- 1Department of Anaesthesiology, Spital Grabs, Grabs, Switzerland
- 2Department of Anaesthesiology and Pain Medicine, Bern University Hospital (Inselspital), University of Bern, Bern, Switzerland
- 3Department of Congenital and Pediatric Heart Surgery, German Heart Center Munich, Technical University, Munich, Germany
- 4Department of Cardiovascular Surgery, Bern University Hospital (Inselspital), Bern, Switzerland
- 5Department of Cardiovascular Surgery, University Hospital Zurich, Zurich, Switzerland
Indoleamine-2,3-dioxygenase (IDO) is the “rate-limiting” enzyme in the kynurenine (Kyn) pathway of the tryptophan (Trp) catabolism. By its immune-modulatory effect, IDO initiates changes to the physiologically balanced immune state and plays a key role in the pathogenesis of various diseases, as well as in the perioperative setting during surgery. In autoimmune processes, highly malignant cancers such as glioblastoma or organ transplantation, IDO’s involvement has been studied extensively. However, in severe systemic infections, as present in sepsis, it is not yet completely understood. Hereafter, in this narrative review, we present the current knowledge of IDO’s implication on such complex immune-related processes. Moreover, we address the role of IDO as a predictive biomarker as well as a therapeutic target for immune-mediated diseases. Finally, we discuss IDO in the setting of surgical trauma-induced stress and highlight its promising use as a biomarker in the pre-operative setting for all disciplines involved in the decision-making process and treatment of patients undergoing surgery.
The Physiological Role of Indoleamine-2,3-Dioxygenase
Physiologically, homeostasis in the immune system is always maintained through a balance between protection from infections versus prevention of excessive autoimmune reactions. Cellular immune response including B- and T-lymphocytes has a major role in the maintenance of an immunological equilibrium within the individual immune system. In addition, they play an important role in self-tolerance (Abbas and Pillai, 2019).
The differentiation of T cells into different subtypes with diverse activities depends greatly on so-called fate-specific cytokines and receptors (Abbas and Pillai, 2019). Nowadays, it is thought that the differentiation of cluster of differentiation (CD-)4 naive cells into T regulatory cells (Tregs) is caused by a combination of T-cell receptor activation, CD-28 signaling, FOX-P3 stimulation (Fontenot et al., 2003) via interleukin (IL-)2, and many other cytokines (IL-15 and IL-7), as well as individual interactions by themselves (not via FOXP3), including NFAT/AP1, ICOS/ICOSL, and thymic stromal lymphopoietin (Watanabe et al., 2005; Mantel et al., 2006; Nazzal et al., 2014).
T regulatory cells then interact with other T cells, natural killer cells, the host cell membrane, B cells, and monocytes. Some important anti-inflammatory mediators mitigating these effects include transforming growth factor beta (TGF-β) IL-2, interaction of programmed death-ligand 1 (PD-L1) and programmed cell death protein 1 (PD-1) (with B cells), cytotoxic T-lymphocyte-associated protein (CTLA-4), and lymphocyte-activation gene 3 (LAG3). CTLA-4 has important downstream effects on dendritic cells (DCs): it decreases antigen-capturing and antigen-presenting capability and induces indoleamine-2,3-dioxygenase (IDO) (Schmidt et al., 2012).
Indoleamine-2,3-dioxygenase catalyzes the rate-limiting step in the Kyn pathway, catabolizing the essential amino acid tryptophan (Trp). Trp catabolism’s Kyn arm is dependent on IDO, which exists in two isoforms: IDO1 and IDO2. IDO2 has a modest enzymatic potential (Pantouris et al., 2014) and has received less attention. Tryptophan-2,3-dioxygenase is the third enzyme engaged in the Kyn pathway. As a result, our review will focus exclusively on the role of IDO1.
This enzyme catalyzes the reaction of Trp to N-formylkynurenine, leading to the production of Kyn metabolites. Kyn metabolites are broken down into metabolites such as picolinic acid, quinolinic acid, and kynurenic acid. These metabolites are actively involved in neuroinflammatory diseases and also serve as a substrate for the generation of nicotinamide adenine dinucleotide (NAD), a key molecule for cellular repair, energy substrate, and fatigue (Figure 1).
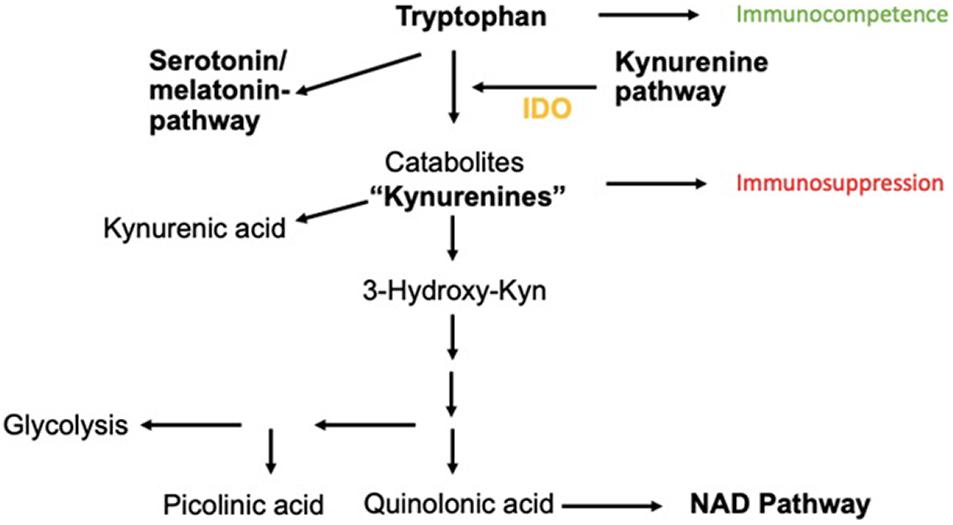
Figure 1. Catabolism of Trp via Kyn-pathway. The neuroinflammatory metabolites kynurenic acid, picolinic acid, and quinolinic acids are shown as well as the transition to the NAD pathway important for cellular energy generation.
Cell-mediated immune response is massively regulated by IDO depending on immunosuppressive effects. It is a potentially ground-breaking pathway that could serve as a target for therapy of many human immune-mediated diseases, ranging from autoimmune disease (Anquetil et al., 2018) to chronic infections. In addition, it may serve as a marker of immune status, e.g., in the perioperative setting.
Which Tissues Express Indoleamine-2,3-Dioxygenase?
Indoleamine-2,3-deoxygenase is expressed and normally active in many tissues, such as placental endothelial cells, parenchymal lung cells, cells of the epithelial lining of the female genital tract, and most relevant for this review, DCs (Théate et al., 2015). There is extensive interest in the inducibility of IDO1. While not constitutively expressed, its transcription can be activated or enhanced under certain conditions; for example, in and around tumor cells or in antigen-presenting cells. In addition, endothelial cells, innate immune cells, mesenchymal cells, lymph nodes draining tumor tissue (Prendergast et al., 2018), and mononuclear blood cells (Meireson et al., 2020) can express IDO. In addition, the expression of IDO increases in elderly people (Marttila et al., 2011).
The mechanisms by which the enhancement of this crucial enzyme is mediated are not completely understood. There are numerous regulatory and modulatory coenzymes involved in the Trp metabolism, influencing a balanced state between serotonin and Kyn. One of these is Coenzyme Q (Abuelezz et al., 2017). Also, stress can induce the Kyn pathway, while exercise can lead to a decrease in stress-induced high Kyn levels (Agudelo et al., 2014; Kirmeier et al., 2019). Flavonoids such as curcumin deactivate IDO interaction with the aryl hydrocarbon receptor (AhR) (Busbee et al., 2013). Through modulation of the AhR, such substances can alter macrophage IDO activity and block interferon (IFN-)gamma-induced IDO activity (Jeong et al., 2009). Although not the main promoting factor for IDO expression (Taylor and Feng, 1991), IFN-y plays an immunomodulatory role by affecting response to viral and bacterial infections, promoting “leukocyte trafficking,” and boosting the processing of antigens and apoptosis of infected cells.
Effector T-cells produce IFN-y in infective states. Together with pattern recognition receptors (PRRs) and cytokines such as tumor necrosis factor (TNF-)alpha, IL-4 lipopolysaccharide, and Type-I interferons, IFN-y then acts as a key initiator of the pro-inflammatory cascade (Kak et al., 2018) (Figure 2).
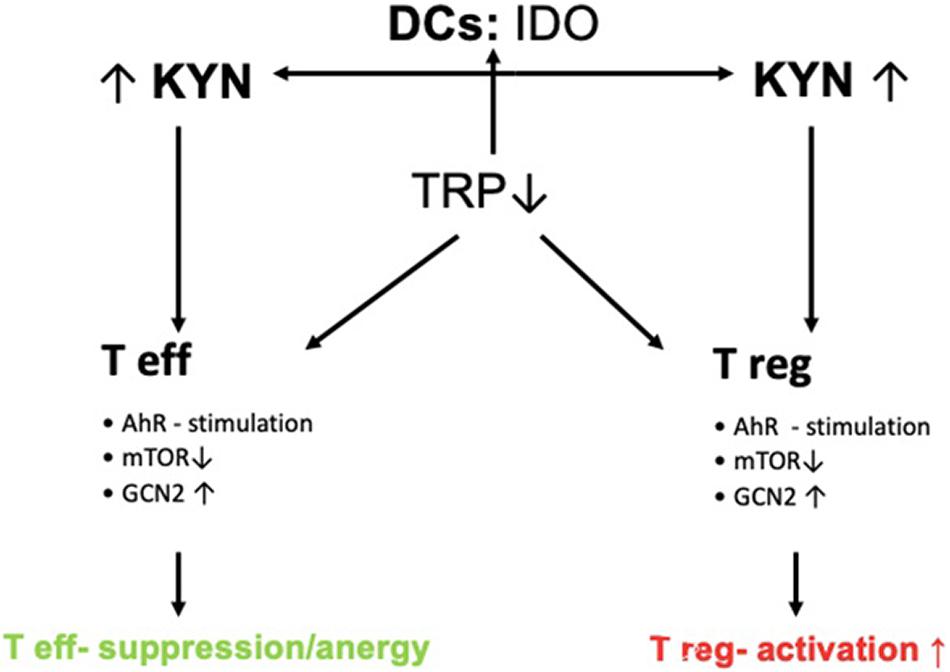
Figure 2. Control of T-cell response by IDO. DCs express IDO thereby leading to increased Kyn-metabolite production. Increased Kyn-metabolites stimulate AhR and GCN2 and inhibit mTOR thereby leading to the activation of Tregs and inducing tolerance via suppression of effector T-cells (Teffs).
In addition, it is a potent initiator of the Kyn pathway. Other IDO-elevating factors include IL-10, IL-27, the cytokine production of Treg cells including TGF-β, IL-10, and adenosine or Treg-expression profile of CTLA-4. These lead to enhanced IDO1 secretion by DCs as well as cyclooxygenase (COX-)2 and prostaglandin E2 (PGE2). COX-2 and PGE2 are associated with IDO1 expression in tumor cells (Hornyák et al., 2018). Once the balance of Trp metabolism is shifted to the Kyn pathway, Kyn products induce a self-enforcing feedback loop involving IDO expression. This paves the way for a vicious cycle during inflammation (Litzenburger et al., 2014) (Figure 3).
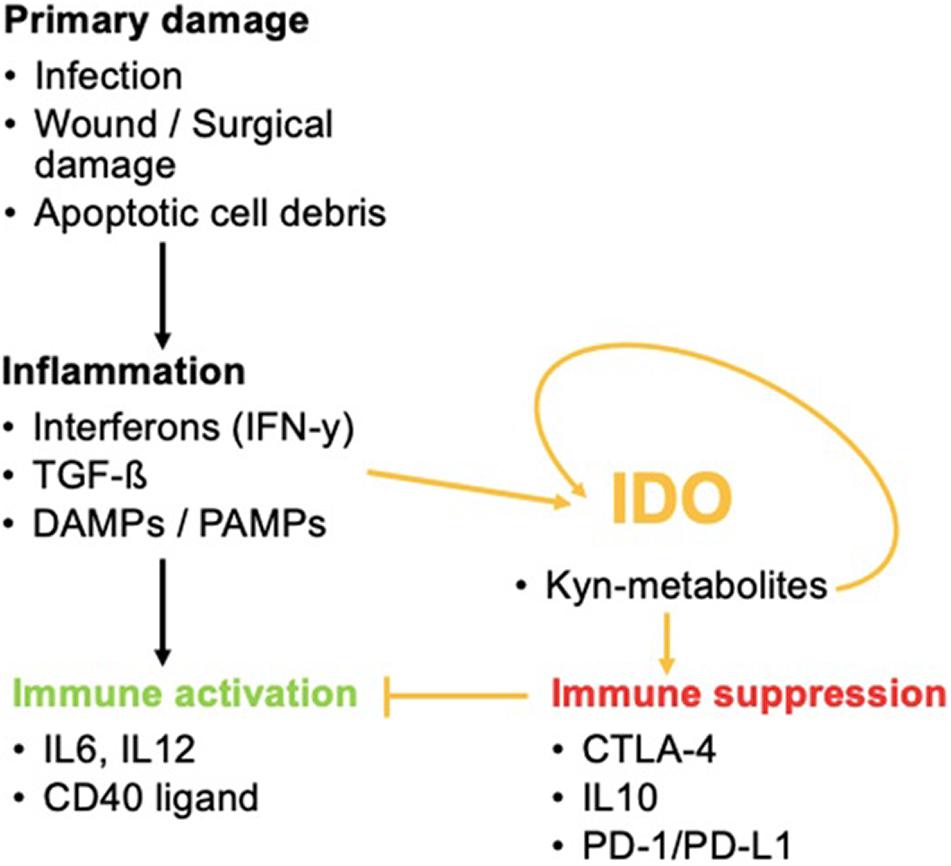
Figure 3. The role of IDO in inflammatory processes. IDO activation by inflammation favors immune tolerance and hinders immune activation that would normally occur via the activation of pro-inflammatory cytokines. IDO can even promote its own activity once activated.
Pathways by Which Immune Regulatory Function Is Mitigated
Once activated, there are three main pathways by which the immune regulatory function of IDO is mitigated. First, Trp catabolites produced by IDO bind to the AhR. This receptor is a cytosolic transcription factor. Via IL-6-induced signal transducer and activator of transcription protein (STAT-)3 signaling, the differentiation of Treg and DCs is promoted and apoptosis of T helper (Th)1 cells is induced. Second, the Kyn pathway is stimulated, activating a positive feedback loop until depletion of this amino acid. Immune cells lacking Trp are suppressed, while tolerogenic immune cells are activated (Zhai et al., 2018). In detail, general control non-depressible 2 (GCN2) kinase and mechanistic target of rapamycin (mTOR, previously referred to as the mammalian target of rapamycin) sense low tryptophan levels and promote anergy as well as differentiation into regulatory types in effector T-cells (Teffs) (Munn and Mellor, 2013). Also, Th17 diffferentiation is altered (Albini et al., 2017). The third pathway of immune modulation that has been identified is executed via nuclear factor kappa-light-chain-enhancer of activated B cells (NF-κB). IDO may act as a signaling protein inducing NF-κB, which then joins TGF-β in favoring the survival of regulatory plasmacytoid DCs. These cells suppress T-cell activity and promote Treg formation, while keeping the production of pro-inflammatory cytokines at a low level. The effect of IDO might not be limited to plasmacytoid DCs, but rather lead via non-enzymic function to an upregulation of Src homology 2 domain phosphatase (SHP-)1 and SHP-2 receptors on DCs. This in turn favors binding of SHP1/2 to immune tyrosine-based inhibitory motif (ITIM)1 over ITIM2. In this way, a cascade is finally activated, leading to long-term expression of the IDO gene in the nucleus of DCs of the plasmacytoid and conventional type (Pallotta et al., 2011).
Lately, IDO has been found to have a direct anti-infective effect by suppressing the replication of certain bacteria and parasites as well as preventing viral spread. Trp depletion also seems to have a bactericidal effect, as found in infections with toxoplasma, or to serve as a virostatic agent through depletion of IFN-y in people infected with cytomegalovirus. This effect could be reversed by the administration of exogenous tryptophan. Altering such regulatory effects of IDO may also help certain pathogens infect human cells without being noticed by the immune system. Uropathogenic Escherichia coli induce IDO in epithelial cells, thereby successfully colonizing urinary epithelium (Schmidt and Schultze, 2014).
The role of IDO is complex, and there are many ongoing studies looking into the effects of various signaling proteins involved in the immune response to IDO. The extent of IFN-y-induced activation of IDO, for example, can be altered by B lymphocyte-induced maturation protein-1 (BLIMP-1) binding to the IFN-y binding site on IDO (Barnes et al., 2009). BLIMP-1 itself is promoted by GCN2, and thereby plays a key role in regulating IDO activation in response to GCN2-sensed Trp-depletion. mTOR-dependent IDO activation via response to Trp depletion, on the other hand, can be decreased by 1-methyl-DL-tryptophan (1-MT). The D isoform acts as a Trp-mimetic at mTOR (Moon et al., 2015).
In view of the fact that the role of IDO is already very complex in physiological states, and given that IDO expression is altered under the circumstances discussed above, the role of IDO in modulating the course of immune-mediated disease is of great interest to many specialties.
Autoimmunity and Neurodegenerative Disorders
Indoleamine-2,3-dioxygenase has been identified as a driving factor in certain human diseases in which the equilibrium between immune response and self-tolerance is disrupted, such as in some autoimmune diseases. For example, altered IDO levels have been found in patients suffering from Type 1 diabetes, with IDO1 being physiologically highly expressed in pancreatic islets β-cells of healthy tissue, but absent in the remainder (Anquetil et al., 2018). Also, in Type 2 diabetes, IDO levels are excessive (Baban et al., 2013). Elevated IDO levels have also been observed in autoimmune neurodegenerative conditions such as multiple sclerosis (MS), in neuropsychiatric diseases such as depression, and in inflammatory bowel disorders such as Crohn’s disease and systemic sclerosis. This may indicate a possible role in the pathogenesis of these conditions (Pallotta et al., 2011).
Organ Transplantation
Autoimmunity or tolerance in a broader sense is also a key factor following organ transplantations. In acute liver transplant rejection, the severity of acute organ rejection amounts to IDO-positive cells in the portal area, where IDO positivity in liver cells was associated with worse host tolerance of a transplanted organ (Sun et al., 2012).
On the contrary, high levels of Trp catabolism were associated with better long-term survival in kidney transplant recipients (Brandacher et al., 2007) or small bowel transplantations. This has also been investigated when IDO activity was externally induced in the recipients by pharmacological measures such as transfusion of IDO-positive DC to hosts of small bowel transplants (Xie et al., 2015). IDO might therefore be a potent target for further improvements in the long-term success of transplantations, once its role in autoimmune balance is better understood.
Glioblastoma
Currently, research on IDO is most advanced in cancer cells, which quite commonly express IDO (Théate et al., 2015). Glioblastoma and its surrounding cells do not normally express IDO; however, this might contribute to the immunocompromised microenvironment of malignant gliomas (Théate et al., 2015).
Aging seems to further decrease IDO expression, making glioblastoma patients at an advanced age less likely to respond well to immunotherapy due to IDO’s immunosuppressive effect (Ladomersky et al., 2018). However, treatment with the pro-inflammatory factor IFN-y can induce IDO in such cancer cells (Zhai et al., 2017).
Promoting IDO is a promising method for targeting glioblastoma, which is highly resistant to most of the commonly used cancer treatments and often requires a combination of surgical resection, chemotherapy, radiotherapy, and/or immunotherapy (Minniti et al., 2009). Directly targeting IDO for therapeutic use is promising, but has mostly been used for cancer treatment so far. Immunotherapy can target the Trp metabolism. In Trp-depleted cancer cells, protein synthesis and thereby proliferation potential is decreased through the activation of an intracellular stress response via GCN-2. CD8-T-cell survival is promoted through GCN-2 activity in Trp-depleted states (Rashidi et al., 2020). However, in vivo Trp levels show very low fluctuations in the brain by an autoregulatory mechanism (Adam et al., 2018). Also, some evidence indicates negative effects of low Trp levels changing HLA-1-molecule presentation of melanoma cells rendering them immunity against the immune system of the host (Bartok et al., 2021). The role of IDO in NAD generation in glioma cells has also been studied extensively, and it is supposed to be highly involved in the development of resistance to therapy (Rosell et al., 2011). Additionally, changes in AhR and AhR target gene levels have been linked to increased IDO1 and TDO2 levels in glioblastoma cells pointing to the fact that increased IDO-activity might promote AhR-mediated changes in glioma cells that enable immune escape and malignant potential (Opitz et al., 2011; Sadik et al., 2020). AhR plays an important role in clonogenic survival (Opitz et al., 2011; Sadik et al., 2020), motility (Opitz et al., 2011; Sadik et al., 2020), promoting pro-oncogenic cytokine production such as TGF-β (Gramatzki et al., 2009), immunosuppression by its effects on T-cells (Ehrlich et al., 2018; Prasad Singh et al., 2020), DCs (Goudot et al., 2017), macrophages (Goudot et al., 2017), natural killer cells (Shin et al., 2013), B-cells (Piper et al., 2019), or glioblastoma-specific tumor-associated macrophages (Takenaka et al., 2019). Finally, AhR and AhR genes induce molecular immune checkpoints such as PD-L1 (Liu et al., 2018).
The mechanisms by which IDO enhancement may help fight glioblastoma cells are still not clear, and agents acting solely on IDO without simultaneously targeting other immune checkpoints such as PD-L1 are not effective (Ladomersky et al., 2018). Whether or not the higher efficacy when IDO-enhancement and PD-L1 blockade are applied jointly might be based on IDOs promoting effect on PD-L1 expression remains unclear. In patients at an advanced age, combining different therapeutic approaches including IDO is less effective than in younger glioblastoma patients (Bulloch et al., 2008). More studies are needed to further develop treatment plans, especially for highly malignant, immune-escaping, and therapy-resistant cancer types such as glioblastoma.
Indoleamine-2,3-Dioxygenase as a Predictive Biomarker
Aside from the therapeutic potential of IDO and its metabolites, it may be a promising biomarker with predictive value in cancer. Predictive biomarkers have a substantial significance because they may allow more accurate expectations for the treatment teams and the patients. These should aid in the selection of the best possible treatment regimen.
Indoleamine-2,3-dioxygenase activity has recently been analyzed with regard to progression of lung cancer. IDO2 was found to have prognostic and predictive value together with PD-L1-expression for NSCLC (Mandarano et al., 2020).
Indoleamine-2,3-dioxygenase 1 levels could be of interest for perioperative decision-making, with some studies suggesting its usefulness in deciding on perioperative immunotherapy directly targeting certain immune checkpoints (Takada et al., 2020). Analysis of IDO level was even effective for decision-making on usefulness of perioperative chemotherapy in early-stage adenocarcinoma of the lung (Takada et al., 2020). Thus IDO provides both therapeutic and diagnostic value in the field of oncology.
Infections
Infections also cause an inflammatory condition that promotes the growth of cancer cells over time. Infections can be chronic or acute, and the Kyn pathway is involved in the development of both. Immune-mediated pathways are inadequate in combating an infective agent in persistent infections. An inflammatory condition is maintained or improperly prolonged.
The role of IDO in infection is to suppress the replication of intracellular pathogens and to create an immune balance by regulating T-cell differentiation (Mbongue et al., 2015). In chronic infections such as hepatitis C, inflammatory mediators such as IFN-β or IFN-y induce IDO expression (Schulz et al., 2015; Yoshio et al., 2016). Hepatitis B replication, on the other hand, can be suppressed by IFN-y via Trp depletion (through activation of IDO), helping infected cells mount anti-viral responses (Mao et al., 2011). Some pathogens use this immunoregulatory response to their advantage and inhibit IDO activity allowing an escape of immunological checkpoints and persist and mature in host cells (Planes and Bahraoui, 2013). Epstein–Barr virus is such a virus that escapes the immune response by interacting with IDO (Liu et al., 2014).
Another more commonly seen problem, especially after surgery, are surgical site infections. Lately, effects of Kyn metabolites have been identified as key drivers in dysregulation of wound healing (Kim et al., 2021), but more profound analyses are needed.
Influenza
Apart from the important role of IDO in chronic infective conditions, the Kyn pathway also contributes to an understanding of acute infections. Lung inflammation induced by influenza might be highly dependent on IDO activity. IDO with its immunosuppressive effect might predispose infected lung tissue to bacterial infection. By inhibiting IDO activity, heterosubtypic T memory cells are favorably activated. These cells then provide cross-protection immunity against influenza virus (Sage et al., 2014).
In view of these findings, the response to yearly influenza vaccines could probably be improved by inhibiting IDO. The promoted activation of T memory cells by IDO inhibition may improve individual immunity against different influenza subtypes.
On the other hand, enhancing IDO could give the immune response support to fight infections with parainfluenza virus. IDO depletes parainfluenza virus of L-Trp and thereby inhibits its metabolism (not by other metabolites of the Kyn pathway, though). Interestingly, 5-hydroxy-tryptophan, a Trp isoform favoring the serotonin pathway, protects parainfluenza virus from this IDO-mediated block in metabolism (Rabbani and Barik, 2017).
Sepsis
Sepsis is a devastating acute infection that creates a state of dysregulation in the immune system in response to an infection with systemic involvement (Raeven et al., 2018). It is difficult to identify and even more difficult to treat once septic shock has occurred. Even for highly competent interdisciplinary teams of treating physicians, it sometimes appears to be a “one-way track” leading to septic shock. It is therefore critical to better understand the mechanics of this acute systemic infection in order to identify therapy targets.
So far, little is known about the roles IDO and the Kyn pathway play in the septic shock spiral. However, increased activity of IDO has been registered lately in patients with severe sepsis and septic shock (Tattevin et al., 2010). Further studies are needed to improve outcome of acute infections – especially sepsis – and to provide evidence-based care.
Surgical Trauma
Indoleamine-2,3-dioxygenase levels are typically elevated during inflammatory reactions, and this makes it a useful biomarker for detecting immune dysregulation. Surgical trauma is a well-known cause of acute stress, as is an acute infection. Because of this, IDO may prove useful as a biomarker to track the recovery process after surgical trauma.
Surgical stress induces endocrine changes as well as cellular tissue damage. Stress hormones such as cortisol, ACTH, and growth hormone regulate the metabolism of glucose, as well as anabolic and catabolic processes, and generally affect homeostasis, with immune cells mitigating an inflammatory response (Desborough, 2000).
Damage to tissue leads to the activation of immune cells that release damage-associated molecular patterns (DAMPs). DAMPs then activate monocytes and neutrophils, which produce pro-inflammatory cytokines. Such cytokines include IL-1, TNF-α, GCS, or TGF-β among others. DAMPs then activate IL-6, which enhances acute-phase proteins such as C-reactive protein (CRP) and macroglobulin (Desborough, 2000) and decreases important mediators of oxidative stress tolerance such as zinc and iron (Sheeran and Hall, 1997).
Pro-inflammatory conditions result as a consequence of this. Patients undergoing cardiac surgery with extracorporeal circuits, which increases the risk of sepsis and infection, are more likely to have additional tissue damage and a persistent severe inflammatory response syndrome (SIRS). More immune cells will be activated as a result of infections with pathogen-associated molecular patterns (PAMPs), which will lead to a recurrence of SIRS and, ultimately, death (Lord et al., 2014).
Not every patient who has general surgery develops SIRS. However, identifying high-risk individuals is still challenging, and even early identification of surgical trauma-induced SIRS that is already “present” is problematic. More research is needed to identify the typical patients which may be at risk.
Indoleamine-2,3-Dioxygenase as a Target in Infective Diseases Related to Oxidative Stress
Indoleamine-2,3-dioxygenase involvement in the perioperative phase and for the treatment of sepsis is still in its early stages. The pro-inflammatory cytokines involved are the same as those found in other immune-mediated diseases where IDO has been implicated, such as acute infections. It would be revolutionary to specifically target immune-modulating enzymes like IDO without falling into a vicious loop of SIRS after surgery. Aside from the use of antibiotics to limit microbial development, no therapies have been found to have a substantial postoperative effect on SIRS/sepsis regulation (Lord et al., 2014).
However, a recent study involving pediatric patients undergoing cardiopulmonary bypass (CPB) found higher IL-6, CRP, and IDO levels in children who developed SIRS following CPB. The plasma IDO level was the best diagnostic forecast (Wang et al., 2018). The authors even suggested that IDO is more sensitive than IL-6 or CRP to predict SIRS upon surgical correction of congenital heart diseases. Predicting such negative outcomes could be a crucial advance in optimal patient management prior to surgery. It would also have a significant impact on treatment plans in sepsis and post-surgical inflammation (SIRS), where a change is desperately needed.
Indoleamine-2,3-dioxygenase can also be blocked by CD11b + l cells in peritonitis and postoperative sepsis. This is a protective benefit in hosts during sepsis, and was even found to improve mortality (Hoshi et al., 2014). Decreasing IDO activity by applying suppressive cytokines such as GM-CSF decreases catabolic Kyn metabolites in sepsis. This could improve the antibacterial defense (Schefold et al., 2010). Finally, the administration of melatonin, an IDO inhibitor, can directly inhibit the proinflammatory Kyn pathway (Moreno et al., 2018).
Other benefits of inhibiting IDO include positive effects on oxygen- and nitrogen-based toxic metabolites, stimulation of antioxidative mechanisms, limitation of free radical generation via more efficient activity of the electron transport chain, increased formation of ATP, and preservation of mitochondrial integrity. This in turn is key for the maintenance of cell functions and survival (Leon et al., 2004). Endothelial cells cultured in laboratory-induced septic environments were found to have altered NF-κB activity, cytokine profiles, mitochondrial membrane potential, and metabolic changes favoring protection against oxidative mitochondrial stress when exposed to structurally similar compounds to IDO (Lowes et al., 2011).
Indoleamine-2,3-Dioxygenase as a Target in Anoxic States Related to Oxidative Stress
Oxidative stress and mitochondrial damage can be found in many other conditions such as Alzheimer’s, Parkinson’s, epileptogenic disease, or reperfusion injury after ischemia (Leon et al., 2004). The latter relates to the observation of adverse consequences of reperfusing organs that were exposed to acute ischemia, either as a pathologic event such as acute myocardial infarction or as a therapeutic strategy such as producing hypothermia to protect brain tissue following unconscious cardiac arrest. Induced hypothermia raised the chance of developing a serious infection/sepsis (Schefold et al., 2016). This was directly related to IDO overexpression and Trp suppression caused by low body temperature. Given these latest findings, such commonly used therapeutic techniques may need to be reevaluated, since they may even impair patient outcomes.
Other studies assessing organ damage after induced anoxia such as in renal reperfusion after transplantations have also found an increase in IDO induction. Both hypoxia and reoxygenation of renal tissue led to significant higher IDO levels (Eleftheriadis et al., 2021). Damage caused by reperfusion is to a vast extent due to inflammatory responses (Eleftheriadis et al., 2021). These inflammatory changes might possibly lead to advanced systemic inflammation just an in sepsis or surgical trauma-induced stress. A promising new approach to the prevention of reperfusion injury is the application of extracellular vesicles (EVs) from mesenchymal cells such as DCs. These EVs can also be produced during sheer-stress on the vasculature as part of short periods of ischemia. In so-called remote ischemic preconditioning, ischemia is induced upon a distal part of the body as, for example, the arm by alternatingly inflating and deflating cuffs (Gassanov et al., 2014; Figure 4). The EVs can then be extracted from the distal area and re-introduced into the patient. They express micro-ribonucleic acid (RNA) and seem to have anti-inflammatory, anti-oxidative, and angiogenic properties. Introduction of EVs helped mitigate damage from reperfusion (Chen et al., 2021). EVs have been utilized as a therapeutic approach in immune-mediated illness, in addition to their application in intentionally induced hypoxia to organs as in transplantation or cardiac surgery. In mice, EVs generated from IDO-positive DCs successfully delayed the onset of collagen-induced arthritis (Bianco et al., 2009). IDO expression in dendritic-cell-derived EVs that were driven into host cells is considered to have reduced this impact.
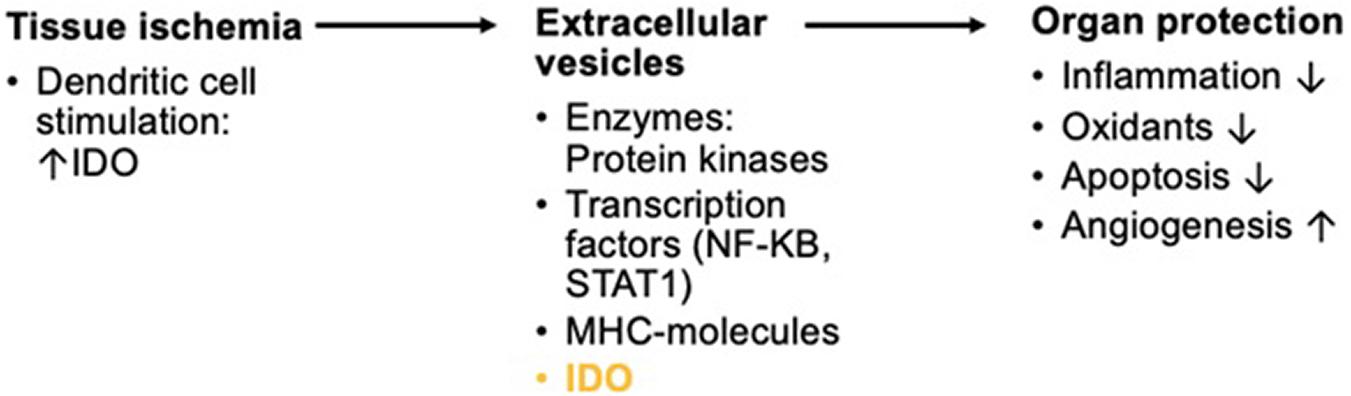
Figure 4. Short overview of the physiologic background in remote ischemic preconditioning for reperfusion of anoxic organs. Ischemia triggers the activation of DCs. Inflammation by tissue ischemia induces IDO in DCs. The production of exosomes with enzymatic composites, transcription factors, major histocompatibility complex (MHC-) molecules, and IDO among many other compounds is triggered. These EVs show protective effects on organs in reperfusion.
The application of such findings in vivo remains a distant dream. However, deriving EVs from IDO-expressing DCs may be a therapeutic target, particularly in an immune-compromised state characterized by such variable facets as SIRS (Raeven et al., 2018). EVs may eventually be used as biomarkers in sepsis, as they contain critical nucleic acids involved in the suppression of inflammatory responses (Raeven et al., 2018). An in-depth examination of the nucleic acid profiles of EVs, with a focus on immune-modulatory enzymes such as IDO, could be used to assess the inflammatory state.
Knowing that oxidative stress plays an important role in surgical trauma response, these findings point to IDO’s potentially therapeutic and prognostic function in addressing the surgical stress cascade. Apart from sepsis, whether the chemicals described above offer a treatment alternative for the oxidative stress-induced inflammatory response by surgical trauma remains unknown, since the complicated role of IDO is currently being investigated. However, surgical trauma response shares several pathways with SIRS, sepsis, and anoxic states. The final common pathway of a vicious circle ending in cell death by necrosis or apoptosis, in which IDO plays an important role, could potentially be altered by targeting IDO early enough. By altering the oxidative damage by surgical trauma, surgical outcome could be significantly improved.
Future Perspectives
In patients undergoing surgery, all sub-specialties of a multidisciplinary team must be involved in order to optimize patient outcomes. Anesthesia has a vital role in decreasing patient stress in the pre- and perioperative settings. On anesthesia induction, strong opioids such as morphine or high-dose fentanyl suppress the postsurgical stress response. However, they are limited by serious ventilatory problems at higher doses (Hall, 1985; Abd Elrazek et al., 1999). Clonidine, a potent alpha-2 agonist, can also positively affect postsurgical sympathetic tone, reducing the stress response (Aantaa and Scheinin, 1993). Regional anesthesia, such as epidural blocking T4-S5, has a positive effect on post-surgical cortisol levels (Engquist et al., 1977). Thoracic epidurals even lower the troponin T (TnT) values in patients undergoing CPB (Loick et al., 1999). However, this effect is limited by frequently concomitant anticoagulant therapy, increasing the risk of epidural hematomas and impaired neurologic outcomes.
Indoleamine-2,3-dioxygenase 1 activation and emptying of Trp levels has been found to be stimulated via cytokine-induction by psychological stress also (Raeven et al., 2018). Thus, anesthetists play a basic role in limiting surgical stress. As there are sufficient data demonstrating increased Trp metabolism already prior to surgery, it should be possible in the near future to use IDO as a biomarker in patient-centered decision-making, providing optimal treatment in the perioperative period (Figure 5; Kiank et al., 2010).
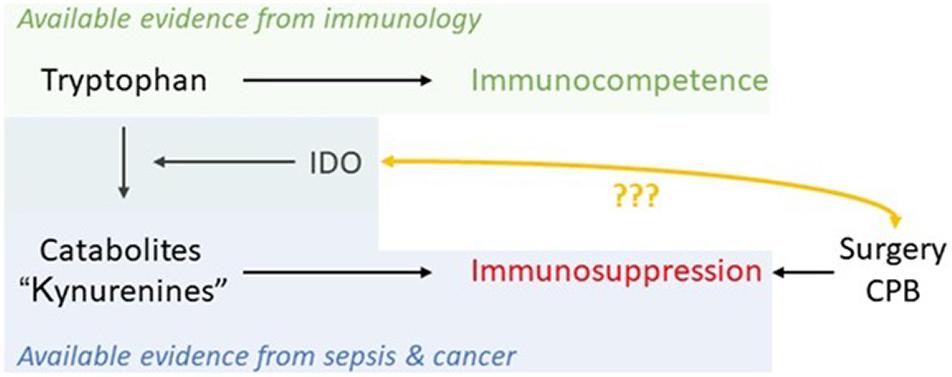
Figure 5. Schematic of available evidence from different fields and the potential of IDO as a marker in the perioperative period to monitor immunosuppression caused by major trauma/physiological derangement such as cardiopulmonary bypass (CPB) in cardiac surgery.
A number of factors, including the fact that most research is conducted on mouse models, complicate the use of IDO as a biomarker and therapy target at the current stage. Especially when it comes to studying myeloid cells in mice, these models might not be representative (Schmidt and Schultze, 2014), as mice seem not to express IDO in myeloid cells (Blumenthal et al., 2012). Also, measuring IDO is laborious. Using Trp levels as a surrogate for IDO activity is not helpful, since Trp can be metabolized either by IDO1, IDO2, or TDO in the Kyn direction or via the serotonin pathway. This limits our ability to draw direct conclusions about IDO activity. Patients with sepsis or SIRS in clinical practice usually show significant variability in factors such as age, presence of underlying or secondary illness, immune system status, and infection severity. There are several pathophysiological processes that can result from an infection, and the name “sepsis” encompasses them all. These characteristics of heterogeneity make it more difficult to detect sepsis quickly and to select the best therapy for each patient. To make therapy decisions and prognosis decisions in infections, a metabolomic approach is preferable. Despite the fact that sepsis metabolism has been studied in the past, a multivariate score utilizing serum amino acid profiling as a sepsis diagnostic biomarker is yet to be developed. Despite several investigations into metabolomics in sepsis, only a small number have looked at serum amino acids as potential biomarkers. A recent study by Ahn et al. (2021) intended to design and verify a sepsis biomarker as a multivariate index based on amino acid profiling. Patients with SIRS were also included, a disease that can be difficult to identify from sepsis when pre-existing sepsis indicators are used (Ahn et al., 2021). The study might help clinicians and researchers better understand how metabolomics can be used in clinical medicine and how multivariate index analysis can be used to generate multivariate biomarkers that function well. More research, particularly with human probes, is urgently required for further understanding IDO in the process as a perioperative marker. Our group has initiated a biobank-derived study on cardiac surgical patients to analyze the impact of IDO as a perioperative marker of the immune system.
Conclusion
Indoleamine-2,3-dioxygenase’s immuno-modulatory function alters the physiologically balanced immune state and has a role in disease etiology as well as perioperative settings after surgery. The role of IDO in autoimmune diseases, malignancies including glioblastoma, and organ transplantation has been widely investigated. But with severe systemic infections like sepsis, it is still not clear. IDO might offer validity as a prognostic biomarker and therapeutic target for immune-mediated illnesses. Finally, IDO offers potential as a pre-operative biomarker for all disciplines involved in the decision-making process and care of patients undergoing surgery.
Author Contributions
CB, PH, MM, TC, and ML searched the literature and wrote the manuscript. All authors contributed to the article and approved the submitted version.
Conflict of Interest
The authors declare that the research was conducted in the absence of any commercial or financial relationships that could be construed as a potential conflict of interest.
Publisher’s Note
All claims expressed in this article are solely those of the authors and do not necessarily represent those of their affiliated organizations, or those of the publisher, the editors and the reviewers. Any product that may be evaluated in this article, or claim that may be made by its manufacturer, is not guaranteed or endorsed by the publisher.
Abbreviations
AhR, aryl hydrocarbon receptor; BLIMP-1, B lymphocyte-induced maturation protein-1; CPB, cardiopulmonary bypass; CD, cluster of differentiation; COX, cyclooxygenase; CRP, C-reactive protein; CTLA-4, cytotoxic T-lymphocyte -associated protein; DAMP, damage-associated molecular pattern; DC, dendritic cell; EV, extracellular vesicle; GCN2, general control non-depressible 2; IDO, indoleamine-2,3-deoxygenase; IFN, interferon; IL, interleukin; ITIM, immune tyrosine-based inhibitory motif; Kyn, kynurenine; LAG3, lymphocyte-activation gene 3; MHC, major histocompatibility complex; mTOR, mechanistic target of rapamycin (previously: mammalian target of rapamycin); MS, multiple sclerosis; NAD, nicotinamide adenine dinucleotide; NF- κ B, nuclear factor kappa-light-chain-enhancer of activated B cells; PAMPs, pathogen-associated molecular patterns; PD-L1, programmed death-ligand 1; PD-1, programmed cell death protein 1; PGE2, prostaglandin E2; PRR, pattern recognition receptor; RNA, ribonucleic acid; SHP, Src homology 2 domain phosphatase; SIRS, severe inflammatory response syndrome; STAT, signal transducer and activator of transcription protein; TDO, tryptophan-2,3-dioxygenase; Teff, effector T-cell; TGF- β, transforming growth factor beta; Th, T helper cell; TNF- α, tumor necrosis factor alpha; TnT, troponin T; Tregs, T regulatory cells; Trp, tryptophan.
References
Aantaa, R., and Scheinin, M. (1993). Alpha 2-adrenergic agents in anaesthesia. Acta Anaesthesiol. Scand. 37, 433–448.
Abbas, A. K. L. A., and Pillai, S. (2019). Basic Immunology: Functions and Disorders of the Immune System, 6th Edn. Philadelphia, PA: Elsevier.
Abd Elrazek, E., Scott, N. B., and Vohra, A. (1999). An epidural scoring scale for arm movements (ESSAM) in patients receiving high thoracic epidural analgesia for coronary artery bypass grafting. Anaesthesia 54, 1104–1109. doi: 10.1046/j.1365-2044.1999.01031.x
Abuelezz, S. A., Hendawy, N., and Magdy, Y. (2017). Targeting oxidative stress, cytokines and serotonin interactions via indoleamine 2, 3 dioxygenase by Coenzyme Q10: role in suppressing depressive like behavior in rats. J. Neuroimmune Pharmacol. 12, 277–291. doi: 10.1007/s11481-016-9712-7
Adam, I., Dewi, D. L., Mooiweer, J., Sadik, A., Mohapatra, S. R., Berdel, B., et al. (2018). Upregulation of tryptophanyl-tRNA synthethase adapts human cancer cells to nutritional stress caused by tryptophan degradation. Oncoimmunology 7:e1486353. doi: 10.1080/2162402X.2018.1486353
Agudelo, L. Z., Femenía, T., Orhan, F., Porsmyr-Palmertz, M., Goiny, M., Martinez-Redondo, V., et al. (2014). Skeletal muscle PGC-1α1 modulates kynurenine metabolism and mediates resilience to stress-induced depression. Cell 159, 33–45. doi: 10.1016/j.cell.2014.07.051
Ahn, S., Lee, S. H., Chung, K. S., Ku, N. S., Hyun, Y. M., Chun, S., et al. (2021). Development and validation of a novel sepsis biomarker based on amino acid profiling. Clin. Nutr. 40, 3668–3676. doi: 10.1016/j.clnu.2021.05.008
Albini, E., Rosini, V., Gargaro, M., Mondanelli, G., Belladonna, M. L., Pallotta, M. T., et al. (2017). Distinct roles of immunoreceptor tyrosine-based motifs in immunosuppressive indoleamine 2,3-dioxygenase 1. J. Cell. Mol. Med. 21, 165–176. doi: 10.1111/jcmm.12954
Anquetil, F., Mondanelli, G., Gonzalez, N., Rodriguez Calvo, T., Zapardiel Gonzalo, J., Krogvold, L., et al. (2018). Loss of IDO1 expression from human pancreatic β-cells precedes their destruction during the development of type 1 diabetes. Diabetes 67, 1858–1866. doi: 10.2337/db17-1281
Baban, B., Liu, J. Y., and Mozaffari, M. S. (2013). Endoplasmic reticulum stress response and inflammatory cytokines in type 2 diabetic nephropathy: role of indoleamine 2,3-dioxygenase and programmed death-1. Exp. Mol. Pathol. 94, 343–351. doi: 10.1016/j.yexmp.2012.11.004
Barnes, N. A., Stephenson, S. J., Tooze, R. M., and Doody, G. M. (2009). Amino acid deprivation links BLIMP-1 to the immunomodulatory enzyme indoleamine 2,3-dioxygenase. J. Immunol. 183, 5768–5777. doi: 10.4049/jimmunol.0803480
Bartok, O., Pataskar, A., Nagel, R., Laos, M., Goldfarb, E., Hayoun, D., et al. (2021). Anti-tumour immunity induces aberrant peptide presentation in melanoma. Nature 590, 332–337.
Bianco, N. R., Kim, S. H., Ruffner, M. A., and Robbins, P. D. (2009). Therapeutic effect of exosomes from indoleamine 2,3-dioxygenase-positive dendritic cells in collagen-induced arthritis and delayed-type hypersensitivity disease models. Arthritis Rheum. 60, 380–389. doi: 10.1002/art.24229
Blumenthal, A., Nagalingam, G., Huch, J. H., Walker, L., Guillemin, G. J., Smythe, G. A., et al. (2012). M. tuberculosis induces potent activation of IDO-1, but this is not essential for the immunological control of infection. PLoS One 7:e37314. doi: 10.1371/journal.pone.0037314
Brandacher, G., Cakar, F., Winkler, C., Schneeberger, S., Obrist, P., Bösmüller, C., et al. (2007). Non-invasive monitoring of kidney allograft rejection through IDO metabolism evaluation. Kidney Int. 71, 60–67. doi: 10.1038/sj.ki.5002023
Bulloch, K., Miller, M. M., Gal-Toth, J., Milner, T. A., Gottfried-Blackmore, A., Waters, E. M., et al. (2008). CD11c/EYFP transgene illuminates a discrete network of dendritic cells within the embryonic, neonatal, adult, and injured mouse brain. J. Comp. Neurol. 508, 687–710. doi: 10.1002/cne.21668
Busbee, P. B., Rouse, M., Nagarkatti, M., and Nagarkatti, P. S. (2013). Use of natural AhR ligands as potential therapeutic modalities against inflammatory disorders. Nutr. Rev. 71, 353–369. doi: 10.1111/nure.12024
Chen, A. Q., Gao, X. F., Wang, Z. M., Wang, F., Luo, S., Gu, Y., et al. (2021). Therapeutic exosomes in prognosis and developments of coronary artery disease. Front. Cardiovasc. Med. 8:691548. doi: 10.3389/fcvm.2021.691548
Desborough, J. P. (2000). The stress response to trauma and surgery. Br. J. Anaesth. 85, 109–117. doi: 10.1093/bja/85.1.109
Ehrlich, A. K., Pennington, J. M., Bisson, W. H., Kolluri, S. K., and Kerkvliet, N. I. (2018). FICZ, and other high affinity AhR ligands dose-dependently determine the fate of CD4+ T cell differentiation. Toxicol. Sci. 161, 310–320. doi: 10.1093/toxsci/kfx215
Eleftheriadis, T., Pissas, G., Golfinopoulos, S., Liakopoulos, V., and Stefanidis, I. (2021). Role of indoleamine 2,3-dioxygenase in ischemia-reperfusion injury of renal tubular epithelial cells. Mol. Med. Rep. 23:472.
Engquist, A., Brandt, M. R., Fernandes, A., and Kehlet, H. (1977). The blocking effect of epidural analgesia on the adrenocortical and hyperglycemic responses to surgery. Acta Anaesthesiol. Scand. 21, 330–335. doi: 10.1111/j.1399-6576.1977.tb01227.x
Fontenot, J. D., Gavin, M. A., and Rudensky, A. Y. (2003). Foxp3 programs the development and function of CD4+CD25+ regulatory T cells. Nat. Immunol. 4, 330–336. doi: 10.1038/ni904
Gassanov, N., Nia, A. M., Caglayan, E., and Er, F. (2014). Remote ischemic preconditioning and renoprotection: From myth to a novel therapeutic option? J. Am. Soc. Nephrol. 25, 216–224. doi: 10.1681/ASN.2013070708
Goudot, C., Coillard, A., Villani, A. C., Gueguen, P., Cros, A., Sarkizova, S., et al. (2017). Aryl hydrocarbon receptor controls monocyte differentiation into dendritic cells versus macrophages. Immunity 47, 582–596.e6. doi: 10.1016/j.immuni.2017.08.016
Gramatzki, D., Pantazis, G., Schittenhelm, J., Tabatabai, G., Köhle, C., Wick, W., et al. (2009). Aryl hydrocarbon receptor inhibition downregulates the TGF-beta/Smad pathway in human glioblastoma cells. Oncogene 28, 2593–2605. doi: 10.1038/onc.2009.104
Hall, G. M. (1985). The anaesthetic modification of the endocrine and metabolic response to surgery. Ann. R. Coll. Surg. Engl. 67, 25–29.
Hornyák, L., Dobos, N., Koncz, G., Karányi, Z., Páll, D., Szabó, Z., et al. (2018). The role of indoleamine-2,3-dioxygenase in cancer development, diagnostics, and therapy. Front. Immunol. 9:151. doi: 10.3389/fimmu.2018.00151
Hoshi, M., Osawa, Y., Ito, H., Ohtaki, H., Ando, T., Takamatsu, M., et al. (2014). Blockade of indoleamine 2,3-dioxygenase reduces mortality from peritonitis and sepsis in mice by regulating functions of CD11b+ peritoneal cells. Infect. Immun. 82, 4487–4495. doi: 10.1128/IAI.02113-14
Jeong, Y. I., Kim, S. W., Jung, I. D., Lee, J. S., Chang, J. H., Lee, C. M., et al. (2009). Curcumin suppresses the induction of indoleamine 2,3-dioxygenase by blocking the Janus-activated kinase-protein kinase Cdelta-STAT1 signaling pathway in interferon-gamma-stimulated murine dendritic cells. J. Biol. Chem. 284, 3700–3708. doi: 10.1074/jbc.M807328200
Kak, G., Raza, M., and Tiwari, B. K. (2018). Interferon-gamma (IFN-gamma): exploring its implications in infectious diseases. Biomol. Concepts 9, 64–79. doi: 10.1515/bmc-2018-0007
Kiank, C., Zeden, J. P., Drude, S., Domanska, G., Fusch, G., Otten, W., et al. (2010). Psychological stress-induced, IDO1-dependent tryptophan catabolism: implications on immunosuppression in mice and humans. PLoS One 5:e11825. doi: 10.1371/journal.pone.0011825
Kim, J., Yang, G. S., Lyon, D., Kelly, D. L., and Stechmiller, J. (2021). Metabolomics: impact of comorbidities and inflammation on sickness behaviors for individuals with chronic wounds. Adv. Wound Care 10, 357–369. doi: 10.1089/wound.2020.1215
Kirmeier, E., Eriksson, L. I., Lewald, H., Jonsson Fagerlund, M., Hoeft, A., Hollmann, M., et al. (2019). POPULAR Contributors. Post-anaesthesia pulmonary complications after use of muscle relaxants (POPULAR): a multicentre, prospective observational study. Lancet Respir. Med. 7, 129–140. doi: 10.1016/S2213-2600(18)30294-7
Ladomersky, E., Zhai, L., Lenzen, A., Lauing, K. L., Qian, J., Scholtens, D. M., et al. (2018). IDO1 inhibition synergizes with radiation and PD-1 blockade to durably increase survival against advanced glioblastoma. Clin. Cancer Res. 24, 2559–2573. doi: 10.1158/1078-0432.CCR-17-3573
Leon, J., Acuna-Castroviejo, D., Sainz, R. M., Mayo, J. C., Tan, D. X., and Reiter, R. J. (2004). Melatonin and mitochondrial function. Life Sci. 75, 765–790.
Litzenburger, U. M., Opitz, C. A., Sahm, F., Rauschenbach, K. J., Trump, S., Winter, M., et al. (2014). Constitutive IDO expression in human cancer is sustained by an autocrine signaling loop involving IL-6, STAT3 and the AHR. Oncotarget 5, 1038–1051. doi: 10.18632/oncotarget.1637
Liu, W. L., Lin, Y. H., Xiao, H., Xing, S., Chen, H., Chi, P. D., et al. (2014). Epstein-Barr virus infection induces indoleamine 2,3-dioxygenase expression in human monocyte-derived macrophages through p38/mitogen-activated protein kinase and NF-κB pathways: impairment in T cell functions. J. Virol. 88, 6660–6671. doi: 10.1128/JVI.03678-13
Liu, Y., Liang, X., Dong, W., Fang, Y., Lv, J., Zhang, T., et al. (2018). Tumor-repopulating cells induce PD-1 expression in CD8+ T cells by transferring kynurenine and AhR activation. Cancer Cell 33, 480–494.e7. doi: 10.1016/j.ccell.2018.02.005
Loick, H. M., Schmidt, C., Van Aken, H., Junker, R., Erren, M., Berendes, E., et al. (1999). High thoracic epidural anesthesia, but not clonidine, attenuates the perioperative stress response via sympatholysis and reduces the release of troponin T in patients undergoing coronary artery bypass grafting. Anesth. Analg. 88, 701–709. doi: 10.1213/00000539-199904000-00001
Lord, J. M., Midwinter, M. J., Chen, Y. F., Belli, A., Brohi, K., Kovacs, E. J., et al. (2014). The systemic immune response to trauma: an overview of pathophysiology and treatment. Lancet 384, 1455–1465. doi: 10.1016/s0140-6736(14)60687-5
Lowes, D. A., Almawash, A. M., Webster, N. R., Reid, V. L., and Galley, H. F. (2011). Melatonin and structurally similar compounds have differing effects on inflammation and mitochondrial function in endothelial cells under conditions mimicking sepsis. Br. J. Anaesth. 107, 193–201. doi: 10.1093/bja/aer149
Mandarano, M., Bellezza, G., Belladonna, M. L., Vannucci, J., Gili, A., Ferri, I., et al. (2020). Indoleamine 2,3-Dioxygenase 2 immunohistochemical expression in resected human non-small cell lung cancer: a potential new prognostic tool. Front. Immunol. 11:839. doi: 10.3389/fimmu.2020.00839
Mantel, P. Y., Ouaked, N., Rückert, B., Karagiannidis, C., Welz, R., Blaser, K., et al. (2006). Molecular mechanisms underlying FOXP3 induction in human T cells. J. Immunol. 176, 3593–3602. doi: 10.4049/jimmunol.176.6.3593
Mao, R., Zhang, J., Jiang, D., Cai, D., Levy, J. M., Cuconati, A., et al. (2011). Indoleamine 2,3-dioxygenase mediates the antiviral effect of gamma interferon against hepatitis B virus in human hepatocyte-derived cells. J. Virol. 85, 1048–1057. doi: 10.1128/JVI.01998-10
Marttila, S., Jylhava, J., Eklund, C., Hervonen, A., Jylha, M., and Hurme, M. (2011). Aging-associated increase in indoleamine 2,3-dioxygenase (IDO) activity appears to be unrelated to the transcription of the IDO1 or IDO2 genes in peripheral blood mononuclear cells. Immun. Ageing 8:9.
Mbongue, J. C., Nicholas, D. A., Torrez, T. W., Kim, N. S., Firek, A. F., and Langridge, W. H. (2015). The role of indoleamine 2, 3-dioxygenase in immune suppression and autoimmunity. Vaccines 3, 703–729. doi: 10.3390/vaccines3030703
Meireson, A., Devos, M., and Brochez, L. I. D. O. (2020). Expression in Cancer: Different Compartment, Different Functionality? Front. Immunol. 11:531491. doi: 10.3389/fimmu.2020.531491
Minniti, G., Muni, R., Lanzetta, G., Marchetti, P., and Enrici, R. M. (2009). Chemotherapy for glioblastoma: current treatment and future perspectives for cytotoxic and targeted agents. Anticancer Res. 29, 5171–5184.
Moon, Y. W., Hajjar, J., Hwu, P., and Naing, A. (2015). Targeting the indoleamine 2,3-dioxygenase pathway in cancer. J. Immunother. Cancer 3:51.
Moreno, A. C. R., Porchia, B. F. M. M., Pagni, R. L., Souza, P. D. C., Pegoraro, R., Rodrigues, K. B., et al. (2018). The Combined Use of Melatonin and an Indoleamine 2,3-Dioxygenase-1 Inhibitor Enhances Vaccine-Induced Protective Cellular Immunity to HPV16-Associated Tumors. Front. Immunol. 9:1914. doi: 10.3389/fimmu.2018.01914
Munn, D. H., and Mellor, A. L. (2013). Indoleamine 2,3 dioxygenase and metabolic control of immune responses. Trends Immunol. 34, 137–143. doi: 10.1016/j.it.2012.10.001
Nazzal, D., Gradolatto, A., Truffault, F., Bismuth, J., and Berrih-Aknin, S. (2014). Human thymus medullary epithelial cells promote regulatory T-cell generation by stimulating interleukin-2 production via ICOS ligand. Cell Death Dis. 5:e1420. doi: 10.1038/cddis.2014.377
Opitz, C. A., Litzenburger, U. M., Sahm, F., Ott, M., Tritschler, I., Trump, S., et al. (2011). An endogenous tumour-promoting ligand of the human aryl hydrocarbon receptor. Nature 478, 197–203. doi: 10.1038/nature10491
Pallotta, M. T., Orabona, C., Volpi, C., Vacca, C., Belladonna, M. L., Bianchi, R., et al. (2011). Indoleamine 2,3-dioxygenase is a signaling protein in long-term tolerance by dendritic cells. Nat. Immunol. 12, 870–878. doi: 10.1038/ni.2077
Pantouris, G., Serys, M., Yuasa, H. J., Ball, H. J., and Mowat, C. G. (2014). Human indoleamine 2,3-dioxygenase-2 has substrate specificity and inhibition characteristics distinct from those of indoleamine 2,3-dioxygenase-1. Amino Acids 46, 2155–2163. doi: 10.1007/s00726-014-1766-3
Piper, C. J. M., Rosser, E. C., Oleinika, K., Nistala, K., Krausgruber, T., Rendeiro, A. F., et al. (2019). Aryl Hydrocarbon Receptor Contributes to the Transcriptional Program of IL-10-Producing Regulatory B Cells. Cell Rep. 29, 1878–1892.e7. doi: 10.1016/j.celrep.2019.10.018
Planes, R., and Bahraoui, E. (2013). HIV-1 Tat protein induces the production of IDO in human monocyte derived-dendritic cells through a direct mechanism: effect on T cells proliferation. PLoS One 8:e74551. doi: 10.1371/journal.pone.0074551
Prasad Singh, N., Nagarkatti, M., and Nagarkatti, P. (2020). From suppressor T cells to regulatory T cells: how the journey that began with the discovery of the toxic effects of TCDD led to better understanding of the role of AhR in immunoregulation. Int. J. Mol. Sci. 21:7849. doi: 10.3390/ijms21217849
Prendergast, G. C., Malachowski, W. J., Mondal, A., Scherle, P., and Muller, A. J. (2018). Indoleamine 2,3-dioxygenase and its therapeutic inhibition in cancer. Int. Rev. Cell Mol. Biol. 336, 175–203. doi: 10.1016/bs.ircmb.2017.07.004
Rabbani, M. A. G., and Barik, S. (2017). 5-Hydroxytryptophan, a major product of tryptophan degradation, is essential for optimal replication of human parainfluenza virus. Virology 503, 46–51. doi: 10.1016/j.virol.2017.01.007
Raeven, P., Zipperle, J., and Drechsler, S. (2018). Extracellular vesicles as markers and mediators in sepsis. Theranostics 8, 3348–3365. doi: 10.7150/thno.23453
Rashidi, A., Miska, J., Lee-Chang, C., Kanojia, D., Panek, W. K., Lopez-Rosas, A., et al. (2020). GCN2 is essential for CD8+ T cell survival and function in murine models of malignant glioma. Cancer Immunol. Immunother. 69, 81–94. doi: 10.1007/s00262-019-02441-6
Rosell, F. I., Kuo, H. H., and Mauk, A. G. (2011). NADH oxidase activity of indoleamine 2,3-dioxygenase. J. Biol. Chem. 286, 29273–29283. doi: 10.1074/jbc.M111.262139
Sadik, A., Somarribas Patterson, L. F., Öztürk, S., Mohapatra, S. R., Panitz, V., Secker, P. F., et al. (2020). IL4I1 is a metabolic immune checkpoint that activates the AHR and promotes tumor progression. Cell 182, 1252–1270.e34. doi: 10.1016/j.cell.2020.07.038
Sage, L. K., Fox, J. M., Mellor, A. L., Tompkins, S. M., and Tripp, R. A. (2014). Indoleamine 2,3-dioxygenase (IDO) activity during the primary immune response to influenza infection modifies the memory T cell response to influenza challenge. Viral Immunol. 27, 112–123. doi: 10.1089/vim.2013.0105
Schefold, J. C., Fritschi, N., Fusch, G., Bahonjic, A., Doehner, W., von Haehling, S., et al. (2016). Influence of core body temperature on Tryptophan metabolism, kynurenines, and estimated IDO activity in critically ill patients receiving target temperature management following cardiac arrest. Resuscitation 107, 107–114. doi: 10.1016/j.resuscitation.2016.07.239
Schefold, J. C., Zeden, J. P., Pschowski, R., Hammoud, B., Fotopoulou, C., Hasper, D., et al. (2010). Treatment with granulocyte-macrophage colony-stimulating factor is associated with reduced indoleamine 2,3-dioxygenase activity and kynurenine pathway catabolites in patients with severe sepsis and septic shock. Scand. J. Infect. Dis. 42, 164–171. doi: 10.3109/00365540903405768
Schmidt, A., Oberle, N., and Krammer, P. H. (2012). Molecular mechanisms of treg-mediated T cell suppression. Front. Immunol. 3:51. doi: 10.3389/fimmu.2012.00051
Schmidt, S. V., and Schultze, J. L. (2014). New Insights into IDO Biology in Bacterial and Viral Infections. Front. Immunol. 5:384. doi: 10.3389/fimmu.2014.00384
Schulz, S., Landi, A., Garg, R., Wilson, J. A., and van Drunen Littel-van den Hurk, S. (2015). Indolamine 2,3-dioxygenase expression by monocytes and dendritic cell populations in hepatitis C patients. Clin. Exp. Immunol. 180, 484–498. doi: 10.1111/cei.12586
Sheeran, P., and Hall, G. M. (1997). Cytokines in anaesthesia. Br. J. Anaesth. 78, 201–219. doi: 10.1093/bja/78.2.201
Shin, J. H., Zhang, L., Murillo-Sauca, O., Kim, J., Kohrt, H. E., Bui, J. D., et al. (2013). Modulation of natural killer cell antitumor activity by the aryl hydrocarbon receptor. Proc. Natl. Acad. Sci. U.S.A. 110, 12391–12396. doi: 10.1073/pnas.1302856110
Sun, X., Gong, Z. J., Wang, Z. W., Li, T., Zhang, J. Y., Sun, H. C., et al. (2012). IDO-competent-DCs induced by IFN-γ attenuate acute rejection in rat liver transplantation. J. Clin. Immunol. 32, 837–847. doi: 10.1007/s10875-012-9681-4
Takada, K., Toyokawa, G., Kinoshita, F., Jogo, T., Kohashi, K., Wakasu, S., et al. (2020). Expression of PD-L1, PD-L2, and IDO1 on tumor cells and density of CD8-positive tumor-infiltrating lymphocytes in early-stage lung adenocarcinoma according to histological subtype. J. Cancer Res. Clin. Oncol. 146, 2639–2650. doi: 10.1007/s00432-020-03250-6
Takenaka, M. C., Gabriely, G., Rothhammer, V., Mascanfroni, I. D., Wheeler, M. A., Chao, C. C., et al. (2019). Control of tumor-associated macrophages and T cells in glioblastoma via AHR and CD39. Nat. Neurosci. 22, 729–740. doi: 10.1038/s41593-019-0370-y
Tattevin, P., Monnier, D., Tribut, O., Dulong, J., Bescher, N., Mourcin, F., et al. (2010). Enhanced indoleamine 2,3-dioxygenase activity in patients with severe sepsis and septic shock. J. Infect. Dis. 201, 956–966.
Taylor, M. W., and Feng, G. S. (1991). Relationship between interferon-gamma, indoleamine 2,3-dioxygenase, and tryptophan catabolism. FASEB J. 5, 2516–2522. doi: 10.1096/fasebj.5.11.1907934
Théate, I., van Baren, N., Pilotte, L., Moulin, P., Larrieu, P., Renauld, J. C., et al. (2015). Extensive profiling of the expression of the indoleamine 2,3-dioxygenase 1 protein in normal and tumoral human tissues. Cancer Immunol. Res. 3, 161–172.
Wang, X., Li, Z. X., Wen, Y. P., and Chang, C. (2018). [Value of indoleamine 2,3-dioxygenase in diagnosis of systemic inflammatory response syndrome after cardiopulmonary bypass in children with congenital heart disease]. Zhongguo Dang Dai Er Ke Za Zhi 20, 28–31. doi: 10.7499/j.issn.1008-8830.2018.01.006
Watanabe, N., Wang, Y. H., Lee, H. K., Ito, T., Wang, Y. H., Cao, W., et al. (2005). Hassall’s corpuscles instruct dendritic cells to induce CD4+CD25+ regulatory T cells in human thymus. Nature 436, 1181–1185. doi: 10.1038/nature03886
Xie, F. T., Cao, J. S., Zhao, J., Yu, Y., Qi, F., and Dai, X. C. (2015). IDO expressing dendritic cells suppress allograft rejection of small bowel transplantation in mice by expansion of Foxp3+ regulatory T cells. Transpl. Immunol. 33, 69–77. doi: 10.1016/j.trim.2015.05.003
Yoshio, S., Sugiyama, M., Shoji, H., Mano, Y., Mita, E., Okamoto, T., et al. (2016). Indoleamine-2,3-dioxygenase as an effector and an indicator of protective immune responses in patients with acute hepatitis B. Hepatology 63, 83–94. doi: 10.1002/hep.28282
Zhai, L., Ladomersky, E., Dostal, C. R., Lauing, K. L., Swoap, K., Billingham, L. K., et al. (2017). Non-tumor cell IDO1 predominantly contributes to enzyme activity and response to CTLA-4/PD-L1 inhibition in mouse glioblastoma. Brain Behav. Immun. 62, 24–29. doi: 10.1016/j.bbi.2017.01.022
Keywords: immune system, cardiac surgery, indoleamine-2, 3-dioxygenase, tryptophan, immunosuppression
Citation: Bello C, Heinisch PP, Mihalj M, Carrel T and Luedi MM (2021) Indoleamine-2,3-Dioxygenase as a Perioperative Marker of the Immune System. Front. Physiol. 12:766511. doi: 10.3389/fphys.2021.766511
Received: 29 August 2021; Accepted: 14 October 2021;
Published: 08 November 2021.
Edited by:
Jan Larmann, Heidelberg University Hospital, GermanyReviewed by:
Sebastian Roth, University Hospital of Düsseldorf, GermanyLaura Kummer, Heidelberg University Hospital, Germany
Hans-Joerg Gillmann, Hannover Medical School, Germany
Copyright © 2021 Bello, Heinisch, Mihalj, Carrel and Luedi. This is an open-access article distributed under the terms of the Creative Commons Attribution License (CC BY). The use, distribution or reproduction in other forums is permitted, provided the original author(s) and the copyright owner(s) are credited and that the original publication in this journal is cited, in accordance with accepted academic practice. No use, distribution or reproduction is permitted which does not comply with these terms.
*Correspondence: Markus M. Luedi, Markus.Luedi2@insel.ch