- 1Laboratorio de Fisiología, Hipoxia y Función Vascular, Departamento de Ciencias Biomédicas, Facultad de Medicina, Universidad Católica del Norte, Coquimbo, Chile
- 2Laboratorio de Fisiología y Fisiopatología del Desarrollo, Programa de Fisiopatología, ICBM, Facultad de Medicina, Universidad de Chile, Santiago, Chile
- 3Centro Internacional de Estudios Andinos (INCAS), Universidad de Chile, Santiago, Chile
The authors previously demonstrated that newborn llama (NBLL) express high levels of α1 adrenergic receptors, which provide a potent vasoconstriction response when compared with newborn sheep (NBSH) gestated at sea level. However, data regarding the impact of chronic gestational hypobaric hypoxia on α-adrenergic vasoconstriction in the neonatal life has not been studied. We evaluated if gestation under chronic hypobaric hypoxia modifies α1-adrenergic vasoconstrictor function in NBLL and NBSH. We compared the vasoconstrictor response induced by potassium and α-adrenergic stimuli in isolated small femoral arteries of NBLL and NBSH gestated at high altitude (HA; 3,600 m) or low altitude (LA; 580 m). The maximal contraction (RMAX) and potency (EC50) to potassium, noradrenaline (NA), and phenylephrine (PHE) were larger in HA-NBLL than LA-NBLL. RMAX to potassium, NA, and PHE were lower in HA-NBSH when compared with LA-NBSH and potency results were similar. Competitive blockade with prazosin showed that RNLL LA/HA have a similar pA2. In contrast, NBSH had increased pA2 values in HA when compared with LA. Finally, small femoral arteries denudated or treated with LNAME in LA and HA lacked NO or endothelium participation in response to PHE stimulation. In contrast, NBSH displayed that denudation or blockade with LNAME support NO or endothelium participation in response to PHE activation. In conclusion, HA chronic hypoxia enhances α1 adrenergic receptor activity in small femoral arteries in NBLL to a higher degree than NBSH, implying a higher vasoconstriction function.
Introduction
When humans and animals are exposed to high-altitude (HA) environments, the low barometric pressure decreases the amount of oxygen in the body (Conkin and Wessel, 2008). Chronic hypobaric hypoxia conditions likely exerted a selective pressure on those species with ancestry at HA, which allowed them to develop cellular and physiologic defense strategies to live under low atmospheric PO2 (Monge and León-Velarde, 1991; Llanos et al., 2007). Camelids are dwellers of the Andean plateau that have a wide distribution between 3,500 and 5,000 m above sea level, and evidence supports an ancestry of over 2 million years in this low PO2 environment (Stanley et al., 1994). Sheep (Ovis aries) and llama (Lama glama) have been compared to evaluate how species manage to dwell at HA; the former as a paradigm of acclimatization, with only 500 years in highlands and the latter of adaptation. Both species have been compared in studies of perinatal physiology and cardiovascular adaptations to chronic hypoxia (Llanos et al., 2003, 2007; Reyes et al., 2020).
The llama is a camelid species that inhabits the Alto Andino plateau. They are often studied for their notable physiological adaptations to hypoxia (Meschia et al., 1960; Banchero and Grover, 1972; Braunitzer, 1980; Llanos et al., 2007), that is the hemoglobin mutation, allowing a higher hemoglobin oxygen affinity (Braunitzer, 1980; Moraga et al., 1996). Additionally, studies performed during fetal life show strong vasoconstriction when the fetus is submitted to acute hypoxia. This response is induced by the release of catecholamines, neuropeptide Y (NPY), and other vasoconstrictors (Giussani et al., 1996, 1999; Reyes et al., 2020). α-Adrenergic vasoconstriction is higher in sea level fetal llama than fetal sheep. It is also critical for blood flow redistribution and survival during acute hypoxia since α-adrenergic blockade elicited death in fetal llama but not in fetal sheep (Giussani et al., 1999). However, in chronically hypoxic fetal sheep, α-adrenergic peripheral vasoconstriction is also enhanced and is critical for survival (Block et al., 1984; Giussani et al., 1996, 1999). We previously demonstrated that llamas living at sea level for several generations deliver newborns with a high femoral vasoconstrictor response to acute hypoxia compared with their sheep counterparts. Also, sea level newborn llamas (NBLL) have greater femoral sensitivity and maximal contraction to norepinephrine and phenylephrine (PHE) than newborn sheep (NBSH). This sensibility was corroborated by competitive inhibition experiments that showed higher prazosin affinity in NBLL than NBSH. This indicates the presence of one high-affinity adrenoceptor, which is consistent with the greater α1B-adrenoceptor transcript expression observed in the small femoral arteries of NBLL compared with NBSH (Moraga et al., 2011). In addition, α1-adrenergic receptor activation by PHE may be counterbalanced by simultaneous NO release from the endothelium in neonatal sheep but not in neonatal llama at lowland (Moraga et al., 2011). However, the effect of chronic hypoxia on the α-adrenergic vasoconstrictor tone has not been compared between species with or without HA ancestry (Longo and Pearce, 1998; Goyal et al., 2014). We hypothesize that HA chronic hypoxia enhanced femoral α-adrenergic vascular reactivity in NBLL but not in NBSH. For this, we used pharmacological approaches to test the role of adrenergic receptors in small femoral arteries by comparing the responses of llama (NBLL) and sheep neonates (NBSH) gestated at low altitude (LA) and HA.
Materials and Methods
All the procedures of animal care, maintenance, and experimentation were performed in accordance with the UK Animals (Scientific Procedures) Act, 1986, and the American Physiological Society “Guiding Principles for Research Involving Animals and Human Beings” (American Physiological Society, 2002), and approved by Faculty of Medicine Ethics Committee of the University of Chile.
Animals
We studied eight NBSH and seven NBLL s gestated, born, and raised at the University of Chile farm, Santiago, located at 580 m above sea level; and six NBSH and six NBLL, gestated, born, and raised at Putre Research Station, International Center for Andean Studies (INCAS), University of Chile, located at 3,600 m above sea level (see Supplementary Table). The ancestry of HA maternal sheep has been estimated at over 50 generations (Herrera et al., 2007) and around 2 million of years for maternal llamas (Stanley et al., 1994). The llama and sheep mothers and their newborns were maintained with access to food and water ad libitum in an open yard. The shed time of both llamas and sheep was programmed so that all deliveries occurred in the same spring–summer season. The installed experimental station is in Putre, which is at 3,500 m. The measured values indicate a low relative humidity close to 20%, with thermal oscillations of 5°C at night and 16°C during the day in the spring–summer season. All the animals used for the study of small arteries were healthy and showed the expected postnatal growth for the altitude where the experiment was performed.
Ex Vivo Small Vessel Wire Myography
All the animals underwent euthanasia using sodium thiopentone (1 g I.V.). We dissected from deep femoral artery where small arteries of approximately 300–350 μm diameter and 1.8–2.0 mm length were cut and placed in ice-cold saline (see Supplementary Table). Arterial rings were mounted in a myograph to perform studies of isometric force (610M, Multimyograph, Danish Myotechnology, Aarhus, Denmark) by continuously recording tension using a data acquisition system connected to a computer (Powerlab/8SP; AD Instruments) (Moraga et al., 2011). The small femoral arteries were evaluated with (+E) or without (–E) endothelium. To eliminate the endothelium from the femoral arteries, a strand of human hair was gently rubbed backward and forward through the lumen of the vessel (Auer and Ward, 1998). To ensure the endothelium was denudated, we evaluated the response to the endothelium-dependent vasodilator ACh (10−5 M) in arteries, previously contracted with 10−5 M PHE. All endothelium-denudated rings lacked relaxation when exposed to the ACh bath, indicating that denudation was successful. Afterward, all small femoral arteries (+E or –E) were incubated in Krebs Ringer Bicarbonate (KRB) at 37°C and gassed (95% O2 and 5% CO2). After a period of incubation (1 h), the optimal diameter was determined for each artery by stretching the artery rings in a stepwise manner until a tension equivalent to the physiological transmural pressure was obtained (Mulvany and Halpern, 1997). In this condition, a dose of 62.5 mM of potassium chloride was added to promote a contraction dependent on the degree of stretch until it gave the maximal vascular response (Moraga et al., 2011; Castillo-Galán et al., 2016). Concentration-response curves (CRC) were performed for potassium chloride by adding 6.25–125 mM of KCl, α-adrenergic agonist NA, and α1-adrenergic agonist PHE, with concentrations ranging from 10−10 to 10−3 M as described previously (Moraga et al., 2011). The participation of endothelium in modulating the vasoconstriction mechanism was evaluated through concentration-response curves for PHE in intact (+E) and endothelium-free (–E) femoral arteries, either in the presence or the absence of the nitric oxide synthase (NOS) inhibitor L-NAME (10−5 M). The relative affinity of prazosin was determined by performing concentration response curves to NA in preincubated vessels for 30 min with prazosin (α1-adrenergic receptor blocker with doses of 10−9, 10−8 and 10−7 M). Each curve was evaluated in triplicate. We collected atleast 12–24 rings of small femoral arteries per animal to carry out the different assays. After finishing one curve, each artery was maintained at rest, with KRB, for at least 30 min, changing KRB solution every 10 min. Before initiating a new curve, contraction induced by 62.5 mM KCl was tested to evaluate the viability of rings. If maximal responses (RMAX) was lower than 80% of contraction obtained after CRC to K+, the artery was discarded.
Solutions and Reagents
KRB contained (in mM) NaCl 118.5, NaHCO3 25, KCl 4.7, KH2PO4 1.2, MgSO4 1.2, CaCl2 2.5, glucose 5.5 with a pH of 7.4. In K-KRB (125 mM K+) NaCl was replaced by an equimolar amount of KCl. Noradrenaline, PHE, L-NAME, and prazosin were obtained from Sigma Chemical Co., USA.
Analysis
Potency (EC50) and RMAX to the different vasoactive agents tested were obtained by fitting the concentration-response curves to a Boltzmann function (Prism 4.0, Graphpad) as described previously (Moraga et al., 2011). RMAX was expressed as tension (N/m) for K+ and/or as a percentage relative to a submaximal dose of 62.5 mM of KCl (expressed, %) for the adrenergic agonists. Values are normalized (%), in accord with sigmoidal shape and the model is assumed to have a standard slope with a Hill slope of 1.0 (Motulsky and Christopoulos, 2003). In this sense, potency was expressed as EC50 (the concentration at which 50% of RMAX was obtained) for K+ or as pEC50 (–logEC50) for the adrenergic agonists. The relative affinity (pA2) for prazosin was estimated through Schild analysis by plotting log (R – 1) values for individual vessels against the antagonist (prazosin) concentration (log [A]), where R is defined as a ratio of the EC50 NA plus antagonist [A] divided by EC50 NAalone (Arunlakshana and Schild, 1959). Slope analysis was performed in each curve, supporting the presence of a competitive antagonist. Then, pA2 values were considered the x-intercept to the Schild slope (Motulsky and Christopoulos, 2003).
Statistical Analysis
Data are expressed as means ± S.E.M. Two-way ANOVA for repeated measures followed by the post hoc test of Newman–Keuls or the Student's t test for unpaired data were used to compare data, as appropriate. Linear regression analysis of the Schild plot was used to estimate pA2 (intercept) values, the slope, and the correlation coefficient of the regression line (Motulsky and Christopoulos, 2003). For all comparisons, differences were considered significant when p < 0.05 (Zar, 1984).
Results
Ex vivo Small Femoral Arteries Wire Myography
Contractile Response to Potassium Chloride in Femoral Arteries of NB Llamas and Sheep of LA and HA
In the analysis of K+ CRC sensitivity, NBLL showed that HA neonates are more sensitive (EC50) than LA neonates. NBSH showed a similar potency (EC50) at LA and HA (Table 1). LA and HA NBSH potency was lower than LA and HA NBLL (p < 0.05) (Table 1; Supplementary Figure 1).
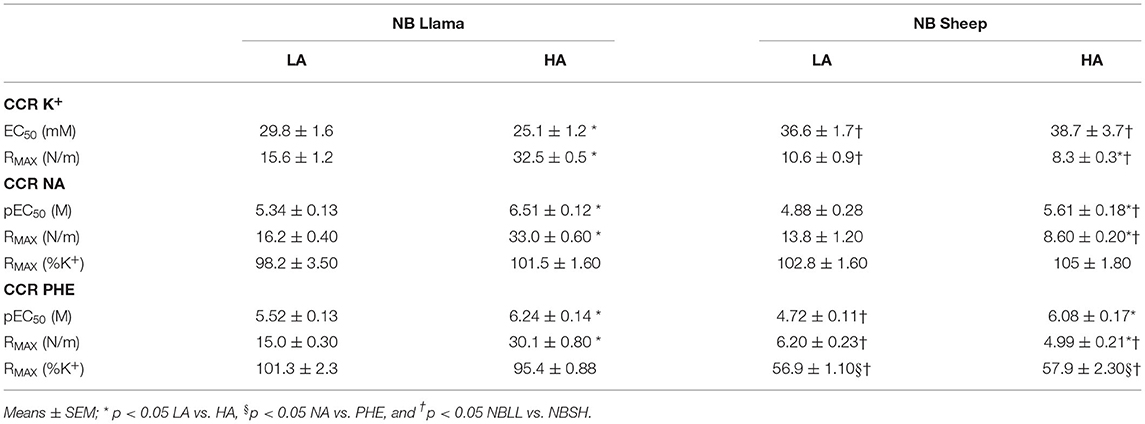
Table 1. Values of CRC in presence of potassium chloride, noradrenaline (NA), phenylephrine (PHE) in NBLL and NBSH at LA and HA.
Regarding the RMAX, LA NBLL was lower than HA NBLL (p < 0.05). In contrast with the NBLL, the LA NBSH had a greater maximal contraction than HA NBSH (p < 0.05) (Table 1). Moreover, LA and HA NBLL had a higher maximal contraction than LA and HA NBSH femoral vessels (p < 0.05) (Table 1).
Contractile Response to Adrenergic Agonists in Femoral Arteries of NB Llamas and Sheep of LA and HA
The absolute tension of HA-NBLL had a greater RMAX to both NA and PHE than their LA counterparts (p < 0.05). However, when normalized to potassium, the RMAX for NA and PHE were similar in LA- and HA-NBLL, but their corresponding pEC50 values were higher in HA- than LA-NBLL (p < 0.05) (Table 1). In NBSH, RMAX for either NA or PHE expressed as absolute values were lower in HA- than LA-animals, but they were similar for K+-normalized values, while pEC50 was larger in HA- than in LA-NBSH (Table 1). When compared, the contraction to PHE was ~57% of the tension elicited by NA in NBSH (Table 1). Additionally, we observed that pEC50 and RMAX to NA (in N/m) are similar between species at LA. However, the response to PHE of pEC50, RMAX (N/m and %K+) in NBSH is lower than NBLL. When comparing both species at HA, we observed that pEC50 and RMAX to NA (N/m and %K+) in NBSH were lower than NBLL (p < 0.05). In addition, the pEC50 in response to PHE was similar to NBLL and RMAX (N/m and %K+) in NBSH and was lower than NBLL at HA (p < 0.05) (Supplementary Figure 2).
The Role of (NOS) and Endothelium in the Vasocontractile Response to PHE in NBLL and NBSH of LA and HA
First, to probe the role of NOS we compared the CRC to PHE in the absence or the presence of NOS inhibition with L-NAME (10−5 M). In NBLL of either LA and HA, L-NAME did not modify RMAX (Figure 1A; Table 2) or pEC50 of PHE (Table 2). In contrast, in NBSH of LA and HA, NOS blockade with L-NAME promoted an RMAX of PHE similar to NA without modification in pEC50 (Figure 1B and Table 2). To examine the role of endothelium, we denudated small femoral arteries in both species and performed CRC again for PHE in the presence and the absence of L-NAME. In NBLL of either LA or HA, denudation had no effect on pEC50 or RMAX to PHE (Figure 1A and Table 2). In contrast, in NBSH of LA and HA, denudation of small femoral arteries increased the RMAX for PHE, with values similar to those in the presence of NA without modification in pEC50 (Figure 1B and Table 2). Lastly, we determined the role of NOS blockade with L-NAME (10−5 M) and arterial denudation in both species. Again, we performed CRC for PHE. In the NBLL of either LA or HA, denudation did not affect pEC50 or RMAX to PHE (Figure 1A and Table 2). NBSH of LA and HA, blocked and with denudation of small femoral arteries, increased RMAX for PHE to values similar to those observed in the presence of NA without modification in pEC50 (Figure 1B, Tables 1, 2). When comparing both species at LA, we observed that pEC50 in NBLL was higher than NBSH in all experimental conditions. However, when comparing both species at HA, values of pEC50 in NBLL were similar to NBSH (Supplementary Figure 3).
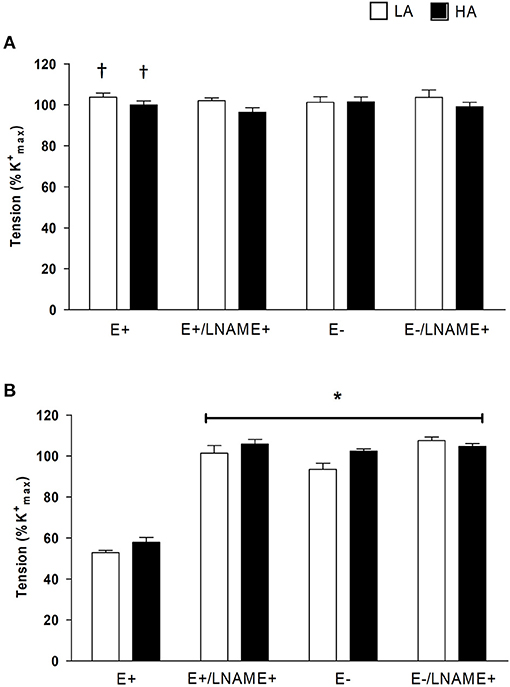
Figure 1. Effect of NG-nitro-L-arginine methyl ester L-NAME and endothelium remove (–E) on PHE-induced contraction. (A) In NBLL (n = 6) and CRC to PHE, and PHE after 30 min of incubation with 10−5 M L-NAME with endothelium (+E) and without endothelium (–E). (B) Effect of L-NAME on PHE-induced contraction in NBSH (n = 6) and CRC to PHE, and PHE after 30 min of incubation with 10−5 M L-NAME with endothelium (+E) and without endothelium (–E), where open bar represent LA and close bar represent HA. Values are expressed as means ± SEM. Asterisk (*) represent a significant difference between NBSH (LA/HA) PHE-induce contraction (+E) vs PHE-induce contraction (+E) plus L-NAME and PHE-induce contraction (–E) (p < 0.05). † Represent significant difference between NBLL vs NBSH (p < 0.05).
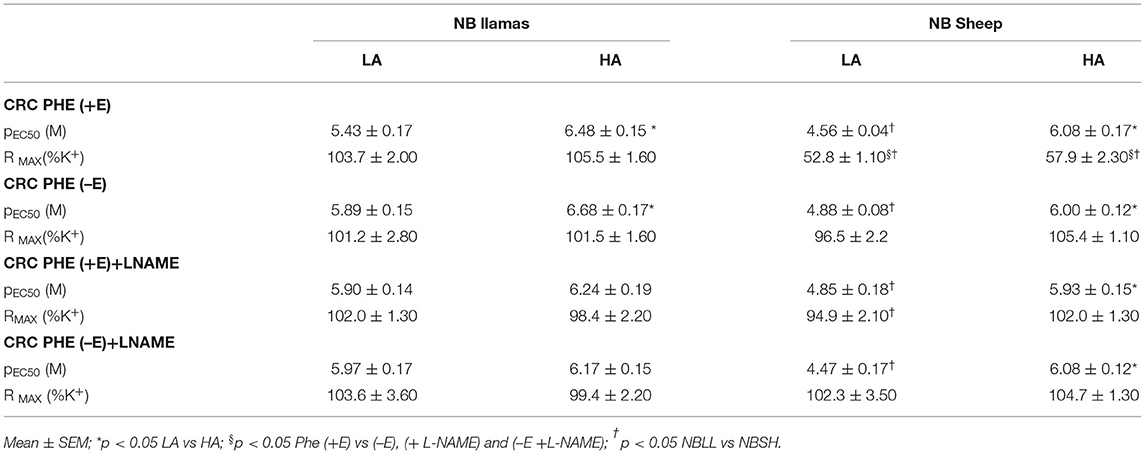
Table 2. Effect of L-NAME blocked and denudation of small femoral arteries in NB LL and NBSH at LA and HA.
Relative Affinity (pA2) to NA in Femoral Arteries in NB Llama and Sheep at LA and HA
The blockade with prazosin, a selective α1-adrenergic antagonist, promoted a concentration-dependent rightward shift of pEC50 for NA, in both LA- and HA-NBLL. This rightward shift is consistent with competitive blockade (Supplementary Figure 4). Competitive inhibition experiments modified RMAX for NA, except at the highest concentration tested in NBLL at LA compared with HA. The Schild plot analysis did not show changes in pA2 values between LA- and HA-NBLL, suggesting that the affinity of the α-adrenergic receptor for prazosin was unchanged (Figure 2A). In both LA- and HA-NBSH, prazosin blockade also promoted a rightward shift of pEC50 for NA as prazosin concentration increased. Increasing prazosin concentration did not decrease RMAX for NA except at the highest concentration tested in NBSH at HA. However, the Schild analysis showed a greater pA2 value in HA- than LA-NBSH, consistent with a greater affinity of the α-adrenergic receptor for prazosin at HA (Figure 2B). Further, when comparing both species at LA and HA, we observed that NBLL values for pA2 were greater than those obtained at LA in NBSH (p < 0.05). However, no difference was observed in NBLL and NBSH at HA.
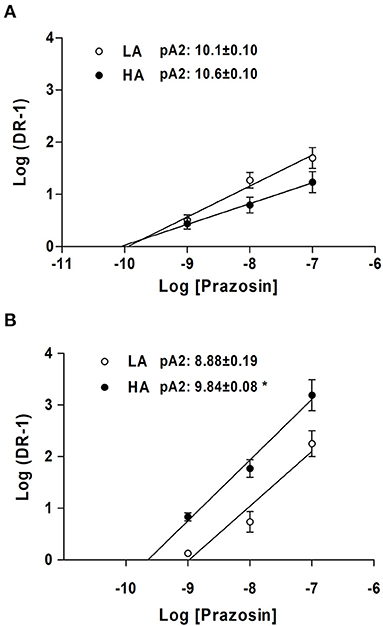
Figure 2. Relative affinity (pA2) in NBLL and NBSH of LA and HA obtained from concentration response curves to NA and NA in the presence of prazosin at 1, 10, and 100 nM and Schild plots analysis. (A) NBLL at LA vs HA and (B) NB sheep. Values are expressed as means ± SEM. Asterisk (*) represent a significant difference between NBSH (LA/HA) (p < 0.05).
Discussion
We found that HA chronic hypoxia-induced enhanced femoral α-adrenergic vascular reactivity in NBLL compared with NBSH.
The enhanced α-adrenergic vascular reactivity found in the HA NBLL is also observed in the fetal and neonatal llama at sea level (Giussani et al., 1999; Moraga et al., 2011; Reyes et al., 2020). The importance of the α-adrenergic signaling during acute hypoxia in the fetus is vital since its blockade causes cardiovascular collapse and death (Giussani et al., 1999).
Our previous work has shown that intense peripheral vasoconstriction is essential for blood flow redistribution in response to acute hypoxia in the llama fetus and that α-adrenergic signaling is necessary for eliciting this response which is critical for fetus survival (Giussani et al., 1999). As indicated, this high peripheral vasoconstrictor tone persists in the neonatal period (Reyes et al., 2018, 2020). Moreover, we described the high femoral vascular resistance either basally or under acute hypoxia in LA-NBLL compared with LA-NBSH (Moraga et al., 2011). We also described that this higher femoral vascular resistance in the LA-NBLL could be explained by a powerful α1 adrenergic tone and the preferential expression of the α1B adrenoceptor subtype. In contrast, the LA-NBSH preferentially expressed the α1A adrenoceptor (Moraga et al., 2011). Our finding of a greater RMAX to NA and PHE in HA-NBLL compared with HA-NBSH llamas are consistent with higher α1B adrenoceptor subtype levels in the former.
In the present study, we have extended our previous work and have now compared the effect of HA chronic hypoxia in the femoral vasoconstrictor response in two species, llama and the sheep, with different ancestry at HA.
Vasoconstriction Mediated by Potassium in NBLL and NBSH at LA/HA
Maximum Response to Potassium Chloride
Regarding increased RMAX for K+ observed in animals with chronic exposure like NBLL at HA, there are no previous studies published on the effect of hypobaric hypoxia on femoral vessels in the NBLL. Exposure of rats to hypoxia of 10% by 24 h and 48 h decreases the contractile response in aorta rings (Auer and Ward, 1998), while pulmonary arterial bed hypoxia promotes vasoconstriction, upregulation of voltage-dependent Ca2+ channels, and a hyperplasic/hypertrophic structural remodeling related with increased RMAX (Wan et al., 2013; Dunham-Snary et al., 2017; Reyes et al., 2018; Gassmann et al., 2021). Other mechanisms such as increased Ca2+ sensitization in vascular smooth muscle can also increase RMAX (Jernigan and Resta, 2014). To the best of our knowledge, hypoxic remodeling, an increase of Ca2+ sensitization, or upregulation of voltage-dependent Ca2+ channels have not been described in femoral vessels; nevertheless, we cannot rule out these possibilities. The mechanism involved in the increase of femoral RMAX to K+ in HA-NBLL may improve the total peripheral contraction capacity and blood flow redistribution to withstand hypoxia in this species.
In contrast, the decreased RMAX described in our study in small femoral arteries NBSH at HA is in agreement with previous observations in the same ovine neonatal model (Herrera et al., 2007) and in the carotid artery in fetal sheep (Nauli et al., 2005). Decreased sensitivity of the contractile smooth muscle machinery to Ca2+ could explain our results (Nauli et al., 2005). The highest function or expression of vasodilator mechanisms, like nitric oxide signaling, cannot be excluded in NBSH at HA (Herrera et al., 2008, 2019; Reyes et al., 2018).
Potency to Potassium (EC50)
NBLL responses increased potency at HA compared with LA. Meanwhile, NBSH showed similar potency values at LA and HA. However, there was a larger potassium potency (low values of EC50) in NBLL at LA or HA than NBSH at LA and HA. In agreement with Nernst Equation, the lower EC50 values to K+ described in NBLL means that it can activate a vasocontraction at a lower membrane potential (see Papamatheakis et al., 2012). This suggests that NBLL, as a species, adapted to chronic HA hypoxia over millions of years, has developed a lower threshold for calcium channel type-L activation (Ghosh et al., 2017; Ottolini et al., 2019).
Vasoconstriction Mediated by α1-Adrenoreceptors in NBLL and NBSH at LA/HA
NBLL at LA and HA
Our study depicted the outcomes of chronic exposure at HA on vasoconstriction mechanisms mediated by α1-adrenoceptors in NBLL compared with LA-NBLL. Vasocontraction mediated by NAor PHE in NBLL are similar to those reported previously in NBLL at LA (Moraga et al., 2011). We observed a similar pattern in NBLL at HA, resulting in increased vasocontraction when HA-NBLL was compared with LA-NBLL. Furthermore, an increased pEC50 was described in NBLL at HA. We did not observe any potentiation in the PHE vasoconstrictor effect using L-NAME or femoral artery denudation in LA- or HA-NBLL. This evidence supports the presence of α1−adrenoceptors in small femoral arteries as the dominant mechanism accounting for the powerful vasoconstrictor tone in NBLL from HA or LA.
To characterize the α1-adrenoceptor we performed competitive inhibition with increased doses of prazosin followed by CRC to NA. Schild analysis performed with NBLL showed similar pA2 value for both LA- and HA-NBLL, indicating the presence of one α1−adrenoceptor subtype under both conditions. In addition, pA2 > 9 value, which suggests the presence of a receptor with a high affinity for prazosin (Graham et al., 1996; Moraga et al., 2011; Docherty, 2019). Nevertheless, competitive blockade was performed using prazosin, a specific α1-adrenoceptor blocker. Therefore, the difference observed in potency to NA and PHE at HA could be explained by the expression of other α1-adrenoceptor subtypes. NBLL at LA have preferential expression of the α1B-adrenoceptor subtype over the α1A-adrenoceptor subtype transcript (Moraga et al., 2011). However, further studies using specific subtype α1-adrenoceptor blockers are required to evaluate subtype expression in NBLL at HA appropriately.
NBSH at LA/HA
RMAX and pEC50 values for the α-adrenergic agonist (NA and PHE) described in small femoral arteries in the present work are similar to values reported in NBSH at LA (Herrera et al., 2008; Moraga et al., 2011). However, NBSH exposed to HA showed increased potency and reduced RMAX to NA and PHE. The RMAX values in response to PHE in NA at LA and HA show the same tendency to previously described results in NBSH at LA (Moraga et al., 2011). We can discard the vasoconstrictor role of α2−adrenoceptor since clonidine does not promote vasoconstriction in small femoral arteries nor at LA or HA in the present study (Moraga et al., 2011). To demonstrate that α1-adrenoceptor could promote NO production by attenuating vasoconstriction at close to 50%, we studied the contraction under NOS inhibition (L-NAME 10−5 M) or after removing the endothelium, both conditions permit 100% vasoconstriction recovery with PHE, similar to that observed with NA in NBSH at LA and HA. A similar pattern was described in the bronchial resistance artery in rabbits (Zschauer et al., 1997) and mesenteric resistances artery in rats (Dora et al., 2000). The proposed mechanism suggests that PHE promotes α1-adrenoceptor activation in endothelium, increasing NO production that opposes vasoconstriction (Tuttle and Falcone, 2001; Raj and Subramani, 2016; Marconi et al., 2020). This result suggests different subtypes of α1-adrenoceptor with a specific location in the small femoral artery, one type of α1-adrenoceptor present in the endothelium, and other α1-adrenoceptors present in smooth muscle cells, as proposed by Moraga et al. (2011).
To characterize the α1-adrenoceptor, we performed competitive inhibition experiments with increasing doses of prazosin followed by CRC to NA. Schild analysis performed to NBSH showed two different pA2 values, suggesting a low-affinity receptor in LA-NBSH, similar to previous reports (Moraga et al., 2011) and according to the nomenclature proposed by Graham et al. (1996) and Docherty (2019). Also, the pA2 > 9 value suggests the presence of a receptor with a high affinity for prazosin in HA-NBSH (Graham et al., 1996; Moraga et al., 2011; Docherty, 2019). These outcomes indicate the presence of two α1−adrenoceptor subtypes in small femoral arteries at LA and HA-NBSH. Nowadays, low-affinity phenotype has been associated with the subtype α1A adrenoceptor (Docherty, 2019). Accordingly, we previously demonstrated that NBSH at LA preferentially expresses subtype α1A-adrenoceptor (Moraga et al., 2011).
In conclusion, the ex vivo results obtained from small femoral arteries of NBLL and NBSH gestated at HA showed that the physiology underlying this difference between species is due to the following (1) enhanced vasoconstrictor reactivity and potency to α-adrenergic agonists in NBLL; (2) an important role of NO and endothelium in NBSH in the modulation of vasoconstriction induced by α1-adrenergic stimuli, and (3) maintenance in pA2 in NBLL at LA/HA. In NBSH, increased pA2 was observed at HA respect LA.
Data Availability Statement
The original contributions presented in the study are included in the article/Supplementary Material, further inquiries can be directed to the corresponding author.
Ethics Statement
The animal study was reviewed and approved by Faculty of Medicine University of Chile.
Author Contributions
FM, RR, and AL conceived and designed the study. FM, GE, and VL supervised the overall study. FM and GE contributed to sample data collections and statistical analysis. All authors drafted the report and contributed to the interpretation of the results, critical revision of the manuscript, and approval of the final manuscript. FM is the guarantor.
Funding
This work was supported by National Fund for Scientific and Technological Development (FONDECYT), Grants 1090355, 1080663, 105047, and 1140647.
Conflict of Interest
The authors declare that the research was conducted in the absence of any commercial or financial relationships that could be construed as a potential conflict of interest.
Publisher's Note
All claims expressed in this article are solely those of the authors and do not necessarily represent those of their affiliated organizations, or those of the publisher, the editors and the reviewers. Any product that may be evaluated in this article, or claim that may be made by its manufacturer, is not guaranteed or endorsed by the publisher.
Acknowledgments
The authors thank Dr. César E. Ulloa for help reading and editing this paper and to Carlos Brito for technical assistance.
Supplementary Material
The Supplementary Material for this article can be found online at: https://www.frontiersin.org/articles/10.3389/fphys.2021.697211/full#supplementary-material
References
American Physiological Society (2002). Guiding principles for research involving animals and human beings. Am. J. Physiol. Regul. Integr. Comp. Physiol. 283, R281–R283. doi: 10.1152/ajpregu.00279.2002
Arunlakshana, O., and Schild, H. O. (1959). Some quantitative uses of drug antagonists. Br. J. Pharmacol. 14:48–58. doi: 10.1111/j.1476-5381.1959.tb00928.x
Auer, G., and Ward, M. E. (1998). Impaired reactivity of rat aorta to phenylephrine and KCl after prolonged hypoxia: role of the endothelium. J. Appl. Physiol. 85, 411–417. doi: 10.1152/jappl.1998.85.2.411
Banchero, N., and Grover, R. F. (1972). Effect of different levels of simulated altitude on O2 transport in llama and sheep. Am. J. Physiol. 222, 1239–1245. doi: 10.1152/ajplegacy.1972.222.5.1239
Block, B. S., Llanos, A. J., and Creasy, R. K. (1984). Responses of the growth-retarded fetus to acute hypoxemia. Am. J. Obstet. Gynecol. 148, 878–885. doi: 10.1016/0002-9378(84)90529-5
Braunitzer, G. (1980). Phosphate-hemoglobin interaction: concerning the respiration of adult man, human fetus, the llama and dromedary. Klin. Wochenschr. 58, 701–708. doi: 10.1007/BF01478457
Castillo-Galán, S., Quezada, S., Moraga, F. A., Ebensperger, G., Herrera, E. A., Beñaldo, F., et al. (2016). 2-Aminoethyldiphenylborinate modifies the pulmonary circulation in pulmonary hypertensive newborn lambs with partial gestation at high altitude. Am. J. Physiol. Lung Cell. Mol. Physiol. 311, L788–L799. doi: 10.1152/ajplung.00230.2016
Conkin, J, and Wessel, J. H. 3rd. (2008). Critique of the equivalent air altitude model. Aviat. Space Environ. Med. 79, 975–982. doi: 10.3357/ASEM.2331.2008
Docherty, J. R. (2019). The pharmacology of α1-adrenoceptor subtypes. Eur. J. Pharmacol. 855, 305–320. doi: 10.1016/j.ejphar.2019.04.047
Dora, K. A., Hinton, J. M., Walker, S. D., and Garland, C. J. (2000). An indirect in?uence of phenylephrine on the release of endothelium-derived vasodilators in rat small mesenteric artery. Br. J. Pharmacol. 129, 381–387. doi: 10.1038/sj.bjp.0703052
Dunham-Snary, K. J., Wu, D., Sykes, E. A., Thakrar, A., Parlow, L. R. G., Jeffrey, D., Mewburn, J. D., et al. (2017). Hypoxic pulmonary vasoconstriction. From molecular mechanisms to medicine. Chest 151, 181–192. doi: 10.1016/j.chest.2016.09.001
Gassmann, M., Cowburn, A., Gu, H., Li, J., Rodriguez, M., Babicheva, A., et al. (2021). Hypoxia-induced pulmonary hypertension—utilising experiments of nature. Br. J. Pharmacol. 178, 121–131. doi: 10.1111/bph.15144
Ghosh, D., Syed, A. U., Prada, M. P., Nystoriak, M. A., Santana, L. F., Nieves-Cintrón, M., et al. (2017). Calcium channels in vascular smooth muscle. Adv. Pharmacol. 78, 49–87. doi: 10.1016/bs.apha.2016.08.002
Giussani, D. A., Riquelme, R. A., Hanson, M. A., Blanco, C. E., Edwards, C. M. B., and Llanos, A. J. (1999). Pronounced increase in plasma neuropeptide Y (NPY) during acute hypoxemia in the llama fetus during late gestation. J. Soc. Gynecol. Invest. 6:106, Abstract.
Giussani, D. A., Riquelme, R. A., Moraga, F. A., McGarrigle, H. H. G., Gaete, C. R, Sanhueza, E. M., et al. (1996). Chemore?ex and endocrine components of cardiovascular responses to acute hypoxemia in the llama fetus. Am. J. Physiol. Regul. Integr. Comp. Physiol. 271, R73–R83. doi: 10.1152/ajpregu.1996.271.1.R73
Goyal, R., Goyal, D., Chu, N., Van Wickle, J., and Longo, L. D. (2014). Cerebral artery alpha-1 AR subtypes: high altitude long-term acclimatization responses. PLoS ONE. 9:e112784. doi: 10.1371/journal.pone.0112784
Graham, R. M., Perez, D. M., Hwa, J., and Piascik, M. T. (1996). alpha 1-adrenergic receptor subtypes. Molecular structure, function, and signaling. Circ. Res. 78, 737–749. doi: 10.1161/01.RES.78.5.737
Herrera, E. A., Ebensperger, G., Hernández, I., Sanhueza, E. M., Llanos, A. J., and Reyes, R. V. (2019). The role of nitric oxide signaling in pulmonary circulation of high- and low-altitude newborn sheep under basal and acute hypoxic conditions. Nitric Oxide 89, 71–80. doi: 10.1016/j.niox.2019.05.003
Herrera, E. A., Pulgar, V. M., Riquelme, R. A., Sanhueza, E. M., Reyes, R. V., Ebensperger, G., et al. (2007). High-altitude chronic hypoxia during gestation and after birth modifies cardiovascular responses in newborn sheep. Am. J. Physiol. Regul. Integr. Comp. Physiol. 292, R2234–R2240. doi: 10.1152/ajpregu.00909.2006
Herrera, E. A., Reyes, R. V., Giussani, D. A., Riquelme, R. A., Sanhueza, E. M., Ebensperger, G., et al. (2008). Carbon monoxide: a novel pulmonary artery vasodilator in neonatal llamas of the Andean altiplano. Cardiovasc. Res. 77, 197–201. doi: 10.1093/cvr/cvm013
Jernigan, N. L., and Resta, T. C. (2014). Calcium homeostasis and sensitization in pulmonary arterial smooth muscle. Microcirculation 21, 259–271. doi: 10.1111/micc.12096
Llanos, A. J., Riquelme, R. A., Herrera, E. A., Ebensperger, G., Krause, B., Reyes, R. V., et al. (2007). Evolving in thin air-lessons from the llama fetus in the altiplano. Respir. Physiol. Neurobiol. 158, 298–306. doi: 10.1016/j.resp.2007.04.012
Llanos, A. J., Riquelme, R. A., Sanhueza, E. M., Hanson, M. A., Blanco, C. E., Parer, J. T., et al. (2003). The fetal llama versus the fetal sheep: different strategies to withstand hypoxia. High Alt. Med. Biol. 4, 193–202. doi: 10.1089/152702903322022794
Longo, L. D., and Pearce, W. J. (1998). High altitude, hypoxic-induced modulation of noradrenergic-mediated responses in fetal and adult cerebral arteries. Comp. Biochem. Physiol. A Mol. Integr. Physiol. 119, 683–694. doi: 10.1016/S1095-6433(98)01006-X
Marconi, K. P., Bharathi, B., Venis, A. M., Raj, R., Amirtham, S. M., and Subramani, S. (2020). Phenylephrine induces relaxation of longitudinal strips from small arteries of goat legs. PLoS ONE 15:e0227316. doi: 10.1371/journal.pone.0227316
Meschia, G., Prytowsky, H., Hellegers, A., Huckabee, W., Metcalfe, J., and Barron, D. H. (1960). Observations on the oxygen supply to the fetal llama. Quart. J. Exp. Physiol. 45, 284–291. doi: 10.1113/expphysiol.1960.sp001473
Monge, C., and León-Velarde, F. (1991). Physiological adaptation to high altitude: oxygen transport in mammals and birds. Physiol. Rev. 71, 1135–1172. doi: 10.1152/physrev.1991.71.4.1135
Moraga, F., Monge, C., Riquelme, R., and Llanos, A. J. (1996). Fetal and maternal blood oxygen affinity: a comparative study in llamas and sheep. Comp. Biochem. Physiol. A Physiol. 115, 111–115. doi: 10.1016/0300-9629(96)00016-3
Moraga, F. A., Reyes, R. V., Herrera, E. A., Riquelme, R. A., Ebensperger, G., Pulgar, V. M., et al. (2011). Role of the α-adrenergic system in femoral vascular reactivity in neonatal llamas and sheep: a comparative study between highland and lowland species. Am. J. Physiol. Regul. Integr. Comp. Physiol. 301, R1153–R1160. doi: 10.1152/ajpregu.00124.2010
Motulsky, H. J., and Christopoulos, A. (2003). Fitting dose response curves. Fitting Models to Biological Data Using Linear and Nonlinear Regression. A Practical Guide To Curve Fitting. San Diego, CA: GraphPad Prism Software, 256–295.
Mulvany, M. J., and Halpern, W. (1997). Contractile properties of small arterial resistance vessels in spontaneously hypertensive and normotensive rats. Circ. Res. 41, 19–26. doi: 10.1161/01.RES.41.1.19
Nauli, S. M., Williams, J. M., Gerthoffer, W. T., and Pearce, W. J. (2005). Chronic hypoxia modulates relations among calcium, myosin light chain phosphorylation, and force differently in fetal and adult ovine basilar arteries. J. Appl. Physiol. 99, 120–127. doi: 10.1152/japplphysiol.01131.2004
Ottolini, M., Hong, K., and Sonkusare, S. K. (2019). Calcium signals that determine vascular resistance. Wiley Interdiscip. Rev. Syst. Biol. Med. 11:e1448. doi: 10.1002/wsbm.1448
Papamatheakis, D. G., Patel, J. J., Blood, Q., Merritt, T. T., Longo, L. D., and Wilson, S. M. (2012). Depolarization-dependent contraction increase after birth and preservation following long-term hypoxia in sheep pulmonary arteries. Pulmonary Circ. 2, 41–53. doi: 10.4103/2045-8932.94832
Raj, R. R., and Subramani, S. (2016). Phenylephrine decreases vascular tension in goat arteries in specific circumstances. PLoS ONE. 11:e0158551. doi: 10.1371/journal.pone.0158551
Reyes, R. V., Díaz, M., Ebensperger, G., Herrera, E. A., Quezada, S. A., Hernandez, I., et al. (2018). The role of nitric oxide in the cardiopulmonary response to hypoxia in highland and lowland newborn llamas. J. Physiol. 596, 5907–5923. doi: 10.1113/JP274340
Reyes, R. V., Herrera, E. A., Ebensperger, G., Sanhueza, E. M., Giussani, D. A., and Llanos, A. J. (2020). Perinatal cardiopulmonary adaptation to the thin air of the Alto Andino by a native Altiplano dweller, the llama. J. Appl. Physiol. 129, 152–161. doi: 10.1152/japplphysiol.00800.2019
Stanley, H., Kadwell, M., and Wheeler, J. C. (1994). Molecular evolution of the family Camelidae: a mitochondrial DNA study. Proc. Biol. Sci. 256, 1–6. doi: 10.1098/rspb.1994.0041
Tuttle, J. L., and Falcone, J. C. (2001). Nitric oxide release during α1-adrenoceptor mediated constriction of arterioles. Am. J. Physiol. Heart Circ. Physiol. 281, H873–H881. doi: 10.1152/ajpheart.2001.281.2.H873
Wan, J., Yamamura, A., Zimnicka, A. M., Voirot, G., Smith, K. A., Tang, H., et al. (2013). Chronic hypoxia selectively enhances L- and T-type voltage-dependent Ca2+ channel activity in pulmonary artery by upregulating Ca1.2 and Ca3.2. Am. J. Physiol. Lung Cell Mol. Physiol. 305, L154–L164. doi: 10.1152/ajplung.00313.2012
Keywords: newborn llama, newborn sheep, sea level, chronic hypoxia, femoral vasoconstriction, noradrenaline, phenylephrine
Citation: Moraga FA, Reyes RV, Ebensperger G, López V and Llanos AJ (2021) Enhanced Vasoconstriction Mediated by α1-Adrenergic Mechanisms in Small Femoral Arteries in Newborn Llama and Sheep Gestated at Low and High Altitudes. Front. Physiol. 12:697211. doi: 10.3389/fphys.2021.697211
Received: 19 April 2021; Accepted: 28 June 2021;
Published: 04 August 2021.
Edited by:
Francisco C. Villafuerte, Universidad Peruana Cayetano Heredia, PeruReviewed by:
Luciana Venturini Rossoni, University of São Paulo, BrazilJulio Alcayaga, University of Chile, Chile
Copyright © 2021 Moraga, Reyes, Ebensperger, López and Llanos. This is an open-access article distributed under the terms of the Creative Commons Attribution License (CC BY). The use, distribution or reproduction in other forums is permitted, provided the original author(s) and the copyright owner(s) are credited and that the original publication in this journal is cited, in accordance with accepted academic practice. No use, distribution or reproduction is permitted which does not comply with these terms.
*Correspondence: Fernando A. Moraga, fmoraga@ucn.cl