- 1Department of Intensive Care, Amsterdam UMC, University of Amsterdam, Amsterdam, Netherlands
- 2Department of Intensive Care, Brugmann University Hospital, Université Libre de Bruxelles, Brussels, Belgium
- 3Department of Intensive Care, Maastricht UMC+, Maastricht, Netherlands
- 4Laboratory of Experimental Intensive Care and Anesthesiology, Amsterdam UMC, University of Amsterdam, Amsterdam, Netherlands
- 5Department of Anesthesiology, Amsterdam UMC, University of Amsterdam, Amsterdam, Netherlands
- 6Mahidol–Oxford Tropical Medicine Research Unit (MORU), Mahidol University, Bangkok, Thailand
- 7Nuffield Department of Medicine, University of Oxford, Oxford, United Kingdom
- 8Department of Respiratory Medicine, Amsterdam UMC, University of Amsterdam, Amsterdam, Netherlands
Background: Recruitment maneuvers (RMs) have heterogeneous effects on lung aeration and have adverse side effects. We aimed to identify morphological, anatomical, and functional imaging characteristics that might be used to predict the RMs on lung aeration in invasively ventilated patients.
Methods: We performed a systemic review. Studies included invasively ventilated patients who received an RM and in whom re-aeration was examined with chest computed tomography (CT), electrical impedance tomography (EIT), and lung ultrasound (LUS) were included.
Results: Twenty studies were identified. Different types of RMs were applied. The amount of re-aerated lung tissue after an RM was highly variable between patients in all studies, irrespective of the used imaging technique and the type of patients (ARDS or non-ARDS). Imaging findings suggesting a non-focal morphology (i.e., radiologic findings consistent with attenuations with diffuse or patchy loss of aeration) were associated with higher likelihood of recruitment and lower chance of overdistention than a focal morphology (i.e., radiological findings suggestive of lobar or segmental loss of aeration). This was independent of the used imaging technique but only observed in patients with ARDS. In patients without ARDS, the results were inconclusive.
Conclusions: ARDS patients with imaging findings suggestive of non-focal morphology show most re-aeration of previously consolidated lung tissue after RMs. The role of imaging techniques in predicting the effect of RMs on re-aeration in patients without ARDS remains uncertain.
Introduction
A lung recruitment maneuver (RM) is a dynamic and transient increase in transpulmonary pressure aiming at (re-)opening collapsed lung parts and increasing end-expiratory lung volume (Lapinsky and Mehta, 2005). In theory, opening of collapsed or “non-aerated” lung areas decreases shunt, improving both oxygenation and removal of CO2 (Hedley-Whyte et al., 1964; Neumann et al., 1999). Furthermore, atelectatic areas might cause stress on, or deformation of, aerated regions, resulting in additional injury of lung parenchyma (Gattinoni et al., 2012). Accordingly, decreasing atelectatic areas with RM could protect the lungs, a strategy often referred to as the “open lung concept” (Hes, 2015).
The value of RMs without the use of any imaging monitoring is disputed, as, so far, clinical studies have failed to show benefit with regard to patient-centered outcomes—and even suggest harm (Cavalcanti et al., 2017). The absence of net benefit might be explained by the heterogeneity and unpredictable effects of RMs on lung aeration (Sahetya and Brower, 2017; Mancebo et al., 2019). The pressure threshold that should be overpassed during RMs to open atelectatic lung units is multifactorial and cannot be calculated precisely (Sahetya and Brower, 2017; Gattinoni et al., 2017). Furthermore, any increase in airways pressure will result in higher pressures in all lung parts, also those that are “open,” and these areas might be harmed by overdistention (Gattinoni et al., 2019). Thus, the benefit of RMs needs to be balanced between re-aeration and overdistention.
Changes in lung morphology indicative of re-aeration or overdistention can be estimated using lung imaging (Gattinoni et al., 2020). Various imaging techniques like chest computed tomography (CT), electrical impedance tomography (EIT), and lung ultrasound (LUS) have been suggested to be useful to evaluate lung morphology and function in an individual patient (Godet et al., 2015). We performed a systematic review to describe imaging-based methods to assess re-aeration after RMs in patients receiving invasive ventilation at the intensive care unit or the operating room. In this review, we focus on the variability of imaging-based method definitions and the clinical utility of baseline imaging characteristics.
Methods
This protocol was designed in accordance with Preferred Reporting Items for Systematic Reviews and Meta-Analyses (PRISMA) guidelines (Liberati et al., 2009). The study protocol has been registered on PROSPERO (CRD42020188056).
Eligibility Criteria
The PICO used to define eligibility criteria are the following: (1) P (population): invasive mechanical ventilation either in the intensive care unit (ICU) or the operating room (OR) with or without ARDS, (2) I (intervention): recruitment maneuver of any sort, (3) C (comparison): LUS and/or EIT and/or CT was used to evaluate re-aeration of previously consolidated lung tissue, (4) O: baseline image characteristics were reported and evaluated for their predictive value of recruitment.
Only original studies written in English were included, whereas animal studies, case reports, comments, letters, and studies that enrolled pediatric patients were not included.
Information Sources and Search
We searched EMBASE using PubMed on December 15, 2020 using the following key words: ((“diagnostic imaging” [Subheading] OR (“diagnostic” [All Fields] AND “imaging” [All Fields]) OR “diagnostic imaging” [All Fields] OR “ultrasound” [All Fields] OR “ultrasonography” [MeSH Terms] OR “ultrasonography” [All Fields] OR “ultrasound” [All Fields] OR “ultrasonics” [MeSH Terms] OR “ultrasonics” [All Fields]) OR (“ct” [All Fields]) OR “computed tomography” [All Fields]) OR ((“IEEE Int Conf Electro Inf Technol” [Journal] OR “eit” [All Fields]) OR “(electrical impedance tomography” [All Fields])) AND ((“positive-pressure respiration” [MeSH Terms] OR (“positive-pressure” [All Fields] AND “respiration” [All Fields]) OR “positive-pressure respiration” [All Fields] OR “peep” [All Fields]) AND Recruitment [All Fields]).
Study Selection
The identified studies were assessed for inclusion criteria based on title and then on abstract. For all selected papers, the full text was read and discussed between two authors (CP and LB). Studies that fulfilled the inclusion criteria were included in this review.
Data Collection
For each included study, we collected data related to patient characteristics and whether they referred to ARDS patients or not. The type of recruitment maneuver that was used was categorized as (a) sustained inflation, (b) sigh, (c) pressure-control ventilation, and (d) variable ventilation (Rocco et al., 2010). We recorded the criteria that were used to define a “responder” to recruitment and the baseline characteristics to identify factors that differentiate between “responders” and “non-responders.” For those studies including patients with ARDS, we documented whether authors classified patients as having “focal” (i.e., radiological attenuations with lobar or segmental distributions) or “non-focal” (i.e., radiological attenuation with diffuse or patchy distribution) abnormal lung morphology.
Bias Assessment
The Quality Assessment of Diagnostic Accuracy Studies-2 (QUADAS-2) was used for the assessment of the methodologic quality of selected studies (Whiting, 2011). The four recommended domains (i.e., patient selection, index test, reference standard, and flow/timing) were assessed for low, high, or unclear risk of bias. As for the reference standard domain, CT was considered the “gold standard” for assessing lung re-aeration. Given the insufficient evidence to classify LUS or EIT as adequate reference tests to assess lung aeration, we considered the risk of bias to be high. Concerns regarding applicability for the first three domains were also assessed and scored as low, high, or unclear.
Synthesis of Results
The following data were combined into a table: patient group that was studied, number of patients, type of recruitment and maximal airway pressure reached, assessment of re-aeration of lung tissue, and criteria to define “responder.” The main findings of the study regarding heterogeneity in re-aerated lung tissue and differences between “responders” and “non-responders” were also shown. We further synthesized the current evidence for heterogeneity and prediction of recruitment response in an overview table, stratified per imaging method that was used. Finally, we linked the morphological characteristics derived from different imaging techniques of responders and non-responders in an overview figure.
Results
Included Studies
The described search resulted in 326 articles of which 249 were excluded based on the title and abstract review. Twenty out of the remaining 77 studies were included in this review based on full text review (Figure 1) and are summarized in Table 1. Seventeen studies included deeply sedated patients, while sedation level was not mentioned in the other three studies. All patients in the included studies were in supine position during RM. The majority of the included studies enrolled ARDS patients exclusively (14 studies, 70%). Three studies (15%) included a mixed population of intensive care unit patients, and in three studies (15%), patients undergoing elective operation were included. Three studies had the primary goal of quantification of potential for lung recruitment (Gattinoni et al., 2006; Camporota et al., 2019) or recruitment prediction (Constantin et al., 2010). Regarding lung imaging techniques, most of the studies (10 studies, 50%) assessed chest CT scan, followed by LUS (five studies, 25%) and EIT (five studies, 25%). Notably, chest CT was only used in studies that included patients with ARDS.
Quality characteristics of the included studies, in relation to the aim of this systematic review, are presented in Supplementary Table 1. In two studies, there was a high concern regarding applicability of population selection. These two studies included a highly selective population, i.e., patients after cardiac surgery (Longo et al., 2017) or patients who underwent tracheostomy (Eichler et al., 2018).
Recruitment Methodology and Identification of “Responders”
In eight studies (42%), a sigh, in six studies (31%), a pressure-control method, and in five studies (26%), a sustained inflation were used for the RM (Table 1). Applied maximum airway pressure varied widely, between 30 and 60 cm H2O. Classification of responders depended on the imaging technique used (Table 2). None of the studies defined the criteria to identify “responders” beforehand. Patients were classified post-hoc as “responders” and “non-responders” based on the median value of the study population in studies that quantified re-aeration by CT imaging. Recruitment “responders” generally had an increase in aeration of non-aerated lung tissue of more than 20% (Figure 2).
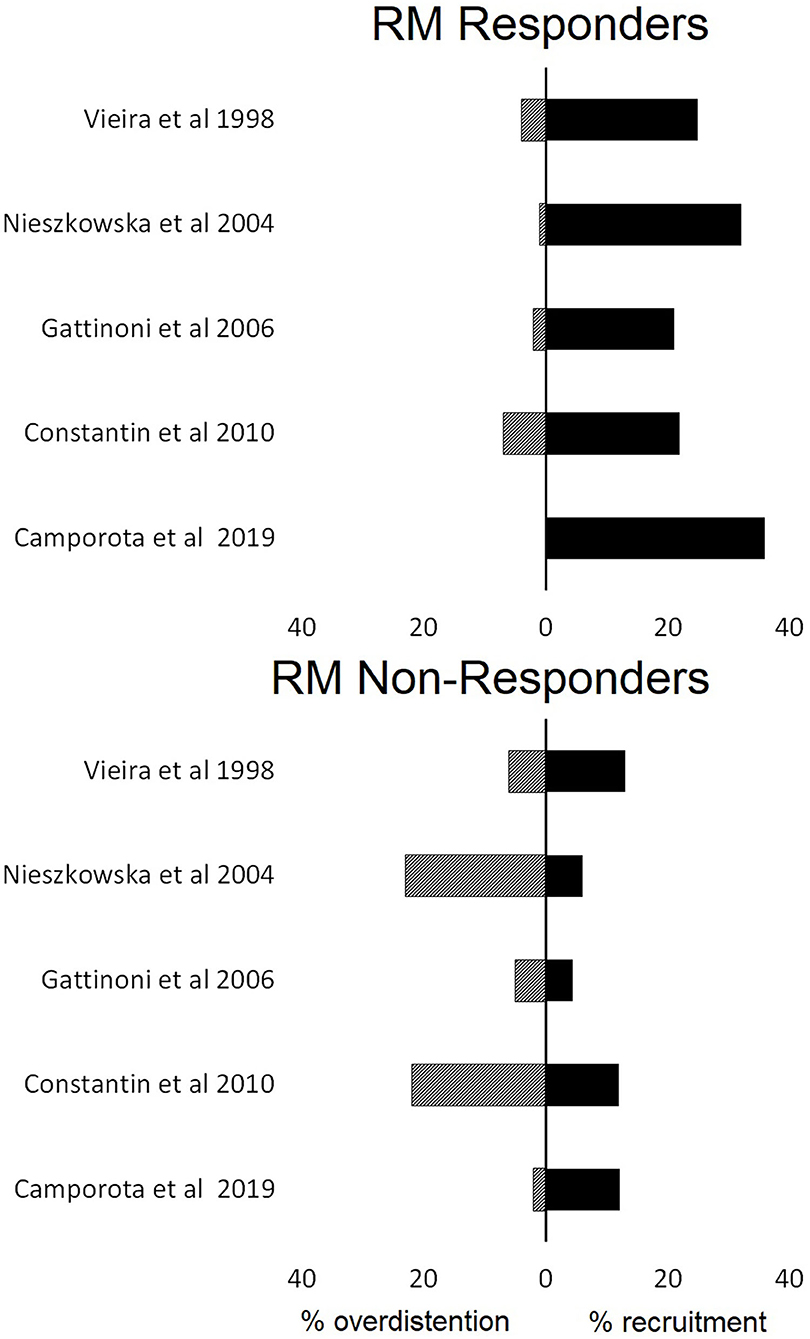
Figure 2. The proportions of lung recruitment and lung overdistention in patients who were characterized responders or not responders to lung recruitment maneuvers (RM) based on computed tomography findings.
Heterogeneity in Re-aeration and Prediction of Positive Response to RM
Re-aeration after RM varied widely between studies, independent of the used image technique (Table 3). Unsurprisingly, most CT imaging studies showed that around 50% of patients are “non-responders” to recruitment because the median value was used as the cutoff value (Borges et al., 2006; Gattinoni et al., 2006; Caironi et al., 2015; Vieira et al., 1999; Camporota et al., 2019). Studies that used other imaging techniques did not mention the proportion of “non-responders,” though recruitment was described as “highly variable” (Karsten et al., 2019; Généreux et al., 2020).
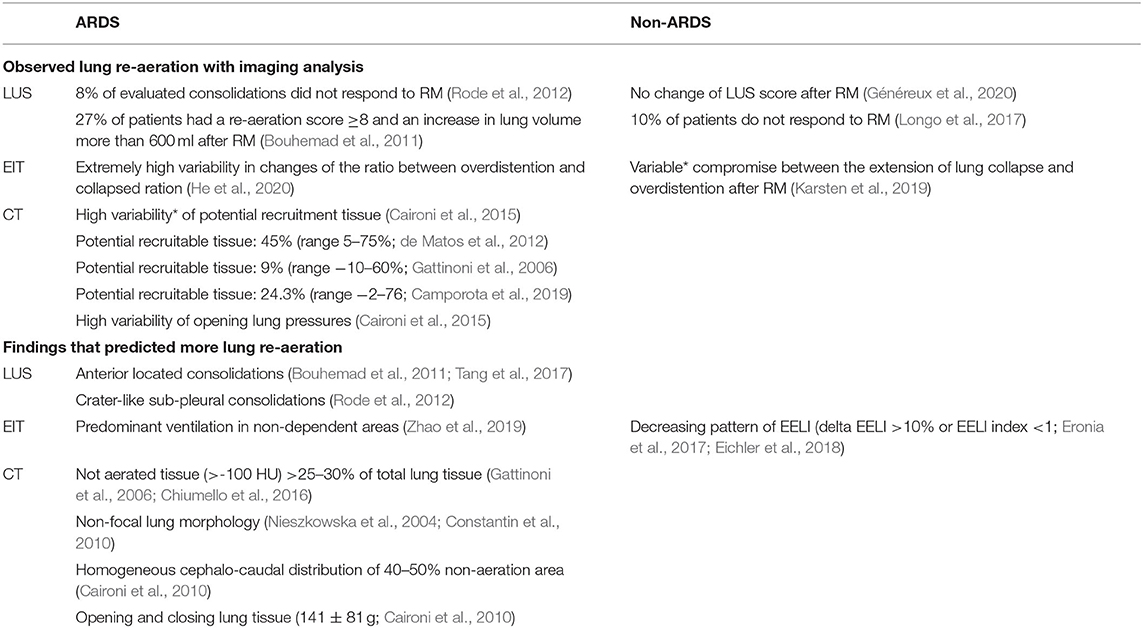
Table 3. Observed recruitment maneuver re-aeration effect and findings related to potential for lung re-aeration after recruitment maneuver according to the imaging module and the presence or not ARDS.
Imaging findings related to the amount of re-aerated lung tissue in patients with ARDS were the extent of lost aeration before RM, the distribution of non-aerated areas (craniocaudal and anteroposterior distribution), the morphology of non-aerated areas (e.g., crater-like consolidation), and functional lung characteristics related to tidal recruitment (tidal opening/closing tissue; Table 3). Findings that are more likely to resemble a diffuse or patchy loss of aeration (i.e., non-focal morphology) were suggestive of an increased likelihood of positive response to RMs (Figure 3). This was independent on the image technique employed.
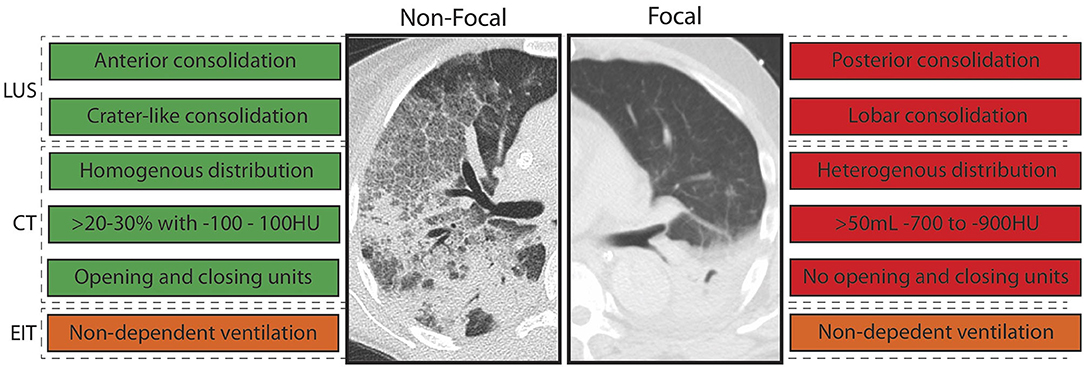
Figure 3. Imaging abnormalities that predicted response to recruitment maneuvers (RM) stratified per morphology. LUS, lung ultrasound; EIT, electrical impedance tomography; CT, computed tomography; HU, Houndsfield units; green, imaging abnormality in line with responder to RM; red, imaging abnormality in line with non-responder to RM; orange, imaging abnormality in line with responder with high uncertainty. Text boxes on the left: consistent with non-focal morphology. Text boxes on the right: consistent with focal morphology.
Only one study addressed the prediction of response to RM in patients in the operating room. A decreasing pattern of end-expiratory lung impedance (EELI) evaluated with EIT was found to be related to the amount of re-aerated lung tissue (Eichler et al., 2018; Table 3).
Overdistention
Overdistention was assessed in studies that used CT or EIT only, as LUS cannot be used for this purpose. Studies employing CT imaging showed the average percentage of overdistended lung volume to vary between 0 and 20% (Figure 2). EIT studies revealed the average overdistention secondary to RMs across patients to range between 5 and 30% (Karsten et al., 2019). Nevertheless, local overdistention in non-dependent areas may exceed 60% of that area (Eronia et al., 2017). “Non-responders” identified by CT had a higher increase in hyperinflated lung tissue compared with “responders” (Figure 2).
Discussion
The results of this systematic review can be summarized as follows: (a) data that quantify the potential for lung recruitment based on imaging are limited, (b) the definition of positive response to RMs was highly variable, and (c) patients with imaging characteristics suggestive for a non-focal morphology of ARDS seemed to show more re-aeration at RMs with moderate inspiratory pressures.
The included studies used a wide range of maximum airway pressures to recruit lung tissue. Most collapsed areas can be opened, but frequently only at very high airway pressures (Cressoni et al., 2017). Borges et al. found opening pressures of 60 cmH2O in patients with ARDS to be common, with coexistence of areas opening at lower and higher pressures in the majority of patients (Borges et al., 2006). In clinical practice, maximum airway pressure is often selected based on the hemodynamic fragility of the patient rather than the expected pressure needed for lung recruitment (Santos et al., 2015). This might explain why CT compared with LUS and EIT studies revealed higher recruitment pressures as transfer for CT imaging requires more hemodynamically stable patients (Constantin et al., 2019). Recent RCTs suggest airway pressures above 50 cm H2O to be associated with serious adverse events, even when the patient is exposed to it for a short period of time (Cavalcanti et al., 2017; Hodgson et al., 2019). As the different components that attribute to the compliance of the respiratory system (compliance of the lung and chest wall as well as intra-abdominal pressure) cannot be easily separated in clinical practice (Umbrello and Chiumello, 2018), assessing the RMs' effect with imaging techniques is important in clinical practice. Rather than defining the pressure at which the lung can be opened, it is more important to determine whether recruitment can be achieved with moderate airway pressures. In other words, when comparing patients with a similar expected risk of side effects due to a transient increase in inspiratory pressures, a patient who responds to the RM with reaeration of previously collapsed lung tissue may still benefit, but a patient without this response may not.
This review also revealed several challenges associated with the quantification of lung re-aeration with image technics: there is poor agreement between imaging techniques, and there is no universal definition of recruitment response. Chiumello et al. found poor agreement between CT and LUS with respect to assessment of re-aeration, which is not unexpected since LUS is a semiquantitative method assessing only the subpleural areas (Chiumello et al., 2018). Furthermore, the role of LUS in assessing overdistention is currently unknown (Bouhemad et al., 2015). Pleural line displacement identified with LUS, as well the number of A-lines are relevant indexes that are currently being studied (Martins and Nogué, 2020; Tonelotto et al., 2020). EIT quantifies collapsed lung units based on local changes in compliance (Costa et al., 2009). However, compliance might be more related to the improvement or deterioration of already ventilated lung units than the real recruitment of atelectatic lung units (Chiumello et al., 2016). Even though CT is considered the gold standard in detecting lung recruitment, defining the degree of re-aeration remains challenging. Potentially recruitable lung tissue, determined by CT, is mainly expressed as percentage of total lung volume since absolute values depend on lung dimensions. However, expressing recruitment as percentage implies mathematical coupling with the total atelectatic volume, which is at least debatable (de Matos et al., 2012). Gattinoni et al. introduced the terms “high” and “low” recruitment responders based on the median percentage of potentially recruitable lung tissue determined by CT (Gattinoni et al., 2006). Worth mentioning, different median percentages of potentially recruitable tissue were reported in later studies (Camporota et al., 2019; de Matos et al., 2012), probably due to heterogeneity in inclusion characteristics and application of various maximum airway pressures. Given that recruitment is a continuous spectrum that depends on applied airway pressure and several imaging characteristics, speaking about “responders” from “non-responders” is a false dichotomization.
We set out to determine the role of imaging techniques in predicting the lung response to RM. The main strength of this review is the systematic and integrative approach. We excluded studies that based assessment of recruitment on mechanical or oxygenation variables as those can be influenced by factors other than recruitment of lung tissue, which is also known as the recruitment paradox (Amato and De Santis Santiago, 2016). We also acknowledge several limitations. First, we had to perform secondary analyses of many included studies as they were not intended to quantify potential for lung re-aeration, limiting statistical comparisons between groups. Second, we did not directly compare imaging techniques. Each method has intrinsic limitations, such as visualization of the subpleural region only for LUS and the need for patient transport for CT, which justify preferential use of one technique over another in specific situations. Of note, the definition and method of recruitment varied between studies even when the same image technique was used, which made direct comparisons impossible. Third, given the undefined role of LUS and EIT in the assessment of recruitment, a significant number of trials had an unclear risk of bias.
All features predictive of increased lung re-aeration after RM are consistent with a non-focal morphology of ARDS. Patients with focal ARDS lack, by definition, ventral consolidations not limited to the subpleural space and show a heterogeneous distribution of consolidation with less opening and closing, which renders them very unlikely to be recruitable. In line with this notion, patients with non-focal morphology were typically recruitable, while patients with focal morphology were not (Puybasset et al., 2000; Constantin et al., 2010). Notably, atelectasis is usually located in the dorsal lung areas in patients without lung injury requiring invasive mechanical ventilation (Longo et al., 2017; Pereira et al., 2018) implying a “focal” morphology. This may explain the lack of RM efficiency to increase lung aeration in invasively ventilated patients in the operating room (Généreux et al., 2020). Although the results of this review are not conclusive for patients without ARDS, it stresses the need for further research into lung morphology and its relation to lung re-aeration with robust imaging technics in these patients.
By integrating data from multiple studies to morphological classifications, we present a framework used to better design and interpret future studies. We have to acknowledge that this classification is imperfect, as one EIT study that only included three patients suggested that predominant ventilation in the non-dependent areas predicted recruitment, while this is not a feature that is consistent with non-focal morphology of ARDS. The relation between re-aeration and improvement in ventilation perfusion mismatch and heart function was not evaluated in this review (Karbing et al., 2020). Furthermore, in this review, we investigated the imaging techniques' role in predicting RM effects in deeply sedated patients without considering the optimal level of PEEP that would be required after recruitment to keep the lung open. Rather than a final classification, we suggest that the morphological classification is a good starting point to further improve from, with the addition of other predictors. Furthermore, more attention should be drawn to the quantification of overdistention rather than measurement of re-aeration alone. Balancing the assessment of negative and positive effects may improve our understanding as to what patients may or may not benefit from RMs.
Conclusions
We conclude that defining positive response to RMs using imaging techniques is challenging and not yet well-elucidated. Variations in RM method, population selection, as well as different imaging techniques should be taken into consideration in future studies. Given the adverse events associated with high maximum airway pressures, only the lungs of specific patients can be re-aerated with moderate maximum airway pressures. Lung ultrasound and CT characteristics consistent with non-focal morphology of ARDS are predictive of more re-aeration in response to recruitment maneuver. The morphological characteristics related to successful response to RMs in patients without ARDS have not been studied to date.
Data Availability Statement
The original contributions presented in the study are included in the article/Supplementary Material, further inquiries can be directed to the corresponding author/s.
Author Contributions
CP performed the literature search, drafted the manuscript, and approved the submitted version of the manuscript. MRS, LH, NH, MH, and FP revised the manuscript for critical content and approved the submitted version of the manuscript. MJS and LB conceived the study, revised the manuscript for critical content, and approved the submitted version of the manuscript. All authors contributed to the article and approved the submitted version.
Conflict of Interest
The authors declare that the research was conducted in the absence of any commercial or financial relationships that could be construed as a potential conflict of interest.
Supplementary Material
The Supplementary Material for this article can be found online at: https://www.frontiersin.org/articles/10.3389/fphys.2021.666941/full#supplementary-material
References
Amato, M. B. P., and De Santis Santiago, R. R. (2016). The recruitability paradox. Am. J. Respir. Crit. Care Med. 193, 1192–1194. doi: 10.1164/rccm.201601-0178ED
Borges, J. B., Okamoto, V. N., Matos, G. F., Caramez, M. P., Arantes, P. R., Barros, F., et al. (2006). Reversibility of lung collapse and hypoxemia in early acute respiratory distress syndrome. Am. J. Respir. Crit. Care Med. 174, 268–278. doi: 10.1164/rccm.200506-976OC
Bouhemad, B., Brisson, H., Le-Guen, M., Arbelot, C., Lu, Q., and Rouby, J. J. (2011). Bedside ultrasound assessment of positive end-expiratory pressure-induced lung recruitment. Am. J. Respir. Crit. Care Med. 183, 341–347. doi: 10.1164/rccm.201003-0369OC
Bouhemad, B., Mongodi, S., Via, G., and Rouquette, I. (2015). Ultrasound for “lung monitoring” of ventilated patients. Anesthesiology 122, 437–447. doi: 10.1097/ALN.0000000000000558
Caironi, P., Carlesso, E., Cressoni, M., Chiumello, D., Moerer, O., Chiurazzi, C., et al. (2015). Lung recruitability is better estimated according to the Berlin definition of acute respiratory distress syndrome at standard 5 cm H2O rather than higher positive end-expiratory pressure: a retrospective cohort study. Crit. Care Med. 43, 781–790. doi: 10.1097/CCM.0000000000000770
Caironi, P., Cressoni, M., Chiumello, D., Ranieri, M., Quintel, M., Russo, S. G., et al. (2010). Lung opening and closing during ventilation of acute respiratory distress syndrome. Am. J. Respir. Crit. Care Med. 181, 578–586. doi: 10.1164/rccm.200905-0787OC
Camporota, L., Caricola, E. V., Bartolomeo, N., Di Mussi, R., Wyncoll, D. L. A., Meadows, C. I. S., et al. (2019). Lung recruitability in severe acute respiratory distress syndrome requiring extracorporeal membrane oxygenation. Crit. Care Med. 47,1177–1183. doi: 10.1097/CCM.0000000000003837
Cavalcanti, A. B., Suzumura, É. A., Laranjeira, L. N., Paisani, D. M., Damiani, L. P., Guimarães, H. P., et al. (2017). Effect of lung recruitment and titrated Positive End-Expiratory Pressure (PEEP) vs low PEEP on mortality in patients with acute respiratory distress syndrome - a randomized clinical trial. JAMA 318, 1335–1345. doi: 10.1001/jama.2017.14171
Chen, L., Del Sorbo, L., Grieco, D. L., Junhasavasdikul, D., Rittayamai, N., Soliman, I., et al. (2019). Potential for lung recruitment estimated by the recruitment-to-inflation ratio in acute respiratory distress syndrome. Am. J. Respir. Crit. Care Med. 201, 178–187. doi: 10.1164/rccm.201902-0334OC
Chiumello, D., Marino, A., Brioni, M., Cigada, I., Menga, F., Colombo, A., et al. (2016). Lung recruitment assessed by respiratory mechanics and computed tomography in patients with acute respiratory distress syndrome what is the relationship? Am. J. Respir. Crit. Care Med. 193, 1254–1263. doi: 10.1164/rccm.201507-1413OC
Chiumello, D., Mongodi, S., Algieri, I., Vergani, G. L., Orlando, A., and Via, G. (2018). Assessment of lung aeration and recruitment by ct scan and ultrasound in acute respiratory distress syndrome patients*. Crit. Care Med. 46, 1761–1768. doi: 10.1097/CCM.0000000000003340
Constantin, J. M., Grasso, S., Chanques, G., Aufort, S., Futier, E., and Sebbane, M. (2010). Lung morphology predicts response to recruitment maneuver in patients with acute respiratory distress syndrome. Crit. Care Med. 38, 1108–1117. doi: 10.1097/CCM.0b013e3181d451ec
Constantin, J. M., Jabaudon, M., Lefrant, J. Y., Jaber, S., Quenot, J. P., Langeron, O., et al. (2019). Personalised mechanical ventilation tailored to lung morphology versus low positive end-expiratory pressure for patients with acute respiratory distress syndrome in France (the LIVE study): a multicentre, single-blind, randomised controlled trial. Lancet Respir. Med. 7, 870–880. doi: 10.1016/S2213-2600(19)30138-9
Costa, E. L., Borges, J. B., Melo, A., Suarez-Sipmann, F., Toufen, C. Jr, Bohm, S. H., et al. (2009). Bedside estimation of recruitable alveolar collapse and hyperdistension by electrical impedance tomography. Intens. Care Med. 35, 1132–1137. doi: 10.1007/s00134-009-1447-y
Cressoni, M., Chiumello, D., Algieri, I., Brioni, M., Chiurazzi, C., Colombo, A., et al. (2017). Opening pressures and atelectrauma in acute respiratory distress syndrome. Intens. Care Med. 43, 603–611. doi: 10.1007/s00134-017-4754-8
de Matos, G. F., Stanzani, F., Passos, R. H., Fontana, M. F., Albaladejo, R., and Caserta, R. E. (2012). How large is the lung recruitability in early acute respiratory distress syndrome: a prospective case series of patients monitored by computed tomography. Crit. Care 16:R4. doi: 10.1186/cc10602
Eichler, L., Mueller, J., Grensemann, J., Frerichs, I., Zöllner, C., and Kluge, S. (2018). Lung aeration and ventilation after percutaneous tracheotomy measured by electrical impedance tomography in non-hypoxemic critically ill patients: a prospective observational study. Ann. Intens. Care 8:110. doi: 10.1186/s13613-018-0454-y
Eronia, N., Mauri, T., Maffezzini, E., Gatti, S., Bronco, A., Alban, L., et al. (2017). Bedside selection of positive end-expiratory pressure by electrical impedance tomography in hypoxemic patients: a feasibility study. Ann. Intens. Care 7:76. doi: 10.1186/s13613-017-0299-9
Gattinoni, L., Caironi, P., Cressoni, M., Chiumello, D., Ranieri, V. M., Quintel, M., et al. (2006). Lung recruitment in patients with the acute respiratory distress syndrome. N. Engl. J. Med. 354, 1775–1786. doi: 10.1056/NEJMoa052052
Gattinoni, L., Carlesso, E., and Caironi, P. (2012). Stress and strain within the lung. Curr. Opin. Crit. Care 18, 42–47. doi: 10.1097/MCC.0b013e32834f17d9
Gattinoni, L., Collino, F., Maiolo, G., Rapetti, F., Romitti, F., Tonetti, T., et al. (2017). Positive end-expiratory pressure: How to set it at the individual level. Ann. Transl. Med. 5:288.
Gattinoni, L., Collino, F., Maiolo, G., Rapetti, F., Romitti, F., Tonetti, T., et al. (2017). Positive end-expiratory pressure: how to set it at the individual level. Ann. Transl. Med. 5:288. doi: 10.21037/atm.2017.06.64
Gattinoni, L., Marini, J. J., and Quintel, M. (2020). Recruiting the acutely injured lung: how and why? Am. J. Respir. Crit. Care Med. 201, 130–132. doi: 10.1164/rccm.201910-2005ED
Généreux, V., Chassé, M., Girard, F., Massicotte, N., Chartrand-Lefebvre, C., and Girard, M. (2020). Effects of positive end-expiratory pressure/recruitment manoeuvres compared with zero end-expiratory pressure on atelectasis during open gynaecological surgery as assessed by ultrasonography: a randomised controlled trial. Br. J. Anaesth. 124, 101–109. doi: 10.1016/j.bja.2019.09.040
Godet, T., Constantin, J. M., Jaber, S., and Futier, E. (2015). How to monitor a recruitment maneuver at the bedside. Curr. Opin. Crit. Care 21, 253–258. doi: 10.1097/MCC.0000000000000195
He, H., Chi, Y., Long, Y., Yuan, S., Frerichs, I., Möller, K., et al. (2020). Influence of overdistension/recruitment induced by high positive end-expiratory pressure on ventilation-perfusion matching assessed by electrical impedance tomography with saline bolus. Crit. Care. 29:586. doi: 10.1186/s13054-020-03301-x
Hedley-Whyte, J., Laver, M. B., and Bendixen, H. H. (1964). Effect of changes in tidal ventilation on physiologic shunting. Am. J. Physiol. 206, 891–897. doi: 10.1152/ajplegacy.1964.206.4.891
Hes, D. R. (2015). Recruitment maneuvers and PEEP titration. Respir. Care 60, 1688–1704. doi: 10.4187/respcare.04409
Hodgson, C. L., Cooper, D. J., Arabi, Y., King, V., Bersten, A., Bihari, S., et al. (2019). Maximal recruitment open lung ventilation in acute respiratory distress syndrome (PHARLAP): a phase II. Multicenter randomized controlled clinical trial. Am. J. Respir. Crit. Care Med. 200, 1363–1372. doi: 10.1164/rccm.201901-0109OC
Karbing, D. S., Panigada, M., Bottino, N., Spinelli, E., Protti, A., Rees, S. E., et al. (2020). Changes in shunt, ventilation/perfusion mismatch, and lung aeration with PEEP in patients with ARDS: a prospective single-arm interventional study. Crit. Care 24, 1–13. doi: 10.1186/s13054-020-2834-6
Karsten, J., Voigt, N., Gillmann, H.-J., and Stueber, T. (2019). Determination of optimal positive end-expiratory pressure based on respiratory compliance and electrical impedance tomography: a pilot clinical comparative trial. Biomed. Eng. Biomed. Tech. 64, 135–145. doi: 10.1515/bmt-2017-0103
Lapinsky, S. E., and Mehta, S. (2005). Bench-to-bedside review: recruitment and recruiting maneuvers. Crit Care 9, 60–65. doi: 10.1186/cc2934
Liberati, A., Altman, D. G., Tetzlaff, J., Mulrow, C., Gøtzsche, P. C., Ioannidis, J. P., et al. (2009). The PRISMA statement for reporting systematic reviews and meta-analyses of studies that evaluate health care interventions: explanation and elaboration. PLoS Med. 21:e1000100. doi: 10.1371/journal.pmed.1000100
Longo, S., Siri, J., Acosta, C., Palencia, A., Echegaray, A., Chiotti, I., et al. (2017). Lung recruitment improves right ventricular performance after cardiopulmonary bypass: a randomised controlled trial. Eur. J. Anaesthesiol. 34, 66–74. doi: 10.1097/EJA.0000000000000559
Mancebo, J., Mercat, A., and Brochard, L. (2019). Maximal lung recruitment in ARDS: a nail in the coffin. Am. J. Respir. Crit. Care Med. 200, 1331–1333. doi: 10.1164/rccm.201908-1615ED
Martins, S. R., and Nogué, R. (2020). Vertical displacement of pleura: a new method for bronchospasm evaluation? Ultrasound J. 12, 10–13. doi: 10.1186/s13089-020-00184-5
Neumann, P., Rothen, H. U., Berglund, J. E., Valtysson, J., Magnusson, A., and Hedenstierna, G. (1999). Positive end-expiratory pressure prevents atelectasis during general anaesthesia even in the presence of a high inspired oxygen concentration. Acta Anaesthesiol. Scand. 43, 295–301. doi: 10.1034/j.1399-6576.1999.430309.x
Nieszkowska, A., Lu, Q., Vieira, S., Elman, M., Fetita, C., and Rouby, J. J. (2004). Incidence and regional distribution of lung overinflation during mechanical ventilation with positive end-expiratory pressure. Crit. Care Med. 32, 1496–1503. doi: 10.1097/01.CCM.0000130170.88512.07
Pereira, S. M., Tucci, M. R., Morais, C. C. A., Simões, C. M., Tonelotto, B. F. F., Pompeo, M. S., et al. (2018). Individual positive end-expiratory pressure settings optimize intraoperative mechanical ventilation and reduce postoperative atelectasis. Anesthesiology 129, 1070–1081. doi: 10.1097/ALN.0000000000002435
Puybasset, L., Gusman, P., Muller, J.-C., Cluzel, P., Coriat, P., Rouby, J.-J., et al. (2000). Regional distribution of gas and tissue in acute respiratory distress syndrome. III. Consequences for the effects of positive end-expiratory pressure. Intens. Care Med. 26, 1215–1227. doi: 10.1007/s001340051340
Rocco, P. R., Pelosi, P., and De Abreu, M. G. (2010). Pros and cons of recruitment maneuvers in acute lung injury and acute respiratory distress syndrome. Expert Rev. Respir. Med. 4, 479–489. doi: 10.1586/ers.10.43
Rode, B., Vučić, M., Siranović, M., Horvat, A., Krolo, H., Kelečić, M., et al. (2012). Positive end-expiratory pressure lung recruitment: comparison between lower inflection point and ultrasound assessment. Wien Klin Wochenschr. 124, 842–847. doi: 10.1007/s00508-012-0303-1
Sahetya, S. K., and Brower, R. G. (2017). Lung recruitment and titrated PEEP in moderate to severe ARDS. JAMA 318:1327. doi: 10.1001/jama.2017.13695
Santos, R. S., Silva, P. L., Pelosi, P., and Rocco, P. R. (2015). Recruitment maneuvers in acute respiratory distress syndrome: the safe way is the best way. World J. Crit. Care Med. 4, 278–286 doi: 10.5492/wjccm.v4.i4.278
Tang, K. Q., Yang, S. L., Zhang, B., Liu, H. X., Ye, D. Y., Zhang, H. Z., et al. (2017). Ultrasonic monitoring in the assessment of pulmonary recruitment and the best positive end-expiratory pressure. Medicine 96:e8168. doi: 10.1097/MD.0000000000008168
Tonelotto, B., Pereira, S. M., Tucci, M. R., Vaz, D. F., Vieira, J. E., Malbouisson, L. M., et al. (2020). Intraoperative pulmonary hyperdistention estimated by transthoracic lung ultrasound: a pilot study. Anaesth. Crit. Care Pain Med. 39, 825–831. doi: 10.1016/j.accpm.2020.09.009
Umbrello, M., and Chiumello, D. (2018). Interpretation of the transpulmonary pressure in the critically ill patient. Ann. Transl. Med. 6, 383–383. doi: 10.21037/atm.2018.05.31
Vieira, S. R., Puybasset, L., Lu, Q., Richecoeur, J., Cluzel, P., Coriat, P., et al. (1999). A scanographic assessment of pulmonary morphology in acute lung injury: significance of the lower inflection point detected on the lung pressure- volume curve. Am. J. Respir. Crit. Care Med. 159, 1612–1623. doi: 10.1164/ajrccm.159.5.9805112
Whiting, P. F. (2011). QUADAS-2: a revised tool for the quality assessment of diagnostic accuracy studies. Ann. Intern. Med. 155:529. doi: 10.7326/0003-4819-155-8-201110180-00009
Keywords: electrical impedance tomography, computed tomography, lung ultrasound, overdistention, recruitment maneuvers, ARDS
Citation: Pierrakos C, Smit MR, Hagens LA, Heijnen NFL, Hollmann MW, Schultz MJ, Paulus F and Bos LDJ (2021) Assessment of the Effect of Recruitment Maneuver on Lung Aeration Through Imaging Analysis in Invasively Ventilated Patients: A Systematic Review. Front. Physiol. 12:666941. doi: 10.3389/fphys.2021.666941
Received: 11 February 2021; Accepted: 20 April 2021;
Published: 04 June 2021.
Edited by:
Lorenzo Ball, University of Genoa, ItalyReviewed by:
Carmen Silvia Valente Barbas, University of São Paulo, BrazilAlysson Roncally Silva Carvalho, University of Porto, Portugal
Copyright © 2021 Pierrakos, Smit, Hagens, Heijnen, Hollmann, Schultz, Paulus and Bos. This is an open-access article distributed under the terms of the Creative Commons Attribution License (CC BY). The use, distribution or reproduction in other forums is permitted, provided the original author(s) and the copyright owner(s) are credited and that the original publication in this journal is cited, in accordance with accepted academic practice. No use, distribution or reproduction is permitted which does not comply with these terms.
*Correspondence: Charalampos Pierrakos, charalampos.pierrakos@chu-brugmann.be