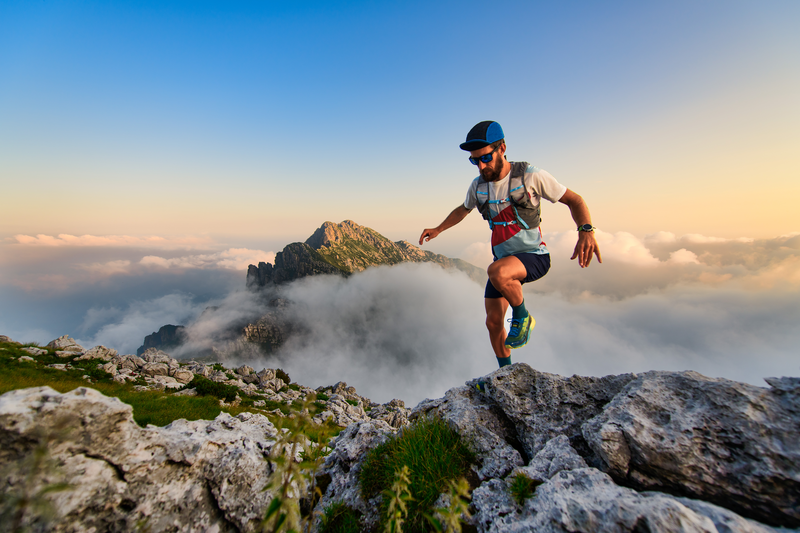
94% of researchers rate our articles as excellent or good
Learn more about the work of our research integrity team to safeguard the quality of each article we publish.
Find out more
ORIGINAL RESEARCH article
Front. Physiol. , 02 December 2021
Sec. Invertebrate Physiology
Volume 12 - 2021 | https://doi.org/10.3389/fphys.2021.768522
This article is part of the Research Topic Insect Physiology Aspects of Environmentally Friendly Strategies for Crop Pests and Insect Vectors Control View all 7 articles
Slow pyrolysis is a process for the thermochemical conversion of biomasses into bio-oils that may contain a rich chemical composition with biotechnological potential. Bio-oil produced from crambe fruits was investigated as to their acaricidal effect. Slow pyrolysis of crambe fruits was performed in a batch reactor at 400°C and chemical composition was analyzed by gas chromatography-mass spectrometry (GC-MS). The bio-oil collected was used in bioassays with larvae and engorged females of the cattle tick Rhipicephalus microplus. Biological assays were performed using the larval packet test (LPT) and adult immersion test. The GC-MS of crambe fruit bio-oil revealed mainly hydrocarbons such as alkanes and alkenes, phenols, and aldehydes. The bio-oil in the LPT exhibited an LC90 of 14.4%. In addition, crambe bio-oil caused female mortality of 91.1% at a concentration of 15% and a high egg-laying inhibition. After ovary dissection of treated females, a significant reduction in gonadosomatic index was observed, indicating that bio-oil interfered in tick oogenesis. Considering these results, it may be concluded that slow pyrolysis of crambe fruit affords a sustainable and eco-friendly product for the control of cattle tick R. microplus.
A major concern in livestock is the infestation in cattle by the tick Rhipicephalus microplus (Canestrini and Fanzago, 1887) (Acari: Ixodidae). This tick can be found in different places in the world with a tropical and subtropical climate and is responsible for a loss of 22–30 billion dollars a year in the livestock industry (Lew-Tabor and Valle, 2016; Ali et al., 2019). Blood spoliation by R. microplus causes a reduction in weight gain of bovine and, consequently, decreases the production of meat and milk. In addition, this tick is a vector of infectious agents that cause babesiosis and anaplasmosis (Araújo et al., 2015).
The most used strategy for the control of R. microplus is using synthetic acaricides. However, these acaricides are mostly toxic to humans, animals, and the environment. In addition, resistance to acaricides has been reported and has become one of the major obstacles in tick control programs. There are populations of ticks with multiple resistance, with cases of resistance to six classes of acaricides also to associations (Fernández-Salas et al., 2012; Reck et al., 2014; Higa et al., 2015; Klafke et al., 2017). Therefore, it becomes necessary to develop new products for the control of R. microplus that are safe for the environment, human, and animal health, and have a low cost.
A new area that has been investigated is the use of products pyrolysis from plant biomass, which has a high biotechnological potential. Plant biomass and agroindustrial residues can be thermochemically converted by pyrolysis. Pyrolysis is a process where an organic matter is subjected to high temperatures (300–1,000°C) in the absence of oxygen (Bridgwater, 2012), generating as products: biochar, pyrolytic liquid, and combustible gases. Pyrolytic liquid can be separated by density difference in an aqueous fraction and a bio-oil (organic fraction) (Czernik and Bridgwater, 2004; Kraiem et al., 2016). The composition of bio-oils varies with the biomass used, being a complex mixture of organic compounds with different chemical functions. Bio-oils obtained from lignocellulosic biomass contain phenolic derivatives, hydroxyaldehydes, hydroxyketones, sugars, and carboxylic acids, mainly acetic acid and formic acid. This chemical composition is due to the depolymerization and fragmentation reactions of the three main constituents of plant biomass: cellulose, hemicellulose, and lignin (Mohan et al., 2006).
Pyrolytic liquids have been the subject of studies for several purposes, mainly as an alternative to fuel production. In recent years, some research groups have revealed the biopesticidal effect of bio-oils against organisms such as insects, bacteria, and fungi (Mattos et al., 2019). The pyrolysis process has great socioenvironmental potential, for generating renewable products and having a reduced emission of greenhouse gases (Silva et al., 2014).
Crambe (Crambe abyssinica Hochst) is an annual herbaceous plant of the Brassicaceae family, native to eastern Africa and the Mediterranean region, which has a low production cost and high tolerance to grow in different climatic conditions (Falasca et al., 2010). Crambe fruit is a small sphere-shaped siliqua about 2 mm in diameter, consisting of a thin pericarp and a single seed that is covered by a thin brown husk. The pericarp, which remains attached to the seed, possesses around 30% of the total mass of fruit, has a high content of lignin (40%) and cellulose (41%). The fruit contains about 21% protein, 16–18% fiber, and 30–44% of a nonedible oil that has a high content of erucic acid (C22:1), a raw material to produce industrial lubricants, synthetic rubber, plastic films, nylon, and adhesives (Falasca et al., 2010; Ionov et al., 2013; Hu et al., 2015; Bassegio et al., 2016; Samarappuli et al., 2020).
Considering the need for alternatives to control the cattle tick R. microplus, the study of the biotechnological potential of pyrolysis bio-oil can provide the development of new products with acaricidal properties. This work aimed to determine the chemical composition of bio-oil derived from slow pyrolysis of crambe fruit, and the acaricidal activity on larvae and engorged females, and the effect on reproduction of R. microplus.
Crambe fruits were supplied by the MS Foundation (Maracajú, Mato Grosso do Sul, Brazil; 21° 37′49″ S, 55°09′37″ W). The bio-oil from crambe fruit (dry fruit consisting of the pericarp, seed, and husk) was obtained through the slow pyrolysis carried out in a batch reactor of the Laboratory of Synthesis, Chromatography, and Environment (SINCROMA). The reactor consists of: A Heraeus R/O 100 oven; a fixed bed of borosilicate type glass with ground joints, dimensions 1.40 cm × 10 mm; a temperature controller and operating time; a liquid collection system consisting of a condenser, a settling funnel (500 ml) and gas scrubber flasks.
Fifty grams of crambe fruit were subjected to slow pyrolysis at 400°C. The biomass was placed in the central region of a cylindrical glass tube which was introduced into the reactor and connected to the condensation system. Nitrogen gas was continuously applied, 500 ml/min, before and throughout the process. The sample was subjected to a heating rate of 10°C/min and the temperature was then held at 400°C for 2 h. The pyrolytic biochar was trapped in the middle of the reactor and collected after cooling. The non-condensable gases passed through a gas scrubber system and were bubbled in water. The condensable gases, when passing through a condenser, generated the pyrolytic liquid. The liquid fractions were separated from the organic phase (bio-oil) by density difference in a separating funnel.
The characterization of bio-oil was carried out by gas chromatography coupled to mass spectrometry (GC-MS), after fractionation by classical liquid chromatography, using hexane and dichloromethane eluents.
The analyzes by GC-MS were carried out in a Shimadzu apparatus (QP2010), automatic sampler QP, coupled to the Mass Spectrometer, using a fused silica capillary column DB5-MS (20 m × 0.18 mm in internal diameter, 0.18 μm of phenyl polydimethylsiloxane). Helium was employed as a carrier gas at a flow rate of 0.6 ml min–1 and the injector (mode split, 1:10) temperature was 280°C. The initial oven temperature was 40°C (5 min hold) and was ramped to 230°C at 5°C min–1 and 230°C during 10 min. The mass detector was operated under the following conditions: the temperature at 250°C, electron ionization energy, 70 eV; scan range, 40–600 Da; MS interface temperature were maintained at 200°C.
Compounds were identified by comparing mass spectra with the National Institute of Standards and Technology 147 mass spectral library, considering a similarity equal to or greater than 85%. The semiquantitative analysis was done by normalizing the areas of the identified substances.
Crambe fruit bio-oil dissolved in an aqueous solution with 5% of tween 80 (v/v) as an emulsifying agent. Solutions were prepared to obtain the concentrations of 25, 20, 15, 10, and 5% (v/v) for larvae test and 25, 15, and 10% (v/v) for female test. The emulsifying solution (5% tween 80) and distilled water were used as the negative controls. Commercial products containing amitraz (Triatox/MSD saúde animal) and deltamethrin (Butox/MSD saúde animal) were used as positive controls at concentrations of 250 and 25 μg/ml, respectively.
Rhipicephalus microplus ticks of the Porto Alegre, Brazil strain were maintained on infested Hereford bovines acquired from a tick-free area. All bovines were housed in individual tick-proof pens on slatted floors in the Faculty of Veterinary from the Federal University of Rio Grande do Sul, Porto Alegre (UFRGS) and Institute of Veterinary Research Desidério Finamor (FEPAGRO), Brazil (Reck et al., 2009; Ali et al., 2016). After the cycle on the host is completed, fully engorged females dropped from calves were thoroughly washed with tap water and dried on a filter paper towel. Part of the engorged females was used for the adult immersion test (AIT), while others were kept in a bio-oxygen demand (BOD) incubator at 28°C and 70–80% relative humidity (RH) for approximately 20 days to obtain eggs and larvae, which were later used in biological assays.
All experiments were conducted following the guidelines of the Ethics Committee on Animal Experimentation of UFRGS and FEPAGRO, Brazil (institutional approval number 14403).
The larval packet test (LPT) was performed following the methodology defined by FAO (2004). The filter paper packages (3 × 3 cm) were impregnated with 180 μl solution uniformly distributed with a pipette on both sides. About 100 tick larvae, aged around 14–21 days old, approximately, were added to each filter paper package, and the ends were sealed with a staple. The packets were placed in a BOD incubator at 28°C and 70–80% RH for 24 h. After 24 h, the envelopes were opened and inspected using a stereoscope, to record the number of live and dead larvae. Larvae with walking ability are considered alive. Larvae that are immobile or that move but cannot walk are classified as dead. The test was repeated three times with different batches of larvae and performed in duplicate.
The AIT was performed as described by Drummond et al. (1973) with minor modifications. Ticks were distributed to groups randomly (15 engorged females per group). The groups of R. microplus were immersed for 1 min in 3 ml solution of the respective concentrations (25, 15, and 10%) of bio-oils. After this period, ticks were removed from the solution with the aid of a sieve, distributed in Petri dishes (9 cm diameter, 1.5 cm high), weighed, and kept in a BOD incubator at a temperature of 28°C and 70–80% RH. The mortality of the females was evaluated daily for 15 days. The dead ticks were diagnosed using parameter increasing cuticle darkness, hemorrhagic skin lesions, and stopped Malpighian tube movement observed in a stereomicroscope (Pirali-Kheirabadi et al., 2009). After 15 days, the eggs laid were placed in a glass tube, weighed, and observed separately, using the same condition of incubation for the next 30 days for visual estimation of hatching rate. This experiment was performed three times in duplicate.
The percentage inhibition of oviposition (IO) was calculated according to Singh et al. (2015), as follows:
Reproductive index (RI) = average weight of eggs laid (mg)/average weight of females before treatment (g).
Percentage inhibition of oviposition (IO%) = (RI of the control group – RI of treated group/RI control group) × 100.
Engorged females of R. microplus were incubated with crambe fruit bio-oil solution at a concentration of 25%, following the methodology of AIT, and then kept in a BOD incubator, with 70–80% RH and 28°C. The ticks were subsequently dissected 24, 48, and 72 h after immersion, with the aid of a stereomicroscope, using a 0.01 M phosphate-buffered saline solution. The ovary was removed and weighed. A total of 45 females/treatments were used.
To quantify the interference of pyrolysis bio-oil in the development of the ovary, the gonadosomatic index (GSI) was calculated by dividing the total weight of the ovary by the mean body weight of each group of females, both in the control and treated groups, for each period evaluation (Barbosa et al., 2016).
The percentage inhibition of ovarian development (IOD%) was calculated as follows:
Data were expressed as the mean ± SD of the mean. The efficacy was assessed by measuring tick mortality (%) and the lethal concentrations for 50% (LC50) and 90% (LC90) with their 95% confidence limits values were estimated by applying regression equation analysis to the probit data of mortality.
Groups were compared using the one-way ANOVA and the Tukey test. A p value less than 0.05 was considered significant. Statistical analysis was performed using GraphPad Prism 6.0 software.
The yield of coal and pyrolysis liquids were estimated from the mass of each product concerning the mass of raw material, and yield values of the gaseous product were obtained by difference. The yield of crambe fruit pyrolysis products is related to the mass of each product compared to the mass of the raw material used in the process, and the yield values of the gaseous products were obtained by difference. The pyrolysis of the crambe fruit generated a higher bio-oil yield than the aqueous fraction (Table 1). The total mass yield of bio-oil was 34% (w/w). The mains organic functions identified by GC-MS of compounds present in bio-oil were: hydrocarbons, phenol, aldehydes, heterocyclic nitrogenous, ketones, nitrile, amides, and ester. Analysis of hexane fraction showed the presence of substances derived mainly from pyrolysis of triacylglycerides contained in the seed, such as linear alkanes, alkenes, and alkynes, alkadienes, mononuclear alkylbenzenes, aldehydes, carboxylic acids, and esters. All alkanes and alkenes identify in this fraction had similarities above 95%. High molecular mass nitriles (C16 – C19) were also identified in this fraction. Semiquantitative analysis of hexane fraction performed by normalizing the areas of all identified compounds showed that major compounds were: heneicos-9-ene, from the thermochemical conversion of erucic acid; heneicos-1-ene; hexadec-11-al; heptadec-1-ene; and heneicosane. We were identified this compounds in a small proportion a phytosterol (β-tocopherol), high mass molar hydrocarbons, and steroids as ergostenol, ergostene, stigmastenol, and cholestanone. Some of these substances have already been identified in crambe fruit bio-oil (Silva et al., 2019). In fraction eluted with dichloromethane, the predominance was phenols and methoxy-phenols from the thermal decomposition of the lignocellulosic part of the pericarp and N-heterocycles compounds such as pyridines, pyrroles, and pyrazines. Nitrogen compounds come from the pyrolysis of crambe fruit seed proteins. Phenolic compounds were the majority in this fraction (Table 2).
Table 2. Identification and quantification by GC-MS of the main compounds present in the crambe fruit bio-oil.
The acaricidal effect of bio-oils was tested using larvae of R. microplus. In LPT it was observed that the crambe fruit bio-oil, in all concentrations, caused significant mortality of R. microplus larvae 24 h after treatment. At a concentration of 15%, crambe bio-oil reached 91.6% of larval mortality (Table 3). The commercial acaricide amitraz was not effective against larvae and deltamethrin caused low mortality of larvae (45%). The calculation of regression analysis of larvae showed an LC50 and LC90 of 7.6 and 14.4%, respectively (Table 4).
Table 3. Evaluation of the larval packet test of Rhipicephalus microplus after treatment with pyrolysis bio-oil of crambe fruit.
Table 4. Lethal concentration of 50% (LC50) and 90% (LC90) obtained through larval packet test of Rhipicephalus microplus treated with bio-oil, 24 h after treatment.
Figure 1 describes the AIT results for the crambe fruit bio-oil in different concentrations showing R. microplus female mortality. The acaricidal effect was elevated in all concentrations tested, causing high female mortality and inhibiting oviposition. At a concentration of 15%, crambe bio-oil exhibited mortality of 91.1% on the 15th day after treatment. When used at 10% concentration (v/v), the effect of bio-oil was less than that found by the commercial acaricide deltamethrin.
Figure 1. Mortality of Rhipicephalus microplus females exposed to different concentrations of crambe fruit bio-oil. (A) Crambe fruit bio-oil in different concentrations; (B) positive and negative controls.
In the AIT the efficacy of treatment against engorged females was also evaluated by measuring egg production. Results showed that the IO of the crambe fruit bio-oil differed significantly from the negative controls (p < 0.0001) at all concentrations tested. The bio-oil at a concentration of 15% showed an IO similar to the commercial acaricide amitraz (Table 5).
Table 5. Percentage of mortality, reproductive index (RI), and inhibition of oviposition (IO) of engorged females of Rhipicephalus microplus exposed to crambe fruit bio-oil in different concentrations.
To analyze the interference of crambe fruit bio-oil in ovarian development, engorged females were treated with bio-oil at a concentration of 25% and dissected 24, 48, and 72 h after treatment. Females treated with bio-oil showed a reduction in GSI in all the periods evaluated, however, only the reduction in 72 h of exposure was significant (Figure 2).
Figure 2. Gonadosomatic index of Rhipicephalus microplus tick females exposed 24, 48, and 72 h to crambe fruit pyrolysis bio-oil (25% concentration). Tween 80 (5%) was used as a negative control. The experimental number was 45 females/treatment. The results are expressed as mean ± SD, **p < 0.05 (two-way ANOVA).
During dissection, it was observed that the ovaries of females in groups treated with crambe fruit bio-oil had small and whitish oocytes after 72 h, therefore, little developed (Figure 3). In addition, there are no mature oocytes in the oviduct, whereas in the ovary of the control group, its presence can be observed.
Figure 3. Ovaries of engorged females treated with 5% tween 80 (negative control) (A) and females treated with the crambe fruit pyrolysis bio-oil (B) obtained after 72 h of treatment. Red arrow: oviduct; green arrow: poorly developed oocytes (increase: 20×). Bar = 1 cm.
Liquid pyrolysis products have been the target of studies evaluating biocidal effects in different organisms. Bio-oils have a high yield and a rich chemical composition, which varies according to the biomass used and the conditions of the thermochemical process. Slow pyrolysis of crambe fruit gave rise to a high bio-oil yield. The general distribution of the yield of crambe fruit pyrolysis products was like that described in the literature (Silva et al., 2019).
Results of this study show that slow pyrolysis of crambe fruit produced a bio-oil with toxic effect in R. microplus. This is the first report demonstrating the effect of pyrolysis bio-oil as an acaricide. A previous study carried out by Lindqvist et al. (2009) evaluated the acaricide effect of a pyrolysis bio-oil (birch tar oil) against Phytonemus pallidus (Acari: Tarsonemidae) and Tetranychus urticae (Acari: Tetranychidae) mites. However, the bio-oil was not toxic to mites. Most studies evaluating the effect of pyrolysis products on arthropods have been carried out with insects and this is an area of research that has not yet been fully explored (Mattos et al., 2019).
Crambe fruit bio-oil caused high mortality of R. microplus larvae in 24 h, with an LC90 of 14.4%. Similarly, some bio-oils also showed the larvicidal effect on larvae of Colorado potato beetle Leptinotarsa decemlineata (Coleoptera: Chrysomelidae). Pyrolysis bio-oil from dried coffee grounds and tobacco-caused 100% mortality of CPB larvae in 48 h at a concentration of 50 and 100%, respectively (Booker et al., 2010; Bedmutha et al., 2011), concentrations higher than that found with the crambe bio-oil in R. microplus. These studies, different from ours, used fast pyrolysis at higher temperatures (400–600°C). The fast pyrolysis process at temperatures above 500°C, although generate a high yield of bio-oil (Bridgwater and Peacocke, 2000; Bridgwater, 2012), typically form polycyclic aromatic hydrocarbons (Simko, 2005; Mohan et al., 2006), toxic substances to humans and environment (EPA, 2008; Wu et al., 2011).
Crambe fruit bio-oil significantly interfered in tick reproduction, inhibiting egg development and egg-laying. At a lower concentration (10%), mortality was gradual, reaching 65.5% mortality only on the 15th day after treatment. However, on the fourth day, which would be the peak of egg-laying (Hitchcock, 1955), only 3% of the females had died, which does not explain the high inhibition of egg-laying observed. These results show that crambe fruit bio-oil, in addition to being toxic to females tick causing their death, also prevents them from laying eggs, contributing to stopping the tick cycle.
Thus, it was investigated if there was a metabolic interference, impairing the development of eggs or whether the organs associated with the egg-laying process (Genet’s organ, for example) may have been affected. Treated R. microplus females were dissected to analyze the ovaries. Crambe fruit bio-oil significantly reduced the GSI by more than half after 72 h. Barbosa et al. (2016) also observed a strong decrease in GSI and the number of oocytes when R. microplus engorged females were treated with 3β-O-tigloylmelianol, a protolimonoid isolated from Guarea kunthiana (Meliaceae).
According to Balashov (1983), oogenesis can be divided into five stages. Phases III and IV of oogenesis consist of the period of formation of yolk granules by oocytes, and at the end of this period, the oocyte is ready for ovulation. During ovulation (stage V), the oocyte passes through the ovary lumen, is moved by peristaltic movements to the oviduct and, finally, to the vagina (Balashov, 1983; Denardi et al., 2004). The oocytes of R. microplus females treated with crambe fruit bio-oil were poorly developed. As the females were incubated after feeding and unformed eggs were seen in the ovary, there may be a change in stage III or IV of oogenesis in females treated with crambe fruit bio-oil. In addition, unlike the control group, there were no oocytes in the oviduct, indicating that the oocytes did not reach the last stage of development (V). Our group also evaluated ovaries of tick females treated with pyrolysis bio-oils from other biomasses, and there was no significant reduction in GSI (data not shown).
Bio-oils have a large number of organic compounds, and this chemical composition is dependent on raw material and the process conditions used. The acaricidal activity found may be associated with a synergistic effect between the various substances present in crambe fruit bio-oil. Phenolic compounds, fatty acids, and acetic acid have been found in liquid pyrolysis products from plant biomass with important insecticidal properties (Yatagai et al., 2002; Bedmutha et al., 2011; Kartal et al., 2011; Suqi et al., 2014; Cáceres et al., 2015). However, there are still few studies evaluating the effect of pyrolysis products on arthropods, and not all have evaluated the chemical composition (Mattos et al., 2019). Crambe fruit bio-oil exhibited many hydrocarbons in its hexane fraction. Hydrocarbons have already been found as major compounds in pyrolysis bio-oils of coffee grounds and macadamia nutshells, and these bio-oils were active against CPB and the termite Coptotermes formosanus, respectively (Yatagai et al., 2002; Bedmutha et al., 2011).
These interesting data show us that crambe fruit pyrolysis bio-oil is a promising acaricide against the cattle tick R. microplus. However, additional research must be carried out to investigate issues related to environmental safety, to develop a safe product for the population.
Slow pyrolysis of crambe fruits produces a bio-oil in high yield, containing several organic compounds being a good source for developing new biotechnological products. This is the first report of the use of pyrolysis bio-oil as an acaricide. The results of this study demonstrate the acaricidal effect of crambe fruit bio-oil on larvae and engorged females of R. microplus. In addition, bio-oil interferes with tick reproduction, inhibiting egg-laying. Crambe fruit bio-oil is, therefore, a potential alternative of sustainable product for control R. microplus.
The raw data supporting the conclusions of this article will be made available by the authors, without undue reservation.
The animal study was reviewed and approved by the Ethics Committee on Animal Experimentation of UFRGS and FEPAGRO, Brazil.
GA, MC, and EF contributed to the conception and design of the study. CM and JA organized the database. CM performed the statistical analysis and wrote the first draft of the manuscript. CM, JA, and NT performed tick experiments. BS performed pyrolysis conversion. BS, CM, MC, and GA performed the chemical analysis. CM and EF wrote the final manuscript. All authors contributed to manuscript revision, read, and approved the submitted version.
The authors are grateful to Fundação de Amparo à Pesquisa do Estado do Rio de Janeiro (FAPERJ), Conselho Nacional de Desenvolvimento Científico e Tecnológico (CNPq), Coordenação de Aperfeiçoamento de Pessoal de Nível Superior (CAPES), and Programa de Fomento à Pesquisa – Universidade Federal Fluminense (FOPESQ–UFF) for their financial support to the present work. This work was also supported by FAPERJ Scholarship E-26/200.584/2021.
The authors declare that the research was conducted in the absence of any commercial or financial relationships that could be construed as a potential conflict of interest.
All claims expressed in this article are solely those of the authors and do not necessarily represent those of their affiliated organizations, or those of the publisher, the editors and the reviewers. Any product that may be evaluated in this article, or claim that may be made by its manufacturer, is not guaranteed or endorsed by the publisher.
The authors are particularly grateful to Itabajara da Silva Vaz Junior, and our group, at the Federal University of Rio Grande do Sul, Porto Alegre (UFRGS) for their provision of ticks used in this work.
Ali, A., Khan, M. A., Zahid, H., Yaseen, P. M., Qayash Khan, M., Nawab, J., et al. (2019). Seasonal dynamics, record of ticks infesting humans, wild and domestic animals and molecular phylogeny of Rhipicephalus microplus in Khyber Pakhtunkhwa Pakistan. Front. Physiol. 10:793. doi: 10.3389/fphys.2019.00793
Ali, A., Parizi, L. F., Ferreira, B. R., and Vaz Junior, I. S. (2016). A revision of two distinct species of Rhipicephalus: R. microplus and R. australis. Cienc. Rural 46, 1240–1248. doi: 10.1590/0103-8478cr20151416
Araújo, L. X., Novato, T. P. L., Zeringota, V., Matos, R. S., Senra, T. O. S., Maturano, R., et al. (2015). Acaricidal activity of thymol against larvae of Rhipicephalus microplus (Acari: Ixodidae) under semi-natural conditions. Parasitol. Res. 114, 3271–3276. doi: 10.1007/s00436-015-4547-3
Balashov, Y. S. (1983). “The female reproductive system,” in An Atlas of Ixodid Tick Ultrastructure, ed. Y. S. Balashov (Annapolis, MD: Entomological Society of America), 98128.
Barbosa, C. S., Borges, L. M. F., Louly, C. C. B., Rocha, T. L., Sabóia-Morais, S. M. T., Miguita, C. H., et al. (2016). In vitro activity of 3β-O-tigloylmelianol from Guarea kunthiana A. Juss (Meliaceae) on oogenesis and ecdysis of the cattle tick Rhipicephalus (Boophilus) microplus (Canestrini) (Acari: Ixodidae). Exp. Parasitol. 164, 5–11. doi: 10.1016/j.exppara.2016.01.015
Bassegio, D., Santos, R. F., Sarto, M. V. M., Bassegio, C., Dias, P. P., Martins, J. D. L., et al. (2016). Short-term green manure effects on crambe yield and oil content. Aust. J. Crop. Sci. 10, 1618–1622. doi: 10.21475/ajcs.2016.10.12.PNE136
Bedmutha, R., Booker, C. J., Ferrante, L., Briens, C., Berruti, F., Yeung, K. K. C., et al. (2011). Insecticidal and bactericidal characteristics of the bio-oil from the fast pyrolysis of coffee grounds. J. Anal. Appl. Pyrol. 90, 224–231. doi: 10.1016/j.jaap.2010.12.011
Booker, C. J., Bedmutha, R., Scott, I. M., Conn, K., Berruti, F., Briens, C., et al. (2010). Bioenergy II: characterization of the pesticide properties of tobacco bio-oil. Int. J. Chem. Reactor Eng. 8, 1–14. doi: 10.2202/1542-6580.1939
Bridgwater, A. V. (2012). Review of fast pyrolysis of biomass and product upgrading. Biomass Bioenerg. 38, 68–94. doi: 10.1016/j.biombioe.2011.01.048
Bridgwater, A. V., and Peacocke, G. V. C. (2000). Fast pyrolysis processes for biomass. Renew Sustain. Energy Rev. 4, 1–73. doi: 10.1016/S1364-0321(99)00007-6
Cáceres, L. A., McGarvey, B. D., Briens, C., Berruti, F., Yeung, K. K. C., and Scott, I. M. (2015). Insecticidal properties of pyrolysis bio-oil from greenhouse tomato residue biomass. J. Anal. Appl. Pyrol. 112, 333–340. doi: 10.1016/j.jaap.2015.01.003
Canestrini, G., and Fanzago, F. (1887). Intorno agli Acari italiani. Inst. Veneto Sci. Lett. Ed. Arti. 4, 69–208.
Czernik, S., and Bridgwater, A. V. (2004). Overview of applications of biomass fast pyrolysis oil. Energy Fuel. 18, 590–598. doi: 10.1021/ef034067u
Denardi, S. E., Bechara, G. H., Oliveira, P. R., Nunes, E. T., Saito, K. C., and Mathias, M. I. (2004). Morphological characterization of the ovary and vitellogenesis dynamics in the tick Amblyomma cajennense (Acari: Ixodidae). Vet. Parasitol. 125, 379–395. doi: 10.1016/j.vetpar.2004.07.015
Drummond, R. E. A., Ernst, S. E., Trevino, J. L., Gladney, W. J., and Graham, O. H. (1973). Boophilus annulatus and B. microplus: laboratory tests of insecticides. J. Econ. Entomol. 66, 130–133. doi: 10.1093/jee/66.1.130
EPA (2008). ). Polycyclic Aromatic Hydrocarbons. Available online at: http://www.epa.gov/osw/hazard/wastemin/minimize/factshts/pahs.pdf (accessed January 22, 2021)
Falasca, S. L., Flores, N., Lamas, M. C., Carballo, S. M., and Anschau, A. (2010). Crambe abyssinica: an almost unknown crop with a promissory future to produce biodiesel in Argentina. Int. J. Hydrog. Energy 35, 5808–5812. doi: 10.1016/j.ijhydene.2010.02.095
FAO (2004). Module 1. Ticks: Acaricide Resistance: Diagnosis Management and Prevention In: Guidelines Resistance Management and Integrated Parasite Control in Ruminants. Rome: FAO Animal Production and Health Division.
Fernández-Salas, A., Rodríguez-Vivas, R. I., and Alonso-Díaz, M. A. (2012). First report of a Rhipicephalus microplus tick population multi-resistant to acaricides and iver-mectin in the Mexican tropics. Vet. Parasitol. 183, 338–342. doi: 10.1016/j.vetpar.2011.07.028
Higa, L. O. S., Garcia, M. V., Barros, J. C., Koller, W. W., and Andreotti, R. (2015). Acaricide resistance status of the Rhipicephalus microplus in Brazil: a literature overview. Med. Chem. 5, 326–333. doi: 10.4172/2161-0444.1000281
Hitchcock, L. F. (1955). Studies of the non-parasitic stages on the cattle tick, Boophilus microplus (Canestrini) (Acarina: Ixodidade). Austr. J. Zool. 3, 295–311. doi: 10.1071/ZO9550295
Hu, J., Deng, Z., Wang, B., Zhi, Y., Pei, B., Zhang, G., et al. (2015). Influence of heavy metals on seed germination and early seedling growth in Crambe abyssinica, a potential industrial oil crop for phytoremediation. Am. J. Plant Sci. 6:150. doi: 10.4236/ajps.2015.61017
Ionov, M., Yuldasheva, N., Ulchenko, N., Glushenkova, A. I., and Heuer, B. (2013). Growth, development and yield of Crambe abyssinica under saline irrigation in the greenhouse. J. Agron. Crop. Sci. 199, 331–339. doi: 10.1111/jac.12027
Kartal, S. N., Terzi, E., Kose, C., Hofmeyr, J., and Imamura, Y. (2011). Efficacy of tar oil recovered during slow pyrolysis of macadamia nutshells. Int. Biodeterior. Biodegradation 65, 369–373. doi: 10.1016/j.ibiod.2010.08.011
Klafke, G., Webster, A., Agnol, B. D., Pradel, E., Silva, J., La Canal, L. H., et al. (2017). Multiple resistance to acaricides in field populations of Rhipicephalus microplus from Rio Grande do Sul State, Southern Brazil. Ticks Tick Borne Dis. 8, 73–80. doi: 10.1016/j.ttbdis.2016.09.019
Kraiem, T., Hassen, A. B., Belayouni, H., and Jeguirim, M. (2016). Production and characterization of bio-oil from the pyrolysis of waste frying oil. Environ. Sci. Pollut. Res. 24, 9951–9961. doi: 10.1007/s11356-016-7704-z
Lew-Tabor, A. E., and Valle, M. R. (2016). A review of reverse vaccinology approaches for the development of vaccines against ticks and tick borne diseases. Ticks Tick Borne Dis. 7, 573–585. doi: 10.1016/j.ttbdis.2015.12.012
Lindqvist, I., Lindqvist, S., Tuovinen, T., Nissinen, A., Korpela, S., Tiilikkala, K., et al. (2009). “The potential of botanical birch tar oil for insect pest control,” in Koivutisle – Kasvinsuojelun Uusi Innovaatio, Vol. 143, eds K. Tiilikkala and M. Segerstedt (Jiokioinen: MTT Agrifood Research Finland), 55–72.
Mattos, C., Veloso, M. C. C., Romeiro, G. A., and Folly, E. (2019). Biocidal applications trends of bio-oils from pyrolysis: characterization of several conditions and biomass, a review. J. Anal. Appl. Pyrol. 139, 1–12. doi: 10.1016/j.jaap.2018.12.029
Mohan, D., Pittman, C. U., and Steele, P. H. (2006). Pyrolysis of wood/biomass for bio-oil: a critical review. Energy Fuel. 20, 848–889. doi: 10.1021/ef0502397
Pirali-Kheirabadi, K., Razzaghi-Abyaneh, M., and Halajian, A. (2009). Acaricidal effect of Pelargonium roseum and Eucalyptus globulus essential oils against adult stage of Rhipicephalus (Boophilus) annulatus in vitro. Vet. Parasitol. 162, 346–349. doi: 10.1016/j.vetpar.2009.03.015
Reck, J., Berger, M., Terra, R. M. S., Marks, F. S., Vaz, I. S. Jr., Guimarães, J. A., et al. (2009). Systemic alterations of bovine hemostasis due to Rhipicephalus (Boophilus) microplus infestation. Res. Vet. Sci. 86, 56–62. doi: 10.1016/j.rvsc.2008.05.007
Reck, J., Klafke, G. M., Webster, A., Dall’Agnol, B., Scheffer, R., Souza, U. A., et al. (2014). First report of fluazuron resistance in Rhipicephalus microplus: a field tick population resistant to six classes of acaricides. Vet. Parasitol. 201, 128–136. doi: 10.1016/j.vetpar.2014.01.012
Samarappuli, D., Zanetti, F., Berzuini, S., and Berti, M. T. (2020). Crambe (Crambe abyssinica Hochst): a non-food oilseed crop with great potential: a review. Agronomy 10:1380. doi: 10.3390/agronomy10091380
Silva, R. V., Pereira, V. B., Stelzer, K. T., Almeida, T. A., Romeiro, G. A., and Azevedo, D. A. (2019). Comprehensive study of the liquid products from slow pyrolysis of crambe seeds: bio-oil and organic compounds of the aqueous phase. Biomass Bioenerg. 123, 78–88. doi: 10.1016/j.biombioe.2019.02.014
Silva, R. V. S., Casilli, A., Sampaio, A. L., Ávila, B. M., Veloso, M. C., Azevedo, D. A., et al. (2014). The analytical characterization of castor seed cake pyrolysis bio-oils by using comprehensive GC coupled to time of flight mass spectrometry. J. Anal. Appl. Pyrol. 106, 152–159. doi: 10.1016/j.jaap.2014.01.013
Simko, P. (2005). Factors affecting elimination of polycyclic aromatic hydrocarbons from smoked meat foods and liquid smoke flavorings. Mol. Nut. Food Res. 49, 637–647. doi: 10.1002/mnfr.200400091
Singh, N. K., Vemu, B., Singh, H., Prerna, M., Daundkar, P. S., Sharma, S. K., et al. (2015). In vitro acaricidal activity of Murraya koenigii (L.) Spreng (Rutaceae) extracts against synthetic pyrethroid-resistant Rhipicephalus (Boophilus) microplus. Parasitol. Res. 114, 1531–1539. doi: 10.1007/s00436-015-4337-y
Suqi, L., Cáceres, L., Schieck, K., Booker, C. J., Mcgarvey, B. M., Yeung, K. K. C., et al. (2014). Insecticidal activity of bio-oil from the pyrolysis of straw from Brassica spp. J. Agr. Food Chem. 62, 3610–3618. doi: 10.1021/jf500048t
Wu, B., Zhang, R., Cheng, S., Ford, T., Li, A., and Zhang, X. (2011). Risk assessment of polycyclic aromatic hydrocarbons in aquatic ecosystems. Ecotoxicology 20, 1124–1130. doi: 10.1007/s10646-011-0653-x
Keywords: slow pyrolysis, bio-oil, crambe fruit, acaricidal activity, cattle tick, ecofriendly
Citation: Mattos C, Andrade J, Salarini Peixoto B, Tavares Moraes NL, da Cunha Veloso MC, Alves Romeiro G and Folly E (2021) Acaricidal Properties of Bio-Oil Derived From Slow Pyrolysis of Crambe abyssinica Fruit Against the Cattle Tick Rhipicephalus microplus (Acari: Ixodidae). Front. Physiol. 12:768522. doi: 10.3389/fphys.2021.768522
Received: 31 August 2021; Accepted: 29 October 2021;
Published: 02 December 2021.
Edited by:
Sylvia Anton, Institut National de la Recherche Agronomique (INRA), FranceReviewed by:
Abid Ali, Abdul Wali Khan University Mardan, PakistanCopyright © 2021 Mattos, Andrade, Salarini Peixoto, Tavares Moraes, da Cunha Veloso, Alves Romeiro and Folly. This is an open-access article distributed under the terms of the Creative Commons Attribution License (CC BY). The use, distribution or reproduction in other forums is permitted, provided the original author(s) and the copyright owner(s) are credited and that the original publication in this journal is cited, in accordance with accepted academic practice. No use, distribution or reproduction is permitted which does not comply with these terms.
*Correspondence: Evelize Folly das Chagas, ZXZlbGl6ZWZvbGx5QHlhaG9vLmNvbS5icg==; ZWZvbGx5QGlkLnVmZi5icg==; orcid.org/0000-0001-6313-2447
Disclaimer: All claims expressed in this article are solely those of the authors and do not necessarily represent those of their affiliated organizations, or those of the publisher, the editors and the reviewers. Any product that may be evaluated in this article or claim that may be made by its manufacturer is not guaranteed or endorsed by the publisher.
Research integrity at Frontiers
Learn more about the work of our research integrity team to safeguard the quality of each article we publish.