- 1University Outpatient Clinic, Sports Medicine and Orthopedics, University of Potsdam, Potsdam, Germany
- 2Faculty of Health Sciences, University of Potsdam, Potsdam, Germany
Background: The relationship between exercise-induced intratendinous blood flow (IBF) and tendon pathology or training exposure is unclear.
Objective: This study investigates the acute effect of running exercise on sonographic detectable IBF in healthy and tendinopathic Achilles tendons (ATs) of runners and recreational participants.
Methods: 48 participants (43 ± 13 years, 176 ± 9 cm, 75 ± 11 kg) performed a standardized submaximal 30-min constant load treadmill run with Doppler ultrasound “Advanced dynamic flow” examinations before (Upre) and 5, 30, 60, and 120 min (U5-U120) afterward. Included were runners (>30 km/week) and recreational participants (<10 km/week) with healthy (Hrun, n = 10; Hrec, n = 15) or tendinopathic (Trun, n = 13; Trec, n = 10) ATs. IBF was assessed by counting number [n] of intratendinous vessels. IBF data are presented descriptively (%, median [minimum to maximum range] for baseline-IBF and IBF-difference post-exercise). Statistical differences for group and time point IBF and IBF changes were analyzed with Friedman and Kruskal-Wallis ANOVA (α = 0.05).
Results: At baseline, IBF was detected in 40% (3 [1–6]) of Hrun, in 53% (4 [1–5]) of Hrec, in 85% (3 [1–25]) of Trun, and 70% (10 [2–30]) of Trec. At U5 IBF responded to exercise in 30% (3 [−1–9]) of Hrun, in 53% (4 [−2–6]) of Hrec, in 70% (4 [−10–10]) of Trun, and in 80% (5 [1–10]) of Trec. While IBF in 80% of healthy responding ATs returned to baseline at U30, IBF remained elevated until U120 in 60% of tendinopathic ATs. Within groups, IBF changes from Upre-U120 were significant for Hrec (p < 0.01), Trun (p = 0.05), and Trec (p < 0.01). Between groups, IBF changes in consecutive examinations were not significantly different (p > 0.05) but IBF-level was significantly higher at all measurement time points in tendinopathic versus healthy ATs (p < 0.05).
Conclusion: Irrespective of training status and tendon pathology, running leads to an immediate increase of IBF in responding tendons. This increase occurs shortly in healthy and prolonged in tendinopathic ATs. Training exposure does not alter IBF occurrence, but IBF level is elevated in tendon pathology. While an immediate exercise-induced IBF increase is a physiological response, prolonged IBF is considered a pathological finding associated with Achilles tendinopathy.
Introduction
Intratendinous blood flow (IBF) and degenerative tendon changes are a common finding in long-distance runners with Achilles tendinopathy (Hirschmüller et al., 2010). In earlier studies IBF has been associated with ingrowth of neo-innervation in a failed tendon healing process and the onset of tendon pain (Alfredson et al., 2003; Rees et al., 2014). More recent studies, however, have reported no direct association of IBF with pain severity or functional impairment (de Vos et al., 2007; De Jonge et al., 2014; De Marchi et al., 2018). By use of high-resolution ultrasound devices and increasing sensitivity to low flow (Koenig et al., 2010; Risch et al., 2016), IBF has also become detectable in up to 35% of asymptomatic Achilles tendons (ATs) (Boesen et al., 2006b; Hirschmüller et al., 2010). A small amount of IBF at rest has been suggested to be of physiological nature (Boesen et al., 2006a,b, 2012). Moreover, IBF has been found to increase in response to tendon loading exercise (Boesen et al., 2006a,b; Fahlström and Alfredson, 2010; Sanz-López et al., 2016; Risch et al., 2018a) assuming a physiological metabolic reaction visualized due to increased flow velocity and/or volume in pre-existing vessels (Boesen et al., 2006a,b, 2012; Risch et al., 2018a). In summary, detectability of IBF itself cannot be considered a pathological finding anymore (Boesen et al., 2006b, 2012; Risch et al., 2018a).
In athletes, several studies have reported an exercise-induced increase of IBF suggesting an adaptation to continuous high strain and mechanical loading (Boesen et al., 2006a, 2012; Malliaras et al., 2008) associated with increased metabolic activity and regeneration (remodeling/repair) (Malliaras et al., 2008; Koenig et al., 2010; Tardioli et al., 2012). This increased metabolic response, has been already reported in the context of elevated collagen synthesis and tendon hypertrophy following strenuous activity (Couppe et al., 2008; Boesen et al., 2011). Increased presence of IBF has been associated with greater hours of training and higher amount of training years, suggesting a long-term adaptation to tendon loading in athletes (Malliaras et al., 2008; Boesen et al., 2012). Then again, the amount of training hours has also been associated with an increased risk of developing Achilles tendinopathy (Knobloch et al., 2008). An acute increase in amount of sonographic detectable intratendinous vessels following exercise has been suggested to represent elevated blood flow in preexisting vessel beds, rising above the sensitivity threshold of Doppler ultrasound (Boesen et al., 2006b; Risch et al., 2018a). Other studies, however, have found contradictory effects of exercise on detectable IBF in terms of persisting, decreased (Malliaras et al., 2012) or absent blood flow (Pingel et al., 2013b). Due to varying sensitivity and imaging quality of ultrasound devices (Boesen et al., 2012), lacking standardization of exercise and examination protocols as well as inconsistent tendon characterization (healthy, tendinopathy, degenerative changes), comparability of previous studies and interpretation of differing results is limited (Risch et al., 2018a). Consequently, the diagnostic value of sonographic detectable IBF considering a pathological or physiological finding remains inconclusive. To differentiate between normal and abnormal Doppler flow, a cut-off value of 1–2 vessels has been proposed, but so far lacks sufficient evidence (Koenig et al., 2010; Boesen et al., 2012).
It remains questionable to what extent training status as well as healthy or pathological tendon condition and symptoms affect detectability of exercise-induced IBF. Therefore, the aim of this study was to investigate the response of sonographic detectable IBF to a standardized acute bout of constant load running exercise in healthy and tendinopathic ATs of runners and recreational participants. This study is the first to examine the acute sonographic detectable IBF response as well as IBF recovery in subsequent ultrasound examinations up to 2 h following exercise. It was hypothesized that exercise leads to an acute, temporary increase of IBF in healthy and tendinopathic tendons. Due to the higher training exposure this response was expected to be more pronounced in runners compared to recreational participants.
Materials and Methods
Study Design
In this cross-sectional study, all participants were assessed on two measurement days (M1/M2) separated by at least 48 h. M1 consisted of a maximum incremental treadmill running test to determine the individual anaerobic threshold (IAT). On M2 participants performed 30 min of constant load treadmill running with an intensity 5% below the IAT, and Doppler ultrasound (DU) examinations directly before (Upre) and 5, 30, 60, and 120 min after exercise (U5, U30, U60, U120, Figure 1). The study protocol has been validated in a prior pilot study (Risch et al., 2018a) and was approved by the local ethics committee.

Figure 1. Study design. ME, medical examination; VISA-A, Victorian Institute of Sports Assessment-Achilles Questionnaire; Upre-U120, Ultrasound examinations at baseline and 5, 30, 60, and 120 min after exercise; IAT, individual anaerobic threshold.
Participants
Fifty participants were included in this study between August 2015 and October 2018 after signing a written informed consent. Two participants dropped out of the study after M1, one healthy recreational participant due to an acute onset of low back pain and one recreational participant with tendinopathy due to a recurrent onset of intense Achilles tendon pain. Finally, forty-eight participants (43 ± 13 years, 176 ± 9 cm, 75 ± 11 kg) were included in the analysis. Participants were recruited from the university outpatient clinic and a local running club. Inclusion criteria were 30 to 65 years of age, with (a) presence of clinically diagnosed mid-portion Achilles tendinopathy and sonographic evident degenerative changes (focal thickening or hypo- and/or hyperechogenic areas) with a running minimum of 30 km/week (Clermont et al., 2019) (Trun, n = 13) or being recreationally active (<10 km running/week, Trec, n = 10) or (b) healthy, asymptomatic ATs (no acute or past history of AT pain or sonographic signs of tendon pathology) with a running minimum of 30 km/week (Hrun, n = 10) or being recreationally active (<10 km running/week, maximum of two recreational training session/week, Hrec, n = 15) (Figure 2 and Table 1). Patients with a history of systemic diseases (e.g., cardiovascular, metabolic or rheumatic disease, hypercholesterolemia), previous AT ruptures (partial or complete tear), previous injections (i.e., corticosteroid or platelet-rich plasma), or surgical interventions of the AT were excluded from the study. Prior to the first measurement all participants received a medical examination by a sports medicine physician (medical history and orthopedic physical examination, resting-ECG) as well as an ultrasound examination of both ATs to ensure correct inclusion and group allocation. Groups were matched for age and gender to account for the evident effect on presence of IBF and Achilles tendinopathy (Boesen et al., 2006b; Malliaras et al., 2012; Wezenbeek et al., 2018). All participants had to avoid physical activity 24 h prior to each measurement to prevent an effect of exercise on the baseline examination (de Vos et al., 2007; Sengkerij et al., 2009). Furthermore, all participants completed the Victorian Institute of Sports Assessment-Achilles (VISA-A) questionnaire to assess severity of Achilles tendinopathy (Robinson et al., 2001).
Exercise Protocol
All running tasks were performed on the same treadmill (Pulsar, h/p/cosmos Sports & Medical, Nussdorf-Traunstein, Germany) with a constant incline of 0.4%, validated to be comparable to outdoor running (Mugele et al., 2018). To determine the IAT on M1, participants performed a stepwise incremental exercise test starting with 4–6 km/h with increments of 1–2 km/h every 3 min until voluntary exhaustion. Starting velocity and increase of stepwise increments (1 or 2 km/h) were adapted to the participants estimated performance capacity. Blood lactate was assessed by taking blood samples from the earlobe at baseline and every 3 min during the test. Heart rate was assessed simultaneously with a chest strap. IAT was determined using the Dickhuth threshold concept (Dickhuth et al., 1999). On M2, participants performed a 30 min constant load running task at a speed 5% below the IAT (IAT – 5% of max. running velocity during incremental exercise test) with a prior 9 min running warm-up starting with 4–6 km/h and an incremental increase of running velocity every 3 min up to the constant load velocity (Moser et al., 2015). The IAT was used to determine the individual running velocity in each participant to account for different training levels and still ensure comparable but individual exercise intensity for a standardized time period. Blood lactate and heart rate were assessed at baseline and every 10 min during constant load running to control for steady state intensity. After running patients were asked to rate their perceived exertion using the Borg-scale (6–20) (Borg, 1982).
Ultrasound Examinations
All ultrasound examinations were performed by the same investigator applying a high-resolution ultrasound device (Xario SSA-660 A, Toshiba, Japan) with a multi-frequency linear transducer at 14 MHz (PLT-120 AT). Participants were examined in prone position with their feet hanging over the distal end of the examination table. On M1, tendon structure (hypo-/hyperechogenic alterations) and tendon anterior-posterior thickness at the thickest location (Fredberg et al., 2008) was assessed in longitudinal scans using B-mode (gain = 80, DR = 65, penetration depth = 3 cm, focus = 0.5 cm), the ankle being passively flexed at 90° angle to the tibia to avoid tendon waving (Fredberg et al., 2008). Doppler ultrasound examinations on M2 were performed with the feet hanging free and relaxed and applying minimal probe pressure to avoid obliteration of vessels (de Vos et al., 2007; Sengkerij et al., 2009; Cassel et al., 2018; Risch et al., 2018a). IBF was assessed with the broadband Doppler ultrasound “Advanced Dynamic Flow” (region of interest 2 × 1 cm, pre-settings: color gain 42, color velocity 1.5 cm/s, pulse repetition frequency 13.7 kHz) saving video sequences of 5 s as avi files (Figure 3). Each examination at Upre-U120 took approximately 5 min. At each measurement time point, ADF-scans were obtained from left and right AT in randomized order, assessing IBF throughout each tendon ranging from the calcaneal insertion to the musculo-tendinous junction of the soleus muscle. Tendons were scanned in medio-lateral direction gradually moving from distal to proximal, recording video sequences while following the course of intratendinous vessels whenever they were detected. IBF was quantified in dynamic scans by counting the total number of vessels [n] (every branch counting as one vessel) throughout each tendon (Risch et al., 2018a,b). The applicability as well as settings and procedure have been tested for validity and reliability in previous studies (Risch et al., 2016, 2018a,b). Doppler examination using “Advanced Dynamic Flow” has revealed high consistency compared with conventional Doppler ultrasound and good reliability of IBF assessment also for low level of observer experience (Risch et al., 2016). The grading procedure (counting number of vessels) has revealed high intra-observer test-retest agreement with a standard error of measurement of 0.99–1.47 vessels (Risch et al., 2018b).
Data and Statistical Analysis
Anthropometric, exercise data, and tendon thickness measures are presented descriptively (mean ± SD). After testing for normal distribution (Shapiro-Wilk), group differences were analyzed using one-way ANOVA and Tukey-Kramer HSD post hoc test (SPSS Statistics 25 and Jmp 9.0). Since IBF data is not normally distributed within groups, IBF is presented descriptively (% of examined ATs, median and minimum-to-maximum range, and boxplots with interquartile range) for each group and measurement time point. Main effects for intra- and inter-group comparison were analyzed with Friedman and Kruskal-Wallis ANOVA (Wilcoxon test and Mann-Whitney-U test for post hoc analysis. Distribution of responding and non-responding ATs in the four groups was compared with contingency table and chi-square analysis. An association between amount of IBF at rest and amount of increase in U5 was analyzed using the Pearson correlation coefficient. Due to inter-individual variability of IBF amount no further statistical testing was performed. Significance level was set to α = 0.05.
After finding no statistically significant side differences between left and right tendon IBF amount in Hrec and Hrun, only right tendons are presented in comparison to the symptomatic tendon of Trec and Trun. In case of bilateral AT pathology in Trec and Trun, ATs with leading/more severe symptoms according to the clinical examination are considered the symptomatic side.
Results
Participants
There were no statistically significant group differences for age, height, and weight whereas kilometers of running per week, VISA-A, tendon thickness measures, and constant load velocity (M2) revealed significant differences between runners and recreational participants as well as between symptomatic and asymptomatic tendons (Table 1). Duration of symptoms in Trun and Trec ranged from 2 months to more than 10 years (mean 26 months).
Exercise Testing
M1 and M2 were separated 7 ± 6 days (2 to 16 days). On M2 all participants performed a constant load running task with a mean heart rate of 158 ± 13 and mean blood lactate of 2.7 ± 1.3 mmol. Subjective perceived exhaustion (Borg scale) after constant load running was reported to be 13 ± 1 in Hrun, 15 ± 2 in Hrec, and 14 ± 2 in Trun as well as Trec (p = 0.12).
Intratendinous Blood Flow
At Upre, IBF was detected in 40% of Hrun (median 3 vessels) and in 53% of Hrec (4 vessels). At U5 IBF responded to exercise in 30% of Hrun (with a median difference to baseline of 3 vessels) and in 53% of Hrec (4 vessels) (Table 2). While the majority of responding ATs showed an exercise-induced increase, number of vessels temporarily decreased in two ATs. After 30 min, IBF returned to baseline values in 9 from 11 (80%) healthy responding ATs. A total of 60% of ATs in Hrun and 33% in Hrec remained without any detectable IBF throughout the investigation. Within groups, changes over measurement time points were significant for Hrec (p < 0.01) between Upre and U5 (p = 0.02) and between U5 and U30-U120 (p < 0.02). Exercise-induced changes of IBF in Hrun did not reach statistical significance (p = 0.80) (Table 3). At all measurement time points, the amount of IBF between groups differed significantly (p < 0.05). IBF was significantly lower in Hrun and Hrec compared to Trec (p < 0.05) and in Hrun compared to Trun (p = 0.04) at Upre-U120. Furthermore, Hrec was significantly lower than Trun at U30-U60 (p < 0.04). No significant differences were found between Hrun and Hrec (p > 0.05) (Table 3).
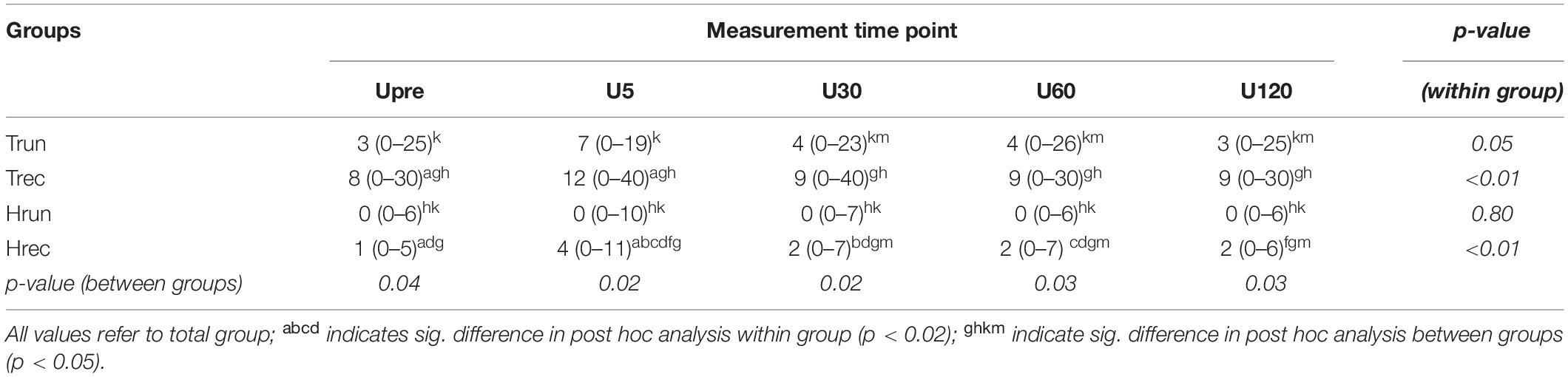
Table 3. IBF at each measurement time point (U5-U120) median [range] (value represent median for whole group).
In tendinopathic participants, baseline examination showed IBF in 85% of Trun (3 vessels), and in 70% of Trec (10 vessels). At U5 IBF responded to exercise in 70% of Trun (with a median difference to baseline of 4 vessels) and in 80% of Trec (5 vessels) (Table 2). One from 17 responding ATs showed a temporary reduction of IBF at U5. In 10 from 17 (60%) responding tendinopathic ATs IBF remained increased after U30. Some ATs remained without detectable IBF throughout the investigation (15% in Trun and 8% in Trec). Within groups, IBF changes over time were significant for Trun (p = 0.05) and Trec (p < 0.01). Post hoc analysis showed statistically significant differences only between Upre and U5 for Trec (p = 0.01) but not for Trun (p = 0.08). No significant between group differences were detected for Trun and Trec at any measurement time point (p > 0.05).
The distribution of ATs responding and not responding to exercise did not differ significantly between groups (p = 0.11). Furthermore, the amount of IBF increase was not significantly correlated to the amount of IBF at baseline (p = 0.56). Boxplots with median number of vessels and interquartile range for each group (in total) and measurement time point is presented in Figure 4. Individual courses of IBF change relative to baseline in healthy and tendinopathic Achilles tendons is presented in Figure 5.
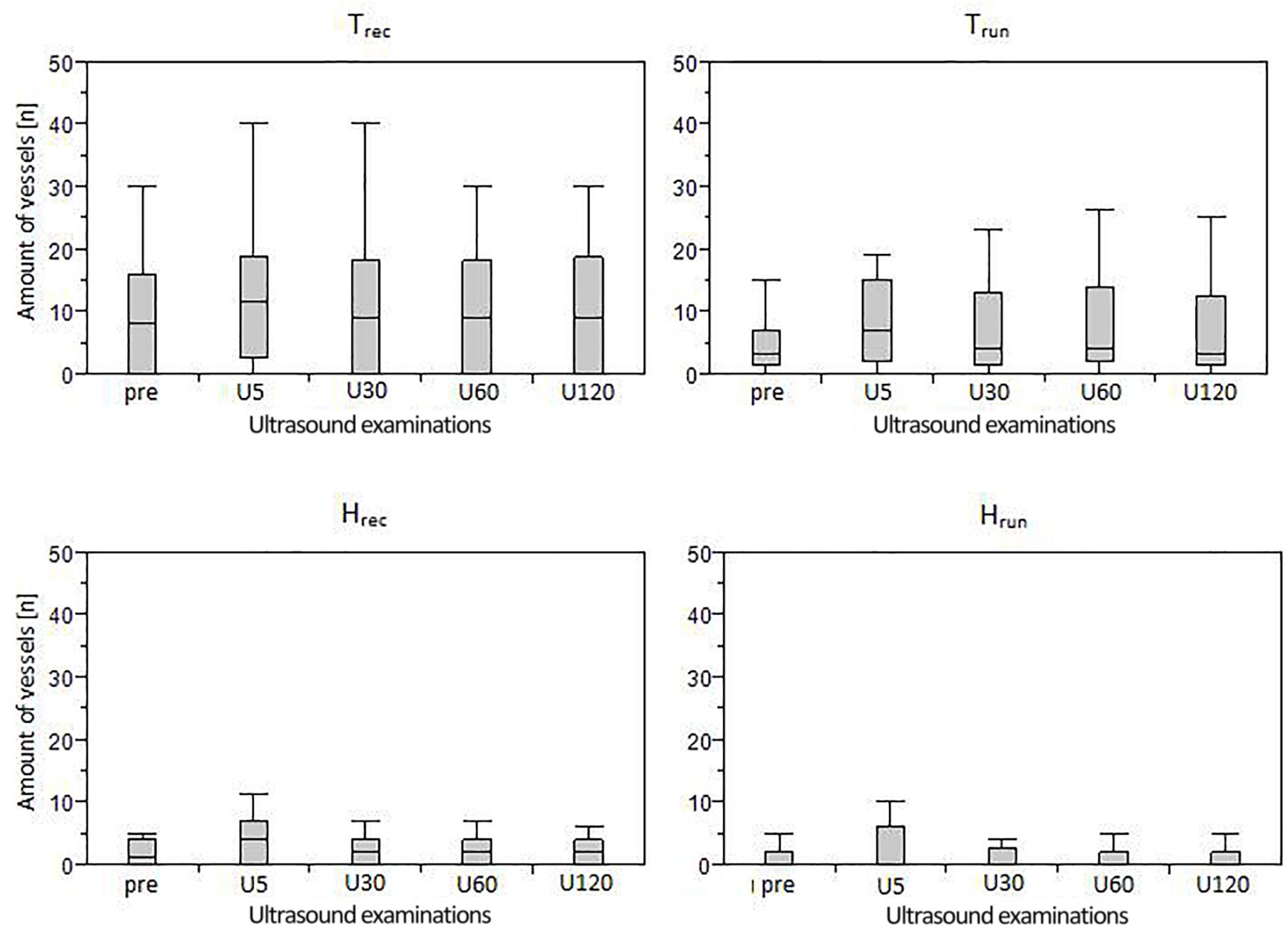
Figure 4. Boxplots (median and interquartile range) of IBF amount in each group assessed during five measurement time points (Upre-U120).
Discussion
This study investigated the acute effect of constant load running on presence of sonographic detectable IBF in runners and recreational participants with and without tendon pathology. Irrespective of training and pathology, some ATs responded with acutely increased IBF 5 min after exercise while others remained unaffected. The amount of IBF increase was similar (median of 3–5 vessels) in all four groups. The immediate increase, however, only reached the level of significance in Hrec and Trec. Contrary to the hypothesis of a pronounced reaction in athletes, runners neither revealed a significantly higher amount of IBF at rest nor following exercise compared to recreational participants. Although healthy and tendinopathic ATs showed a similar IBF increase directly after exercise, the overall amount of intratendinous vessels was significantly higher in tendinopathic compared to healthy ATs at all measurement time points. Moreover, the duration of increased IBF after exercise differed between healthy and tendinpathic ATs. While IBF increase in Hrec was only detectable 5 min after exercise and returned to baseline values within 30 min, IBF increase detected in Trec did not show a significant decrease at U30-U120.
The role of exercise-induced IBF increase in tendinopathy patients has been discussed controversially. On the one hand, IBF was assumed to represent a pathological finding and exercise was necessary to reveal total amount of vascularity (Cook et al., 2005). On the other hand, it has been discussed to be a physiological reaction associated to increased metabolic demands (e.g., oxygen demand and collagen synthesis) (Boushel et al., 2000; Kjaer et al., 2005; Boesen et al., 2006b). While an acute short-term increase directly following exercise has been considered to represent a physiological effect, a prolonged elevation seen in 60% of responding tendinopathic ATs, is hypothesized to be an indicator of morphological changes and tissue turnover and might represent a possible discrimination factor between healthy and pathological ATs. In this context, a post-exercise Doppler ultrasound examinations performed after 30 min of rest would exclude physiological exercise-induced IBF (Risch et al., 2018a). However, since the observation of a prolonged IBF response did not reach statistical significance, it requires confirmation in further investigations with a larger sample size.
Irrespective of exercise, between group comparison showed a significantly higher IBF level in tendinopathic compared to healthy ATs, which is similar to findings from Boesen et al. (2006b) and Pingel et al. (2013a). In patients with Achilles tendinopathy, occurrence of resting IBF (2 to 30 vessels) in 70–85% of ATs is in line with previous reports ranging from 50 to 88% (Tol et al., 2012). However, prospective studies have shown that the presence and amount of IBF as well as structural alterations of tendon tissue in tendinopathy patients are not necessarily associated with the degree of tendon pain and functional impairment (de Vos et al., 2007; De Jonge et al., 2014; Ryan and Cped, 2015). IBF is rather seen as part of a failed tendon healing process including matrix change and ingrowth of sensory and sympathetic nerves (Andersson et al., 2007; Tol et al., 2012). Although degenerated tendons frequently reveal higher amount of IBF at rest, examinations using microdialysis have reported high lactate levels suggesting an anaerobic metabolism in a persisting hypoxic environment (Alfredson et al., 2002; Järvinen, 2020). Therefore, Järvinen (2020) has proposed that sonographic detectable intratendinous vessels in degenerated tendons represent hypoxia-induced, non-functional neo-vessels that fail to supply tendon tissue with oxygen and nutrients required for tissue healing. On the other hand, some papers have discussed IBF to be a positive finding indicating a reparative healing response e.g., following eccentric training and sclerosing injections, which remains to be clarified in further studies (Alfredson and Ohberg, 2006; Pingel et al., 2013a; De Marchi et al., 2018).
In healthy athletes, increased amount of resting IBF has been proposed to represent a long-term physiological metabolic adaptation to prolonged sports performance involving high and repetitive mechanical loading (Boesen et al., 2006a, 2011, 2012; Malliaras et al., 2008; Koenig et al., 2010). It has been suggested that this elevated vascularization indicates a continuous regenerative process during resting phases in between training sessions (Boesen et al., 2006a). Investigations regarding the acute effect of exercise in athletes have reported persisting or increased IBF following sport performance in elite badminton players (Boesen et al., 2006a, 2011) and recreational floorball players (Fahlström and Alfredson, 2010), assuming a physiological remodeling process and reaction to hypoxic environment in direct response to tendon loading (Koenig et al., 2010; Boesen et al., 2011; Malliaras et al., 2012). On the other hand, IBF was discussed to indicate overloading and pathology (Ohberg et al., 2001; Boesen et al., 2006a, 2011) which would implicate a number of asymptomatic athletes with pathological tendons (Boesen et al., 2006a, 2011; Tardioli et al., 2012). Since the present study does not show significantly altered resting IBF or pronounced exercise-induced IBF increase in runners compared to recreational participants, the previously suggested long-term adaptation regarding increased detectability of IBF in athletes (Malliaras et al., 2008; Boesen et al., 2012) cannot be supported.
Temporary increased IBF solely detectable directly following tendon loading exercise may be considered a physiological response irrespective of training status or tendon pathology. A comparable acute response to exercise has already been reported in a prior pilot study with healthy recreational participants (Risch et al., 2018a) as well as by Boesen et al. (2006b) finding resting-IBF in 30% (“mean color fraction” 0.05) of ATs in 10 healthy non-trained participants and increased or sustained IBF in 80% (mean color fraction 0.14) after a 5 km run. Furthermore, elevated IBF reported in elite badminton players (Boesen et al., 2006a) and recreational floorball players (Fahlström and Alfredson, 2010) directly after competition and in Achilles tendinopathy patients directly after eccentric training (Boesen et al., 2006b) support this assumption. It is also known from microdialysis, Xenon clearance technique, and contrast-enhanced ultrasound examinations that physiological tendon blood flow can increase up to 12-fold following tendon loading exercise (Kjaer et al., 2006; Pingel et al., 2013a,b). Therefore, detectability of exercise-induced IBF increase in the present study is assumed to represent a physiologically increased flow in preexisting vessel beds temporarily rising above the sensitivity threshold of the applied ultrasound device (Boesen et al., 2006b; Risch et al., 2018a). Present and previous findings indicate that there are “responders” and “non-responders” regarding sonographic detectable IBF changes following acute exercise. Although the percentage of responders is lower in healthy compared to tendinopathic ATs, statistical analysis revealed no significant differences between groups. If varying responsiveness regarding the sonographic detectable increase of IBF is a consequence of inter-individual differences in tendon vascularization (Boesen et al., 2006b; Risch et al., 2018a) can only be speculated.
In healthy ATs the occurrence of 1 to 6 vessels in 40–53% of ATs was higher than the previously reported detectability in 29–35% of ATs in comparable participants (Boesen et al., 2006b; Hirschmüller et al., 2010; Risch et al., 2018a). Boesen et al. (2006b, 2011) have suggested that low amount of sonographic detected resting IBF (grade 1 with 1 or 2 “small color foci”) represents a physiological finding whereas higher IBF amount was considered pathological. A specific threshold between pathological and physiological IBF, however, has not been established yet (Boesen et al., 2006b, 2011, 2012; Koenig et al., 2010; Genovese et al., 2011). On the other hand, it is known that tendon blood flow commonly exists below the sensitivity threshold of conventional Doppler ultrasound devices (Tol et al., 2012; Pingel et al., 2013b). Improving technology with increased sensitivity, e.g., use of Doppler ultrasound mode “Advanced Dynamic Flow”(Risch et al., 2016), has likely resulted in detection of physiological, low vascularization (Boesen et al., 2012; Tol et al., 2012) not visible in earlier studies (Ohberg et al., 2001). For the interpretation of detectable IBF, presence of pain and tendon tissue alterations should be taken into account. Data of the present study suggest that in the absence of tendon pain in history, pain on palpation and sonographic detectable pathology, the observed 6 vessels at rest and an increase up to 10 vessels following exercise assessed with “Advanced Dynamic Flow” can be considered a physiological finding. The determination of a specific threshold for physiological versus pathological IBF, however, requires further investigation and is likely to be device-dependent (Boesen et al., 2006b).
Limitations
To ensure comparable intensity of cardiovascular, metabolic and mechanical stress, the constant load running exercise was standardized based on running velocity and exercise duration, taking into account that the distance covered varied between individual participants. Standardized device settings, reliability of measurement procedure and data analysis have been tested previously (Risch et al., 2016, 2018a,b). To optimize reliability, all examinations have been performed by the same experienced investigator (Risch et al., 2016, 2018b). It was taken into account that due to the clinical setting of this study, the examiner was not blinded to the participant and measurement time point. Nevertheless, some consecutive post-exercise Doppler ultrasound examinations in Hrec and Hrun revealed slight variations of one vessel which were most likely due to ultrasound handling and are considered negligible due to measurement error. A temporary reduction of 10 vessels in one tendon of the Trun group at U5, however, was remarkable. Other studies have reported this occurrence in some tendons as well but had no conclusive explanation either (Boesen et al., 2006b; Malliaras et al., 2012).
Due to a lack of comparable and available data, no power-analysis was performed prior to this study. Therefore the aim was to include a minimum of 10 participants per group, comparable to sample sizes in previous studies investigating exercise-induced IBF (Boesen et al., 2006b; Malliaras et al., 2012; Pingel et al., 2013b), with an age and gender matched composition. The relatively small sample size may influence the generalizability of the presented results and main effects. Furthermore, the inter-subject variability concerning amount of IBF as well as supposed presence of responders and non-responders to exercise limit the ability to perform statistical testing: Due to lack of normal distribution, statistical analysis was performed using a non-parametric ANOVA which does not test for an interaction effect. Nevertheless, the described varying IBF occurrence is comparable to findings in previous literature (Hirschmüller et al., 2010; Risch et al., 2016). Previous investigations applying conventional Doppler ultrasound commonly involve rather imprecise and “noisy” depiction of IBF due to the so-called “blooming effect” (Heling et al., 2004). The use of the ADF-mode with highly standardized assessment settings has enabled a very detailed and reliable imaging and quantification of IBF. Therefore, the results are considered clinically relevant. However, it should be noted that the response of IBF presented in this study merely refers to sonographic detectable blood flow and excludes changes in microvascular volume existing below the sensitivity threshold of the applied Doppler mode.
Conclusion
In conclusion, this study showed that exercise predominantly results in a slight immediate increase of sonographic detectable IBF irrespective of training status and tendon pathology. In all groups there are responders and non-responders to tendon loading activity regarding changes of IBF. It is not feasible to discriminate between IBF in runners and recreational participants since runners did not show an elevated amount of resting-IBF or a pronounced response following exercise. Although tendinopathic ATs reveal an overall higher level of IBF compared to healthy ATs at all times, the amount of increase following exercise was similar. While a temporary exercise-induced IBF increase solely detectable after 5 min is considered a physiological response, prolonged IBF elevation may indicate morphological changes and tendon tissue turnover which has to be confirmed in further studies. In healthy ATs, the present study found presence of up to 6 vessels at rest and up to 10 vessels following exercise which is considered a physiological finding. However, the determination of a specific threshold to differentiate between physiological (low) and pathological (high) IBF requires further investigation.
Data Availability Statement
The raw data supporting the conclusions of this article will be made available by the authors, without undue reservation.
Ethics Statement
The studies involving human participants were reviewed and approved by the Ethics Committee of the University of Potsdam. The patients/participants provided their written informed consent to participate in this study.
Author Contributions
LR, FM, and MC involved in the development of study design, interpretation, and revising the manuscript. LR involved in the data collection, data analysis, and preparation of the manuscript. All authors contributed to the article and approved the submitted version.
Conflict of Interest
The authors declare that the research was conducted in the absence of any commercial or financial relationships that could be construed as a potential conflict of interest.
Acknowledgments
We acknowledge the support of the “Deutsche Forschungsge- meinschaft” and Open Access Publishing Fund of the University of Potsdam. We thank all participants for their support and Janine Merten for her technical assistance.
References
Alfredson, H., Bjur, D., Thorsen, K., Lorentzon, R., and Sandström, P. (2002). High intratendinous lactate levels in painful chronic Achilles tendinosis. An investigation using microdialysis technique. J. Orthop. Res. 20, 934–938. doi: 10.1016/S0736-0266(02)00021-9
Alfredson, H., and Ohberg, L. (2006). Increased intratendinous vascularity in the early period after sclerosing injection treatment in Achilles tendinosis?: a healing response? Knee Surg. Sports Traumatol. Arthrosc. 14, 399–401. doi: 10.1007/s00167-006-0720-7
Alfredson, H., Ohberg, L., and Forsgren, S. (2003). Is vasculo-neural ingrowth the cause of pain in chronic Achilles tendinosis? An investigation using ultrasonography and colour Doppler, immunohistochemistry, and diagnostic injections. Knee Surg. Sports Traumatol. Arthrosc. 11, 334–338. doi: 10.1007/s00167-003-0391-6
Andersson, G., Danielson, P., Alfredson, H., and Forsgren, S. (2007). Nerve-related characteristics of ventral paratendinous tissue in chronic Achilles tendinosis. Knee Surg. Sports Traumatol. Arthrosc. 15, 1272–1279. doi: 10.1007/s00167-007-0364-2
Boesen, A. P., Boesen, M. I., Koenig, M. J., Bliddal, H., Torp-Pedersen, S., and Langberg, H. (2011). Evidence of accumulated stress in Achilles and anterior knee tendons in elite badminton players. Knee Surg. Sports Traumatol. Arthrosc. 19, 30–37. doi: 10.1007/s00167-010-1208-z
Boesen, A. P., Boesen, M. I., Torp-Pedersen, S., Christensen, R., Boesen, L., Hölmich, P., et al. (2012). Associations between abnormal ultrasound color Doppler measures and tendon pain symptoms in badminton players during a season: a prospective cohort study. Am. J. Sports Med. 40, 548–555. doi: 10.1177/0363546511435478
Boesen, M. I., Boesen, A., Koenig, M. J., Bliddal, H., and Torp-Pedersen, S. (2006a). Ultrasonographic investigation of the Achilles tendon in elite badminton players using color Doppler. Am. J. Sports Med. 34, 2013–2021. doi: 10.1177/0363546506290188
Boesen, M. I., Koenig, M. J., Torp-Pedersen, S., Bliddal, H., and Langberg, H. (2006b). Tendinopathy and Doppler activity: the vascular response of the Achilles tendon to exercise. Scand. J. Med. Sci. Sports. 16, 463–469. doi: 10.1111/j.1600-0838.2005.00512.x
Borg, G. A. (1982). Psychophysical bases of perceived exertion. Med. Sci. Sports Exerc. 14, 377–381.
Boushel, R., Langberg, H., Green, S., Skovgaard, D., Jens, B., and Kjòr, M. (2000). Blood flow and oxygenation in peritendinous tissue and calf muscle during dynamic exercise in humans. J. Physiol. 524(Pt 1), 305–313. doi: 10.1111/j.1469-7793.2000.t01-2-00305.x
Cassel, M., Risch, L., Intziegianni, K., Mueller, J., Stoll, J., Brecht, P., et al. (2018). Incidence of achilles and patellar tendinopathy in adolescent elite athletes. Int. J. Sports Med. 39, 726–732. doi: 10.1055/a-0633-9098
Clermont, C. A., Phinyomark, A., Osis, S. T., and Ferber, R. (2019). Classification of higher- and lower-mileage runners based on running kinematics. J. Sport. Health Sci. 8, 249–257. doi: 10.1016/j.jshs.2017.08.003
Cook, J. L., Kiss, Z. S., Ptasznik, R., and Malliaras, P. (2005). Is vascularity more evident after exercise? Implications for tendon imaging. AJR Am. J. Roentgenol. 185, 1138–1140. doi: 10.2214/AJR.04.1205
Couppe, C., Kongsgaard, M., Aagaard, P., Hansen, P., Bojsen-Moller, J., Kjaer, M., et al. (2008). Habitual loading results in tendon hypertrophy and increased stiffness of the human patellar tendon. J. Appl. Physiol. 105, 805–810. doi: 10.1152/japplphysiol.90361.2008
Dickhuth, H. H., Yin, L., Niess, A., Röcker, K., Mayer, F., Heitkamp, H. C., et al. (1999). Ventilatory, lactate-derived and catecholamine thresholds during incremental treadmill running: relationship and reproducibility. Int. J. Sports Med. 20, 122–127. doi: 10.1055/s-2007-971105
Fahlström, M., and Alfredson, H. (2010). Ultrasound and Doppler findings in the Achilles tendon among middle-aged recreational floor-ball players in direct relation to a match. Br. J. Sports Med. 44, 140–143. doi: 10.1136/bjsm.2008.047316
Fredberg, U., Bolvig, L., Andersen, N. T., and Stengaard-Pedersen, K. (2008). Ultrasonography in evaluation of Achilles and patella tendon thickness. Ultraschall Med. 29, 60–65. doi: 10.1055/s-2007-963027
Genovese, E., Ronga, M., Recaldini, C., Fontana, F., Callegari, L., Maffulli, N., et al. (2011). Analysis of achilles tendon vascularity with second-generation contrast-enhanced ultrasound. J. Clin. Ultrasound. 39, 141–145. doi: 10.1002/jcu.20789
Heling, K. S., Chaoui, R., and Bollmann, R. (2004). Advanced dynamic flow – a new method of vascular imaging in prenatal medicine. A pilot study of its applicability. Ultraschall Med. 25, 280–284. doi: 10.1055/s-2004-813383
Hirschmüller, A., Frey, V., Deibert, P., Konstantinidis, L., Mayer, F., Südkamp, N., et al. (2010). [Achilles tendon power Doppler sonography in 953 long distance runners - a cross sectional study]. Ultraschall Med. 31, 387–393. doi: 10.1055/s-0029-1245189
Järvinen, T. A. H. (2020). Neovascularisation in tendinopathy: from eradication to stabilisation? Br. J. Sports Med. 54, 1–2. doi: 10.1136/bjsports-2019-100608
De Jonge, S., Warnaars, J. L. F., De Vos, R. J., Weir, A., van Schie, H. T., Bierma-Zeinstra, S. M., et al. (2014). Relationship between neovascularization and clinical severity in Achilles tendinopathy in 556 paired measurements. Scand. J. Med. Sci. Sports. 24, 773–778. doi: 10.1111/sms.12072
Kjaer, M., Langberg, H., Miller, B. F., Boushel, R., Crameri, R., Koskinen, S., et al. (2005). Metabolic activity and collagen turnover in human tendon in response to physical activity. J. Musculoskelet. Neuronal Interact. 5, 41–52.
Kjaer, M., Magnusson, P., Krogsgaard, M., BoysenMøller, J., Olesen, J., Heinemeier, K., et al. (2006). Extracellular matrix adaptation of tendon and skeletal muscle to exercise. J. Anat. 208, 445–450. doi: 10.1111/j.1469-7580.2006.00549.x
Knobloch, K., Yoon, U., and Vogt, P. M. (2008). Acute and overuse injuries correlated to hours of training in master running athletes. Foot Ankle Int. 29, 671–676. doi: 10.3113/FAI.2008.0671
Koenig, M. J., Torp-Pedersen, S., Boesen, M. I., Holm, C. C., and Bliddal, H. (2010). Doppler ultrasonography of the anterior knee tendons in elite badminton players?: colour fraction before and after match. Br. J. Sports Med. 2, 134–139. doi: 10.1136/bjsm.2007.039743
Malliaras, P., Chan, O., Simran, G., Martinez de Albornoz, P., Morrissey, D., and Maffulli, N. (2012) Doppler ultrasound signal in Achilles tendinopathy reduces immediately after activity. Int. J. Sports Med. 33, 480–484. doi: 10.1055/s-0032-1304636
Malliaras, P., Richards, P. J., Garau, G., and Maffulli, N. (2008). Achilles tendon Doppler flow may be associated with mechanical loading among active athletes. Am. J. Sports Med. 36, 2210–2215. doi: 10.1177/0363546508319052
De Marchi, A., Pozza, S., Cenna, E., Cavallo, F., Gays, G., Simbula, L., et al. (2018). In Achilles tendinopathy, the neovascularization, detected by contrast-enhanced ultrasound (CEUS), is abundant but not related to symptoms. Knee Surg. Sports Traumatol. Arthroshrosc. 26, 2051–2058. doi: 10.1007/s00167-017-4710-8
Moser, O., Tschakert, G., Mueller, A., Groeschl, W., Pieber, T. R., Obermayer-Pietsch, B., et al. (2015). Effects of high-intensity interval exercise versus moderate continuous exercise on glucose homeostasis and hormone response in patients with type 1 diabetes mellitus using novel ultra-long-acting insulin. PLoS One 10:e0136489. doi: 10.1371/journal.pone.0136489
Mugele, H., Plummer, A., Baritello, O., Towe, M., Brecht, P., and Mayer, F. (2018). Accuracy of training recommendations based on a treadmill multistage incremental exercise test. PLoS One 13:e0204696. doi: 10.1371/journal.pone.0204696
Ohberg, L., Lorentzon, R., and Alfredson, H. (2001). Neovascularisation in Achilles tendons with painful tendinosis but not in normal tendons: an ultrasonographic investigation. Knee Surg. Sports Traumatol. Arthrosc. 9, 233–238. doi: 10.1007/s001670000189
Pingel, J., Harrison, A., Simonsen, L., Suetta, C., Bülow, J., and Langberg, H. (2013a). The microvascular volume of the Achilles tendon is increased in patients with tendinopathy at rest and after a 1-hour treadmill run. Am. J. Sports. Med. 41, 2400–2408. doi: 10.1177/0363546513498988
Pingel, J., Harrison, A., Suetta, C., Simonsen, L., Langberg, H., and Bülow, J. (2013b). The acute effects of exercise on the microvascular volume of Achilles tendons in healthy young subjects. Clin. Physiol. Funct. Imaging. 33, 252–257. doi: 10.1111/cpf.12021
Rees, J. D., Stride, M., and Scott, A. (2014). Tendons – time to revisit in fl ammation. Br. J. Sports Med. 48, 1553–1557. doi: 10.1136/bjsports-2012-091957
Risch, L., Cassel, M., and Mayer, F. (2018a). Acute effect of running exercise on physiological Achilles tendon blood flow. Scand. J. Med. Sci. Sports 28, 138–143. doi: 10.1111/sms.12874
Risch, L., Cassel, M., Messerschmidt, J., Intziegianni, K., Fröhlich, K., Kopinski, S., et al. (2016). Is sonographic assessment of intratendinous blood flow in achilles tendinopathy patients reliable? Ultrasound Int. Open 2, E13–E18. doi: 10.1055/s-0035-1569286
Risch, L., Wochatz, M., Messerschmidt, J., Engel, T., Mayer, F., and Cassel, M. (2018b). Reliability of evaluating Achilles tendon vascularization assessed with doppler ultrasound advanced dynamic flow. J. Ultrasound Med. 37, 737–744. doi: 10.1002/jum.14414
Robinson, J. M., Cook, J. L., Purdam, C., Visentini, P. J., Ross, J., Maffulli, N., et al. (2001). The VISA-A questionnaire: a valid and reliable index of the clinical severity of Achilles tendinopathy. Br. J. Sports. Med. 35, 335–341. doi: 10.1136/bjsm.35.5.335
Ryan, M., and Cped, C. (2015). Should we care about tendon structure?? the disconnect between structure and symptoms in tendinopathy. J. Orthop. Sports Phys. Ther. 45, 823–825. doi: 10.2519/jospt.2015.0112
Sanz-López, F., Berzosa Sánchez, C., Hita-Contreras, F., Cruz-Diaz, D., and Martínez-Amat, A. (2016). Ultrasound changes in Achilles tendon and gastrocnemius medialis muscle on squat eccentric overload and running performance. J. strength Cond. Res. 30, 2010–2018. doi: 10.1519/JSC.0000000000001298
Sengkerij, P. M., de Vos, R.-J., Weir, A., van Weelde, B. J. G., and Tol, J. L. (2009). Interobserver reliability of neovascularization score using power Doppler ultrasonography in midportion Achilles tendinopathy. Am. J. Sports Med. 37, 1627–1631. doi: 10.1177/0363546509332255
Tardioli, A., Malliaras, P., and Maffulli, N. (2012). Immediate and short-term effects of exercise on tendon structure: biochemical, biomechanical and imaging responses. Br. Med. Bull. 103, 169–202. doi: 10.1093/bmb/ldr052
Tol, J. L., Spiezia, F., and Maffulli, N. (2012). Neovascularization in Achilles tendinopathy: have we been chasing a red herring? Knee Surg. Sports Traumatol. Arthrosc. 20, 1891–1894. doi: 10.1007/s00167-012-2172-6
de Vos, R.-J., Weir, A., Cobben, L. P. J., and Tol, J. L. (2007). The value of power Doppler ultrasonography in Achilles tendinopathy: a prospective study. Am. J. Sports Med. 35, 1696–1701. doi: 10.1177/0363546507303116
Keywords: neovascularization, tendinopathy, Doppler ultrasound, Advanced Dynamic Flow, athlete, sonography
Citation: Risch L, Mayer F and Cassel M (2021) Doppler Flow Response Following Running Exercise Differs Between Healthy and Tendinopathic Achilles Tendons. Front. Physiol. 12:650507. doi: 10.3389/fphys.2021.650507
Received: 07 January 2021; Accepted: 23 February 2021;
Published: 23 March 2021.
Edited by:
Beat Knechtle, Universitätsspital Zürich, SwitzerlandReviewed by:
Christoph Spang, Umeå University, SwedenJennifer Zellers, Washington University School of Medicine in St. Louis, United States
Francesco Oliva, University of Rome Tor Vergata, Italy
Rene Svensson, Bispebjerg Hospital, Denmark
Rod S. Barrett, Griffith University, Australia
Copyright © 2021 Risch, Mayer and Cassel. This is an open-access article distributed under the terms of the Creative Commons Attribution License (CC BY). The use, distribution or reproduction in other forums is permitted, provided the original author(s) and the copyright owner(s) are credited and that the original publication in this journal is cited, in accordance with accepted academic practice. No use, distribution or reproduction is permitted which does not comply with these terms.
*Correspondence: Lucie Risch, lrisch@uni-potsdam.de