- 1College of Life Sciences, Anhui Normal University, Wuhu, China
- 2State Key Laboratory for Biology of Plant Diseases and Insect Pests, Institute of Plant Protection, Chinese Academy of Agricultural Sciences, Beijing, China
- 3MoA-CABI Joint Laboratory for Bio-safety, Institute of Plant Protection, Chinese Academy of Agricultural Sciences, Beijing, China
- 4Anhui Provincial Key Laboratory for the Conservation and Exploitation of Biology Resources, College of Life Sciences, Anhui Normal University, Wuhu, China
The brown marmorated stink bug, Halyomorpha halys (Hemiptera: Pentatomidae), is a serious agricultural and urban pest that has become an invasive species in many parts of the world. Olfaction plays an indispensable role in regulating insect behaviors, such as host plant location, partners searching, and avoidance of predators. In this study, we sequenced and analyzed the antennal transcriptomes of both male and female adults of H. halys to better understand the olfactory mechanisms in this species. A total of 241 candidate chemosensory genes were identified, including 138 odorant receptors (ORs), 24 ionotropic receptors (IRs), 15 gustatory receptors (GRs), 44 odorant-binding proteins (OBPs), 17 chemosensory proteins (CSPs), and three sensory neuron membrane proteins (SNMPs). The results of semi-quantitative reverse transcription PCR (RT-PCR) assays showed that some HhalOBP and HhalCSP genes have tissue-specific and sex-biased expression patterns. Our results provide an insight into the molecular mechanisms of the olfactory system in H. halys and identify potential novel targets for pest control strategies.
Introduction
Natural environments are characterized by a wide variety of odors, and insects have developed corresponding olfactory systems that enable them to recognize and interpret the complicated odorant information (Su et al., 2009). The complex chemosensory system enables insects to sense the volatile odors of host plants, conspecific individuals, and natural enemies, which allows them to locate food sources, mating partners, and predators (Field et al., 2000; Hansson, 2002; Bruce et al., 2005; Asahina et al., 2008; Sato and Touhara, 2009). The recognition of odor molecules by insects is a very sophisticated process. The odorants are detected by olfactory receptor neurons (ORNs) distributed in cuticular sensilla, which are mainly housed in the antennae wherein the chemical signals are converted into electrical signals that are further transmitted to the antennal lobe. The electrical signals then activate the central nervous system and ultimately guide the insect to respond accordingly (Hansson, 2002; Anton et al., 2003; Sato and Touhara, 2009; Rospars et al., 2010; Fleischer et al., 2018).
In the process by which chemical signals are converted into electrical signals at the peripheral nerve level, both receptor and non-receptor gene families are involved. The former includes odorant receptors (ORs), ionotropic receptors (IRs), and gustatory receptors (GRs), whereas the latter includes at least odorant-binding proteins (OBPs), chemosensory proteins (CSPs), and sensory neuron membrane proteins (SNMPs) (Dunipace et al., 2001; Dahanukar et al., 2005; Jin et al., 2008; Vogt et al., 2009; Koh et al., 2014; Wicher, 2015; Agnihotri et al., 2016; Sun et al., 2016; Du et al., 2018).
Insect OR is a type of transmembrane receptor that has seven transmembrane domains (TMDs; Clyne et al., 1999; Smart et al., 2008) and is mainly expressed in the dendritic membrane of the ORNs. The ORs form a heterodimer composed of a conserved, non-conventional OR co-receptor (Orco) and a variable, conventional ORx (Benton et al., 2006; Sato et al., 2008; Leal, 2013). IRs were originally discovered by expression of olfactory neurons and belong to the ionotropic glutamate receptor (iGluR) gene family, a highly conserved family of ligand-gated ion channels (Benton et al., 2009; Croset et al., 2010; Rytz et al., 2013). Recent functional studies indicate that IRs have diverse functions in chemical reception and participate in the sensation of odorants, temperature, humidity, and salt (Chen et al., 2015; Zhang et al., 2019). GRs play key roles in the sensing of CO2, sugars, bitter compounds, salts, and some gustatory pheromones (Dahanukar et al., 2007; Kwon et al., 2007; Wanner and Robertson, 2008; Kent and Robertson, 2009; Xu et al., 2012; Mang et al., 2016; Fleischer et al., 2018).
In addition to the three types of receptors, there are a variety of non-receptor proteins involved in insects olfactory perception including OBPs, CSPs, and SNMPS. OBPs and CSPs are small hydrophilic proteins that are abundant in the sensillum lymph. OBPs have six conserved cysteines, which are known as their most prominent characteristic, and can bind to the hydrophobic odor molecules and finally transport them through the sensory lymph to the ORNs around the membrane, activating ORs (Brito et al., 2016). CSPs belong to another class of relatively low-molecular-weight hydrophilic proteins, which are ubiquitous in the sensillum lymph (Jin et al., 2005). CSPs also are expressed in non-olfactory tissues (Jin et al., 2006) and are involved in insect growth and development. The SNMPs are homologous to mammalian CD36 proteins family (Rogers et al., 2001; Nichols and Vogt, 2008). Three subgroups of SNMPs (SNMP1, SNMP2, and SNMP3) have been identified in many insects orders (Rogers et al., 2001; Nichols and Vogt, 2008; Vogt et al., 2009; Gu et al., 2013b; Jiang et al., 2016; Yang et al., 2017; Zhang et al., 2020). SNMP1 plays a role in the detection of sex pheromones, but the exact functions of SNMP2 and SNMP3 are presently unknown (Gomez-Diaz et al., 2016).
The brown marmorated stink bug Halyomorpha halys (Hemiptera: Pentatomidae) is a major agricultural pest that is native to much of Asia. In the past 20 years, this pest has invaded parts of North America, South America, and Europe, causing great economic losses and degrading the ecosystem dynamics (Acebes-Doria et al., 2018). H. halys is a polyphagous pest that can feed on at least 88 host plants including fruits, vegetables, crops, nuts, weeds, and wild plants (Bergmann et al., 2016; Botch and Delfosse, 2018). This pest often invades human dwellings and commercial buildings and secretes volatile compounds that have a detrimental effect on human skin and eyes (Anderson et al., 2012; Shen and Hu, 2017). Two previous studies have reported transcriptome datasets from distinct developmental stages of H. halys, but neither of these studies involved the antennal transcriptome, especially chemosensory genes (Ioannidis et al., 2014; Sparks et al., 2014). Paula et al. (2016) identified a total of 30 full-length putative OBP genes in the antennal transcriptome of H. halys and examined their expression in the presence of food, aggregation pheromone, and alarm pheromone stimuli, but they did not analyze other olfactory genes. In this study, we identified 241 candidate olfactory genes in H. halys comprising 138 ORs, 24 IRs, 15 GRs, 44 OBPs, 17 CSPs, and three SNMPs in the adult H. halys antennae. The sequence and phylogenetic analyses were performed on these important olfactory genes. Tissue-specific expression patterns of the OBP and CSP genes were determined using semi-quantitative reverse transcription PCR (RT-PCR). The results of this study not only lay a foundation for the functional characterization of these chemosensory genes but also provide a theoretical basis for the future development of new technology to control H. halys using insect-related olfactory genes as targets.
Materials and Methods
Insect Rearing and Tissue Collection
Overwintering H. halys adults were collected from Baiwang Mountain Forest Park (116°21′43″–116°28′12″E, 39°57′52″–40°02′11″), Beijing, China. Male and female adults were identified by sexing. The antennae were removed with tweezers, flash frozen in liquid nitrogen, and stored at −70°C until use.
cDNA Library Construction and Sequencing
Total RNA was extracted from one pool each of 30 male antennae and 30 female antennae of H. halys using TRIzol reagent, following the manufacturer’s instructions (Invitrogen, Carlsbad, CA, United States). The purity and concentration of the RNA samples were determined using a NanoDrop ND-2000 Spectrophotometer (NanoDrop Technologies, Inc., Wilmington, DE, United States), and RNA integrity was verified by gel electrophoresis. Two micrograms of RNA from each sample was used for cDNA library construction. The construction and sequencing of the two H. halys antennae cDNA libraries (male and female) were performed at Beijing Genomics Institute (Shenzhen, China). The insert sequence length was ∼280 bp. The two libraries were pair-end sequenced using PE150 strategy in Illumina HiSeq 4000 platform (Illumina, San Diego, CA, United States).
Transcriptome Assembly and Functional Annotation
Datasets of clean reads were generated from the raw reads by removing adaptor sequences, poly-N-containing reads, and low-quality sequences. The clean reads of the two transcriptomes produced in this study are stored in the National Center for Biotechnology Information (NCBI) Sequence Read Archive (SRA) database under the accession numbers SRR11748354 (female antennae) and SRR11747758 (male antennae). The clean-read datasets of male and female antenna were fed to Trinity (release-20130225) for de novo transcriptome assembly using the pair-end reads mode with default parameters (Grabherr et al., 2011). The Trinity outputs were clustered using TGICL V2.1 (Pertea et al., 2003). The consensus cluster sequences and singletons made up the final unigene dataset.
Identification of Chemosensory Genes
Unigenes were annotated using blastx searches against the NCBI non-redundant (nr) database with an e-value < 1e–5. Candidate unigenes encoding putative ORs, IRs, GRs, OBPs, CSPs, and SNMPs were identified based on to the nr annotation results. All candidate chemosensory genes were then manually checked using the blastx program against the nr database. And all predicted olfactory gene sequences possessed overlapping regions with low identity and, therefore, likely represent unigenes.
Sequence and Phylogenetic Analyses
The open reading frames (ORFs) of all genes were predicted using ExPASy server1. Putative N-terminal signal peptides of OBPs and CSPs were predicted by SignalP 4.02 in default parameters (Petersen et al., 2011). The TMDs of ORs, IRs, and GRs were predicted using the TMHMM server version 2.03. Amino acid sequence alignments were performed with MAFFT4. Phylogenetic trees of CSPs were constructed by RAxML version 8 using the Jones–Taylor–Thornton (JTT) amino acid substitution model (Stamatakis, 2014), and a bootstrap procedure of 1000 replicates was used to evaluate the node support. The OR phylogenetic tree was constructed using the total of 319 ORs from three Hemiptera species: 138 ORs from H. halys, 122 from Oncopeltus fasciatus, and 59 from Tessaratoma papillosa (Wu et al., 2017; Panfilio et al., 2019). For IRs, a phylogenetic analysis was conducted using a dataset containing all 24 IRs in H. halys together with other insects including 39 IRs from Nilaparvata lugens, 37 from O. fasciatus, 32 from Rhodnius prolixus 12 from T. papillosa, and 80 from Drosophila melanogaster (Benton et al., 2009; Mesquita et al., 2015; Wu et al., 2017; He et al., 2018; Panfilio et al., 2019). For GRs, a phylogenetic analysis that included 15 GRs from H. halys, 28 from N. lugens, 169 from O. fasciatus, and 31 from R. prolixus was performed (Mesquita et al., 2015; He et al., 2018; Panfilio et al., 2019). The OBP phylogenetic tree was constructed using 44 OBPs from our H. halys, 17 from R. prolixus, 33 from T. papillosa, five from Acyrthosiphon pisum, and 10 from Cyrtorhinus lividipennis (De Biasio et al., 2015; Mesquita et al., 2015; Wang G.Y. et al., 2017; Wu et al., 2017). For CSPs, the phylogenetic tree was constructed using 17 CSPs from H. halys, nine from Aphis gossypii, 11 from N. lugens, and eight from R. prolixus (Gu et al., 2013a; Mesquita et al., 2015; Xue et al., 2016).
Semi-Quantitative Reverse Transcription PCR
Reverse transcription PCR was performed to examine the expression of candidate OBP and CSP genes in different tissues and both sexes of H. halys. Thirty pairs of antennae, 10 heads (without antennae), five thoraxes, five abdomens, and 36 legs (including the fore, median, and hind legs) were collected from male and female H. halys adults. Total RNA was extracted as described above. The cDNA was synthesized from total RNA using the RevertAid First Strand cDNA Synthesis Kit (Thermo Fisher Scientific, Pittsburgh, PA, United States). Gene-specific primers were designed using Primer Premier 5.0 software (PREMIER Biosoft, Palo Alto, CA, United States) and synthesized by Sangon Biotech (Shanghai, China). An actin gene fragment was used as a reference. The sequences of primers are listed in Supplementary Table S1. The RT-PCR assays were conducted with a Veriti Thermal Cycler (Applied Biosystems, Carlsbad, CA, United States) and performed in a 20-μl reaction system, which contained 10 μl of 2 × Taq MasterMix (CWBIO, Beijing, China), 1 μl of each primer (10 μM), 1 μl of cDNA, and 7 μl of deionized water. The PCRs were 94°C for 3 min, followed by 27–35 cycles of 94°C for 30 s, 53–58°C (primer-dependent) for 30 s, and 72°C for 30 s, with a final extension at 72°C for 10 min. The PCR products were separated by electrophoresis on 2% agarose gels, stained by ethidium bromide (EB), and photographed under UV light using a Gel Doc XR + System with Image Lab Software (Bio-Rad, Hercules, CA, United States). The RT-PCR was repeated three times using one group of RNA samples (three technical replicates).
Results
Sequencing and Unigene Assembly
The antennal transcriptomes of female and male H. halys adults were constructed and sequenced separately on Illumina HiSeq 4000 platform. A total of 80.33 million and 85.57 million raw reads were obtained from the male and female antennae, respectively. After filtering, 74.26 million and 78.97 million clean reads were generated from the male and female H. halys antennae transcriptomes, respectively. The de novo assemblies led to the generation of 48,875 and 58,935 unigenes from the female antenna and male antenna, respectively. After merging and clustering, a final transcript dataset with 65,914 unigenes was produced. The dataset was 71.5 megabases in size with a mean length of 1085 nt and N50 of 2342 nt (Supplementary Table S2).
Identification of Candidate Odorant Receptors
Candidate ORs were identified using key word searches of the blastx annotation. We identified 138 putative OR genes in H. halys. Of these candidate ORs, 93 unigenes were full-length putative OR genes with complete ORFs and an average length of 1200 bp and five to eight predicted TMDs, which is characteristic of typical insect ORs. The OR co-receptor, named HhalOrco, was also found. Other putative ORs (HhalOR1–HhalOR137) were given names followed by a numeral in descending order of the length of their coding regions. Information including unigene reference, length, and best blastx hit of all putative ORs is listed in Supplementary Table S3. The amino acid sequences of the ORs identified in this study are provided in Supplementary File S1.
The phylogeny of H. halys ORs is shown in Figure 1. The phylogenetic tree was constructed using the candidate ORs of H. halys and other Hemipteran ORs containing T. papillosa and R. prolixus. As expected, the highly conserved HhalOrco belonged to the same clade as the orthologous proteins from the two other Hemiptera species (Figure 1). Compared with OfasORs, HhalORs were clustered more closely to TpapORs. Compared with the ORs from the other two species, we found five expansions in the number of ORs in H. halys, which were marked as HhalOR-clade 1 to HhalOR-clade 5 in Figure 1.
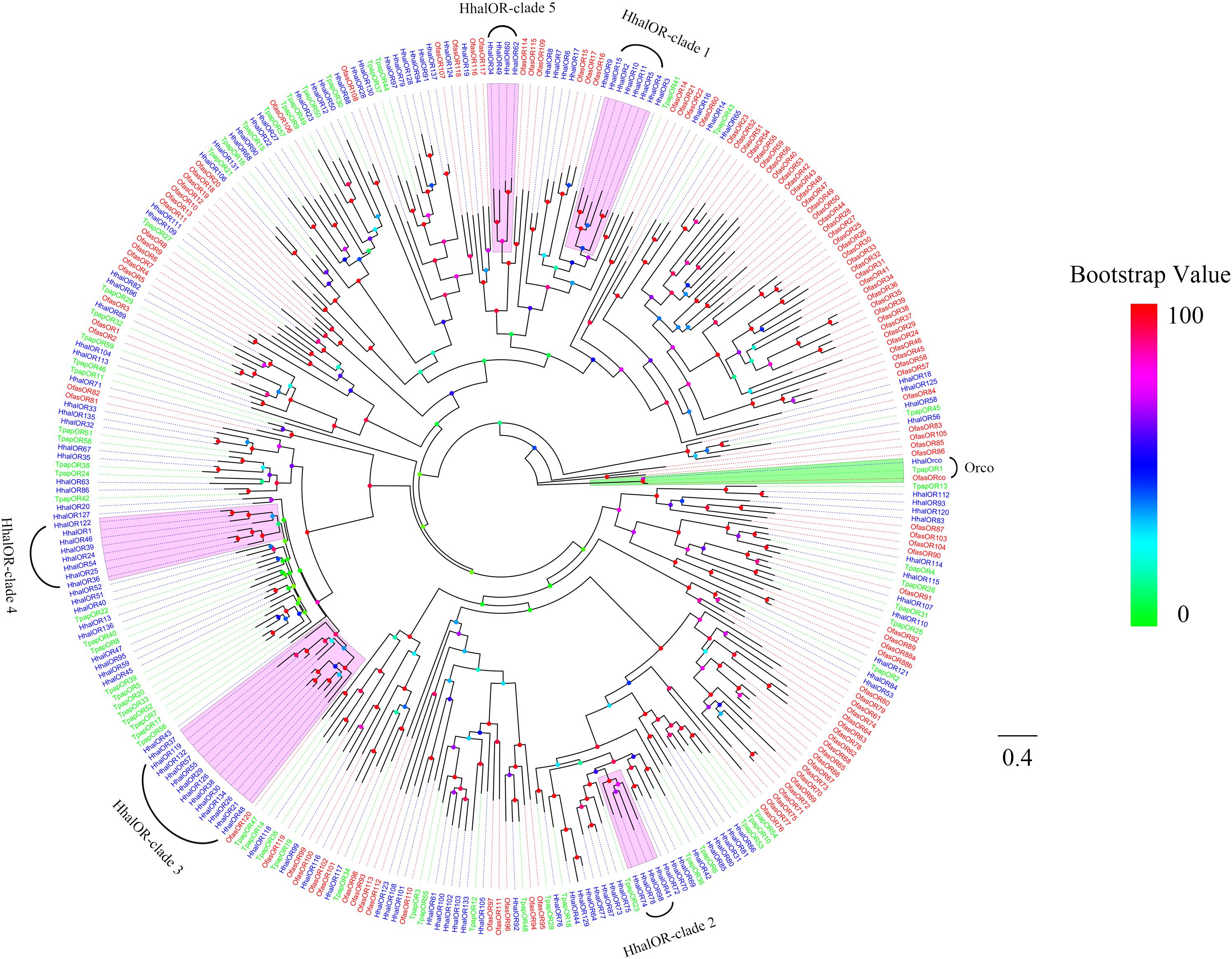
Figure 1. Phylogenetic tree of putative HhalORs with known Hemiptera odorant receptors (ORs). Hhal, Halyomorpha halys (blue); Tpap, Tessaratoma papillosa (green); Ofas, Oncopeltus fasciatus (red). The clade in green indicates the Orco and in lavender the H. halys expansions ORs. The scale bar equals 0.4 substitutions per sequence position. The bootstrap values are shown in the circles of different colors.
Identification of Candidate Ionotropic Receptors
The second type of olfactory receptor, IR, belongs to an ancient chemosensory receptor family. In the H. halys transcriptome in the present study, 24 putative IRs were identified based on their similarity to known insect IRs. Of these IRs, eight sequences contained full-length ORFs, and the remaining 16 sequences were incomplete owing to lacking a 5′ and/or 3′ terminus. A total of 17 IRs contain more than three TMDs as predicted by TMHMM 2.0 (Supplementary Table S3), which is consistent with the characteristics of insect IRs. The two IR co-receptors HhalIR8a and HhalIR25a were readily detected. Eight HhalIRs (HhalIR21a.1, 21a.2, 60f, 68a, 75d.1, 75d.2, 76b, and 93a) were named based on their orthologous relationships with IRs from D. melanogaster. Other putative IRs were named based on their homology from O. fasciatus and R. prolixus or by a numeral in descending order of the length of their corresponding coding regions. Information including unigene reference, length, and best blastx hit of all putative IRs is listed in Supplementary Table S4. The amino acid sequences of the IRs identified in this study are provided in Supplementary File S1.
We performed a phylogenetic analysis using the candidate HhalIRs and IRs of T. papillosa, O. fasciatus, R. prolixus, N. lugens, and D. melanogaster (Figure 2). Obviously, the co-receptors Hhal8a and IR25a clustered to form the IR8a and IR25a evolutionary clades, respectively. The remaining IRs clustered on different branches of other species, and almost all IRs are convergent to T. papillosa and O. fasciatus. Significant separation of iGluRs from IRs was found in the phylogenetic tree. A unique HhalIR2 gene that has no homologous gene in other close species was found (Figure 2).
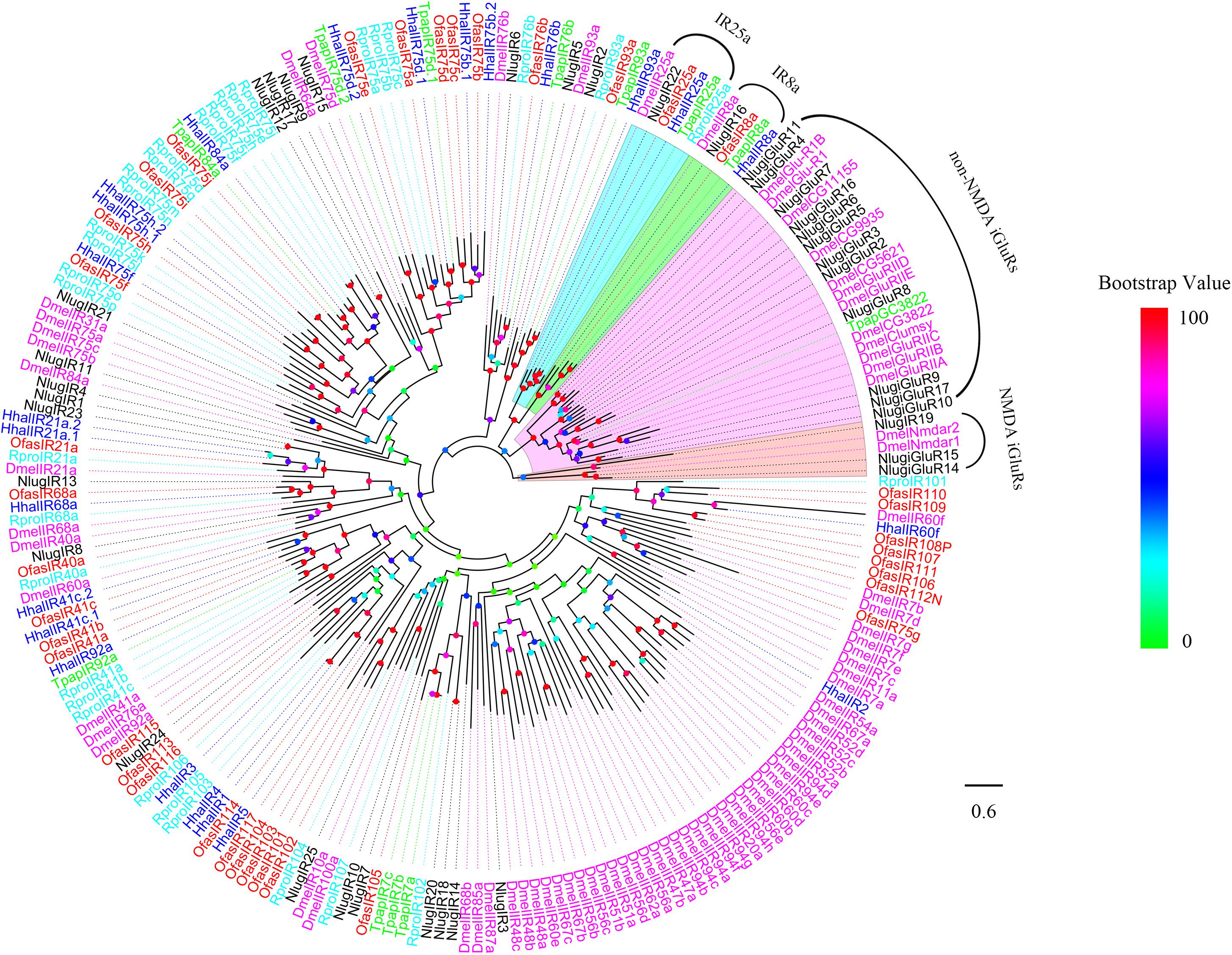
Figure 2. Phylogenetic tree of putative HhalIRs with known Hemiptera ionotropic receptors (IRs). Hhal, Halyomorpha halys (blue); Tpap, Tessaratoma papillosa (green); Ofas, Oncopeltus fasciatus (red); Rpro, Rhodnius prolixus (cyan); Dmel, Drosophila melanogaster; Nlug, Nilaparvata lugens (black). The clade in violet indicates the non-NMDA ionotropic glutamate receptors, in orange indicates NMDA ionotropic glutamate receptors, in cyan indicates the IR25a, and in green indicates the IR8a. The scale bar equals 0.6 substitutions per sequence position. The bootstrap values are shown in the circles of different colors.
Identification of Candidate Gustatory Receptors
Fifteen putative GRs were identified in the H. halys transcriptome. Four GR sequences contained a full-length ORF; the remaining 11 sequences were incomplete owing to the absence of the 5′ and/or 3′ terminus (Supplementary Table S5). All putative GRs (HhalGR1–HhalGR15) were given a name followed by a numeral in descending order of the length of their coding regions. Information including unigene reference, length, and best blastx hit of all putative GRs is listed in Supplementary Table S5. The amino acid sequences of the GRs identified in this study are provided in Supplementary File S1.
To identify GRs in H. halys, the putative proteins were analyzed phylogenetically against known Hemipteran GRs including sugar receptor and CO2 receptor from O. fasciatus, R. prolixus, and N. lugens (Figure 3). The phylogenetic analysis showed that HhalGR1 and HhalGR2 are in a clade with the sugar receptors, whereas the highly similar HhalGR3, HhalGR5, and HhalGR10-13 were members of the candidate CO2 receptor. There was no GR in H. halys that clustered with the fructose lineage. HhalGR6 clustered with OfasGR42a; and HhalGR15 clustered together with OfasGR56; and both clusters had high bootstrap support. We identified a species-specific branch that included four HhalGRs: HhalGR7–9 and HhalGR14.
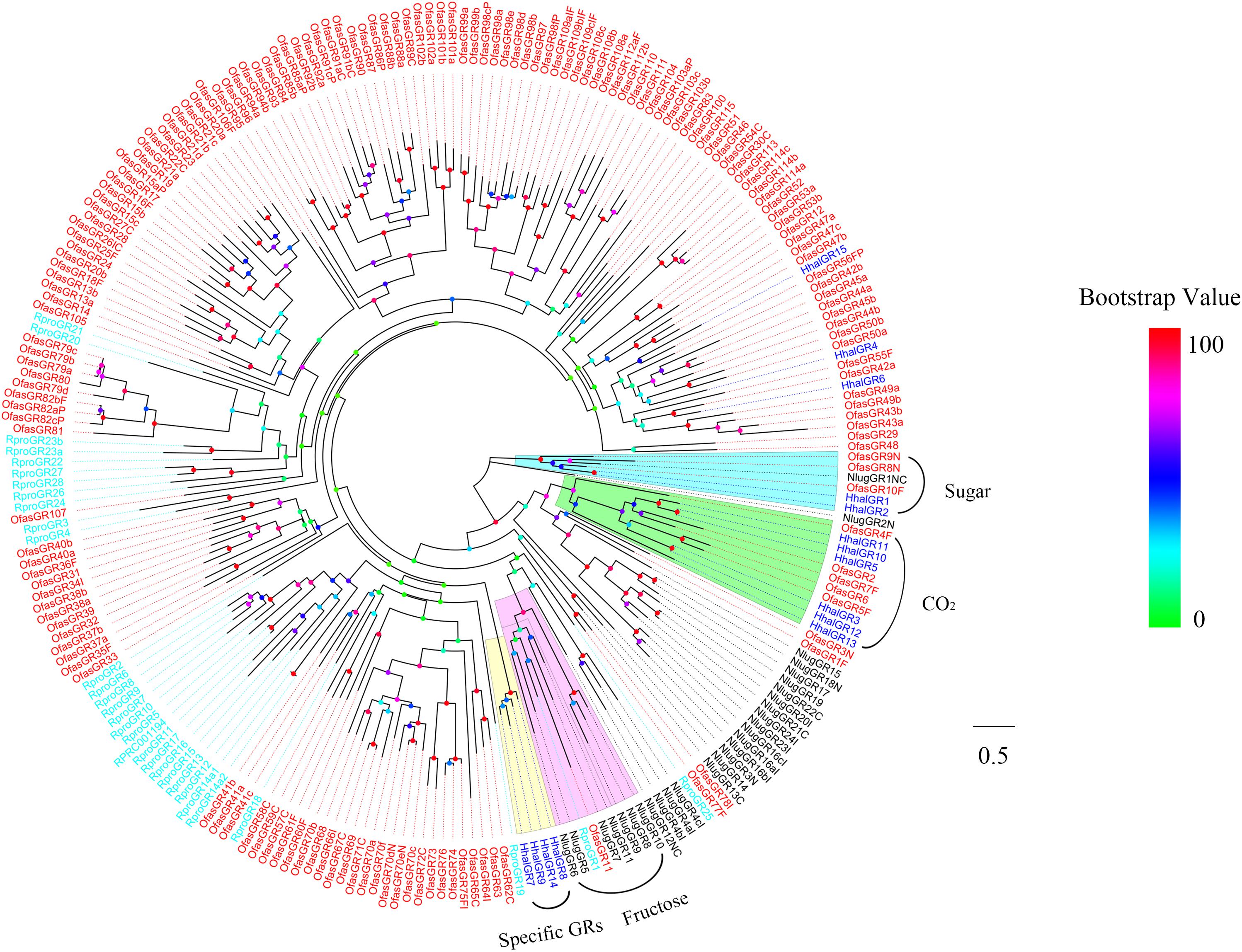
Figure 3. Phylogenetic tree of putative HhalGRs with known Hemiptera gustatory receptors (GRs). Hhal, Halyomorpha halys (blue); Ofas, Oncopeltus fasciatus (red); Rpro, Rhodnius prolixus (cyan); Nlug, Nilaparvata lugens (black). The clade in cyan indicates the sugar receptors, in green the CO2 receptors, in violet the fructose receptors, and in yellow the H. halys specific GRs. The scale bar equals 0.5 substitutions per sequence position. The bootstrap values are shown in the circles of different colors.
Identification of Candidate Odorant-Binding Proteins
Forty-four putative unigenes encoding OBPs were identified from the H. halys transcriptome. Among them, 37 were full-length putative OBP genes, and the remaining seven were incomplete owing to the absence of the 5′ or 3′ terminus (Supplementary Table S6). All the 30 OBPs (HhalOBP1–30) identified in the previous study were found in our transcriptome, and we also found an additional 14 new OBPs (HhalOBP31–HhalOBP44), which were named using the same convention for the ORs, IRs, and GRs. Information including unigene reference, length, and best blastx hit for all putative OBPs is listed in Supplementary Table S6. The amino acid sequences of the OBPs identified in this study are provided in Supplementary File S1.
The phylogenetic tree of HhalOBPs was constructed using the candidate HhalOBPs and OBPs from other Hemipteran species including A. pisum, R. prolixus, T. papillosa, and C. lividipennis (Figure 4). Based on the number of conserved cysteines of each OBP, 12 HhalOBPs (HhalOBP1, 3, 4, 9, 10, 13, 18, 28, 29, 31, 32, and 33) were classified as Plus-C OBPs (Supplementary Figure S1), and the remaining 32 OBPs are classified as classic OBPs (Supplementary Figure S1). Minus-C OBP was not found in H. halys. Moreover, six HhalOBPs (HhalOBP36, 39, 40, 41, 42, and 43) were clustered into the same clade, which may be related to their unique functions.
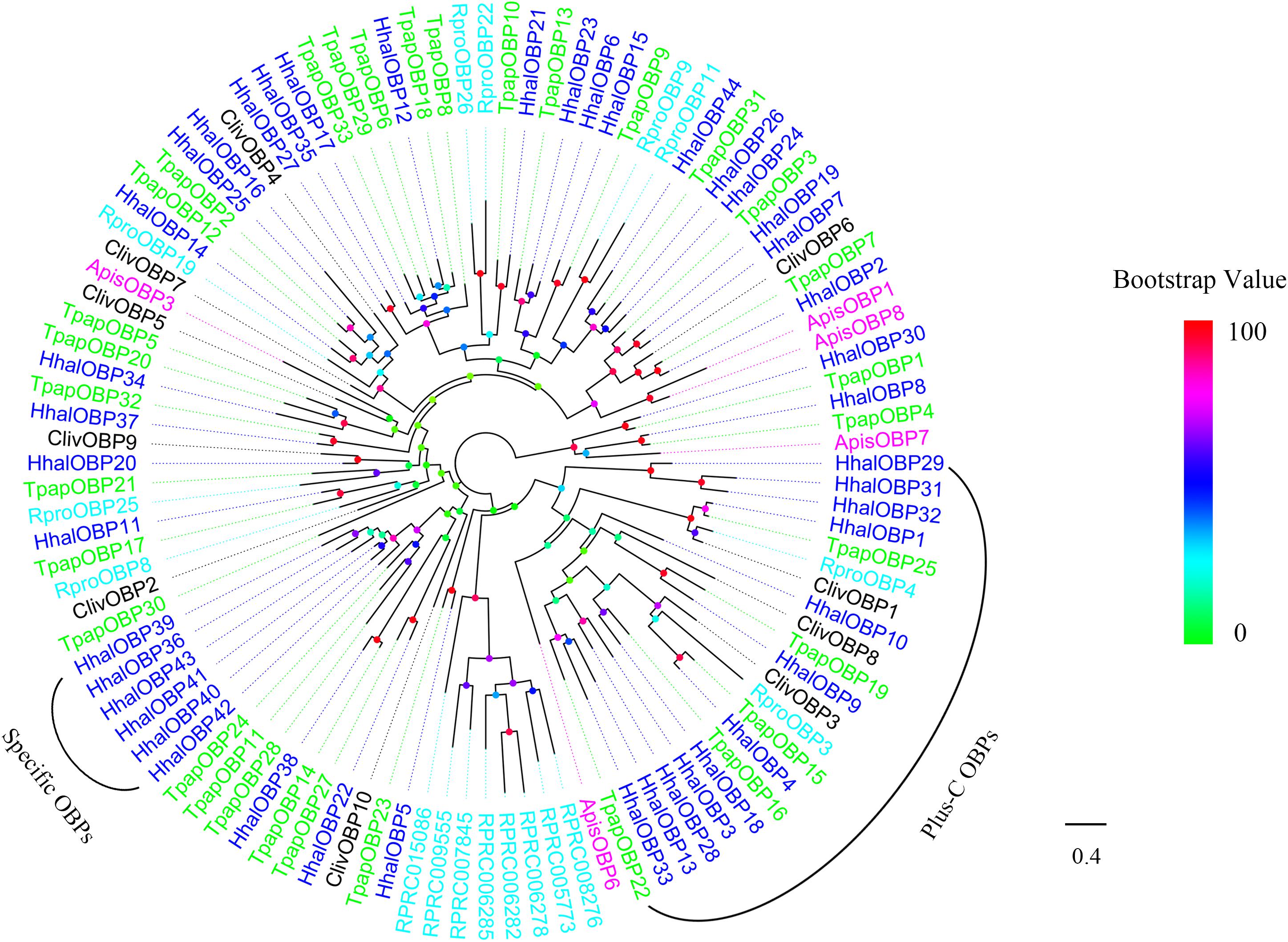
Figure 4. Phylogenetic tree of putative HhalOBPs with known Hemiptera odorant-binding proteins (OBPs). Hhal, Halyomorpha halys (blue); Tpap, Tessaratoma papillosa (green); Rpro, Rhodnius prolixus (cyan); Apis, Acyrthosiphon pisum (violet); Cliv, Cyrtorhinus lividipennis (black). The scale bar equals 0.4 substitutions per sequence position. The bootstrap values are shown in the circles of different colors.
Identification of Candidate Chemosensory Proteins
Seventeen putative unigenes encoding CSPs were identified in the antennal transcriptome of H. halys. Among these unigenes, 15 sequences were predicted to encode full-length putative CSP proteins because they had complete ORFs and four cysteines, a characteristic of typical insect CSPs. The remaining two sequences were incomplete owing to the lack of 5′ or 3′ terminus (Supplementary Table S7). All putative CSPs (HhalCSP1–HhalCSP17) were named as described above. The information including unigene reference, length, and best blastx hit of all putative CSPs is listed in Supplementary Table S7. All of the identified amino acid sequences have the highly conserved four-cysteine profiles (Supplementary Figure S2). The amino acid sequences of the CSPs identified in this study are provided in Supplementary File S1.
A phylogenetic tree was built using all of the putative H. halys CSPs and those from A. gossypii, R. prolixus, and N. lugens (Figure 5). HhalCSP5 clustered with NlugCSP9, HhalCSP9 clustered with RproCSP16, and HhalCSP10 clustered with AgosCSP7. The remaining HhalCSPs did not cluster with CSPs from other Hemiptera species.
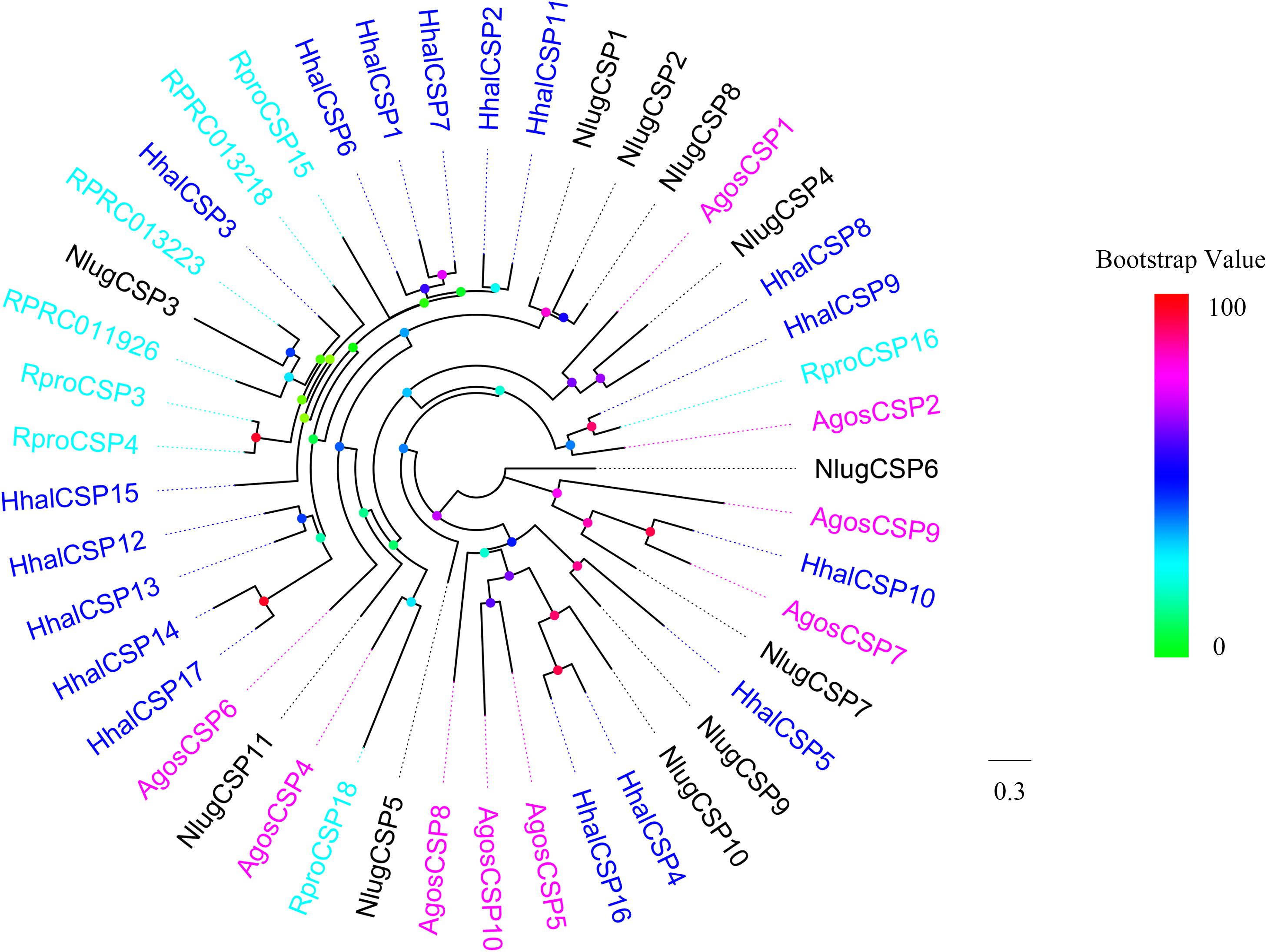
Figure 5. Phylogenetic tree of putative HhalCSPs with known Hemiptera chemosensory proteins (CSPs). Hhal, Halyomorpha halys (blue); Agos, Aphis gossypii (violet); Rpro, Rhodnius prolixus (cyan); Nlug, Nilaparvata lugens (black). The scale bar equals 0.3 substitutions per sequence position. The bootstrap values are shown in the circles of different colors.
Identification of Candidate Sensory Neuron Membrane Proteins
Three unigenes encoding SNMPs (HhalSNMP1.1, HhalSNMP1.2, and HhalSNMP2) were identified in the H. halys transcriptome. Two unigenes were predicted to encode full-length SNMP proteins as they contain complete ORFs that are > 2000 bp long. HhalSNMP1.2 was incomplete owing to a lack of the 5′ terminus. Two HhalSNMP1s (HhalSNMP1.1 and HhalSNMP1.2) were found in our transcriptome with the supporting of blastx results. Information including unigene reference, length, and best blastx hit of all putative SNMPs is listed in Supplementary Table S8. The amino acid sequences of the SNMPs identified in this study are provided in Supplementary File S1.
Tissue- and Sex-Specific Expression of Candidate OBP and CSP Genes in Halyomorpha halys
In order to better understand the role of OBPs and CSPs in the olfactory system in H. halys, we studied the expression patterns of the 44 HhalOBP and 17 HhalCSP genes in antennae, head (without antennae), thorax, abdomen, and legs in adults of both sexes using the semi-quantitative RT-PCR. The results showed that 20 of the 44 HhalOBP genes (including OBP3–5, 8, 11, 13–16, 20, 22, 25, 28, 29, 34, 36–39, and 43) are specifically expressed in male and female antenna (Figure 6). We also found that 13 HhalOBP genes (OBP1, 2, 6, 7, 9, 12, 17, 19, 27, 30, 33, 35, and 40) are highly expressed in the antennae, but they are also weakly or highly expressed in other tissues (Figure 6). Four HhalOBP genes (OBP10, 21, 26, and 32) are poorly expressed in the antennae but were highly expressed in belly tissues of both sexes (Figure 6). Furthermore, HhalOBP10 and HhalOBP40 are only expressed in the male antennae, and no expression is found in the female antennae (Figure 6).
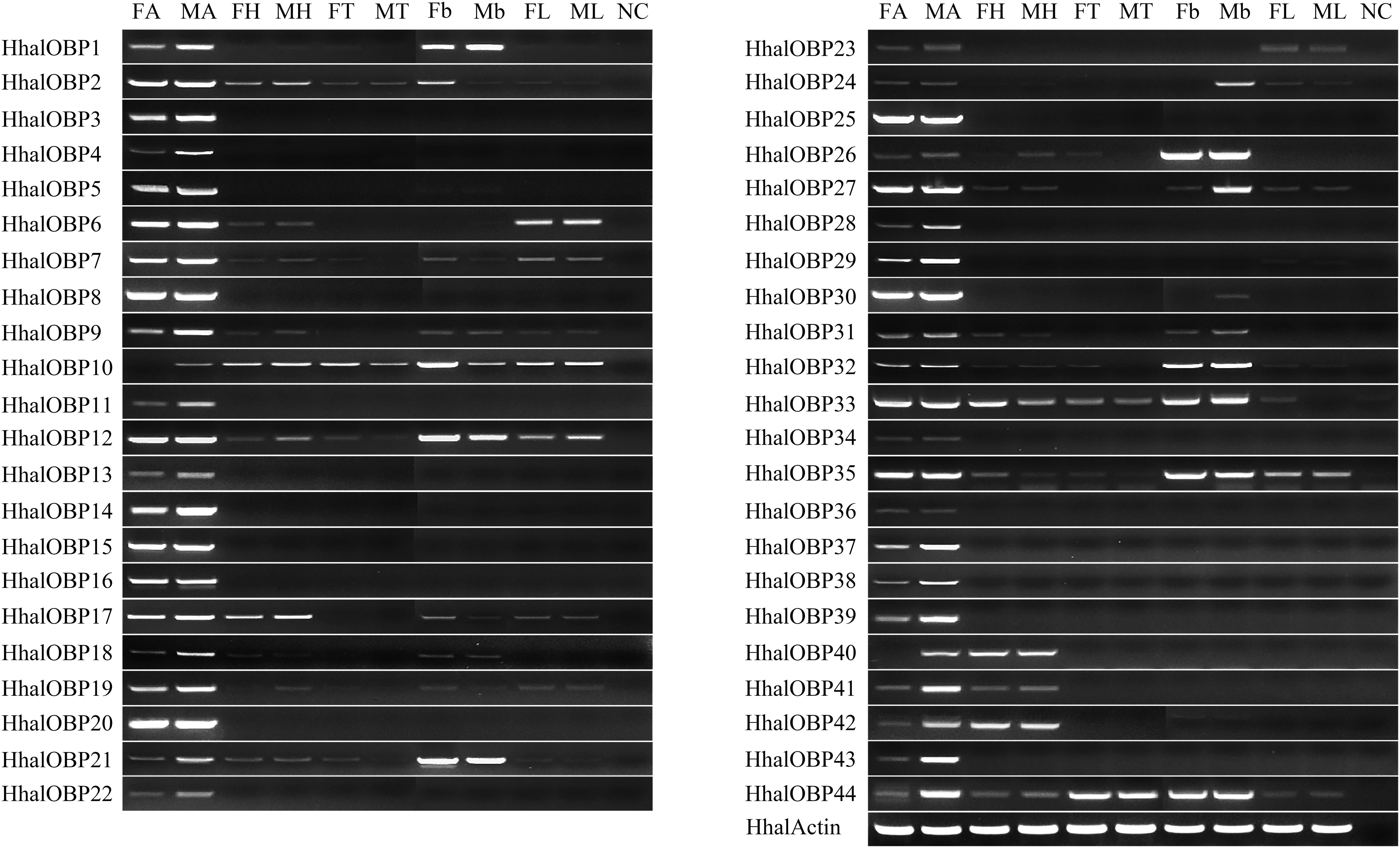
Figure 6. The expression levels of HhalOBP genes in different tissues of Halyomorpha halys as estimated by RT-PCR. FA, female antennae; MA, male antennae; FH, female head; MH, male head; FT, female thorax; MT, male thorax; Fb, female belly; Mb, male belly; FL, female legs; ML, male legs; NC, no template control. HhalActin was used as a reference gene.
As shown in Figure 7, all of the HhalCSP genes are expressed in the antennae of male and female H. halys; HhalCSP1, HhalCSP6–7, and HhalCSP10–12 are expressed in all of these tested tissues; HhalCSP4–5 and HhalCSP13–17 are only expressed in the male and female antennae, not in any other tissues; HhalCSP2 is expressed in the abdomen of both sexes in addition to the antennae; and HhalCSP3 is expressed in all of the tested tissues of both sexes except the thorax. HhalCSP10 is highly expressed in the abdomen of both sexes, and HhalCSP11 is highly expressed in the thorax and abdomen of both sexes of H. halys (Figure 7).
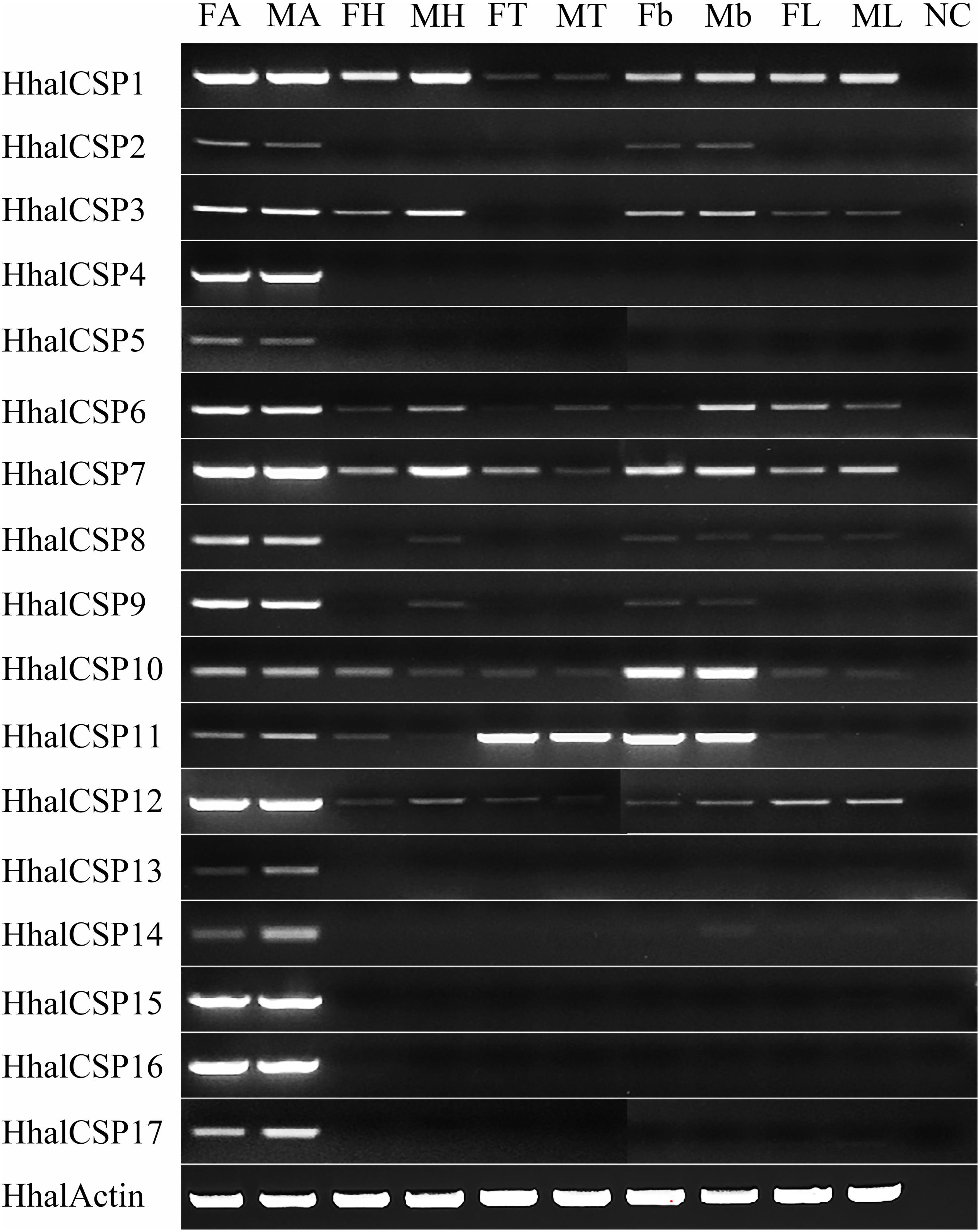
Figure 7. The expression levels of HhalCSP genes in different tissues of Halyomorpha halys as estimated by RT-PCR. FA, female antennae; MA, male antennae; FH, female head; MH, male head; FT, female thorax; MT, male thorax; Fb, female belly; Mb, male belly; FL, female legs; ML, male legs; NC, no template control. HhalActin was used as a reference gene.
Discussion
H. halys is an important agricultural pest of global concern. This invasive pest is native to Asia, but it has been introduced into many countries in Europe, the Americas, and Oceania, where it causes enormous economic losses and is a nuisance to humans, especially during overwintering (Lee et al., 2013). Recent decades have witnessed the rapid development of insect antennal transcriptome studies, but only a few studies have focused on the Hemiptera, especially the Pentatomidae family (stink bugs). Investigations into the mechanisms of olfaction in H. halys will be useful for functional characterization of olfaction genes and could ultimately lead to the identification of new targets for olfactory disruption and development of environmentally friendly pest control strategies. Previously, Paula et al. (2016) identified 30 OBP from H. halys antennae, but they did not examine other olfactory genes. In this study, we reported the sequencing, assembly, and annotation of antennal transcriptomes in H. halys; and we identified 138 ORs, 24 IRs, 15 GRs, 44 OBPs, 17 CSPs, and three SNMPs. We also assayed the expression profiles of 44 OBP and 17 CSP genes in different tissues of H. halys using RT-PCR.
Odorant receptors are crucial to the insect olfactory system, as they determine the sensitivity and specificity of odorant reception (Wang B. et al., 2017). In this study, we identified a total of 138 OR genes in H. halys antennal transcriptomes, which was more than those in O. fasciatus (122) and in T. papillosa (59). These differences in the numbers of identified OR genes could be attributed to the differences in sequencing methods and depth or sample preparation between this study and the other studies. The number of olfactory genes identified here may not reflect all of the olfactory genes in H. halys, because some olfactory genes are expressed in tissues other than antenna. Orco is a highly conserved olfactory co-receptor that plays important roles in insect olfaction and does not function directly in odor recognition but rather forms the obligate co-receptor for all ORs (Larsson et al., 2004; Vosshall and Hansson, 2011). The results of the phylogenetic analysis showed that HhalORs are more closely related to TpapORs than they are to OfasORs, which is consistent with the evolutionary relationship among these three species: H. halys, T. papillosa, and O. fasciatus (Yuan et al., 2015). Although the sequences of insect ORs are highly diverse, we found some ORs from H. halys and T. papillosa that have high sequence similarities. HhalOR45/TpapOR58 and HhalOR89/TpapOR32 share 80.24 and 82.16% sequence similarity, respectively, suggesting that they have some common and possibly identical olfactory functions.
A total of 24 IRs were identified in the antennal transcriptomes of H. halys. This is considerably lower than those numbers found in N. lugens (39), O. fasciatus (37), and R. prolixus (32), but more than those in T. papillosa (12). It is possible that some IR genes are not expressed in antennae or, alternatively, that the number of IRs is species specific and is dependent on natural habitats. Similar to Orco, both IR8a and IR25a are predicted to act as co-receptors present in the IR group because they were co-expressed along with other IRs (Benton et al., 2009; Sheng et al., 2017; Du et al., 2018). TheiGluRs are a highly conserved family of ligand-gated ion channels that mediate chemical communication between neurons at synapses (Croset et al., 2010; Abuin et al., 2011). And in the phylogenetic tree, IR8a and IR25a formed two conserved IR clades.
Members of the GR family of insect chemoreceptors are diverse and demonstrate a broad ligand selectivity for several molecules including receptors for sugars, deterrents, salts, fructose, bitter compounds, CO2, and other compounds (Xu et al., 2012; Agnihotri et al., 2016; Fleischer et al., 2018). This type of receptor plays a critical role in chemo-sensation and influences the insect’s behavior. However, the functions of GRs are largely unknown. In the present study, we identified 15 GRs in the H. halys antennal transcriptome. The number of predicted GRs in H. halys is less than that in N. lugens (28) and R. prolixus (31) and far fewer than the number in O. fasciatus (122). A phylogenetic analysis indicated that HhalGR3, HhalGR5, and HhalGR10–13 may play a role in CO2 detection. HhalGR1 and HhalGR2 are predicted to be candidate sugar receptors that are related to host plant selection and oviposition (Lombarkia and Derridj, 2008; Wang J. et al., 2017).
Odorant-binding proteins are mainly present in insect sensillum lymph, and they can package odor molecules and finally transport them by the sensory lymph to the ORNs around the membrane, activating ORs or IRs (Brito et al., 2016). Among the 44 putative OBPs identified in the present study, 12 HhalOBPs (HhalOBP1, 3, 4, 9, 10, 13, 18, 28, 29, and 31–33) were clustered with the Plus-C OBPs from other insect species. The remaining 32 HhalOBPs were clustered with the classic OBPs, which are characterized by a conserved six-cysteine-residue pattern. In contrast, Paula et al. (2016) identified 30 HhalOBPs from the antennae of 3-day-old H. halys males and females, including 22 classic OBPs and eight plus-C ones. A possible explanation is that these two studies used different sequencing platforms; that is, HiSeq 4000 platform was used in our study, whereas Illumina HiSeq 2500 was used by Paula et al. (2016). The skills for de novo transcriptome assembly and annotation can also result in different numbers of OBPs. Of the 44 OBPs, 20 candidate HhalOBP genes seem to be expressed only in the male and female antennae, suggesting their roles in host plant location. Six HhalOBPs (HhalOBP36 and 39–43) were clustered in one branch, and these OBPs may have similar functions in H. halys. In addition, HhalOBP10 and HhalOBP40 were only found in male antenna, indicating their potential function in finding mates. Thirteen HhalOBP genes were highly expressed in the antennae and were also expressed in other tissues, indicating their involvement in the binding of non-odorant molecules in addition to the recognition process of odorants. HhalOBPs may have functions to bind H. halys pheromones. The expression of 21 HhalOBPs was enhanced in response to alarm pheromone and two HhalOBPs (HhalOBP4 and HhalOBP8) to aggregation pheromone (Paula et al., 2016). We found that HhalOBP8, 16, 25, and 30 were highly expressed in H. halys antennae. Coincidently, these four HhalOBPs had higher binding activities to the major component of the alarm pheromone, (E)-2-decenal, of H. halys (Zhong et al., 2018). The epoxides (3S,6S,7R,10S)-10,11-epoxy-1-bisabolen-3-ol and (3R,6S,7R,10S)-10,11-epoxy-1-bisabolen-3-ol were identified as the main components of the aggregation pheromone of H. halys (Khrimian et al., 2014; Weber et al., 2014). HhalOBP4 and HhalOBP8 may be the proteins that bind the aggregation pheromone of H. halys. However, these assumptions remain to be tested. Our findings, together with those of Paula et al. (2016) and Zhong et al. (2017, 2018), would provide a basis to understand the physiological functions of HhalOBPs and may facilitate to develop approaches for behavioral interference of the pest.
Chemosensory proteins are a class of low-molecular-weight proteins widely found in sensillum lymph, and all of them have a highly conserved four-cysteine profile (Jin et al., 2005). We identified 17 CSPs in the H. halys antennal transcriptome. The number of HhalCSPs is higher than in other Hemiptera species, such as A. gossypii (nine CSPs), N. lugens (11 CSPs), and R. prolixus (eight CSPs). The RT-PCR results showed that HhalCSP4–5, HhalCSP8–9, and HhalCSP13–17 are enriched in the antennae and may be involved in the chemosensory process (Zhang et al., 2014).
There are three SNMPs (SNMP1, SNMP2, and SNMP3) subfamilies identified in insects (Vogt et al., 2009; Zhang et al., 2020). In our study, we found three SNMPs (SNMP1.1, SNMP1.2, and SNMP2) belonging to two subfamilies in the antennal transcriptome of H. halys. SNMP3 was recently identified in Lepidoptera, and it is highly expressed in midguts (Zhang et al., 2020). We failed to identify SNMP3 in our transcriptomic analysis in antenna of H. halys.
The expression of olfactory genes in non-olfactory tissues is very common among insect species (Sheng et al., 2017; Kang et al., 2018). These olfactory genes are assumed to be linked to host plant location or the synthesis of insect pheromone. In the study, we also found that many OBPs and CSPs are highly expressed in non-olfactory tissues. HhalOBP1, HhalOBP24, HhalOBP32, and HhalCSP10 are highly expressed in the abdomen, whereas HhalOBP40–42 are highly expressed in legs, suggesting some special functions of these HhalOBPs (Zhang et al., 2016; Bin et al., 2017; Wang G.Y. et al., 2017).
Conclusion
In summary, we sequenced and annotated the chemosensory gene profiles in the antennal transcriptome of male and female adults of the brown marmorated stink bug, H. halys. A total of 241 chemosensory genes including 138 ORs, 24 IRs, 15 GRs, 44 OBPs, 17 CSPs, and three SNMPs were identified in the antennal transcriptome. We also found tissue-specific expression of HhalOBP1, HhalOBP24, HhalOBP32, HhalOBP40–42, and HhalCSP2, as well as sex-specific expression of HhalOBP10 and HhalOBP40. The huge number of olfactory genes, as well as tissue- and sex-specific expression of some CSPs in H. halys antennal transcriptome, suggests a range of diverse functions of insect antennae, which, to a greater degree, may facilitate the survival of insects in environments full of infochemicals from hosts, mates, and enemies. Data from the present study may also provide a basis for additional insight into olfactory mechanisms at the molecular level and for the development of environmentally friendly management strategies for H. halys in the future.
Data Availability Statement
The clean reads of the two transcriptomes produced in this study are stored in the NCBI SRA database under the accession numbers SRR11748354 (female antennae) and SRR11747758 (male antennae).
Author Contributions
DS, YH, ZQ, and HZ conducted the experiment. DS, YH, ZQ, YL, and SY wrote the manuscript. JZ, YL, and SY conceived the experiment. YL and DS analyzed the data. YH revised the manuscript and interpreted the data. All authors read and approved the final manuscript.
Funding
This project was supported by the National Key R&D Program of China (2017YFD0200400), the National Natural Science Foundation of China (31070338 and 31672095), and the Foundation of Provincial Key Laboratory of Biotic Environment and Ecological Safety in Anhui Province. The funders had no role in the study design, data collection and analysis, decision to publish, or preparation of the manuscript.
Conflict of Interest
The authors declare that the research was conducted in the absence of any commercial or financial relationships that could be construed as a potential conflict of interest.
Supplementary Material
The Supplementary Material for this article can be found online at: https://www.frontiersin.org/articles/10.3389/fphys.2020.00876/full#supplementary-material
FIGURE S1 | Sequence alignment of HhalOBPs. Conserved cysteines of HhalOBPs were marked with “C.” Plus-C OBPs: green; Classic OBPs: blue.
FIGURE S2 | Sequences alignment of HhalCSPs. Conserved cysteines of HhalCSPs were shown by C1–C4 which marked with a black triangle, respectively.
TABLE S1 | Primers for RT-PCR expression analyses of HhalOBP and HhalCSP genes.
TABLE S2 | Assembly summary of Halyomorpha halys transcriptome.
TABLE S3 | Unigenes of candidate olfactory receptors in Halyomorpha halys.
TABLE S4 | Unigenes of candidate ionotropic receptors in Halyomorpha halys.
TABLE S5 | Unigenes of candidate gustatory receptors in Halyomorpha halys.
TABLE S6 | Unigenes of candidate odorant-binding proteins in Halyomorpha halys.
TABLE S7 | Unigenes of candidate chemosensory proteins in Halyomorpha halys.
TABLE S8 | Unigenes of candidate sensory neuron membrane proteins in Halyomorpha halys.
FILE S1 | The amino acid sequences of the chemosensory proteins identified in this study.
Footnotes
- ^ http://web.expasy.org/translate/
- ^ http://www.cbs.dtu.dk/services/SignalP/
- ^ http://www.cbs.dtu.dk/services/TMHMM/
- ^ https://www.ebi.ac.uk/Tools/msa/mafft/
References
Abuin, L., Bargeton, B., Ulbrich, M. H., Isacoff, E. Y., Kellenberger, S., and Benton, R. (2011). Functional architecture of olfactory ionotropic glutamate receptors. Neuron 69, 44–60. doi: 10.1016/j.neuron.2010.11.042
Acebes-Doria, A. L., Morrison, W. R. III, Short, B. D., Rice, K. B., Bush, H. G., Kuhar, T. P., et al. (2018). Monitoring and biosurveillance tools for the brown marmorated stink bug, Halyomorpha halys (Stal) (Hemiptera: Pentatomidae). Insects 9:82. doi: 10.3390/insects9030082
Agnihotri, A. R., Roy, A. A., and Joshi, R. S. (2016). Gustatory receptors in Lepidoptera: chemosensation and beyond. Insect. Mol. Biol. 25, 519–529. doi: 10.1111/imb.12246
Anderson, B. E., Miller, J. J., and Adams, D. R. (2012). Irritant contact dermatitis to the brown marmorated stink bug, Halyomorpha halys. Dermatitis 23, 170–172. doi: 10.1097/DER.0b013e318260d7be
Anton, S., Van Loon, J. J., Meijerink, J., Smid, H. M., Takken, W., and Rospars, J. P. (2003). Central projections of olfactory receptor neurons from single antennal and palpal sensilla in mosquitoes. Arthropod. Struct. Dev. 32, 319–327. doi: 10.1016/j.asd.2003.09.002
Asahina, K., Pavlenkovich, V., and Vosshall, L. B. (2008). The survival advantage of olfaction in a competitive environment. Curr. Biol. 18, 1153–1155. doi: 10.1016/j.cub.2008.06.075
Benton, R., Sachse, S., Michnick, S. W., and Vosshall, L. B. (2006). Atypical membrane topology and heteromeric function of Drosophila odorant receptors in vivo. PLoS Biol. 4:e20. doi: 10.1371/journal.pbio.0040020
Benton, R., Vannice, K. S., Gomez-Diaz, C., and Vosshall, L. B. (2009). Variant ionotropic glutamate receptors as chemosensory receptors in Drosophila. Cell 136, 149–162. doi: 10.1016/j.cell.2008.12.001
Bergmann, E. J., Venugopal, P. D., Martinson, H. M., Raupp, M. J., and Shrewsbury, P. M. (2016). Host plant use by the invasive Halyomorpha halys (Stal) on woody ornamental trees and shrubs. PLoS One 11:e0149975. doi: 10.1371/journal.pone.0149975
Bin, S. Y., Qu, M. Q., Pu, X. H., Wu, Z. Z., and Lin, J. T. (2017). Antennal transcriptome and expression analyses of olfactory genes in the sweetpotato weevil Cylas formicarius. Sci. Rep. 7:11073. doi: 10.1038/S41598-017-11456-X
Botch, P. S., and Delfosse, E. S. (2018). Host-acceptance behavior of trissolcus japonicus (hymenoptera: scelionidae) reared on the invasive Halyomorpha halys (Heteroptera: Pentatomidae) and nontarget species. Environ. Entomol. 47, 403–411. doi: 10.1093/ee/nvy014
Brito, N. F., Moreira, M. F., and Melo, A. C. A. (2016). A look inside odorant-binding proteins in insect chemoreception. J. Insect. Physiol. 95, 51–65. doi: 10.1016/j.jinsphys.2016.09.008
Bruce, T. J. A., Wadhams, L. J., and Woodcock, C. M. (2005). Insect host location: a volatile situation. Trends Plant Sci. 10, 269–274. doi: 10.1016/j.tplants.2005.04.003
Chen, C., Buhl, E., Xu, M., Croset, V., Rees, J. S., Lilley, K. S., et al. (2015). Drosophila Ionotropic Receptor 25a mediates circadian clock resetting by temperature. Nature 527, 516–520. doi: 10.1038/nature16148
Clyne, P. J., Warr, C. G., Freeman, M. R., Lessing, D., Kim, J., and Carlson, J. R. (1999). A novel family of divergent seven-transmembrane proteins: candidate odorant receptors in Drosophila. Neuron 22, 327–338. doi: 10.1016/S0896-6273(00)81093-4
Croset, V., Cummins, S. F., and Benton, R. (2010). Ancient protostome origin of chemosensory ionotropic glutamate receptors and the evolution of insect taste and olfaction. J. Neurogenet. 24, 30–31. doi: 10.1371/journal.pgen.1001064
Dahanukar, A., Hallem, E. A., and Carlson, J. R. (2005). Insect chemoreception. Curr. Opin. Neurobiol. 15, 423–430. doi: 10.1016/j.conb.2005.06.001
Dahanukar, A., Lei, Y.-T., Kwon, J. Y., and Carlson, A. J. R. (2007). Two Gr genes underlie sugar reception in Drosophila. Neuron 56, 503–516. doi: 10.1016/j.neuron.2007.10.024
De Biasio, F., Riviello, L., Bruno, D., Grimaldi, A., Congiu, T., Sun, Y. F., et al. (2015). Expression pattern analysis of odorant-binding proteins in the pea aphid Acyrthosiphon pisum. Insect. Sci. 22, 220–234. doi: 10.1111/1744-7917.12118
Du, L. X., Liu, Y., Zhang, J., Gao, X. W., Wang, B., and Wang, G. R. (2018). Identification and characterization of chemosensory genes in the antennal transcriptome of Spodoptera exigua. Comp. Biochem. Physiol. D 27, 54–65. doi: 10.1016/j.cbd.2018.05.001
Dunipace, L., Meister, S., McNealy, C., and Amrein, H. (2001). Spatially restricted expression of candidate taste receptors in the Drosophila gustatory system. Curr. Biol. 11, 822–835. doi: 10.1016/s0960-9822(01)00258-5
Field, L. M., Pickett, J. A., and Wadhams, L. J. (2000). Molecular studies in insect olfaction. Insect. Mol. Biol. 9, 545–551. doi: 10.1046/j.1365-2583.2000.00221.x
Fleischer, J., Pregitzer, P., Breer, H., and Krieger, J. (2018). Access to the odor world: olfactory receptors and their role for signal transduction in insects. Cell Mol. Life. Sci. 75, 485–508. doi: 10.1007/s00018-017-2627-5
Gomez-Diaz, C., Bargeton, B., Abuin, L., Bukar, N., Reina, J. H., Bartoi, T., et al. (2016). A CD36 ectodomain mediates insect pheromone detection via a putative tunnelling mechanism. Nat. Commun. 7:11866. doi: 10.1038/ncomms11866
Grabherr, M. G., Haas, B. J., Yassour, M., Levin, J. Z., and Thompson, D. A. (2011). Full-length transcriptome assembly from RNA-Seq data without a reference genome. Nat. Biotechnol. 29, 644–652. doi: 10.1038/nbt.1883
Gu, S. H., Wu, K. M., Guo, Y. Y., Field, L. M., Pickett, J. A., Zhang, Y. J., et al. (2013a). Identification and expression profiling of odorant binding proteins and chemosensory proteins between two wingless morphs and a winged morph of the cotton aphid Aphis gossypii glover. PLoS One 8:e73524. doi: 10.1371/journal.pone.0073524
Gu, S. H., Yang, R. N., Guo, M. B., Wang, G. R., Wu, K. M., Guo, Y. Y., et al. (2013b). Molecular identification and differential expression of sensory neuron membrane proteins in the antennae of the black cutworm moth Agrotis ipsilon. J. Insect Physiol. 59, 430–443. doi: 10.1016/j.jinsphys.2013.02.003
Hansson, B. S. (2002). A bug’s smell–research into insect olfaction. Trends Neurosci. 25, 270–274. doi: 10.1016/S0166-2236(02)02140-9
He, P., Engsontia, P., Chen, G. L., Yin, Q., Wang, J., Lu, X., et al. (2018). Molecular characterization and evolution of a chemosensory receptor gene family in three notorious rice planthoppers, Nilaparvata lugens, Sogatella furcifera and Laodelphax striatellus, based on genome and transcriptome analyses. Pest Manag Sci. 15:4912. doi: 10.1002/ps.4912
Ioannidis, P., Lu, Y., Kumar, N., Creasy, T., Daugherty, S., Chibucos, M. C., et al. (2014). Rapid transcriptome sequencing of an invasive pest, the brown marmorated stink bug Halyomorpha halys. BMC Genomics 15:738. doi: 10.1186/1471-2164-15-738
Jiang, X., Pregitzer, P., Grosse-Wilde, E., Breer, H., and Krieger, J. (2016). Identification and characterization of two “Sensory Neuron Membrane Proteins” (SNMPs) of the desert locust, Schistocerca gregaria (Orthoptera: Acrididae). J. Insect. Sci. 16:33. doi: 10.1093/jisesa/iew015
Jin, X., Brandazza, A., Navarrini, A., Ban, L., Zhang, S., Steinbrecht, R. A., et al. (2005). Expression and immunolocalisation of odorant-binding and chemosensory proteins in locusts. Cell Mol. Life Sci. 62, 1156–1166. doi: 10.1007/s00018-005-5014-6
Jin, X., Ha, T. S., and Smith, D. P. (2008). SNMP is a signaling component required for pheromone sensitivity in Drosophila. Proc. Natl. Acad. Sci. U.S.A. 105, 10996–11001. doi: 10.1073/pnas.0803309105
Jin, X., Zhang, S. G., and Zhang, L. (2006). Expression of odorant-binding and chemosensory proteins and spatial map of chemosensilla on labial palps of Locusta migratoria (Orthoptera: Acrididae). Arthropod. Struct. Dev. 35, 47–56. doi: 10.1016/j.asd.2005.11.001
Kang, Z. W., Liu, F. H., Pang, R. P., Yu, W. B., Tan, X. L., Zheng, Z. Q., et al. (2018). The identification and expression analysis of candidate chemosensory genes in the bird cherry-oat aphid Rhopalosiphum padi (L.). Bull. Entomol. Res. 108, 645–657. doi: 10.1017/S0007485317001171
Kent, L. B., and Robertson, H. M. (2009). Evolution of the sugar receptors in insects. BMC Evol. Biol. 9:41. doi: 10.1186/1471-2148-9-41
Khrimian, A., Zhang, A., Weber, D. C., Ho, H. Y., Aldrich, J. R., Vermillion, K. E., et al. (2014). Discovery of the aggregation pheromone of the brown marmorated stink bug (Halyomorpha halys) through the creation of stereoisomeric libraries of 1-bisabolen-3-ols. J. Nat Prod. 77, 1708–1717. doi: 10.1021/np5003753
Koh, T. W., He, Z., Gorur-Shandilya, S., Menuz, K., Larter, N. K., Stewart, S., et al. (2014). The Drosophila IR20a clade of ionotropic receptors are candidate taste and pheromone receptors. Neuron 83, 850–865. doi: 10.1016/j.neuron.2014.07.012
Kwon, J. Y., Dahanukar, A., Weiss, L. A., and Carlson, J. R. (2007). The molecular basis of CO2 reception in Drosophila. Proc. Natl. Acad. Sci. U.S.A. 104, 3574–3578. doi: 10.1073/pnas.0700079104
Larsson, M. C., Domingos, A. I., Jones, W. D., Chiappe, M. E., Amrein, H., and Vosshall, L. B. (2004). Or83b encodes a broadly expressed odorant receptor essential for Drosophila olfaction. Neuron 43, 703–714. doi: 10.1016/j.neuron.2004.08.019
Leal, W. S. (2013). Odorant reception in insects: roles of receptors, binding proteins, and degrading enzymes. Annu. Rev. Entomol. 58, 373–391. doi: 10.1146/annurev-ento-120811-153635
Lee, D. H., Short, B. D., Joseph, S. V., Bergh, J. C., and Leskey, T. C. (2013). Review of the biology, ecology, and management of Halyomorpha halys (Hemiptera: Pentatomidae) in China, Japan, and the Republic of Korea. Environ. Entomol. 42, 627–641. doi: 10.1603/EN13006
Lombarkia, N., and Derridj, S. (2008). Resistance of apple trees to Cydia pomonella egg-laying due to leaf surface metabolites. Entomol. Exp. Appl. 128, 57–65. doi: 10.1111/j.1570-7458.2008.00741.x
Mang, D., Shu, M., Tanaka, S., Nagata, S., Takada, T., Endo, H., et al. (2016). Expression of the fructose receptor BmGr9 and its involvement in the promotion of feeding, suggested by its co-expression with neuropeptide F1 in Bombyx mori. Insect. Biochem. Molec. 75, 58–69. doi: 10.1016/j.ibmb.2016.06.001
Mesquita, R. D., Vionette-Amaral, R. J., Lowenberger, C., Rivera-Pomar, R., Monteiro, F. A., and Minx, P. (2015). Genome of Rhodnius prolixus, an insect vector of Chagas disease, reveals unique adaptations to hematophagy and parasite infection. Proc. Natl. Acad. Sci. U.S.A. 112, 14936–14941. doi: 10.1073/pnas.1506226112
Nichols, Z., and Vogt, R. G. (2008). The SNMP/CD36 gene family in Diptera, Hymenoptera and Coleoptera: Drosophila melanogaster, D. pseudoobscura, Anopheles gambiae, Aedes aegypti, Apis mellifera, and Tribolium castaneum. Insect. Biochem. Molec. 38, 398–415. doi: 10.1016/j.ibmb.2007.11.003
Panfilio, K. A., Vargas Jentzsch, I. M., Benoit, J. B., Erezyilmaz, D., Suzuki, Y., Colella, S., et al. (2019). Molecular evolutionary trends and feeding ecology diversification in the Hemiptera, anchored by the milkweed bug genome. Genome Biol. 20:64. doi: 10.1186/s13059-019-1660-0
Paula, D. P., Togawa, R. C., Costa, M. M., Grynberg, P., Martins, N. F., and Andow, D. A. (2016). Identification and expression profile of odorant-binding proteins in Halyomorpha halys (Hemiptera: Pentatomidae). Insect. Mol. Biol. 25, 580–594. doi: 10.1111/imb.12243
Pertea, G., Huang, X., Liang, F., Antonescu, V., Sultana, R., Karamycheva, S., et al. (2003). TIGR Gene Indices clustering tools (TGICL): a software system for fast clustering of large EST datasets. Bioinformatics 19, 651–652. doi: 10.1093/bioinformatics/btg034
Petersen, T. N., Brunak, S., von Heijne, G., and Nielsen, H. (2011). SignalP 4.0: discriminating signal peptides from transmembrane regions. Nat. Methods 8, 785–786. doi: 10.1038/nmeth.1701
Rogers, M. E., Krieger, J., and Vogt, R. G. (2001). Antennal SNMPs (sensory neuron membrane proteins) of Lepidoptera define a unique family of invertebrate CD36-like proteins. J. Neurobiol. 49, 47–61. doi: 10.1002/neu.1065
Rospars, J. P., Gu, Y., Gremiaux, A., and Lucas, P. (2010). Odour transduction in olfactory receptor neurons. Chinese J. Physiol. 53, 364–372. doi: 10.4077/CJP.2010.AMM038
Rytz, R., Croset, V., and Benton, R. (2013). Ionotropic receptors (IRs): chemosensory ionotropic glutamate receptors in Drosophila and beyond. Insect. Biochem. Molec. 43, 888–897. doi: 10.1016/j.ibmb.2013.02.007
Sato, K., Pellegrino, M., Nakagawa, T., Nakagawa, T., Vosshall, L. B., and Touhara, K. (2008). Insect olfactory receptors are heteromeric ligand-gated ion channels. Nature 452, 1002–1006. doi: 10.1038/nature06850
Sato, K., and Touhara, K. (2009). Insect olfaction: receptors, signal transduction, and behavior. Results Probl. Cell Differ. 47, 121–138. doi: 10.1007/400_2008_10
Shen, Y. S., and Hu, C. C. (2017). Irritant contact keratitis caused by the bodily fluids of a brown marmorated stink bug. Taiwan J. Ophthalmol. 7, 221–223. doi: 10.4103/tjo.tjo_32_17
Sheng, S., Liao, C. W., Zheng, Y., Zhou, Y., Xu, Y., Song, W. M., et al. (2017). Candidate chemosensory genes identified in the endoparasitoid Meteorus pulchricornis (Hymenoptera: Braconidae) by antennal transcriptome analysis. Comp. Biochem. Physiol. D 22, 20–31. doi: 10.1016/j.cbd.2017.01.002
Smart, R., Kiely, A., Beale, M., Vargas, E., Carraher, C., Kralicek, A. V., et al. (2008). Drosophila odorant receptors are novel seven transmembrane domain proteins that can signal independently of heterotrimeric G proteins. Insect. Biochem. Molec. 38, 770–780. doi: 10.1016/j.ibmb.2008.05.002
Sparks, M. E., Shelby, K. S., Kuhar, D., and Gundersen-Rindal, D. E. (2014). Transcriptome of the invasive brown marmorated stink bug, Halyomorpha halys (Stal) (Heteroptera: Pentatomidae). PLoS One 9:e111646. doi: 10.1371/journal.pone.0111646
Stamatakis, A. (2014). RAxML version 8: a tool for phylogenetic analysis and post-analysis of large phylogenies. Bioinformatics 30, 1312–1313. doi: 10.1093/bioinformatics/btu033
Su, C. Y., Menuz, K., and Carlson, J. R. (2009). Olfactory perception: receptors, cells, and circuits. Cell 139, 45–59. doi: 10.1016/j.cell.2009.09.015
Sun, X., Zeng, F. F., Yan, M. J., Zhang, A., Lu, Z. X., and Wang, M. Q. (2016). Interactions of two odorant-binding proteins influence insect chemoreception. Insect. Mol. Biol. 25, 712–723. doi: 10.1111/imb.12256
Vogt, R. G., Miller, N. E., Litvack, R., Fandino, R. A., Sparks, J., Staples, J., et al. (2009). The insect SNMP gene family. Insect. Biochem. Molec. 39, 448–456. doi: 10.1016/j.ibmb.2009.03.007
Vosshall, L. B., and Hansson, B. S. (2011). A unified nomenclature system for the insect olfactory coreceptor. Chem. Sens. 36, 497–498. doi: 10.1093/chemse/bjr022
Wang, B., Liu, Y., and Wang, G. R. (2017). Chemosensory genes in the antennal transcriptome of two syrphid species, Episyrphus balteatus and Eupeodes corollae (Diptera: Syrphidae). BMC Genomics 18:586. doi: 10.1186/s12864-017-3939-4
Wang, G. Y., Zhu, M. F., Jiang, Y. D., Zhou, W. W., Liu, S., Heong, K. L., et al. (2017). Identification of candidate odorant-binding protein and chemosensory protein genes in Cyrtorhinus lividipennis (Hemiptera: Miridae), a key predator of the rice planthoppers in Asia. Environ. Entomol. 46, 654–662. doi: 10.1093/ee/nvx075
Wang, J., Hu, P., Gao, P., Tao, J., and Luo, Y. (2017). Antennal transcriptome analysis and expression profiles of olfactory genes in Anoplophora chinensis. Sci. Rep. 7:15470. doi: 10.1038/s41598-017-15425-2
Wanner, K. W., and Robertson, H. M. (2008). The gustatory receptor family in the silkworm moth Bombyx mori is characterized by a large expansion of a single lineage of putative bitter receptors. Insect. Mol. Biol. 17, 621–629. doi: 10.1111/j.1365-2583.2008.00836.x
Weber, D. C., Leskey, T. C., Walsh, G. C., and Khrimian, A. (2014). Synergy of aggregation pheromone with methyl (E,E,Z) -2,4,6-decatrienoate in attraction of Halyomorpha halys (Hemiptera: Pentatomidae). J. Econ. Entomol. 107, 1061–1068. doi: 10.1603/ec13502
Wicher, D. (2015). Olfactory signaling in insects. Prog. Mol. Biol. Transl. 130, 37–54. doi: 10.1016/j.cois.2014.10.006
Wu, Z. Z., Qu, M. Q., Pu, X. H., Cui, Y., Xiao, W. Y., Zhao, H. X., et al. (2017). Transcriptome sequencing of Tessaratoma papillosa antennae to identify and analyze expression patterns of putative olfaction genes. Sci. Rep. 7:3070. doi: 10.1038/s41598-017-03306-7
Xu, W., Zhang, H. J., and Anderson, A. (2012). A sugar gustatory receptor identified from the foregut of cotton bollworm Helicoverpa armigera. J. Chem. Ecol. 38, 1513–1520. doi: 10.1007/s10886-012-0221-8
Xue, W., Fan, J., Zhang, Y., Xu, Q., Han, Z., Sun, J., et al. (2016). Identification and expression analysis of candidate odorant-binding protein and chemosensory protein genes by antennal transcriptome of Sitobion avenae. PLoS One 11:e0161839. doi: 10.1371/journal.pone.0161839
Yang, S. Y., Cao, D. P., Wang, G. R., and Liu, Y. (2017). Identification of genes involved in chemoreception in Plutella xyllostella by antennal transcriptome analysis. Sci. Rep. 7:11941. doi: 10.1038/S41598-017-11646-7
Yuan, M. L., Zhang, Q. L., Guo, Z. L., Wang, J., and Shen, Y. Y. (2015). Comparative mitogenomic analysis of the superfamily Pentatomoidea (Insecta: Hemiptera: Heteroptera) and phylogenetic implications. BMC Genomics 16:460. doi: 10.1186/s12864-015-1679-x
Zhang, H. J., Xu, W., Chen, Q. M., Sun, L. N., Anderson, A., Xia, Q. Y., et al. (2020). A phylogenomics approach to characterizing sensory neuron membrane proteins (SNMPs) in Lepidoptera. Insect. Biochem. Molec. 118:103313. doi: 10.1016/j.ibmb.2020.103313
Zhang, J., Bisch-Knaden, S., Fandino, R. A., Yan, S., Obiero, G. F., Grosse-Wilde, E., et al. (2019). The olfactory coreceptor IR8a governs larval feces-mediated competition avoidance in a hawkmoth. Proc. Natl. Acad. Sci. U.S.A. 116, 21828–21833. doi: 10.1073/pnas.1913485116
Zhang, L. W., Kang, K., Jiang, S. C., Zhang, Y. N., Wang, T. T., Zhang, J., et al. (2016). Analysis of the antennal transcriptome and insights into olfactory genes in Hyphantria cunea (Drury). PLoS One 11:e0164729. doi: 10.1371/journal.pone.01647
Zhang, Y. N., Ye, Z. F., Yang, K., and Dong, S. L. (2014). Antenna-predominant and male-biased CSP19 of Sesamia inferens is able to bind the female sex pheromones and host plant volatiles. Gene 536, 279–286. doi: 10.1016/j.gene.2013.12.011
Zhong, Y. Z., Tang, R., Zhang, J. P., Yang, S. Y., Chen, G. H., He, K. L., et al. (2018). Behavioral evidence and olfactory reception of a single alarm pheromone component in Halyomorpha halys. Front. Physiol. 9:1610. doi: 10.3389/fphys.2018.01610
Keywords: Halyomorpha halys, antennal transcriptome, chemosensory genes, expression patterns, odorant-binding protein, chemosensory protein
Citation: Sun D, Huang Y, Qin Z, Zhan H, Zhang J, Liu Y and Yang S (2020) Identification of Candidate Olfactory Genes in the Antennal Transcriptome of the Stink Bug Halyomorpha halys. Front. Physiol. 11:876. doi: 10.3389/fphys.2020.00876
Received: 29 February 2020; Accepted: 29 June 2020;
Published: 24 July 2020.
Edited by:
Sylvia Anton, Institut National de la Recherche Agronomique (INRA), FranceReviewed by:
Alisha Anderson, Commonwealth Scientific and Industrial Research Organisation (CSIRO), AustraliaWilliam Benjamin Walker III, Swedish University of Agricultural Sciences, Sweden
Copyright © 2020 Sun, Huang, Qin, Zhan, Zhang, Liu and Yang. This is an open-access article distributed under the terms of the Creative Commons Attribution License (CC BY). The use, distribution or reproduction in other forums is permitted, provided the original author(s) and the copyright owner(s) are credited and that the original publication in this journal is cited, in accordance with accepted academic practice. No use, distribution or reproduction is permitted which does not comply with these terms.
*Correspondence: Yang Liu, eWFuZ2xpdUBpcHBjYWFzLmNu; Shiyong Yang, c2hpeWFuQGFobnUuZWR1LmNu