- 1Department of Zoology, Faculty of Science, Assiut University, Assiut, Egypt
- 2Department of Zoology, Faculty of Science, Al Azhar University (Assiut Branch), Assiut, Egypt
- 3Department of Zoology, Faculty of Science, Sohag University, Sohag, Egypt
The current study aims at evaluating the toxicity of hydroxychloroquine (HCQ) as a pharmaceutical residue in catfish (Clarias gariepinus) and the protective role of Spirulina platensis (SP). Four groups were used in this study: (1) a control group, (2) a group exposed to 3.16 mg/l of HCQ, (3) a group exposed to 3.16 mg/l of HCQ + 10 mg/l of SP, and (4) a group exposed to 3.16 mg/l of HCQ + 20 mg/l of SP for 15 days of exposure. The HCQ-treated group showed a significant decline in the hematological indices and glucose, total protein, and antioxidant levels in relation to the control group, whereas the HCQ-treated group showed a significant increase in the levels of creatinine, uric acid, aspartate aminotransferase (AST), and alanine aminotransferase (ALT) as well as the percentage of poikilocytosis and nuclear abnormalities of RBCs in relation to the control group. The histopathological evaluation of the liver indicated dilation of the central vein, vacuolization, degeneration of hepatocytes and pyknotic nuclei, as well as reduction of glomeruli, dilation of Bowman’s space, and degeneration of renal tubules in the kidney of the HCQ-treated group. Spirulina platensis (SP) rendered the hematological and biochemical indexes as well as antioxidant levels and the histological architecture to normal status in a dose-dependent manner. Accordingly, the current study recommends the use of SP to remedy the toxic effects of HCQ.
Highlights
- Hydroxychloroquine (HCQ) induced changes in biochemical and antioxidant parameters.
- Hydroxychloroquine (HCQ) prompted abnormalities in erythron profile of catfish
- Hydroxychloroquine (HCQ) induces damages in the histopathological structure of catfish
- Spirulina supplementation protects against Hydroxychloroquine (HCQ) deleterious effects.
Introduction
The increasing global use of pharmaceutical and personal-care products is leading to the increasing contamination of surface water and groundwater, which raises a significant ecotoxicological concern (Schwarzenbach et al., 2006; Hughes et al., 2013; Margot et al., 2015). Conventional wastewater treatment plants (WWTPs) are designed to remove solids, suspended solids, and easily (bio-)degradable organic material. Consequently, many of these pharmaceutical products are only partially removed in current WWTPs (Lindroos et al., 2019). This problem is especially significant in developing countries, where untreated effluents from the hospitals and homes result in the release of high quantities into the environment (Larsson et al., 2007; Carlsson et al., 2009; Rana et al., 2017).
Hydroxychloroquine (HCQ) is used in the treatment protocols of malaria and various inflammatory diseases like rheumatoid arthritis and systemic lupus erythematosus (Borba et al., 2004), as well as management of a number of viral diseases, such as influenza A/H5N1, SARS-CoV, and HIV (Takano et al., 2013). Recently, national and international medical organizations over the world allowed the treatment of Coronavirus (COVID-19) in certain hospitalized patients with chloroquine and HCQ. This certainly results in the discharge of large quantities of wastewaters contaminated with HCQ into the environment (Bensalah et al., 2020). The behavior and fate of pharmaceutical HCQ in the aquatic environment are significantly unexplored (Kumar et al., 2021).
HCQ has a serious chronic threat to the aquatic environment as the drug belongs to a group of quinolone derivatives that are recalcitrant, toxic, persistent, teratogenic, and carcinogenic for the aquatic organisms (Ramesh et al., 2018). Although the mechanism of chloroquine or HCQ toxic effects has not been precisely identified yet, some researchers are pointing at the formation of reactive oxygen species (El Shishtawy et al., 2013).
Spirulina is a freshwater microscopic single-cell microalga (Sharoud, 2015) that contains antioxidant enzymes with the ability to downregulate free radical generation (Seshadri et al., 1991). Furthermore, Spirulina platensis (SP) has been verified to protect against the toxicity of some known drugs, such as paracetamol (Sharoud, 2015), D-galactosamine, acetaminophen (Sabina et al., 2009; Lu et al., 2010; Zeenat et al., 2017), methotrexate (Khafaga and El-Sayed, 2018), aspirin (Mahmoud and Abd El-Ghffar, 2019), isoniazid, and rifampicin (Martin and Sabina, 2016). However, little is known about the potential protective mechanisms of Spirulina against HCQ toxicity in fish and other animals.
Catfish (Clarias gariepinus) is used in toxicology and bioremediation research due to its high growth rate, and hardiness, including its high resistance to handling and stress (Sayed and Soliman, 2018). The current study aims to evaluate the toxicity of HCQ as pharmaceutical residue in catfish (Clarias gariepinus) and the protective role of SP.
Materials and Methods
Chemicals
HCQ [Plaquenil, white, round, film-coated 200-mg tablets, Sanofi®] was obtained from a drug store. A stock solution of HCQ was prepared (3,000 mg/l deionized water).
SP tablets were bought from Japan Algae Co., Ltd., Spirulina contains proteins, sugariness, fatty acids, and vitamins, as well as plenty of minerals, such as calcium, magnesium, iron, and zinc. Spirulina tablets dissolved in water, and their bioactive ingredients became available in the water column for fish to absorb via the gastrointestinal canal.
Fish Exposure
The fish Clarias gariepinus (weight of 478.85 ± 3.03 g and a mean length of 32 ± 0.3 cm, n = 96, male) were obtained from a fish farm at Assiut University and transported to the Fish Biology and Pollution Laboratory, Assiut University. They were healthy and parasite-free according to AFS-FHS (2004). The fish were kept in ≈200-l glass tanks (92 cm × 46 cm × 46 cm) containing 100 l dechlorinated tap water and air pumps under laboratory circumstances for 4 weeks for acclimatization. The physicochemical properties of the test water were recorded as follows: conductivity 260.8 μM cm–1, pH 7.56, dissolved oxygen 6.9 mg l–1, temperature 29.5°C, and photoperiod 12:12 light:dark. Four groups (24 fish/group) were assigned in three replicates for each treatment group (eight fish/glass aquarium according to Cash et al., 2016) during the experimental period. The first group was a control group; the second group were exposed to 3.16 mg/l of HCQ according to Ramesh et al. (2018)—this concentration is lower than LC50 > 100 mg/l according to SANOFI (2020); the third group was exposed to 3.16 mg/l of HCQ + 10 mg/l of SP; and the fourth group was exposed to 3.16 mg/l of HCQ + 20 mg/l of SP for 15 days. After the exposure, six fish per replicate from each group were randomly selected and anesthetized with ice (Hamed et al., 2019a). Blood samples (1.5 ml) were collected from the caudal vein, one part for the erythron profile and hematological indices, and the other part centrifuged under cooling for biochemical and antioxidant biomarkers. Liver and kidney tissues were used for histopathological studies. The experimental setup, guidelines, and fish handling were approved by the Research and Ethical Committee of the Faculty of Science, Assiut University, Assiut, Egypt.
Hemato-Biochemical Parameters
The hematological parameters, i.e., white blood cell count (WBC), red blood cell count (RBC), thrombocytes, hemoglobin (Hb), hematocrit (HCT), mean corpuscular hemoglobin (MCH), mean corpuscular volume (MCV), and mean corpuscular hemoglobin concentration (MCHC), were performed according to Fazio et al. (2014). Likewise, biochemical parameters, i.e., uric acid, creatinine, glucose, ALT, AST, and total protein, were assessed as described by Hamed et al. (2019a) using a spectrophotometer (Jasco V-530, Ottawa, Canada).
Measurement of Antioxidant Biomarkers
Superoxide dismutase (SOD) was measured based on its ability to inhibit the phenazine methosulfate-mediated reduction of nitroblue tetrazolium dye to form a red product (Nishikimi et al., 1972). Catalase (CAT) was determined based on the fact that 3,5-dichloro-2-hydroxybenzene sulfonic acid could rapidly terminate the degradation reaction of hydrogen peroxide catalyzed by CAT and react with the residual hydrogen peroxide to generate a yellow product (Aebi, 1984). The TAC assay measured the capacity of the biological fluids to inhibit the production of thiobarbituric acid reactive substances (TBARS) from sodium benzoate under the influence of the free oxygen radicals derived from Fenton’s reaction. A solution of 1 mmol/l uric acid was used as standard (Koracevic et al., 2001).
Erythron Profile (Poikilocytosis and Nuclear Abnormalities of RBCs)
Blood smears were prepared, stained with hematoxylin–eosin, selected, coded, randomized, and scored blindly for erythrocyte alterations and nuclear abnormalities following the criteria of Schmid (1975) and Al-Sabti and Metcalfe (1995).
Histopathological Studies
The liver and kidney samples were taken and then fixed in 10% neutral buffered formalin. Fixed samples were processed routinely using a paraffin embedding technique, then sectioned at 5 μm in thickness and stained with Harris’ hematoxylin and eosin (H&E). Sections were examined using an Olympus microscope model BX50F4 (Olympus Optical Co., Ltd., Tokyo, Japan).
Statistical Analysis
Data were analyzed using the SPSS package (SPSS, 1998), and 0.05 was considered the point of significance. Data were then tested for normality (Shapiro–Wilk test). Then, the homogeneity of variances was tested (Levene’s test) following the one-way analysis of variance (ANOVA). Fisher’s LSD post hoc test was used in case of variance equality to compare the treated groups in relation to the control group. Dunnett’s post hoc test was used in case of variance inequality to compare the treated groups in relation to the control group.
Results
Hematological Parameters
The hematological indices showed a significant decrease (P < 0.05) after exposure to 3.16 mg/l of HCQ for 15 days in comparison with the control group, whereas MCV, MCHC, and MCH showed a non-significant increase (P < 0.05) after exposure to 3.16 mg/l of HCQ for 15 days. Co-treatment with Spirulina significantly improved the hematological indices which were decreased by HCQ in a dose-dependent manner (Table 1).
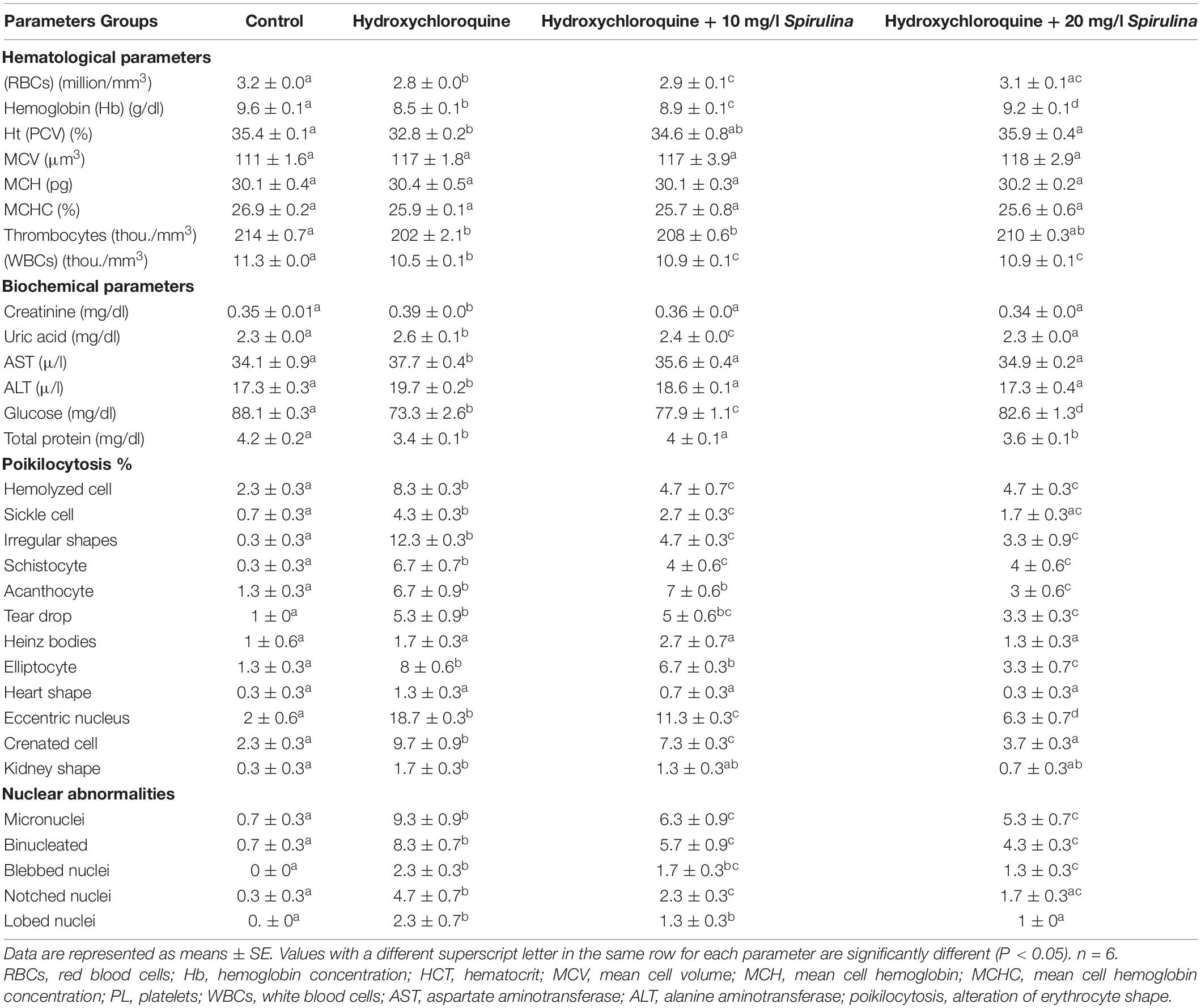
Table 1. Hematological, biochemical parameters, percentage of poikilocytosis, and nuclear abnormalities of the African catfish (Clarias gariepinus) after hydroxychloroquine (HCQ) exposure for 15 days and treatment with SP (in 10 and 20 mg l–1 water).
Biochemical Parameters
Kidney functions, i.e., uric acid and creatinine, as well as liver functions, i.e., AST and ALT, showed a significant increase (P < 0.05) after exposure to 3.16 mg/l of HCQ for 15 days compared to the control group, whereas other biochemical parameters, like total protein and glucose, showed a significant decrease (P < 0.05) after exposure to 3.16 mg/l of HCQ for 15 days compared to the control group (Table 1). Co-treatment with Spirulina significantly rendered these biochemical parameters to their normal levels induced by HCQ in a dose-dependent manner (Table 1).
Antioxidant Enzymes
The levels of antioxidant biomarkers, i.e., TAC, CAT, and SOD, were significantly depleted in the catfish treated with HCQ. Co-treatment with Spirulina significantly increased the levels of antioxidants in HCQ-treated groups in a dose-dependent manner (Figure 1).
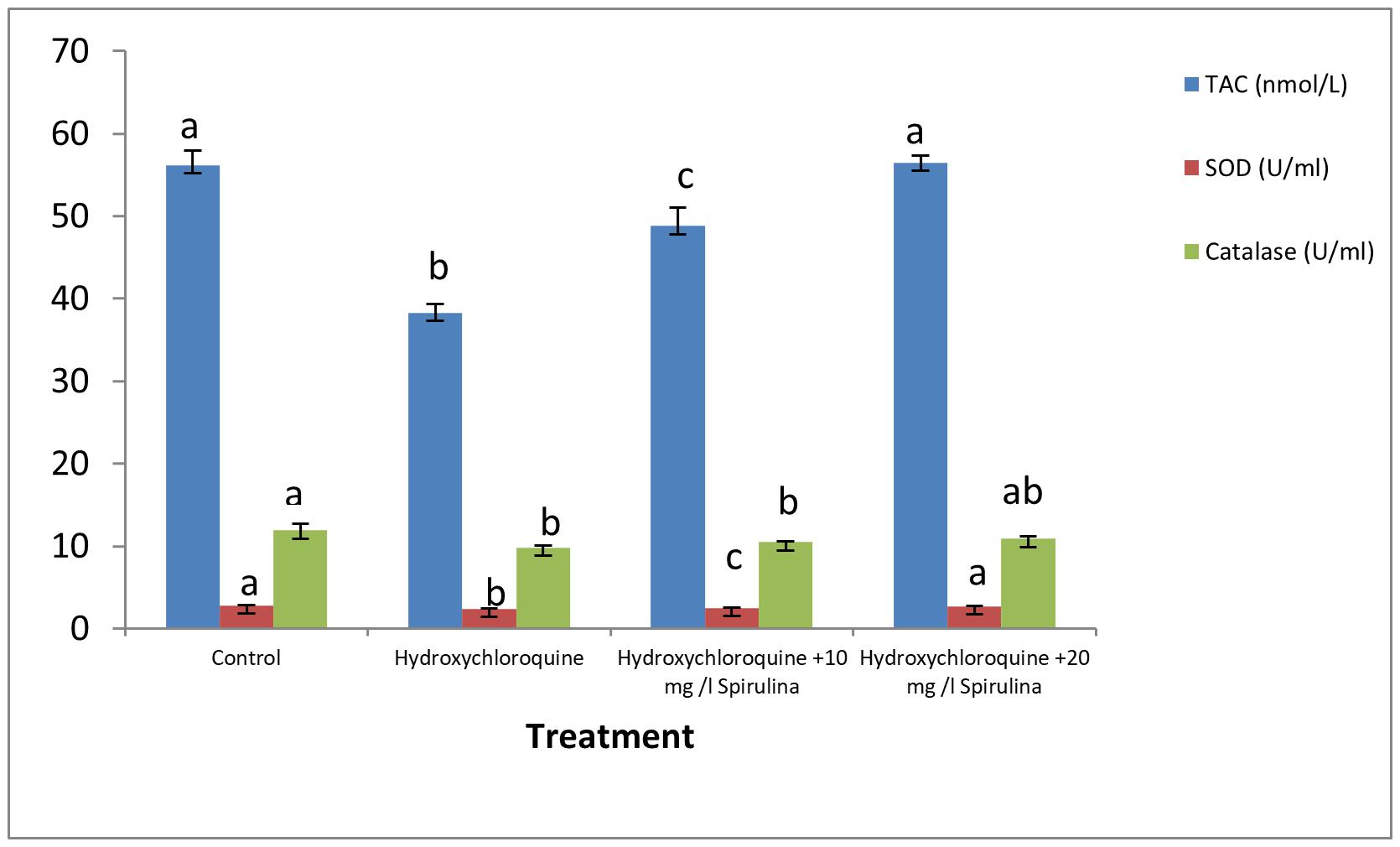
Figure 1. Effect of hydroxychloroquine (HCQ) exposure for 15 days on antioxidant parameters of the African catfish (Clarias gariepinus) and treatment with SP (in 10 and 20 mg l– 1 water). Bars with different superscript letters for each parameters are significantly different (P < 0.05).
Erythron Profile
HCQ (3.16 mg/l) caused a significant increase in the percentage of poikilocytosis and nuclear abnormalities of RBCs in relation to the control group (Table 1). The blood smears of control fish showed erythrocytes, which are ellipsoidal in shape with a centrally located ellipsoidal nucleus (Figure 2A). The blood smear of HCQ-treated catfish (C. gariepinus) showed poikilocytosis of erythrocytes. The major alterations of RBCs are teardrop-like cells (Tr), whose shape looks like a tear with pointed apices; sickle cells (Sk), which are elongated, crescent-shaped RBCs; eccentric nuclei, which are nuclei deviating or departing from the center of the cell; acanthocytes (Ac), where the red blood cells develop an irregular cell surface with numerous projections; crenated cells (Cr); with fewer projections from the surface; schistocytes (fragmented RBCs); and hemolyzed cells (Figures 2B,C). Likewise, there are still some alterations of RBCs in the fish exposed to HCQ and co-treated with Spirulina (10 and 20 mg/l), such as tear drop-like cells, acanthocytes, and eccentric nuclei (Figures 2D,E).
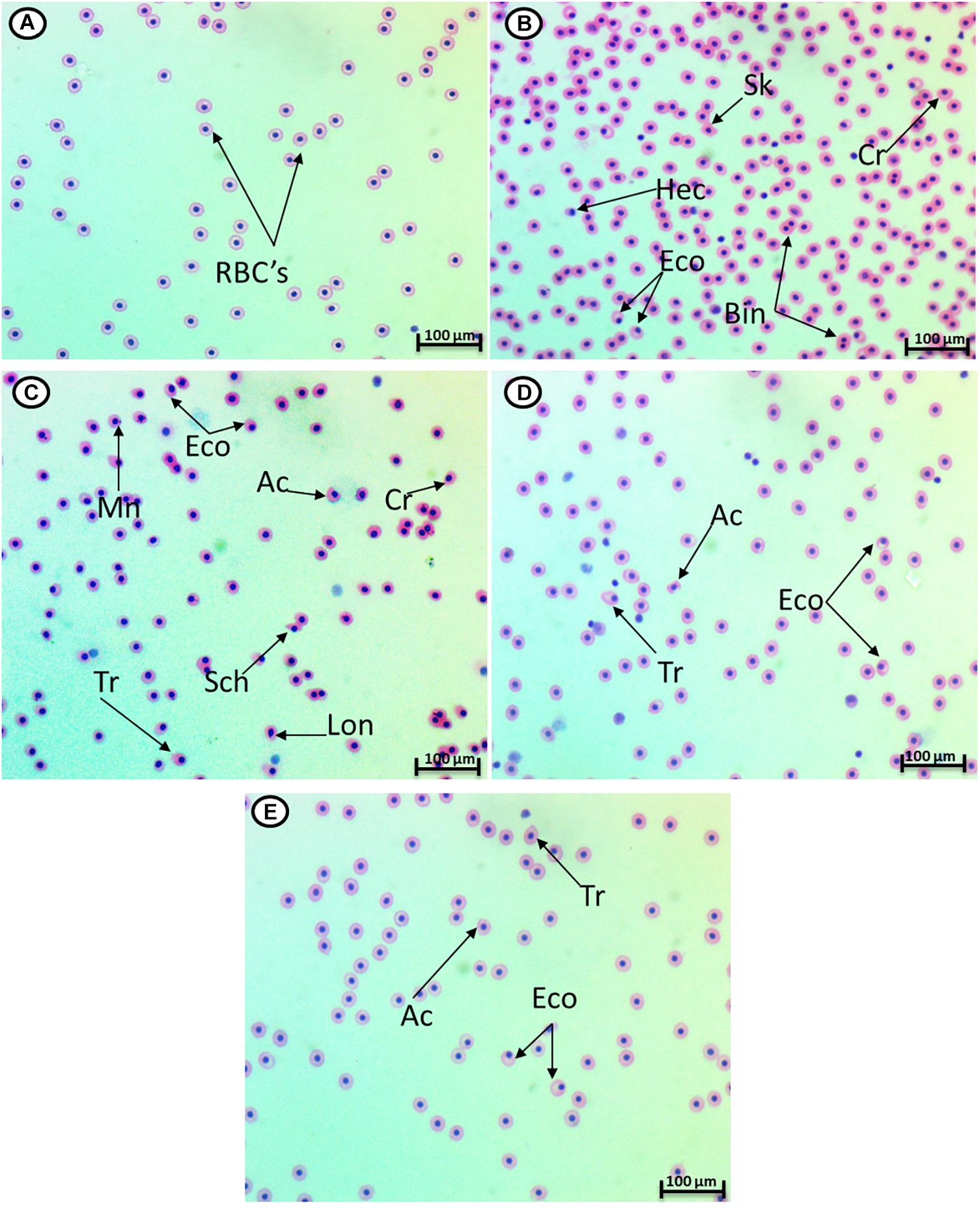
Figure 2. Hematoxylin–eosin-stained blood smears from catfish showing I: the normal erythrocytes (A), the deformed ones after exposure to hydroxychloroquine for 15 days (B,C), the deformed ones after exposure to hydroxychloroquine + 10 mg/l Spirulina for 15 days (D), and the deformed ones after exposure to hydroxychloroquine + 20 mg/l Spirulina for 15 days (E). Tr, tear-drop cell; Cr, crenated cell; Ac, acanthocyte; Eco, eccentric nucleus; Hec, hemolyzed cells; Mn, micronucleus; Bin, bionuclei; Lon, lobed nucleus; Shc, schistocytes; Sk, sickle cell (magnification: 1,000×).
The major alterations of nuclei of RBCs in the fish treated with HCQ are shown by micronuclei (Mn), binuclei (Bin), and lobed nucleus (Lon) and having a large invagination of the nuclear envelope that has no clear shape or definition (Figures 2B,C).
Hepato-Nephropathological Alterations
The liver of the control group showed normal hepatocytes and blood sinusoids (Figure 3A). The liver of HCQ-exposed fish showed some histopathological alterations, such as dilation of the central vein, vacuolization, degeneration of hepatocytes, and pyknotic nuclei (Figure 3B). The liver of HCQ-exposed fish and fish co-treated with SP (10 and 20 mg l–1 water) showed mild and strong improvement of the histoarchitecture of the liver in a dose-dependent manner (Figures 3C,D).
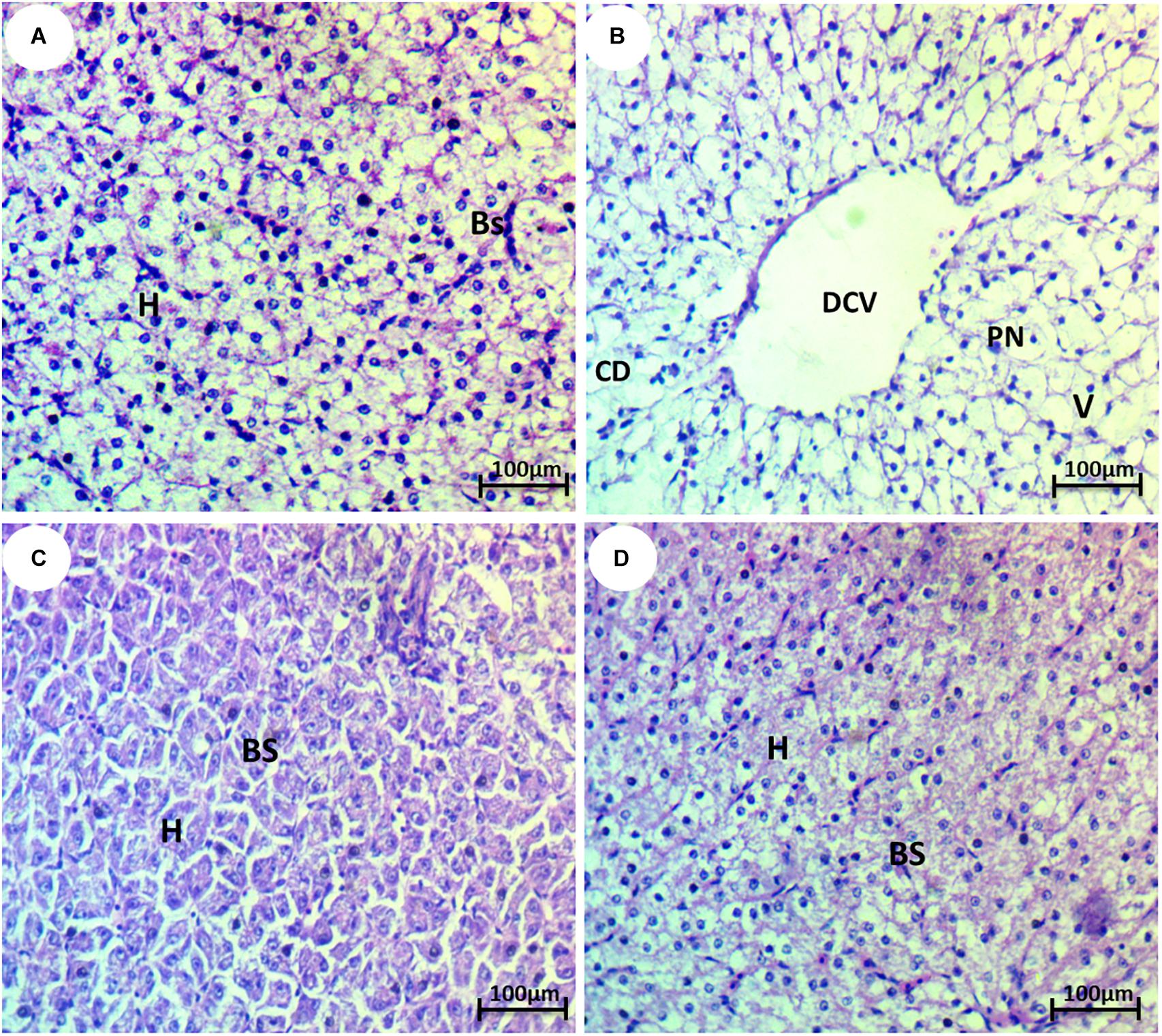
Figure 3. Photomicrograph of liver tissue of the African catfish (Clarias gariepinus) showing (A) normal architecture of liver hepatocytes (H) and normal blood sinusoids (BS); (B) fish exposed to hydroxychloroquine showing complete degeneration (CD), pyknosis of nuclei (PN), dilation of the central vein (DCV), and vacuolization (V); (C) fish exposed to hydroxychloroquine + 10 mg/l Spirulina showing mild improvement of hepatocytes (H) and blood sinusoids (BS); and (D) fish exposed to hydroxychloroquine + 20 mg/l Spirulina showing strong improvement of hepatocytes (H) and blood sinusoids (BS) (H&E stain, scale bar 50 μm).
The normal morphology of glomeruli, renal tubule, and Bowman’s capsule was shown in the kidney tissues of the control group (Figure 4A). The HCQ-exposed group showed several anomalies, such as reduction of glomeruli, dilation of Bowman’s space, and deterioration of renal tubules (Figure 4B). The kidney of HCQ-exposed fish and fish co-treated with SP (10 and 20 mg l–1 water) showed mild and moderate improvement of the histoarchitecture of kidney in a dose-dependent manner (Figures 4C,D).
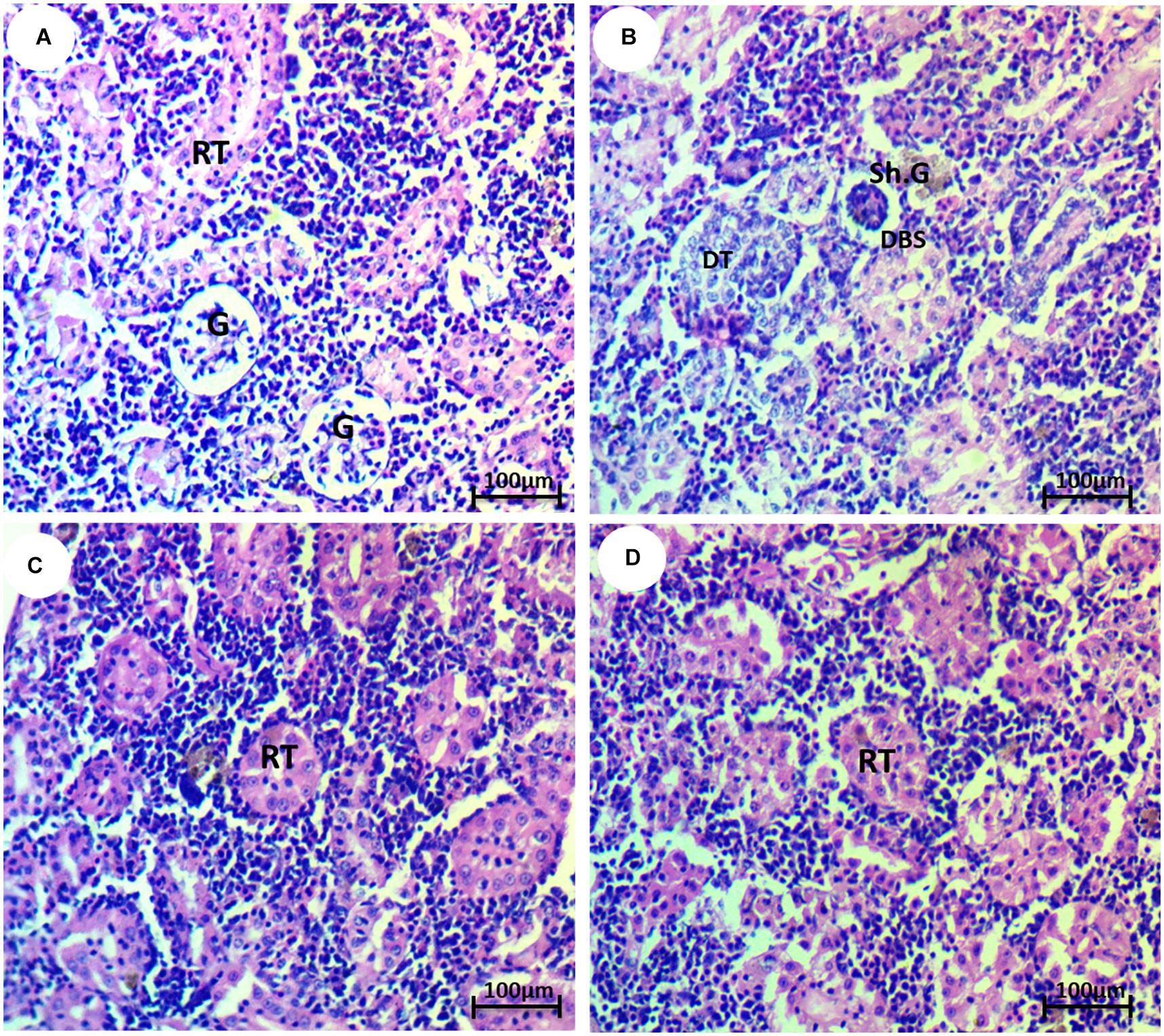
Figure 4. Photomicrograph of kidney tissue of the African catfish (Clarias gariepinus) showing (A) normal architecture of glomerulus (H) and renal tubule (RT); (B) fish exposed to hydroxychloroquine showing degeneration of renal tubule (DT), shrinkage of the glomerulus (Sh.G), and dilation of Bowman’s space (DBS); (C) fish exposed to hydroxychloroquine + 10 mg/l Spirulina showing mild improvement of the renal tubule (RT); and (D) fish exposed to hydroxychloroquine + 20 mg/l Spirulina showing moderate improvement of the renal tubule (RT) (H&E stain, scale bar 50 μm).
Discussion
Environmental release and accumulation of pharmaceutical products are a global concern in view of increased awareness of ecotoxicological effects (Soliman et al., 2019; Soliman and Sayed, 2020). In the present study, most of the hematological indices showed a significant decrease after exposure to 3.16 mg/l of HCQ for 15 days in relation to the control group. Besides, Nagaratnam et al. (1978) observed that patients treated with chloroquine for malaria suffered fever and severe anemia and had a palpable spleen, indicating that chloroquine interferes with hemoglobin (Thomé et al., 2013). Current results showed that co-treatment with Spirulina significantly inhibits the decrease in the hematological indices induced by HCQ because S. platensis consists of iron and vitamins, thus controlling the production of red blood cells (Hemalatha, 2012). S. platensis contains polysaccharides which induce RBC regeneration (Mohamed et al., 2014). Moreover, a pigment, phycocyanin, induces the erythropoietin (EPO) hormone which is responsible for erythropoiesis (Zhang, 1994). Spirulina contains B-carotene which enhances RBC recovery to decrease cell lysis and alterations (Stivala et al., 1996; Sayed and Soliman, 2018; Hamed et al., 2019b).
The current data showed alterations (increase or decrease) in some biochemical parameters after exposure to 3.16 mg/l of HCQ. The increased level of ALT and AST reflects damage to hepatocytes (Komatsu et al., 2002) or damage in the protein and carbohydrate metabolism (Ramesh et al., 2018). Murugavel and Pari (2004) reported that high doses of chloroquine increased serum uric acid and creatinine which are regarded as biomarkers for renal failure. Co-treatment with Spirulina significantly inhibits the increase of these biochemical parameters induced by HCQ, which was sustained by the limited extent of histological changes in the present study. Moreover, Bhat and Madyastha (2001) stated that the existence of blue pigment phycocyanin in Spirulina decreases the hepatotoxicity caused by paracetamol-induced free radicals because it contains enzyme SOD, β-carotene, selenium, or vitamins which have immunostimulant activities and protective effects (Alam et al., 2013). The nephroprotective ability of Spirulina recorded in the present study may be due to the presence of antioxidant compounds like triterpenes and flavonoids or because of its free radical scavenging effects (Sharoud, 2015).
Antioxidant biomarkers, i.e., TAC, CAT, and SOD, were depleted significantly in the catfish treated with HCQ, which is in accordance with Pari and Murugan (2006), Kumar Mishra et al. (2013), and Asha et al. (2019). A decrease in the activity of CAT may be due to the excess of superoxide anion radical as a consequence of a reduction in the activity of SOD (Kumar Mishra et al., 2013). Besides, the decreased activities of SOD and CAT may be due to decreased synthesis of enzymes or inhibition of enzyme activity by enhanced lipid peroxidation after stress (Murugavel and Pari, 2004). Co-treatment with Spirulina increased the levels of antioxidants significantly in HCQ-treated groups. This indicated the ability of Spirulina to protect from the oxidative stress caused by HCQ for it has been reported to possess strong antioxidant activity (phycocyanin) and to provoke the free radical scavenging enzyme system (Sabina et al., 2009).
In the current study, HCQ triggered a significant increase in the percentage of poikilocytosis, and a nuclear abnormality of RBCs in relation to the control group, which was reported before in patients treated with chloroquine for malaria, showed iso-poikilocytosis and hypochromia (Nagaratnam et al., 1978). HCQ is assumed to interact with erythrocytes and inhibit dehydrogenase of delta-amino levulinic acid, causing plasma membrane disruption and leading to poikilocytosis in fish (Hamed et al., 2019b). Extensive improvement in the alterations after SP treatment was observed, which indicated the protective role of SP against the alterations in erythrocytes. Spirulina is described as an antioxidant and anti-mutagenic due to the presence of alpha-tocopherol and beta-carotene (Araldi et al., 2014).
The histopathological evaluation of the liver showed dilation of the central vein, vacuolization, degeneration of hepatocytes, and pyknosis of nuclei, which are in accordance with Pari and Amali (2005) who stated that the histopathological observations in CQ-treated rats showed feathery degeneration and a microvesicular type of fatty generation with sinusoidal dilation and focal necrosis. This could be due to the formation of highly reactive radicals because of the oxidative threat caused by CQ (Pari and Murugan, 2006). Likewise, El Shishtawy et al. (2013) observed sinusoidal congestion, hepatoportal and hydropic degeneration, cloudy swelling, cellular necrosis (karyolysis, karyorrhexis, and nuclear pyknosis), and inflammatory cellular infiltration in chloroquine and HCQ treated-albino rats. Moreover, Ramesh et al. (2018) noticed severe morphological anomalies, such as vacuolization, nuclear degeneration, cellular edema, necrosis, altered hepatocyte, and increased sinusoidal space, which appeared in CQ-treated Cyprinus carpio fingerlings. Histopathological results reveal the incidence of degeneration of liver cells of albino rats upon oral administration of chloroquine (Asha et al., 2019).
The histopathological evaluation of the kidney showed a reduction of glomeruli, dilation of Bowman’s space, and deterioration of renal tubules, which is in accordance with Pari and Murugan (2006), who stated the histopathological observations in chloroquine-treated rat kidneys showing multiple foci of hemorrhage and necrosis with cloudy swelling of tubules, which could be due to the formation of highly reactive radicals as a consequence of oxidative threat caused by CQ (Murugavel and Pari, 2004). Besides, El Shishtawy et al. (2013) mentioned that cloudy swelling, vascular congestion, hydropic degeneration, hemorrhage, inflammation, focal tubular necrosis, and hypercellularity of glomeruli were observed in chloroquine- and HCQ-treated albino rats. In addition, Ramesh et al. (2018) noticed various anomalies, such as tubular cell necrosis, thickening of Bowman’s capsule, reduction of glomeruli, glomerular necrosis, tubular degeneration, hyaline droplet degeneration, congestion in the renal parenchyma, cloudy swelling, renal tubular separation, and reduction of lumens, in the kidney of fish exposed to CQ. Co-treatment with Spirulina restored the histological structure to a normal state. Spirulina reinforces the stabilization of the plasma membrane, thereby preserving the structural integrity of cells as well as the repair of tissue damage (Stivala et al., 1996).
Conclusion
The present investigation indicates that HCQ induced hematological, biochemical, and histological changes as well as antioxidant depletion and poikilocytosis in catfish. In addition, Spirulina remedies the toxic effects of HCQ due to its bioactive components. Therefore, Spirulina can be used in aquaculture for protecting fish from pharmaceutical residue side effects.
Data Availability Statement
The raw data supporting the conclusions of this article will be made available by the authors, without undue reservation.
Ethics Statement
The animal study was reviewed and approved by the Assiut University committee.
Author Contributions
All authors listed have made a substantial, direct and intellectual contribution to the work, and approved it for publication.
Conflict of Interest
The authors declare that the research was conducted in the absence of any commercial or financial relationships that could be construed as a potential conflict of interest.
Supplementary Material
The Supplementary Material for this article can be found online at: https://www.frontiersin.org/articles/10.3389/fphys.2021.683669/full#supplementary-material
References
Aebi, H. (1984). Catalase in vitro. Methods Enzymol. 105, 121–126. doi: 10.1016/s0076-6879(84)05016-3
AFS-FHS. (2004). Suggested Procedures for the Detection and Identification of Certain Finfish and Shellfish Pathogens. American Fisheries Society: Bethesda.
Alam, M. A., Haider, N., Ahmed, S., Alam, M. T., Aziz, A., and Praveen, A. (2013). Tahlab (Spirulina) and few other medicinal plants having anti-oxidant &immunomodulatory properties described in Unani medicine- A review. Int. J. Pharm. Sci. Res. 4, 4158–4164.
Al-Sabti, K., and Metcalfe, C. D. (1995). Fish micronuclei for assessing genotoxicity in water. Mutat Res. 343, 121–135. doi: 10.1016/0165-1218(95)90078-0
Araldi, R. P., Rechiutti, B. M., Mendes, T. B., Ito, E. T., and Souza, E. B. (2014). Mutagenic potential of Cordia ecalyculata alone and in association with Spirulina maxima for their evaluation as candidate anti-obesity drugs. Genet. Mol. Res. 13, 5207–5220. doi: 10.4238/2014.july.7.14
Asha, S., Thirunavukkarasu, P., and Taju, G. (2019). Curcumin pretreated hepatoprotectivity against antimalarial drug chloroquine induced hepatotoxicity in albino rats. Eurasian J. BioSci. 13, 41–48.
Bensalah, N., Midassi, S., Ahmad, M. I., and Bedoui, A. (2020). Degradation of hydroxychloroquine by electrochemical advanced oxidation processes. Chem. Eng. J. 402:126279. doi: 10.1016/j.cej.2020.126279
Bhat, V. B., and Madyastha, K. M. (2001). Scavenging of Peroxynitrite by Phycocyanin and Phycocyanobilin from Spirulina platensis: protection against Oxidative Damage to DNA. Biochem. Biophys. Res. Commun. 285, 262–266. doi: 10.1006/bbrc.2001.5195
Borba, E. F., Turrini-Filho, J. R., Kuruma, K. A., Bertola, C., Pedalini, M. E., Lorenzi, M. C., and Bonfá, E. (2004). Chloroquine gestational use in systemic lupus erythematosus: assessing the risk of child ototoxicity by pure tone audiometry. Lupus 13, 223–227. doi: 10.1191/0961203304lu528oa
Carlsson, G., Örn, S., and Larsson, D. J. (2009). Effluent from bulk drug production is toxic to aquatic vertebrates. Environ. Toxicol. Chem. 28, 2656–2662. doi: 10.1897/08-524.1
Cash, P., Stanković, T., and Štorga, M. (2016). “An introduction to experimental design research,” in Experimental Design Research, eds P. Cash, T. Stanković, and M. Štorga (Cham: Springer), 3–12. doi: 10.1007/978-3-319-33781-4_1
El Shishtawy, M., Elgendy, F. S., and Ramzy, R. (2013). Comparative Toxicity Study of Chloroquine and Hydroxychloroquine on Adult Albino Rats. Mansoura J. Forensic Med. Clin. Toxicol. 21, 15–28. doi: 10.21608/mjfmct.2013.47674
Fazio, F., Piccione, G., Tribulato, K., Ferrantelli, V., Giangrosso, G., Arfuso, F., and Faggio, C. (2014). Bioaccumulation of heavy metals in blood and tissue of striped mullet in two Italian lakes. J. Aquat. Anim. Health 26, 278–284. doi: 10.1080/08997659.2014.938872
Hamed, M., Soliman, H. A. M., Osman, A. G. M., and Sayed, A. E. H. (2019a). Assessment the effect of exposure to microplastics in Nile Tilapia (Oreochromis niloticus) early juvenile: I. blood biomarkers. Chemosphere 228, 345–350. doi: 10.1016/j.chemosphere.2019.04.153
Hamed, M., Soliman, H. A. M., and Sayed, A. E. D. H. (2019b). Ameliorative effect of Spirulina platensis against lead nitrate-induced cytotoxicity and genotoxicity in catfish Clarias gariepinus. Environ. Sci. Pollut. Res. Int. 26, 20610–20618. doi: 10.1007/s11356-019-05319-3
Hemalatha, K. (2012). Studies on the protective efficacy of Spirulinaagainst lead acetate induced hepatotoxicity in Rattusnorvegicus. Int. J. Chem. Anal. Sci. 3, 1509–1512.
Hughes, S. R., Kay, P., and Brown, L. E. (2013). Global synthesis and critical evaluation of pharmaceutical data sets collected from river systems. Environ. Sci. Technol. 47, 661–677. doi: 10.1021/es3030148
Khafaga, A. F., and El-Sayed, Y. S. (2018). Spirulina ameliorates methotrexate hepatotoxicity via antioxidant, immune stimulation, and proinflammatory cytokines and apoptotic proteins modulation. Life Sci. 196, 9–17. doi: 10.1016/j.lfs.2018.01.010
Komatsu, T., Yamazaki, H., Nakajima, M., and Yokoi, T. (2002). Identification of catalase in human livers as a factor that enhances phenytoin dihydroxy metabolite formation by human liver microsomes. Biochem. Pharmacol. 63, 2081–2090. doi: 10.1016/s0006-2952(02)01024-9
Koracevic, D., Koracevic, G., Djordjevic, V., Andrejevic, S., and Cosic, V. (2001). Method for the measurement of antioxidant activity in human fluids. J. Clin. Pathol. 54, 356–361. doi: 10.1136/jcp.54.5.356
Kumar Mishra, S., Singh, P., and Rath, S. K. (2013). Protective effect of quercetin on chloroquine-induced oxidative stress and hepatotoxicity in mice. Malar. Res. Treat. 2013:141734.
Kumar, R., Sharma, A., Srivastava, J. K., Siddiqui, M. H., Uddin, M. S., and Aleya, L. (2021). Hydroxychloroquine in COVID-19: therapeutic promises, current status, and environmental implications. Environ. Sci. Pollut. Res. 1–14. doi: 10.1007/s11356-020-12200-1 [Epub online ahead of print].
Larsson, D. J., de Pedro, C., and Paxeus, N. (2007). Effluent from drug manufactures contains extremely high levels of pharmaceuticals. J. Hazard. Mat. 148, 751–755. doi: 10.1016/j.jhazmat.2007.07.008
Lindroos, M., Hörnström, D., Larsson, G., Gustavsson, M., and van Maris, A. J. (2019). Continuous removal of the model pharmaceutical chloroquine from water using melanin-covered Escherichia coli in a membrane bioreactor. J. Hazard. Mat. 365, 74–80. doi: 10.1016/j.jhazmat.2018.10.081
Lu, J., Ren, D. F., Wang, J. Z., Sanada, H., and Egashira, Y. (2010). Protection by dietary Spirulina platensis against D-galactosamine–and acetaminophen-induced liver injuries. Br. J. Nutr. 103, 1573–1576. doi: 10.1017/s0007114509993758
Mahmoud, Y. I., and Abd El-Ghffar, E. A. (2019). Spirulina ameliorates aspirin-induced gastric ulcer in albino mice by alleviating oxidative stress and inflammation. Biomed. Pharmacother. 109, 314–321. doi: 10.1016/j.biopha.2018.10.118
Margot, J., Rossi, L., Barry, D. A., and Holliger, C. (2015). A review of the fate of micropollutants in wastewater treatment plants. WIREs Water 2, 457–487. doi: 10.1002/wat2.1090
Martin, S. J., and Sabina, E. P. (2016). Amelioration of anti-tuberculosis drug induced oxidative stress in kidneys by Spirulina fusiformis in a rat model. Ren Fail. 38, 1115–1121. doi: 10.1080/0886022x.2016.1184940
Mohamed, W. A., Ismail, S. A., and el-Hakim, Y. M. A. (2014). Spirulina platensis ameliorative effect against GSM 900-MHz cellular phoneradiation-induced genotoxicity in male Sprague-Dawley rats. Comp. Clin. Pathol. 23, 1719–1726. doi: 10.1007/s00580-014-2003-x
Murugavel, P., and Pari, L. (2004). Attenuation of chloroquine-induced renal damage by alpha-lipoic acid: possible antioxidant mechanism. Ren Fail. 26, 517–524. doi: 10.1081/jdi-200031761
Nagaratnam, N., Chetiyawardana, A. D., and Rajiyah, S. (1978). Aplasia and leukaemia following chloroquine therapy. Postgrad. Med. J. 54, 108–112. doi: 10.1136/pgmj.54.628.108
Nishikimi, M., Appaji, N., and Yagi, K. (1972). The occurrence of superoxide anion in the reaction of reduced phenazine methosulfate and molecular oxygen. Biochem. Biophys. Res. Commun. 46, 849–854. doi: 10.1016/s0006-291x(72)80218-3
Pari, L., and Amali, D. R. (2005). Protective role of tetrahydrocurcumin (THC) an active principle of turmeric on chloroquine induced hepatotoxicity in rats. J. Pharm. Pharm. Sci. 8, 115–123.
Pari, L., and Murugan, P. (2006). Tetrahydrocurcumin: effect on chloroquine-mediated oxidative damage in rat kidney. Basic Clin. Pharmacol. Toxicol. 99, 329–334. doi: 10.1111/j.1742-7843.2006.pto_503.x
Ramesh, M., Anitha, S., Poopal, R. K., and Shobana, C. (2018). Evaluation of acute and sublethal effects of chloroquine (C(18)H(26)CIN(3)) on certain enzymological and histopathological biomarker responses of a freshwater fish Cyprinus carpio. Toxicol. Rep. 5, 18–27. doi: 10.1016/j.toxrep.2017.11.006
Rana, R. S., Singh, P., Kandari, V., Singh, R., Dobhal, R., and Gupta, S. (2017). A review on characterization and bioremediation of pharmaceutical industries’ wastewater: an Indian perspective. Appl. Water Sci. 7, 1–12. doi: 10.1007/s13201-014-0225-3
Sabina, E., Samuel, J., RajappaRamya, S., Patel, S., Mandal, N., Pranatharthiiharan, P., and Rasool, M. (2009). Hepatoprotective and antioxidant potential of Spirulinafusiformis on acetaminophen-induced hepatotoxicity in mice. Int. J. Integr. Biol. 6, 1–5.
SANOFI. (2020). Important information on Plaquenil® /Quensyl® / Plaquinol® (hydroxychloroquine) and COVID-19. Available online at: https://www.sanofi.com/en/about-us/our-stories/importantinformation- on-plaquenil-quensyl-plaquinol-COVID-19 [Accessed Nov 15 2020].
Sayed, A. E. H., and Soliman, H. A. M. (2018). Modulatory effects of green tea extract against the hepatotoxic effects of 4-nonylphenol in catfish (Clarias gariepinus). Ecotoxicol. Environ. Saf. 149, 159–165. doi: 10.1016/j.ecoenv.2017.11.007
Schwarzenbach, R. P., Escher, B. I., Fenner, K., Hofstetter, T. B., Johnson, C. A., Von Gunten, U., and Wehrli, B. (2006). The challenge of micropollutants in aquatic systems. Science 313, 1072–1077. doi: 10.1126/science.1127291
Seshadri, C. V., Umesh, B. V., and Manoharan, R. (1991). Beta-carotene studies in Spirulina. Bioresour. Technol. 38, 111–113. doi: 10.1016/0960-8524(91)90140-f
Sharoud, M. N. (2015). Protective effect of Spirulina against paracetamol-induced hepatic injury in rats. J. Exp. Biol. Agric. Sci. 3, 44–53.
Soliman, H. A. M., and Sayed, A. E. H. (2020). Poikilocytosis and tissue damage as negative impacts of tramadol on juvenile of Tilapia (Oreochromis niloticus). Environ. Toxicol. Pharmacol. 78:103383. doi: 10.1016/j.etap.2020.103383
Soliman, H. A. M., Hamed, M., Lee, J. S., and Sayed, A. E. H. (2019). Protective effects of a novel pyrazolecarboxamide derivative against lead nitrate induced oxidative stress and DNA damage in Clarias gariepinus. Environ. Pollut. 247, 678–684. doi: 10.1016/j.envpol.2019.01.074
Stivala, L. A., Savio, M., Cazzalini, O., Pizzala, R., Rehak, L., Bianchi, L., Vannini, V., and Prosperi, E. (1996). Effect of beta-carotene on cell cycle progression of human fibroblasts. Carcinogenesis 17, 2395–2401. doi: 10.1093/carcin/17.11.2395
Takano, T., Katoh, Y., Doki, T., and Hohdatsu, T. (2013). Effect of chloroquine on feline infectious peritonitis virus infection in vitro and in vivo. Antiviral Res. 99, 100–107. doi: 10.1016/j.antiviral.2013.04.016
Thomé, R., Lopes, S. C., Costa, F. T., and Verinaud, L. (2013). Chloroquine: modes of action of an undervalued drug. Immunol. Lett. 153, 50–57. doi: 10.1016/j.imlet.2013.07.004
Zeenat, M., Sharmin, S., Islam, M. K., Rashid, M. B., Saha, T., and Islam, M. T. (2017). Counteracting Effect of SpirulinaAgainst Acetaminophen on Some Selected Blood Parameters and Liver Histotexture of Mice. BD Vet. Med. Rec. 3:1317.
Keywords: Spirulina, hydroxychloroquine, Clarias gariepinus, AST, TAC
Citation: Sayed AH, Hamed M and Soliman HAM (2021) Spirulina platensis Alleviated the Hemotoxicity, Oxidative Damage and Histopathological Alterations of Hydroxychloroquine in Catfish (Clarias gariepinus). Front. Physiol. 12:683669. doi: 10.3389/fphys.2021.683669
Received: 21 March 2021; Accepted: 24 May 2021;
Published: 06 July 2021.
Edited by:
Samad Rahimnejad, University of South Bohemia in České Budìjovice, CzechiaReviewed by:
Neeraj Kumar, National Institute of Abiotic Stress Management (ICAR), IndiaMohammad Mahmoud Nabih Authman, National Research Centre, Egypt
Mahmoud A. O. Dawood, Kafrelsheikh University, Egypt
Copyright © 2021 Sayed, Hamed and Soliman. This is an open-access article distributed under the terms of the Creative Commons Attribution License (CC BY). The use, distribution or reproduction in other forums is permitted, provided the original author(s) and the copyright owner(s) are credited and that the original publication in this journal is cited, in accordance with accepted academic practice. No use, distribution or reproduction is permitted which does not comply with these terms.
*Correspondence: Alaa El-Din H. Sayed, alaasayed@aun.edu.eg; alaa_h254@yahoo.com
†These authors have contributed equally to this work