- 1Institute of Cardiovascular Diseases of PLA, The Second Affiliated Hospital, Third Military Medical University (Army Medical University), Chongqing, China
- 2Department of Cardiology, The Second Affiliated Hospital, Third Military Medical University (Army Medical University), Chongqing, China
- 3Department of Geriatric Cardiology, Chinese PLA General Hospital, Beijing, China
- 4Department of Medical Ultrasonics, The Second Affiliated Hospital, Third Military Medical University (Army Medical University), Chongqing, China
The aims of this study were to explore the effect of high-altitude (HA) exposure on the incidence, determinants, and impacts of right ventricular dyssynchrony (RVD). In our study, 108 healthy young men were enrolled, and physiological and echocardiographic variables were recorded at both sea level and 4,100 m. By using two-dimensional speckle-tracking echocardiography, RVD was evaluated by calculating the R–R interval-corrected standard deviation of the time-to-peak systolic strain for the four mid-basal RV segments (RVSD4) and defined by RVSD4 > 18.7 ms. After HA exposure, RVSD4 was significantly increased, and the incidence of RVD was approximately 32.4%. Subjects with RVD showed lower oxygen saturation (SaO2) and RV global longitudinal strain and higher systolic pulmonary artery pressure than those without RVD. Moreover, myocardial acceleration during isovolumic contraction was increased in all subjects and those without RVD, but not in those with RVD. Multivariate logistic regression revealed that SaO2 is an independent determinant of RVD at HA (odds ratio: 0.72, 95% CI: 0.56–0.92; P = 0.009). However, the mean pulmonary artery pressure was linearly correlated with the magnitude of RVD in the presence of Notch. No changes were found in RV fractional area change, tricuspid annular motion, or tricuspid s’ velocity between subjects with and without RVD. Collectively, we demonstrated for the first time that HA exposure could induce RVD in healthy subjects, which may be mainly attributed to the decline in SaO2 as well as RV overload; the incidence of RVD was associated with reduced RV regional function and blunted myocardial acceleration.
Introduction
Traveling to a high altitude (HA) for sports, work, or recreational purposes is becoming popular but poses some physiological challenges to the cardiovascular system, leading to hypoxic pulmonary vasoconstriction, pulmonary vascular remodeling, and increases in pulmonary artery pressure (PAP) (Xu and Jing, 2009). In turn, elevated right ventricular (RV) afterload may predispose the RV to remodeling, hypertrophy, or even failure if HA hypoxia is sustained (Penaloza and Arias-Stella, 2007). Although it has been proposed that RV systolic function is well preserved and that its diastolic function is sometimes altered under acute HA hypoxia (Naeije, 2010), exposure to HA at 8,000 m or acute hypoxia results in RV dilatation, an increased RV Tei index, and a decreased RV free wall longitudinal systolic strain (Kurdziel et al., 2017; Netzer et al., 2017). Moreover, significant decreases in tricuspid annular plane systolic excursion (TAPSE) and RV global longitudinal strain (RVGLS) have been found in healthy lowlanders after ascending to an HA of 5,050 m, suggesting that RV longitudinal systolic function is impaired (Stembridge et al., 2014). Therefore, although elevated PAP and HA hypoxia may potentially threaten RV performance, the effects of acute HA exposure on RV global or regional functions are controversial and inconclusive.
Evaluation of RV global or regional function at HA is of considerable importance because RV function (such as the Tei index) and elevated PAP are associated with impaired exercise capacity (Naeije et al., 2010; Yang et al., 2015). Moreover, a previous report showed that subjects with previous HA pulmonary edema developed HA right-heart failure with severe tricuspid regurgitation (TR), dilatation of the RV chamber, and RV postsystolic shortening (Huez et al., 2007). The latter reflects RV dyssynchrony (RVD), which was calculated by the R–R interval-corrected standard deviation (SD) of the time-to-peak systolic strain for the four mid-basal RV segments (RVSD4) as detected by speckle-tracking echocardiography (STE). The presence of RVD usually suggests the variability of time differences in regional contraction through RV strain curves and is a more accurate estimate of RV function than the tissue Doppler method (Murata et al., 2017). In patients with pulmonary hypertension (PH), the presence of RVD is always associated with the symptomatology, functional state, exercise performance, and clinical outcomes (Murata et al., 2017; Rehman et al., 2018). Recent evidence also showed that RVD occurred during hypoxia but not during exercise, suggesting the combined contributions of mechanical (RV afterload) and systemic (hypoxia) factors (Pezzuto et al., 2018). Based on these reasons, we hypothesize that RVD is present under acute HA exposure and can be explained by HA hypoxia or increased RV afterload.
We therefore conducted the present study to determine whether acute HA exposure can induce regional heterogeneity of RV contraction or RVD and to explore the determinants and impacts.
Materials and Methods
Participants and Study Procedures
Our research was approved by the Clinical Research Ethics Board of the Third Military Medical University (Army Medical University) (No. 2012015), was registered with the Center of Chinese Clinical Trial Registration (No. ChiCTR-RCS-12002232)1, and complied with the Declaration of Helsinki. Informed consent was obtained from each participant in our study. Young Chinese male soldiers who permanently lived in lowland areas were enrolled in 2013 at sea level (SL) (<500 m) in Chongqing and experienced a stair-type ascension to 4,100 m (Litang, Sichuan, China) from 400 m (Yanggongqiao, Chongqing, China) by bus within 7 days. Data collection was performed at SL and 5 ± 2 h after arrival at 4,100 m. Exclusion criteria were known cardiovascular and pulmonary diseases, any cardiovascular and pulmonary therapy, hematologic diseases, malignant tumors, liver or kidney dysfunction, history of HA-related diseases, or exposure to 2,500 m above SL in the past 6 months.
Echocardiographic Assessments
An echocardiographic study was performed using a CX50 ultrasound system (Philips Ultrasound System, Andover, MA, United States) equipped with a 2.5-MHz adult transducer by registered sonographers according to a standardized protocol (Lang et al., 2015). Standard two-dimensional (2D) and Doppler images were obtained in the left lateral decubitus position after 10 min of rest, stored for three consecutive beats, and then analyzed off-line on a digital image analysis system (QLAB 10.5, Philips Healthcare, Andover, MA, United States) by two independent investigators in a blind fashion.
The following parameters were analyzed or calculated: right atrial (RA) area, RV end-diastolic area (RVEDA), RV end-systolic area (RVESA), and RV fractional area change [RV FAC, (RVEDA − RVESA)/RVEDA × 100]. Pulsed Doppler RV inflow recordings were performed in the apical four-chamber view. The peak early (E) and late (A) diastolic flow velocities were measured, and the E/A ratio was calculated from the transtricuspid inflow. From a standard apical four-chamber window, tricuspid annular motion (TAM) was measured to evaluate RV longitudinal function according to guidelines from the American Society of Echocardiography (Rudski et al., 2010).
Pulsed-wave Doppler recordings of pulmonary flow were performed from a modified basal short-axis view. Peak pulmonary artery velocity (PAV), the time of the pre-ejection period (PEP), pulmonary artery acceleration time (PAAT), pulmonary artery ejection time (PAET), and the presence of Notch were measured accordingly (Vonk Noordegraaf et al., 2015). The mean PAP (mPAP) was calculated from PAAT because it was present in all subjects; moreover, it is closely correlated with and substantially more feasible to measure than the maximal velocity of the TR jet (TRV) for the estimation of PAP: when PAAT was more than 120 ms, mPAP = 79 − (0.45 × AT), and when PAAT was less than 120 ms, mPAP = 90 − (0.62 × AT) (Wierzbowska-Drabik et al., 2019). Systolic PAP (SPAP) was calculated by the modified Bernoulli equation: 4 × TRV2 + 5 mmHg (an estimated central venous pressure) (Rudski et al., 2010).
With the use of color tissue Doppler imaging (TDI), the peak systolic velocity (tricuspid s’), early diastolic velocity (tricuspid e’), isovolumetric velocity (tricuspid IVV), and isovolumetric acceleration time (tricuspid IVAT) were measured at the lateral and septal tricuspid annular, and isovolumetric accelerated velocity (tricuspid IVA = IVV/IVAT) was calculated (Rudski et al., 2010).
2D STE
For STE analysis (QLAB 10.5), standard grayscale 2D images in the RV-focused apical four-chamber view were obtained (frame rate >60 fps) and digitally stored for three consecutive cardiac cycles. Speckles were tracked frame by frame throughout the RV wall automatically, and segments that failed to track were manually adjusted by the researchers. Any segment with persistently inadequate tracking was excluded. The peak systolic myocardial strain measured by 2D STE was assessed in six RV segments including 2D strain (2DS) basal, middle, apical (the average of septum and free wall), and RVGLS. The time-to-peak systolic strain was measured as the time from onset of QRS complexes on ECG to maximum RV shortening by strain. The SD of the time-to-peak systolic strain was calculated as a parameter of mechanical dyssynchrony in a model with four mid-basal RV segments according to Bazett’s formula; this measurement was called RVSD4 (Figure 1A). Using the upper 95% limit of young healthy subjects at SL in our study, a cutoff value of 18.7 ms was defined as the criterion for RVD (Badagliacca et al., 2015).
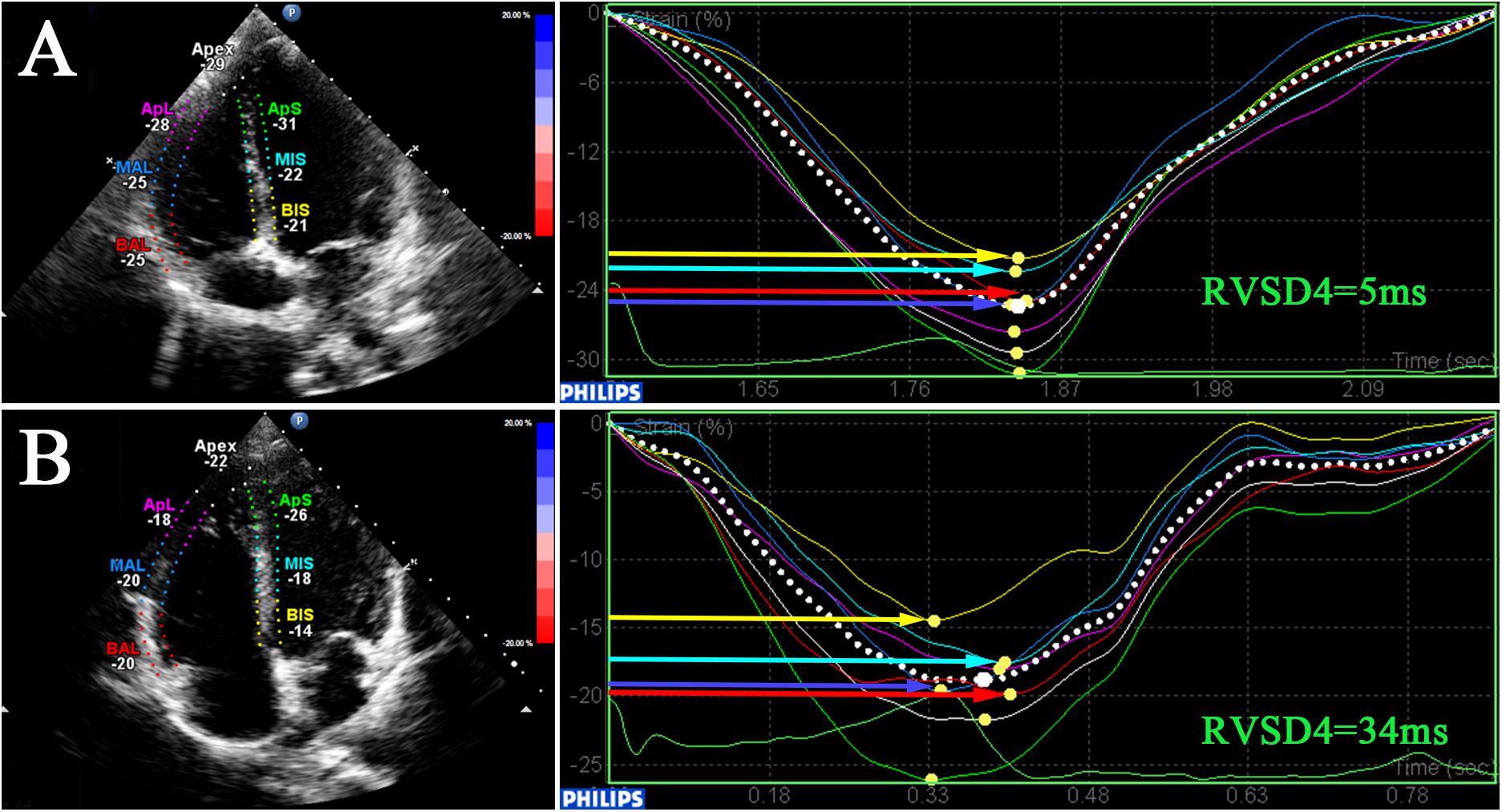
Figure 1. Dyssynchrony evaluation by speckle-tracking analysis. 2D speckle-tracking imaging in the RV-focused view in a participant at SL (A) and a participant at HA (B). Curves represent longitudinal strain curves, which were used to measure RVD and contractile function. RV, right ventricular; RVSD4, SD of the time-to-peak systolic strain for the four mid-basal RV segments.
Reproducibility
Observer reliability of RVSD4 was assessed in 20 randomly selected subjects: 10 from SL and 10 from HA. Interobserver variability was analyzed by two separate observers. Assessments of intraobserver variability were conducted in a random order at least 1 week apart. The Bland–Altman method was used to evaluate the intraobserver variability [0.05 ± 2.74 (95% CI: −5.33 to + 5.43)] and interobserver variability [0.28 ± 3.26 (95% CI: −6.12 to + 6.68)], which is considered acceptable for our study (Figures 2A,B). The observer variability for the other echocardiographic parameters is presented in Supplementary Table 1.
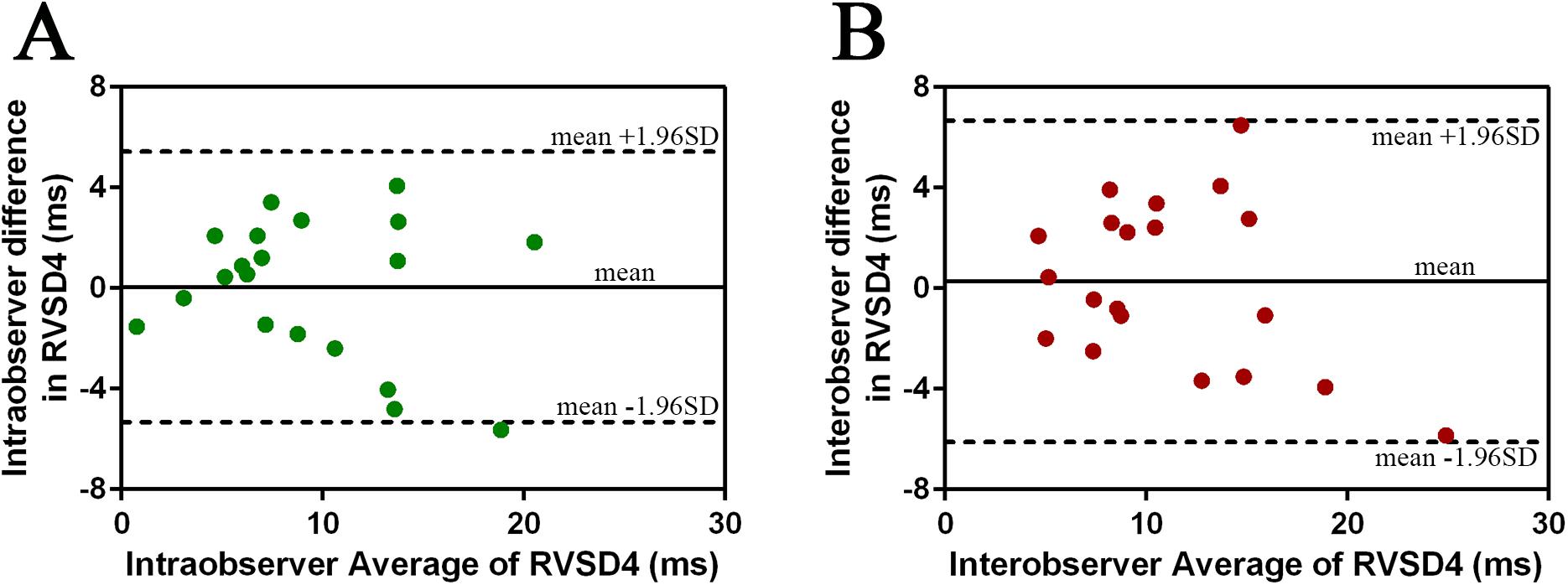
Figure 2. Intraobserver and interobserver variability in RVSD4 measurements assessed by the Bland–Altman method. (A) Intraobserver variability. (B) Interobserver variability. Abbreviations as in Figure 1.
Covariates
Age, height, weight, Han ethnic status, and smoking status were obtained by using interviews or physical examinations. Body mass index (BMI) (kg/m2) was calculated by weight (kg)/height2 (m2). Heart rate (HR) was recorded from synchronous ECG when echocardiography was performed. Oxygen saturation (SaO2) was measured with warmed hands after signal stabilization by finger-pulse oximetry (Nonin ONYX OR9500, United States). Systolic and diastolic blood pressure (SBP and DBP) levels were taken in the supine position after 10 min of rest by an Omron HEM-6200 (Japan). Venous blood samples were collected after a 5-min rest, and routine blood tests were performed at SL and HA for the assessment of hemoglobin (Hb) concentration and hematocrit by a BC-3000 plus automated hematology corpuscle analyzer (Shenzhen, China).
Statistical Analysis
SPSS 22.0 software (IBM Corp., Armonk, NY, United States) was used to perform all statistical analyses. Continuous data were represented as the mean ± SD or medians (25th, 75th percentiles) based on their normality distribution according to the Kolmogorov–Smirnov test, and categorical data were represented as count and percentage. Differences between groups were tested by using an independent-sample t-test or the Mann–Whitney U test for unpaired variables. The chi-square test was used for categorical variables. A paired t-test or the Wilcoxon test was used for comparisons of continuous variables between SL and HA. The risk factors for the incidence of RVD at HA were analyzed by univariate logistic regression analysis followed by a stepwise algorithm (P < 0.20 for entry and P < 0.05 for stay). Relationships between continuous variables were measured by linear regression analysis. The figures in this study were made by using GraphPad Prism 6 software (GraphPad Prism Software Inc., La Jolla, CA, United States). All tests were two-sided, and P-values <0.05 were considered statistically significant.
Results
Basic Characteristics and Physiological Parameters
In this preliminary study, a total of 108 subjects aged 20 (19, 22) years with sufficient quality echocardiographic images were included. Representative pictures showed that RVSD4 was increased after acute HA exposure (Figures 1A,B). Using the cutoff value of 18.7 ms as the criterion for RVD, the incidence of RVD at HA was 32.4%. There were no significant differences between the RVD− and RVD + groups in age, BMI, Han ethnicity, or smoking status. After acute HA exposure, HR, SBP, DBP, Hb concentration, and hematocrit were increased, but the values of SaO2 decreased in all RVD− and RVD + groups. However, subjects in the RVD + group had a lower SaO2 [88.2 ± 2.7 vs. 89.0 ± 2.7%, P < 0.05] than those in the RVD- group after acute HA exposure (Table 1 and Figure 3A).
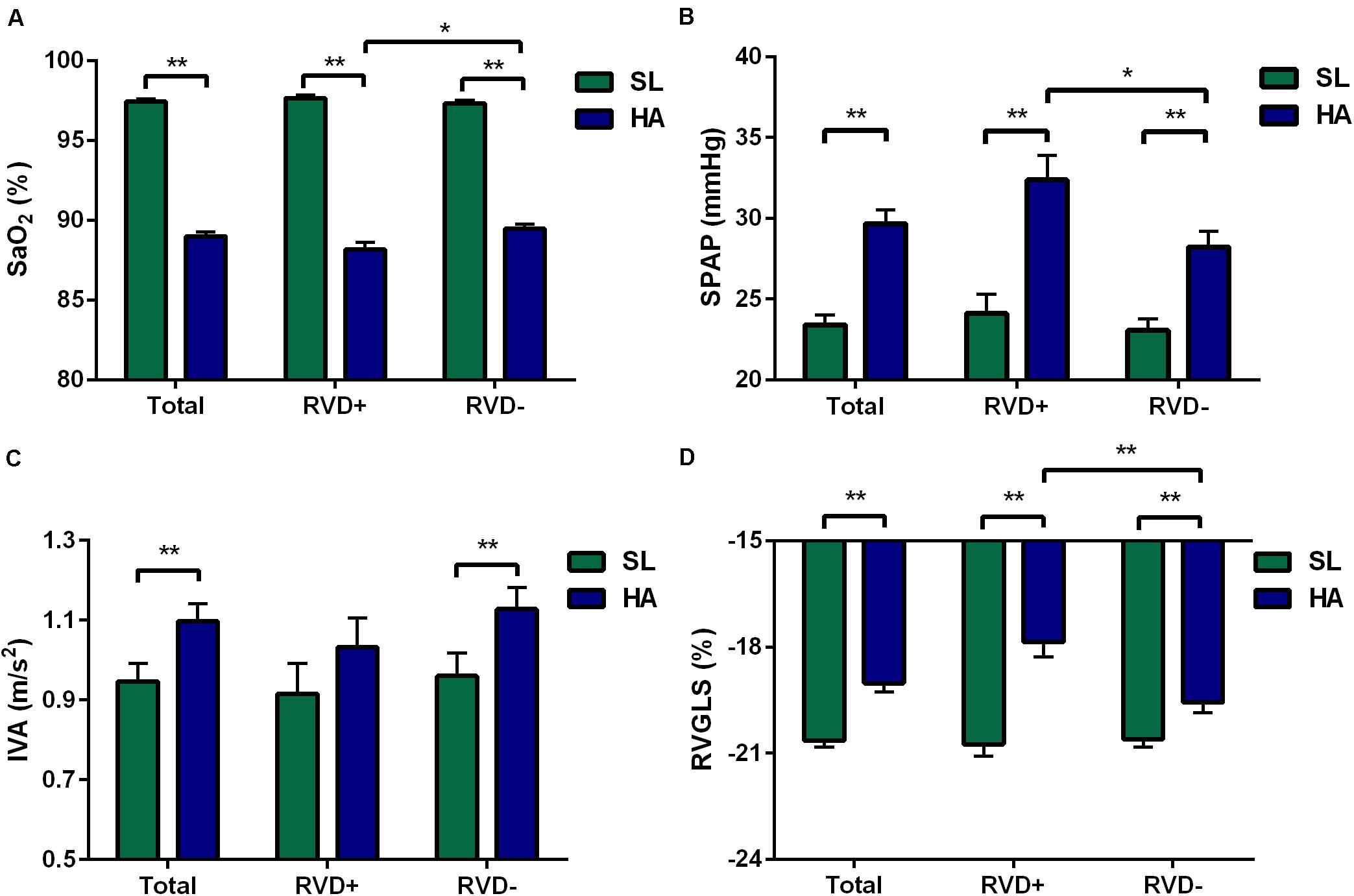
Figure 3. Differences in the total, RVD+, and RVD− subgroups. Different levels of SaO2 (A), SPAP (B), IVA (C), and RVGLS (D) in the total, RVD+, and RVD− subgroups. Values represent the mean ± standard error of the mean. *P < 0.05, **P < 0.01. Abbreviations are as in Tables 1–3.
RV Function
After acute HA exposure, RVSD4 was significantly increased in all subjects [7.8 (2.9, 11.0) vs. 13.9 (6.7, 20.7) ms, P < 0.001] and in subjects with RVD [7.2 (3.3, 9.8) vs. 22.7 (20.9, 30.9) ms, P < 0.001]. In all subjects, RA area, RV EDA, RV FAC, TAM, tricuspid E/A ratio, RVGLS, and 2DS (basal, middle, and apical) were decreased, whereas tricuspid IVV and IVA were increased following acute HA exposure. However, RVGLS [−18.6 (−19.5, −16.6) vs. −19.6 (−21.3, −18.5)%, P < 0.01] and 2DS basal [−18.6 (−19.8, −15.9) vs. −19.0 (−20.4, −17.9)%, P < 0.05] were even lower in subjects with RVD than in those without RVD. Moreover, increases in tricuspid IVV and IVA were found in subjects without RVD but not in those with RVD (Table 2 and Figures 3C,D).
Hemodynamics in Pulmonary Circulation
In all subjects, the values of PAAT, PAET, PAAT/PAET, PAAT/PEP, and PAV were decreased, whereas PEP, mPAP, and the incidence of TR, TRV, and SPAP were increased following acute HA exposure. Additionally, 25 (23.1%) subjects with Notch were identified at HA. However, subjects with RVD showed higher TRV [259 (231, 293) vs. 236 (218, 263) cm/s, P < 0.05] and higher SPAP [30.7 (26.3, 39.4) vs. 27.2 (24.0, 32.7) mmHg, P < 0.05] than those without RVD at HA (Table 3 and Figure 3B).
Factors Associated With the Incidence of RVD at HA
The results from univariate logistic regression showed that SaO2, RVGLS, and SPAP at HA were associated with the incidence of RVD at HA. Multivariate logistic regression identified two independent factors: SaO2 [odds ratio (OR): 0.72, 95% CI: 0.56–0.92; P = 0.009] and RVGLS (OR: 1.78, 95% CI: 1.31–2.41; P < 0.001) (Table 4).
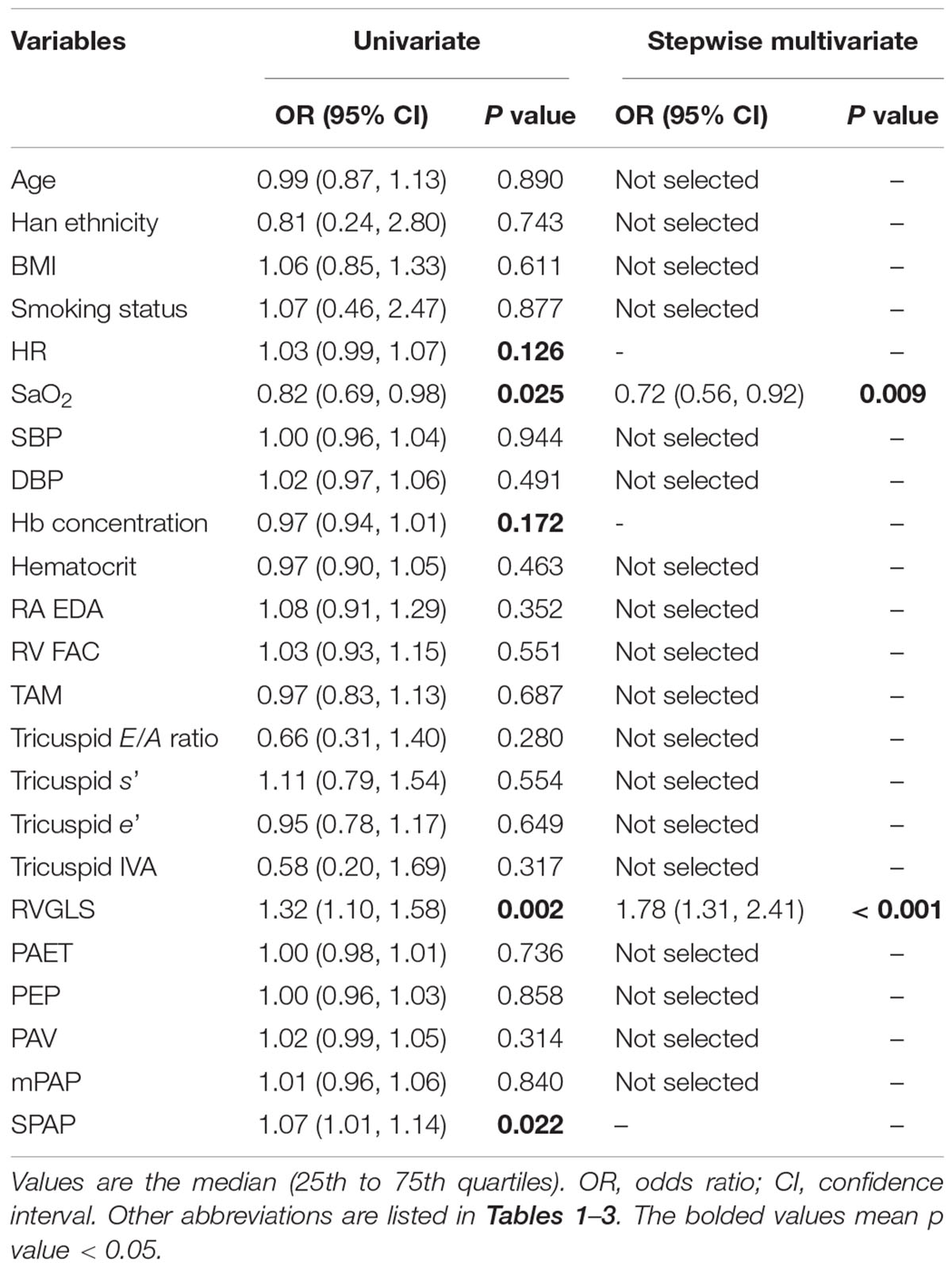
Table 4. Logistic regression analysis of clinical factors for the incidence of RVD at high altitude.
Associations of mPAP With RVSD4 or RVGLS
The linear regression analysis demonstrated that there was no significant correlation between the levels of mPAP and RVSD4 in all subjects at HA (Figure 4A). However, mPAP was linearly associated with RVSD4 at HA in the presence of Notch (r = 0.403, p = 0.046) (Figure 4B). Furthermore, RVSD4 was linearly associated with RVGLS at HA (r = 0.383, p < 0.001) (Figure 4C). Although there was no significant correlation between mPAP and RVGLS in all subjects at HA, mPAP was linearly associated with RVGLS at HA in subjects with RVD (r = 0.361, p = 0.028) (Figures 4D,E).
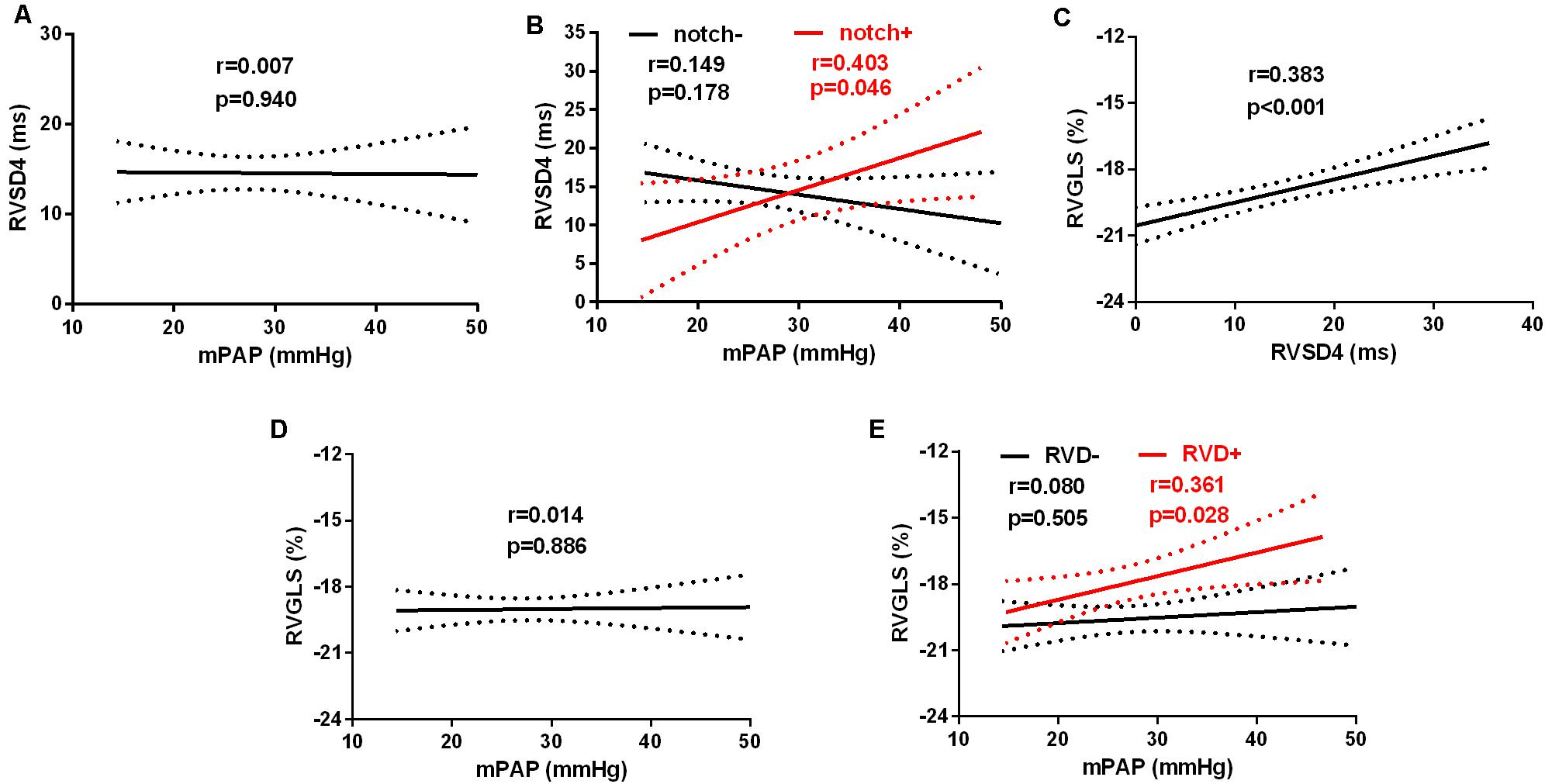
Figure 4. Linear relationships between mPAP and RVGLS or RVSD4 at HA. The linear relationship between mPAP and RVSD4 at HA in (A) all subjects and (B) subjects with Notch (red) and without Notch (black). The linear relationship between RVGLS and RVSD4 at HA in all subjects (C). The linear relationships between mPAP and RVGLS at HA in (D) all subjects and (E) subjects with RVD (red) and without RVD (black). Abbreviations are as in Figure 1 and Tables 1, 2.
Discussion
This is the first study to investigate RVD at HA in healthy subjects and shows that (1) the incidence of RVD after acute HA exposure at 4,100 m is approximately 32.4% in healthy young men; (2) SaO2 is an independent determinant of RVD at HA; (3) mPAP is linearly correlated with the magnitude of RVD in the presence of Notch; and (4) the presence and magnitude of RVD at HA result in reduced RVGLS and blunted tricuspid IVA.
RVD Measurements by STE Analysis
Cardiac magnetic resonance (CMR) is considered a good method for the quantitative assessment of the severity of electromechanical dyssynchrony (Yim et al., 2018). However, unlike portable echocardiography, CMR is not flexible and convenient for field studies at HA. In addition, the lower temporal resolution of CMR compared with echocardiography may affect the analysis of time-to-peak metrics (Schafer et al., 2018). On the other hand, compared to the conventional TDI method, STE analysis can provide some advantages of angle independence and improved signal-to-noise ratio. Moreover, using the longitudinal strain analysis of STE is more accurate than using the wall velocities of TDI for evaluating intraventricular dyssynchrony (Rudski et al., 2010). Additionally, considering the excessive variability in the apical segments for assessing the time-to-peak strain, even in healthy subjects, RVSD4 but not the SD of time-to-peak strain from six segments (RVSD6) was used to estimate RVD (Murata et al., 2017).
Determinants of HA Exposure-Induced RVD
It has been demonstrated that RVD is mainly determined by the delayed contraction of the RV free wall at basal and mid-segments due to the non-uniformly distributed RV wall stress (Kalogeropoulos et al., 2008). RVD is always associated with elevated RV afterload, especially in patients with different kinds of PH. RVD has been shown to be attributed to an increase in RV afterload (Badagliacca et al., 2015). HA exposure induces a decrease in SaO2 as well as an increase in PAP; however, our present results suggest that there are lower levels of SaO2 in subjects with RVD and a significant association between SaO2 and RVD incidence by univariate and multivariate logistic regression, indicating that HA hypoxia may play a critical role in HA exposure-induced RVD independent of RV overload. Not surprisingly, the absence of RV overload as a contributor to the incidence of RVD may be explained by the fact that the elevated PAP during the first few days at HA was moderate. Consistently, recent results also showed that acute hypoxia for several minutes, but not exercise, could induce regional inhomogeneity (or dyssynchrony) of RV contraction, although both acute hypoxia and exercise led to a comparable increase in RV afterload, suggesting that acute hypoxia was the main determinant of RVD but not RV afterload (Pezzuto et al., 2018). Hypobaric hypoxia is the main characteristic of the HA environment but may result in the reduction in cardiac phosphocreatine (PCr)/ATP in healthy volunteers (Holloway et al., 2011). In an animal model, hypoxia-induced RV dysfunction is attributed to the disturbance of fatty acid oxidation and mitochondrial respiration, which could not be explained by RV overload, suggesting a direct impairment of hypoxia on RV (Gomez-Arroyo et al., 2013). Considering that the RV free wall is very thin and may be more sensitive to HA hypoxia, HA hypoxia-triggered RVD in the present study may be attributed to disturbed energy metabolism. However, the exact mechanisms involved need further investigation.
In our study, RV afterload (mPAP and SPAP) was not associated with the incidence of RVD. Consistently, a previous report showed that patients with borderline PH exhibited marked heterogeneity in RV contraction without any correlation with RV afterload (Lamia et al., 2017). However, our results showed that SPAP was higher in subjects with RVD than in those without RVD and that SPAP was associated with the incidence of RVD by univariate logistic regression, which suggested a weak association between SPAP and RVD. The mPAP in our study was also not a contributor to the development of RVD; however, mPAP was linearly associated with RVSD4 in subjects with Notch formation, which was attributed to pulmonary artery reflected waves under an elevated RV afterload, according to recent guidelines from the American Society of Echocardiography (Bossone et al., 2013), suggesting an underlying relationship between mPAP and RVD. Previous results showed that RV size was another contributing factor to RVD (Hardziyenka et al., 2009). In our study, RV size was not associated with the incidence of RVD, although the RA area and RVEDA had decreased after HA exposure due to hypovolemia, especially after several days of acclimatization, similar to our research setting (Stembridge et al., 2016). Similarly, the elevated afterload seemed to be an important cause of LV dyssynchrony in normal subjects with the absence of other confounding factors, such as ventricular size (Miura et al., 1993).
RV Function
In our present study, the increases in Hb concentration and hematocrit suggested that the blood volume was significantly decreased, which may lead to the reduction in ventricular filling (Stembridge et al., 2016). Thus, the reduced RV preload due to hypovolemia resulted in a significant but slight decline in RV FAC, which is a load-dependent estimation of RV systolic function (Rudski et al., 2010). Consistent with previous results, although the P value is 0.06, the comparable values of FAC with ours showed a trend toward a decline, which may be attributed to the limited sample size (Maufrais et al., 2017). Moreover, the TAM and RV strain also decreased after HA exposure, which was consistent with a previous study (Stembridge et al., 2014). Taken together, these results indicate that although parameters such as FAC and TAM were load dependent, the regional function was altered based on the decreased RV strain.
Although data from PAH patients showed that RVD was strongly correlated with impaired RV function and adverse RV remodeling (Hill et al., 2012), we did not find any additional changes in the decreased FAC and TAM in subjects with RVD. However, subjects with RVD showed lower values of RVGLS than subjects without RVD, which was mainly attributed to the decline in RV strain at basal segments, suggesting that regional RV function was more affected by RVD. Similarly, it has been demonstrated that the presence of RVD was associated with reduced RVGLS in children with PAH as evaluated by CMR (Schafer et al., 2018). Furthermore, we also found a significant increase in tricuspid IVA in all subjects and RVD- subjects but not in RVD + subjects after HA exposure, which indicated that RV IVA was blunted by RVD. It has been suggested that IVA is an ideal method for evaluating acute changes in RV function in a preload- and afterload-independent manner (Vogel et al., 2002). Furthermore, RV IVA has been considered a useful method for identifying early RV systolic alterations and predicting RV contractile dysfunction in the future (Suran et al., 2016). However, whether the blunted RV IVA in subjects with RVD after acute HA exposure persisted or was reversible remains to be investigated.
Study Limitations
There are some limitations in the present study. First, all the included subjects were young healthy men, and whether the present conclusion could extend to others is still unknown. Second, 2D STE is mainly based on plane selection and therefore may be inferior to 3D STE for evaluating RV function (Smith et al., 2014). Third, echocardiography instead of RV catheterization was used to evaluate the PAP because invasive procedures are unnecessary and unethical in healthy individuals despite right-heart catheterization being the gold standard.
Conclusion
For the first time, we demonstrated that acute HA exposure could induce RVD in healthy subjects, which may be mainly attributed to the decline in SaO2 as well as the increase of RV afterload; the incidence of RVD at HA was associated with reduced RV regional function and blunted myocardial acceleration. Our findings may provide novel insight into the understanding of RV adaptation to HA exposure. Further investigations are needed to clarify the clinical relevance of these findings.
Data Availability Statement
The datasets generated for this study are available on request to the corresponding author.
Ethics Statement
The studies involving human participants were reviewed and approved by the Clinical Research Ethics Board at the Third Military Medical University (Army Medical University). The patients/participants provided their written informed consent to participate in this study.
Author Contributions
LH conceived and designed the study. YY, CL, JT, XD, SB, JY, ZQ, JK, FY, CZ, and RR performed the experiments. YY, CL, and JT analyzed the data and wrote the manuscript. LH critically reviewed the manuscript. All authors approved the final manuscript.
Funding
This work was supported by grants from the National Natural Science Foundation of China (Grant No. 81730054); Military Logistics Research Project, PLA (Grant No. BLJ18J007); and the Special Health Research Project, Ministry of Health of PR China (No. 201002012).
Conflict of Interest
The authors declare that the research was conducted in the absence of any commercial or financial relationships that could be construed as a potential conflict of interest.
Supplementary Material
The Supplementary Material for this article can be found online at: https://www.frontiersin.org/articles/10.3389/fphys.2020.00703/full#supplementary-material
Footnotes
References
Badagliacca, R., Reali, M., Poscia, R., Pezzuto, B., Papa, S., Mezzapesa, M., et al. (2015). Right intraventricular dyssynchrony in idiopathic, heritable, and anorexigen-induced pulmonary arterial hypertension: clinical impact and reversibility. JACC Cardiovasc. Imaging 8, 642–652. doi: 10.1016/j.jcmg.2015.02.009
Bossone, E., D’andrea, A., D’alto, M., Citro, R., Argiento, P., Ferrara, F., et al. (2013). Echocardiography in pulmonary arterial hypertension: from diagnosis to prognosis. J. Am. Soc. Echocardiogr. 26, 1–14. doi: 10.1016/j.echo.2012.10.009
Gomez-Arroyo, J., Mizuno, S., Szczepanek, K., Van Tassell, B., Natarajan, R., Dos Remedios, C. G., et al. (2013). Metabolic gene remodeling and mitochondrial dysfunction in failing right ventricular hypertrophy secondary to pulmonary arterial hypertension. Circ. Heart Fail. 6, 136–144. doi: 10.1161/circheartfailure.111.966127
Hardziyenka, M., Campian, M. E., Bouma, B. J., Linnenbank, A. C., De Bruin-Bon, H. A., Kloek, J. J., et al. (2009). Right-to-left ventricular diastolic delay in chronic thromboembolic pulmonary hypertension is associated with activation delay and action potential prolongation in right ventricle. Circ. Arrhythm. Electrophysiol. 2, 555–561. doi: 10.1161/circep.109.856021
Hill, A. C., Maxey, D. M., Rosenthal, D. N., Siehr, S. L., Hollander, S. A., Feinstein, J. A., et al. (2012). Electrical and mechanical dyssynchrony in pediatric pulmonary hypertension. J. Heart Lung Transplant. 31, 825–830. doi: 10.1016/j.healun.2012.04.004
Holloway, C. J., Montgomery, H. E., Murray, A. J., Cochlin, L. E., Codreanu, I., Hopwood, N., et al. (2011). Cardiac response to hypobaric hypoxia: persistent changes in cardiac mass, function, and energy metabolism after a trek to Mt. Everest Base Camp. FASEB J. 25, 792–796. doi: 10.1096/fj.10-172999
Huez, S., Faoro, V., Vachiery, J. L., Unger, P., Martinot, J. B., and Naeije, R. (2007). Images in cardiovascular medicine. High-altitude-induced right-heart failure. Circulation 115, e308–e309.
Kalogeropoulos, A. P., Georgiopoulou, V. V., Howell, S., Pernetz, M. A., Fisher, M. R., Lerakis, S., et al. (2008). Evaluation of right intraventricular dyssynchrony by two-dimensional strain echocardiography in patients with pulmonary arterial hypertension. J. Am. Soc. Echocardiogr. 21, 1028–1034. doi: 10.1016/j.echo.2008.05.005
Kurdziel, M., Wasilewski, J., Gierszewska, K., Kazik, A., Pytel, G., Waclawski, J., et al. (2017). Echocardiographic assessment of right ventricle dimensions and function after exposure to extreme altitude: is an expedition to 8000 m hazardous for right ventricular function? High Alt. Med. Biol. 18, 330–337. doi: 10.1089/ham.2017.0019
Lamia, B., Muir, J. F., Molano, L. C., Viacroze, C., Benichou, J., Bonnet, P., et al. (2017). Altered synchrony of right ventricular contraction in borderline pulmonary hypertension. Int. J. Cardiovasc. Imaging 33, 1331–1339. doi: 10.1007/s10554-017-1110-6
Lang, R. M., Badano, L. P., Mor-Avi, V., Afilalo, J., Armstrong, A., Ernande, L., et al. (2015). Recommendations for cardiac chamber quantification by echocardiography in adults: an update from the American society of echocardiography and the European association of cardiovascular imaging. Eur. Heart J. Cardiovasc. Imaging 16, 233–270.
Maufrais, C., Rupp, T., Bouzat, P., Doucende, G., Verges, S., Nottin, S., et al. (2017). Heart mechanics at high altitude: 6 days on the top of Europe. Eur. Heart J. Cardiovasc. Imaging 18, 1369–1377. doi: 10.1093/ehjci/jew286
Miura, T., Bhargava, V., Guth, B. D., Sunnerhagen, K. S., Miyazaki, S., Indolfi, C., et al. (1993). Increased afterload intensifies asynchronous wall motion and impairs ventricular relaxation. J. Appl. Physiol. 75, 389–396. doi: 10.1152/jappl.1993.75.1.389
Murata, M., Tsugu, T., Kawakami, T., Kataoka, M., Minakata, Y., Endo, J., et al. (2017). Right ventricular dyssynchrony predicts clinical outcomes in patients with pulmonary hypertension. Int. J. Cardiol. 228, 912–918. doi: 10.1016/j.ijcard.2016.11.244
Naeije, R. (2010). Physiological adaptation of the cardiovascular system to high altitude. Prog. Cardiovasc. Dis. 52, 456–466. doi: 10.1016/j.pcad.2010.03.004
Naeije, R., Huez, S., Lamotte, M., Retailleau, K., Neupane, S., Abramowicz, D., et al. (2010). Pulmonary artery pressure limits exercise capacity at high altitude. Eur. Respir. J. 36, 1049–1055. doi: 10.1183/09031936.00024410
Netzer, N. C., Strohl, K. P., Hogel, J., Gatterer, H., and Schilz, R. (2017). Right ventricle dimensions and function in response to acute hypoxia in healthy human subjects. Acta Physiol. 219, 478–485. doi: 10.1111/apha.12740
Penaloza, D., and Arias-Stella, J. (2007). The heart and pulmonary circulation at high altitudes: healthy highlanders and chronic mountain sickness. Circulation 115, 1132–1146. doi: 10.1161/circulationaha.106.624544
Pezzuto, B., Forton, K., Badagliacca, R., Motoji, Y., Faoro, V., and Naeije, R. (2018). Right ventricular dyssynchrony during hypoxic breathing but not during exercise in healthy subjects: a speckle tracking echocardiography study. Exp. Physiol. 103, 1338–1346. doi: 10.1113/ep087027
Rehman, M. B., Howard, L. S., Christiaens, L. P., Gill, D., Gibbs, J. S. R., and Nihoyannopoulos, P. (2018). Resting right ventricular function is associated with exercise performance in PAH, but not in CTEPH. Eur. Heart J. Cardiovasc. Imaging 19, 185–192. doi: 10.1093/ehjci/jex002
Rudski, L. G., Lai, W. W., Afilalo, J., Hua, L., Handschumacher, M. D., Chandrasekaran, K., et al. (2010). Guidelines for the echocardiographic assessment of the right heart in adults: a report from the American society of echocardiography endorsed by the European association of echocardiography, a registered branch of the European society of cardiology, and the Canadian society of echocardiography. J. Am. Soc. Echocardiogr. 23, 685–713. doi: 10.1016/j.echo.2010.05.010
Schafer, M., Collins, K. K., Browne, L. P., Ivy, D. D., Abman, S., Friesen, R., et al. (2018). Effect of electrical dyssynchrony on left and right ventricular mechanics in children with pulmonary arterial hypertension. J. Heart Lung Transplant. 37, 870–878. doi: 10.1016/j.healun.2018.01.1308
Smith, B. C., Dobson, G., Dawson, D., Charalampopoulos, A., Grapsa, J., and Nihoyannopoulos, P. (2014). Three-dimensional speckle tracking of the right ventricle: toward optimal quantification of right ventricular dysfunction in pulmonary hypertension. J. Am. Coll. Cardiol. 64, 41–51.
Stembridge, M., Ainslie, P. N., Hughes, M. G., Stohr, E. J., Cotter, J. D., Nio, A. Q., et al. (2014). Ventricular structure, function, and mechanics at high altitude: chronic remodeling in Sherpa vs. short-term lowlander adaptation. J. Appl. Physiol. 117, 334–343. doi: 10.1152/japplphysiol.00233.2014
Stembridge, M., Ainslie, P. N., and Shave, R. (2016). Mechanisms underlying reductions in stroke volume at rest and during exercise at high altitude. Eur. J. Sport Sci. 16, 577–584. doi: 10.1080/17461391.2015.1071876
Suran, D., Sinkovic, A., and Naji, F. (2016). Tissue Doppler imaging is a sensitive echocardiographic technique to detect subclinical systolic and diastolic dysfunction of both ventricles in type 1 diabetes mellitus. BMC Cardiovasc. Disord. 16:72. doi: 10.1186/s12872-016-0242-2
Vogel, M., Schmidt, M. R., Kristiansen, S. B., Cheung, M., White, P. A., Sorensen, K., et al. (2002). Validation of myocardial acceleration during isovolumic contraction as a novel noninvasive index of right ventricular contractility: comparison with ventricular pressure-volume relations in an animal model. Circulation 105, 1693–1699. doi: 10.1161/01.cir.0000013773.67850.ba
Vonk Noordegraaf, A., Haddad, F., Bogaard, H. J., and Hassoun, P. M. (2015). Noninvasive imaging in the assessment of the cardiopulmonary vascular unit. Circulation 131, 899–913. doi: 10.1161/circulationaha.114.006972
Wierzbowska-Drabik, K., Picano, E., Bossone, E., Ciampi, Q., Lipiec, P., and Kasprzak, J. D. (2019). The feasibility and clinical implication of tricuspid regurgitant velocity and pulmonary flow acceleration time evaluation for pulmonary pressure assessment during exercise stress echocardiography. Eur. Heart J. Cardiovasc. Imaging 20, 1027–1034. doi: 10.1093/ehjci/jez029
Xu, X. Q., and Jing, Z. C. (2009). High-altitude pulmonary hypertension. Eur. Respir. Rev. 18, 13–17.
Yang, T., Li, X., Qin, J., Li, S., Yu, J., Zhang, J., et al. (2015). High altitude-induced borderline pulmonary hypertension impaired cardiorespiratory fitness in healthy young men. Int. J. Cardiol. 181, 382–388. doi: 10.1016/j.ijcard.2014.12.044
Yim, D., Hui, W., Larios, G., Dragulescu, A., Grosse-Wortmann, L., Bijnens, B., et al. (2018). Quantification of right ventricular electromechanical dyssynchrony in relation to right ventricular function and clinical outcomes in children with repaired tetralogy of fallot. J. Am. Soc. Echocardiogr. 31, 822–830. doi: 10.1016/j.echo.2018.03.012
Keywords: high altitude, right ventricular dyssynchrony, hypoxemia, pulmonary artery pressure, speckle-tracking echocardiography
Citation: Yang Y, Liu C, Tian J, Ding X, Yu S, Bian S, Yang J, Qin Z, Zhang J, Ke J, Yuan F, Zhang C, Rao R and Huang L (2020) Preliminary Study of Right Ventricular Dyssynchrony Under High-Altitude Exposure: Determinants and Impacts. Front. Physiol. 11:703. doi: 10.3389/fphys.2020.00703
Received: 15 October 2019; Accepted: 28 May 2020;
Published: 02 July 2020.
Edited by:
Hanns-Christian Gunga, Charité – Universitätsmedizin Berlin, GermanyReviewed by:
Fabian Hoffmann, University Hospital Cologne, GermanyJörn Rittweger, Helmholtz Association of German Research Centers (HZ), Germany
Copyright © 2020 Yang, Liu, Tian, Ding, Yu, Bian, Yang, Qin, Zhang, Ke, Yuan, Zhang, Rao and Huang. This is an open-access article distributed under the terms of the Creative Commons Attribution License (CC BY). The use, distribution or reproduction in other forums is permitted, provided the original author(s) and the copyright owner(s) are credited and that the original publication in this journal is cited, in accordance with accepted academic practice. No use, distribution or reproduction is permitted which does not comply with these terms.
*Correspondence: Lan Huang, huanglan260@126.com
†These authors have contributed equally to this work