- 1Anesthesia and Intensive Care, San Martino Policlinico Hospital, Istituto di Ricovero e Cura a Carattere Scientifico (IRCCS) for Oncology and Neurosciences, Genoa, Italy
- 2Department of Surgical Sciences and Integrated Diagnostics (DISC), University of Genoa, Genoa, Italy
- 3Humanitas Clinical and Research Center—Istituto di Ricovero e Cura a Carattere Scientifico (IRCCS), Rozzano, Italy
- 4Radiology Department San Martino Policlinico Hospital, Istituto di Ricovero e Cura a Carattere Scientifico (IRCCS) for Oncology and Neurosciences, Genoa, Italy
- 5Laboratory of Pulmonary Investigation, Carlos Chagas Filho Institute of Biophysics, Federal University of Rio de Janeiro, Rio de Janeiro, Brazil
Background: The pathophysiological effects of positive end-expiratory pressure (PEEP) on respiratory mechanics, lung recruitment, and intracranial pressure (ICP) in acute brain-injured patients have not been completely elucidated. The primary aim of this study was to assess the effects of PEEP augmentation on respiratory mechanics, quantitative computed lung tomography (qCT) findings, and its relationship with ICP modifications. Secondary aims included the assessment of the correlations between different factors (respiratory mechanics and qCT features) with the changes of ICP and how these factors at baseline may predict ICP response after greater PEEP levels.
Methods: A prospective, observational study included mechanically ventilated patients with acute brain injury requiring invasive ICP and who underwent two-PEEP levels lung CT scan. Respiratory system compliance (Crs), arterial partial pressure of carbon dioxide (PaCO2), mean arterial pressure (MAP), data from qCT and ICP were obtained at PEEP 5 and 15 cmH2O.
Results: Sixteen examinations (double PEEP lung CT and neuromonitoring) in 15 patients were analyzed. The median age of the patients was 54 years (interquartile range, IQR = 39–65) and 53% were men. The median Glasgow Coma Scale (GCS) at intensive care unit (ICU) admission was 8 (IQR = 3–12). Median alveolar recruitment was 2.5% of total lung weight (−1.5 to 4.7). PEEP from 5 to 15 cmH2O increased ICP [median values from 14.0 (11.2–17.5) to 23.5 (19.5–26.8) mmHg, p < 0.001, respectively]. The amount of recruited lung tissue on CT was inversely correlated with the change (Δ) in ICP (rho = −0.78; p = 0.0006). Additionally, ΔCrs (rho = −0.77, p = 0.008), ΔPaCO2 (rho = 0.81, p = 0.0003), and ΔMAP (rho = −0.64, p = 0.009) were correlated with ΔICP. Baseline Crs was not predictive of ICP response to PEEP.
Conclusions: The main factors associated with increased ICP after PEEP augmentation included reduced Crs, lower MAP and lung recruitment, and increased PaCO2, but none of these factors was able to predict, at baseline, ICP response to PEEP. To assess the potential benefits of increased PEEP in patients with acute brain injury, hemodynamic status, respiratory mechanics, and lung morphology should be taken into account.
Introduction
A substantial number of patients with acute brain injury require mechanical ventilation (Borsellino et al., 2016) due to both neurological and respiratory causes (Della Torre et al., 2017). The aim of mechanical ventilation is to optimize oxygen delivery and minimize lung and brain injury (Frisvold et al., 2019). The use of lung-protective ventilation strategies has been shown to reduce morbidity and mortality in acute respiratory distress syndrome (ARDS) and non-ARDS critically ill patients (Sutherasan et al., 2014; Serpa Neto et al., 2015; Simonis et al., 2018). However, strategies comprising the use of high positive end-expiratory pressure (PEEP) have been challenged in brain-injured patients because of concerns regarding their effects on cerebral hemodynamics (Nemer et al., 2011; Borsellino et al., 2016; Robba et al., 2020), in particular intracranial pressure (ICP). Possible mechanisms responsible for ICP augmentation after PEEP application include alveolar overdistension with the increase of arterial partial pressure of carbon dioxide (PaCO2) levels, and hemodynamic instability (Caricato et al., 2005; Mascia et al., 2005; Nemer et al., 2011). To date, the pathophysiological interplay between intracranial changes, respiratory system mechanics, and alveolar recruitment has not been completely elucidated, and no specific recommendations are available regarding the optimal levels of PEEP to be applied in acute brain-injured patients (Robba et al., 2020). We, therefore, conducted an observational study whose primary aim was to investigate the effects of two levels of PEEP (5 and 15 cmH2O) on respiratory mechanics, quantitative lung computed tomography (qCT) findings, and its relationship with ICP changes in brain-injured patients. Secondary aims included the assessment of the correlation between different factors (respiratory mechanics and qCT features) with the changes of ICP, and how these factors at baseline may predict ICP response after greater PEEP levels. Finally, we explored whether non-invasive neuromonitoring tools are able to assess changes of ICP following augmented PEEP levels. We hypothesized that the effect of greater PEEP levels on ICP depends on the amount of alveolar recruitment and respiratory mechanics.
Methods
We followed the “Strengthening the Reporting of Observational Studies in Epidemiology (STROBE)” statement guidelines for observational cohort studies (Supplementary Table 1) (von Elm et al., 2014). This study was performed at San Martino Policlinico Hospital, Genoa, Italy, a tertiary academic hospital with neurocritical care facilities, from August 1, 2020, to March 8, 2021. The study was approved by the ethics review board “Comitato Etico Regione Liguria” (protocol n. CER Liguria: 23/2020). According to the local regulations, written consent was obtained from next of kin of the patients, as all patients were unconscious at the time of inclusion.
Inclusion and Exclusion Criteria
Inclusion criteria were critically ill adult patients who required intubation and invasive mechanical ventilation following acute brain injury (traumatic brain injury, TBI; subarachnoid hemorrhage, SAH; intracranial hemorrhage, ICH) admitted to the intensive care unit (ICU), requiring invasive ICP and other neuromonitoring tools (Transcranial Doppler, TCD and optic nerve sheath diameter, ONSD) and who underwent two-PEEP CT scan based on clinical indication with PEEP 5 and 15 cmH2O. Exclusion criteria were the absence of informed consent; the absence of indications for invasive ICP monitoring (i.e., coagulopathy); the absence of temporal window for TCD evaluation; basal skull fracture with the cerebrospinal fluid leak, or ocular trauma for ONSD measurement; patients requiring contrast medium during CT for clinical reasons or having contraindications to higher PEEP (e.g., emphysema and undrained pneumothorax), or judged too instable to be safely transported to the CT facility (e.g., hemodynamic instability, need for high doses vasopressors, or acute and refractory increased ICP).
Data Collection and Patients' Management
Demographic, epidemiologic, and clinical data were obtained from electronic medical records of patients and collected by physicians trained in critical care at admission to the ICU and on the day when a double PEEP CT scan was obtained. Recorded data included admission demographics [age, gender, and body mass index (BMI)], comorbidities (asthma, chronic respiratory disease, hypertension, chronic cardiac disease diabetes mellitus, chronic kidney injury, and previous neurological disease), type of brain injury, neurological status at ICU admission (Glasgow Coma Scale, GCS), type of ICP monitoring (intraparenchymal and external ventricular drain), ICU complications, ICU length of stay (LOS), and Glasgow Outcome Score (GOS) at ICU discharge. Patients were sedated with propofol and/or midazolam and fentanyl, targeting the tidal volume of 6–8 ml per kg of predicted body weight (PBW), but increases were tolerated based on the driving pressure. The respiratory rate was titrated to maintain pH between 7.35 and 7.45. On the day of CT scan, ventilatory data, respiratory mechanics, and blood gases parameters (i.e., inspired fraction of oxygen (FiO2), PEEP, plateau pressure (Pplat), respiratory system compliance (Crs), tidal volume (VT), respiratory rate (RR), arterial and venous saturation of oxygen (SaO2 and SvO2), arterial pH (pHa), partial pressure of oxygen (PaO2 and PaCO2) were obtained at PEEP = 5 cmH2O (T0) and at PEEP = 15 cmH2O (T1). Vital signs, such as mean arterial pressure (MAP) and neuromonitoring parameters [ICP, ONSD, systolic, mean, and diastolic flow velocities (FVs, FVd, and FVm)] were also collected at T0 and T1.
Clinical Rationale for PEEP Test
The decision to perform a PEEP test was based on the judgment of the treating physician, if optimization of mechanical ventilation was required. PEEP test was performed in Volume-Controlled Ventilation aiming to target the tidal volume of 6–8 ml per kg/PBW. To date, no universal recommendations are available concerning the optimal PEEP levels in the invasively ventilated brain-injured patients (Robba et al., 2020). Therefore, in our institution, a PEEP test is performed to increasing PEEP from 5 to 15 cmH2O, assessing both respiratory mechanics and cerebral hemodynamics. These values have previously been demonstrated to be safe and can lead to increased brain oxygenation, without the increase in ICP (Nemer et al., 2011). However, as greater PEEP levels may result in worsening of the respiratory mechanics with eventually increased alveolar hyperdistention (Mascia et al., 2005), two-PEEP CT, when possible, has become part of our routine clinical evaluation and has been performed in our institution in other groups of patients (Ball et al., 2021). Evaluation and calculation of gas exchanges, respiratory mechanics, and details on the protocol for two-PEEP CT acquisition and analysis are described in the ESM.
CT Scan Acquisition and Analysis
Images were acquired during expiratory breath-hold at 5 and 15 cmH2O. The two scans were acquired in sequence, interleaved by 1–2 min of uninterrupted ventilation at PEEP 15 cmH2O (Ball et al., 2021). This time of ventilation at 15 cmH2O of PEEP was applied before repeating the CT scan (T1). For safety reasons, no recruitment maneuver was performed.
Lung segmentation was performed excluding big airway, vessels, and pleural effusion. Segmentations were performed using ITKSnap (http://www.itksnap.org), image analysis was performed with Matlab scripts (Mathworks, MA, USA), based on widely adopted numerical methods (Malbouisson et al., 2001). Alveolar recruitment was defined as the difference in the non-aerated compartment from PEEP 5–15 cmH2O, divided by total lung weight at PEEP of 5 cmH2O (Gattinoni et al., 2006).
Neuromonitoring
The indications for invasive ICP placement followed the latest Brain Trauma Foundation Guidelines (Carney et al., 2016). Ultrasound measurement was performed by a selected group of experienced operators (CR, SN, and DB) using a standardized insonation technique to reduce inter-operator variability. Ultrasound measurements were performed after PEEP augmentation and after repeating the second CT.
Transcranial Doppler was performed bilaterally on the middle cerebral artery (MCA) through the temporal window using a traditional 2-MHz transducer (Philips SparQ®) as previously described (Robba et al., 2017b). Non-invasive ICP estimation using TCD (ICPTCD) was obtained using a previously validated formula (Rasulo et al., 2017). Ultrasound examination of the ONSD was performed using a 7.5 MHz linear ultrasound probe (Philips SparQ®) using the lowest possible acoustic power that could measure the ONSD. The probe was oriented perpendicularly in the vertical plane and at around 30° in the horizontal plane on the closed eyelids of both eyes of subjects in the supine position. Ultrasound gel was applied on the surface of each eyelid, and the measurements were made in the axial and sagittal planes of the widest diameter visible 3 mm behind the retina in both eyes. The final ONSD value was calculated as previously described (Robba et al., 2016, 2017a).
Statistical Analysis
An a priori sample size calculation was not feasible due to the lack of data on quantitative CT analysis in brain-injured patients, but our sample size was similar to previous physiologic studies regarding PEEP augmentation in ARDS or brain-injured patients (Mascia et al., 2005; Nemer et al., 2011; Mauri et al., 2016, 2020). Data are reported as median (interquartile range, IQR), if not otherwise specified. We compared data between groups with the Mann–Whitney U or Fisher's exact test, as appropriate. Variables acquired at two-PEEP levels were compared with the Wilcoxon signed-rank test. Changes of variables from PEEP 5 to PEEP 15 were calculated as Δ (value at PEEP 15 cmH2O–value at PEEP 5 cmH2O). Correlations were sought using Spearman's rho. A linear regression analysis was performed using ΔICP (invasive ICP) as the dependent variable and alveolar recruitment, ΔMAP, Crs, and PaCO2 as independent variables. As an exploratory analysis, we modeled ΔICP as a function of clinically sound covariates using linear regression, adopting a variance inflation factor threshold of 5 as an acceptable limit for multi-collinearity. All statistical analyses were performed in SPSS Statistics, Version 25.0 (IBM Corp., Armonk, NY, USA). Significance was assumed at two-tailed p < 0.05.
Results
General Characteristics
A total of 16 examinations (double PEEP quantitative CT and neuromonitoring, including invasive ICP, ONSD, and TCD) from 15 patients were included in the analysis (in one patient, the measures were obtained twice). The median age of the patients was 54 years (IQR = 39–65); 53.3% were men. Six patients (40%) were admitted for SAH, six after TBI (40%), and three (20%) for ICH (Table 1). The median GCS was eight (IQR = 3–12); intraparenchymal and intraventricular monitoring were inserted in seven (46.6%) and eight (53.3%) cases, respectively. One patient died in ICU (6.6%) and the median ICU LOS was 16 (IQR = 13–21) days.
Effect of PEEP Augmentation on Respiratory Mechanics, Quantitative CT Findings, and ICP
Figure 1 shows two representative examples of CT images at 5 and 15 cmH2O of PEEP in two patients with low and high alveolar recruitment. After greater PEEP levels, systemic oxygenation [PaO2, from 96.4 (81–108) to 98 (85.4–148) mmHg; p = 0.039], and PaCO2 [from 40 (36.8–44.9) to 44 (41.4–48.6) mmHg; p = 0.034] increased, while median Crs did not change (Table 2 and Figure 2). Total lung volume was augmented after greater PEEP levels, as also the gas volume, but not total lung weight (Table 2). Median alveolar recruitment was 2.5% (−1.5–4.7). Figure 3 illustrates the frequency distribution of Hounsfield units at 5 and 15 cmH2O of PEEP. The increase of PEEP from 5 to 15 cmH2O resulted in higher median invasive ICP values [14 (11.2–17.5) vs. 23.5 (19.5–26.8) mmHg, p < 0.001; Figure 2] and non-invasive ICP measured through TCD and of ONSD values. Higher PEEP also resulted in a significant reduction of cerebral perfusion pressure (CPP) [78 (71–81.7) vs. 63 (57.8–74.8) mmHg, p = 0.001; Table 2].
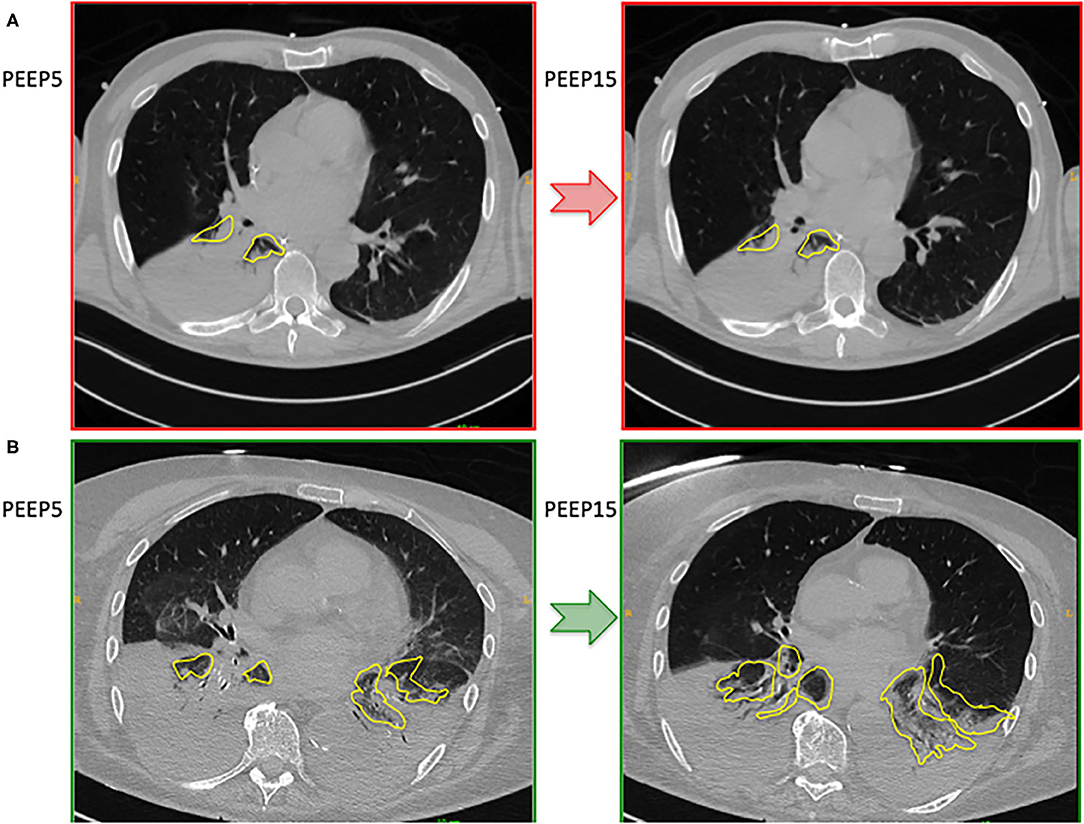
Figure 1. Representative cases representing CT images at 5 and 15 cmH2O of PEEP in a patient with poor alveolar recruitment (A) and good alveolar recruitment (B).
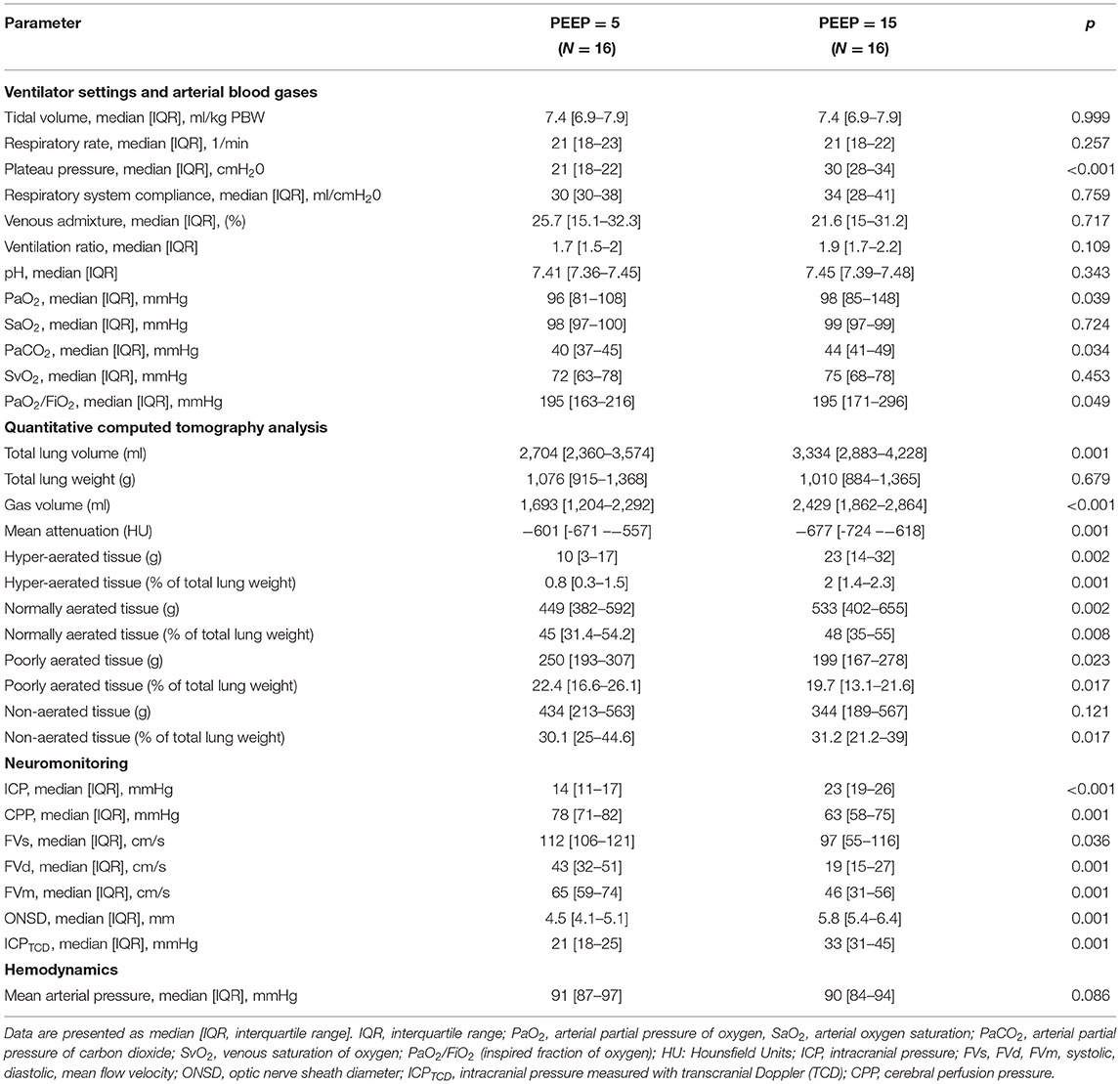
Table 2. Ventilator settings, respiratory mechanics, arterial blood gases, quantitative CT analysis, neuromonitoring data, and hemodynamics in our cohort at PEEP = 5 and 15 cmH2O.
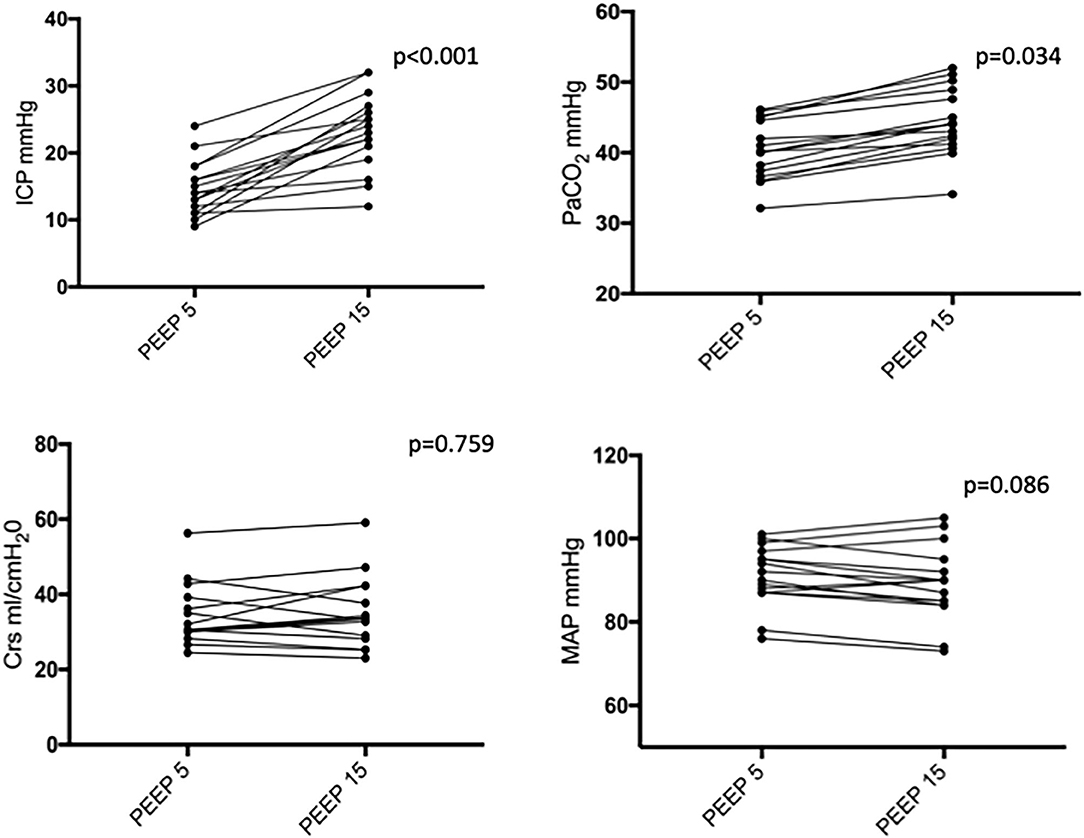
Figure 2. Intracranial pressure (ICP), partial pressure of carbon dioxide (PaCO2), respiratory system compliance (Crs), and mean arterial pressure (MAP) at PEEP of 5 and 15 cmH2O. Black dots and lines represent individual patient data. PEEP, positive end-expiratory pressure.
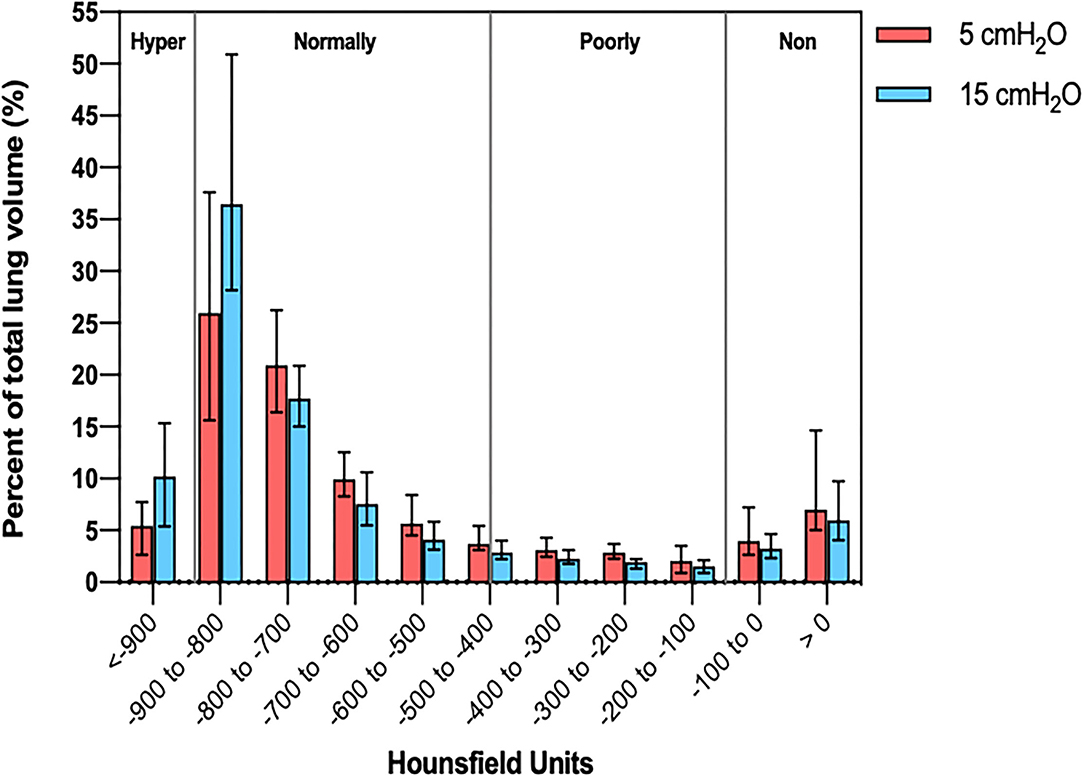
Figure 3. Histogram distribution of lung volume aeration at 5 and 15 cmH2O of PEEP. PEEP, positive end-expiratory pressure.
Correlation Between the Changes of Quantitative CT Variables, Respiratory Mechanics, and ICP
The variations of lung volume and gas volume evaluated at qCT analysis were not correlated with the changes of ICP (rho = 0.05; p = 0.86 and rho = −0.07; p = 0.80, respectively). However, the amount of recruited tissue was inversely correlated with ΔICP (rho = −0.78; p = 0.0006). ΔCrs, ΔPlateau pressure, ΔPaCO2, and ΔMAP were significantly correlated with ΔICP (rho = −0.77; p = 0.008; rho = 0.54; p = 0.0002; rho = 0.81; p = 0.0003; rho = −0.64; p = 0.009, respectively; Figure 4).
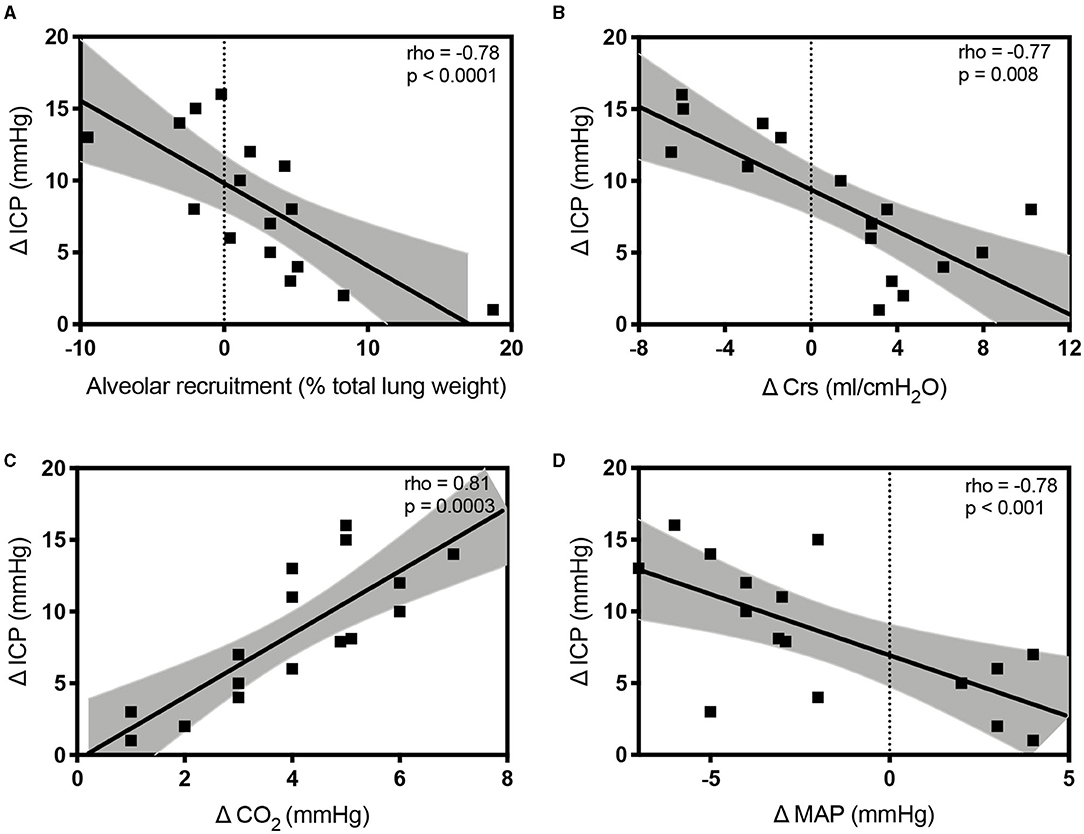
Figure 4. Scatterplots showing the linear association and correlation between alveolar recruitment (A), Δ respiratory system compliance (Crs; B), Δ carbon dioxide (PaCO2; C), Δ mean arterial pressure (MAP; D) vs. Δ intracranial pressure (ICP) at different study time points. Dotted lines represent the 95% CIs for the linear regression.
At linear regression analysis, ΔPaCO2 (regression coefficient B = 0.96, 95% CI from 0.1 to 1.8, p = 0.028) and ΔCrs (B = −0.41, 95% CI from −0.647 to −0.183, p = 0.02) were the only independently variables associated with ΔICP. We did not observe correlations between the ICP increase and the following parameters assessed at PEEP 5 cmH2O: Crs, CO2, MAP, ONSD, ICPTCD, and invasive ICP (p > 0.40 in all correlations with ΔICP).
Correlation analysis between qCT variables, respiratory mechanics, and non-invasive ICP estimated through TCD and ONSD are presented in Supplementary Table 2. A significant correlation was found between the changes of ONSD and ICP (rho = 0.8096; p = 0.0003), but not between ICPTCD and ICP (Supplementary Figure 1).
Discussion
In a population of mechanically ventilated patients with acute brain injury, we found that (1) PEEP augmentation from 5 to 15 cmH2O may lead to higher oxygenation, PaCO2, and ICP values, with alveolar recruitment of 2.5% of total lung weight; (2) ICP increase with PEEP was correlated to higher PaCO2, poor alveolar recruitment, reduction of Crs, and decreased MAP; (3) baseline values of Crs, PaCO2, MAP, and ICP are not predictive for ICP increase with PEEP; and (4) changes in ONSD, but not ICPTCD, are correlated to changes in ICP.
To our knowledge, this is the first study quantitatively assessing alveolar recruitment and its distribution in the whole lung in mechanically ventilated patients with acute brain injury and its associations with changes in ICP and main physiological and clinical parameters.
Acute brain-injured patients with a clinical indication for chest CT, such as respiratory failure, and/or suspect of pneumonia were included in the study. This explains why the alveolar recruitment induced by PEEP was 2.5% of total lung weight, similar to a previous cohort of patients with COVID-19 (Ball et al., 2021). In fact, in the healthy population, the average lung weight is 930 g and gas volume 4,000 ml (Cressoni et al., 2013) in patients with ARDS-COVID-19, average lung weight is 1,500 g and gas volume 1,360 ml (Ball et al., 2021), whereas in our cohort of acute brain-injured patients average lung weight is 1,076 g and gas volume 1,693 ml.
Patients with acute brain injury admitted to ICU frequently require mechanical ventilation, and PEEP is often useful to support oxygenation, with the aim to ensure reliable oxygen delivery (Stevens et al., 2008; Borsellino et al., 2016; Del Sorbo et al., 2017) and minimize lung injury. However, mechanical ventilation and increased intrathoracic pressure can exert harmful effects on the brain due to complex physiological interactions between brain and lung compartments. The optimization of PEEP application in the general ICU population (Sutherasan et al., 2014; Algera et al., 2020) and in particular in patients with acute brain injury is still controversial. Recently, a systematic review of the literature revealed only marginal evidence for a specific ventilatory strategy in this group of patients (Robba et al., 2020), and only a few small physiologic studies have explored the effect of PEEP on intracranial dynamics (Caricato et al., 2005; Nemer et al., 2011). The use of higher PEEP may lead to possible negative hemodynamic effects, which could potentially lead to a reduction of MAP and therefore CPP. However, two small studies suggested that slow and progressive PEEP augmentation may be safe and can improve systemic and cerebral oxygenation without significant changes in ICP and CPP (Huynh et al., 2002; Nemer et al., 2011). Another important pathophysiological mechanism is the effect of respiratory mechanics on ICP. Caricato et al. (2005) demonstrated that in patients with low Crs (those with greater severity of lung injury and requiring higher PEEP), PEEP application had no important effects on cerebral and systemic hemodynamics. However, this finding was not confirmed in the present study. We observed a correlation between changes in ICP and worsening of Crs, but the absolute value of Crs at lower PEEP was not predictive for ICP increase with PEEP. We speculate that Crs measured at lower PEEP alone might not identify patients that will increase ICP at higher PEEP is not necessarily associated with the potential for lung recruitment. Patients with greater lung recruitment will improve lung gas distribution not resulting in ICP increase, while non-recruiters will over-inflate already aerated areas with a negative impact on dead space and possibly on venous return. In a prospective study that has 12 brain-injured patients, where 5 and 10 cmH2O of PEEP was randomly applied, patients defined as recruiters increased Crs and PaO2, while in non-recruiters Crs decreased and PaCO2 increased. Furthermore, ICP and jugular saturation remained constant in recruiters but significantly increased in non-recruiters, showing a significant correlation between changes in ICP, compliance, and PaCO2.
This suggests that PEEP may have a detrimental effect on ICP only when it causes alveolar hyperinflation leading to a significant increase in PaCO2, whereas when PEEP leads to good alveolar recruitment, ICP does not change.
Our results and previous evidence suggest that a precise evaluation of respiratory mechanics and gas exchange modifications may be of great importance in the assessment of recruitment. Comparative studies have shown that the only possible method to evaluate the amount of collapsed lung tissue regaining inflation is the CT scan (Gattinoni et al., 2017), thus making our study unique in the description of the pathophysiological effects of PEEP on the intracranial compartment, based on the characteristics of lung morphology. Our results show that in patients with acute brain injury increased PaCO2 and reduction of CPP and Crs with PEEP are the main factors associated with increased ICP. In fact, the potential mechanisms related to the overall ICP increase observed in our cohort might be related to the increase of PaCO2 and reduction of CPP. However, as MAP did not change, this latter mechanism was probably related to a reduction of intracranial compliance consequent to the supine position, thus possibly reducing jugular venous outflow.
We also demonstrated that the amount of alveolar recruitment is an important determinant of changes in ICP, thus suggesting that in patients with good response to alveolar recruitment, which leads to the improvement of Crs without affecting hemodynamic status and without causing alveolar hyperdistension of patients and therefore increased PaCO2, PEEP augmentation might be safe.
All in it, the principles for PEEP safety and titration in patients with acute brain injury seem not to be importantly different from those applied in the general ICU population and should take into account hemodynamic status, respiratory mechanics, and CT findings of patients (Ball et al., 2021). Indeed, a recent expert consensus (Robba et al., 2020) suggested that in brain-injured patients the levels of PEEP should be the same as for the general critically ill population. Similarly, a survey of the European Society of Intensive Care (Stocchetti et al., 2014) and a recent large multicenter study (Tejerina et al., 2021) suggest that moderate-high levels of PEEP are currently part of the clinical practice of neurocritical care physicians.
Finally, we explored the potential role of non-invasive ICP methods for the evaluation of changes of ICP after PEEP application. We found no significant correlation between non-invasive methods and qCT or respiratory mechanics data. This suggests that invasive ICP methods should be always considered as the gold standard for the evaluation of cerebral hemodynamics (Robba et al., 2015). However, changes in ONSD seem to be correlated with changes in ICP, thus making this tool a promising method for the bedside assessment of intracranial modifications when ICP is not available or contraindicated (Robba et al., 2018).
There are several limitations in our study that deserve to be mentioned. First, the sample size is small, despite similar to previous physiological studies exploring the effect of PEEP on lung recruitment (Mascia et al., 2005; Nemer et al., 2011; Mauri et al., 2016, 2020).
Second, in our center, a CT scan with double PEEP is routinely performed in selected patients with acute brain-injured patients, but only when CT is clinically indicated and in sufficiently stable patients. Therefore, patients were affected by brain damage of different nature and were heterogeneous as for comorbidities and lung damage.
Third, we cannot exclude that different ventilator setting may have led to different results (Gattinoni et al., 2006). However, we standardized mechanical ventilator settings, respiratory mechanics evaluation, and arterial blood gases measurement. In addition, we used a relatively short time for high PEEP exposure before repetition of CT. However, studies showed that most changes in volume and recruitment occur in this timeframe and that most respiratory units recruit below 30 cmH2O (Katz et al., 1981; Crotti et al., 2001). In fact, we were able to detect a clear recruitment effect in some patients. In addition, more data and details regarding hemodynamics and cardiac performance would have added greater insights regarding the effect of PEEP on cardiac function; however, unfortunately, we do not routinely perform in our institution echocardiography or carotid flow assessment during PEEP challenge. Finally, patients were in a supine position during CT, and this might have led to an increase of ICP regardless of the implementation of PEEP.
Conclusions
Quantitative CT can help in the assessment of lung recruitability and the effect of different PEEP levels on ICP. The main factors associated with an increase of ICP after PEEP augmentation include worsening of Crs, reduction of MAP, low lung recruitment, and increased PaCO2. The potential benefits of PEEP augmentation in acute brain-injured patients should take into account hemodynamic status, respiratory mechanics, and lung morphology of patients. Further research is warranted to assess the effect of PEEP on ICP and the application of non-invasive ICP methods in this context.
Data Availability Statement
The raw data supporting the conclusions of this article will be made available by the authors, without undue reservation.
Ethics Statement
The studies involving human participants were reviewed and approved by Comitato Etico Genova, Liguria. The patients/participants provided their written informed consent to participate in this study.
Author Contributions
CR, LB, PR, and PP designed the study and the methods. CR, SN, and DB collected the data. LB and CR performed the statistical analysis. All the authors participated in data evaluation, analysis, manuscript revision, and final manuscript approval.
Conflict of Interest
The authors declare that the research was conducted in the absence of any commercial or financial relationships that could be construed as a potential conflict of interest.
Publisher's Note
All claims expressed in this article are solely those of the authors and do not necessarily represent those of their affiliated organizations, or those of the publisher, the editors and the reviewers. Any product that may be evaluated in this article, or claim that may be made by its manufacturer, is not guaranteed or endorsed by the publisher.
Supplementary Material
The Supplementary Material for this article can be found online at: https://www.frontiersin.org/articles/10.3389/fphys.2021.711273/full#supplementary-material
References
Algera, A. G., Pisani, L., Serpa Neto, A., den Boer, S. S., Bosch, F. F. H., Bruin, K., et al. (2020). Effect of a lower vs. higher positive end-expiratory pressure strategy on ventilator-free days in ICU patients without ARDS: a randomized clinical trial. J. Am. Med. Assoc. 324, 2509–2520. doi: 10.1001/jama.2020.23517
Ball, L., Robba, C., Maiello, L., Herrmann, J., Gerard, S. E., Xin, Y., et al. (2021). Computed tomography assessment of PEEP-induced alveolar recruitment in patients with severe COVID-19 pneumonia. Crit. Care. 25:81. doi: 10.1186/s13054-021-03477-w
Borsellino, B., Schultz, M. J., Gama de Abreu, M., Robba, C., and Bilotta, F. (2016). Mechanical ventilation in neurocritical care patients: a systematic literature review. Expert. Rev. Respir. Med. 10, 1123–1132. doi: 10.1080/17476348.2017.1235976
Caricato, A., Conti, G., Corte, F., Della, M, A., Santilli, F., Sandroni, C., et al. (2005). Effects of PEEP on the intracranial system of patients with head injury and subarachnoid hemorrhage: the role of respiratory system compliance. J. Trauma Inj. Infect. Crit. Care 58, 571–576. doi: 10.1097/01.TA.0000152806.19198.DB
Carney, N., Totten, A. M., O'Reilly, C., Ullman, J. S., Hawryluk, G. W. J., Bell, M. J., et al. (2016). Guidelines for the management of severe traumatic brain injury, fourth edition. Neurosurgery 2016, 1–10. doi: 10.1227/NEU.0000000000001432
Cressoni, M., Gallazzi, E., Chiurazzi, C., Marino, A., Brioni, M., Menga, F., et al. (2013). Limits of normality of quantitative thoracic CT analysis. Crit. Care. 17:R93. doi: 10.1186/cc12738
Crotti, S., Mascheroni, D., Caironi, P., Pelosi, P., Ronzoni, G., Mondino, M., et al. (2001). Recruitment and derecruitment during acute respiratory failure. Am. J. Respir. Crit. Care Med. 164, 131–140. doi: 10.1164/ajrccm.164.1.2007011
Del Sorbo, L., Goligher, E. C., McAuley, D. F., Rubenfeld, G. D., Brochard, L. J., Gattinoni, L., et al. (2017). Mechanical ventilation in adults with acute respiratory distress syndrome: summary of the experimental evidence for the clinical practice guideline. Ann. Am. Thorac Soc. 14, S261–S270. doi: 10.1513/AnnalsATS.201704-345OT
Della Torre, V., Badenes, R., Corradi, F., Racca, F., Lavinio, A., Matta, B., et al. (2017). Acute respiratory distress syndrome in traumatic brain injury: how do we manage it? J. Thorac Dis. 9, 5369–5381. doi: 10.21037/jtd.2017.11.03
Frisvold, S. K., Robba, C., and Guérin, C. (2019). What respiratory targets should be recommended in patients with brain injury and respiratory failure? Intens. Care Med. 45, 683–686. doi: 10.1007/s00134-019-05556-7
Gattinoni, L., Caironi, P., Cressoni, M., Chiumello, D., Ranieri, V. M., Quintel, M., et al. (2006). Lung recruitment in patients with the acute respiratory distress syndrome. N. Engl. J. Med. 354, 1775–1786. doi: 10.1056/NEJMoa052052
Gattinoni, L., Collino, F., Maiolo, G., Rapetti, F., Romitti, F., Tonetti, T., et al. (2017). Positive end-expiratory pressure: how to set it at the individual level. Ann. Transl. Med. 5:288. doi: 10.21037/atm.2017.06.64
Huynh, T., Messer, M., Sing, R. F., Miles, W., Jacobs, D. G., Thomason, M. H., et al. (2002). Positive end-expiratory pressure alters intracranial and cerebral perfusion pressure in severe traumatic brain injury. J. Trauma 53, 488–492. doi: 10.1097/00005373-200209000-00016
Katz, J. A., Ozanne, G. M., Zinn, S. E., and Fairley, H. B. (1981). Time course and mechanisms of lung-volume increase with PEEP in acute pulmonary failure. Anesthesiology 54, 9–16. doi: 10.1097/00000542-198101000-00003
Malbouisson, L. M., Muller, J. C., Constantin, J. M., Lu, Q., Puybasset, L., Rouby, J. J., et al. (2001). Computed tomography assessment of positive end-expiratory pressure-induced alveolar recruitment in patients with acute respiratory distress syndrome. Am. J. Respir. Crit. Care Med. 163, 1444–1450. doi: 10.1164/ajrccm.163.6.2005001
Mascia, L., Grasso, S., Fiore, T., Bruno, F., Berardino, M., and Ducati, A. (2005). Cerebro-pulmonary interactions during the application of low levels of positive end-expiratory pressure. Intens. Care Med. 31, 373–379. doi: 10.1007/s00134-004-2491-2
Mauri, T., Eronia, N., Turrini, C., Battistini, M., Grasselli, G., Rona, R., et al. (2016). Bedside assessment of the effects of positive end-expiratory pressure on lung inflation and recruitment by the helium dilution technique and electrical impedance tomography. Intens. Care Med. 42, 1576–1587. doi: 10.1007/s00134-016-4467-4
Mauri, T., Spinelli, E., Scotti, E., Colussi, G., Basile, M. C., Crotti, S., et al. (2020). Potential for lung recruitment and ventilation-perfusion mismatch in patients with the acute respiratory distress syndrome from coronavirus disease 2019. Crit. Care Med. 48, 1129–1134. doi: 10.1097/CCM.0000000000004386
Nemer, S., Santos, R., Caldeira, J., Reis, P., Guimarães, B., Loureiro, T., et al. (2011). Positive end-expiratory pressure can increase brain tissue oxygen pressure in hypoxemic severe traumatic brain injury patients. Crit. Care. 15:P41. doi: 10.1186/cc10189
Rasulo, F. A., Bertuetti, R., Robba, C., Lusenti, F., Cantoni, A., Bernini, M., et al. (2017). The accuracy of transcranial Doppler in excluding intracranial hypertension following acute brain injury: a multicenter prospective pilot study. Crit. Care. 21:44. doi: 10.1186/s13054-017-1632-2
Robba, C., Bragazzi, N. L., Bertuccio, A., Cardim, D., Donnelly, J., Sekhon, M., et al. (2017a). Effects of prone position and positive end-expiratory pressure on noninvasive estimators of ICP: a pilot study. J. Neurosurg. Anesthesiol. 29, 243–250. doi: 10.1097/ANA.0000000000000295
Robba, C., Cardim, D., Donnelly, J., Bertuccio, A., Bacigaluppi, S., Bragazzi, N., et al. (2016). Effects of pneumoperitoneum and Trendelenburg position on intracranial pressure assessed using different non-invasive methods. Br. J. Anaesth. 117, 783–791. doi: 10.1093/bja/aew356
Robba, C., Cardim, D., Tajsic, T., Pietersen, J., Bulman, M., Donnelly, J., et al. (2017b). Ultrasound non-invasive measurement of intracranial pressure in neurointensive care: a prospective observational study. PLoS Med. 14:1002356. doi: 10.1371/journal.pmed.1002356
Robba, C., Donnelly, J., Bertuetti, R., Cardim, D., Sekhon, M. S., Aries, M., et al. (2015). Doppler non-invasive monitoring of ICP in an animal model of acute intracranial hypertension. Neurocrit. Care 23, 419–426. doi: 10.1007/s12028-015-0163-4
Robba, C., Poole, D., McNett, M., Asehnoune, K., Bösel, J., Bruder, N., et al. (2020). Mechanical ventilation in patients with acute brain injury: recommendations of the European Society of Intensive Care Medicine consensus. Intens. Care Med. 46, 2397–2410. doi: 10.1007/s00134-020-06283-0
Robba, C., Santori, G., Czosnyka, M., Corradi, F., Bragazzi, N., Padayachy, L., et al. (2018). Optic nerve sheath diameter measured sonographically as non-invasive estimator of intracranial pressure: a systematic review and meta-analysis. Intens. Care Med. 44, 1284–1294. doi: 10.1007/s00134-018-5305-7
Serpa Neto, A., Hemmes, S. N. T., Barbas, C. S. V., Beiderlinden, M., Biehl, M., Binnekade, J. M., et al. (2015). Protective versus conventional ventilation for surgery: a systematic review and individual patient data meta-analysis. Anesthesiology 123, 66–78. doi: 10.1097/ALN.0000000000000706
Simonis, F. D., Serpa Neto, A., Binnekade, J. M., Braber, A., Bruin, K. C. M., Determann, R. M., et al. (2018). Effect of a low vs intermediate tidal volume strategy on ventilator-free days in intensive care unit patients without ARDS. J. Am. Med. Assoc. 320, 1872–1880. doi: 10.1001/jama.2018.14280
Stevens, R. D., Lazaridis, C., and Chalela, J. A. (2008). The role of mechanical ventilation in acute brain injury. Neurol. Clin. 26, 543–563. doi: 10.1016/j.ncl.2008.03.014
Stocchetti, N., Picetti, E., Berardino, M., Buki, A., Chesnut, R. M., Fountas, K. N., et al. (2014). Clinical applications of intracranial pressure monitoring in traumatic brain injury : report of the Milan consensus conference. Acta Neurochir. 156, 1615–1622. doi: 10.1007/s00701-014-2127-4
Sutherasan, Y., Vargas, M., and Pelosi, P. (2014). Protective mechanical ventilation in the non-injured lung: review and meta-analysis. Crit. Care. 18:211. doi: 10.1186/cc13778
Tejerina, E. E., Pelosi, P., Robba, C., Peñuelas, O., Muriel, A., Barrios, D., et al. (2021). Evolution over time of ventilatory management and outcome of patients with neurologic disease. Crit. Care Med. 49, 1095–1106. doi: 10.1097/CCM.0000000000004921
von Elm, E., Altman, D. G., Egger, M., Pocock, S. J., Gøtzsche, P. C., and Vandenbroucke, J. P. (2014). The strengthening the reporting of observational studies in epidemiology (STROBE) statement: guidelines for reporting observational studies. Int. J. Surg. 12, 1495–1499. doi: 10.1016/j.ijsu.2014.07.013
Keywords: positive end expiratory pressure, intracranial pressure, brain injured patients, quantitative computed tomography, mechanical ventilation
Citation: Robba C, Ball L, Nogas S, Battaglini D, Messina A, Brunetti I, Minetti G, Castellan L, Rocco PRM and Pelosi P (2021) Effects of Positive End-Expiratory Pressure on Lung Recruitment, Respiratory Mechanics, and Intracranial Pressure in Mechanically Ventilated Brain-Injured Patients. Front. Physiol. 12:711273. doi: 10.3389/fphys.2021.711273
Received: 18 May 2021; Accepted: 09 September 2021;
Published: 18 October 2021.
Edited by:
Christopher G. Wilson, Loma Linda University, United StatesReviewed by:
Sophie Lalande, University of Texas at Austin, United StatesGabriele Valli, Azienda Ospedaliera San Giovanni Addolorata, Italy
Copyright © 2021 Robba, Ball, Nogas, Battaglini, Messina, Brunetti, Minetti, Castellan, Rocco and Pelosi. This is an open-access article distributed under the terms of the Creative Commons Attribution License (CC BY). The use, distribution or reproduction in other forums is permitted, provided the original author(s) and the copyright owner(s) are credited and that the original publication in this journal is cited, in accordance with accepted academic practice. No use, distribution or reproduction is permitted which does not comply with these terms.
*Correspondence: Chiara Robba, a2lhcm9iYmFAZ21haWwuY29t
†These authors have contributed equally to this work