- Department of Neurology, University of Iowa, Iowa City, IA, United States
Neural circuits extending from the cerebral cortex to the bladder maintain urinary continence and allow voiding when it is socially appropriate. Injuries to certain brain regions produce a specific disruption known as urge incontinence. This neurologic symptom is distinguished by bladder spasticity, with sudden urges to void and frequent inability to maintain continence. The precise localization of neural circuit disruptions responsible for urge incontinence remains poorly defined, partly because the brain regions, cell types, and circuit connections that normally maintain continence are unknown. Here, we review what is known about the micturition reflex circuit and about forebrain control of continence from experimental animal studies and human lesion data. Based on this information, we hypothesize that urge incontinence results from damage to a descending pathway that normally maintains urinary continence. This pathway begins with excitatory neurons in the prefrontal cortex and relays subcortically, through inhibitory neurons that may help suppress reflex micturition during sleep and until it is safe and socially appropriate to void. Identifying the specific cell types and circuit connections that constitute the continence-promoting pathway, from the forebrain to the brainstem, will help us better understand why some brain lesions and neurodegenerative diseases disrupt continence. This information is needed to pave the way toward better treatments for neurologic patients suffering from urge incontinence.
Introduction
The ability to inhibit urination despite a full bladder develops during childhood and is taken for granted by most people. However, 17 million people think about this on a daily, if not minute by minute basis, as they have lost this ability and experience urinary incontinence, the involuntary leakage of urine (Minassian et al., 2003). Urinary incontinence is more common in the elderly. In fact, one-fifth of people over the age of 65 will lose some degree of continent control (Landefeld et al., 2008), and adult diapers are beginning to outsell infant diapers (Richa and Ritsuko, 2019). Urinary incontinence contributes to caregiver burden, is a common consideration in institutionalization, and can leave the affected individual with feelings of isolation and depression (Jonas and Brown, 1975; Stewart et al., 2003; Irwin et al., 2006).
One type of urinary incontinence – commonly referred to as “urge incontinence” or more formally as “urgency urinary incontinence” – is a sudden impulse to urinate with the inability to overcome this urge and hold one’s bladder (Drake, 2018). Associated with bladder hypercontractility and loss of control of the external urethral sphincter (EUS), this form of urinary incontinence typically results from changes in the brain. Overactive bladder (OAB) symptoms in general can be treated with anticholinergic drugs, which may reduce bladder contractility and unpleasant sensations (Finney et al., 2006). However, these medications are less effective in many neurologic patients, and they can produce significant cognitive and other side effects (Mintzer and Burns, 2000; Sakakibara, 2015). Mirabegron, a beta-3-adrenoreceptor antagonist, reduces OAB symptoms without cognitive side effects (Cui et al., 2014), but without greater efficacy for neurologic patients with urge incontinence. Therefore, many neurologic patients require diapers. Our current lack of knowledge regarding the fundamental neural circuits maintaining urinary continence is hindering better treatments for patients with urge incontinence.
In this article, after briefly reviewing the reflex micturition circuit between the brainstem and spinal cord, we discuss the neurologic basis of urinary urgency and incontinence caused by lesions and other abnormalities in the human brain. We do not cover other types of urinary incontinence, including stress incontinence, that result from urologic or peripheral neuropathic changes. Instead, we focus exclusively on the central neurologic basis of urge incontinence.
Neural Circuit Control of Reflex Micturition
While we understand very little about the central neurologic basis of continence, more is known about the basic neural circuit controlling the micturition reflex. In the 1920s, J. F. Barrington produced brainstem lesions in cats. Combining these lesions with behavioral monitoring and urodynamics, he found a small region in the dorsal pons that, when lesioned, caused urinary retention (Barrington, 1925, 1927). This region, previously referred to as the “pontine micturition center,” is now known as Barrington’s nucleus (Barrington, 1925, 1927; Verstegen et al., 2017). Bar neurons project axons to the sacral spinal cord and coordinate bladder (detrusor) contraction with EUS relaxation (Loewy et al., 1979; Blok et al., 1997a; Blok and Holstege, 1997; Fowler et al., 2008; Hou et al., 2016; Verstegen et al., 2017; Keller et al., 2018). As the bladder fills with urine, mechanosensors in the bladder wall progressively activate an ascending pathway through the sacral spinal cord and through relay neurons in the midbrain periaqueductal gray (PAG), which then trigger reflex activation of Bar neurons (Fowler et al., 2008; Stone et al., 2011).
The past century of work on this circuit between the spinal cord and upper brainstem helps explain reflexive fill-void cycles, but does not explain how we can overcome this reflex during sleep or retain urine until it is socially acceptable to void. More specifically, information about the brainstem and spinal neurons required to trigger micturition does not explain why urge incontinence results from focal injuries in the forebrain (Andrew and Nathan, 1964; Andrew et al., 1966; Sakakibara, 2015). This suggests that descending neural projections to Bar may be responsible for the suppression and timely activation of the micturition reflex to maintain continence. Here we use animal and human findings to highlight what is known about the micturition reflex, brain regions that project to Bar, and how disruptions in specific forebrain regions may lead to incontinence.
Barrington’s Nucleus Synchronizes Bladder Contraction and Sphincter Relaxation
Bar as a whole is known to elicit reflexive voiding in mice, and distinguishing the different cell types that comprise Bar has provided promising information on micturition control, loss of which leads to urinary retention. Bar is made up of excitatory, glutamatergic neurons, and is differentiated from several surrounding cell groups based on the expression of certain neuronal markers. For example, Bar borders the locus coeruleus (LC), whose catecholaminergic neurons are easily distinguishable by their expression of tyrosine hydroxylase (Verstegen et al., 2017). Bar itself is made up of at least two neuronal subpopulations that send axonal projections directly to the distal spinal cord.
The first subpopulation, which expresses the neuropeptide co-transmitter corticotropin releasing hormone (CRH), is referred to as BarCRH (Valentino et al., 1994; Verstegen et al., 2017). A homologous area involved in micturition, analogous to Bar in rodents, has been identified in the human pons, with cells expressing corticotrophin releasing hormone (Ruggiero et al., 1999) and where a focal injury can eliminate the ability to void (Sakakibara et al., 1998). In rats and mice, BarCRH neurons make up roughly half of all Bar neurons that project axons to the sacral spinal cord (Valentino et al., 1995; Verstegen et al., 2017). Optogenetic and chemogenetic experiments shows that BarCRH neurons promote bladder contraction (Hou et al., 2016; Keller et al., 2018; Verstegen et al., 2019). Other recent studies verify that optogenetic stimulation of BarCRH neurons drives bladder contraction, but typically does not result in urinary excretion (Ito et al., 2020; Verstegen et al., 2019). However, detrusor contraction is dependent on how full the bladder is, and BarCRH excitation typically leads to voiding when the bladder is more than 50% full (Ito et al., 2020). Importantly, despite evidence that BarCRH neurons augment bladder contraction, ablating these neurons does not significantly change voiding behavior or bladder physiology (Verstegen et al., 2019).
A second, non-CRH subgroup of Bar neurons must control voiding because, in contrast to ablating the BarCRH subgroup, eliminating all glutamatergic neurons here causes severe urinary retention (Verstegen et al., 2019), similar to conventional lesions in rats and cats (1925; Satoh et al., 1978). Many neurons in this non-CRH subgroup are identified by their estrogen receptor expression (BarESR1). BarESR1 neurons project their axons primarily to a central region of the lumbosacral spinal cord (Keller et al., 2018), where inhibitory interneurons are thought to allow voiding by phasically inhibiting motor neurons that normally constrict the EUS (Blok et al., 1997a, 1998; Grill et al., 1999). In contrast to BarCRH neurons, optogenetically stimulating BarESR1 neurons not only increases bladder pressure, but relaxes the EUS and reliably triggers voiding (96% of BarESR1 stimulation trials vs. 37% in BarCRH cases) (Keller et al., 2018). Even when the bladder is empty, stimulating BarESR1 neurons causes EMG bursts in the EUS, similar to spontaneous voiding (Keller et al., 2018). While additional work is required to learn whether and how these or other Bar neurons control internal urethral sphincter (IUS) smooth muscle, these results suggest that that the BarESR1 subpopulation is a key node for controlling voluntary initiation of micturition.
Descending Inputs to Barrington’s Nucleus
BarESR1 neurons can trigger voiding and BarCRH neurons augment bladder contraction, but voluntary micturition control requires input from the forebrain. Many brain regions provide direct input projections to Bar, including the lateral hypothalamic area (LHA), medial preoptic area (POA), bed nucleus of the stria terminalis (BNST), PAG, anterior cingulate cortex (ACC), prelimbic cortex, and primary and secondary motor areas (Moga et al., 1990; Ennis et al., 1991; Allen and Cechetto, 1992; Rizvi et al., 1994; Valentino et al., 1994; Hou et al., 2016; Yao et al., 2018; Verstegen et al., 2019). Recent experimental work has focused largely on excitatory inputs that promote voiding.
For example, Bar receives dense, excitatory input from both the PAG and the LHA. Glutamatergic input from both brain regions produces excitatory post-synaptic responses on both BarCRH and non-CRH neurons without preference for either subgroup (Verstegen et al., 2019). Optogenetically stimulating glutamatergic axons from the PAG causes immediate voiding with an incontinent phenotype – mice void a small amount, immediately, when stimulated. Stimulating glutamatergic input from the LHA also led to voiding, often with a delay; these mice produced fewer, larger voids. Bar also receives direct input from the mouse primary motor cortex (M1), which could allow volitional initiation of voiding (Yao et al., 2018). It is not yet clear whether axons from any of these afferent sites selectively target one or the other Bar subpopulation.
These projections offer different pathways for initiating micturition, but not for suppressing the micturition reflex to maintain continent control. We have very little information on the role of inhibitory input to Bar or how urinary continence is maintained in general. Classic studies exploring the brain with electrical stimulation noted that some sites in the medial POA or BNST triggered bladder contractions, while stimulating the lateral POA caused bladder relaxation (Kabat et al., 1936). It remains unclear whether these effects resulted from neurons in those regions or axons passing through them, but the latter finding suggests that inhibitory input from the LPOA to Bar could be important for maintaining continence.
Rather than descending inhibitory input to Bar, one alternative model proposes that PAG interneurons form an inhibitory “switch” that normally constrains the micturition reflex (Liu et al., 2004; Fowler et al., 2008; Benarroch, 2010). Possibly at odds with this model, injuring the PAG causes urinary retention, not incontinence (Yaguchi et al., 2004). Another model, based on a limited number of cat experiments, suggests that a lateral (“L”) region of the pons, separate from Bar, sends axonal projections directly to the sacral spinal cord, enhancing the excitatory tone of EUS motor neurons and thus forming a separate, continence-promoting pathway that parallels the micturition-promoting projections that originate medially, from Bar (Holstege et al., 1986). Retrograde tracing in rodents has not identified a homologous “L” pathway (Verstegen et al., 2017), and it is unclear whether this arrangement exists in other species. Yet another model speculates that “fibers that arise in the frontal lobes” descend directly to the spinal cord and control EUS motor neurons (Ropper and Samuels, 2009). Whatever the pathway, maintaining continence probably requires inhibiting Bar neurons that initiate micturition, and it is clear from transection experiments in cats (Langworthy and Kolb, 1933; Tang, 1955) and lesion analysis in the human brain (Figure 1) that the integrity of certain forebrain regions is essential for maintaining continence.
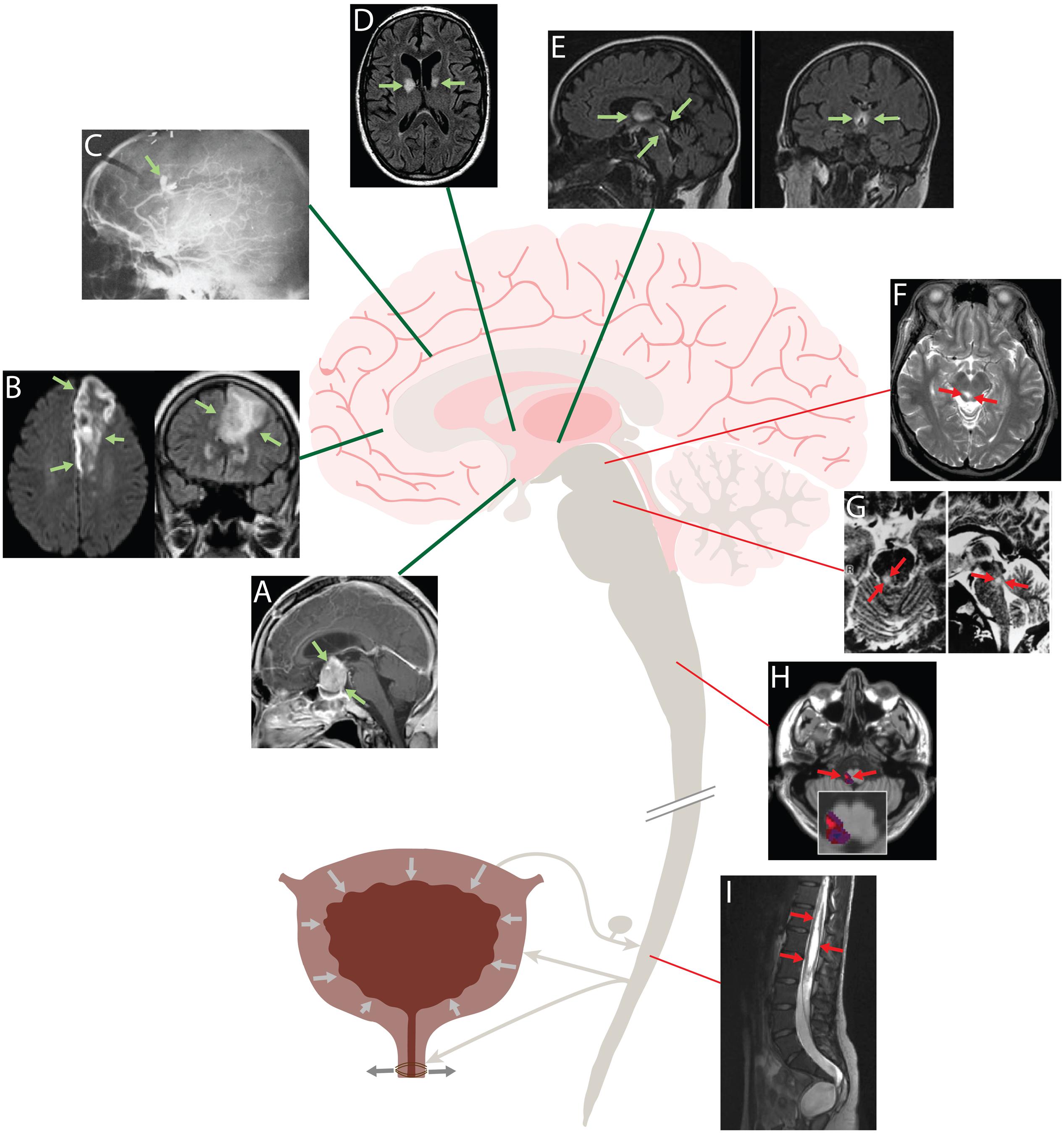
Figure 1. Human brain lesions that produce urgency and incontinence (A–E) localize to forebrain regions, while lesions that cause urinary retention (F–I) are located in the brainstem and spinal cord. Urge incontinence: (A) MRI showing a large, contrast-enhancing pituitary adenoma compressing the hypothalamus. This patient presented with urgency, frequency and daytime incontinence (Yamamoto et al., 2005). (B) Left anterior cerebral artery stroke causing urinary incontinence. Axial diffusion-weighted image (DWI) at left; coronal fluid-attenuated inversion recovery (FLAIR) image at right (Lakhotia et al., 2016). (C) Carotid arteriogram showing a ruptured, bilobed aneurysm of the left pericallosal artery. This patient had florid urinary incontinence, and a 2 cm hematoma was found in the left cingulate gyrus (Andrew et al., 1966). (D) Axial FLAIR image showing ischemic strokes centered on the internal capsule genu bilaterally in a patient who presented clinically with urinary incontinence and behavioral abnormalities. (E) MR images of a patient with Wernicke’s encephalopathy and urinary incontinence. Sagittal FLAIR image at left shows hyperintense periventricular abnormalities extending from the diencephalon through the periaqueductal gray matter; coronal image at shows symmetric hyperintensity in the thalamus and hypothalamus (Kuhn et al., 2012). Retention: (F) T2-weighted MR images showing hyperintense lesion in the right PAG. This patient presented with an inability to void, which improved with steroid treatment (Yaguchi et al., 2004). (G) T2-weighted MR images showing a hyperintense lesion in right dorsolateral tegmentum of the rostral pons in a patient with urinary retention after recovery from rhomboencephalitis (Sakakibara, 2015). (H) Overlap analysis of small strokes in the lateral medulla that caused urinary retention. Red indicates areas damaged more frequently in retentive relative to non-retentive patients after medullary strokes (Cho et al., 2015). (I) Sagittal T2-weighted MRI showing an extensive cystic lesion in the lumbosacral spinal cord of a patient with urinary retention (Kemp et al., 2014).
Human Brain Lesions That Disrupt Continence
Neurologic patients often suffer micturition deficits following strokes, tumors, or other focal brain lesions. Collectively, these are referred to as “lower urinary tract symptoms” (LUTS) and include OAB, urgency, nocturia, and urinary incontinence. A study in 2005 found that 40–60% of stroke patients had LUTS immediately following admission, and 25% still had symptoms at hospital discharge (Thomas et al., 2005). Even 1 year later, 15% of these patients still had LUTS.
The precise localization of human brain regions responsible for micturition symptoms remains elusive, because more than one site may be affected by neurologic diseases like multiple sclerosis, tumors, strokes, hydrocephalus, traumatic brain injuries, epilepsy, and Parkinson’s disease. Further, LUTS may result from peripheral neuropathies or even non-neurologic structural changes, in addition to diseases of the central nervous system. To approach the neurologic localization of brain regions that control micturition, most previous work has focused on the inherently correlational findings of functional brain imaging (Fowler and Griffiths, 2010). Here, we will instead focus on information derived from the analysis of focal brain injuries that produce acute-onset symptoms, which offers cause-and-effect information about the regions necessary for normal urinary continence.
Similar to experimental animal studies, lesions in the pons-midbrain, medulla, and spinal cord produce urinary retention with detrusor underactivity (Figure 1, red). In one case, acute urinary retention and decreased bladder sensation was caused by a lesion in the midbrain PAG (Yaguchi et al., 2004). Another study saw that in patients with multiple sclerosis, bladder hyporeflexia correlated to pontine lesions (Araki et al., 2003). And a patient with herpetic brainstem encephalitis causing a unilateral lesion in the upper pons developed urinary retention (Sakakibara et al., 1998). Two studies that looked at the medulla found that lesions producing urinary retention typically involve the lateral medulla, which contains axons running from Bar to the spinal cord (Cho et al., 2015; Lee et al., 2017). With regards to these descending tracts, there have been many case reports demonstrating that lesions to the spinal cord cause urinary retention (Head and Riddoch, 1917; Poeze et al., 1999; Kemp et al., 2014; Chern et al., 2017).
In contrast to brainstem and spinal cord lesions, forebrain lesions that alter micturition typically cause urgency and incontinence, not urinary retention (Figure 1, green). Andrews and Nathan spearheaded this area of investigation in the 1960s, presenting 37 patients with frontal lobe lesions that included tumors, aneurysms, penetrating wounds, and leukotomies (Andrew and Nathan, 1964; Andrew et al., 1966). Of these, 34 (92%) had hypertonic bladders that emptied at low volumes, typical urodynamic findings in patients with urinary urgency incontinence. Their incontinence appeared acutely and did not result from cognitive or gait impairments that can also arise with frontal lobe damage. They found that most lesions involved an anteromedial part of either frontal lobe, in an area that included the ACC and subcortical white matter tracts, near the genu of the corpus callosum (Andrew and Nathan, 1964).
Also in the 1960s, Ueki reported that urinary incontinence develops more often with tumors in the frontal lobe (10 of 76 patients) relative to other parts of the cerebral hemispheres (0/14 “central,” 2/32 parietal, 0/35 temporal, and 0/10 occipital). He also noted that lesions in the pons often inhibited micturition, consistent with Barrington’s experiments in cats, while frontal lobe lesions caused a loss of inhibition (incontinence). Ueki therefore proposed that the pons provides an important positive influence on micturition, with inhibitory input from the frontal lobe (Ueki, 1960).
These early observations are consistent with subsequent work. Sakakibara et al. (1996) analyzed 72 patients after acute stroke and found that 53% of these patients had LUTS (1996). Symptoms were more common when the lesion included the anterior and medial surfaces of the frontal lobe, anterior edge of the periventricular white matter, or genu of the internal capsule. Urodynamic studies in these patients revealed that detrusor overactivity (bladder contraction) was seen when the lesion involved the frontal lobe and basal ganglia, while sphincter relaxation, which is normally under voluntary control, was disinhibited when the lesion involved the frontal lobe (Sakakibara et al., 1996). Khan also reported on 33 post-stroke patients with LUTS and found that the majority had frontal cortex or internal capsule lesions Khan et al. (1990). Several reports confirm the common clinical observation that large anterior cerebral artery infarctions often cause severe urinary urgency and incontinence, sometimes with fecal incontinence as well (Bogousslavsky and Regli, 1990; Kumral et al., 2002; Lakhotia et al., 2016). Something in the anteromedial frontal lobe, therefore, is clearly important for maintaining urinary continence, though it cannot act alone.
Between the frontal lobes and brainstem is a continuum of regions through the deep hemispheric white matter, internal capsule, and diencephalon, where lesions similarly produce urge incontinence. For example, Andrews and Nathan reported one patient with a hypothalamic tumor causing urinary incontinence. This patient presented with painful detrusor contractions that occurred when the bladder was not full, and symptoms disappeared after resecting her tumor, which had occupied the anterior hypothalamus and stretched the optic nerves (Andrew and Nathan, 1965). In 1950, Brouwer presented a patient with a glioma in the hypothalamus and involuntary micturition was the first symptom Brouwer (1950). More recently, Yamamoto observed three patients with pituitary adenomas that spread to and compressed the hypothalamus. All three had urinary urgency, frequency, and incontinence (typically at night) accompanied by detrusor overactivity. Two patients also had urinary retention, with detrusor underactivity once voiding had been initiated leading to difficulty voiding and excess residual urine (Yamamoto et al., 2005). These results indicate that in addition to the anteromedial frontal lobes, the diencephalon (probably the hypothalamus) also contains neurons or axonal tracts that are critical for continent control of micturition.
Besides focal lesions, several other neurologic disorders can cause urinary incontinence. Microvascular ischemic disease (MVID), also known as white matter disease (WMD) is defined by progressive, patchy injury to white matter, typically in the deep hemispheric, periventricular region. MVID/WMD commonly affects elderly patients, and may cause “vascular incontinence” (Sakakibara et al., 2012a). In 1999, Sakakibara reported that 75% of patients with WMD had urinary frequency and 40% had urinary incontinence, with urodynamic studies identifying detrusor overactivity (Sakakibara et al., 1999). Subsequent work confirmed a significant relationship between OAB/urinary incontinence and WMD (Tadic et al., 2010). Wernicke’s encephalopathy, a nutritional deficiency that causes periventricular injury along the third ventricle and cerebral aqueduct typically including the hypothalamus, can include severe urge incontinence as a symptom (Sakakibara et al., 1997; Kuhn et al., 2012). Several other neurologic diseases cause incontinence, but it is frequently unclear whether or why a particular lesion or disease process produces micturition deficits.
Interestingly, night-time incontinence is very common in patients with brain lesions causing urgency with urinary frequency, even without daytime incontinence (Andrew et al., 1966; Sakakibara et al., 2012a). This is of interest because recordings in the monkey cerebral cortex have identified many neurons the anteromedial frontal lobe, near the genu of the corpus callosum, which increase their firing rates 4-fold during sleep relative to wakefulness (Rolls et al., 2003; Gabbott and Rolls, 2013). In the human brain, resting state fMRI analysis identified functional connectivity between a large region of the ventromedial prefrontal cortex and an anterior region of the hypothalamus that contains sleep-active inhibitory neurons (Boes et al., 2018). These regions with sleep-active neurons overlap frontal and hypothalamic regions that, when injured, cause incontinence and nocturnal enuresis, suggesting that they help maintain urinary continence during sleep.
Discussion
Complementary findings from experimental animal studies and human brain lesions indicate that (1) the neurons and axonal projections necessary for triggering micturition are contained within the brainstem and spinal cord, while (2) neurons and projections that are critical for maintaining continence are located somewhere within a poorly defined continuum of forebrain regions, running from the prefrontal cortex through the hypothalamus.
Excitatory neurons in the prefrontal cortex send heavy axonal projections to the hypothalamus (Hurley et al., 1991; Takagishi and Chiba, 1991; Vertes, 2004; Myers et al., 2014). The hypothalamus contains many inhibitory neurons and supplies heavy, direct input to Bar (Valentino et al., 1994; Kuipers et al., 2006; Venner et al., 2016). Based on connectivity data derived from animal studies, paired with the observation that lesions in the cortex and hypothalamus produce similar disinhibition of the micturition reflex, the most parsimonious hypothesis for a continence pathway that begins in the mPFC is that it relays through inhibitory neurons in or near the hypothalamus, which tonically inhibit Bar (and thereby the micturition reflex) until it is safe and socially appropriate to void. Alternatively, “hypothalamic” lesions that cause incontinence may simply impinge on mPFC axons coursing past the diencephalon to reach inhibitory relay neurons in the upper brainstem. In either case, identifying the forebrain neurons and circuit connections that inhibit reflex micturition is necessary to understand the neural control of continence. It is also important that we determine how this descending pathway interacts with other, excitatory inputs to Bar (Verstegen et al., 2019), whether it influences the IUS smooth muscle, and how it interacts with the hypothesized “switch” circuitry inside the PAG, which is considered important for reflex micturition (Fowler et al., 2008; Benarroch, 2010; Green et al., 2012; Griffiths and Fowler, 2013).
Overall, the neural control of urinary continence remains an understudied area of neuroscience research. Much work is needed to fill major knowledge gaps in this area. As examples, formal lesion-symptom mapping in the human brain can better define the specific forebrain regions where injuries may produce urinary urgency or incontinence (and conversely, which brain regions have nothing to do with urinary continence). This information is critical for guiding future work investigating the function of specific neurons in each region and the circuit connections between them and Bar. Another unanswered question in this area involves the laterality of micturition circuitry in the human brain. Functional imaging studies suggest a prominent role for the non-dominant (typically right) cerebral hemisphere (Blok et al., 1997b; Sasaki et al., 2007; Sakakibara et al., 2012b), but this correlation needs to be tested in a sufficiently powered lesion-symptom mapping study. Knowing the precise locations of neurons and axons that maintain continence would also accelerate our understanding of how exactly “non-localizing” or multifocal diseases like normal pressure hydrocephalus, multiple sclerosis, and white matter ischemic disease lead to incontinence in some patients, but not others. Finally, this information will guide targeted neurologic therapies to help boost or restore continent control in neurologic patients with urge incontinence. Similar to electrode stimulation of Bar in animal studies, unilateral deep brain stimulation (DBS) of the upper pons in a human patient triggered voiding and detrusor over-activity (Aviles-Olmos et al., 2011), so targeting DBS to an appropriate forebrain site may hold the potential to improve continence.
Ethics Statement
Our use of the de-identified image in panel (D), for academic purposes, is covered under a blanket policy for patient data at our academic hospital (UIHC). In addition, Dr. Geerling obtained signed consent from the patient to use their (de-identified) case history and images for research and teaching purposes, including scientific publications.
Author Contributions
MT and JG planned the manuscript and figure and edited the text and figure. MT drafted the text and figure. Both authors contributed to the article and approved the submitted version.
Funding
This work was supported by the Hydrocephalus Association Network for Discovery Science (HANDS), Aging Mind and Brain Initiative, and the University of Iowa Center for Aging (JG) (NIH K08 NS099425).
Conflict of Interest
The authors declare that the research was conducted in the absence of any commercial or financial relationships that could be construed as a potential conflict of interest.
References
Allen, G. V., and Cechetto, D. F. (1992). Functional and anatomical organization of cardiovascular pressor and depressor sites in the lateral hypothalamic area: I. Descending projections. J. Comp. Neurol. 315, 313–332. doi: 10.1002/cne.903150307
Andrew, J., and Nathan, P. W. (1964). Lesions on the anterior frontal lobes and disturbances of micturition and defaecation. Brain 87, 233–262. doi: 10.1093/brain/87.2.233
Andrew, J., and Nathan, P. W. (1965). The cerebral control of micturition. Proc. R. Soc. Med. 58, 553–555. doi: 10.1177/003591576505800733
Andrew, J., Nathan, P. W., and Spanos, N. C. (1966). Disturbances of micturition and defaecation due to aneurysms of anterior communicating or anterior cerebral arteries. J. Neurosurg. 24, 1–10. doi: 10.3171/jns.1966.24.1.0001
Araki, I., Matsui, M., Ozawa, K., Takeda, M., and Kuno, S. (2003). Relationship of bladder dysfunction to lesion site in multiple sclerosis. J. Urol. 169, 1384–1387. doi: 10.1097/01.ju.0000049644.27713.c8
Aviles-Olmos, I., Foltynie, T., Panicker, J., Cowie, D., Limousin, P., Hariz, M., et al. (2011). Urinary incontinence following deep brain stimulation of the pedunculopontine nucleus. Acta Neurochir. 153, 2357–2360. doi: 10.1007/s00701-011-1155-6
Barrington, F. J. (1927). Affections of micturition resulting from lesions of the nervous system. Proc. R. Soc. Med. 20, 722–727. doi: 10.1177/0035915727020005125
Barrington, F. J. F. (1925). The effect of lesions of the hind- and mid-brain on micturition in the cat. Exp. Physiol. 15, 81–102. doi: 10.1113/expphysiol.1925.sp000345
Benarroch, E. E. (2010). Neural control of the bladder: recent advances and neurologic implications. Neurology 75, 1839–1846. doi: 10.1212/wnl.0b013e3181fdabba
Blok, B. F., de Weerd, H., and Holstege, G. (1997a). The pontine micturition center projects to sacral cord GABA immunoreactive neurons in the cat. Neurosci. Lett. 233, 109–112. doi: 10.1016/s0304-3940(97)00644-7
Blok, B. F., Willemsen, A. T., and Holstege, G. (1997b). A PET study on brain control of micturition in humans. Brain 120(Pt 1), 111–121. doi: 10.1093/brain/120.1.111
Blok, B. F., and Holstege, G. (1997). Ultrastructural evidence for a direct pathway from the pontine micturition center to the parasympathetic preganglionic motoneurons of the bladder of the cat. Neurosci. Lett. 222, 195–198. doi: 10.1016/s0304-3940(97)13384-5
Blok, B. F., van Maarseveen, J. T., and Holstege, G. (1998). Electrical stimulation of the sacral dorsal gray commissure evokes relaxation of the external urethral sphincter in the cat. Neurosci. Lett. 249, 68–70. doi: 10.1016/s0304-3940(98)00382-6
Boes, A. D., Fischer, D., Geerling, J. C., Bruss, J., Saper, C. B., and Fox, M. D. (2018). Connectivity of sleep- and wake-promoting regions of the human hypothalamus observed during resting wakefulness. Sleep 41:zsy108.
Bogousslavsky, J., and Regli, F. (1990). Anterior cerebral artery territory infarction in the Lausanne stroke registry. Clinical and etiologic patterns. Arch. Neurol. 47, 144–150.
Brouwer, B. (1950). Positive and negative aspects of hypothalamic disorders. J. Neurol. Neurosurg. Psychiatry 13, 16–23. doi: 10.1136/jnnp.13.1.16
Chern, B. Z. H., Rajaraman, S., Verma, G., and Heng, K. W. J. (2017). Unusual case of acute urinary retention in a young female. BMJ Case Rep. 2017:bcr2017221411.
Cho, H. J., Kang, T. H., Chang, J. H., Choi, Y. R., Park, M. G., Choi, K. D., et al. (2015). Neuroanatomical correlation of urinary retention in lateral medullary infarction. Ann. Neurol. 77, 726–733. doi: 10.1002/ana.24379
Cui, Y., Zong, H., Yang, C., Yan, H., and Zhang, Y. (2014). The efficacy and safety of mirabegron in treating OAB: a systematic review and meta-analysis of phase III trials. Int. Urol. Nephrol. 46, 275–284. doi: 10.1007/s11255-013-0509-9
Drake, M. J. (2018). Fundamentals of terminology in lower urinary tract function. Neurourol. Urodyn. 37, S13–S19.
Ennis, M., Behbehani, M., Shipley, M. T., Van Bockstaele, E. J., and Aston-Jones, G. (1991). Projections from the periaqueductal gray to the rostromedial pericoerulear region and nucleus locus coeruleus: anatomic and physiologic studies. J. Comp. Neurol. 306, 480–494. doi: 10.1002/cne.903060311
Finney, S. M., Andersson, K. E., Gillespie, J. I., and Stewart, L. H. (2006). Antimuscarinic drugs in detrusor overactivity and the overactive bladder syndrome: motor or sensory actions? BJU Int. 98, 503–507. doi: 10.1111/j.1464-410x.2006.06258.x
Fowler, C. J., Griffiths, D., and de Groat, W. C. (2008). The neural control of micturition. Nat. Rev. Neurosci. 9, 453–466.
Fowler, C. J., and Griffiths, D. J. (2010). A decade of functional brain imaging applied to bladder control. Neurourol. Urodyn. 29, 49–55.
Gabbott, P. L., and Rolls, E. T. (2013). Increased neuronal firing in resting and sleep in areas of the macaque medial prefrontal cortex. Eur. J. Neurosci. 37, 1737–1746. doi: 10.1111/ejn.12171
Green, A. L., Stone, E., Sitsapesan, H., Turney, B. W., Coote, J. H., Aziz, T. Z., et al. (2012). Switching off micturition using deep brain stimulation at midbrain sites. Ann. Neurol. 72, 144–147. doi: 10.1002/ana.23571
Griffiths, D. J., and Fowler, C. J. (2013). The micturition switch and its forebrain influences. Acta Physiol. 207, 93–109. doi: 10.1111/apha.12019
Grill, W. M., Bhadra, N., and Wang, B. (1999). Bladder and urethral pressures evoked by microstimulation of the sacral spinal cord in cats. Brain Res. 836, 19–30. doi: 10.1016/s0006-8993(99)01581-4
Head, H., and Riddoch, G. (1917). The automatic bladder, excessive sweating and some other reflex conditions, in gross injuries of the spinal cord. Brain 40, 188–263. doi: 10.1093/brain/40.2-3.188
Holstege, G., Griffiths, D., de Wall, H., and Dalm, E. (1986). Anatomical and physiological observations on supraspinal control of bladder and urethral sphincter muscles in the cat. J. Comp. Neurol. 250, 449–461. doi: 10.1002/cne.902500404
Hou, X. H., Hyun, M., Taranda, J., Huang, K. W., Todd, E., Feng, D., et al. (2016). Central control circuit for context-dependent micturition. Cell 167, 73–86.e12. doi: 10.1016/j.cell.2016.08.073
Hurley, K. M., Herbert, H., Moga, M. M., and Saper, C. B. (1991). Efferent projections of the infralimbic cortex of the rat. J. Comp. Neurol. 308, 249–276. doi: 10.1002/cne.903080210
Irwin, D. E., Milsom, I., Kopp, Z., Abrams, P., and Cardozo, L. (2006). Impact of overactive bladder symptoms on employment, social interactions and emotional well-being in six European countries. BJU Int. 97, 96–100. doi: 10.1111/j.1464-410x.2005.05889.x
Ito, H., Sales, A. C., Fry, C. H., Kanai, A. J., Drake, M. J., and Pickering, A. E. (2020). Probabilistic, spinally-gated control of bladder pressure and autonomous micturition by Barrington’s nucleus CRH neurons. eLife 9:e56605. doi: 10.7554/eLife.56605
Jonas, S., and Brown, J. (1975). Neurogenic bladder in normal pressure hydrocephalus. Urology 5, 44–50. doi: 10.1016/0090-4295(75)90300-3
Kabat, H., Magoun, H. W., and Ranson, S. W. (1936). Reaction of the bladder to stimulation of points in the forebrain and mid-brain. J. Comp. Neurol. 63, 211–239. doi: 10.1002/cne.900630204
Keller, J. A., Chen, J., Simpson, S., Wang, E. H., Lilascharoen, V., George, O., et al. (2018). Voluntary urination control by brainstem neurons that relax the urethral sphincter. Nat. Neurosci. 21, 1229–1238. doi: 10.1038/s41593-018-0204-3
Kemp, J., Guzman, M. A., Fitzpatrick, C. M., and Elbabaa, S. K. (2014). Holocord syringomyelia secondary to tethered spinal cord associated with anterior sacral meningocele and tailgut cyst: case report and review of literature. Childs Nerv. Syst. 30, 1141–1146. doi: 10.1007/s00381-014-2379-6
Khan, Z., Starer, P., Yang, W. C., and Bhola, A. (1990). Analysis of voiding disorders in patients with cerebrovascular accidents. Urology 35, 265–270. doi: 10.1016/0090-4295(90)80048-r
Kuhn, A. L., Hertel, F., Boulanger, T., and Diederich, N. J. (2012). Vitamin B1 in the treatment of Wernicke’s encephalopathy due to hyperemesis after gastroplasty. J. Clin. Neurosci. 19, 1303–1305. doi: 10.1016/j.jocn.2011.11.030
Kuipers, R., Mouton, L. J., and Holstege, G. (2006). Afferent projections to the pontine micturition center in the cat. J. Comp. Neurol. 494, 36–53. doi: 10.1002/cne.20775
Kumral, E., Bayulkem, G., Evyapan, D., and Yunten, N. (2002). Spectrum of anterior cerebral artery territory infarction: clinical and MRI findings. Eur. J. Neurol. 9, 615–624. doi: 10.1046/j.1468-1331.2002.00452.x
Lakhotia, M., Pahadiya, H. R., Prajapati, G. R., Choudhary, A., Gandhi, R., and Jangid, H. (2016). A case of anterior cerebral artery A1 segment hypoplasia syndrome presenting with right lower limb monoplegia, abulia, and urinary incontinence. J. Neurosci. Rural Pract. 7, 189–191. doi: 10.4103/0976-3147.168438
Landefeld, C. S., Bowers, B. J., Feld, A. D., Hartmann, K. E., Hoffman, E., Ingber, M. J., et al. (2008). National Institutes of Health state-of-the-science conference statement: prevention of fecal and urinary incontinence in adults. Ann. Intern. Med. 148, 449–458.
Langworthy, O. R., and Kolb, L. C. (1933). The encephalic control of tone in the musculature of the urinary bladder. Brain 56, 371–382. doi: 10.1093/brain/56.4.371
Lee, H. S., Choi, J. G., and Shin, J. H. (2017). Urological disturbance and its neuroanatomical correlate in patients with chronic brainstem stroke. Neurourol. Urodyn. 36, 136–141. doi: 10.1002/nau.22889
Liu, Z., Sakakibara, R., Nakazawa, K., Uchiyama, T., Yamamoto, T., Ito, T., et al. (2004). Micturition-related neuronal firing in the periaqueductal gray area in cats. Neuroscience 126, 1075–1082. doi: 10.1016/j.neuroscience.2004.04.033
Loewy, A. D., Saper, C. B., and Baker, R. P. (1979). Descending projections from the pontine micturition center. Brain Res. 172, 533–538. doi: 10.1016/0006-8993(79)90584-5
Minassian, V. A., Drutz, H. P., and Al-Badr, A. (2003). Urinary incontinence as a worldwide problem. Int. J. Gynaecol. Obstet. 82, 327–338. doi: 10.1016/s0020-7292(03)00220-0
Mintzer, J., and Burns, A. (2000). Anticholinergic side-effects of drugs in elderly people. J. R. Soc. Med. 93, 457–462. doi: 10.1177/014107680009300903
Moga, M. M., Herbert, H., Hurley, K. M., Yasui, Y., Gray, T. S., and Saper, C. B. (1990). Organization of cortical, basal forebrain, and hypothalamic afferents to the parabrachial nucleus in the rat. J. Comp. Neurol. 295, 624–661. doi: 10.1002/cne.902950408
Myers, B., Mark Dolgas, C., Kasckow, J., Cullinan, W. E., and Herman, J. P. (2014). Central stress-integrative circuits: forebrain glutamatergic and GABAergic projections to the dorsomedial hypothalamus, medial preoptic area, and bed nucleus of the stria terminalis. Brain Struct. Funct. 219, 1287–1303. doi: 10.1007/s00429-013-0566-y
Poeze, M., Herpers, M. J., Tjandra, B., Freling, G., and Beuls, E. A. (1999). Intramedullary spinal teratoma presenting with urinary retention: case report and review of the literature. Neurosurgery 45, 379–385. doi: 10.1097/00006123-199908000-00038
Richa, N., and Ritsuko, A. (2019). Diaper Rush: Conquering a $9 Billon Market no One wants to Talk About. London: Thomson Reuters.
Rizvi, T. A., Ennis, M., Aston-Jones, G., Jiang, M., Liu, W. L., Behbehani, M. M., et al. (1994). Preoptic projections to Barrington’s nucleus and the pericoerulear region: architecture and terminal organization. J. Comp. Neurol. 347, 1–24. doi: 10.1002/cne.903470102
Rolls, E. T., Inoue, K., and Browning, A. (2003). Activity of primate subgenual cingulate cortex neurons is related to sleep. J. Neurophysiol. 90, 134–142. doi: 10.1152/jn.00770.2002
Ropper, A. H., and Samuels, M. A. (2009). Adams and Victor’s Principles of Neurology. New York, NY: McGraw-Hill Medical.
Ruggiero, D. A., Underwood, M. D., Rice, P. M., Mann, J. J., and Arango, V. (1999). Corticotropic-releasing hormone and serotonin interact in the human brainstem: behavioral implications. Neuroscience 91, 1343–1354. doi: 10.1016/s0306-4522(98)00703-9
Sakakibara, R. (2015). Lower urinary tract dysfunction in patients with brain lesions. Handb. Clin. Neurol. 130, 269–287. doi: 10.1016/b978-0-444-63247-0.00015-8
Sakakibara, R., Hattori, T., Fukutake, T., Mori, M., Yamanishi, T., and Yasuda, K. (1998). Micturitional disturbance in herpetic brainstem encephalitis; contribution of the pontine micturition centre. J. Neurol. Neurosurg. Psychiatry 64, 269–272. doi: 10.1136/jnnp.64.2.269
Sakakibara, R., Hattori, T., Uchiyama, T., and Yamanishi, T. (1999). Urinary function in elderly people with and without leukoaraiosis: relation to cognitive and gait function. J. Neurol. Neurosurg. Psychiatry 67, 658–660. doi: 10.1136/jnnp.67.5.658
Sakakibara, R., Hattori, T., Yasuda, K., and Yamanishi, T. (1996). Micturitional disturbance after acute hemispheric stroke: analysis of the lesion site by CT and MRI. J. Neurol. Sci. 137, 47–56. doi: 10.1016/0022-510x(95)00322-s
Sakakibara, R., Hattori, T., Yasuda, K., Yamanishi, T., Tojo, M., and Mori, M. (1997). Micturitional disturbance in Wernicke’s encephalopathy. Neurourol. Urodyn. 16, 111–115. doi: 10.1002/(sici)1520-6777(1997)16:2<111::aid-nau4>3.0.co;2-h
Sakakibara, R., Panicker, J., Fowler, C. J., Tateno, F., Kishi, M., Tsuyuzaki, Y., et al. (2012a). Vascular incontinence: incontinence in the elderly due to ischemic white matter changes. Neurol. Int. 4:e13.
Sakakibara, R., Uchida, Y., Ishii, K., Kazui, H., Hashimoto, M., Ishikawa, M., et al. (2012b). Correlation of right frontal hypoperfusion and urinary dysfunction in iNPH: a SPECT study. Neurourol. Urodyn. 31, 50–55. doi: 10.1002/nau.21222
Sasaki, H., Ishii, K., Kono, A. K., Miyamoto, N., Fukuda, T., Shimada, K., et al. (2007). Cerebral perfusion pattern of idiopathic normal pressure hydrocephalus studied by SPECT and statistical brain mapping. Ann. Nucl. Med. 21, 39–45. doi: 10.1007/bf03033998
Satoh, K., Shimizu, N., Tohyama, M., and Maeda, T. (1978). Localization of the micturition reflex center at dorsolateral pontine tegmentum of the rat. Neurosci. Lett. 8, 27–33. doi: 10.1016/0304-3940(78)90092-7
Stewart, W. F., Van Rooyen, J. B., Cundiff, G. W., Abrams, P., Herzog, A. R., Corey, R., et al. (2003). Prevalence and burden of overactive bladder in the United States. World J. Urol. 20, 327–336. doi: 10.1007/s00345-002-0301-4
Stone, E., Coote, J. H., Allard, J., and Lovick, T. A. (2011). GABAergic control of micturition within the periaqueductal grey matter of the male rat. J. Physiol. 589, 2065–2078. doi: 10.1113/jphysiol.2010.202614
Tadic, S. D., Griffiths, D., Murrin, A., Schaefer, W., Aizenstein, H. J., and Resnick, N. M. (2010). Brain activity during bladder filling is related to white matter structural changes in older women with urinary incontinence. Neuroimage 51, 1294–1302. doi: 10.1016/j.neuroimage.2010.03.016
Takagishi, M., and Chiba, T. (1991). Efferent projections of the infralimbic (area 25) region of the medial prefrontal cortex in the rat: an anterograde tracer PHA-L study. Brain Res. 566, 26–39. doi: 10.1016/0006-8993(91)91677-s
Tang, P. C. (1955). Levels of brain stem and diencephalon controlling micturition reflex. J. Neurophysiol. 18, 583–595. doi: 10.1152/jn.1955.18.6.583
Thomas, L. H., Barrett, J., Cross, S., French, B., Leathley, M., Sutton, C., et al. (2005). Prevention and treatment of urinary incontinence after stroke in adults. Cochrane Database Syst. Rev. 2005:CD004462.
Ueki, K. (1960). Disturbances of micturition observed in some patients with brain tumor. Neurologia 2, 25–33. doi: 10.2176/nmc.2.25
Valentino, R. J., Page, M. E., Luppi, P. H., Zhu, Y., Van Bockstaele, E., and Aston-Jones, G. (1994). Evidence for widespread afferents to Barrington’s nucleus, a brainstem region rich in corticotropin-releasing hormone neurons. Neuroscience 62, 125–143. doi: 10.1016/0306-4522(94)90320-4
Valentino, R. J., Pavcovich, L. A., and Hirata, H. (1995). Evidence for corticotropin-releasing hormone projections from Barrington’s nucleus to the periaqueductal gray and dorsal motor nucleus of the vagus in the rat. J. Comp. Neurol. 363, 402–422. doi: 10.1002/cne.903630306
Venner, A., Anaclet, C., Broadhurst, R. Y., Saper, C. B., and Fuller, P. M. (2016). A novel population of wake-promoting GABAergic neurons in the ventral lateral hypothalamus. Curr. Biol. 26, 2137–2143. doi: 10.1016/j.cub.2016.05.078
Verstegen, A. M. J., Klymko, N., Zhu, L., Mathai, J. C., Kobayashi, R., Venner, A., et al. (2019). Non-Crh glutamatergic neurons in Barrington’s nucleus control micturition via glutamatergic afferents from the midbrain and hypothalamus. Curr. Biol. 29, 2775–2789.e7. doi: 10.1016/j.cub.2019.07.009
Verstegen, A. M. J., Vanderhorst, V., Gray, P. A., Zeidel, M. L., and Geerling, J. C. (2017). Barrington’s nucleus: neuroanatomic landscape of the mouse “pontine micturition center”. J. Comp. Neurol. 525, 2287–2309. doi: 10.1002/cne.24215
Vertes, R. P. (2004). Differential projections of the infralimbic and prelimbic cortex in the rat. Synapse 51, 32–58. doi: 10.1002/syn.10279
Yaguchi, H., Soma, H., Miyazaki, Y., Tashiro, J., Yabe, I., Kikuchi, S., et al. (2004). A case of acute urinary retention caused by periaqueductal grey lesion. J. Neurol. Neurosurg. Psychiatry 75, 1202–1203. doi: 10.1136/jnnp.2003.027516
Yamamoto, T., Sakakibara, R., Uchiyama, T., Liu, Z., Ito, T., Yamanishi, T., et al. (2005). Lower urinary tract function in patients with pituitary adenoma compressing hypothalamus. J. Neurol. Neurosurg. Psychiatry 76, 390–394. doi: 10.1136/jnnp.2004.044644
Keywords: incontinence, urgency, continence, LUTS, micturition, voiding, urination, Barrington
Citation: Tish MM and Geerling JC (2020) The Brain and the Bladder: Forebrain Control of Urinary (In)Continence. Front. Physiol. 11:658. doi: 10.3389/fphys.2020.00658
Received: 20 November 2019; Accepted: 25 May 2020;
Published: 03 July 2020.
Edited by:
Laurival Antonio De Luca Jr., São Paulo State University, BrazilReviewed by:
Maria Luisa Sanchez-Ferrer, University of Murcia, SpainKarl-Erik-Andersson, Wake Forest University, United States
Copyright © 2020 Tish and Geerling. This is an open-access article distributed under the terms of the Creative Commons Attribution License (CC BY). The use, distribution or reproduction in other forums is permitted, provided the original author(s) and the copyright owner(s) are credited and that the original publication in this journal is cited, in accordance with accepted academic practice. No use, distribution or reproduction is permitted which does not comply with these terms.
*Correspondence: Joel C. Geerling, am9lbC1nZWVybGluZ0B1aW93YS5lZHU=
†ORCID: Joel C. Geerling, orcid.org/0000-0001-9956-4006