- 1Department of Women’s and Children’s Health, Karolinska Institutet, Stockholm, Sweden
- 2Astrid Lindgren Children′s Hospital Karolinska University Hospital, Stockholm, Sweden
Because of the polysystemic nature of coronavirus disease 2019 (COVID-19), during the present pandemic, there have been serious concerns regarding pregnancy, vertical transmission, and intrapartum risk. The majority of pregnant patients with COVID-19 infection present with mild or asymptomatic course of the disease. Some cases were hospitalized, and few needed intensive care unit admission, or mechanical ventilation. There have also been scarce case reports where neonates required mechanical ventilation post COVID-19 pregnancies. Without approved therapies other than dexamethasone, advanced mesenchymal cell therapy is one immunomodulatory therapeutic approach that is currently explored and might hold great promise. We suggest that the circulating fetal stem cells might have an immune-protective effect to mothers and contribute to the often mild and even asymptomatic post-COVID-19 pregnancies. Thus, COVID-19 pregnancies come forth as a paradigm to be further and more comprehensively approached, to understand both the mechanism and action of circulating stem cells in immunoprotection and hypoxia in microcirculation.
Introduction
Maternal–fetal transmission of viral diseases may occur transvaginally or through the hematogenic, i.e., the transplacental transmission pathway. In the latter, the virus circulating in the maternal blood vessels may reach and enter the placenta across chorionic villous and non-villous structures fetal blood vessels and be transmitted to the fetus. This mechanism of vertical transmission was not reported after the infection of pregnant women with the coronaviruses, severe acute respiratory syndrome coronavirus 1 (SARS-CoV-1) and Middle East respiratory syndrome coronavirus (MERS-CoV). Despite the fact that pregnant women may be infected by these coronaviruses other than severe pneumonia, they may face complications such as early pregnancy loss or even death (Schwartz and Graham, 2020).
The uterine enlargement during pregnancy is known to bring major changes in maternal physiology. These can be both mechanical, such as reduced functional residual volumes and diaphragm elevation, and cellular, such as altered cellular immunity. The maternal immune system may tolerate fetal antigens suppressing cell-mediated immunity while retaining normal humoral immunity, changes known to occur locally at the maternal–fetal interface, which could affect systemic immune responses to infection (Jamieson et al., 2006). This may render pregnant women more vulnerable to viral infections, especially in cases where the infections may have an effect on the cardiorespiratory system during pregnancy and could enhance progression to respiratory failure (Dashraath et al., 2020). The ability to increase ventilation is reduced, when pregnant. Therefore, there is an increased risk of inadequate response to environmental stressors such as upper/lower respiratory tract infections and hypoxic and hypercapnic respiratory failure (Lapinsky et al., 2014). During the SARS epidemic, up to 35% of the infected pregnant patients required mechanical ventilation, and mortality rates reached 18%; in the case of MERS, the numbers reached 41 and 25%, respectively (Dashraath et al., 2020; Liu et al., 2020; Schwartz and Graham, 2020).
SARS–Coronavirus 2 in Pregnancy
Coronavirus disease 2019 (COVID-19) is a polysystemic disease, aggravated by compromised immune response, increased body mass index, and other comorbidities. Thus, there have been serious concerns regarding the pregnancy and the vertical transmission and intrapartum risk (Ferrazzi et al., 2020; Gupta et al., 2020).
The majority of pregnant patients with COVID-19 infection present with a mild or asymptomatic course of the disease (Ferrazzi et al., 2020). Some cases were hospitalized, and few needed intensive care unit admission or mechanical ventilation; some patients received oxygen support, whereas others were treated with antibiotics, antivirals, systemic corticosteroids, and other treatment combinations (Breslin et al., 2020; Chen H. et al., 2020; Dashraath et al., 2020; Friedman et al., 2020; Hecht et al., 2020; Iqbal et al., 2020; Liu et al., 2020; Oncel et al., 2020; Savasi et al., 2020; Takemoto et al., 2020), while several clinical trials are ongoing to evaluate the choice of treatment in pregnant or breastfeeding COVID-19 patients (Dashraath et al., 2020; Pastick et al., 2020). There have also been some case reports where neonates required mechanical ventilation post-COVID-19 pregnancies (Alwardi et al., 2020; Amaral et al., 2020; Gale et al., 2020; Gregorio-Hernández et al., 2020; Kirtsman et al., 2020; Oncel et al., 2020; Savasi et al., 2020). The studies that concern maternal–fetal transmission of virus have some limitations. First, analyses of SARS coronavirus 2 (SARS-CoV-2) in newborns are often delayed; thus, there is an increased risk of extrauterine transmission. Second, the majority of the present case reports include the analysis of late vaginal smears (Zeng L. et al., 2020), instead of the sampling of amniotic fluid. The sampling of amniotic fluid could have provided an indication that vertical transmission might have occurred. A few studies investigated SARS-CoV-2-positive placental sampling to document direct viral involvement or vertical transmission (Alamar et al., 2020; Schwartz and Morotti, 2020; Smithgall et al., 2020; Taglauer et al., 2020). Although there have been case reports of histomorphologic evidence of maternal/fetal vascular malperfusions (Smithgall et al., 2020), there is still no concrete proof that SARS-CoV-2 placental invasion may lead to fetal pathology.
Different studies have shown that prevalence of COVID-19 test positivity among small studies of neonates vary, from less than 1% and up to 5% (Ashraf et al., 2020; Dumitriu et al., 2020; Khoury et al., 2020; Remaeus et al., 2020; Verma et al., 2020; Walker et al., 2020). Meanwhile, several study groups are actively investigating the subject of SARS-CoV-2 vertical transmission of infection, focusing on sample collection as early as possible and ideally within the first 12 h after birth. One of them is the periCOVID (COVID-19 Clinical Research Coalition, 2021) study, set up in the United Kingdom under the umbrella of Public Health England, also recruiting in Africa. The primary objective of the periCOVID surveillance study is to assess the risk of COVID-19 vertical transmission and identify and determine the routes, collecting samples from breast milk, placenta, and cord blood at birth for reverse transcriptase–polymerase chain reaction (RT-PCR) and sequencing followed by sequential sampling of maternal and neonatal urine and feces and blood samples for serology.
Some case reports and small studies have indicated that maternal–fetal transmission might occur (Table 1). They report different combinations of positive RT-PCR testing of neonatal nasopharyngeal swabs at birth followed with negative serology and later seroconversion of the mother (Alzamora et al., 2020), negative RT-PCR results of nasopharyngeal swab (Dong et al., 2020; He et al., 2020; Zeng H. et al., 2020) and positive neonatal blood serology immediately after birth, and several other combinations of COVID-19 diagnostic results. Unfortunately, neither amniotic fluid and placenta nor cord blood was tested in most cases. Immunoglobulin M (IgM) against SARS-CoV-2 proteins has been detected in some newborn case reports. Thus, as IgM does not cross the placental barrier, it raises the possibility of vertical transmission of the virus leading to IgM production by the fetus. However, this is not conclusive evidence and may also be due to placental alterations allowing the passage of IgM, or false-positive testing.
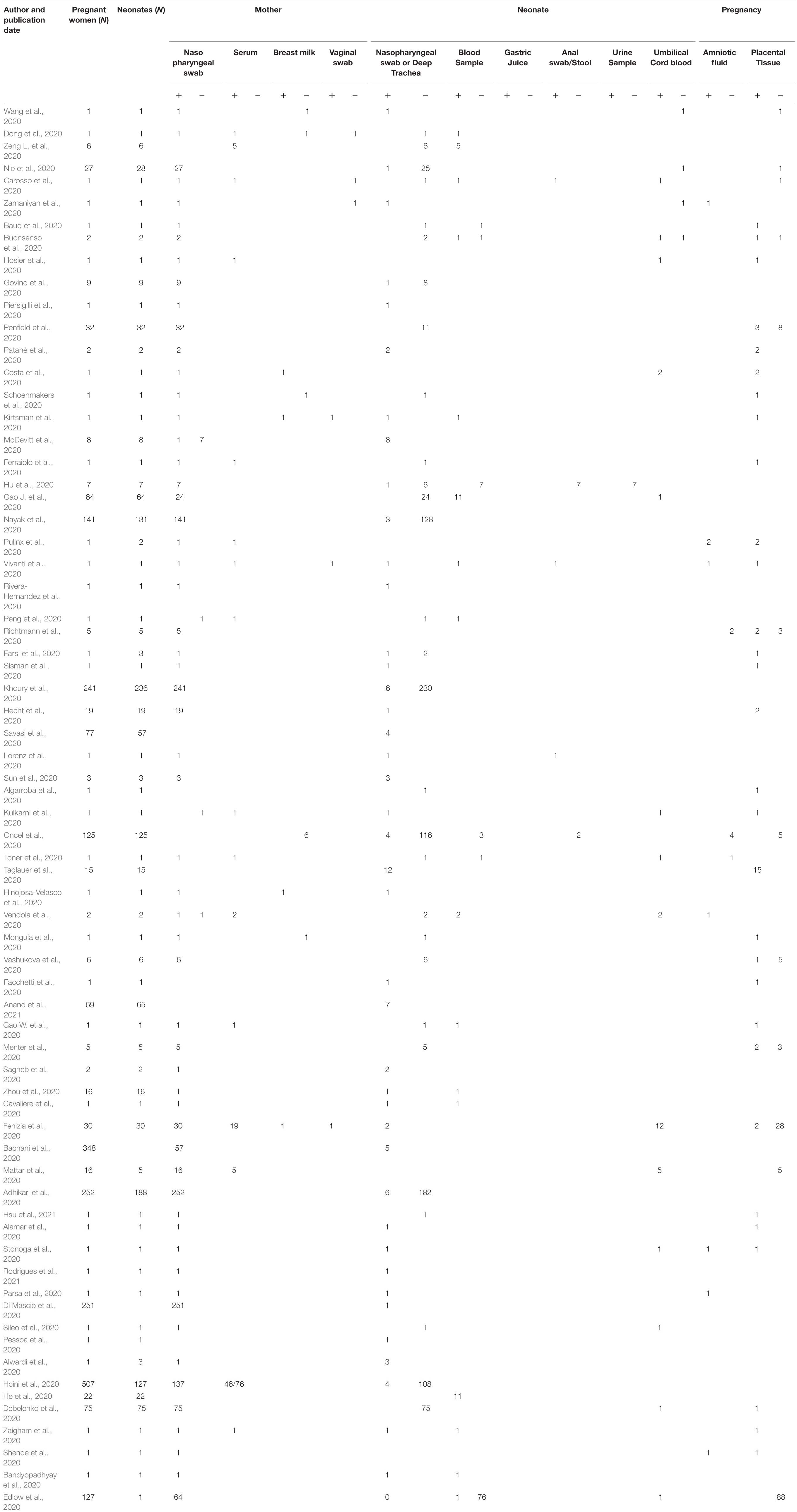
Table 1. List of publications providing information on laboratory tests of mothers and neonates who tested positive with COVID-19.
As expected, SARS-CoV-2 RT-PCR-positive diagnosed mothers with IgG antibodies against SARS-CoV-2 transfer these to the fetus (Gao J. et al., 2020). However, there have also been some reports where IgM were detected in the fetus. These include case reports and small studies from China [2 preterm (Dong et al., 2020; Wu et al., 2020d), 1 term (Zhou et al., 2020), 2 term, 10 term (Gao J. et al., 2020), 2 preterm-1 term (He et al., 2020)], the United States [one term (Edlow et al., 2020)], Italy [one preterm (Fenizia et al., 2020), one term (Cavaliere et al., 2020)], and Sweden (three term Herlenius et al. at Karolinska University Hospital, Stockholm, in progress) that also exhibited IgG against SARS-CoV-2 nucleocapsid and S-protein antibodies 0–2 days after birth.
SARS-CoV-2 Compromised Immunity
Because of the unprecedented participation of volunteers, supported by huge private and governmental investments, both phase III clinical trials of the vaccines developed by BioNTech/Pfizer and Moderna concluded in November 2020. At the time of writing, Pfizer’s vaccine was approved for emergency use in the European Union, United Kingdom, Canada, and the United States, whereas Moderna’s vaccine was also approved for use in the United States. However, pregnant women were excluded from the vaccine clinical trials; thus, the vaccines were not authorized for use during pregnancy.
Vaccination plans take into account several factors, among which are age and comorbidities. Not only progression in COVID-19 patients but also percentages of asymptomatic prevalence vary considerably. A proof-of-principle example of this comes from a study from Stockholm, Sweden, where among patients presenting in labor at Karolinska University Hospital from March 25 to July 24, 2020, 65% of those diagnosed as RT-PCR-positive were asymptomatic (Ahlberg et al., 2020); a similar small study between February and March 2020 in Stockholm showed that 31% of the children reporting no symptoms were seropositive (Herlenius et al., 2020).
However, the highest COVID-19-related morbidity is observed in the group of old-age patients with co-morbidities. Because of their age and medical history, both the immune and the tissue regenerative capacity of these patients are compromised, resulting in this subgroup being affected the most by coronavirus.
Old-aged and other critically ill patients suffer the effects of the aberrant systemic inflammatory response known as the cytokine release syndrome, or the infamous “COVID-19 cytokine storm.” This cytokine storm clinically presents with a sharp rise of cytokines within a short time and may, among others, cause lung injury, which in turn may progress into acute lung injury or its more severe form, acute respiratory distress syndrome (ARDS). The exact pathology and the mechanism of lung injury proceeding to respiratory failure and ARDS in COVID-19 patients are not yet fully understood; yet, it leads to low oxygen saturation levels. Overproduction of proinflammatory cytokines is one of the parameters and a major cause of mortality in COVID-19 (Chen N. et al., 2020; Huang et al., 2020; Lai et al., 2020).
Coronavirus disease 2019 disease progression presents with distinct symptoms, suggesting diversified host immune responses, with one of the villains being the dysfunctional interferon system. A recent study showed that excessive inflammatory response in severe and critical patients was associated with persistent viremia associated with the expression of the nuclear transcription factor nuclear factor-κB; patients presented with type I interferon deficiency, increased production, and altered signaling of tumor necrosis factor-α and interleukin 6 (IL-6; Hadjadj et al., 2020). Such data suggest that combined therapeutic approaches, which could alleviate severe COVID-19 and hasten recovery of the patients who are critically ill, are vital.
One of the main challenges when treating COVID-19 is that the documented exacerbated anti-SARS-CoV-2 immune response can cause severe disease if it remains uncontrolled. The cytokine storm is this inflammatory cascade associated with this exaggerated response of innate immunity, which might cause a late or ineffective response of adaptive immunity (Ragab et al., 2020). It seems to lead to dysfunction of the microcirculation that becomes the villain of SARS-CoV-2 infection (Colantuoni et al., 2020). Thus, a number of therapies aim to target the immune system, but dexamethasone is so far the only drug that can ameliorate disease outcome also in intubated patients (RECOVERY Collaborative Group et al., 2020). Dexamethasone has a long half-life, acts on glucocorticoid receptors, and reduces inflammation through a broad-pathway approach that has been associated, among others, with immunosuppression, hospital-acquired infections, and neuromuscular weakness, even with short courses. Monoclonal antibody therapies of Eli Lilly and Regeneron have also been granted emergency use approval in the United States, for use in mild and moderate cases. IL-6 antagonists have not yet been proven successful (Scherger et al., 2020).
The attenuated deficient immune system is the major risk factor in COVID-19. However, pregnant women also have an attenuated immune system, due to the fetal allograft, but they do not display increased vulnerability under COVID-19. But it might be that there is, currently explored, one immunomodulatory therapeutic approach that might hold great promise toward answering this question. That is advanced mesenchymal cell therapies, i.e., the application of stem cells to treat patients with COVID-19 (Saldanha-Araujo et al., 2020).
Mesenchymal Stem Cells
Exogenous mesenchymal stem cells (MSCs) have been used for decades to treat other diseases caused by viruses. Examples include the acute lung injury caused by influenza virus the immunological compromises caused by the human immunodeficiency virus and the chronic hepatitis caused by hepatitis B virus (Thanunchai et al., 2015). MSCs are a type of highly proliferative adult stem cells with multilineage differentiation capacity. Initially isolated from the bone marrow, they subsequently identified in other tissues, such as the dental pulp, umbilical cord and placenta, adipose tissue and even periosteum and skeletal muscle. They can be found in various autologous and allogenic sources.
Several lines of evidence indicate that one of the main therapeutic mechanisms of MSC administration is via immunomodulation. MSC-mediated immunomodulation operates through a cohort of cell contact-dependent mechanisms including gap junctions and soluble factors (Wu et al., 2017; de Witte et al., 2018; Naji et al., 2019). The main advantage of the use of MSCs for clinical research lies with their hypoimmunogenicity, and they are thus known as “immune-privileged cells.” They do not express human leukocyte antigen (HLA) class II molecules or costimulatory molecules such as CD40, CD40L, CD80, and CD86 and express low levels of HLA class I molecules. These characteristics permits the MSCs to escape the cytotoxic effects of lymphocytic T cells, B cells, and natural killer cells (Rasmusson, 2006; Stagg, 2007; Weiss, 2014; Can and Coskun, 2020; Li et al., 2020). Furthermore, they are able to detect injury signals in their microenvironment and signal regeneration (Stappenbeck and Miyoshi, 2009; Le Blanc and Mougiakakos, 2012; Qin and Zhao, 2020).
Not only MSC-secreted cytokine-mediated effects but also apoptotic, metabolically inactivated, or even fragmented MSCs were shown to exert an immunomodulatory effect, but the roles of regulatory T cells and monocytes in the equation remain under investigation (see review Weiss and Dahlke, 2019). The MSCs’ immunomodulatory properties affect proliferation, activation, and function of various immune cells (Harrell et al., 2019) and may thus alter the innate and adoptive immune responses (Li et al., 2016). The underlying cellular and molecular mechanisms of the long-term effects of MSC-mediation are yet not fully clarified, but regenerative and immunomodulatory effects have been observed in various diseases and tissue types (Aguiar et al., 2020; Qin and Zhao, 2020; Sharma et al., 2020; Tao and Chen, 2020). Although studies describe MSCs as short-lived (Eggenhofer et al., 2012), unable to cross the lung capillary network after intravenous infusion (Fischer et al., 2009), there have been preclinical reports that survival of exogenous MSCs could be detected on site up to 4 months after their direct transplantation; however, few of these cells developed the tissue phenotype of the resident cells (Muñoz et al., 2018).
Mesenchymal stem cells may also use a connexin-43-dependent mechanism to aid compromised cells via mitochondrial transfer, increasing their survival (Islam et al., 2012). Older and recent studies have documented that MSC transplantation and transdifferentiation (Dilger et al., 2020) is dependent on gap junctional cell coupling and the intricate interplay and changes in expression levels of connexin family members. Pannexins might also be involved (Swayne et al., 2020) and thus MSCs might modulate the inflammasome via gap junctional dependent mechanisms.
Mesenchymal stem cells can also suppress chronic inflammation and promote tissue regeneration via the secretion of exosomes, which may in turn regulate macrophage polarization (Ti et al., 2015). Their extracellularly secreted vesicles (termed EVs, which includes both exosomes and microvesicles) are exploited as a cell-free therapeutic tool due to their paracrine and/or endocrine effects (Meirelles Lda et al., 2009). Their mode of action involves either binding to extracellular receptors of targeted cells, merging with the membrane and secreting EV contents, or entering the target cell as endocytic vesicles. MSC-derived EV therapies come with further clinical advantages as they pass across small blood capillaries due because of their small size; they have low chances of tumor formation as they are non-proliferative, and they are immune privileged as they are HLA-I and HLA-II negative.
COVID-19 and MSCs
Because of these immunomodulatory properties of MSCs, 66 stem cell therapy clinical protocols against SARS-CoV-2/COVID-19 have been registered, and six trials have now been completed (details are available at www.clinicaltrials.gov). The use of stem cells as possible therapeutic approaches against COVID-19, including not only MSCs but also MSC-derived exosomes, as therapeutic opportunities for COVID-19 has gained momentum (Jacob et al., 2020; Jayaramayya et al., 2020). As mentioned, most intravenously injected MSCs seem to get trapped in the lung. However, their role in the injured lung may be anti-inflammatory and antiviral and aiding in tissue repair utilizing cell-to-cell contacts and without engraftment into the damaged tissue (Galleu et al., 2017; Armitage et al., 2018), But in addition, and more importantly, in vivo MSCs survive in a hypoxic niche where oxygen tensions are oftenless than 10%.
Many of the protocols submitted to treat COVID-19 involve MSCs derived from the umbilical cord and Wharton jelly. It is known that fetal MSCs are more potent in comparison to those derived from adult sources, but there are ethical considerations regarding fetal tissue as a cellular source. However, perinatal tissues are easily accessible for isolation, highly abundant, and not posing any major ethical concerns. More importantly, a number of clinical trials documented no adverse events after the infusion of MSCs; thus, these cells are considered safe for clinical use (Lalu et al., 2012). MSCs from perinatal tissues have stronger immunomodulatory properties than adult bone marrow-derived MSCs (BM-MSCs; Li et al., 2014). Therefore, MSCs isolated from umbilical cord (UC-MSC) and the fetal part of the placenta (PL-MSCs) share many similarities with BM-MSCs. Compared to adult BM-MSCs, UC-MSCs are superior in colony-forming capacity and differentiation potential; PL-MSCs have a lower colony-forming capacity but similar or less differentiation potential (Beeravolu et al., 2017).
Discussion
Mesenchymal stem cell-induced modulation of immune response in COVID-19 patients seems to be a feasible therapeutic treatment. Currently (December 21, 2020), results from seven single case studies and five small studies (7, 9, 12, 16, and 27 patients, respectively) have been published, where MSCs were employed as therapy on COVID-19 patients (Feng et al., 2020; Liang et al., 2020; Meng et al., 2020; Scherger et al., 2020; Sengupta et al., 2020; Shu et al., 2020; Soler Rich et al., 2020; Tao et al., 2020; Yilmaz et al., 2020; Zengin et al., 2020; Zhang et al., 2020). The phase I clinical trial with the 27 patients (Wu et al., 2020c) with COVID-19 followed the first case study (Wu et al., 2020a) of infusion of MSC-like cells [called human embryonic stem cell-derived immunity- and matrix-regulatory cells (Wu et al., 2020b)] that show higher immunomodulatory potential than standard adult MSCs. These results, although from few patients, suggest that intravenous infusion of MSCs in COVID-19 patients does not cause severe side effects. In addition, MSC transplantation or MSC-derived exosome transplantation is associated with reduced inflammation, also in critically and severely ill COVID-19 patients (n = 70). Interestingly, a case study showed that MSC transplantation, also ameliorated and treated the central nervous system infection, and the authors propose simultaneous systemic and intrathecal administration, as MSCs can thus cross the blood–brain barrier (Yilmaz et al., 2020). Moreover, the outcome after stem cell administration included improvement in lung function. Therefore, transplantation of MSCs seems to attenuate the inflammatory response and possibly promote tissue repair and regeneration, leading to improved outcome of COVID-19 patients (Leng et al., 2020). It seems that due to the MSC-induced immunosuppression, several proinflammatory cytokines and chemokines were reduced in the serum, including IL-6 and C-reactive protein (CRP), while lymphocyte count returned to normal levels faster (Shu et al., 2020). We hypothesize that the anti-inflammatory MSC effect attenuated the recruitment of both macrophages and mononuclear cells to the inflamed lung tissue, further inducing more regulatory dendritic cells; these combined with increased IL-10 and vascular endothelial growth factor might have promoted lung repair. The effect of secreted exosomes has also been documented on antigen presentation functions, differentiation, and maturation of dendritic cells, neutrophils, and other immune cells (for details see recent reviews Abraham and Krasnodembskaya, 2020; Li et al., 2020).
These results suggest that the MSCs may help to reverse the outcome of the COVID-19 cytokine storm. Therefore, we hypothesize that the circulating fetal stem cells might have an immune-protective effect to mothers and contribute to the mild and even asymptomatic COVID-19 pregnancies.
The mechanisms of action, the life span, and the effect of circulating stem cells have been under investigation for decades. Several reports documented the presence of large numbers of fetal cells in healthy or wounded tissue (Bianchi et al., 1996; Khosrotehrani et al., 2004; Nguyen Huu et al., 2007; Zeng et al., 2010). One such study demonstrated that years after the pregnancy, presumably fetal male cells were located in pathological lung and thymus surgical specimens, and the authors speculated they were either recruited from bone marrow or had been proliferated locally (O’Donoghue et al., 2008). Moreover, the results of a gene expression analysis of fetal cells located in the murine maternal lung during pregnancy support this view (Pritchard et al., 2012). These findings back our hypothesis that the presence of placental and fetal MSCs may have an effect on prepartum and post partum maternal health.
Coronavirus disease 2019 pregnancies come forth as a paradigm to be further and more comprehensively investigated. This to understand both the mechanism and action of circulating stem cells in immune modulation and protection as well as their role in hypoxia. Non-invasive methods to monitor human pregnancies and animal models would help answer crucial aspects of both the COVID-19 disease and a possible and feasible therapeutic approaches and non-invasive treatment. When and how early fetal circulating stem cells are recruited to the lungs and what factors signal cell migration toward the maternal circulation might be answered with such studies. Moreover, this type of approaches might reveal the onset of an immunoprotective cell migration as a response to placental vascular malperfusions because of increased uterine and intervillous blood flow. Moreover, the cell migration mechanisms themselves could provide hints on blood–brain approachability for future studies and therapies.
Administration of MSCs in COVID-19 patients seems to be a promising tool in the treatment by reducing hyperinflammation (Gentile et al., 2020). MSCs from COVID-19 patients of different disease severity should be isolated and analyzed. Adipose tissue-derived stem cells (ADSCs), and their exosomes, are considered superior regarding non-invasiveness, accessibility, and abundance when compared to other sources (Jin et al., 2013). They can also be maintained and expanded in culture for long without losing their differentiation capacity, expanded thus to the large quantities needed for cell therapy purposes. ADSCs maintain their differentiation potential to differentiate, and they have low immunogenicity combined with modulatory effects, with less than 1% of them expressing human leukocyte antigen – DR isotype (HLA-DR), rendering them suitable for clinical allogeneic transplantation (Puissant et al., 2005; Strem et al., 2005; Dominici et al., 2006; McIntosh, 2011).
The route of administration and MSC preconditioning with cytokines or hypoxia should be further explored and fine-tuned. Both the COVID-19 pregnancies and the outcome of the clinical trials that investigate the possible therapeutic role of MSC transplantation will provide solid evidence of how MSCs influence the indicators of proinflammatory cytokines and further elucidate the impairment of the interferon pathway in COVID-19 patients.
Thus, studying the effect of maternal and fetal MSCS in COVID-19 pregnancies may act as a proof-of-principle approach, pave the understanding of the role of fetal circulating stem cells in the mother–fetus dyad, and elucidate the use of MSC infusion for therapy beyond COVID-19.
Data Availability Statement
The original contributions presented in the study are included in the article/supplementary material, further inquiries can be directed to the corresponding author.
Author Contributions
AS conceptualized the study, wrote first draft, and revised the manuscript. EH revised the manuscript. Both authors contributed to the article and approved the submitted version.
Funding
This study was supported by the Swedish Research Council, the Stockholm County Council (ALF projects 2019-0400 and 2019-0974), the Karolinska Institutet, and VINNOVA, and grants from the Swedish Brain (2019-0087) and Swedish National Heart and Lung (2018-0212) Foundations. The funders of the study played no role in the study design, data collection, data analysis, data interpretation, or writing of the report.
Conflict of Interest
The authors declare that the research was conducted in the absence of any commercial or financial relationships that could be construed as a potential conflict of interest.
References
Abraham, A., and Krasnodembskaya, A. (2020). Mesenchymal stem cell-derived extracellular vesicles for the treatment of acute respiratory distress syndrome. Stem Cells Transl. Med. 9, 28–38. doi: 10.1002/sctm.19-0205
Adhikari, E. H., Moreno, W., Zofkie, A. C., MacDonald, L., McIntire, D. D., Collins, R. R. J., et al. (2020). Pregnancy outcomes among women with and without severe acute respiratory syndrome coronavirus 2 infection. JAMA Netw. Open 3:e2029256. doi: 10.1001/jamanetworkopen.2020.29256
Aguiar, F. S., Melo, A. S., Araújo, A. M. S., Cardoso, A. P., de Souza, S. A. L., Lopes-Pacheco, M., et al. (2020). Autologous bone marrow-derived mononuclear cell therapy in three patients with severe asthma. Stem Cell Res. Ther. 11:167. doi: 10.1186/s13287-020-01675-x
Ahlberg, M., Neovius, M., Saltvedt, S., Söderling, J., Pettersson, K., Brandkvist, C., et al. (2020). Association of SARS-CoV-2 test status and pregnancy outcomes. JAMA 324, 1782–1785. doi: 10.1001/jama.2020.19124
Alamar, I., Abu-Arja, M. H., Heyman, T., Roberts, D. J., Desai, N., Narula, P., et al. (2020). A possible case of vertical transmission of severe acute respiratory syndrome coronavirus 2 (SARS-CoV-2) in a newborn with positive placental in situ hybridization of SARS-CoV-2 RNA. J. Pediatric. Infect. Dis. Soc. 9, 636–639. doi: 10.1093/jpids/piaa109
Algarroba, G. N., Hanna, N. N., Rekawek, P., Vahanian, S. A., Khullar, P., Palaia, T., et al. (2020). Confirmatory evidence of the visualization of severe acute respiratory syndrome coronavirus 2 invading the human placenta using electron microscopy. Am. J. Obstet. Gynecol. 223, 953–954. doi: 10.1016/j.ajog.2020.08.106
Alwardi, T. H., Ramdas, V., Al Yahmadi, M., Al Aisari, S., Bhandari, S., Saif Al Hashami, H., et al. (2020). Is vertical transmission of SARS-CoV-2 infection possible in preterm triplet pregnancy? A case series. Pediatr. Infect. Dis. J. 39, e456–e458. doi: 10.1097/INF.0000000000002926
Alzamora, M. C., Paredes, T., Caceres, D., Webb, C. M., Valdez, L. M., and La Rosa, M. (2020). Severe COVID-19 during pregnancy and possible vertical transmission. Am. J. Perinatol. 37, 861–865. doi: 10.1055/s-0040-1710050
Amaral, W. N. D., Moraes, C. L., Rodrigues, A. P. D. S., Noll, M., Arruda, J. T., and Mendonça, C. R. (2020). Maternal coronavirus infections and neonates born to mothers with SARS-CoV-2: a systematic review. Healthcare 8:511. doi: 10.3390/healthcare8040511
Anand, P., Yadav, A., Debata, P., Bachani, S., Gupta, N., and Gera, R. (2021). Clinical profile, viral load, management and outcome of neonates born to COVID 19 positive mothers: a tertiary care centre experience from India. Eur. J. Pediatr. 180, 547–559. doi: 10.1007/s00431-020-03800-7
Armitage, J., Tan, D. B. A., Troedson, R., Young, P., Lam, K. V., Shaw, K., et al. (2018). Mesenchymal stromal cell infusion modulates systemic immunological responses in stable COPD patients: a phase I pilot study. Eur. Respir. J. 51:1702369. doi: 10.1183/13993003.02369-2017
Ashraf, M. A., Keshavarz, P., Hosseinpour, P., Erfani, A., Roshanshad, A., Pourdast, A., et al. (2020). Coronavirus disease 2019 (COVID-19): a systematic review of pregnancy and the possibility of vertical transmission. J. Reprod. Infertil. 21, 157–168.
Bachani, S., Arora, R., Dabral, A., Marwah, S., Anand, P., Reddy, K. S., et al. (2020). Clinical profile, viral load, maternal-fetal outcomes of pregnancy with COVID-19: 4-week retrospective, tertiary care single-centre descriptive study. J. Obstet. Gynaecol. Can. doi: 10.1016/j.jogc.2020.09.021 Online ahead of print
Bandyopadhyay, T., Sharma, A., Kumari, P., Maria, A., and Choudhary, R. (2020). Possible early vertical transmission of COVID-19 from an infected pregnant female to her neonate: a case report. J. Trop. Pediatr. doi: 10.1093/tropej/fmaa094 Online ahead of print
Baud, D., Greub, G., Favre, G., Gengler, C., Jaton, K., Dubruc, E., et al. (2020). Second-trimester miscarriage in a pregnant woman with SARS-CoV-2 infection. JAMA 323, 2198–2200. doi: 10.1001/jama.2020.7233
Beeravolu, N., McKee, C., Alamri, A., Mikhael, S., Brown, C., Perez-Cruet, M., et al. (2017). Isolation and characterization of mesenchymal stromal cells from human umbilical cord and fetal placenta. J. Vis. Exp. 3:55224. doi: 10.3791/55224
Bianchi, D. W., Zickwolf, G. K., Weil, G. J., Sylvester, S., and DeMaria, M. A. (1996). Male fetal progenitor cells persist in maternal blood for as long as 27 years postpartum. Proc. Natl. Acad. Sci. U.S.A. 93, 705–708. doi: 10.1073/pnas.93.2.705
Breslin, N., Baptiste, C., Miller, R., Fuchs, K., Goffman, D., Gyamfi-Bannerman, C., et al. (2020). Coronavirus disease 2019 in pregnancy: early lessons. Am. J. Obstet. Gynecol. MFM. 2:100111. doi: 10.1016/j.ajogmf.2020.100111
Buonsenso, D., Costa, S., Sanguinetti, M., Cattani, P., Posteraro, B., Marchetti, S., et al. (2020). Neonatal Late onset infection with severe acute respiratory syndrome coronavirus 2. Am. J. Perinatol. 37, 869–872. doi: 10.1055/s-0040-1710541
Can, A., and Coskun, H. (2020). The rationale of using mesenchymal stem cells in patients with COVID-19-related acute respiratory distress syndrome: what to expect. Stem Cells Transl Med. 9, 1287–1302. doi: 10.1002/sctm.20-0164
Carosso, A., Cosma, S., Borella, F., Marozio, L., Coscia, A., Ghisetti, V., et al. (2020). Pre-labor anorectal swab for SARS-CoV-2 in COVID-19 pregnant patients: is it time to think about it? Eur. J. Obstet. Gynecol. Reprod. Biol. 249, 98–99. doi: 10.1016/j.ejogrb.2020.04.023
Cavaliere, A. F., Marchi, L., Aquilini, D., Brunelli, T., and Vasarri, P. L. (2020). Passive immunity in newborn from SARS-CoV-2-infected mother. J. Med. Virol. doi: 10.1002/jmv.26609 Online ahead of print
Chen, H., Guo, J., Wang, C., Luo, F., Yu, X., Zhang, W., et al. (2020). Clinical characteristics and intrauterine vertical transmission potential of COVID-19 infection in nine pregnant women: a retrospective review of medical records. Lancet 395, 809–815. doi: 10.1016/S0140-6736(20)30360-3
Chen, N., Zhou, M., Dong, X., Qu, J., Gong, F., Han, Y., et al. (2020). Epidemiological and clinical characteristics of 99 cases of 2019 novel coronavirus pneumonia in Wuhan, China: a descriptive study. Lancet 395, 507–513. doi: 10.1016/S0140-6736(20)30211-7
Colantuoni, A., Martini, R., Caprari, P., Ballestri, M., Capecchi, P. L., Gnasso, A., et al. (2020). COVID-19 sepsis and microcirculation dysfunction. Front. Physiol. 11:747. doi: 10.3389/fphys.2020.00747
Costa, S., Posteraro, B., Marchetti, S., Tamburrini, E., Carducci, B., Lanzone, A., et al. (2020). Excretion of SARS-CoV-2 in human breast milk. Clin. Microbiol. Infect. 26, 1430–1432. doi: 10.1016/j.cmi.2020.05.027
COVID-19 Clinical Research Coalition (2021). Available online at: https://www.pericovid.com/ (accessed January 31, 2021).
Dashraath, P., Wong, J. L. J., Lim, M. X. K., Lim, L. M., Li, S., Biswas, A., et al. (2020). Coronavirus disease 2019 (COVID-19) pandemic and pregnancy. Am. J. Obstet. Gynecol. 222, 521–531. doi: 10.1016/j.ajog.2020.03.021
de Witte, S. F. H., Luk, F., Sierra Parraga, J. M., Gargesha, M., Merino, A., Korevaar, S. S., et al. (2018). Immunomodulation by therapeutic mesenchymal stromal cells (MSC) is triggered through phagocytosis of MSC By monocytic cells. Stem Cells 36, 602–615. doi: 10.1002/stem.2779
Debelenko, L., Katsyv, I., Chong, A. M., Peruyero, L., Szabolcs, M., and Uhlemann, A. C. (2020). Trophoblast damage with acute and chronic intervillositis: disruption of the placental barrier by severe acute respiratory syndrome coronavirus 2. Hum Pathol. 109, 69–79. doi: 10.1016/j.humpath.2020.12.004
Di Mascio, D., Sen, C., Saccone, G., Galindo, A., Grünebaum, A., Yoshimatsu, J., et al. (2020). Risk factors associated with adverse fetal outcomes in pregnancies affected by Coronavirus disease 2019 (COVID-19): a secondary analysis of the WAPM study on COVID-19. J. Perinat. Med. 48, 950–958. doi: 10.1515/jpm-2020-0355
Dilger, N., Neehus, A. L., Grieger, K., Hoffmann, A., Menssen, M., and Ngezahayo, A. (2020). Gap junction dependent cell communication is modulated during transdifferentiation of mesenchymal stem/stromal cells towards neuron-like cells. Front. Cell Dev. Biol. 8:869. doi: 10.3389/fcell.2020.00869
Dominici, M., Le Blanc, K., Mueller, I., Slaper-Cortenbach, I., Marini, F., Krause, D., et al. (2006). Minimal criteria for defining multipotent mesenchymal stromal cells. The international society for cellular therapy position statement. Cytotherapy 8, 315–317. doi: 10.1080/14653240600855905
Dong, L., Tian, J., He, S., Zhu, C., Wang, J., Liu, C., et al. (2020). Possible vertical transmission of SARS-CoV-2 from an infected mother to her newborn. JAMA 323, 1846–1848. doi: 10.1001/jama.2020.4621
Dumitriu, D., Emeruwa, U. N., Hanft, E., Liao, G. V., Ludwig, E., Walzer, L., et al. (2020). Outcomes of neonates born to mothers with severe acute respiratory syndrome coronavirus 2 infection at a large medical center in New York City. JAMA Pediatr. 12:e204298. doi: 10.1001/jamapediatrics.2020.4298
Edlow, A. G., Li, J. Z., Collier, A. Y., Atyeo, C., James, K. E., Boatin, A. A., et al. (2020). Assessment of Maternal and neonatal SARS-CoV-2 viral load, transplacental antibody transfer, and placental pathology in pregnancies during the COVID-19 pandemic. JAMA Netw. Open 3:e2030455. doi: 10.1001/jamanetworkopen.2020.30455
Eggenhofer, E., Benseler, V., Kroemer, A., Popp, F. C., Geissler, E. K., Schlitt, H. J., et al. (2012). Mesenchymal stem cells are short-lived and do not migrate beyond the lungs after intravenous infusion. Front. Immunol. 3:297. doi: 10.3389/fimmu.2012.00297
Facchetti, F., Bugatti, M., Drera, E., Tripodo, C., Sartori, E., Cancila, V., et al. (2020). SARS-CoV2 vertical transmission with adverse effects on the newborn revealed through integrated immunohistochemical, electron microscopy and molecular analyses of Placenta. EBioMedicine 59:102951. doi: 10.1016/j.ebiom.2020.102951
Farsi, Z., Derakhsh, N. T., Bassirnia, M., Ahmadi, L., Shiva, M., and Yousefzadegan, S. (2020). Coronavirus disease-2019 infection in neonates of an infected pregnant mother with triplets. Iran. J. Neonatol. 11, 120–122. doi: 10.22038/ijn.2020.49218.1856
Feng, Y., Huang, J., Wu, J., Xu, Y., Chen, B., Jiang, L., et al. (2020). Safety and feasibility of umbilical cord mesenchymal stem cells in patients with COVID-19 pneumonia: a pilot study. Cell Prolif. 53:e12947. doi: 10.1111/cpr.12947
Fenizia, C., Biasin, M., Cetin, I., Vergani, P., Mileto, D., Spinillo, A., et al. (2020). Analysis of SARS-CoV-2 vertical transmission during pregnancy. Nat. Commun. 11:5128. doi: 10.1038/s41467-020-18933-4
Ferraiolo, A., Barra, F., Kratochwila, C., Paudice, M., Vellone, V. G., Godano, E., et al. (2020). Report of positive placental swabs for SARS-CoV-2 in an asymptomatic pregnant woman with COVID-19. Medicina 56:306. doi: 10.3390/medicina56060306
Ferrazzi, E., Beretta, P., Bianchi, S., Cetin, I., Guarnerio, P., Locatelli, A., et al. (2020). SARS-CoV-2 infection testing at delivery: a clinical and epidemiological priority. J. Matern. Fetal Neonatal Med. 13, 1–3. doi: 10.1080/14767058.2020.1788532
Fischer, U. M., Harting, M. T., Jimenez, F., Monzon-Posadas, W. O., Xue, H., Savitz, S. I., et al. (2009). Pulmonary passage is a major obstacle for intravenous stem cell delivery: the pulmonary first-pass effect. Stem Cells Dev. 18, 683–692. doi: 10.1089/scd.2008.0253
Friedman, D. M., Kim, M., Costedoat-Chalumeau, N., Clancy, R., Copel, J., Phoon, C. K., et al. (2020). Electrocardiographic QT intervals in infants exposed to hydroxychloroquine throughout gestation. Circ. Arrhythm. Electrophysiol. 13:e008686. doi: 10.1161/CIRCEP.120.008686
Gale, C., Quigley, M. A., Placzek, A., Knight, M., Ladhani, S., Draper, E. S., et al. (2020). Characteristics and outcomes of neonatal SARS-CoV-2 infection in the UK: a prospective national cohort study using active surveillance. Lancet Child Adolesc. Health doi: 10.1016/S2352-4642(20)30342-4 Online ahead of print
Galleu, A., Riffo-Vasquez, Y., Trento, C., Lomas, C., Dolcetti, L., Cheung, T. S., von Bonin, M., et al. (2017). Apoptosis in mesenchymal stromal cells induces in vivo recipient-mediated immunomodulation. Sci. Transl. Med. 9:eaam7828. doi: 10.1126/scitranslmed.aam7828
Gao, J., Li, W., Hu, X., Wei, Y., Wu, J., Luo, X., et al. (2020). Disappearance of SARS-CoV-2 antibodies in infants born to women with COVID-19, Wuhan, China. Emerg. Infect. Dis. 26, 2491–2494. doi: 10.3201/eid2610.202328
Gao, W., Deng, Z., Zeng, L., Yang, Y., Gong, H., Liu, J., et al. (2020). A newborn with normal IgM and elevated IgG antibodies born to an asymptomatic infection mother with COVID-19. Aging 12, 16672–16674. doi: 10.18632/aging.103346
Gentile, P., Sterodimas, A., Pizzicannella, J., Calabrese, C., and Garcovich, S. (2020). Research progress on mesenchymal stem cells (MSCs), adipose-derived mesenchymal stem cells (AD-MSCs), drugs, and vaccines in inhibiting COVID-19 disease. Aging Dis. 11, 1191–1201. doi: 10.14336/AD.2020.0711
Govind, A., Essien, S., Karthikeyan, A., Fakokunde, A., Janga, D., Yoong, W., et al. (2020). Re: novel coronavirus COVID-19 in late pregnancy: outcomes of first nine cases in an inner city London hospital. Eur. J. Obstet. Gynecol. Reprod. Biol. 251, 272–274. doi: 10.1016/j.ejogrb.2020.05.004
Gregorio-Hernández, R., Escobar-Izquierdo, A. B., Cobas-Pazos, J., and Martínez-Gimeno, A. (2020). Point-of-care lung ultrasound in three neonates with COVID-19. Eur. J. Pediatr. 179, 1279–1285. doi: 10.1007/s00431-020-03706-4
Gupta, A., Madhavan, M. V., Sehgal, K., Nair, N., Mahajan, S., Sehrawat, T. S., et al. (2020). Extrapulmonary manifestations of COVID-19. Nat. Med. 26, 1017–1032. doi: 10.1038/s41591-020-0968-3
Hadjadj, J., Yatim, N., Barnabei, L., Corneau, A., Boussier, J., Smith, N., et al. (2020). Impaired type I interferon activity and inflammatory responses in severe COVID-19 patients. Science 369, 718–724. doi: 10.1126/science.abc6027
Harrell, C. R., Sadikot, R., Pascual, J., Fellabaum, C., Jankovic, M. G., Jovicic, N., et al. (2019). Mesenchymal stem cell-based therapy of inflammatory lung diseases: current understanding and future perspectives. Stem Cells Int. 2019:4236973. doi: 10.1155/2019/4236973
Hcini, N., Maamri, F., Picone, O., Carod, J. F., Lambert, V., Mathieu, M., et al. (2020). Maternal, fetal and neonatal outcomes of large series of SARS-CoV-2 positive pregnancies in peripartum period: a single-center prospective comparative study. Eur J Obstet Gynecol Reprod Biol. 257, 11–18. doi: 10.1016/j.ejogrb.2020.11.068
He, Z., Fang, Y., Zuo, Q., Huang, X., Lei, Y., Ren, X., et al. (2020). Vertical transmission and kidney damage in newborns whose mothers had coronavirus disease 2019 during pregnancy. Int. J. Antimicrob. Agents 9:106260. doi: 10.1016/j.ijantimicag.2020.106260
Hecht, J. L., Quade, B., Deshpande, V., Mino-Kenudson, M., Ting, D. T., Desai, N., et al. (2020). SARS-CoV-2 can infect the placenta and is not associated with specific placental histopathology: a series of 19 placentas from COVID-19-positive mothers. Mod. Pathol. 33, 2092–2103. doi: 10.1038/s41379-020-0639-4
Herlenius, E., Åkerstedt, S. O., Herrting, O., Ottosson, L., Ramadan, L., and Hildenwall, H. (2020). SARS-CoV-2 Exposure, Symptoms and Antibody Response in Children in Stockholm during the COVID-19 pandemic. Abstract Presented at “State of the Art Covid-19” on 15-16 December. Available online at: https://www.sls.se/state-of-the-art-covid-19/abstracts/Abstract-book/ (accessed October 30, 2020).
Hinojosa-Velasco, A., de Oca, P. V. B., García-Sosa, L. E., Mendoza-Durán, J. G., Pérez-Méndez, M. J., Dávila-González, E., et al. (2020). A case report of newborn infant with severe COVID-19 in Mexico: detection of SARS-CoV-2 in human breast milk and stool. Int. J. Infect. Dis. 100, 21–24. doi: 10.1016/j.ijid.2020.08.055
Hosier, H., Farhadian, S., Morotti, R., Deshmukh, U., Lu-Culligan, A., Campbell, K. H., et al. (2020). First case of placental infection with SARS-CoV- 2. medRxiv [Preprint] doi: 10.1101/2020.04.30.20083907
Hsu, A. L., Guan, M., Johannesen, E., Stephens, A. J., Khaleel, N., Kagan, N., et al. (2021). Placental SARS-CoV-2 in a pregnant woman with mild COVID-19 disease. J. Med. Virol. 93, 1038–1044. doi: 10.1002/jmv.26386
Hu, X., Gao, J., Luo, X., Feng, L., Liu, W., Chen, J., et al. (2020). Severe acute respiratory syndrome coronavirus 2 (SARS-CoV-2) vertical transmission in neonates born to mothers with coronavirus disease 2019 (COVID-19) pneumonia. Obstet. Gynecol. 136, 65–67. doi: 10.1097/AOG.0000000000003926
Huang, C., Wang, Y., Li, X., Ren, L., Zhao, J., Hu, Y., et al. (2020). Clinical features of patients infected with 2019 novel coronavirus in Wuhan, China. Lancet 395, 497–506. doi: 10.1016/S0140-6736(20)30183-5
Iqbal, S. N., Overcash, R., Mokhtari, N., Saeed, H., Gold, S., Auguste, T., et al. (2020). An Uncomplicated delivery in a patient with Covid-19 in the United States. N. Engl. J. Med. 382:e34. doi: 10.1056/NEJMc2007605
Islam, M. N., Das, S. R., Emin, M. T., Wei, M., Sun, L., Westphalen, K., et al. (2012). Mitochondrial transfer from bone-marrow-derived stromal cells to pulmonary alveoli protects against acute lung injury. Nat. Med. 18, 759–765. doi: 10.1038/nm.2736
Jacob, F., Pather, S. R., Huang, W. K., Zhang, F., Wong, S. Z. H., Zhou, H. et al. (2020). Human pluripotent stem cell-derived neural cells and brain organoids reveal SARS-CoV-2 neurotropism predominates in choroid plexus epithelium. Cell Stem Cell. 27:937.e9–950.e9. doi: 10.1016/j.stem.2020.09.016
Jamieson, D. J., Theiler, R. N., and Rasmussen, S. A. (2006). Emerging infections and pregnancy. Emerg. Infect. Dis. 12, 1638–1643. doi: 10.3201/eid1211.060152
Jayaramayya, K., Mahalaxmi, I., Subramaniam, M. D., Raj, N., Dayem, A. A., Lim, K.M. et al. (2020). Immunomodulatory effect of mesenchymal stem cells and mesenchymal stem-cell-derived exosomes for COVID-19 treatment. BMB Rep. 53, 400–412. doi: 10.5483/BMBRep.2020.53.8.121
Jin, H. J., Bae, Y. K., Kim, M., Kwon, S. J., Jeon, H. B., Choi, S. J., et al. (2013). Comparative analysis of human mesenchymal stem cells from bone marrow, adipose tissue, and umbilical cord blood as sources of cell therapy. Int. J. Mol. Sci. 14, 17986–18001. doi: 10.3390/ijms140917986
Khosrotehrani, K., Johnson, K. L., Cha, D. H., Salomon, R. N., and Bianchi, D. W. (2004). Transfer of fetal cells with multilineage potential to maternal tissue. JAMA. 292, 75–80. doi: 10.1001/jama.292.1.75
Khoury, R., Bernstein, P. S., Debolt, C., Stone, J., Sutton, D. M., Simpson, L. L., et al. (2020). Characteristics and outcomes of 241 births to women with severe acute respiratory syndrome coronavirus 2 (SARS-CoV-2) infection at five New York city medical centers. Obstet. Gynecol. 136, 273–282. doi: 10.1097/AOG.0000000000004025
Kirtsman, M., Diambomba, Y., Poutanen, S. M., Malinowski, A. K., Vlachodimitropoulou, E., Parks, W. T., et al. (2020). Probable congenital SARS-CoV-2 infection in a neonate born to a woman with active SARS-CoV-2 infection. CMAJ 192, E647–E650. doi: 10.1503/cmaj.200821
Kulkarni, R., Rajput, U., Dawre, R., Valvi, C., Nagpal, R., Magdum, N., et al. (2020). Early-onset symptomatic neonatal COVID-19 infection with high probability of vertical transmission. Infection doi: 10.1007/s15010-020-01493-6 Online ahead of print.
Lai, C. C., Shih, T. P., Ko, W. C., Tang, H. J., and Hsueh, P. R. (2020). Severe acute respiratory syndrome coronavirus 2 (SARS-CoV-2) and coronavirus disease-2019 (COVID-19): the epidemic and the challenges. Int. J. Antimicrob. Agents. 55:105924. doi: 10.1016/j.ijantimicag.2020.105924
Lalu, M. M., McIntyre, L., Pugliese, C., Fergusson, D., Winston, B. W., Marshall, J. C., et al. (2012). Safety of cell therapy with mesenchymal stromal cells (SafeCell): a systematic review and meta-analysis of clinical trials. PLoS One 7:e47559. doi: 10.1371/journal.pone.0047559
Lapinsky, S. E., Tram, C., Mehta, S., and Maxwell, C. V. (2014). Restrictive lung disease in pregnancy. Chest 145, 394–398. doi: 10.1378/chest.13-0587
Le Blanc, K., and Mougiakakos, D. (2012). Multipotent mesenchymal stromal cells and the innate immune system. Nat. Rev. Immunol. 12, 383–396. doi: 10.1038/nri3209
Leng, Z., Zhu, R., Hou, W., Feng, Y., Yang, Y., Han, Q., et al. (2020). Transplantation of ACE2– mesenchymal stem cells improves the outcome of patients with COVID-19 pneumonia. Aging Dis. 11, 216–228. doi: 10.14336/AD.2020.0228
Li, X., Bai, J., Ji, X., Li, R., Xuan, Y., and Wang, Y. (2014). Comprehensive characterization of four different populations of human mesenchymal stem cells as regards their immune properties, proliferation and differentiation. Int. J. Mol. Med. 34, 695–704. doi: 10.3892/ijmm.2014.1821
Li, Y., Xu, J., Shi, W., Chen, C., Shao, Y., Zhu, L., et al. (2016). Mesenchymal stromal cell treatment prevents H9N2 avian influenza virus-induced acute lung injury in mice. Stem Cell Res Ther. 7:159. doi: 10.1186/s13287-016-0395-z
Li, Z., Niu, S., Guo, B., Gao, T., Wang, L., Wang, Y., et al. (2020). Stem cell therapy for COVID-19, ARDS and pulmonary fibrosis. Cell Prolif. 53:e12939. doi: 10.1111/cpr.12939
Liang, B., Chen, J., Li, T., Wu, H., Yang, W., Li, Y., et al. (2020). Clinical remission of a critically ill COVID-19 patient treated by human umbilical cord mesenchymal stem cells: a case report. Medicine 99:e21429. doi: 10.1097/MD.0000000000021429
Liu, D., Li, L., Wu, X., Zheng, D., Wang, J., Yang, L., et al. (2020). Pregnancy and perinatal outcomes of women with coronavirus disease (COVID-19) pneumonia: a preliminary analysis. AJR Am. J. Roentgenol. 215, 127–132. doi: 10.2214/AJR.20.23072
Lorenz, N., Treptow, A., Schmidt, S., Hofmann, R., Raumer-Engler, M., Heubner, G., et al. (2020). Neonatal early-onset infection with SARS-CoV-2 in a newborn presenting with encephalitic symptoms. Pediatr. Infect. Dis. J. 39:e212. doi: 10.1097/INF.0000000000002735
Mattar, C. N., Kalimuddin, S., Sadarangani, S. P., Tagore, S., Thain, S., Thoon, K. C., et al. (2020). Pregnancy outcomes in COVID-19: a prospective cohort study in Singapore. Ann. Acad. Med. Singap. 49, 857–869.
McDevitt, K. E. M., Ganjoo, N., Mlangeni, D., and Pathak, S. (2020). Outcome of universal screening of neonates for COVID-19 from asymptomatic mothers. J. Infect. 81, 452–482. doi: 10.1016/j.jinf.2020.06.037
McIntosh, K. R. (2011). Evaluation of cellular and humoral immune responses to allogeneic adipose-derived stem/stromal cells. Methods Mol. Biol. 702, 133–150. doi: 10.1007/978-1-61737-960-4_11
Meirelles Lda, S., Fontes, A. M., Covas, D. T., and Caplan, A. I. (2009). Mechanisms involved in the therapeutic properties of mesenchymal stem cells. Cytokine Growth Factor Rev. 20, 419–427. doi: 10.1016/j.cytogfr.2009.10.002
Meng, F., Xu, R., Wang, S., Xu, Z., Zhang, C., Li, Y., et al. (2020). Human umbilical cord-derived mesenchymal stem cell therapy in patients with COVID-19: a phase 1 clinical trial. Signal Transduct Target Ther. 5:172. doi: 10.1038/s41392-020-00286-5
Menter, T., Mertz, K. D., Jiang, S., Chen, H., Monod, C., Tzankov, A., et al. (2020). Placental pathology findings during and after SARS-CoV-2 infection: features of villitis and malperfusion. Pathobiology 88, 69–77. doi: 10.1159/000511324
Mongula, J. E., Frenken, M. W. E., van Lijnschoten, G., Arents, N. L. A., de Wit-Zuurendonk, L. D., Schimmel-de Kok, A. P. A., et al. (2020). COVID-19 during pregnancy: non-reassuring fetal heart rate, placental pathology and coagulopathy. Ultrasound. Obstet. Gynecol. 56, 773–776. doi: 10.1002/uog.22189
Muñoz, M. F., Argüelles, S., Guzman-Chozas, M., Guillén-Sanz, R., Franco, J. M., Pintor-Toro, J. A., et al. (2018). Cell tracking, survival, and differentiation capacity of adipose-derived stem cells after engraftment in rat tissue. J. Cell Physiol. 233, 6317–6328. doi: 10.1002/jcp.26439
Naji, A., Favier, B., Deschaseaux, F., Rouas-Freiss, N., Eitoku, M., and Suganuma, N. (2019). Mesenchymal stem/stromal cell function in modulating cell death. Stem Cell Res Ther. 10:56. doi: 10.1186/s13287-019-1158-4
Nayak, A. H., Kapote, D. S., Fonseca, M., Chavan, N., Mayekar, R., Sarmalkar, M., et al. (2020). Impact of the coronavirus infection in pregnancy: a preliminary study of 141 patients. J. Obstet. Gynaecol. India 70, 256–261. doi: 10.1007/s13224-020-01335-3
Nguyen Huu, S., Oster, M., Uzan, S., Chareyre, F., Aractingi, S., and Khosrotehrani, K. (2007). Maternal neoangiogenesis during pregnancy partly derives from fetal endothelial progenitor cells. Proc. Natl. Acad. Sci. U.S.A. 104, 1871–1876. doi: 10.1073/pnas.0606490104
Nie, R., Wang, S.-S., Yang, Q., Fan, C.-F., Liu, Y.-L., He, W.-C., et al. (2020). Clinical features and the maternal and neonatal outcomes of pregnant women with coronavirus disease 2019. medRxiv [Preprint] doi: 10.1101/2020.03.22.20041061
O’Donoghue, K., Sultan, H. A., Al-Allaf, F. A., Anderson, J. R., Wyatt-Ashmead, J., and Fisk, N. M. (2008). Microchimeric fetal cells cluster at sites of tissue injury in lung decades after pregnancy. Reprod. Biomed. Online 16, 382–390. doi: 10.1016/s1472-6483(10)60600-1
Oncel, M. Y., Akın, I. M., Kanburoglu, M. K., Tayman, C., Coskun, S., Narter, F., et al. (2020). A multicenter study on epidemiological and clinical characteristics of 125 newborns born to women infected with COVID-19 by Turkish Neonatal Society. Eur. J. Pediatr. 10, 1–10. doi: 10.1007/s00431-020-03767-5
Parsa, Y., Shokri, N., Jahedbozorgan, T., Naeiji, Z., Zadehmodares, S., and Moridi, A. (2020). Possible vertical transmission of COVID-19 to the newborn; a case report. Arch. Acad. Emerg. Med. 9:e5.
Pastick, K. A., Nicol, M. R., Smyth, E., Zash, R., Boulware, D. R., Rajasingham, R., et al. (2020). A systematic review of treatment and outcomes of pregnant women with COVID-19-a call for clinical trials. Open Forum. Infect. Dis. 7:ofaa350. doi: 10.1093/ofid/ofaa350
Patanè, L., Morotti, D., Giunta, M. R., Sigismondi, C., Piccoli, M. G., Frigerio, L., et al. (2020). Vertical transmission of coronavirus disease 2019: severe acute respiratory syndrome coronavirus 2 RNA on the fetal side of the placenta in pregnancies with coronavirus disease 2019-positive mothers and neonates at birth. Am. J. Obstet. Gynecol. MFM. 2:100145. doi: 10.1016/j.ajogmf.2020.100145
Penfield, C. A., Brubaker, S. G., Limaye, M. A., Lighter, J., Ratner, A. J., Thomas, K. M., et al. (2020). Detection of severe acute respiratory syndrome coronavirus 2 in placental and fetal membrane samples. Am. J. Obstet. Gynecol. MFM. 2:100133. doi: 10.1016/j.ajogmf.2020.100133
Peng, J., Li, R., Yin, H., Tang, F., Xie, H., Li, M., et al. (2020). A case report of a pregnant woman infected with coronavirus disease 2019 pneumonia. Medicine 99:e21335. doi: 10.1097/MD.0000000000021335
Pessoa, F. S., Vale, M. S. D., Marques, P. F., Figueira, S. D. S., Salgado, I. A. D. S. C., and Mochel, R. S. W. C. (2020). Probable vertical transmission identified within six hours of life. Rev. Assoc. Med. Bras. 66, 1621–1624. doi: 10.1590/1806-9282.66.12.1621
Piersigilli, F., Carkeek, K., Hocq, C., van Grambezen, B., Hubinont, C., Chatzis, O., et al. (2020). COVID-19 in a 26-week preterm neonate. Lancet Child Adolesc. Health. 4, 476–478. doi: 10.1016/S2352-4642(20)30140-1
Pritchard, S., Wick, H. C., Slonim, D. K., Johnson, K. L., and Bianchi, D. W. (2012). Comprehensive analysis of genes expressed by rare microchimeric fetal cells in the maternal mouse lung. Biol. Reprod. 87:42. doi: 10.1095/biolreprod.112.101147
Puissant, B., Barreau, C., Bourin, P., Clavel, C., Corre, J., Bousquet, C., et al. (2005). Immunomodulatory effect of human adipose tissue-derived adult stem cells: comparison with bone marrow mesenchymal stem cells. Br. J. Haematol. 129, 118–129. doi: 10.1111/j.1365-2141.2005.05409.x
Pulinx, B., Kieffer, D., Michiels, I., Petermans, S., Strybol, D., Delvaux, S., et al. (2020). Vertical transmission of SARS-CoV-2 infection and preterm birth. Eur. J. Clin. Microbiol. Infect. Dis. 39, 2441–2445. doi: 10.1007/s10096-020-03964-y
Qin, H., and Zhao, A. (2020). Mesenchymal stem cell therapy for acute respiratory distress syndrome: from basic to clinics. Protein Cell. 11, 707–722. doi: 10.1007/s13238-020-00738-2
Ragab, D., Salah Eldin, H., Taeimah, M., Khattab, R., and Salem, R. (2020). The COVID-19 cytokine storm; what we know so far. Front. Immunol. 11:1446. doi: 10.3389/fimmu.2020.01446
Rasmusson, I. (2006). Immune modulation by mesenchymal stem cells. Exp Cell Res. 312, 2169–2179. doi: 10.1016/j.yexcr.2006.03.019
RECOVERY Collaborative Group, Horby, P., Lim, W. S., Emberson, J. R., Mafham, M., Bell, J. L., et al. (2020). Dexamethasone in hospitalized patients with Covid-19 - preliminary report. N. Engl. J. Med. doi: 10.1056/NEJMoa2021436 Online ahead of print
Remaeus, K., Savchenko, J., Brismar Wendel, S., Brusell Gidlöf, S., Graner, S., Jones, E., et al. (2020). Characteristics and short-term obstetric outcomes in a case series of 67 women test-positive for SARS-CoV-2 in Stockholm, Sweden. Acta Obstet. Gynecol. Scand. 99, 1626–1631. doi: 10.1111/aogs.14006
Richtmann, R., Torloni, M. R., Oyamada Otani, A. R., Levi, J. E., Crema Tobara, M., and de Almeida Silva, C. (2020). Fetal deaths in pregnancies with SARS-CoV-2 infection in Brazil: a case series. Case Rep. Womens Health. 27:e00243. doi: 10.1016/j.crwh.2020.e00243
Rivera-Hernandez, P., Nair, J., Islam, S., Davidson, L., Chang, A., and Elberson, V. (2020). Coronavirus disease 2019 in a premature infant: vertical transmission and antibody response or lack thereof. AJP Rep. 10, e224–e227. doi: 10.1055/s-0040-1715176
Rodrigues, M. L., Gasparinho, G., Sepúlveda, F., and Matos, T. (2021). Signs suggestive of congenital SARS-CoV-2 infection with intrauterine fetal death: a case report. Eur. J. Obstet. Gynecol. Reprod. Biol. 256, 508–509. doi: 10.1016/j.ejogrb.2020.11.042
Sagheb, S., Lamsehchi, A., Jafary, M., Atef-Yekta, R., and Sadeghi, K. (2020). Two seriously ill neonates born to mothers with COVID-19 pneumonia- a case report. Ital. J. Pediatr. 46:137. doi: 10.1186/s13052-020-00897-2
Saldanha-Araujo, F., Melgaço Garcez, E., Silva-Carvalho, A. E., and Carvalho, J. L. (2020). Mesenchymal stem cells: a new piece in the puzzle of COVID-19 treatment. Front. Immunol. 11:1563. doi: 10.3389/fimmu.2020.01563
Savasi, V. M., Parisi, F., Patanè, L., Ferrazzi, E., Frigerio, L., Pellegrino, A., et al. (2020). Clinical findings and disease severity in hospitalized pregnant women with coronavirus disease 2019 (COVID-19). Obstet. Gynecol. 136, 252–258. doi: 10.1097/AOG.0000000000003979
Scherger, S., Henao-Martínez, A., Franco-Paredes, C., and Shapiro, L. (2020). Rethinking interleukin-6 blockade for treatment of COVID-19. Med. Hypotheses. 144:110053. doi: 10.1016/j.mehy.2020.110053
Schoenmakers, S., Snijder, P., Verdijk, R. M., Kuiken, T., Kamphuis, S. S. M., Koopman, L. P., et al. (2020). SARS-CoV-2 placental infection and inflammation leading to fetal distress and neonatal multi-organ failure in an asymptomatic woman. medRxiv [Preprint] doi: 10.1101/2020.06.08.20110437
Schwartz, D. A., and Graham, A. L. (2020). Potential maternal and infant outcomes from (Wuhan) coronavirus 2019-nCoV infecting pregnant women: lessons from SARS, MERS, and other human coronavirus infections. Viruses 12:194. doi: 10.3390/v12020194
Schwartz, D. A., and Morotti, D. (2020). Placental pathology of COVID-19 with and without fetal and neonatal infection: trophoblast necrosis and chronic histiocytic intervillositis as risk factors for transplacental transmission of SARS-CoV-2. Viruses 12:1308. doi: 10.3390/v12111308
Sengupta, V., Sengupta, S., Lazo, A., Woods, P., Nolan, A., and Bremer, N. (2020). Exosomes derived from bone marrow mesenchymal stem cells as treatment for severe COVID-19. Stem Cells Dev. 29, 747–754. doi: 10.1089/scd.2020.0080
Sharma, A. K., Sane, H. M., Kulkarni, P. P., Gokulchandran, N., Biju, H., and Badhe, P. B. (2020). Autologous bone marrow mononuclear cell transplantation in patients with chronic traumatic brain injury- a clinical study. Cell Regen. 9:3. doi: 10.1186/s13619-020-00043-7
Shende, P., Gaikwad, P., Gandhewar, M., Ukey, P., Bhide, A., Patel, V., et al. (2020). Persistence of SARS-CoV-2 in the first trimester placenta leading to transplacental transmission and fetal demise from an asymptomatic mother. Hum. Reprod. doi: 10.1093/humrep/deaa367 Online ahead of print
Shu, L., Niu, C., Li, R., Huang, T., Wang, Y., Huang, M., et al. (2020). Treatment of severe COVID-19 with human umbilical cord mesenchymal stem cells. Stem Cell Res. Ther. 11:361. doi: 10.1186/s13287-020-01875-5
Sileo, F. G., Tramontano, A. L., Leone, C., Meacci, M., Gennari, W., Ternelli, G., et al. (2020). Pregnant woman infected by coronavirus disease (COVID-19) and calcifications of the fetal bowel and gallbladder: a case report. Minerva Ginecol. doi: 10.23736/S0026-4784.20.04717-6 Online ahead of print
Sisman, J., Jaleel, M. A., Moreno, W., Rajaram, V., Collins, R. R. J., Savani, R. C., et al. (2020). Intrauterine transmission of SARS-COV-2 infection in a preterm infant. Pediatr. Infect. Dis. J. 39, e265–e267. doi: 10.1097/INF.0000000000002815
Smithgall, M. C., Liu-Jarin, X., Hamele-Bena, D., Cimic, A., Mourad, M., Debelenko, L., et al. (2020). Third-trimester placentas of severe acute respiratory syndrome coronavirus 2 (SARS-CoV-2)-positive women: histomorphology, including viral immunohistochemistry and in-situ hybridization. Histopathology 77, 994–999. doi: 10.1111/his.14215
Soler Rich, R., Rius Tarruella, J., and Melgosa Camarero, M. T. (2020). Expanded Mesenchymal Stem Cells: a novel therapeutic approach of SARS-CoV-2 pneumonia (COVID-19). Concepts regarding a first case in Spain. Med. Clin. 155, 318–319. doi: 10.1016/j.medcli.2020.06.018
Stagg, J. (2007). Immune regulation by mesenchymal stem cells: two sides to the coin. Tissue Antigens. 69, 1–9. doi: 10.1111/j.1399-0039.2006.00739.x
Stappenbeck, T. S., and Miyoshi, H. (2009). The role of stromal stem cells in tissue regeneration and wound repair. Science 324, 1666–1669. doi: 10.1126/science.1172687
Stonoga, E. T. S., de Almeida Lanzoni, L., Rebutini, P. Z., Permegiani, de Oliveira, A. L., Chiste, J. A., et al. (2020). Intrauterine Transmission of SARS-CoV-2. Emerg. Infect. Dis. 27, 638–641. doi: 10.3201/eid2702.203824
Strem, B. M., Hicok, K. C., Zhu, M., Wulur, I., Alfonso, Z., Schreiber, R. E., et al. (2005). Multipotential differentiation of adipose tissue-derived stem cells. Keio. J. Med. 54, 132–141. doi: 10.2302/kjm.54.132
Sun, M., Xu, G., Yang, Y., Tao, Y., Pian-Smith, M., Madhavan, V., et al. (2020). Evidence of mother-to-newborn infection with COVID-19. Br. J. Anaesth. 125, e245–e247. doi: 10.1016/j.bja.2020.04.066
Swayne, L. A., Johnstone, S. R., Ng, C. S., Sanchez-Arias, J. C., Good, M. E., Penuela, S., et al. (2020). Consideration of Pannexin 1 channels in COVID-19 pathology and treatment. Am. J. Physiol. Lung Cell Mol. Physiol. 319, L121–L125. doi: 10.1152/ajplung.00146.2020
Taglauer, E., Benarroch, Y., Rop, K., Barnett, E., Sabharwal, V., Yarrington, C., et al. (2020). Consistent localization of SARS-CoV-2 spike glycoprotein and ACE2 over TMPRSS2 predominance in placental villi of 15 COVID-19 positive maternal-fetal dyads. Placenta 100, 69–74. doi: 10.1016/j.placenta.2020.08.015
Takemoto, M., Menezes, M. O., Andreucci, C. B., Knobel, R., Sousa, L., Katz, L., et al. (2020). Clinical characteristics and risk factors for mortality in obstetric patients with severe COVID-19 in Brazil: a surveillance database analysis. BJOG. 127, 1618–1626. doi: 10.1111/1471-0528.16470
Tao, J., Nie, Y., Wu, H., Cheng, L., Qiu, Y., Fu, J., et al. (2020). Umbilical cord blood-derived mesenchymal stem cells in treating a critically ill COVID-19 patient. J. Infect. Dev. Ctries. 14, 1138–1145. doi: 10.3855/jidc.13081
Tao, Y. C., and Chen, E. Q. (2020). Clinical application of stem cell in patients with end-stage liver disease: progress and challenges. Ann. Transl. Med. 8:564. doi: 10.21037/atm.2020.03.153
Thanunchai, M., Hongeng, S., and Thitithanyanont, A. (2015). Mesenchymal stromal cells and viral infection. Stem. Cells Int. 2015:860950. doi: 10.1155/2015/860950
Ti, D., Hao, H., Tong, C., Liu, J., Dong, L., Zheng, J., et al. (2015). LPS-preconditioned mesenchymal stromal cells modify macrophage polarization for resolution of chronic inflammation via exosome-shuttled let-7b. J. Transl. Med. 13:308. doi: 10.1186/s12967-015-0642-6
Toner, L. E., Gelber, S. E., Pena, J. A., Fox, N. S., and Rebarber, A. (2020). A case report to assess passive immunity in a COVID positive pregnant patient. Am. J. Perinatol. 37, 1280–1282. doi: 10.1055/s-0040-1715643
Vashukova, M. A., Tsinzerling, V. A., Semenova, N. Y., Lugovskaya, N. A., Narkevich, T. A., and Sukhanova, Y. V. (2020). Is perinatal COVID-19 possible: first results. J. Infectol. 12, 51–55. doi: 10.22625/2072-6732-2020-12-3-51-55
Vendola, N., Stampini, V., Amadori, R., Gerbino, M., Curatolo, A., and Surico, D. (2020). Vertical transmission of antibodies in infants born from mothers with positive serology to COVID-19 pneumonia. Eur. J. Obstet. Gynecol. Reprod. Biol. 253, 331–332. doi: 10.1016/j.ejogrb.2020.08.023
Verma, S., Bradshaw, C., Auyeung, N. S. F., Lumba, R., Farkas, J. S., Sweeney, N. B., et al. (2020). Outcomes of maternal-newborn dyads after maternal SARS-CoV-2. Pediatrics 146:e2020005637. doi: 10.1542/peds.2020-005637
Vivanti, A. J., Vauloup-Fellous, C., Prevot, S., Zupan, V., Suffee, C., Do Cao, J., et al. (2020). Transplacental transmission of SARS-CoV-2 infection. Nat. Commun. 11:3572. doi: 10.1038/s41467-020-17436-6
Walker, K. F., O’Donoghue, K., Grace, N., Dorling, J., Comeau, J. L., Li, W., et al. (2020). Maternal transmission of SARS-COV-2 to the neonate, and possible routes for such transmission: a systematic review and critical analysis. BJOG 127, 1324–1336. doi: 10.1111/1471-0528.16362
Wang, S., Guo, L., Chen, L., Liu, W., Cao, Y., Zhang, J., et al. (2020). A case report of neonatal 2019 coronavirus disease in China. Clin. Infect. Dis. 71, 853–857. doi: 10.1093/cid/ciaa225
Weiss, A. R. R., and Dahlke, M. H. (2019). Immunomodulation by mesenchymal stem cells (MSCs): mechanisms of action of living, apoptotic, and dead MSCs. Front. Immunol. 10:1191. doi: 10.3389/fimmu.2019.01191
Weiss, D. J. (2014). Concise review: current status of stem cells and regenerative medicine in lung biology and diseases. Stem Cells 32, 16–25. doi: 10.1002/stem.1506
Wu, J., Hu, Z., Wang, L., Tan, Y., Hou, W., Li, Z., et al. (2020a). First case of COVID-19 infused with hESC derived immunity- and matrix-regulatory cells. Cell Prolif. 53:e12943. doi: 10.1111/cpr.12943
Wu, J., Song, D., Li, Z., Guo, B., Xiao, Y., Liu, W., et al. (2020b). Immunity-and-matrix-regulatory cells derived from human embryonic stem cells safely and effectively treat mouse lung injury and fibrosis. Cell Res. 30, 794–809. doi: 10.1038/s41422-020-0354-1
Wu, J., Zhou, X., Tan, Y., Wang, L., Li, T., Li, Z., et al. (2020c). Phase 1 trial for treatment of COVID-19 patients with pulmonary fibrosis using hESC-IMRCs. Cell Prolif. 53:e12944. doi: 10.1111/cpr.12944
Wu, Y. T., Liu, J., Xu, J. J., Chen, Y. F., Yang, W., Chen, Y., et al. (2020d). Neonatal outcome in 29 pregnant women with COVID-19: a retrospective study in Wuhan, China. PLoS Med. 17:e1003195. doi: 10.1371/journal.pmed.1003195
Wu, Y., Hoogduijn, M. J., Baan, C. C., Korevaar, S. S., de Kuiper, R., Yan, L., et al. (2017). Adipose tissue-derived mesenchymal stem cells have a heterogenic cytokine secretion profile. Stem Cells Int. 2017:4960831. doi: 10.1155/2017/4960831
Yilmaz, R., Adas, G., Cukurova, Z., Kart Yasar, K., Isiksacan, N., Oztel, O. N., et al. (2020). Mesenchymal stem cells treatment in COVID-19 patient with multi-organ involvement. Bratisl. Lek. Listy. 121, 847–852. doi: 10.4149/BLL_2020_139
Zaigham, M., Holmberg, A., Karlberg, M. L., Lindsjö, O. K., Jokubkiene, L., Sandblom, J., et al. (2020). Intrauterine Transmission of SARS-CoV-2 - A Confirmed Case. Abstract Presented at “State of the Art Covid-19” on 15-16 December 2020. Available online at: https://www.sls.se/state-of-the-art-covid-19/abstracts/Abstract-book/ (accessed October 30, 2020).
Zamaniyan, M., Ebadi, A., Aghajanpoor, S., Rahmani, Z., Haghshenas, M., and Azizi, S. (2020). Preterm delivery, maternal death, and vertical transmission in a pregnant woman with COVID-19 infection. Prenat Diagn. 40, 1759–1761. doi: 10.1002/pd.5713
Zeng, H., Xu, C., Fan, J., Tang, Y., Deng, Q., Zhang, W., et al. (2020). Antibodies in infants born to mothers with COVID-19 pneumonia. JAMA 323, 1848–1849. doi: 10.1001/jama.2020.4861
Zeng, L., Xia, S., Yuan, W., Yan, K., Xiao, F., Shao, J., et al. (2020). Neonatal early-onset infection with SARS-CoV-2 in 33 neonates born to mothers with COVID-19 in Wuhan, China. JAMA Pediatr. 174, 722–725. doi: 10.1001/jamapediatrics.2020.0878
Zeng, X. X., Tan, K. H., Yeo, A., Sasajala, P., Tan, X., Xiao, Z. C., et al. (2010). Pregnancy-associated progenitor cells differentiate and mature into neurons in the maternal brain. Stem Cells Dev. 19, 1819–1830. doi: 10.1089/scd.2010.0046
Zengin, R., Beyaz, O., Koc, E. S., Akinci, I. O., Kocagoz, S., Sagcan, G., et al. (2020). Mesenchymal stem cell treatment in a critically ill COVID-19 patient: a case report. Stem Cell Investig. 7:17. doi: 10.21037/sci-2020-024
Zhang, Y., Ding, J., Ren, S., Wang, W., Yang, Y., Li, S., et al. (2020). Intravenous infusion of human umbilical cord Wharton’s jelly-derived mesenchymal stem cells as a potential treatment for patients with COVID-19 pneumonia. Stem Cell Res. Ther. 11:207. doi: 10.1186/s13287-020-01725-4
Keywords: COVID-19, SARS-CoV-2, neonate, pregnancy, vertical, mesenchymal, MSCs
Citation: Samara A and Herlenius E (2021) Is There an Effect of Fetal Mesenchymal Stem Cells in the Mother–Fetus Dyad in COVID-19 Pregnancies and Vertical Transmission? Front. Physiol. 11:624625. doi: 10.3389/fphys.2020.624625
Received: 31 October 2020; Accepted: 30 December 2020;
Published: 11 February 2021.
Edited by:
Adriana Castello Costa Girardi, University of São Paulo, BrazilReviewed by:
David Zurakowski, Harvard Medical School, United StatesVenkata Garikipati, The Ohio State University, United States
Copyright © 2021 Samara and Herlenius. This is an open-access article distributed under the terms of the Creative Commons Attribution License (CC BY). The use, distribution or reproduction in other forums is permitted, provided the original author(s) and the copyright owner(s) are credited and that the original publication in this journal is cited, in accordance with accepted academic practice. No use, distribution or reproduction is permitted which does not comply with these terms.
*Correspondence: Athina Samara, athina.samara@ki.se
†ORCID: Eric Herlenius, orcid.org/0000-0002-6859-0620