- 1Department of Cardiology, Fuwai Hospital, Chinese Academy of Medical Sciences, Shenzhen, China
- 2Department of Cardiovascular Medicine, The Second Affiliated Hospital of Nanchang University, Nanchang, China
- 3Huazhong University of Science and Technology Union Shenzhen Hospital, Shenzhen, China
The present study was undertaken to examine the association between intermittent hypoxia and left ventricular (LV) remodeling and explore which parameter of intermittent hypoxia is most relevant to LV remodeling in patients with obstructive sleep apnea-hypopnea syndrome (OSAHS). Two hundred eighty six patients underwent polysomnographic examination were enrolled. Based on apnea-hypoxia index (AHI), patients were divided into no, mild, moderate and severe OSAHS groups. Between-group differences in LV remodeling and the association between parameters of intermittent hypoxia and LV remodeling was evaluated. Patients with severe OSAHS were more likely to have hypertension, and higher values of LV mass (LVM) and LVM index (LVMI). In univariate regression analysis, male, body mass index (BMI), systolic and diastolic blood pressure (BP), statins, antihypertensive drugs, creatinine, and parameters of intermittent hypoxia (AHI, obstructive apnea index [OAI], lowest oxygen saturation [LSpO2], oxygen desaturation index [ODI], time spent below oxygen saturation of 90% [TS90%], and mean nocturnal oxygen saturation [MSpO2]) were associated with LVMI. After multivariate regression analyses, only male gender, BMI, systolic BP, creatinine, and ODI remained significantly associated with LVMI. Compared to those without LV hypertrophy (LVH), patients with LVH had higher ODI. Compared to patients with normal LV, concentric remodeling and eccentric LVH, those with concentric LVH had higher ODI. In conclusion, intermittent hypoxia was significantly associated with left ventricular remodeling; and among various parameters of intermittent hypoxia, ODI was the most relevant to LV remodeling.
Introduction
With the epidemic of overweight and obesity (Twig et al., 2016; Mouton et al., 2020), the incidence and prevalence of obstructive sleep apnea-hypopnea syndrome (OSAHS) is continuously increasing around the world including China (Cai et al., 2017; Osman et al., 2018). Numerous studies have demonstrated that OSAHS patients have a higher risk of target organ damage (TOD), cardiovascular disease, and all-cause mortality even after adjustment for traditional risk factors and comorbidities (Wang et al., 2016; Floras, 2018; Perger et al., 2019). Unfortunately, up till now, despite promising results in observational studies, there is no evidence from randomized controlled trials demonstrating that OSAHS management by continuous positive airway pressure (CPAP) can improve clinical outcomes (McEvoy et al., 2016; Da Silva Paulitsch and Zhang, 2019; Patil et al., 2019). Therefore, better understanding the mechanisms by which OSAHS causes TOD may help to prevent disease progression and reduce cardiovascular events and mortality.
Left ventricular hypertrophy (LVH) is a sensitive and specific marker of left ventricular remodeling (Huang et al., 2017; Sha et al., 2017). LVH progression results in the development of congestive heart failure, which is associated with great health and economic burdens (Yancy et al., 2013; Ponikowski et al., 2016; Cai et al., 2018). Prior studies have shown that the prevalence of LVH is higher in people with OSAHS than their counterparts without OSAHS (Sekizuka et al., 2017; Hanlon et al., 2019; Maitikuerban et al., 2019). In addition, there is a linear relationship between the severity of OSAHS and LVH (Cuspidi et al., 2019, 2020; Krasińska et al., 2019). The mechanisms are partly attributable to OSAHS-induced blood pressure elevation and intrathoracic mechanical pressure increase (Cai et al., 2016; Cuspidi et al., 2019, 2020; Krasińska et al., 2019). Notably, due to intermittent obstruction of upper respiratory airway, people with OSAHS experience intermittent hypoxia, which causes reactive oxygen species production and endothelial dysfunction (Perrini et al., 2017; Sozer et al., 2018). Numerous studies have reported the adverse effects of intermittent hypoxia on cardiovascular system (Beaudin et al., 2017; Perrini et al., 2017; Bostanci et al., 2018), however, whether there is an independent association between intermittent hypoxia and LVH is unknown. In addition, which parameter of intermittent hypoxia is the most clinically relevant to LVH development is also unknown. This entity is important because if confirmed, intervention to intermittent hypoxia may help to retard LV remodeling progression and prevent LVH development, which in turn reduces the incidence of congestive heart failure. Furthermore, the most relevant parameter of intermittent hypoxia identified can be used as a specific marker to quantify the risk of developing LVH.
Herein, leveraging data from our ongoing prospective cohort study, we conducted a cross-sectional analysis to evaluate that: (1) the association between intermittent hypoxia and LV remodeling; (2) which parameter of intermittent hypoxia is most relevant to LV remodeling in patients with OSAHS.
Materials and Methods
Study Participants
Participants who were admitted to our hospital for polysomnography (PSG) examination were screened, and the exclusion criteria were as follow: (1) prior diagnosis of central sleep apnea; (2) secondary hypertension except for OSAHS-induced hypertension; (3) coexisting cardiovascular disease which could influence left ventricle structure and/or function: coronary heart disease, rheumatic heart disease, congenital heart disease, cardiomyopathy, or left ventricular ejection fraction <50%; (4) coexisting pulmonary disease: chronic obstructive pulmonary disease, acute asthma, moderate to severe pleural effusion, interstitial lung disease, or severe pulmonary infection; (5) the duration of PSG examination <7 h or poor quality of PSG images; (6) lack of important baseline clinical data. Between September 2015 and November 2017, a total of 286 people who had underwent PSG examination and qualified for the study criteria were enrolled (Figure 1). The scoring for obstructive apneas and hypopneas attributed to either central or obstructive events was performed according to The AASM Manual for the Scoring of Sleep and Associated Events Version 2.4 (Berry et al., 2017). The current study was approved by the Clinical Research Ethic Committee of the Second Affiliated Hospital of NanChang University. Signed informed consent was obtained before participant's enrollment.
Baseline Characteristics Collection
Baseline characteristics, including age, sex, body weight and height, smoking status and alcohol consumption, prior medical history and current medications used, were collected via the electronic health record. Fasting venous blood was drawn for the analysis of uric acid, creatinine, blood urea nitrogen (BUN), lipid panel, fasting plasma glucose (FPG) and homocysteine. Body mass index (BMI) was calculated using weight in kilograms divided by height in squared meters, and BMI ≥28 kg/m2 was defined as obesity based on the World Health Organization criteria for Asian population (WHO Expert Consultation, 2004). Estimated glomerular filtration rate (eGFR) was calculated using the Modification of Diet in Renal Disease (MDRD) formula.
PSG Examination
PSG examination was performed to evaluate the apnea-hypopnea index (AHI) using Philips Respironics Alice PDx. Participants were informed about the detailed procedures of PSG examination. Twenty-four hours before PSG examination, sedative medications, alcohol, tea and coffee were not allowed. The total duration of PSG examination must be >7 h. If the airflow was completely blocked for more than 10 s or >50% reduction in respiratory airflow accompanying >3% reduction in oxygen saturation (SaO2) for more than 10 s, the apnea or hypopnea events would be recorded. Based on the the AASM Manual for the Scoring of Sleep and Associated Events Version 2.4 (Berry et al., 2017), participants with AHI of 5–15 events/h were defined as mild, 16–30 events/h were moderate, and 30 events/h were severe OSAHS. In addition, other relevant intermittent hypoxia parameters, including obstructive apnea index (OAI), lowest oxygen saturation (LSpO2), oxygen desaturation index (ODI), time spent below oxygen saturation of 90% (TS90%), and mean nocturnal oxygen saturation (MSpO2) were also collected concurrently.
Echocardiogram and LVH Evaluation
Echocardiogram was performed by experienced cardiologists using the Phillip iE33. Left ventricular ejection fraction (LVEF), left ventricular internal diameter in diastole (LVIDd), left ventricular internal diameter in systole (LVIDs), interventricular septal thickness (IVS), and left ventricular posterior wall thickness (LVPW) were measured in accordance to the guideline recommendation (Badano et al., 2018; Heart Failure Group of Chinese Society of Cardiology of Chinese Medical Association, 2018). Relative wall thickness (RWT) was calculated as follow: (IVS + LVPW)/LVIDd. Left ventricular mass (LVM) was calculated as follow: 0.8 × {1.04 × [(LVIDd + LVPW + IVS)3 – (LVIDd)3]} + 0.6 g. The prevalence of obesity was high in current analysis, therefore, LVM index (LVMI) was adjusted for height2.7 and the formula was as follow: LVMI-height2.7 = LVM/height2.7 g/m2.7. LVMI-height2.7 > 49.2 g/m2.7 in men and LVMI-height2.7 > 46.7 g/m2.7 in women was defined as LVH. Based on the Ganau criteria (Badano et al., 2018), LV remodeling was classified as follows: normal (without LVH and RWT < 0.42), concentric remodeling (without LVH and RWT ≥ 0.42), eccentric hypertrophy (LVH and RWT < 0.42), and concentric hypertrophy (LVH and RWT ≥ 0.42).
Statistical Analysis
Continuous variables were presented as mean ± standard deviation (SD) or median (interquartile range; IQR), and between-group difference was compared using one-way ANOVA for variables with normal distribution, and variables with skewed distribution were compared using Kruskal-Wallis H. Categorial variables were presented as number and percentage and between-group difference was compared using the Chi-square. Univariate regression analyses and stepwise multivariate regression analyses were performed to evaluate factors associated with LVMI-height2.7. All the analyses were performed using the IBM SPSS statistic 22.0 and a P-value < 0.05 was considered as statistical significance.
Results
Selection of Study Participants
From March 2015 to November 2017, 532 patients underwent PSG examination in the cardiology department, and a total of 286 patients were included into final analysis in accordance to inclusion and exclusion criteria (Figure 1). Among these patients, 5.6, 13.3, 31.1, and 50% were diagnosed as no OSAHS, mild, moderate and severe OSAHS, respectively.
Baseline Characteristics Comparisons
As presented in Table 1, compared to patients with mild or moderate OSAHS, those with severe OSAHS were older, and had higher BMI and diastolic blood pressure (P < 0.05). Patients with severe OSAHS were more likely to have hypertension and to use angiotensin converting enzyme inhibitor/angiotensin receptor blocker (ACEI/ARB; P < 0.05). Serum level of uric acid was also higher in patients with severe OSAHS than those with mild or moderate OSAHS (P < 0.05). No significant differences in other baseline characteristics were observed.
Echocardiographic Parameters Comparisons
As presented in Table 2, compared to patients with mild, moderate and severe OSAHS, right ventricular internal diameter and LVIDd were smaller while the E/A ratio was higher in patients with no OSAHS (P < 0.05). Compared to patients without OSAHS, mild or moderate OSAHS, those with severe OSAHS had higher values of IVS, LVIDs, LVM, LVMI-height2.7, A wave velocity and e' wave velocity (P < 0.05).
Polysomnographic Parameters Comparisons
As presented in Table 3, compared to patients without OSAHS, mild or moderate OSAHS, those with severe OSAHS had higher AHI, OAI, ODI, and TS90% (P < 0.05), while MSpO2 and LSpO2 was lower in patients with severe OSAHS (P < 0.05).
Factors Associated With LVMI-Height2.7
As presented in Table 4, in univariate linear regression analysis, male, BMI, systolic and diastolic blood pressure, hypertension, statins, antihypertensive drugs, BUN, creatinine, eGFR, AHI, OAI, LSpO2, ODI, TS90%, and MSpO2 were significantly associated with LVMI-height2.7. However, after multivariate linear regression analyses, only male, BMI, systolic blood pressure, BUN, creatinine, and ODI remained significantly associated with LVMI-height2.7.
Comparison of ODI According to LV Remodeling
As presented in Figure 2A, compared to those without LVH, patients with LVH had higher ODI (P < 0.05). In addition, as presented in Figure 2B, compared to patients with normal LV, concentric remodeling, and eccentric LVH, those with concentric LVH had higher ODI (P < 0.05).
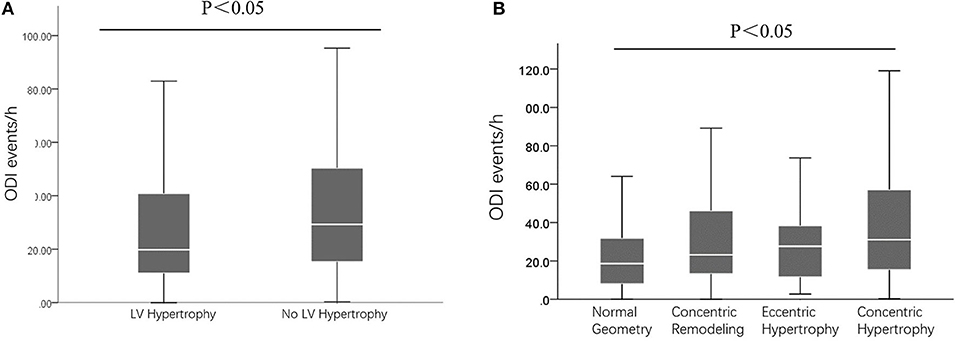
Figure 2. The ODI events between/among different patients. (A) Comparisons of ODI between those with and without LV hypertrophy; (B) Comparisons of ODI between those with different LV remodeling. ODI, oxygen desaturation index; LV, left ventricular.
Discussion
In the present study, we evaluated the association of intermittent hypoxia and LV remodeling in Chinese populations. There are two main findings of current study. First, intermittent hypoxia was significantly associated with left ventricular remodeling as reflected in increased LVMI-height2.7. Second, among various parameters of intermittent hypoxia, ODI was the most relevant to LVMI-height2.7. These findings together suggest that intervention of intermittent hypoxia may help to retard LV remodeling progression and prevent LVH development. ODI may be a useful marker to predict LVH development and to reflect the efficacy of intervention for intermittent hypoxia.
OSAHS has been considered as an important comorbidity for a variety of cardiovascular diseases (Wang et al., 2016; Floras, 2018; Perger et al., 2019). Numerous studies have shown that increased AHI, an indicator of OSAHS severity, is independently associated with TOD and cardiovascular diseases. For example, Chami et al. (2008) reported that compared to patients with AHI <5 events/h, those with AHI >30 events/h had 1.8-fold higher prevalence of LVH. Cai et al. (2018) reported that increased AHI was associated with higher prevalence of congestive heart failure event after adjusted for other risk factors. Sun et al. (2014) also reported that compared to patients without OSAHS, those with OSAHS had higher IVS, LVIDs, and LVIDd, reflecting a significant LV remodeling. Consistent to prior reports, the current analysis also showed that the values of IVS and LVIDs were higher in patients with severe OSAHS than those without OSAHS or those with less severe OSAHS. Furthermore, we also observed that the LVM and LVMI-height2.7 were also significantly higher in patients with severe OSAHS. These findings together strongly indicate that presence of OSAHS is significantly associated with LV remodeling, which presented in a dose-dependent fashion. Furthermore, the velocity of A wave was faster while the lateral mitral annulus e' wave velocity was lower in patients with severe OSAHS, suggesting an impaired diastolic LV dysfunction, which we considered might be due to LV hypertrophic remodeling. With increasing prevalence and incidence of overweight and obesity, it is prudent to screen people with unrecognized or undiagnosed OSAHS, and timely and effective intervention should be implemented to prevent LV remodeling and associated cardiovascular disease.
Various parameters of intermittent oxygen have been developed to assess the severity of OSAHS. The AHI has been the most common use to diagnose and define the severity of OSAHS in clinical practice. However, there are also some limitations of AHI, and the most important one is that the AHI cannot quantitatively reflect the oxygen desaturation during intermittent hypoxia (Kendzerska and Leung, 2016; Kapoor, 2018; Camporro et al., 2019). In the current study, we included six common use parameters of intermittent hypoxia and evaluated their association with LVMI-height2.7. As presented in Table 3, the changes of these six parameters were in accordance to increasing severity of OSAHS. In addition, in the univariate linear regression analysis, all these parameters were significantly associated with LVMI-height2.7, suggesting each individual parameter is a relevant marker to LV remodeling. Nonetheless, after adjustment for other potential confounding factors including gender, BMI, blood pressure and among others, only ODI remained significantly associated with LVMI-height2.7. These findings highlight that compared to other parameters of intermittent hypoxia, ODI might be the most relevant marker related to LV remodeling. Indeed, results from prior reports also support our findings. For example, Tkacova et al. (2014) reported that in hypertensive patients with OSAHS, only ODI was associated with LV remodeling in multivariate regression model. The reasons for the superiority of ODI in relation to LV remodeling are likely multiple, including as follows (Jung Da et al., 2016; Kim et al., 2016; Myllymaa et al., 2016): first, ODI reflects the frequency of intermittent hypoxia, and increased ODI is directly associated with increased hypoxia-reoxygenation injury, causing endothelial dysfunction, arterial constriction, blood pressure elevation, and LV afterload increase; second, increased ODI is associated with incrementally reduced oxygen supply to cardiac tissue, which leaded to cardiomyocytes necrosis and cardiac fibrosis accumulation; third, increased ODI is also associated with renin-angiotensin-aldosterone axis activation, resulting in LV remodeling and hypertrophy. These several lines of evidence suggest that in patients with OSAHS, assessment and monitoring of ODI might provide valuable information to evaluate LV remodeling progression and LVH development. Up to date, there is no clinical data assessing the effects of direct oxygen supplementation on the clinical outcomes for OSAHS treatment. However, most studies performed the CPAP to indirectly improve the oxygen supplementation, which may improve the LVH in the OSAHS patients. Studies showed that CPAP could reverse the LVH in the patients with severe OSA (Cloward et al., 2003; Shivalkar et al., 2006). Colish et al. (2012) demonstrated that both systolic and diastolic abnormalities in patients with OSA could be reversed as early as 3 months into CPAP therapy, with progressive improvement in cardiovascular remodeling over 1 year. In addition, Marin et al. (2005) showed that CPAP treatment reduced the risk of fatal and non-fatal cardiovascular events in the male patients with OSAHS. The improvement of AHI and LSpO2 are the main indicators for assessing treatment outcomes of patients with OSAHS, and our results suggest that the decrease in ODI value may be more effective in predicting the improvement of LVH compared with AHI and LSpO2. Further studies are needed to evaluate whether improvement of ODI is associated with improvement of LV remodeling and lower risk of LVH. Beyond the level of oxygen saturation achieved, LVH may be caused by a different background sensitivity of the chemoreflex system to hypoxia, and patients with similar ODI may have different degree of LVH depending on the chemoreflex (Deacon-Diaz and Malhotra, 2018). In addition, hypoxia may drive to chemoreflex mediated adrenergic discharge and thus LVH, which still requires future investigations.
There are some limitations of current study needed to address. First, this is a cross-sectional study, and therefore causal relationship should not be drawn. Second, despite we have extensively adjusted for potential confounding factors, unmeasured and undetected factors might still exist and influence the association of ODI and LVMI-height2.7. Third, this is a single-center study conducted in Chinese populations and these findings might not be extrapolated to other racial/ethnic populations. Fourth, all participants did not receive any intervention and therefore these findings might not be applied those received treatment, such as continuous positive airway pressure (CPAP). Fifth, the severity of the sample is large with 50% of patients classified as severe, as the data in this study was mainly collected from the patients suspected with OSAHS and these patients were then subjected to the PSG. Studies from Yamaguchi et al. (2016) showed that the percentage of patients with AHI >30 events/h was ≥63%, which was similar to our study. However, large sample size should be considered in the future studies. Last but not the least, consistent to study design, people with cardiovascular and pulmonary diseases were excluded. Therefore, these findings might not be extrapolated to those with cardiovascular or pulmonary diseases.
Conclusion
In conclusion, the current study shows that among patients with untreated OSAHS, intermittent hypoxia is significantly associated with LV remodeling; and among various parameters of intermittent hypoxia, ODI was the most relevant to LVH. Future studies are needed to evaluate whether improvement of ODI by CPAP treatment can prevent or ameliorate LV remodeling.
Data Availability Statement
The raw data supporting the conclusions of this article will be made available by the authors, without undue reservation.
Ethics Statement
The studies involving human participants were reviewed and approved by the Clinical Research Ethic Committee of the Second Affiliated Hospital of NanChang University. The patients/participants provided their written informed consent to participate in this study.
Author Contributions
MD and X-sC: conception and design of the research. MD, Y-tH, and J-qX: recruiting the volunteers and performing the clinical experiments. XK and Y-fD: interpreted the results. X-sC: draft the manuscript. All authors read and approved the final manuscript.
Funding
This study was supported by the Sanming Project of Medicine in Shenzhen of China (SZSM20162048).
Conflict of Interest
The authors declare that the research was conducted in the absence of any commercial or financial relationships that could be construed as a potential conflict of interest.
Acknowledgments
We appreciate very much for all participants to join in current study.
Abbreviations
AHI, apnea-hypopnea index; BMI, body mass index; BUN, blood urea nitrogen; CPAP, continuous positive airway pressure; eGFR, estimated glomerular filtration rate; FPG, fasting plasma glucose; IQR, interquartile range; IVS, interventricular septal thickness; LSpO2, lowest oxygen saturation; LV, left ventricular; LVEF, left ventricular ejection fraction; LVH, left ventricular hypertrophy; LVIDd, left ventricular internal diameter in diastole; LVIDs, left ventricular internal diameter in systole; LVM, left ventricular mass; LVPW, left ventricular posterior wall thickness; MDRD, modification of diet in renal disease; MSpO2, mean nocturnal oxygen saturation; OAI, obstructive apnea index; ODI, oxygen desaturation index; OSAHS, obstructive sleep apnea-hypopnea syndrome; PSG, polysomnography; RWT, relative wall thickness; SaO2, oxygen saturation; SD, standard deviation; TOD, target organ damage; TS90%, oxygen saturation of 90%.
References
Badano, L. P., Kolias, T. J., Muraru, D., Abraham, T. P., Aurigemma, G., Edvardsen, T., et al. (2018). Standardization of left atrial, right ventricular, and right atrial deformation imaging using two-dimensional speckle tracking echocardiography: a consensus document of the EACVI/ASE/Industry Task Force to standardize deformation imaging. Eur. Heart J. Cardiovasc. Imaging 19, 591–600. doi: 10.1093/ehjci/jey042
Beaudin, A. E., Waltz, X., Hanly, P. J., and Poulin, M. J. (2017). Impact of obstructive sleep apnoea and intermittent hypoxia on cardiovascular and cerebrovascular regulation. Exp. Physiol. 102, 743–763. doi: 10.1113/EP086051
Berry, R. B., Brooks, R., Gamaldo, C., Harding, S. M., Lloyd, R. M., Quan, S. F., et al. (2017). AASM scoring manual updates for 2017 (version 2.4). J. Clin. Sleep Med. 13, 665–666. doi: 10.5664/jcsm.6576
Bostanci, A., Bozkurt, S., and Turhan, M. (2018). Impact of age on intermittent hypoxia in obstructive sleep apnea: a propensity-matched analysis. Sleep Breath 22, 317–322. doi: 10.1007/s11325-017-1560-z
Cai, A., Wang, L., and Zhou, Y. (2016). Hypertension and obstructive sleep apnea. Hypertens. Res. 39, 391–395. doi: 10.1038/hr.2016.11
Cai, A., Zhang, J., Wang, R., Chen, J., Huang, B., Zhou, Y., et al. (2018). Joint effects of obstructive sleep apnea and resistant hypertension on chronic heart failure: a cross-sectional study. Int. J. Cardiol. 257, 125–130. doi: 10.1016/j.ijcard.2017.10.089
Cai, A., Zhou, Y., Zhang, J., Zhong, Q., Wang, R., and Wang, L. (2017). Epidemiological characteristics and gender-specific differences of obstructive sleep apnea in a Chinese hypertensive population: a cross-sectional study. BMC Cardiovasc. Disord. 17:8. doi: 10.1186/s12872-016-0447-4
Camporro, F. A., Kevorkof, G. V., and Alvarez, D. (2019). Sleep-disordered breathing: looking beyond the apnea/hypopnea index. Arch. Bronconeumol. 55, 396–397. doi: 10.1016/j.arbres.2018.12.003
Chami, H. A., Devereux, R. B., Gottdiener, J. S., Mehra, R., Roman, M. J., Benjamin, E. J., et al. (2008). Left ventricular morphology and systolic function in sleep-disordered breathing: the Sleep Heart Health Study. Circulation 117, 2599–2607. doi: 10.1161/CIRCULATIONAHA.107.717892
Cloward, T. V., Walker, J. M., Farney, R. J., and Anderson, J. L. (2003). Left ventricular hypertrophy is a common echocardiographic abnormality in severe obstructive sleep apnea and reverses with nasal continuous positive airway pressure. Chest 124, 594–601. doi: 10.1378/chest.124.2.594
Colish, J., Walker, J. R., Elmayergi, N., Almutairi, S., Alharbi, F., Lytwyn, M., et al. (2012). Obstructive sleep apnea: effects of continuous positive airway pressure on cardiac remodeling as assessed by cardiac biomarkers, echocardiography, and cardiac MRI. Chest 141, 674–681. doi: 10.1378/chest.11-0615
Cuspidi, C., Sala, C., and Tadic, M. (2019). Obstructive sleep apnea and left ventricular hypertrophy: more questions than answers. J. Clin. Hypertens. 21, 1908–1909. doi: 10.1111/jch.13718
Cuspidi, C., Tadic, M., Sala, C., Gherbesi, E., Grassi, G., and Mancia, G. (2020). Targeting concentric left ventricular hypertrophy in obstructive sleep apnea syndrome. A Meta-analysis of Echocardiographic Studies. Am. J. Hypertens. 33, 310–315. doi: 10.1093/ajh/hpz198
Da Silva Paulitsch, F., and Zhang, L. (2019). Continuous positive airway pressure for adults with obstructive sleep apnea and cardiovascular disease: a meta-analysis of randomized trials. Sleep Med. 54, 28–34. doi: 10.1016/j.sleep.2018.09.030
Deacon-Diaz, N., and Malhotra, A. (2018). Inherent vs. induced loop gain abnormalities in obstructive sleep apnea. Front. Neurol. 9:896. doi: 10.3389/fneur.2018.00896
Floras, J. S. (2018). Sleep apnea and cardiovascular disease: an enigmatic risk factor. Circ. Res. 122, 1741–1764. doi: 10.1161/CIRCRESAHA.118.310783
Hanlon, C. E., Binka, E., Garofano, J. S., Sterni, L. M., and Brady, T. M. (2019). The association of obstructive sleep apnea and left ventricular hypertrophy in obese and overweight children with history of elevated blood pressure. J. Clin. Hypertens. 21, 984–990. doi: 10.1111/jch.13605
Heart Failure Group of Chinese Society of Cardiology of Chinese Medical Association Chinese Heart Failure Association of Chinese Medical Doctor Association, and Editorial Board of Chinese Journal of Cardiology. (2018). Chinese guidelines for the diagnosis and treatment of heart failure 2018. Zhonghua Xin Xue Guan Bing Za Zhi 46, 760–789. doi: 10.3760/cma.j.issn.0253-3758.2018.10.004
Huang, Y., Tang, S., Huang, C., Chen, J., Li, J., Cai, A., et al. (2017). Circulating miRNA29 family expression levels in patients with essential hypertension as potential markers for left ventricular hypertrophy. Clin. Exp. Hypertens. 39, 119–125. doi: 10.1080/10641963.2016.1226889
Jung Da, W., Hwang, S. H., Lee, Y. J., Jeong, D. U., and Park, K. S. (2016). Oxygen desaturation index estimation through unconstrained cardiac sympathetic activity assessment using three ballistocardiographic systems. Respiration 92, 90–97. doi: 10.1159/000448120
Kapoor, M. (2018). Discrepancy between oxygen desaturation index and apnea-hypopnea index: what do you do with the results? J. Clin. Sleep Med. 14:1437. doi: 10.5664/jcsm.7296
Kendzerska, T., and Leung, R. S. (2016). Going beyond the apnea-hypopnea index. Chest 149, 1349–1350. doi: 10.1016/j.chest.2016.02.671
Kim, T. J., Ko, S. B., Jeong, H. G., Lee, J. S., Kim, C. K., Kim, Y., et al. (2016). Nocturnal desaturation in the stroke unit is associated with wake-up ischemic stroke. Stroke 47, 1748–1753. doi: 10.1161/STROKEAHA.116.013266
Krasińska, B., Cofta, S., Szczepaniak-Chicheł, L., Rzymski, P., Trafas, T., Paluszkiewicz, L., et al. (2019). The effects of eplerenone on the circadian blood pressure pattern and left ventricular hypertrophy in patients with obstructive sleep apnea and resistant hypertension–a randomized controlled trial. J. Clin. Med. 8:1671. doi: 10.3390/jcm8101671
Maitikuerban, B., Sun, X., Li, Y., Chen, Y., Zhang, J., Simayi, Z., et al. (2019). Deletion polymorphism of angiotensin converting enzyme gene is associated with left ventricular hypertrophy in uighur hypertension-obstructive sleep apnea hypopnea syndrome (OSAHS) patients. Med. Sci. Monit. 25, 3390–3396. doi: 10.12659/MSM.916019
Marin, J. M., Carrizo, S. J., Vicente, E., and Agusti, A. G. (2005). Long-term cardiovascular outcomes in men with obstructive sleep apnoea-hypopnoea with or without treatment with continuous positive airway pressure: an observational study. Lancet 365, 1046–1053. doi: 10.1016/S0140-6736(05)71141-7
McEvoy, R. D., Antic, N. A., Heeley, E., Luo, Y., Ou, Q., Zhang, X., et al. (2016). CPAP for prevention of cardiovascular events in obstructive sleep apnea. N. Engl. J. Med. 375, 919–931. doi: 10.1056/NEJMoa1606599
Mouton, A. J., Li, X., Hall, M. E., and Hall, J. E. (2020). Obesity, hypertension, and cardiac dysfunction: novel roles of immunometabolism in macrophage activation and inflammation. Circ. Res. 126, 789–806. doi: 10.1161/CIRCRESAHA.119.312321
Myllymaa, K., Myllymaa, S., Leppänen, T., Kulkas, A., Kupari, S., Tiihonen, P., et al. (2016). Effect of oxygen desaturation threshold on determination of OSA severity during weight loss. Sleep Breath 20, 33–42. doi: 10.1007/s11325-015-1180-4
Osman, A. M., Carter, S. G., Carberry, J. C., and Eckert, D. J. (2018). Obstructive sleep apnea: current perspectives. Nat. Sci. Sleep 10, 21–34. doi: 10.2147/NSS.S124657
Patil, S. P., Ayappa, I. A., Caples, S. M., Kimoff, R. J., Patel, S. R., and Harrod, C. G. (2019). Treatment of adult obstructive sleep apnea with positive airway pressure: an American Academy of Sleep Medicine Clinical Practice guideline. J. Clin. Sleep. Med. 15, 335–343. doi: 10.5664/jcsm.7640
Perger, E., Gonzaga-Carvalho, C., Inami, T., Ryan, C. M., and Lyons, O. D. (2019). Obstructive sleep apnea and cardiovascular disease. Am. J. Respir. Crit. Care Med. 199, 377–379. doi: 10.1164/rccm.201709-1875RR
Perrini, S., Cignarelli, A., Quaranta, V. N., Falcone, V. A., Kounaki, S., Porro, S., et al. (2017). Correction of intermittent hypoxia reduces inflammation in obese subjects with obstructive sleep apnea. JCI Insight 2:e94379. doi: 10.1172/jci.insight.94379
Ponikowski, P., Voors, A. A., Anker, S. D., Bueno, H., Cleland, J. G.F., Coats, A. J.S., et al. (2016). 2016 ESC Guidelines for the diagnosis and treatment of acute and chronic heart failure: The Task Force for the diagnosis and treatment of acute and chronic heart failure of the European Society of Cardiology (ESC) developed with the special contribution of the Heart Failure Association (HFA) of the ESC. Eur. Heart J. 37, 2129–2200. doi: 10.1093/eurheartj/ehw128
Sekizuka, H., Osada, N., and Akashi, Y. J. (2017). Impact of obstructive sleep apnea and hypertension on left ventricular hypertrophy in Japanese patients. Hypertens. Res. 40, 477–482. doi: 10.1038/hr.2016.170
Sha, T., Huang, Y. Q., Cai, A. P., Huang, C., Zhang, Y., Chen, J. Y., et al. (2017). Prevalence and determinants of left ventricular geometric abnormalities in hypertensive patients: a study based on the updated classification system of left ventricular geometry. Hellenic J. Cardiol. 58, 124–132. doi: 10.1016/j.hjc.2016.12.011
Shivalkar, B., Van De Heyning, C., Kerremans, M., Rinkevich, D., Verbraecken, J., De Backer, W., et al. (2006). Obstructive sleep apnea syndrome: more insights on structural and functional cardiac alterations, and the effects of treatment with continuous positive airway pressure. J. Am. Coll. Cardiol. 47, 1433–1439. doi: 10.1016/j.jacc.2005.11.054
Sozer, V., Kutnu, M., Atahan, E., Caliskaner Ozturk, B., Hysi, E., Cabuk, C., et al. (2018). Changes in inflammatory mediators as a result of intermittent hypoxia in obstructive sleep apnea syndrome. Clin. Respir. J. 12, 1615–1622. doi: 10.1111/crj.12718
Sun, Y., Yuan, H., Zhao, M. Q., Wang, Y., Xia, M., and Li, Y. Z. (2014). Cardiac structural and functional changes in old elderly patients with obstructive sleep apnoea-hypopnoea syndrome. J. Int. Med. Res. 42, 395–404. doi: 10.1177/0300060513502890
Tkacova, R., Mcnicholas, W. T., Javorsky, M., Fietze, I., Sliwinski, P., Parati, G., et al. (2014). Nocturnal intermittent hypoxia predicts prevalent hypertension in the European Sleep Apnoea Database cohort study. Eur. Respir. J. 44, 931–941. doi: 10.1183/09031936.00225113
Twig, G., Yaniv, G., Levine, H., Leiba, A., Goldberger, N., Derazne, E., et al. (2016). Body-mass index in 2.3 million adolescents and cardiovascular death in adulthood. N. Engl. J. Med. 374, 2430–2440. doi: 10.1056/NEJMoa1503840
Wang, L., Cai, A., Zhang, J., Zhong, Q., Wang, R., Chen, J., et al. (2016). Association of obstructive sleep apnea plus hypertension and prevalent cardiovascular diseases: a cross-sectional study. Medicine (Baltimore) 95:e4691. doi: 10.1097/MD.0000000000004691
WHO Expert Consultation (2004). Appropriate body-mass index for Asian populations and its implications for policy and intervention strategies. Lancet 363, 157–163. doi: 10.1016/S0140-6736(03)15268-3
Yamaguchi, T., Takata, Y., Usui, Y., Asanuma, R., Nishihata, Y., Kato, K., et al. (2016). Nocturnal intermittent hypoxia is associated with left ventricular hypertrophy in middle-aged men with hypertension and obstructive sleep apnea. Am. J. Hypertens. 29, 372–378. doi: 10.1093/ajh/hpv115
Yancy, C. W., Jessup, M., Bozkurt, B., Butler, J., Casey, D. E. Jr., Drazner, M. H., et al. (2013). 2013 ACCF/AHA guideline for the management of heart failure: executive summary: a report of the American College of Cardiology Foundation/American Heart Association Task Force on practice guidelines. Circulation 128, 1810–1852. doi: 10.1161/CIR.0b013e31829e8807
Keywords: obstructive sleep apnea-hypopnea syndrome, left ventricular remodeling, intermittent hypoxia, oxygen desaturation index, apnea-hypoxia index
Citation: Deng M, Huang Y-t, Xu J-q, Ke X, Dong Y-f and Cheng X-s (2021) Association Between Intermittent Hypoxia and Left Ventricular Remodeling in Patients With Obstructive Sleep Apnea-Hypopnea Syndrome. Front. Physiol. 11:608347. doi: 10.3389/fphys.2020.608347
Received: 20 September 2020; Accepted: 18 December 2020;
Published: 12 February 2021.
Edited by:
Alberto Giannoni, Sant'Anna School of Advanced Studies, ItalyReviewed by:
Sergio L. Cravo, Federal University of São Paulo, BrazilAmalia Peix, Instituto de Cardiología y Cirugía Cardiovascular, Cuba
Copyright © 2021 Deng, Huang, Xu, Ke, Dong and Cheng. This is an open-access article distributed under the terms of the Creative Commons Attribution License (CC BY). The use, distribution or reproduction in other forums is permitted, provided the original author(s) and the copyright owner(s) are credited and that the original publication in this journal is cited, in accordance with accepted academic practice. No use, distribution or reproduction is permitted which does not comply with these terms.
*Correspondence: Xiao-shu Cheng, xiaoshumenfan126@163.com
†These authors have contributed equally to this work