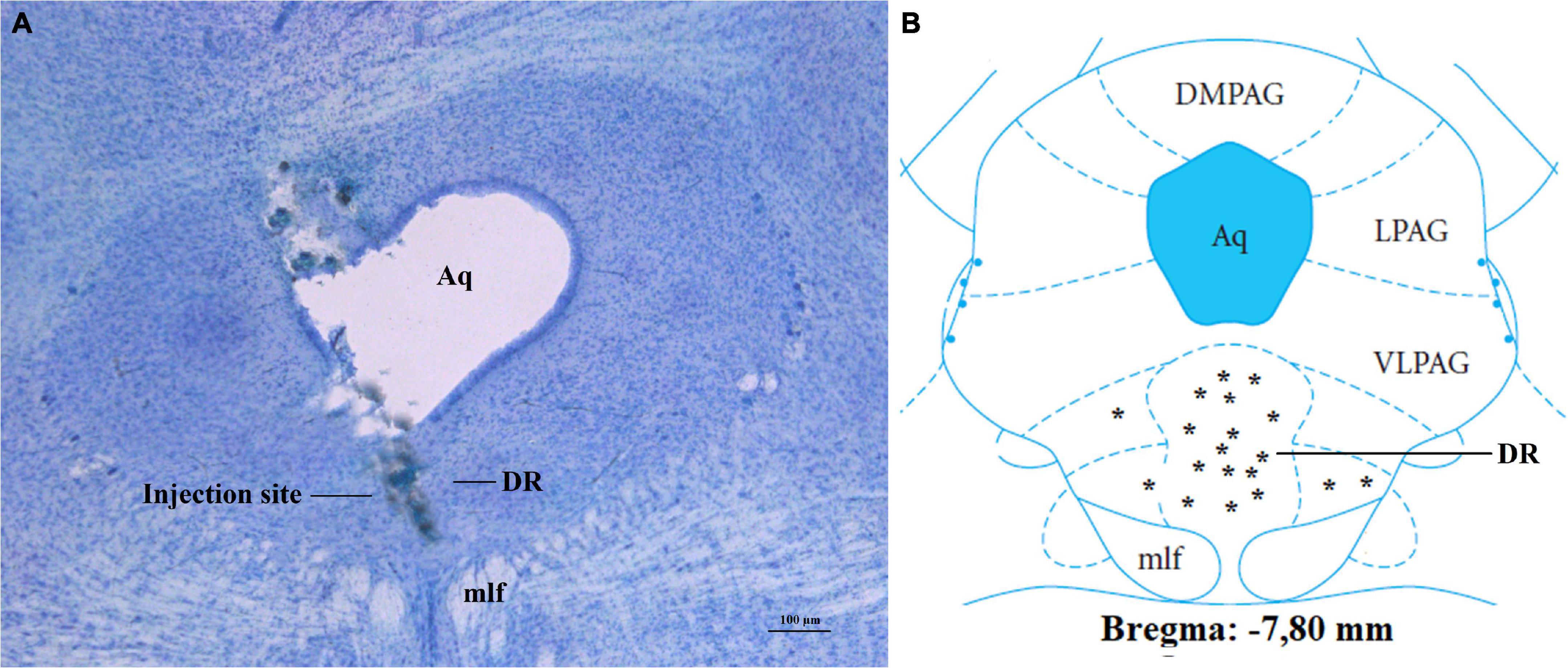
Figure 1. Confirmation of injection sites into DR of fasted rats. (A) Photomicrograph of a stained section, showing injection site into the DR. (B) Injection sites at the bregma level:7, 80 mm; other injection sites were located at-7.32 to-8.04 mm to bregma. Aq, aqueduct; DR, dorsal raphe nucleus; DMPAG, dorsomedial periaqueductal gray; LPAG, lateral periaqueductal gray; VLPAG, ventrolateral periaqueductal gray; mlf, medial longitudinal fasciculus. Scale bar = 100 μm. *, location of each injection site.
Changes in Feeding and Non-feeding Behaviors After Injection of Adrenaline (AD) Into the DR of Fasted Rats
AD injection of 20 and 60 nmol doses into the DR decreased food intake in fasted rats [F(3, 22) = 22.30, p < 0.0001] (Figure 2A), as well as feeding duration [F(3, 24) = 21.12, p < 0.0001], when compared with the control group (vehicle injection; Table 2). Feeding frequency and latency to start feeding were not affected by AD injection (Table 2). Water intake also decreased after injection of AD 60 nmol [F(3, 22) = 7.97, p = 0.0009] (Figure 2B). In addition, there was a positive correlation (r = 0.82; p < 0.0001) between the amount of water intake and the amount of food consumed (Figure 2C). The duration of immobility behavior was increased [F(3, 21) = 26.40, p = 0.01] after AD injection of 20 and 60 nmol doses (Table 3). Other non-ingestive behaviors were not changed by AD treatment (Table 3).
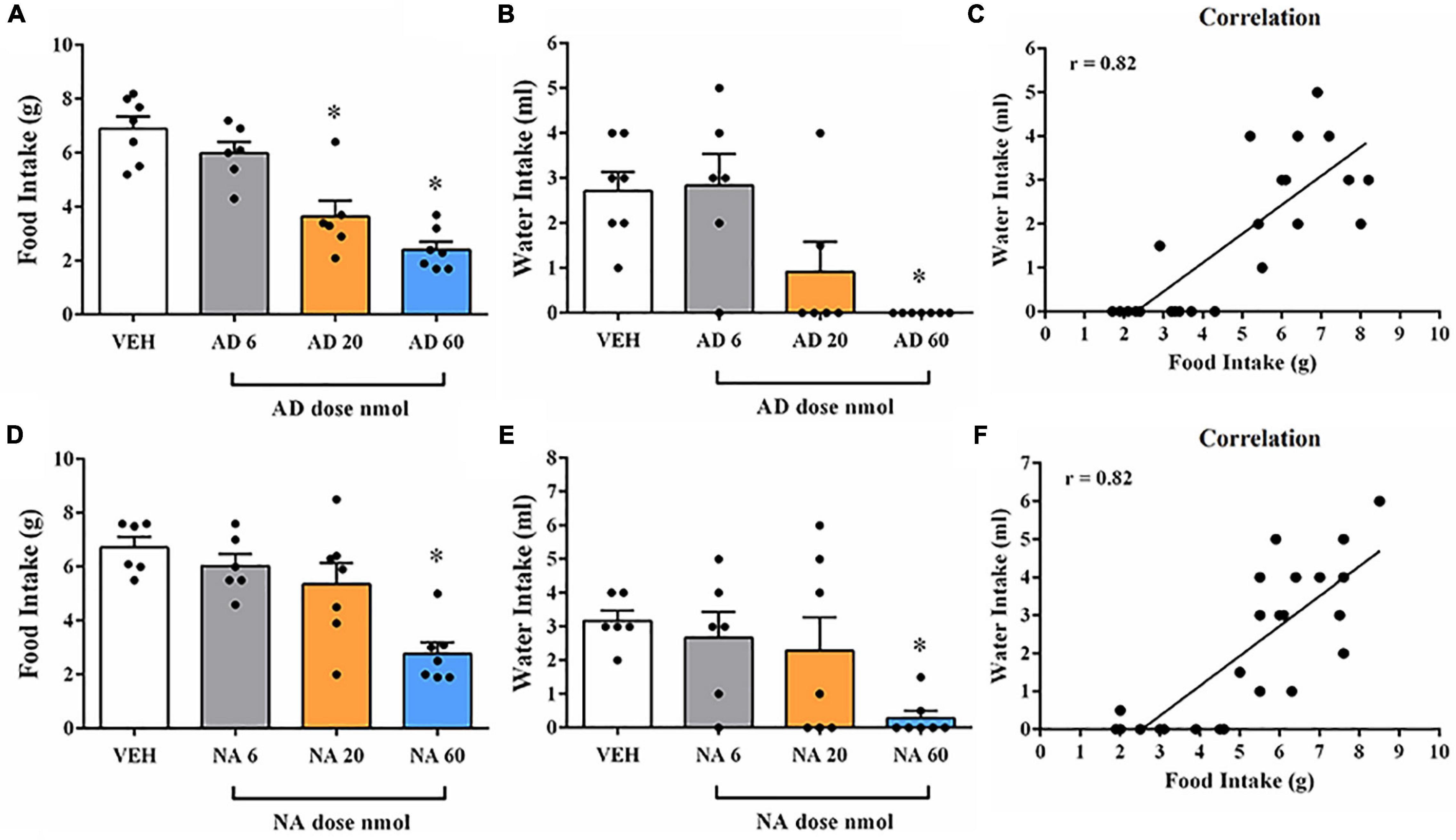
Figure 2. Food and water intake after injection of adrenaline (AD) or noradrenaline (NA) into DR of fasted rats. (A) Changes in the amount of food intake after injection with vehicle (VEH) or AD at 6, 20, and 60 nmol doses into DR of fasted rats. (B) Changes in the amount of water intake after treatment with VEH or AD at 6, 20, and 60 nmol doses into DR of fasted rats. (C) Correlation between water and food intake after administration of AD (6, 20, 60 nmol) or VEH into DR. (D) Changes in the amount of food intake after injection with VEH or NA at 6, 20, and 60 nmol doses into DR of fasted rats. (E) Changes in the amount of water intake after injection with VEH or NA at 6, 20, and 60 nmol doses into DR of fasted rats. (F) Correlation between water and food intake after administration of NA (6, 20, 60 nmol) or VEH into DR. In all experiments, separate rats were used for each dose; each rat received only a single injection of drug or vehicle. Data represent the mean ± SEM *p < 0.05 vs. vehicle group. One-way ANOVA followed by Tukey’s post hoc test (n = 6–8 per group). Pearson’s correlation test *p < 0.05.
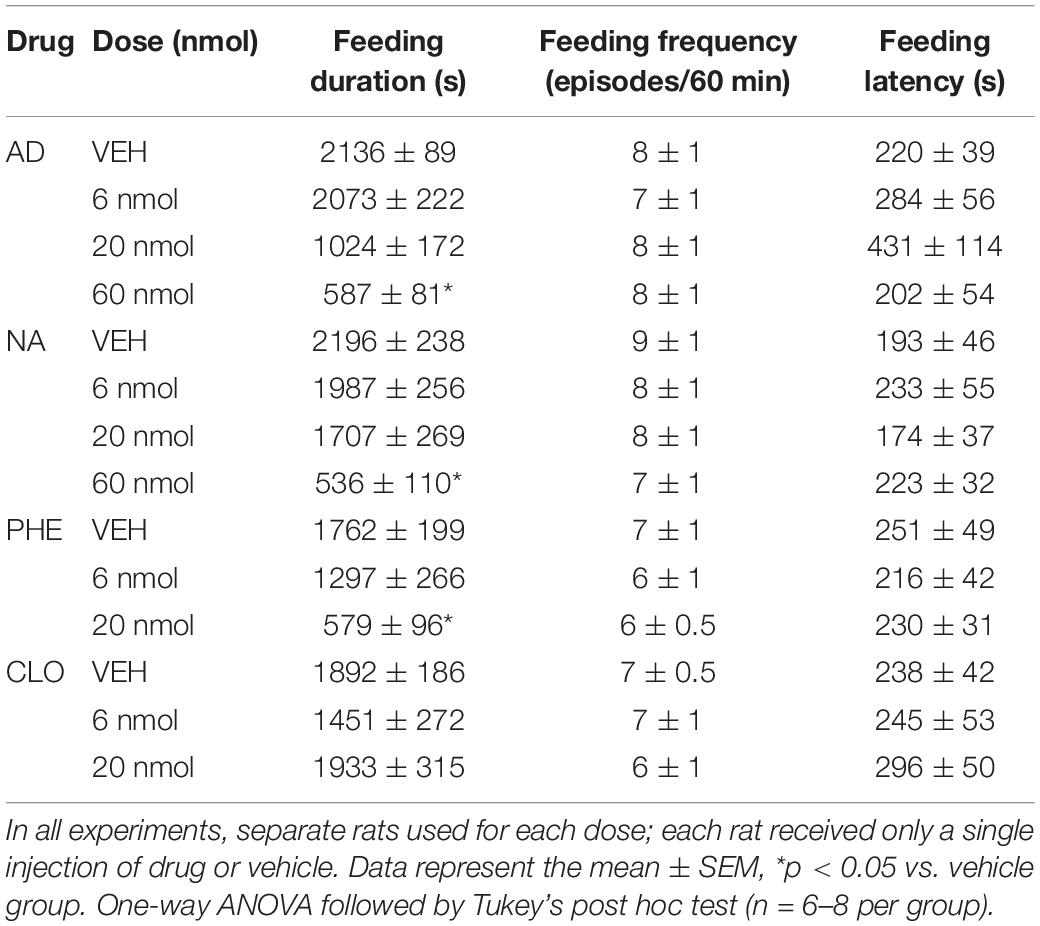
Table 2. Feeding duration, feeding frequency and feeding latency during 60 min of recording after injection of adrenaline (AD), noradrenaline (NA), phenylephrine (PHE), clonidine (CLO), or vehicle (VEH) into DR of fasted rats.
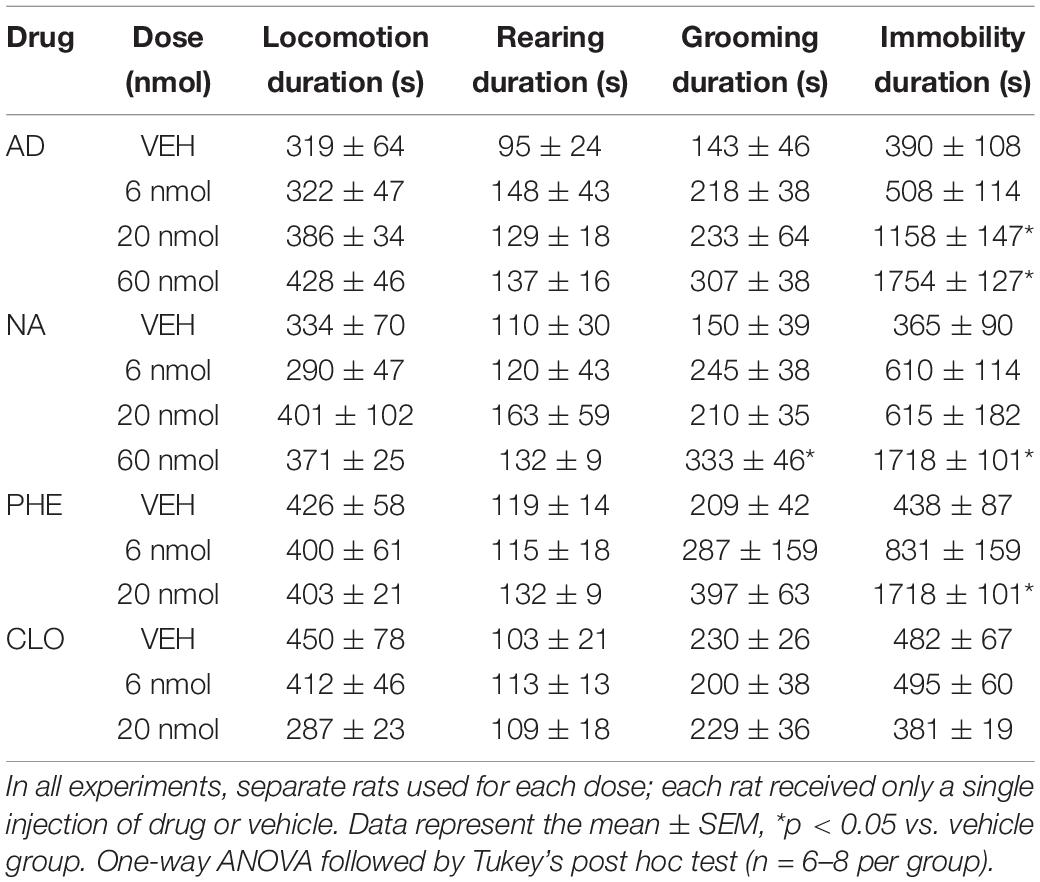
Table 3. Duration of non-ingestive behaviors during 60 min of recording after injection of adrenaline (AD), noradrenaline (NA), phenylephrine (PHE), clonidine (CLO), or vehicle (VEH) into DR of fasted rats.
Changes in Feeding and Non-feeding Behaviors After Injection of Noradrenaline (NA) Into the DR of Fasted Rats
NA injection of 60 nmol dose into the DR decreased food intake in fasted rats [F(3, 22) = 9.85, p = 0.0003] (Figure 2D), as well as feeding duration [F(3, 22) = 11.20, p = 0.0001], when compared with control group (vehicle injection; Table 2). Feeding frequency and latency to start feeding were not affected by NA injection (Table 2). Water intake was also decreased after injection of NA 60 nmol dose [F(3, 22) = 3.69, p = 0.0273] (Figure 2E) with a positive correlation (r = 0.82; p < 0.0001) between the amount of water intake and the amount of food consumed (Figure 2F). Lower doses of NA did not affect ingestive behaviors or water intake. The duration of immobility [F(3, 19) = 22.26, p = 0.01] and grooming [F(3, 19) = 5.56, p = 0.006] behaviors were increased after NA injection of 60 nmol dose (Table 3). Other non-ingestive behaviors were not affected by NA treatment (Table 3).
Changes in Feeding and Non-feeding Behaviors After Injection of Phenylephrine (PHE) Into the DR of Fasted Rats
PHE injection of 20 nmol dose into the DR decreased food intake in fasted rats [F(2, 19) = 6.13, p = 0.0088] (Figure 3A), as well as feeding duration [F(2, 19) = 8.97, p = 0.0018] (Table 2). Similar to the results found in the AD and NA experiments, feeding frequency and latency to start feeding were also not affected by PHE injection (Table 2). Water intake (Figure 3B) decreased after PHE injection of 6 and 20 nmol doses [F(2, 19) = 13.34, p = 0.0002] with a positive, albeit small, correlation (r = 0.68; p = 0.0005) between the amount of water intake and the amount of food intake. Regarding non-ingestive behaviors, the duration of immobility behavior was increased after PHE injection of 20 nmol dose [F(2, 19) = 31.49, p = 0.004] (Table 3). Other non-ingestive behaviors were not affected by PHE treatment (Table 3).
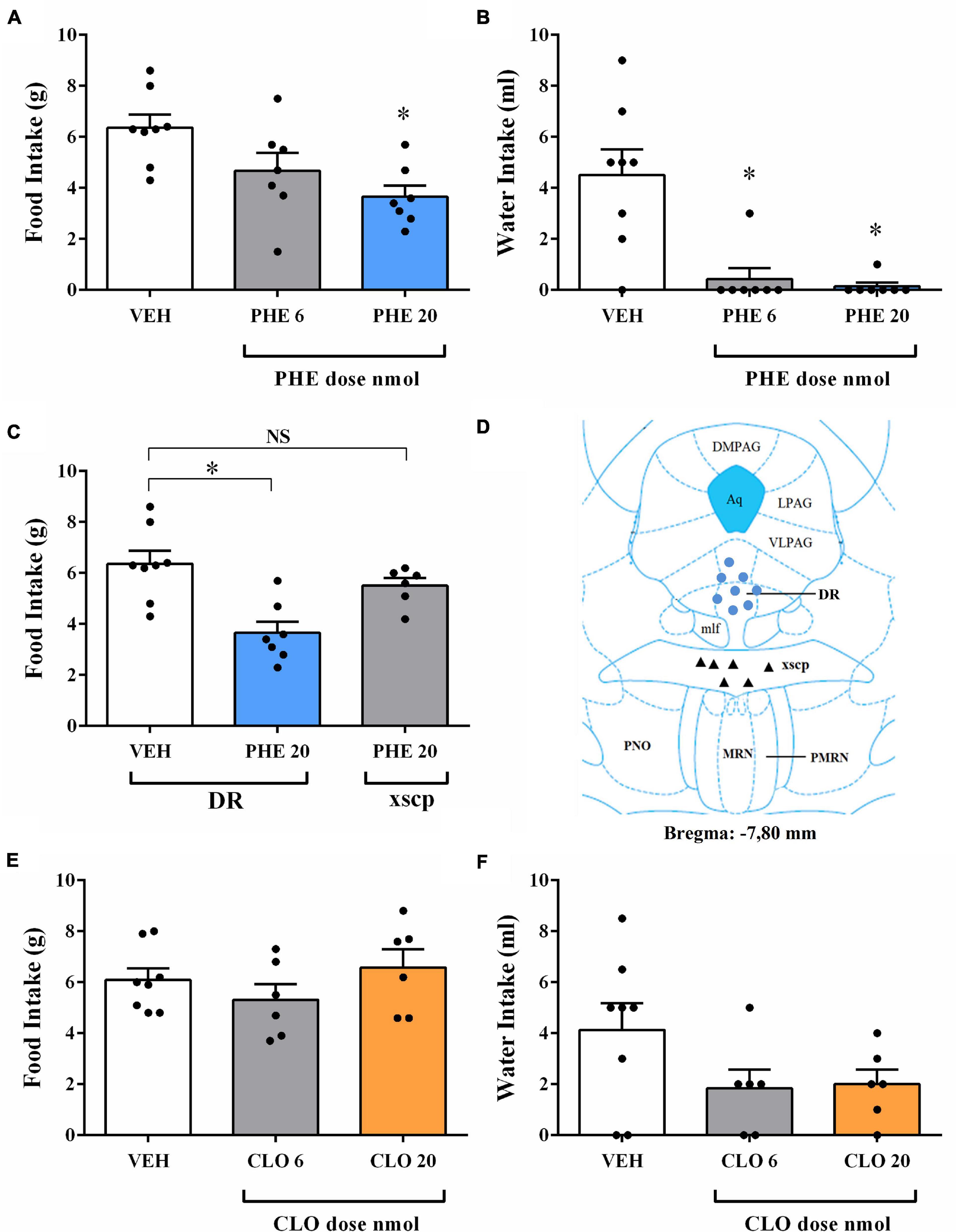
Figure 3. Food and water intake after injection of the α-1 adrenoceptor agonist phenylephrine (PHE) or the α-2 adrenoceptor agonist clonidine (CLO) into DR of fasted rats. (A) Changes in the amount of food intake after injection with vehicle (VEH) or PHE at 6 and 20 nmol doses into DR of fasted rats. (B) Changes in the amount of water intake after treatment with VEH or PHE at 6 and 20 nmol doses into DR of fasted rats. (C,D) Effect of the PHE 20 nmol injection into the decussation of the superior cerebellar peduncle (xscp) on food intake in fasted rats. MR = median raphe nucleus; Aq = cerebral aqueduct; DR = dorsal raphe nucleus; NS = non-significant. (E) Changes in the amount of food intake after injection with VEH or CLO at 6 and 20 nmol doses into DR of fasted rats. (F) Changes in the amount of water intake after injection with VEH or CLO at 6 and 20 nmol doses into DR of fasted rats. In all experiments, separate rats were used for each dose; each rat received only a single injection of drug or vehicle. Data represent the mean ± SEM *p < 0.05 vs. vehicle group. One-way ANOVA followed by Tukey’s post hoc test (n = 6–8 per group).
In order to determine if this finding is specific for correct injections in the DR, an additional group of rats received injections of PHE 20 nmol in the decussation of the superior cerebellar peduncle (xscp), a mesopontine area located between the DR and median raphe nucleus (MR). Statistical analyses revealed that PHE 20 nmol injection in the xscp did not affect ingestive behaviors when compared to the intra-DR vehicle group (Figures 3C,D). Previous work reported that PHE injection of 20 nmol dose into median raphe nucleus (MR) decreased food intake in fasted rats (Ribas et al., 2012). Thus, the effects on food intake could be the sum of the drug effects in these two nuclei due the close localization in the mesopontine tegmentum. However, the lack of effect on food intake of PHE injections into the xscp indicates that the possibility of diffusion of the drug from DR to MR is unlikely.
Changes in Feeding and Non-feeding Behaviors After Injection of Clonidine (CLO) Into the DR of Fasted Rats
Ingestive and non-ingestive behaviors were not significantly affected by CLO injection into the DR at either 6 and 20 nmol doses (Figure 3E and Tables 2, 3). Also, water intake remained unchanged after CLO treatment in the DR when compared to the control group (Figure 3F).
Discussion
In the present study, we investigated the effects of pharmacological manipulations of α-adrenergic agonists in the DR on food intake in fasted rats. Overall, we observed that acute injections of NA or AD into DR evoked reduced food intake in fasted rats. α-adrenoceptors are present in the DR, therefore our assumption is that the decrease in food consumption after NA or AD infusions might be due to the activation of these receptors. Strengthening this notion, injection of specific α-1 agonists PHE into DR of fasted rats decreased food intake similarly to response induced by NA or AD injection. The injection of specific α-2 agonist CLO into DR does not affect feeding, indicating that the activation of α-1 postsynaptic adrenoceptors in this nucleus has an inhibitory influence on feeding in fasted rats.
Interestingly, in a previous study opposite feeding responses were induced by injection of α-adrenoceptors agonists into the DR of satiated rats. In the fed state, AD, NA, or CLO injection increased food intake while PHE treatment did not change feeding behavior (Flores et al., 2021). Based on these findings, the hyperphagic effect was attributed to the inhibition of NA release in DR noradrenergic terminals by the activation of α-2 presynaptic auto-receptors. This activation removes a possible endogenous α-1 adrenergic stimulatory tone on DR serotonergic neurons of fed animals, leading to a decrease in 5-HT release in projection areas, which could favor ingestive behaviors (Flores et al., 2021). In fact, 5-HT release decreases after CLO injection into DR, and lesion with DSP-4 abolishes these effects (Bortolozzi and Artigas, 2003). These data are, in part, corroborated by the experiments in the present work. The hypophagia caused by PHE injection into the DR of fasted rats is comparable to hypophagia induced by NA or AD injections. On the other hand, injection of CLO does not change food intake in these rats, suggesting that the action of AD or NA is mediated by α-1 adrenoceptors in this case. Due to this difference in feeding depending on whether the animal is fed or not, we believe the intensity of this endogenous noradrenergic activity, mediated by α-1 adrenoceptors in the DR, seems to decline in fasted rats.
Several studies demonstrated that 5-HT acts as a satiety signal in hypothalamic nuclei, such as the arcuate and the paraventricular nuclei, as well as other areas such as the parabrachial nucleus and nucleus of the solitary tract (Voigt and Fink, 2015). Stimulation of DR neurons increases extracellular 5-HT levels in the hypothalamus (De Fanti et al., 2000) and manipulation of adrenoceptor activity in the DR induces FOS expression in discrete populations of arcuate and paraventricular nucleus neurons (Flores et al., 2021). Thus, it is possible that activation of DR α-1 adrenoceptors by PHE results in 5-HT release in fasted animals, since facilitatory control of 5-HT release is attributed to these receptors (Bortolozzi and Artigas, 2003). In agreement with this suggestion, a study demonstrated that PHE injections into the median raphe nucleus (MR), another major serotonergic cell group with α-adrenoceptors (Adell and Artigas, 1999), also evoked hypophagia in fasted rats (Ribas et al., 2012). Also, serotonergic activity is low in food restricted rats and, especially in the DR, food restriction decreases the optical density of 5-HT positive neurons when compared to fed rats (Haider and Haleem, 2000; Kang et al., 2001). Therefore, our hypothesis is that in fasted animals the effect of AD or NA injection into the DR is mainly mediated by α-1 adrenoceptor, stimulating 5-HT release in the projection areas and consequently decreasing food intake (Figure 4). However, further experiments using adrenergic antagonists in fasted and fed rats are necessary to better understand the role of these DR adrenergic receptors in feeding behavior.
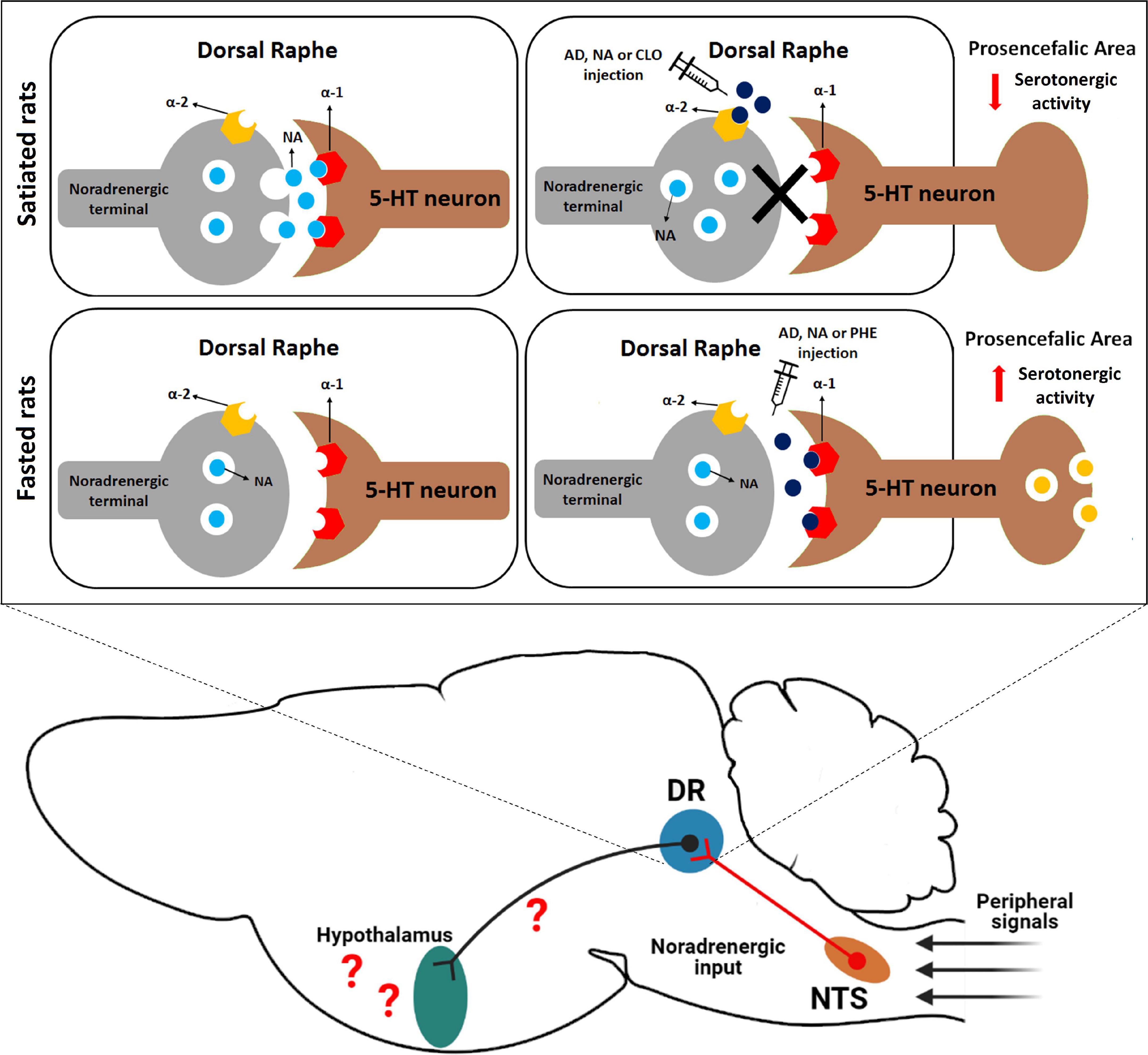
Figure 4. Proposed role of noradrenergic circuits in the DR in food intake regulation in rats. In the fed state, there is a tonic activation of α-1 adrenoceptors into this nucleus, which facilitates the release of a signal that inhibits food intake (possibly via 5-HT release into proensephalic areas) into prosencephalic areas. On the other hand, in fasted rats the intensity of this endogenous noradrenergic activity seems to decline. We also speculate that peripheral satiety signals, such as CCK, could indirectly modify neuronal activity in the DR through noradrenergic neurons located in the NTS that send neuronal input to DR neurons. DR, dorsal raphe nucleus; NTS, nucleus of the solitary tract; AD, adrenaline; NA, noradrenaline; CLO, clonidine; PHE, phenylephrine.
DR is a neurochemically heterogeneous structure containing distinct clusters of 5-HT neurons and several other differentially distributed major neurotransmitters and neuropeptides (Calizo et al., 2011). Some DR subregions display large proportions of GABAergic, dopaminergic, glutamatergic and neurons with a mixed glutamatergic/serotonergic phenotype (Hioki et al., 2010; Calizo et al., 2011; Soiza-Reilly and Commons, 2011). It has been reported that ICV injection of AD 20 nmol evoked serotonergic and non-serotonergic neuronal activation in the DR (Flores et al., 2018). In a recent study conducted by Nectow et al. (2017), treatments that enhance GABAergic tone within DR lead to an increase in food intake, while activation of DR glutamatergic neurons decreases feeding. α-1 adrenoceptors have been reported to be robustly expressed only in 5-HT DR neurons and in a small population of DR GABAergic neurons that expresses the type α-1b receptor (Day et al., 2004). There was no evidence, until the present study, that α-adrenoceptors are expressed in glutamatergic DR neurons. However, the functional data presented in this work does not support activation through GABAergic α-1b receptors, since these neurons stimulate food intake when activated. These observations further support the idea that decreased food intake involves the participation of 5-HT DR neurons.
In addition to the effects observed on food intake, AD, NA and PHE significantly decreased water intake. Generally, water intake occurs in conjunction with food intake, i.e., animals often drink fluids during or right after a meal (Kissileff, 1969; Mecawi et al., 2015). Indeed, in this study water and food intake after AD and NA microinjections were strongly correlated. However, intra-DR PHE administration affected water intake in the smaller dose used, which was not sufficient to affect food intake. This result indicates that activation of α-1 receptors within the DR may inhibit water intake irrespective of food intake. Previous studies have shown that electrolytic lesion of the DR or depletion of 5-HT synthesis induce water intake in rats. This increase in water intake is accompanied by decreased urinary volume, and several endocrine alterations that culminate with water retention, indicating participation of DR serotoninergic systems in hydromineral balance (Reis et al., 1994). Additionally, acute administration of a 5-HT1a agonist, which decreases endogenous 5-HT release, potently induced water intake in rats (Fonseca et al., 2009). These studies indicate that activation of DR 5-HT neurons decreases water intake. Thus, the effects of PHE administration to decrease water intake further supports the idea that α-1 activation increases the activity of DR 5-HT neurons that, in turn, affects the ingestive behaviors in rats.
Binding studies also demonstrated that NA shows some affinity for dopaminergic receptors, with a low to moderate potency to bind and activate D4-class receptors (Lanau et al., 1997; Newman-Tancredi et al., 1997; Sánchez-Soto et al., 2016). In contrast, some authors report low to moderate levels of D2 receptor expression in DR 5-HT neurons (Dawson et al., 1986; Mansour et al., 1990; Meador-Woodruff et al., 1991; Ferré and Artigas, 2006). Despite the possibility of NA acting on D2 receptors, the similarity between the responses evoked after NA injection and the specific α-1 agonist PHE, support the hypothesis that the effects of NA are mediate by α-1 receptors. Additionally, although some activity via D2 receptors is possible, NA shows higher affinity for α and β adrenoceptors; consequently, the probability of NA acting at these receptors is greater. Furthermore, to our knowledge, there is no evidence that DR dopaminergic receptors affect feeding behavior in rats.
Manipulations that interfere with the serotonergic system can potentially influence a variety of behaviors in addition to food intake (Lucki, 1998). However, DR injections of adrenergic agonists only modified immobility behavior. The neurochemical mechanism by which AD or NA may reduce food intake during food deprivation can be attributed to an anticipation of satiety signals, a result consistent with the postulated inhibitory role for 5-HT in controlling eating behavior (Hutson et al., 1986; Blundell, 1991; Leibowitz and Alexander, 1998; Wirtshafter, 2001). Therefore, these results indicate that the treatments used may have anticipated the behavioral sequence of satiety, which is characterized by the increase in immobility after food consumption (Halford et al., 1998), thus inducing the end of the meal. Adrenergic agonist injections into DR decreased feeding duration and this response has been linked to changes in mechanisms that end the meal (Ritter and Epstein, 1975; Blundell, 1986). Several studies have documented increased neuronal activation in A2/C2 catecholaminergic neurons in the brainstem in response to anorexic peptides such as cholecystokinin (Rinaman et al., 1993; Blevins et al., 2003). As previously mentioned, the DR receives robust NTS noradrenergic input (Peyron et al., 1996). Based on these findings, peripheral satiety signals from gut could induce satiety via an increase in the activity of NTS noradrenergic neurons that innervate the DR (Figure 4). However, further studies using specific chemogenetic or optogenetic approaches are required to better understand the neural circuits involved.
In conclusion, the data presented in this study indicate that activation of α-1 receptors in the DR reduces food intake in hungry animals, while activation of α-2 does not affect hunger-induced food intake. Interestingly, these effects differ from those observed in ad libitum fed rats, in which α-2 activation induces food intake. Taken together, these results suggest that an endogenous release of adrenalin/noradrenalin by DR neurons mediates satiety in fed rats, while in the overnight fasted rats the intensity of this endogenous noradrenergic activity mediated by α-1 adrenoceptors seems to decline.
Data Availability Statement
The raw data supporting the conclusions of this article will be made available by the authors, without undue reservation.
Ethics Statement
The animal study was reviewed and approved by the Ethics Committee for the use of Experimental Animals (CEUA) of the Federal University of Santa Catarina, SC, Brazil (CEUA protocol: PP0075).
Author Contributions
RF and MP conceived the study and designed the experiments. RF performed all the experiments with the participation of RS and JA-R in the behavioral tests. RD-S and IR-S contributed to data analysis. RF wrote the manuscript with input from all other authors and revision. All authors contributed to the article and approved the submitted version.
Funding
This study was funded by Brazilian Governmental agency CNPq. RD-S was supported by grant #2019/01260-2, São Paulo Research Foundation (FAPESP).
Conflict of Interest
The authors declare that the research was conducted in the absence of any commercial or financial relationships that could be construed as a potential conflict of interest.
Publisher’s Note
All claims expressed in this article are solely those of the authors and do not necessarily represent those of their affiliated organizations, or those of the publisher, the editors and the reviewers. Any product that may be evaluated in this article, or claim that may be made by its manufacturer, is not guaranteed or endorsed by the publisher.
Acknowledgments
We would like to thank Multiuser Laboratory of Studies in Biology (LAMEB/UFSC) staff for the technical assistance.
References
Adell, A., and Artigas, F. (1999). Regulation of the release of 5-hydroxytryptamine in the median raphe nucleus of the rat by catecholaminergic afferents. Eur. J. Neurosci. 11, 2305–2311. doi: 10.1046/j.1460-9568.1999.00642.x
Adell, A., Celada, P., Abellán, M. T., and Artigas, F. (2002). Origin and functional role of the extracellular serotonin in the midbrain raphe nuclei. Brain Res. Rev. 39, 154–180. doi: 10.1016/S0165-0173(02)00182-0
Anderberg, R. H., Richard, J. E., Eerola, K., López-Ferreras, L., Banke, E., Hansson, C., et al. (2017). Glucagon-Like peptide 1 and its analogs act in the dorsal raphe and modulate central serotonin to reduce appetite and body weight. Diabetes 66, 1062–1073. doi: 10.2337/db16-0755
Baraban, J. M., and Aghajanian, G. K. (1980). Suppression of firing activity of 5-HT neurons in the dorsal raphe by alpha-adrenoceptor antagonists. Neuropharmacology 19, 355–363. doi: 10.1016/0028-3908(80)90187-2
Berger, M., Gray, J. A., and Roth, B. L. (2009). The expanded biology of serotonin. Annu. Rev. Med. 60, 355–366. doi: 10.1146/annurev.med.60.042307.110802
Blevins, J. E., Eakin, T. J., Murphy, J. A., Schwartz, M. W., and Baskin, D. G. (2003). Oxytocin innervation of caudal brainstem nuclei activated by cholecystokinin. Brain Res. 993, 30–41. doi: 10.1016/j.brainres.2003.08.036
Blundell, J. (1991). Pharmacological approaches to appetite suppression. Trends Pharmacol. Sci. 12, 147–157. doi: 10.1016/0165-6147(91)90532-W
Blundell, J. E. (1986). Serotonin manipulations and the structure of feeding behaviour. Appetite 7, 39–56. doi: 10.1016/S0195-6663(86)80051-4
Blundell, J. E., and Latham, C. J. (1979). Serotonergic influences on food intake: effect of 5-hydroxytryptophan on parameters of feeding behaviour in deprived and free-feeding rats. Pharmacol. Biochem. Behav. 11, 431–437. doi: 10.1016/0091-3057(79)90120-5
Bortolozzi, A., and Artigas, F. (2003). Control of 5-Hydroxytryptamine release in the dorsal raphe nucleus by the noradrenergic system in rat brain. Role of α-adrenoceptors. Neuropsychopharmacology 28, 421–434. doi: 10.1038/sj.npp.1300061
Bruschetta, G., Jin, S., Liu, Z.-W., Kim, J. D., and Diano, S. (2020). MC4R signaling in dorsal raphe nucleus controls feeding, anxiety, and depression. Cell Rep. 33:108267. doi: 10.1016/j.celrep.2020.108267
Calizo, L. H., Akanwa, A., Ma, X., Pan, Y., Lemos, J. C., Craige, C., et al. (2011). Raphe serotonin neurons are not homogenous: electrophysiological, morphological and neurochemical evidence. Neuropharmacology 61, 524–543. doi: 10.1016/j.neuropharm.2011.04.008
Dawson, T., Gehlert, D., McCabe, R., Barnett, A., and Wamsley, J. (1986). D-1 dopamine receptors in the rat brain: a quantitative autoradiographic analysis. J. Neurosci. 6, 2352–2365. doi: 10.1523/JNEUROSCI.06-08-02352.1986
Day, H. E. W., Greenwood, B. N., Hammack, S. E., Watkins, L. R., Fleshner, M., Maier, S. F., et al. (2004). Differential expression of 5HT-1A, ?1b adrenergic, CRF-R1, and CRF-R2 receptor mRNA in serotonergic, ?-aminobutyric acidergic, and catecholaminergic cells of the rat dorsal raphe nucleus. J. Comp. Neurol. 474, 364–378. doi: 10.1002/cne.20138
Day, H. E., Campeau, S., Watson, S. J., and Akil, H. (1997). Distribution of α 1a-, α1b- and α1d-adrenergic receptor mRNA in the rat brain and spinal cord. J. Chem. Neuroanat. 13, 115–139. doi: 10.1016/S0891-0618(97)00042-2
De Fanti, B. A., Gavel, D. A., Hamilton, J. S., and Horwitz, B. A. (2000). Extracellular hypothalamic serotonin levels after dorsal raphe nuclei stimulation of lean (Fa/Fa) and obese (fa/fa) Zucker rats. Brain Res. 869, 6–14. doi: 10.1016/s0006-8993(00)02308-8
dos Santos, R. L. D., Mansur, S. S., Steffens, S. M., Faria, M. S., Marino-Neto, J., and Paschoalini, M. A. (2009). Food intake increased after injection of adrenaline into the median raphe nucleus of free-feeding rats. Behav. Brain Res. 197, 411–416. doi: 10.1016/j.bbr.2008.10.008
Ferré, S., and Artigas, F. (2006). Dopamine D2 receptor-mediated regulation of serotonin extracellular concentration in the dorsal raphe nucleus of freely moving rats. J. Neurochem. 61, 772–775. doi: 10.1111/j.1471-4159.1993.tb02187.x
Fletcher, P. J., and Davies, M. (1990). Dorsal raphe microinjection of 5-HT and indirect 5-HT agonists induces feeding in rats. Eur. J. Pharmacol. 184, 265–271. doi: 10.1016/0014-2999(90)90618-G
Flores, R. A., da Silva, E. S., Ribas, A. S., Taschetto, A. P. D., Zampieri, T. T., Donato, J., et al. (2018). Evaluation of food intake and Fos expression in serotonergic neurons of raphe nuclei after intracerebroventricular injection of adrenaline in free-feeding rats. Brain Res. 1678, 153–163. doi: 10.1016/j.brainres.2017.10.021
Flores, R. A., Steinbach, R., Pedroso, J. A. B., Metzger, M., Donato, J., and Paschoalini, M. A. (2021). Injections of the α-2 adrenoceptor agonist clonidine into the dorsal raphe nucleus increases food intake in satiated rats. Neuropharmacology 182:108397. doi: 10.1016/j.neuropharm.2020.108397
Fonseca, F. V., Mecawi, A. S., Araujo, I. G., Almeida-Pereira, G., Magalhães-Nunes, A. P., Badauê-Passos, D., et al. (2009). Role of the 5-HT(1A) somatodendritic autoreceptor in the dorsal raphe nucleus on salt satiety signaling in rats. Exp. Neurol. 217, 353–360. doi: 10.1016/j.expneurol.2009.03.015
Haider, S., and Haleem, D. J. (2000). Decreases of brain serotonin following a food restriction schedule of 4 weeks in male and female rats. Med. Sci. Monit. 6, 1061–1067.
Halford, J. C., Wanninayake, S. C., and Blundell, J. E. (1998). Behavioral satiety sequence (BSS) for the diagnosis of drug action on food intake. Pharmacol. Biochem. Behav. 61, 159–168. doi: 10.1016/s0091-3057(98)00032-x
Hioki, H., Nakamura, H., Ma, Y.-F., Konno, M., Hayakawa, T., Nakamura, K. C., et al. (2010). Vesicular glutamate transporter 3-expressing nonserotonergic projection neurons constitute a subregion in the rat midbrain raphe nuclei. J. Comp. Neurol. 518, 668–686. doi: 10.1002/cne.22237
Hutson, P. H., Dourish, C. T., and Curzon, G. (1986). Neurochemical and behavioural evidence for mediation of the hyperphagic action of 8-OH-DPAT by 5-HT cell body autoreceptors. Eur. J. Pharmacol. 129, 347–352. doi: 10.1016/0014-2999(86)90445-0
Kang, M., Park, C., Ahn, H., and Huh, Y. (2001). Ectopic expression of serotonin-positive neurons in the hypothalamus associated with a significant serotonin decrease in the midbrain of food restricted rats. Neurosci. Lett. 314, 25–28. doi: 10.1016/S0304-3940(01)02279-0
Kissileff, H. R. (1969). Food-associated drinking in the rat. J. Comp. Physiol. Psychol. 67, 284–300. doi: 10.1037/h0026773
Lanau, F., Zenner, M. T., Civelli, O., and Hartman, D. S. (1997). Epinephrine and norepinephrine act as potent agonists at the recombinant human dopamine D4 receptor. J. Neurochem. 68, 804–812. doi: 10.1046/j.1471-4159.1997.68020804.x
Leibowitz, S. F., and Alexander, J. T. (1998). Hypothalamic serotonin in control of eating behavior, meal size, and body weight. Biol. Psychiatry 44, 851–864. doi: 10.1016/s0006-3223(98)00186-3
Lucki, I. (1998). The spectrum of behaviors influenced by serotonin. Biol. Psychiatry 44, 151–162. doi: 10.1016/s0006-3223(98)00139-5
Mansour, A., Meador-Woodruff, J., Bunzow, J., Civelli, O., Akil, H., and Watson, S. (1990). Localization of dopamine D2 receptor mRNA and D1 and D2 receptor binding in the rat brain and pituitary: an in situ hybridization- receptor autoradiographic analysis. J. Neurosci. 10, 2587–2600. doi: 10.1523/JNEUROSCI.10-08-02587.1990
Mansur, S. S., Terenzi, M. G., Neto, J. M., Faria, M. S., and Paschoalini, M. A. (2010). Changes in food intake and anxiety-like behaviors after clonidine injected into the median raphe nucleus. Behav. Brain Res. 212, 71–77. doi: 10.1016/j.bbr.2010.03.046
McCune, S. K., Voigt, M. M., and Hill, J. M. (1993). Expression of multiple alpha adrenergic receptor subtype messenger RNAs in the adult rat brain. Neuroscience 57, 143–151.
Meador-Woodruff, J. H., Mansour, A., Healy, D. J., Kuehn, R., Zhou, Q. Y., Bunzow, J. R., et al. (1991). Comparison of the distributions of D1 and D2 dopamine receptor mRNAs in rat brain. Neuropsychopharmacology 5, 231–242.
Mecawi, A. S., Ruginsk, S. G., Elias, L. L. K., Varanda, W. A., and Antunes-Rodrigues, J. (2015). Neuroendocrine regulation of hydromineral homeostasis. Compr. Physiol. 5, 1465–1516. doi: 10.1002/cphy.c140031
Muzerelle, A., Scotto-Lomassese, S., Bernard, J. F., Soiza-Reilly, M., and Gaspar, P. (2016). Conditional anterograde tracing reveals distinct targeting of individual serotonin cell groups (B5–B9) to the forebrain and brainstem. Brain Struct. Funct. 221, 535–561. doi: 10.1007/s00429-014-0924-4
Nectow, A. R., Schneeberger, M., Zhang, H., Field, B. C., Renier, N., Azevedo, E., et al. (2017). Identification of a brainstem circuit controlling feeding. Cell 170, 429–442.e11. doi: 10.1016/j.cell.2017.06.045
Newman-Tancredi, A., Audinot-Bouchez, V., Gobert, A., and Millan, M. J. (1997). Noradrenaline and adrenaline are high affinity agonists at dopamine D4 receptors. Eur. J. Pharmacol. 319, 379–383. doi: 10.1016/s0014-2999(96)00985-5
Nicholas, A. P., Pieribone, V., and Hökfelt, T. (1993). Distributions of mRNAs for alpha-2 adrenergic receptor subtypes in rat brain: an in situ hybridization study. J. Comp. Neurol. 328, 575–594. doi: 10.1002/cne.903280409
Ottoni, E. B. (2000). EthoLog 2.2: a tool for the transcription and timing of behavior observation sessions. Behav. Res. Methods Instrum. Comput. 32, 446–449. doi: 10.3758/BF03200814
Paxinos, G., and Watson, C. (2005). The Rat Brain in Stereotaxic Coordinates, 6th Edn. London: Elsevier Academic Press.
Peyron, C., Luppi, P.-H., Fort, P., Rampon, C., and Jouvet, M. (1996). Lower brainstem catecholamine afferents to the rat dorsal raphe nucleus. J. Comp. Neurol. 364, 402–413.
Pieribone, V., Nicholas, A., Dagerlind, A., and Hokfelt, T. (1994). Distribution of alpha 1 adrenoceptors in rat brain revealed by in situ hybridization experiments utilizing subtype-specific probes. J. Neurosci. 14, 4252–4268. doi: 10.1523/JNEUROSCI.14-07-04252.1994
Pudovkina, O. L., Cremers, T. I. F. H., and Westerink, B. H. C. (2003). Regulation of the release of serotonin in the dorsal raphe nucleus by ?1 and ?2 adrenoceptors. Synapse 50, 77–82. doi: 10.1002/syn.10245
Pytliak, M., Vargová, V., Mechírová, V., and Felšöci, M. (2011). Serotonin receptors - from molecular biology to clinical applications. Physiol. Res. 60, 15–25. doi: 10.33549/physiolres.931903
Reis, L. C., Ramalho, M. J., Favaretto, A. L., Gutkowska, J., McCann, S. M., and Antunes-Rodrigues, J. (1994). Participation of the ascending serotonergic system in the stimulation of atrial natriuretic peptide release. Proc. Natl. Acad. Sci. U.S.A. 91, 12022–12026. doi: 10.1073/pnas.91.25.12022
Ren, J., Isakova, A., Friedmann, D., Zeng, J., Grutzner, S. M., Pun, A., et al. (2019). Single-cell transcriptomes and whole-brain projections of serotonin neurons in the mouse dorsal and median raphe nuclei. Elife 8:e49424. doi: 10.7554/eLife.49424
Ribas, A. S., Flores, R. A., de Nazareth, A. M., Faria, M. S., Terenzi, M. G., Marino-Neto, J., et al. (2012). Feeding behaviour after injection of α-adrenergic receptor agonists into the median raphe nucleus of food-deprived rats. Physiol. Behav. 105, 220–229. doi: 10.1016/j.physbeh.2011.08.028
Rinaman, L., Verbalis, J. G., Stricker, E. M., and Hoffman, G. E. (1993). Distribution and neurochemical phenotypes of caudal medullary neurons activated to express cFos following peripheral administration of cholecystokinin. J. Comp. Neurol. 338, 475–490. doi: 10.1002/cne.903380402
Ritter, R. C., and Epstein, A. N. (1975). Control of meal size by central noradrenergic action. Proc. Natl. Acad. Sci. U.S.A. 72, 3740–3743. doi: 10.1073/pnas.72.9.3740
Rosin, D. L., Zeng, D., Stornetta, R. L., Norton, F. R., Riley, T., Okusa, M. D., et al. (1993). Immunohistochemical localization ofα2a-adrenergic receptors in catecholaminergic and other brainstem neurons in the rat. Neuroscience 56, 139–155. doi: 10.1016/0306-4522(93)90569-2
Sánchez-Soto, M., Bonifazi, A., Cai, N. S., Ellenberger, M. P., Newman, A. H., Ferré, S., et al. (2016). Evidence for noncanonical neurotransmitter activation: norepinephrine as a dopamine D2-Like receptor agonist. Mol. Pharmacol. 89, 457–466. doi: 10.1124/mol.115.101808
Scheinin, M., Lomasney, J. W., Hayden-Hixson, D. M., Schambra, U. B., Caron, M. G., Lefkowitz, R. J., et al. (1994). Distribution of α2-adrenergic receptor subtype gene expression in rat brain. Mol. Brain Res. 21, 133–149. doi: 10.1016/0169-328X(94)90386-7
Schneeberger, M., Parolari, L., Das Banerjee, T., Bhave, V., Wang, P., Patel, B., et al. (2019). Regulation of energy expenditure by brainstem GABA neurons. Cell 178, 672–685.e12. doi: 10.1016/j.cell.2019.05.048
Soiza-Reilly, M., and Commons, K. G. (2011). Glutamatergic drive of the dorsal raphe nucleus. J. Chem. Neuroanat. 41, 247–255. doi: 10.1016/j.jchemneu.2011.04.004
Stachniak, T. J., Ghosh, A., and Sternson, S. M. (2014). Chemogenetic synaptic silencing of neural circuits localizes a hypothalamus→midbrain pathway for feeding behavior. Neuron 82, 797–808. doi: 10.1016/j.neuron.2014.04.008
Talley, E. M., Rosin, D. L., Lee, A., Guyenet, P. G., and Lynch, K. R. (1996). Distribution of?2A-adrenergic receptor-like immunoreactivity in the rat central nervous system. J. Comp. Neurol. 372, 111–134.
Unnerstall, J. R., Fernandez, I., and Orensanz, L. M. (1985). The alpha-adrenergic receptor: radiohistochemical analysis of functional characteristics and biochemical differences. Pharmacol. Biochem. Behav. 22, 859–874. doi: 10.1016/0091-3057(85)90538-6
Vandermaelen, C. P., and Aghajanian, G. K. (1983). Electrophysiological and pharmacological characterization of serotonergic dorsal raphe neurons recorded extracellularly and intracellularly in rat brain slices. Brain Res. 289, 109–119. doi: 10.1016/0006-8993(83)90011-2
Voigt, J.-P., and Fink, H. (2015). Serotonin controlling feeding and satiety. Behav. Brain Res. 277, 14–31. doi: 10.1016/j.bbr.2014.08.065
Wirtshafter, D. (2001). The control of ingestive behavior by the median raphe nucleus. Appetite 36, 99–105. doi: 10.1006/appe.2000.0373
Keywords: dorsal raphe (DR), adrenergic receptor, hunger, food intake, phenylephrine
Citation: Flores RA, Dos-Santos RC, Steinbach R, Rodrigues-Santos I, de Jesus AA, Antunes-Rodrigues J and Paschoalini MA (2021) α-1 Adrenoceptor Activation in the Dorsal Raphe Nucleus Decreases Food Intake in Fasted Rats. Front. Physiol. 12:775070. doi: 10.3389/fphys.2021.775070
Received: 13 September 2021; Accepted: 21 October 2021;
Published: 25 November 2021.
Edited by:
Kathleen S. Curtis, Oklahoma State University Center for Health Sciences, United StatesReviewed by:
Débora Simões A. Colombari, São Paulo State University, BrazilZhi Yi Ong, University of New South Wales, Australia
Copyright © 2021 Flores, Dos-Santos, Steinbach, Rodrigues-Santos, de Jesus, Antunes-Rodrigues and Paschoalini. This is an open-access article distributed under the terms of the Creative Commons Attribution License (CC BY). The use, distribution or reproduction in other forums is permitted, provided the original author(s) and the copyright owner(s) are credited and that the original publication in this journal is cited, in accordance with accepted academic practice. No use, distribution or reproduction is permitted which does not comply with these terms.
*Correspondence: Rafael Appel Flores, cmFmYWVsYXBwZWxmbG9yZXNAZ21haWwuY29t