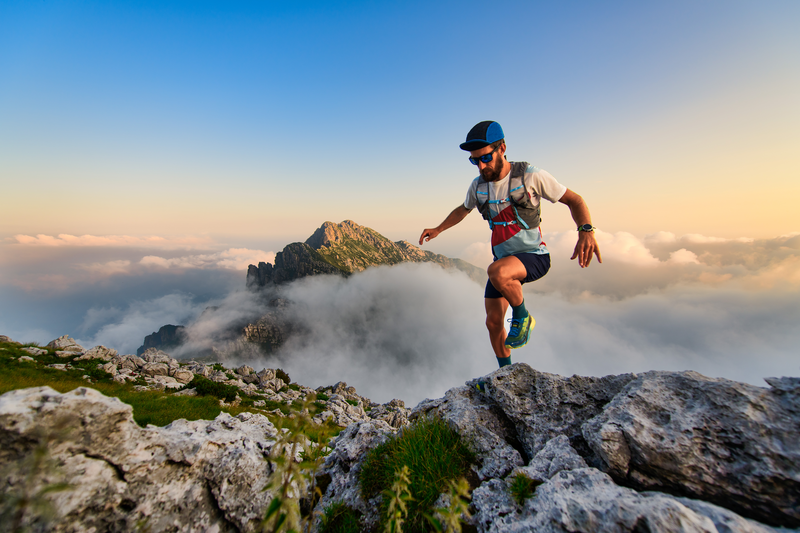
94% of researchers rate our articles as excellent or good
Learn more about the work of our research integrity team to safeguard the quality of each article we publish.
Find out more
ORIGINAL RESEARCH article
Front. Physiol. , 19 August 2021
Sec. Computational Physiology and Medicine
Volume 12 - 2021 | https://doi.org/10.3389/fphys.2021.582037
This article is part of the Research Topic Cardiac Pacemaking in Health and Disease: From Genes to Function View all 17 articles
The cardiac hyperpolarization-activated “funny” current (If), which contributes to sinoatrial node (SAN) pacemaking, has a more negative half-maximal activation voltage and smaller fully-activated macroscopic conductance in human than in rabbit SAN cells. The consequences of these differences for the relative roles of If in the two species, and for their responses to the specific bradycardic agent ivabradine at clinical doses have not been systematically explored. This study aims to address these issues, through incorporating rabbit and human If formulations developed by Fabbri et al. into the Severi et al. model of rabbit SAN cells. A theory was developed to correlate the effect of If reduction with the total inward depolarising current (Itotal) during diastolic depolarization. Replacing the rabbit If formulation with the human one increased the pacemaking cycle length (CL) from 355 to 1,139 ms. With up to 20% If reduction (a level close to the inhibition of If by ivabradine at clinical concentrations), a modest increase (~5%) in the pacemaking CL was observed with the rabbit If formulation; however, the effect was doubled (~12.4%) for the human If formulation, even though the latter has smaller If density. When the action of acetylcholine (ACh, 0.1 nM) was considered, a 20% If reduction markedly increased the pacemaking CL by 37.5% (~27.3% reduction in the pacing rate), which is similar to the ivabradine effect at clinical concentrations. Theoretical analysis showed that the resultant increase of the pacemaking CL is inversely proportional to the magnitude of Itotal during diastolic depolarization phase: a smaller If in the model resulted in a smaller Itotal amplitude, resulting in a slower pacemaking rate; and the same reduction in If resulted in a more significant change of CL in the cell model with a smaller Itotal. This explained the mechanism by which a low dose of ivabradine slows pacemaking rate more in humans than in the rabbit. Similar results were seen in the Fabbri et al. model of human SAN cells, suggesting our observations are model-independent. Collectively, the results of study explain why low dose ivabradine at clinically relevant concentrations acts as an effective bradycardic agent in modulating human SAN pacemaking.
The pacemaker activity of sinoatrial node (SAN) cells in the mammalian heart arises from the integrated action of multiple sarcolemmal ionic channel currents and the interaction between the intracellular calcium handling and sarcolemmal electrogenic processes (Irisawa et al., 1993; Mangoni and Nargeot, 2008; Lakatta et al., 2010). The hyperpolarization-activated “funny” current, If, present in the SAN and other regions of the cardiac conduction system (Boyett, 2009; Difrancesco, 2010), is produced by the hyperpolarization-activated cyclic nucleotide gated (HCN) channel isoforms (of which there are four: HCN1-4), each of which is comprised of six transmembrane domains with four subunits combining to produce functional tetrameric channels, as occurs for voltage-gated potassium channels (Bois et al., 2007; Difrancesco, 2010). Previous studies of the rabbit SAN have shown that although HCN isoforms 1, 2, and 4 are all expressed in the heart, HCN4 is the most abundant in the SAN and the If density within SAN sub-regions correlates strongly with HCN4 expression levels (Thollon et al., 2007; Brioschi et al., 2009). The unique features of HCN channels lie in the fact that they are activated not on depolarization but on hyperpolarization of cell membrane potential (to voltages negative to ~−40 to ~−50 mV) (Hagiwara and Irisawa, 1989; Accili et al., 1997; Baruscotti et al., 2005) and are permeable to both Na+ and K+ ions, with an approximate reversal potential of −30 mV (Van Ginneken and Giles, 1991; Verkerk et al., 2009a). Upon hyperpolarization, HCN channels generate an inward current over the pacemaking potential range which, together with the current generated by other electrogenic processes of the intracellular calcium handling (i.e., the Ca2+ clock), contributes to the genesis of intrinsic pacemaker activity of the SAN (Lakatta and Difrancesco, 2009). HCN channels are also modulated by adrenergic agonists via cAMP (Bucchi et al., 2003; Craven and Zagotta, 2006).
If channels are also present in the human SAN. It has been shown that HCN4 is strongly expressed in the human SAN, with the measured mRNA level of other isoforms accounting for <16% (with HCN3 being negligible, accounting for only 0.5%) of the total mRNA measurement (Chandler et al., 2009). Though the HCN expression in the human SAN is similar to that in the rabbit, properties and kinetics of If are clearly different. It has been shown the fully-activated If conductance in the human is about 3–4 times smaller than that in the rabbit (Verkerk et al., 2007a). Additionally, If in human SAN cells has a more negative half-maximal activation voltage, and a greater time constant of deactivation/activation process which is also negatively shifted (Verkerk et al., 2007a). With such a marked species differences in If conductance and kinetics between the rabbit and human, a question arises as to whether a smaller If in the human SAN cells plays the same important role in regulating cardiac pacemaking activity as in the rabbit SAN?
Although If in human SAN cells is much smaller than those in other mammals, it may play a comparable role to that in the rabbit in modulating cardiac pacemaking. In their study, (Verkerk et al., 2007b) observed about a 26% increase in pacemaking cycle length in human SAN cells on complete block of If by using 2 mM Cs+, which is close to that seen in the rabbit (Verkerk and Wilders, 2010; Fabbri et al., 2017). Pharmacological targeting of If by ivabradine has also shown the clinical value of If in controlling the heart rate in patients who need heart rate control in conditions of coronary artery disease (CAD) (Tardif et al., 2005; Camici et al., 2016; Niccoli et al., 2017) and heart failure (HF) (Bohm et al., 2015; Yancy et al., 2016). In both conditions, slowing down the heart rate by ivabradine increases the diastolic interval, thereby reducing the metabolic load on the working myocardium; this reduces the risks of ischemia of the heart leading to reduced risk of sudden death (Niccoli et al., 2017).
Although inhibition of If by ivabradine provides an efficient pharmacological control of heart rate in the clinic, it is still unclear how the clinical concentration range of ivabradine [about 20–140 nM (Choi et al., 2013; Jiang et al., 2013); blocking If < 20% (Bois et al., 1996; Bucchi et al., 2002)] can produce a significant effect in reducing human heart rate. In pre-clinical animal model studies, ivabradine has been reported to inhibit If in SAN cells with a half-maximal inhibitory concentration of 1.5–2.8 μM (Bois et al., 1996; Bucchi et al., 2002), and recombinant HCN4 channels with an IC50 values between 0.5 and 2.0 μM (Bucchi et al., 2002, 2006, 2013). In rabbit SAN cells, 1 μM ivabradine has been observed to reduce the pacemaking rate by 12.3% (Thollon et al., 1994), whilst about 16.2 and 23.8% of heart rate reduction at 3 μM have been seen (Thollon et al., 1994; Bucchi et al., 2007).
When ivabradine was administered intravenously (0.2 mg kg−1) to patients with normal baseline electrophysiology, mean heart rate reductions of 12.9 and 14.1 beats min−1 (at 0.5 and 1 h respectively following administration) were observed (Camm and Lau, 2003). The drug is usually administered orally, however, and after repeated oral dosing at 5 mg, mean maximal plasma (Cmax) levels of 11–16 ng ml−1 (23.5–34.1 nM) have been measured, whilst for repeated dosing at 10 mg, mean Cmax levels of 29–42 ng ml−1 (61.8–89.6 nM) have been seen (Choi et al., 2013; Jiang et al., 2013). Repeated dosing with a high ivabradine concentration of 20 mg has been associated with a Cmax of 137 nM (Jiang et al., 2013). On the basis of the pre-clinically observed concentration-dependence for If inhibition, comparatively low levels of If block might be expected at such plasma levels (Thollon et al., 1994; Bucchi et al., 2007). However, clinical concentrations of ivabradine produce about 18–20% reduction in human heart rate (Camm and Lau, 2003; Doesch et al., 2007).
Previous animal model studies (Difrancesco, 1991, 2010) have found that inhibition of If by ivabradine slowed down the spontaneous firing rate of the rabbit SAN cell in a use-dependent manner (Bois et al., 1996), and about 15% reduction of the pacemaking rate was observed at a concentration of 3 μM, which produced about 60% If reduction at membrane potential of −92 mV, and about 41% If reduction at physiologically relevant membrane potentials (~60 mV) (Yaniv et al., 2012). Numerically, in a recent in silico exploration of the role of If in SAN pacemaking using a rabbit SAN model, the effect of ivabradine was simulated through implementing a 66% reduction of If (mimicking an experimentally reported effect of 3 μM ivabradine), leading to a 22% reduction in spontaneous rate (Severi et al., 2012). However, due to the non-linear concentration-dependent action of ivabradine on If, it is hard (if not impossible) to derive the effect of a low concentration of ivabradine from that of a high concentration on modulating cardiac pacemaking rate. To date, therefore, the effect of blocking If at the level of If reduction at clinical plasma levels of ivabradine (<20%) on pacemaking rate of the rabbit SAN has not been elucidated, as is how such concentration can produce a marked effect on the human SAN, in which If is much smaller than that in the rabbit SAN. Most importantly, it is unclear either how clinical concentrations of ivabradine affect cardiac pacemaking activity in vivo as compared to those predicted by single cell experiments in vitro, in which vagal tone modulation of cardiac pacemaking is missing. The aim of the present study was therefore to investigate through simulations and theoretical analysis the effect of If reduction over a wide range of values on cardiac pacemaking activity in the presence and absence of vagal tone modulation of cardiac pacemaking activity by acetylcholine.
In this study, the contemporary model of rabbit SAN cells developed by Severi et al. (2012) was used as a basal model. The model was chosen as it represents the most updated progress in the model development of rabbit SAN cells, in particular it incorporates an updated If formulation based on recently available experimental data (Altomare et al., 2003; Barbuti et al., 2007). The basal model code was downloadable from cellML at the following URL: https://models.physiomeproject.org/e/139; and the source codes used for this study are available on request to: aGVuZ2d1aS56aGFuZ0BtYW5jaGVzdGVyLmFjLnVr. In brief, the dynamics of the membrane action potential the SAN cell were modeled as:
Where V is the membrane potential, Cm the membrane capacitance, t the time, Itotal the total membrane current generated by potassium (IKr, IKs, Isus, Ito), calcium (ICaL, ICaT), sodium (INa), Na+-K+ pump (INaK), Na+-Ca2+ exchanger (INaCa), and funny (If) channels. More details of the basal model are documented in the study of Severi et al. (2012).
Previous experimental studies have shown some distinctive differences in the maximal macroscopic conductance, the steady-state activation curve and the time constant of the channel activation of If between the rabbit and the human SAN cells (Difrancesco et al., 1989; Altomare et al., 2003; Barbuti et al., 2007; Verkerk et al., 2007b). In order to take into consideration the reported species difference in If properties, in our simulations we implemented two different sets of If formulations, one is the original model formulations developed by Severi et al. (2012) (rabbit-like formulation), and the other is Fabbri et al. formulation (Fabbri et al., 2017) based on the human If data (Verkerk et al., 2007b) (human-like formulation), which takes the form:
where If,Na and If,K are Na+ and K+ components of If, gf,Na (0.00268 μS) and gf,K (0.00159 μS) conductance of If,Na and If,K. ENa and EK the equilibrium potential for Na+ and K+. y∞ is the steady state activation variable, τy the time constant of activation variable (y).
To determine and validate the parameters in the equations of human-like and rabbit-like If formulations, the equations for the steady-state activation curves (Figure 1A), and the equation for the activation time constant (Figure 1B) were fitted to experimental data obtained from human and rabbit SAN cells respectively (Difrancesco et al., 1989; Altomare et al., 2003; Barbuti et al., 2007; Verkerk et al., 2007b). The developed If formulations were validated by their ability to reproduce experimental I-V relationship data (Figure 1C) obtained by running a series of voltage-clamp commands (Figure 1D) for both the human-like and rabbit-like formulations. Figure 1 shows clearly that If in the human SAN has a more negative half-maximal activation voltage (Figure 1A), a greater activation time constant (i.e., slower activation process (Figure 1B) and a smaller current density (Figure 1C) than that in the rabbit SAN.
Figure 1. Kinetics of If from experimental and mathematical modeling data. (A) Steady-state activation curves in rabbit and human and SAN cells, which were used to fit If formulations for the Severi et al. (2012) rabbit (solid lines) and the modified Fabbri et al. (2017) human (dashed lines) models. Experimental data from Altomare et al. (2003) (▴), Barbuti et al. (2007) (▾), and Verkerk et al. (2007b) (♦) are also shown. (B) Corresponding activation time constant of If for human and rabbit SAN cells. Experimental data from Difrancesco et al. (1989) (□) and Verkerk et al. (2007b) (■) are also shown. (C) Computed I-V relationship for the human- (dashed line) and rabbit-like (solid line) models, which are compared with experimental data from the study of Verkerk et al. (2007b) (□) for the human SAN cells, Zaza et al. (1996) (■) and Goethals et al. (1993) (▴) for the rabbit SAN cells. (D,E) Voltage-clamp protocol and simulated time traces of If during voltage-clamp for the rabbit and human If model. (F) Dose-dependent effect of ivabradine on If in rabbit SAN cells. Experimental data of Bois et al. (1996) (•) and Bucchi et al. (2002) (▴) were based to develop the concentration-response curves as shown by the solid line and dashed line respectively.
It has been shown that ivabradine blocks If without affecting channel kinetics, with block leading to a constant level of If reduction after a period of transition (Bucchi et al., 2002, 2013). Therefore, in this study, we implemented a pore-block theory (Yuan et al., 2015) to simulate the steady-state effect of If blocking by ivabradine over a wide concentration range by reducing its conductance by a factor k (k∈ (0,1), mimicking 0–100% (Bucchi et al., 2013) of If reduction. With varying levels of If inhibition, by the pore-block theory the If conductance became:
In vivo, ACh released from vagal activity slows down the spontaneous pacing rate of the SAN mainly by inhibiting If and ICaL (Boyett et al., 1995), and activating acetylcholine-dependent K+ current (IKACh) (Voigt et al., 2014). Vagal activity may also play a significant role in slowing down the pacing rate when ivabradine blocks If. To test the combined action of ivabradine and ACh, we simulated the ACh effect on SAN spontaneous APs, based on the formulations of Severi et al. (2012) for If and ICaL inhibition, as well as IKACh activation. In simulations, the values of gK,ACh used were the same as those used in the Severi et al. (2012) and Fabbri et al. models (Fabbri et al., 2017) for the human-like model (Severi model with human-like If) ((Fabbri et al., 2017) and the rabbit-like model (i.e., the Fabbri et al. model with rabbit-like If; see details in the Supplementary Material) (Severi et al., 2012). Effects of ACh on pacemaking APs were qualitatively analyzed and compared with the implementation of rabbit-like and human-like If formulation, as well as If blocking. Details of the IKACh formulation were listed in the Supplementary Material.
In order to test the model-dependence of results, simulations were also conducted in the Fabbri et al. model of the human SAN cell (Fabbri et al., 2017), the source code of which was downloadable from cellML at the following URL: https://models.physiomeproject.org/e/568?searchterm=human++si. In this case, the Fabbri et al. model with the human-like If formulation was taken as the basal model, which was then modified by replacing the If formulation by the rabbit-like one.
Although simulated action potentials from the original Fabbri et al. (2017) model closely match experiment data of AP properties and calcium transient of human SAN cells, some modification was necessary as most of the ionic currents in the model (except If, IKr, and IKs) were based on rabbit SAN cell model, densities of which were modified by automatic optimization to match simulated action potential characteristics to experimental data. Such automatic optimization of model parameters may deviate from physiological relevance, resulting in some limitations. For example, a full block of ICaT in the model abolished the pacemaking action potential. Though there are no direct experimental data from human SAN cells to validate the simulation result, data from rabbit sinoatrial node (Hagiwara et al., 1988; Takeda et al., 2004) and human patients (Madle et al., 2001) suggested a more modest change in the pacemaking cycle length when ICaT was blocked. Therefore, we updated the model to address some of the limitations. Details about model updating and validations are presented in Supplementary Figure 1. Using the updated model, simulations in the Serveri et al. model were repeated in the Fabbri et al. model, and results are included in the Supplementary Material to support the major conclusion of the study.
A fourth-order Runge-Kutta-Merson numerical integration method was used to solve the ordinary differential equations of the model. The time step was 5 × 10−6 s, which gives a stable solution of the equations and maintains the accuracy of the computation of membrane current and potential. In simulations, action potentials after the 20th one were recorded for analysis. For solving the Severi et al. model with the human If formulation, a set of initial values were used, which were taken from the recorded state variables when the model reached its steady state (see details about the initial values for solving the model in the Supplementary Material). This allows the secondary effect of different If formulations to other channel variables of the models to be considered in the simulations.
Theoretical analysis of the effect of If block on altered cardiac pacemaking cycle length (CL) was conducted following a similar approach as implemented in previous studies (Rocchetti et al., 2000; Zaza and Lombardi, 2001; Monfredi et al., 2014; Winter and Shattock, 2016; Zaza, 2016).
Figure 2 shows a schematic illustration of a cycle of action potentials of SAN cells. During the time course of the action potential, the voltage difference between the MDP and the voltage at the beginning of AP upstroke (Vup) (ΔVm) can be discretized as many small steps by ΔVi, each taking a time period DIi to complete. Here dVi/dt represents the local diastolic depolarization rate (DDR). During the diastolic depolarization phase, with small time interval (dt), |dVi/dt| can be approximately considered as a constant and denoted by |dV/dt|. And the total diastolic interval (DI) can be expressed as:
Considering Equation (2), the pacemaking cycle length (CL) can be denoted as:
Where Itotal denotes total membrane currents during the diastolic depolarization phase.
Figure 2. Schematic illustration of a cycle of SAN cell action potentials. During one cycle, the time interval between two consecutive action potentials measures the intrinsic pacemaking CL, which can be considered as a sum of the AP duration (APD) and the diastolic interval (DI) which starts from the maximum diastolic potential (MDP) to the beginning of AP upstroke [Vup (Fabbri et al., 2017)]. ΔVm denotes the voltage difference between the MPD and the Vup. During the DI, multiple time intervals (dt) were needed for the membrane potential to transit from the MDP to the Vup, during each of which a ΔVi is generated.
In response to If block, a new total ion channel current during the diastolic depolarization phase I' total is generated, which takes the form
ΔI is the change of Itotal caused by If reduction. This produces a new pacing cycle length (CL′), which can be represented as:
where APD' is the new action potential duration in response to If blockade. As a small If block in response to a low dose of ivabradine mainly affects the diastolic depolarization phase and has little or no impact on the AP duration and the MDP, APD′ is approximately equal to APD. Therefore, the increased cycle length (ΔCL) can be represented as
Then the relative change of the pacing cycle length is:
By denoting p as If current block potency (p = |ΔI/If|), and x as the proportion of If to Itotal during the diastolic phase (x = |If/Itotal|), then we have:
By setting
where C1 (C1 > 0) can be considered as a constant during the diastolic depolarization phase with a fixed level of If reduction (i.e., p is fixed), which has no significant effect on the difference between MDP and the Vup (i.e., Δ Vm), then we have:
Where C2 also can be seen as a constant related to C1 and p, also with a fixed level of If reduction.
With If block, the resultant relative change of the CL predicted by Equation (3) is inversely proportional to the amplitude of Itotal during the diastolic depolarization phase, which determines the intrinsic CL of the pacemaking action potential. It is also related to the level of If reduction and the ratio between If and Itotal. With a small level of If block, the resultant relative change of CL is greater for a smaller Itotal (i.e., greater when the CL is larger or the heart rate is lower).
Figure 3 shows the simulated action potentials from the Severi et al. model with rabbit (Figures 3Ai–Fi) and human (Figures 3Aii–Fii) If formulations. In the figure, action potentials (Figures 3Ai,Aii) are shown together with membrane currents during the genesis of action potentials, including ICaL (Figures 3Bi,Bii), If (Figures 3Ci,Cii), INa (Figures 3Di,Dii), ICaT (Figures 3Ei,Eii), INaCa (Figures 3Fi,Fii), IKr (Figures 3Gi,Gii), IKs (Figures 3Hi,Hii), Ito (Figures 3Ii,Iii), and INaK (Figures 3Ji,Jii). By replacing the rabbit If formulation with the human If formulation, the pacemaking activity was slowed down, with a pacemaking CL that increased from 355 ms to 1,139 ms, which was associated with a slight increase in INa and ICaT at the late period of the diastolic depolarization phase (DDP) (with no noticeable difference at the initial period of the DDP), and a slight decrease in IKr, IKs, Ito, and INaK during the DDP. Such a small increase in the above-mentioned inward currents and a decrease in the outward currents, though contributory factors, are not the major determinants of the prolonged diastolic depolarization phase. The slowing down in the pacemaking activity of the human-like formulation model is mainly attributable to the smaller If, ICaL, and INaCa during the diastolic depolarization phase.
Figure 3. Simulated spontaneous action potentials and underlying currents by the Severi et al. model with rabbit If (Ai–Fi) and human If (Aii–Fii) formulations. Effects of blocking If by 20% on action potentials and underlying ionic currents (dotted lines) are also shown and superimposed with those in control condition (solid lines). (Ai,Aii): action potentials; (Bi,Bii): ICaL; (Ci,Cii): If; (Di,Dii): INa; (Ei,Eii): ICaT; (Fi,Fii): INaCa; (Gi,Gii): IKr; (Hi,Hii): IKs; (Ii,Iii): Ito; (K) increase of pacing CL.
In Figure 3, effects of reducing If by 20% on the pacemaking activity of the two models are also shown.
Reduction of If by 20% produced an increase of the CL in the rabbit-like If formulation model by about 4.6% (Figure 3K). This is in agreement with experimental data from isolated rabbit SAN cells, which showed that a low level of If block by ivabradine [<0.5 μM, a concentration not affecting SAN ICaL which only slightly decreased by 18.12 ± 0.66% at 10 μM (Bois et al., 1996)] produced only a slight slowing down of the pacemaking rate, while >50% If blockade by 3 μM ivabradine (see Figure 1F) only reduced the spontaneous pacing rate by 11–17.7% (Bucchi et al., 2007; Yaniv et al., 2012).
However, in the human-like If formulation model, If reduction by 20% produced a more than 2-fold increase in the pacemaking CL by 12.4% (i.e., equivalent to about 11.1% reduction in the heart rate) as compared to the rabbit-like model (Figure 3K). The pacing rate reduction though was slightly less than the effect of intravenous administration of ivabradine by 0.2 mg·kg−1 (~23.5–34.1 nM of mean maximal ivabradine plasma levels) produced a reduction of heart rate by 18–20% (i.e., mean heart rate reductions of 12.9 and 14.1 beats min−1; Camm and Lau, 2003; Jiang et al., 2013), but close to experimental data of the pacing rate reduction when If was blocked by 3 μM ivabradine in rabbit SAN (Bucchi et al., 2007; Yaniv et al., 2012). This illustrates that the small human-like If has a greater effect on slowing down the pacing rate than the rabbit-like one when If is inhibited by ivabradine.
The results above suggested that the increased CL induced by 20% If reduction is proportional to the intrinsic cycle length of the model, i.e., the greater the intrinsic CL (e.g., the model with human-like If formulation) the greater the increase of the CL. This observation was model-independent as shown in Supplementary Figure 3, in which Fabbri et al. model of the human SAN was implemented by using rabbit-like and human-like If formulations. In the basal condition (Fabbri et al. model with human-like If formulation), the CL was 810 ms, which was increased by 44 ms with 20% If reduction (i.e., 5.5%). When the rabbit-like If formulation was used, the pacemaking rate was increased due to a larger If, resulting a CL of 355 ms. With 20% If reduction, the CL was increased by 18 ms (i.e., 4.7%), which was smaller than that when the human-like If formulation was used.
As shown in Figures 3Ci,Cii, a 20% reduction in the channel conductance did not necessarily produce 20% reduction in If amplitude during the time course of action potential, due to the dependence of If on membrane voltage. To further investigate this, we computed the average If during the diastolic phase before and after 20% reduction in its channel conductance. Results are shown in Supplementary Figure 2. It was found that 20% reduction in the channel conductance produced a similar decrease in the avarage If in the rabbit-like (by 0.34 pA) and the human-like model (by 0.22 pA), but the relative change was greater in the latter model because of its smaller If in the control condition. Such difference in the relative change of If may also be one of the important reasons for the more pronounced prolongation of the diastolic phase in model with human If formulation. Note that in both models (rabbit-like and human-like models), the relative change of If was smaller than 20% though the channel conductance was reduced by 20%, due to the voltage-dependence of the channel's activation.
Further simulations were carried out to analyze possible effects of the cross talk between the membrane clock (If) and Ca2+ clock on modulating pacemaking action potentials in response to If reduction. Figure 4 shows results for the rabbit-like (Figures 4Ai–Ei) and human-like (Figures 4Aii–Eii) If formulation models. Blocking If by 20% decreased intracellular (cytoplasmic) Ca2+ transients ([Ca2+]i, Figures 4Bi,Bii), the intracellular subspace Ca2+ concentration ([Ca2+]sub, Figures 4Ci,Cii) and the Ca2+ content in the network SR ([Ca2+]nsr, Figures 4Ei,Eii). The diastolic level of [Ca2+]sub was reduced by 2.9 and 5% in the rabbit-like and human-like If formulation model respectively, the decreased [Ca]sub during the diastolic depolarization phase of the action potential (Figures 4Ci,Cii) leads to a decreased INaCa (Figures 3Fi,Fii) and ICaL (Figures 3Bi,Bii), especially during the later phase of diastolic depolarization, which prolonged the later phase of the diastolic depolarization in the human-like If formulation model (Figure 4Aii). There was a negligible change in INa (Figures 3Di,Dii) and ICaT (Figures 3Ei,Eii) during the diastolic phase. Taken together with the observation of changes in INaCa (Figure 3F), our simulation results imply that the slowing down of the spontaneous action potentials in response to a low level of If block (<20%) may be mainly attributable to If reduction (i.e., membrane clock), with some contribution from a crosstalk between the membrane clock and Ca2+ clock in the later diastolic depolarization phase.
Figure 4. Effect of If block on the Ca2+ transient in rabbit-like (Ai–Ei) and human-like (Aii–Eii) SAN cell models. (Ai,Aii): the action potentials; (Bi,Bii): Ca2+ transient in the myoplasmic space; (Ci,Cii): Ca2+ transient in the myoplasmic sub-space; (Di,Dii): Ca2+ transients in the junctional SR; (Ei,Eii): Ca2+ transient in the network SR space; (Fi,Fii): intracellular Na+ concentration. Simulation results in control (solid line) are superimposed on those of If blocking (dotted lines).
Effects were also investigated of how a systematic change in If density affects spontaneous pacemaker activity. Results are shown in Figure 5, in which the computed CL (Figure 5A) and its increase (Figure 5B) with If being blocked from 0 to 100% with 1% increment for the rabbit-like (closed circles) and human-like If formulations (open circles). It was shown that over the range 0–80%, If block produced a greater CL increase with the human-like formulation than in the rabbit-like model. With a low level of If reduction, a linear relationship between the CL increase and If block was seen (Figure 5B). However, the relationship changed to an exponential one (Figure 5B) when a high level of If block was implemented. With about 66% If reduction, the CL was increased by about 26% (reduced pacemaking rate by 21%) in the rabbit-like model, which is consistent with experimental data (Thollon et al., 1994; Bucchi et al., 2007), but by about 42% in the human If formulation. A low level of If reduction (<20%) resulted in a negligible change in the MDP (<-0.3 mV in both models) in both rabbit-like and human-like If formulation models, and 100% If reduction hyperpolarised MDP by only 3.2 mV in the latter model (Figure 5C). Corresponding changes in APD90 (Figure 5D) and the voltage at the beginning of AP upstroke (Figure 5E) were also shown (also see Supplementary Table 1). While a high level of If reduction hyperpolarized the MDP, it had modest secondary effect on APD90 (mainly prolonging the late repolarization phase which may be due to the decreased IKr and INaK as shown in Supplementary Figures 4Gi,Ji, and there was no significant change in the fundamental morphology of the action potential as shown in Supplementary Figure 4Ai) and AP upstroke potential in the rabbit-like model.
Figure 5. Simulated effect of If block on the CL increase. (A,B) Pacing cycle length and the relative percentage changes of CL when If blocked from 0 to 100% with 1% increment in the human-like (open circle) and rabbit-like (closed circle) SAN cell models; (C,D) the maximum diastolic potential (MDP) and action potential duration at 90% of repolarization (APD90); (E) change of the beginning of action potential upstroke voltage, which was calculated as the diastolic depolarization rate reached about 50% of its maximum value. If block produced greater effect in the human-like (gray circle) and rabbit-like (black square) SAN cell models.
Changes of the membrane currents and ion concentrations with a systematic change in If density in the two models were also investigated (Supplementary Figures 3, 4). During the diastolic depolarization phase of the action potentials, [Ca]sub decreased as If density gradually decreased (Supplementary Figures 3Ci,Cii), further leading to a slower activation of the ICaL (Supplementary Figures 4Bi,Bii) and a decrease in INaCa (Supplementary Figures 4Fi,Fii) during this phase. Reduction of INaCa decreased [Na]i (Supplementary Figures 3Fi,Fii), leading to a reduction in INaK (Supplementary Figures 4Ji,Jii) which further exacerbated the reduction of [Na]i. The outward currents (IKr, IKs, Ito, INaK) also showed a gradual decrease with a decreased If density during the diastolic depolarization phase (Supplementary Figures 4Gi–Ji,Gii–Jii).
The simulated action potentials from the two models showed some differences when If was fully blocked (Figure 5A), with the rabbit-like model failing to generate spontaneous action potentials. Such differences may be attributable to the use of different sets of initial values recorded from their steady state variables as there was no other changes in model equations or parameters, except for the use of rabbit-like If or human-like If formulations. In order to determine potential factors contributing to such differences or contributing to the pacemaking action potentials in the two models, further analyses on membrane currents and the intracellular Ca2+ transients between control and 99% reduction of If were conducted. Results are shown in Supplementary Figures 3, 4. It was shown that in both models, in addition to If, ICaT, INaCa, and INa contributed to the diastolic depolarization. With a high level of If reduction (99%), there was a significant decrease in [Ca]sub during the diastolic depolarization phase (Supplementary Figure 3Ci), causing a significant reduction in INaCa (Supplementary Figure 4Fi). Consequently, the spontaneous membrane depolarization was not able to reach the ICaL activation potential, terminating the action potentials in the rabbit-like model.
In the human-like model, a reduction of [Ca]sub was also observed with a high level of If reduction, resulting in a decreased INaCa. However INaCa was sufficient to maintain the spontaneous depolarization to generate a full action potential.
The focus of this study was on the action of a modest extent (i.e., <20%) of If bock, mimicking the clinical use of ivabradine, rather than on the action of a large percentage of If block. With <50% If block, the increase in CL was about <30%, which is reasonably close to the experimental data observed in rabbit SAN cells when If is blocked by use of Cs+ (Nikmaram et al., 1997), validating the physiological relevance of the results obtained.
Further simulations were conducted to investigate the combined action of If reduction by ivabradine and actions of ACh (Boyett et al., 1995), mimicking the autonomic regulation of cardiac pacemaking in vivo. In simulations, acetylcholine-dependent inhibition of If and ICaL, and activation of K+ current (IK,ACh) (Voigt et al., 2014) were considered. Results are shown in Figure 6, in which computed time courses of APs in control (with both rabbit-like and human-like If formulations), If reduction alone (by 20%) and If reduction together with actions of 0.1 nM ACh were compared (Figures 6A,B). It was shown that ACh augmented the effect of If reduction on the increase of CL in the model with both the rabbit-like and human-like If formulations. With the action of 0.1 nM ACh, 20% of If block increased the CL by 37.5% (about 27.3% reduction the pacing rate) (Figure 6C) in the model with human-like If formulation, which is remarkably greater than that of 10.1% (about 9.1% reduction in the pacing rat) in the model with the rabbit-like If formulation. This observation held true when different ACh concentrations were considered (Figure 6D). These results illustrate that the combined action of IKACh and If reduction further slowed down the pacemaking AP due to a reduced total depolarization current during diastolic depolarization phase, resulting in a greater CL prolongation. It suggested that the clinical observed effect of low ivabradine on reducing pacing rate may partially result from the action of ACh due to active vagal tone in vivo. Similar observations were also seen in the Fabbri et al. model as shown in Supplementary Figure 4, in which ACh augmented more the effect of If reduction on pacemaking rate in the model with human-like If formulation than that with rabbit-like If formulation. Results from the Fabbri et al. model were similar, as shown in Supplementary Figure 5.
Figure 6. Effects of combined action of ACh and 20% If reduction on sponteneous action potentials in the Severi et al. model with the rabbit-like and human-like If formulations. (A,B) Time courses of action potentials in control, If reduction and combined effect of If reduction and ACh (0.1 nM). (C) The increase of pacemaking CL in the conditions of 20% If block alone and combined action with ACh (0.1 nM). (D) Combined effect of variant concentrations of ACh (from 0.1 to 10 nM) and 20% If block on pacemaking CL in the rabbit-like and human-like If formulations models.
The simulation results presented above showed that If block produced a greater impact on slowing down the pacemaking rate with the human-like If formulation than that in the rabbit-like cell model in both the Severi et al. and Fabbri et al. model (see Supplementary Material). This is paradoxical as the If density over the pacemaker range with the human-like formulation is much smaller than that in the rabbit-like model, and one would expect a smaller If contribution to the spontaneous action potentials (therefore a smaller CL increase with If block). However, such a paradoxical effect of If reduction on the increase of CL as observed in the two models matched the theoretical prediction shown in the Method section (Equation 2), which showed an inverse relationship between an increased CL and the amplitude of total ionic membrane currents during the diastolic depolarization phase. With the theoretical prediction, a greater CL increase in the human-like If formulation SAN cell model can be attributed to a smaller Itotal during the diastolic depolarization phase.
To test the theoretical prediction, further analyses were conducted to compute the averaged Itotal amplitude during the DDP. Results from the Severi et al. model are shown in Figure 7 for control and 20% If reduction for action potentials (Figures 7Ai,Aii), the time course of Itotal (Figures 7Bi,Bii) and the averaged amplitude of Itotal (Figure 7C) during the DDP. It was found that during the time course of diastolic depolarization, the averaged Itotal amplitude in the cell model with the human If formulations was much smaller (<30%) than that in the rabbit-like model, which produced a slower pacemaking rate (i.e., longer CL) and greater CL increase in response to 20% If reduction, matching the theoretical prediction. The changes in currents and Ca2+ ion concentration associated with the change in Itotal (Figures 7Bi,Bii) are shown in Figures 3, 4. A similar observation was also seen in the Fabbri et al. model as shown in Supplementary Figure 6C.
Figure 7. Simulation of the inverse relationship of Itotal and the increasing of CL in control (solid lines) and 20% If reduction (dotted lines) to validate theoretical analysis result (Equation 2) in method section (p = 0.2, modeling 20% If reduction). (Ai,Aii) Time course of action potentials for rabbit-like and human-like SAN cell models respectively (gray lines represents diastolic depolarization voltage changes from the MDP to the voltage at the beginning of AP upstroke in control condition); (Bi,Bii) time courses of Itotal (gray lines represents diastolic depolarization voltage changes from the MDP to the voltage at the beginning of AP upstroke in control condition). (C) Averaged value of Itotal amplitude during the diastolic depolarization phase for the rabbit-like (black) and human-like (gray) SAN cell models. In each case, the integral interval was set to the time interval between the MDP and beginning of the upstroke membrane potential (Vup).
In theoretical analysis it was shown that the relative increase of CL was also influenced by a factor of , which was related to the ratio of If to Itotal (x) and If block potency (p). In simulations, we further computed the values of and for control and If reduction cases. Results from the Severi et al. model are shown in Figure 8, in which the time courses of (Figures 8Ai,Aii) and (Figures 8Bi,Bii) were plotted for the control (black) and 20% If reduction (p = 0.2) for the rabbit-like (left panels) and human-like (the right panels) models. The computed (acting as a piecewise function) was set to when |1/x − 0.2| was smaller than 1 × 10-3 to avoid the value close to positive infinity. It was shown that in both models with a small If block the difference in the computed value of between control (solid line) and 20% If reduction (dotted line) was very small during diastolic depolarization phase, and also small when the value was normalized against Itotal (). This provides support for the notion that the relative increase of CL was mainly determined by the amplitude of Itotal during the diastolic depolarisation phase in response to If block.
Figure 8. Computed values of and to validate theoretical analysis result (Equation 2) in method section (p = 0.2, modeling 20% If reduction). The highlighted lines shown in this figure are during the diastolic depolarization phase of the action potentials of control (solid lines) and 20% If reduction (dotted lines) cases for the rabbit-like (Ai,Bi) and human-like (Aii,Bii) SAN cell models.
Note that during the last period of diastolic depolarisation phase (i.e., during time period of 310–387 ms as shown in the figure for the rabbit-like model and 890–1,331 ms for the human-like model), the difference in the computed values of and became more noticeable. This may be attributable to the different timings by which the upstroke of pacemaking actions potentials occurred between control and If reduction conditions. Note that the value of was also greater in the human-like model than that in the rabbit, which amplified the contribution of toward a relative increase of CL. Results from the Fabbri et al. model were similar, as shown in Supplementary Figure 7.
A marked difference in the V1/2 of the steady-state activation relationship (y∞) of If between the rabbit and the human SAN cells has been observed (Difrancesco et al., 1989; Altomare et al., 2003; Barbuti et al., 2007; Verkerk et al., 2007b). In this study, we used V1/2 of −52.5 and −97.1 mV for the rabbit-like and the human-like If formulation respectively. In order to systematically determine a possible role of varying V1/2 in modulating If amplitude, and thus the Itotal and the effect of If reduction on the increase of CL, we changed V1/2 of y∞ in the human-like If formulation in a border range from −50 to −70 mV. Results from the Serveri et al. model are shown in Figure 9 for the pacemaking CL (Figure 9A), the averaged Itotal during diastolic depolarization phase (Figure 8B), and increase of CL (Figure 9C) with 20% If block. Shifting the V1/2 from −50 mV (about rabbit V1/2) to −70 mV (about human V1/2), the Itotal was decreased (Figure 9B), which were correlated with an increased CL (Figure 9A) as well as an increased effect of If reduction on CL (Figure 9C). These results supported our theoretical analysis on that a smaller If in the model resulted in a smaller Itotal amplitude, resulting in a slower pacemaking rate; and as such the same reduction in If resulted in a more significant change of CL in the cell model with a smaller Itotal. Similar results were also observed using the Fabbri et al. model as shown in Supplementary Figure 8.
Figure 9. (A–C) Effect of varied half-activation voltage (V1/2) of the steady state activation variable (y∞) on pacemaking CL in the Serveri et al. model with human-like If formulation. V1/2 changed from −50 to −70 mV, covering the experimental data range for rabbit (−50 mV) and human (−97.1 mV) SAN cells.
This study was conducted to determine the mechanism by which a low level of If block by clinical drug (ivabradine) concentration is able to reduce the heart rate of patients by about 18–20%. In a previous study, Verkerk and Wilders found that though If has a small magnitude in the human SA node cells, it has an equally important role as in the rabbit (Verkerk and Wilders, 2010). In another study, Maltsev and Lakatta argued that If provides a relatively modest contribution to spontaneous beating rate regulation of human and rabbit sinoatrial node cells, and its major role in human SAN cells is to prevent excessive hyperpolarization during AP repolarization, thus representing an anti-bradycardic mechanism, rather than a primary rate regulatory mechanism (Maltsev and Lakatta, 2010). Though these previous studies addressed how a smaller If can produce the same effect in humans with respect to rabbit (Verkerk and Wilders, 2010; Fabbri et al., 2017) on complete If block, however, the question on how <20% If block produces a marked heart rate reduction in humans in vivo had remained unclear. This is due to the nonlinear relationship between the extent of If block and heart rate reduction (see Figure 5); effects of partial If block cannot be predicted with certainty from the complete block data already in the literature.
The principal contributions of the work are insights into how a small extent of If block (<20%; as may occur during clinical use of ivabradine) produces a marked heart rate reduction in human SAN, greater than that predicted by single cell experiments in the rabbit though the latter has a greater If density. Our principal findings are: (i) blocking If by 20% resulted in only about a 4.6 % increase in the CL in the rabbit-like SAN cell model, but about 12.4% in the cell model with human If formulation. This finding suggests that If block has a greater effect with the human-like SAN cell If formulation than with rabbit-like If formulation, despite the fact that the former has a smaller If current density over diastolic potentials, based on which one might expect a smaller contribution of If to pacemaking; (ii) there is a cross-talk between the membrane clock and Ca2+ clock with 20% If block in the later phase of the diastolic depolarization of the action potential; (iii) a theoretical analysis matching the simulation data has been produced, providing a numerical formalism explaining the relationship between If block effects and its contribution to total current during diastolic phase. In this study, both numerical simulations and theoretical analysis here have attributed the paradoxical effect of If reduction (i.e., a greater effect in SAN cells with smaller If current density and therefore a slower heart rate) to an inverse relationship between the relative increase of CL and the amplitude of the total current during the diastolic depolarization phase in response to If block; and (iv) significantly, vagal tone activity via ACh augments the effects of ivabradine on heart rate reduction, providing a possible mechanism(s) by which the clinical concentrations can have larger effects in vivo than those predicted by single cell experiments in vitro. It has been shown that combined action of ACh (0.1 nM) and 20% If reduction markedly increase the pacemaking CL by 37.5%, close to the clinical effect of ivabradine when human-like If formulation was used, which is significantly greater than that of 10.1% when the rabbit-like If formulation was used. These findings were also observed in the Fabbri et al. model.
The results of the present study demonstrate and explain why a smaller human If has a greater effect on prolonging the diastolic depolarization phase when it is partially blocked, using both mathematical theoretical analysis and computer simulation, which is clearly distinct from the previous studies (Verkerk and Wilders, 2010; Fabbri et al., 2017). Collectively, they add mechanistic insight into the understanding of how a low dose of clinical use of ivabradine (<137 nM) can effectively slow down the human heart rate by about 18–20% (Camm and Lau, 2003; Joannides et al., 2006; Doesch et al., 2007; Jiang et al., 2013), which contrasts with a negligible effect predicted by experimental studies in the rabbit (<4% at 10 min after administration of ivabradine; Thollon et al., 2007).
If channels encoded by HCN genes are richly expressed in cardiac conduction systems including the primary pacemaker, the SAN (Altomare et al., 2003; Ravagli et al., 2016). Previous studies from animal models have suggested an important role of If in the SAN (Difrancesco and Noble, 2012; Baruscotti et al., 2016; Kozasa et al., 2018). It has been shown that a complete block of If by Cs+ (2 mM) slowed down the pacemaking rate by 30% in the rabbit SAN cells (Denyer and Brown, 1990), a 17.6% reduction of pacemaking rate also seen when blocking of If with 0.5 mmol/L Cs+ (Liu et al., 2008). In transgenic mice, knocking down HCN4 produced bradycardiac effects as well as atrioventricular node conduction block (Herrmann et al., 2007; Hoesl et al., 2008; Baruscotti et al., 2011). All of this evidence demonstrates that If, together with the more recently identified Ca2+ clock [arising from the coupling between the intracellular Ca2+ cycling and electrogenesis of membrane currents (e.g., INaCa) (Maltsev and Lakatta, 2008; Lakatta and Difrancesco, 2009)], provide a major driving force for generating the spontaneous depolarization during the diastolic phase that leads to intrinsic pacemaker activity.
If is also present in human SAN cells (Verkerk et al., 2009b; Li et al., 2015) and contributes to pacemaking. It has been shown that loss-of-function of HCN channel mutations is associated with sick sinus syndrome, which manifests with symptoms of bradycardia and conduction block (Schweizer et al., 2014; Verkerk and Wilders, 2014, 2015). However, the functional role of If in generating pacemaking action potentials of human SAN is less well-characterized as compared to that from small mammals. Limited data have shown that If in the human SAN cells has a smaller current density, more negative depolarised membrane potential of half maximal activation and slower activation rate as compared to the rabbit (Verkerk et al., 2007a,b, 2009b). All of these suggested a smaller If current during the diastolic depolarization phase, which may result in a slower heart rate. Indeed this is the case as shown in the present simulation study. In the Severi et al. model (Severi et al., 2012) with the rabbit-like If formulation, the measured pacemaking cycle length was 354.8 ms. However, when the rabbit-like If formulation was replaced by the human one, the pacemaking rate was slowed down and the pacemaking cycle length increased to 1,139.4 ms, greater than the intrinsic pacemaking cycle length of native human SAN cells [about 828 ± 21 ms (Verkerk et al., 2007b)]. Note that in the model, such a significant increase of the pacemaking CL from the one of rabbit SAN cells to the one close to human SAN cells was mainly attributable to a small If, as no change or negligible secondary changes of other ion channels were implemented. This suggests that If exerts a strong influence on pacemaker rhythm. Note that there is a marked difference in the pacemaking CL between the human-like model (1,139.4 ms) and native human SAN cells [about 828 ± 21 ms (Verkerk et al., 2007b)], which may be due to possible species differences in the properties of other membrane currents (Fabbri et al., 2017) and Ca2+ clock (Tsutsui et al., 2018). With the increase of If blocking, the pacemaking CL increased non-linearly in the model with either rabbit-like or human-like If formulations.
In simulations, the action of 100% If block abolished the pacemaking in the model with rabbit-like If formulations. This may over emphasize the role of If in the rabbit SAN pacemaking and is non-physiological. However, the focus of this study is on the action of small If block (i.e., <20%), mimicking the use of effect of ivabradine in practice, rather than on the action of large percentage of If block. With <50% If block, the increase in CL is about <30%, which is reasonably close to the experimental data observed in rabbit SAN cells when If is blocked by use of Cs+ (Nikmaram et al., 1997).
Our theoretical and numerical simulation results have shown that a low level of If block, mimicking the clinical concentrations of ivabradine, produced a more marked effect in reducing the heart rate of the human-like If formulation SAN cell model than the rabbit-like If formulation SAN cell model. When the action of ACh (0.1 nM) was considered, there was a further reduction of sponteneous pacing rate (reduced upto by 27.3%), which is close to the effect of ivabradine at clinical concentration. Results from the Fabbri et al. model were similar, showing that these observations are model-independent. All these results suggest that a combined action of If reduction by ivabradine at clinical concentrations and ACh are attributable to the heart rate reduction as seen clinically (Camm and Lau, 2003; Doesch et al., 2007).
It is possible that ivabradine regulates the heart rate by a cross-talk between the membrane clock and Ca2+ clock via the electrogenic Na+-Ca2+ exchangers (Yaniv et al., 2013). While the direct action of ivabradine on the intracellular Ca2+ handling is unclear, our simulation results showed that a 20% If reduction produced secondary modulations of other ionic currents (e.g., INaCa) and the intracellular Ca2+ handling, suggesting there is a cross-talk between the membrane clock and Ca2+ clock in the late phase of the diastolic depolarization of the action potential (Figures 4Bii–Eii). All these illustrated that ivabradine affected the heart rate at clinical concentrations mainly through regulating membrane clock and Ca2+ clock, and combining with the action of ACh.
Possible limitations of the Severi et al. model of the rabbit SAN cells have been well-discussed and documented (Severi et al., 2012). For example, as highlighted by Verkerk and Wilders (2014), the reversal potential of If in the Severi et al. model (−4.39 mV) was more positive than that experimentally reported (about −30 mV) (Difrancesco et al., 1986; Van Ginneken and Giles, 1991; Verkerk et al., 2009a), which is a limitation of the Severi et al. model, and therefore also of studies employing this model. However, we did not modify the If equation of the Severi et al. model for the following reasons: (1) the simulated I-V relationship data shown in (Figure 1C) lie within the range of experimental data, and If is very small when the voltage is more positive than −40 mV; (2) when the voltage is more positive than −30 mV, the activation of ICaL is dominant, which contributes mainly to the upstroke phase (non-diastolic depolarization phase) of the action potential. Therefore, even if the reversal potential of If is changed from −30 mV to −4.39 mV, it has negligible effect on the diastolic depolarization of spontaneous action potentials. Moreover, in simulations in which the rabbit-like If was replaced by the human-like formulations in the Severi et al. model, the reversal potential of If used was −27.5 mV, close to the experimentally determined value of −22.1 ± 2.4 mV (Verkerk et al., 2007b).
Another potential limitation of the present study relates to modification of the Severi et al. model to incorporate the human If formulation developed by Fabbri et al. based on experimental data from human SAN cells. Due to lack of experimental data from human SAN cells, equations and parameters for other ion channels and transporters in the Severi et al. model were not updated. Note that experimental data on the Ca2+ clock of the human SAN became available recently (Tsutsui et al., 2018), which are not incorporated in the model yet. This may explain why the computed CL of the human-like SAN cell is greater than that of natural human SAN cells, and consequently the smaller effect of If blocking on the reduction of the heart rate the clinical data. In addition, the action of low dose ivabradine was simulated by considering its action on blocking If only, and did not incorporate its possible actions on IKr as seen in some experimental studies on ventricular cells/tissue at low dose (Melgari et al., 2015) or high doses (Lees-Miller et al., 2015). However, the present study deliberately focused on the difference in direct effects of ivabradine on If in the SAN cells between species (If in the rabbit SAN and in the human-like SAN). Whilst it is necessary to point out these potential limitations, nevertheless, the simulation data strongly supported the mechanism demonstrated by the theoretical analysis in showing the inverse correlation between Itotal during the diastolic depolarization phase and the relative increase of the CL. Therefore, these limitations do not alter our major conclusion on the role of If block in modulating cardiac pacemaking activities in the human SAN by low concentrations of ivabradine.
An inverse correlation between the relative increase of CL and the amplitude of the total ion channel current during the diastolic depolarization phase has been observed. Both theoretical analysis and simulations have shown that a low level of If block (<20%) can produce a more marked reduction of in the pacemaking rate of the human-like SAN cell model than the rabbit-like one due to its smaller Itotal during the diastolic depolarization phase. This was particularly the case when ACh actions were considered, which amplified the pacemaking cycle length prolongation. This study thus provides a mechanistic explanation into how a low level of If block by the clinical concentrations of ivabradine can effectively reduce the heart rate in humans but produce a small or negligible effect in the rabbit.
The original contributions presented in the study are included in the article/Supplementary Material, further inquiries can be directed to the corresponding author/s.
The code for the models employed is available on request to Henggui Zhang at aGVuZ2d1aS56aGFuZ0BtYW5jaGVzdGVyLmFjLnVr.
HZ and JH conceived the study. XB conducted simulation and data analysis. HZ and MB contributed to theoretical analysis. XB, KW, MB, JH, and HZ wrote the paper. All authors contributed to the article and approved the submitted version.
This work was supported by grants from the British Heart Foundation FS/14/5/30533, EPSRC (UK) (EP/J00958X/1; EP/I029826/1), Shenzhen Science and Technology Innovation Committee (JCYJ20151029173639477; JSGG2016022912504 9615), and Scientific research plan projects of Shaanxi Education Department (20JK0917). JH received a University of Bristol Research Fellowship.
The authors declare that the research was conducted in the absence of any commercial or financial relationships that could be construed as a potential conflict of interest.
All claims expressed in this article are solely those of the authors and do not necessarily represent those of their affiliated organizations, or those of the publisher, the editors and the reviewers. Any product that may be evaluated in this article, or claim that may be made by its manufacturer, is not guaranteed or endorsed by the publisher.
We are grateful to Dr. Severi's group for providing us the source code of the Severi et al. model.
The Supplementary Material for this article can be found online at: https://www.frontiersin.org/articles/10.3389/fphys.2021.582037/full#supplementary-material
Accili, E. A., Robinson, R. B., and Difrancesco, D. (1997). Properties and modulation of If in newborn versus adult cardiac SA node. Am. J. Physiol. 272, H1549–H1552. doi: 10.1152/ajpheart.1997.272.3.H1549
Altomare, C., Terragni, B., Brioschi, C., Milanesi, R., Pagliuca, C., Viscomi, C., et al. (2003). Heteromeric HCN1-HCN4 channels: a comparison with native pacemaker channels from the rabbit sinoatrial node. J. Physiol. 549, 347–359. doi: 10.1113/jphysiol.2002.027698
Barbuti, A., Baruscotti, M., and Difrancesco, D. (2007). The pacemaker current: from basics to the clinics. J. Cardiovasc. Electrophysiol. 18, 342–347. doi: 10.1111/j.1540-8167.2006.00736.x
Baruscotti, M., Bianco, E., Bucchi, A., and Difrancesco, D. (2016). Current understanding of the pathophysiological mechanisms responsible for inappropriate sinus tachycardia: role of the If “funny” current. J. Interv. Card Electrophysiol. 46, 19–28. doi: 10.1007/s10840-015-0097-y
Baruscotti, M., Bucchi, A., and Difrancesco, D. (2005). Physiology and pharmacology of the cardiac pacemaker (“funny”) current. Pharmacol. Ther. 107, 59–79. doi: 10.1016/j.pharmthera.2005.01.005
Baruscotti, M., Bucchi, A., Viscomi, C., Mandelli, G., Consalez, G., Gnecchi-Rusconi, T., et al. (2011). Deep bradycardia and heart block caused by inducible cardiac-specific knockout of the pacemaker channel gene Hcn4. Proc. Natl. Acad. Sci. U. S. A. 108, 1705–1710. doi: 10.1073/pnas.1010122108
Bohm, M., Robertson, M., Ford, I., Borer, J. S., Komajda, M., Kindermann, I., et al. (2015). Influence of cardiovascular and noncardiovascular co-morbidities on outcomes and treatment effect of heart rate reduction with ivabradine in stable heart failure (from the SHIFT trial). Am. J. Cardiol. 116, 1890–1897. doi: 10.1016/j.amjcard.2015.09.029
Bois, P., Bescond, J., Renaudon, B., and Lenfant, J. (1996). Mode of action of bradycardic agent, S 16257, on ionic currents of rabbit sinoatrial node cells. Br. J. Pharmacol. 118, 1051–1057. doi: 10.1111/j.1476-5381.1996.tb15505.x
Bois, P., Guinamard, R., Chemaly, A. E., Faivre, J. F., and Bescond, J. (2007). Molecular regulation and pharmacology of pacemaker channels. Curr. Pharm. Des. 13, 2338–2349. doi: 10.2174/138161207781368729
Boyett, M. R. (2009). “And the beat goes on.” The cardiac conduction system: the wiring system of the heart. Exp. Physiol. 94, 1035–1049. doi: 10.1113/expphysiol.2009.046920
Boyett, M. R., Kodama, I., Honjo, H., Arai, A., Suzuki, R., and Toyama, J. (1995). Ionic basis of the chronotropic effect of acetylcholine on the rabbit sinoatrial node. Cardiovasc. Res. 29, 867–878. doi: 10.1016/S0008-6363(96)88625-1
Brioschi, C., Micheloni, S., Tellez, J. O., Pisoni, G., Longhi, R., Moroni, P., et al. (2009). Distribution of the pacemaker HCN4 channel mRNA and protein in the rabbit sinoatrial node. J. Mol. Cell Cardiol. 47, 221–227. doi: 10.1016/j.yjmcc.2009.04.009
Bucchi, A., Baruscotti, M., and Difrancesco, D. (2002). Current-dependent block of rabbit sino-atrial node If channels by ivabradine. J. Gen. Physiol. 120, 1–13. doi: 10.1085/jgp.20028593
Bucchi, A., Baruscotti, M., Nardini, M., Barbuti, A., Micheloni, S., Bolognesi, M., et al. (2013). Identification of the molecular site of ivabradine binding to HCN4 channels. PLoS ONE 8:e53132. doi: 10.1371/journal.pone.0053132
Bucchi, A., Baruscotti, M., Robinson, R. B., and Difrancesco, D. (2003). If-dependent modulation of pacemaker rate mediated by cAMP in the presence of ryanodine in rabbit sino-atrial node cells. J. Mol. Cell Cardiol. 35, 905–913. doi: 10.1016/S0022-2828(03)00150-0
Bucchi, A., Baruscotti, M., Robinson, R. B., and Difrancesco, D. (2007). Modulation of rate by autonomic agonists in SAN cells involves changes in diastolic depolarization and the pacemaker current. J. Mol. Cell Cardiol. 43, 39–48. doi: 10.1016/j.yjmcc.2007.04.017
Bucchi, A., Tognati, A., Milanesi, R., Baruscotti, M., and Difrancesco, D. (2006). Properties of ivabradine-induced block of HCN1 and HCN4 pacemaker channels. J. Physiol. 572, 335–346. doi: 10.1113/jphysiol.2005.100776
Camici, P. G., Gloekler, S., Levy, B. I., Skalidis, E., Tagliamonte, E., Vardas, P., et al. (2016). Ivabradine in chronic stable angina: effects by and beyond heart rate reduction. Int. J. Cardiol. 215, 1–6. doi: 10.1016/j.ijcard.2016.04.001
Camm, A. J., and Lau, C. P. (2003). Electrophysiological effects of a single intravenous administration of ivabradine (S 16257) in adult patients with normal baseline electrophysiology. Drugs R D 4, 83–89. doi: 10.2165/00126839-200304020-00001
Chandler, N. J., Greener, I. D., Tellez, J. O., Inada, S., Musa, H., Molenaar, P., et al. (2009). Molecular architecture of the human sinus node: insights into the function of the cardiac pacemaker. Circulation 119, 1562–1575. doi: 10.1161/CIRCULATIONAHA.108.804369
Choi, H. Y., Noh, Y. H., Cho, S. H., Ghim, J. L., Choe, S., Kim, U. J., et al. (2013). Evaluation of pharmacokinetic and pharmacodynamic profiles and tolerability after single (2.5, 5, or 10 mg) and repeated (2.5, 5, or 10 mg bid for 4.5 days) oral administration of ivabradine in healthy male Korean volunteers. Clin. Ther. 35, 819–835. doi: 10.1016/j.clinthera.2013.04.012
Craven, K. B., and Zagotta, W. N. (2006). CNG and HCN channels: two peas, one pod. Annu. Rev. Physiol. 68, 375–401. doi: 10.1146/annurev.physiol.68.040104.134728
Denyer, J. C., and Brown, H. F. (1990). Pacemaking in rabbit isolated sino-atrial node cells during Cs+ block of the hyperpolarization-activated current if. J. Physiol. 429, 401–409. doi: 10.1113/jphysiol.1990.sp018264
Difrancesco, D. (1991). The contribution of the “pacemaker” current (if) to generation of spontaneous activity in rabbit sino-atrial node myocytes. J. Physiol. 434, 23–40. doi: 10.1113/jphysiol.1991.sp018457
Difrancesco, D. (2010). The role of the funny current in pacemaker activity. Circ. Res. 106, 434–446. doi: 10.1161/CIRCRESAHA.109.208041
Difrancesco, D., Ducouret, P., and Robinson, R. B. (1989). Muscarinic modulation of cardiac rate at low acetylcholine concentrations. Science 243, 669–671. doi: 10.1126/science.2916119
Difrancesco, D., Ferroni, A., Mazzanti, M., and Tromba, C. (1986). Properties of the hyperpolarizing-activated current (if) in cells isolated from the rabbit sino-atrial node. J. Physiol. 377, 61–88. doi: 10.1113/jphysiol.1986.sp016177
Difrancesco, D., and Noble, D. (2012). The funny current has a major pacemaking role in the sinus node. Heart Rhythm 9, 299–301. doi: 10.1016/j.hrthm.2011.09.021
Doesch, A. O., Celik, S., Ehlermann, P., Frankenstein, L., Zehelein, J., Koch, A., et al. (2007). Heart rate reduction after heart transplantation with beta-blocker versus the selective If channel antagonist ivabradine. Transplantation 84, 988–996. doi: 10.1097/01.tp.0000285265.86954.80
Fabbri, A., Fantini, M., Wilders, R., and Severi, S. (2017). Computational analysis of the human sinus node action potential: model development and effects of mutations. J. Physiol. 595, 2365–2396. doi: 10.1113/JP273259
Goethals, M., Raes, A., and Van Bogaert, P. P. (1993). Use-dependent block of the pacemaker current I(f) in rabbit sinoatrial node cells by zatebradine (UL-FS 49). On the mode of action of sinus node inhibitors. Circulation 88, 2389–2401. doi: 10.1161/01.CIR.88.5.2389
Hagiwara, N., and Irisawa, H. (1989). Modulation by intracellular Ca2+ of the hyperpolarization-activated inward current in rabbit single sino-atrial node cells. J. Physiol. 409, 121–141. doi: 10.1113/jphysiol.1989.sp017488
Hagiwara, N., Irisawa, H., and Kameyama, M. (1988). Contribution of two types of calcium currents to the pacemaker potentials of rabbit sino-atrial node cells. J. Physiol. 395, 233–253. doi: 10.1113/jphysiol.1988.sp016916
Herrmann, S., Stieber, J., Stockl, G., Hofmann, F., and Ludwig, A. (2007). HCN4 provides a “depolarization reserve” and is not required for heart rate acceleration in mice. EMBO J. 26, 4423–4432. doi: 10.1038/sj.emboj.7601868
Hoesl, E., Stieber, J., Herrmann, S., Feil, S., Tybl, E., Hofmann, F., et al. (2008). Tamoxifen-inducible gene deletion in the cardiac conduction system. J. Mol. Cell Cardiol. 45, 62–69. doi: 10.1016/j.yjmcc.2008.04.008
Irisawa, H., Brown, H. F., and Giles, W. (1993). Cardiac pacemaking in the sinoatrial node. Physiol. Rev. 73, 197–227. doi: 10.1152/physrev.1993.73.1.197
Jiang, J., Tian, L., Huang, Y., Li, Y., and Xu, L. (2013). Pharmacokinetic and safety profile of ivabradine in healthy Chinese men: a phase I, randomized, open-label, increasing single- and multiple-dose study. Clin. Ther. 35, 1933–1945. doi: 10.1016/j.clinthera.2013.10.007
Joannides, R., Moore, N., Iacob, M., Compagnon, P., Lerebours, G., Menard, J. F., et al. (2006). Comparative effects of ivabradine, a selective heart rate-lowering agent, and propranolol on systemic and cardiac haemodynamics at rest and during exercise. Br. J. Clin. Pharmacol. 61, 127–137. doi: 10.1111/j.1365-2125.2005.02544.x
Kozasa, Y., Nakashima, N., Ito, M., Ishikawa, T., Kimoto, H., Ushijima, K., et al. (2018). HCN4 pacemaker channels attenuate the parasympathetic response and stabilize the spontaneous firing of the sinoatrial node. J. Physiol. 596, 809–825. doi: 10.1113/JP275303
Lakatta, E. G., and Difrancesco, D. (2009). What keeps us ticking: a funny current, a calcium clock, or both? J. Mol. Cell Cardiol. 47, 157–170. doi: 10.1016/j.yjmcc.2009.03.022
Lakatta, E. G., Maltsev, V. A., and Vinogradova, T. M. (2010). A coupled SYSTEM of intracellular Ca2+ clocks and surface membrane voltage clocks controls the timekeeping mechanism of the heart's pacemaker. Circ. Res. 106, 659–673. doi: 10.1161/CIRCRESAHA.109.206078
Lees-Miller, J. P., Guo, J., Wang, Y., Perissinotti, L. L., Noskov, S. Y., and Duff, H. J. (2015). Ivabradine prolongs phase 3 of cardiac repolarization and blocks the hERG1 (KCNH2) current over a concentration-range overlapping with that required to block HCN4. J. Mol. Cell Cardiol. 85, 71–78. doi: 10.1016/j.yjmcc.2015.05.009
Li, N., Csepe, T. A., Hansen, B. J., Dobrzynski, H., Higgins, R. S., Kilic, A., et al. (2015). Molecular mapping of sinoatrial node HCN channel expression in the human heart. Circ. Arrhythm Electrophysiol. 8, 1219–1227. doi: 10.1161/CIRCEP.115.003070
Liu, J., Noble, P. J., Xiao, G., Abdelrahman, M., Dobrzynski, H., Boyett, M. R., et al. (2008). Role of pacemaking current in cardiac nodes: insights from a comparative study of sinoatrial node and atrioventricular node. Prog. Biophys. Mol. Biol. 96, 294–304. doi: 10.1016/j.pbiomolbio.2007.07.009
Madle, A., Linhartova, K., and Koza, J. (2001). Effects of the T-type calcium channel blockade with oral mibefradil on the electrophysiologic properties of the human heart. Med. Sci. Monit. 7, 74–77.
Maltsev, V. A., and Lakatta, E. G. (2008). Dynamic interactions of an intracellular Ca2+ clock and membrane ion channel clock underlie robust initiation and regulation of cardiac pacemaker function. Cardiovasc. Res. 77, 274–284. doi: 10.1093/cvr/cvm058
Maltsev, V. A., and Lakatta, E. G. (2010). Funny current provides a relatively modest contribution to spontaneous beating rate regulation of human and rabbit sinoatrial node cells. J. Mol. Cell Cardiol. 48, 804–806. doi: 10.1016/j.yjmcc.2009.12.009
Mangoni, M. E., and Nargeot, J. (2008). Genesis and regulation of the heart automaticity. Physiol. Rev. 88, 919–982. doi: 10.1152/physrev.00018.2007
Melgari, D., Brack, K. E., Zhang, C., Zhang, Y., El Harchi, A., Mitcheson, J. S., et al. (2015). hERG potassium channel blockade by the HCN channel inhibitor bradycardic agent ivabradine. J. Am. Heart Assoc. 4:1813. doi: 10.1161/JAHA.115.001813
Monfredi, O., Lyashkov, A. E., Johnsen, A. B., Inada, S., Schneider, H., Wang, R., et al. (2014). Biophysical characterization of the underappreciated and important relationship between heart rate variability and heart rate. Hypertension 64, 1334–1343. doi: 10.1161/HYPERTENSIONAHA.114.03782
Niccoli, G., Borovac, J. A., Vetrugno, V., Camici, P. G., and Crea, F. (2017). Ivabradine in acute coronary syndromes: protection beyond heart rate lowering. Int. J. Cardiol. 236, 107–112. doi: 10.1016/j.ijcard.2017.02.046
Nikmaram, M. R., Boyett, M. R., Kodama, I., Suzuki, R., and Honjo, H. (1997). Variation in effects of Cs+, UL-FS-49, and ZD-7288 within sinoatrial node. Am. J. Physiol. 272, H2782–H2792. doi: 10.1152/ajpheart.1997.272.6.H2782
Ravagli, E., Bucchi, A., Bartolucci, C., Paina, M., Baruscotti, M., Difrancesco, D., et al. (2016). Cell-specific Dynamic Clamp analysis of the role of funny If current in cardiac pacemaking. Prog. Biophys. Mol. Biol. 120, 50–66. doi: 10.1016/j.pbiomolbio.2015.12.004
Rocchetti, M., Malfatto, G., Lombardi, F., and Zaza, A. (2000). Role of the input/output relation of sinoatrial myocytes in cholinergic modulation of heart rate variability. J. Cardiovasc. Electrophysiol. 11, 522–530. doi: 10.1111/j.1540-8167.2000.tb00005.x
Schweizer, P. A., Schroter, J., Greiner, S., Haas, J., Yampolsky, P., Mereles, D., et al. (2014). The symptom complex of familial sinus node dysfunction and myocardial noncompaction is associated with mutations in the HCN4 channel. J. Am. Coll. Cardiol. 64, 757–767. doi: 10.1016/j.jacc.2014.06.1155
Severi, S., Fantini, M., Charawi, L. A., and Difrancesco, D. (2012). An updated computational model of rabbit sinoatrial action potential to investigate the mechanisms of heart rate modulation. J. Physiol. 590, 4483–4499. doi: 10.1113/jphysiol.2012.229435
Takeda, K., Yamagishi, R., Masumiya, H., Tanaka, H., and Shigenobu, K. (2004). Effect of cilnidipine on L- and T-type calcium currents in guinea pig ventricle and action potential in rabbit sinoatrial node. J. Pharmacol. Sci. 95, 398–401. doi: 10.1254/jphs.SCJ04001X
Tardif, J. C., Ford, I., Tendera, M., Bourassa, M. G., Fox, K., and Investigators, I. (2005). Efficacy of ivabradine, a new selective If inhibitor, compared with atenolol in patients with chronic stable angina. Eur. Heart J. 26, 2529–2536. doi: 10.1093/eurheartj/ehi586
Thollon, C., Bedut, S., Villeneuve, N., Coge, F., Piffard, L., Guillaumin, J. P., et al. (2007). Use-dependent inhibition of hHCN4 by ivabradine and relationship with reduction in pacemaker activity. Br. J. Pharmacol. 150, 37–46. doi: 10.1038/sj.bjp.0706940
Thollon, C., Cambarrat, C., Vian, J., Prost, J. F., Peglion, J. L., and Vilaine, J. P. (1994). Electrophysiological effects of S 16257, a novel sino-atrial node modulator, on rabbit and guinea-pig cardiac preparations: comparison with UL-FS 49. Br. J. Pharmacol. 112, 37–42. doi: 10.1111/j.1476-5381.1994.tb13025.x
Tsutsui, K., Monfredi, O. J., Sirenko-Tagirova, S. G., Maltseva, L. A., Bychkov, R., Kim, M. S., et al. (2018). A coupled-clock system drives the automaticity of human sinoatrial nodal pacemaker cells. Sci. Signal 11:aap7608. doi: 10.1126/scisignal.aap7608
Van Ginneken, A. C., and Giles, W. (1991). Voltage clamp measurements of the hyperpolarization-activated inward current I(f) in single cells from rabbit sino-atrial node. J. Physiol. 434, 57–83. doi: 10.1113/jphysiol.1991.sp018459
Verkerk, A. O., Den Ruijter, H. M., Bourier, J., Boukens, B. J., Brouwer, I. A., Wilders, R., et al. (2009a). Dietary fish oil reduces pacemaker current and heart rate in rabbit. Heart Rhythm. 6, 1485–1492. doi: 10.1016/j.hrthm.2009.07.024
Verkerk, A. O., Van Borren, M. M., Peters, R. J., Broekhuis, E., Lam, K. Y., Coronel, R., et al. (2007a). Single cells isolated from human sinoatrial node: action potentials and numerical reconstruction of pacemaker current. Conf. Proc. IEEE Eng. Med. Biol. Soc. 2007, 904–907. doi: 10.1109/IEMBS.2007.4352437
Verkerk, A. O., Van Ginneken, A. C., and Wilders, R. (2009b). Pacemaker activity of the human sinoatrial node: role of the hyperpolarization-activated current, If. Int. J. Cardiol. 132, 318–336. doi: 10.1016/j.ijcard.2008.12.196
Verkerk, A. O., and Wilders, R. (2010). Relative importance of funny current in human versus rabbit sinoatrial node. J. Mol. Cell Cardiol. 48, 799–801. doi: 10.1016/j.yjmcc.2009.09.020
Verkerk, A. O., and Wilders, R. (2014). Pacemaker activity of the human sinoatrial node: effects of HCN4 mutations on the hyperpolarization-activated current. Europace 16, 384–395. doi: 10.1093/europace/eut348
Verkerk, A. O., and Wilders, R. (2015). Pacemaker activity of the human sinoatrial node: an update on the effects of mutations in HCN4 on the hyperpolarization-activated current. Int. J. Mol. Sci. 16, 3071–3094. doi: 10.3390/ijms16023071
Verkerk, A. O., Wilders, R., Van Borren, M. M. G. J., Peters, R. J. G., Broekhuis, E., Lam, K., et al. (2007b). Pacemaker current (lf) in the human sinoatrial node. Eur. Heart J. 28, 2472–2478. doi: 10.1093/eurheartj/ehm339
Voigt, N., Abu-Taha, I., Heijman, J., and Dobrev, D. (2014). Constitutive activity of the acetylcholine-activated potassium current IK,ACh in cardiomyocytes. Adv. Pharmacol. 70, 393–409. doi: 10.1016/B978-0-12-417197-8.00013-4
Winter, J., and Shattock, M. J. (2016). Geometrical considerations in cardiac electrophysiology and arrhythmogenesis. Europace 18, 320–331. doi: 10.1093/europace/euv307
Yancy, C. W., Jessup, M., Bozkurt, B., Butler, J., Casey, D. E. Jr., Colvin, M. M., et al. (2016). 2016 ACC/AHA/HFSA focused update on new pharmacological therapy for heart failure: an update of the 2013 ACCF/AHA guideline for the management of heart failure: a report of the American College of Cardiology/American Heart Association Task Force on Clinical Practice Guidelines and the Heart Failure Society of America. J. Am. Coll. Cardiol. 68, 1476–1488. doi: 10.1016/j.jacc.2016.05.011
Yaniv, Y., Maltsev, V. A., Ziman, B. D., Spurgeon, H. A., and Lakatta, E. G. (2012). The “funny” current (If) inhibition by ivabradine at membrane potentials encompassing spontaneous depolarization in pacemaker cells. Molecules 17, 8241–8254. doi: 10.3390/molecules17078241
Yaniv, Y., Sirenko, S., Ziman, B. D., Spurgeon, H. A., Maltsev, V. A., and Lakatta, E. G. (2013). New evidence for coupled clock regulation of the normal automaticity of sinoatrial nodal pacemaker cells: bradycardic effects of ivabradine are linked to suppression of intracellular Ca2+ cycling. J. Mol. Cell Cardiol. 62, 80–89. doi: 10.1016/j.yjmcc.2013.04.026
Yuan, Y., Bai, X., Luo, C., Wang, K., and Zhang, H. (2015). The virtual heart as a platform for screening drug cardiotoxicity. Br. J. Pharmacol. 172, 5531–5547. doi: 10.1111/bph.12996
Zaza, A., and Lombardi, F. (2001). Autonomic indexes based on the analysis of heart rate variability: a view from the sinus node. Cardiovasc. Res. 50, 434–442. doi: 10.1016/S0008-6363(01)00240-1
Keywords: human and rabbit sinoatrial node, funny current, bradycardic agent (ivabradine), electrophysiological simulation, theoretical analysis
Citation: Bai X, Wang K, Boyett MR, Hancox JC and Zhang H (2021) The Functional Role of Hyperpolarization Activated Current (If) on Cardiac Pacemaking in Human vs. in the Rabbit Sinoatrial Node: A Simulation and Theoretical Study. Front. Physiol. 12:582037. doi: 10.3389/fphys.2021.582037
Received: 10 July 2020; Accepted: 23 July 2021;
Published: 19 August 2021.
Edited by:
Gerard J. J. Boink, University of Amsterdam, NetherlandsReviewed by:
Thomas Hund, The Ohio State University, United StatesCopyright © 2021 Bai, Wang, Boyett, Hancox and Zhang. This is an open-access article distributed under the terms of the Creative Commons Attribution License (CC BY). The use, distribution or reproduction in other forums is permitted, provided the original author(s) and the copyright owner(s) are credited and that the original publication in this journal is cited, in accordance with accepted academic practice. No use, distribution or reproduction is permitted which does not comply with these terms.
*Correspondence: Henggui Zhang, aGVuZ2d1aS56aGFuZ0BtYW5jaGVzdGVyLmFjLnVr
Disclaimer: All claims expressed in this article are solely those of the authors and do not necessarily represent those of their affiliated organizations, or those of the publisher, the editors and the reviewers. Any product that may be evaluated in this article or claim that may be made by its manufacturer is not guaranteed or endorsed by the publisher.
Research integrity at Frontiers
Learn more about the work of our research integrity team to safeguard the quality of each article we publish.